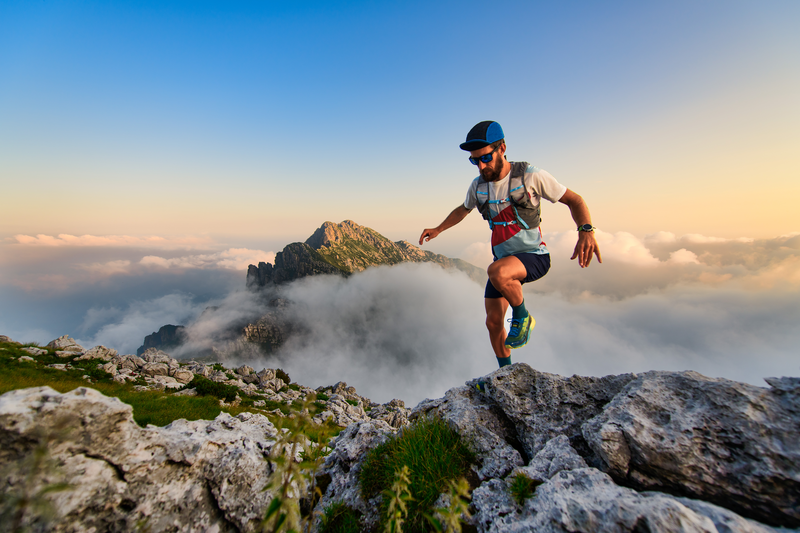
94% of researchers rate our articles as excellent or good
Learn more about the work of our research integrity team to safeguard the quality of each article we publish.
Find out more
OPINION article
Front. Neurol. , 11 August 2014
Sec. Pediatric Neurology
Volume 5 - 2014 | https://doi.org/10.3389/fneur.2014.00150
This article is part of the Research Topic Improving outcomes in cerebral palsy with early intervention: new translational approaches View all 15 articles
In a companion article of the same Research Topic, we present findings on the relationship of fetal adaptive brain shut-down and neuroinflammation during hypoxic acidemia (1). The findings are derived from the chronically instrumented non-anesthetized near-term ovine fetus model with and without chronic hypoxia (defined as arterial O2Sat < 55%) subjected to umbilical cord occlusions (UCOs) of increasing severity. This model mimics human labor and is useful for studying the process of worsening acidemia that may precipitate perinatal brain injury. While the neuroinflammation overall decreases between 24 and 48 h post UCOs, the relationship between the degree of neuroinflammation and the timing of the adaptive brain shut-down reverses between these two time points, raising the question as to the underlying physiology.
We propose that adaptive brain shut-down in the fetus, evidenced by changes in EEG, may be mediated via adenosine monophosphate kinase (AMPK) signaling due to its controlling influence over cellular metabolism and interaction with inflammatory signaling pathways. By way of background, consider that the intracellular energy-sensor AMPK plays a key role in cellular metabolism, increases in cellular AMP/ATP ratio result in activation of AMPK via its phosphorylation (2, 3). pAMPK reduces ATP-consuming processes and promotes ATP-producing processes. Consequently, neuronal pAMPK decreases during the relatively less energy-consuming NREM sleep state, which is associated with increased EEG delta wave activity and ATP increase in adult rats (4). Fetal adaptive neuronal shut-down with worsening acidemia may also be mediated via adenosine A1 receptors (1, 5, 6). Notably, a combination of both A1 and AMPK signaling is also a plausible mechanism leading to adaptive brain shut-down. First, Gadalla et al. observed that 5-aminoimidazole-4-carboxamide riboside (AICA riboside), a compound with neuroprotective properties thanks to the AMPK activation, has an additional neuroprotective effect under metabolic stress via competition with adenosine for uptake by the nucleoside transporter leading to an increase of extracellular adenosine and subsequent activation of A1 receptors (7). Second, endogenous extracellular adenosine in physiological concentrations is, in turn, equally able to activate AMPK, an effect requiring active nucleoside transporters, such as CNT2 (8, 9). Both AMPK and A1 receptor activation result in suppression of the more energy-consuming glutamatergic excitatory synaptic neurotransmission (i.e., as opposed to GABAergic inhibitory signaling contributing only ~20% to the neuronal oxidative energy metabolism) (10, 11). Either way, the result would be a relative increase of intracellular ATP and decreasing AMPK levels.
Sag et al. demonstrated in vitro that AMPK signaling and pro-inflammatory mediators in macrophages are mutually coupled via negative feedback. AMPK suppresses pro-inflammatory responses such as lipopolysaccharide (LPS)-induced production of TNF-α and IL-6 and promotes macrophage polarization to an anti-inflammatory functional phenotype with increased production of IL-10 (12). Exposure of macrophages to pro-inflammatory cytokines increases AMPK dephosphorylation, while exposure to anti-inflammatory cytokines results in rapid AMPK phosphorylation, i.e., activation (12). Activation of toll-like receptor (TLR) 4 on macrophages by LPS and resultant NF-κB pathway activation lead to a loss of AMPK phosphorylation (13). Hence, the effects of AMPK on the regulation of inflammatory status indicate that the presence of AMPK and its activation are important to counteract inflammation. Similarly, in vivo AMPK is down-regulated in all immune cells during experimental autoimmune encephalomyelitis (EAE), the animal model of the autoimmune disease multiple sclerosis (14). Neuronal AMPK is widely expressed in the embryonic and adult rat brains in situ and promotes neuronal survival under conditions of hypoglycemia in vitro (15).
Adenosine monophosphate kinase activity and its anti-inflammatory consequences have been studied in the context of chronic hypoxia. Chronic hypoxia up-regulates pAMPK in vitro in healthy neonatal rat neuronal slice cultures, in the human glioblastoma cells and in vivo in the adult rats’ pulmonary vasculature (7, 16, 17). Lactate is a principal energy source for neurons, especially in the developing brain (11, 18, 19). However, excess lactate within the extracellular space of the brain contributes to neuronal injury (3, 19). Recently, AMPK was also shown to play an important role in controlling the degree of cellular inflammation in various cell types including glial cells, thus linking cellular metabolism and inflammation (2, 3, 20). Brain regional lactate acidosis increases neuronal intracellular pAMPK levels (21). At the same time, pAMPK also restricts microglial activation via the IFN-γ signaling pathway decreasing expression of STAT1-inducible inflammatory cytokines in adult mice (2) and, anti-inflammatory effects of AMPK activation have been demonstrated on NF-κB pathway in the primary glial cultures, notably from 1 to 3 days old rat pups, and in vivo in adult rats (22).
In seeming contrast to the above cited work, acute brain ischemia in adult male gerbils results in regional (CA1) transient pAMPK and lactate increases, ATP depletion, neuronal death, and microglial activation [as opposed to suppression of microglial secretory cytokine activity shown by Meares et al. and Giri et al. (3)]. These traits are reversed if an AMPK inhibitor is administered (3). Notably, these authors provided indirect evidence that cortical neuronal pAMPK increases within 90 min post insult, probably to compensate for lack of ATP, while the glial AMPK induction follows within 5 days, when neuronal death is observed. The seemingly contradictory findings regarding the effect of AMPK on neuroinflammation may result from the different animal models used (septic versus aseptic neuroinflammation), varying temporal profiles (acute versus chronic), and neuroinflammatory phenotyping (cytokine secretion versus cell morphology).
In light of the discussed AMPK physiology, it is intriguing to speculate that at 24 h post UCOs, AMPK-mediated neuronal shut-down correlates to decreased brain regional lactate levels, leading to a pronounced decrease in neuroinflammation. In contrast, at 48 h post UCOs, the relationship between the degree of neuroinflammation and the timing of the adaptive brain shut-down may be reversed due to several reasons. First, the fetal brain may be less capable of metabolizing lactate under conditions of pre-existing hypoxia with reduced metabolism and ATP reserves unable to sustain pAMPK activation beyond an acute response. Second, at 48 h post UCOs, regional lactate accumulation may have occurred, in addition to AMPK and inflammatory mediators. Third, one of the side effects of AMPK activation may be an increase in lactate production due to glycolysis, which may contribute to tissue injury (3). While future studies will have to validate these mechanisms in the perinatal brain, it seems plausible that lactic acidosis has the potential to induce variable degrees of microglial activation and neuronal shut-down in an AMPK-dependent manner, in chronically hypoxic fetuses with worsening acidemia. Further investigations are needed into the potential of intrapartum EEG–FHR monitoring to aid detection of adaptive brain shut-down to improve early postnatal diagnostic and therapeutic strategies, such as selecting at-risk newborns for hypothermic interventions to decrease cerebral metabolism (6, 23).
Martin G. Frasch is an inventor of the related patent application entitled “EEG Monitor of Fetal Health” including U.S. Patent Application Serial No. 12/532,874 and CA 2681926 National Stage Entries of PCT/CA08/00580 filed March 28, 2008, with priority to US provisional patent application 60/908,587, filed March 28, 2007. No other disclosures have been made.
Source of Financial Support: FRQS; Women’s Development Council, London Health Sciences Centre, London, ON, Canada.
1. Xu A, Durosier L, Ross MG, Hammond R, Richardson BS, Frasch MG. Adaptive brain shut-down counteracts neuroinflammation in the near-term ovine fetus. Front Neurol (2014) 5:110. doi: 10.3389/fneur.2014.00110
2. Meares GP, Qin H, Liu Y, Holdbrooks AT, Benveniste EN. AMP-activated protein kinase restricts IFN-gamma signaling. J Immunol (2013) 190:372–80. doi:10.4049/jimmunol.1202390
3. Nam HG, Kim W, Yoo DY, Choi JH, Won MH, Hwang IK, et al. Chronological changes and effects of AMP-activated kinase in the hippocampal CA1 region after transient forebrain ischemia in gerbils. Neurol Res (2013) 35:395–405. doi:10.1179/1743132813Y.0000000158
4. Dworak M, Mccarley RW, Kim T, Kalinchuk AV, Basheer R. Sleep and brain energy levels: ATP changes during sleep. J Neurosci (2010) 30:9007–16. doi:10.1523/JNEUROSCI.1423-10.2010
5. Hunter CJ, Bennet L, Power GG, Roelfsema V, Blood AB, Quaedackers JS, et al. Key neuroprotective role for endogenous adenosine A1 receptor activation during asphyxia in the fetal sheep. Stroke (2003) 34:2240–5. doi:10.1161/01.STR.0000083623.77327.CE
6. Frasch MG, Keen AE, Gagnon R, Ross MG, Richardson BS. Monitoring fetal electrocortical activity during labour for predicting worsening acidemia: a prospective study in the ovine fetus near term. PLoS One (2011) 6:e22100. doi:10.1371/journal.pone.0022100
7. Gadalla AE, Pearson T, Currie AJ, Dale N, Hawley SA, Sheehan M, et al. AICA riboside both activates AMP-activated protein kinase and competes with adenosine for the nucleoside transporter in the CA1 region of the rat hippocampus. J Neurochem (2004) 88:1272–82. doi:10.1046/j.1471-4159.2003.02253.x
8. Aymerich I, Foufelle F, Ferre P, Casado FJ, Pastor-Anglada M. Extracellular adenosine activates AMP-dependent protein kinase (AMPK). J Cell Sci (2006) 119:1612–21. doi:10.1242/jcs.02865
9. Rose JB, Coe IR. Physiology of nucleoside transporters: back to the future. Physiology (Bethesda) (2008) 23:41–8. doi:10.1152/physiol.00036.2007
10. Patel AB, De Graaf RA, Mason GF, Rothman DL, Shulman RG, Behar KL. The contribution of GABA to glutamate/glutamine cycling and energy metabolism in the rat cortex in vivo. Proc Natl Acad Sci U S A (2005) 102:5588–93. doi:10.1073/pnas.0501703102
11. Mangia S, Giove F, Tkac I, Logothetis NK, Henry PG, Olman CA, et al. Metabolic and hemodynamic events after changes in neuronal activity: current hypotheses, theoretical predictions and in vivo NMR experimental findings. J Cereb Blood Flow Metab (2009) 29:441–63. doi:10.1038/jcbfm.2008.134
12. Sag D, Carling D, Stout RD, Suttles J. Adenosine 5’-monophosphate-activated protein kinase promotes macrophage polarization to an anti-inflammatory functional phenotype. J Immunol (2008) 181:8633–41. doi:10.4049/jimmunol.181.12.8633
13. Viollet B, Horman S, Leclerc J, Lantier L, Foretz M, Billaud M, et al. AMPK inhibition in health and disease. Crit Rev Biochem Mol Biol (2010) 45:276–95. doi:10.3109/10409238.2010.488215
14. Nath N, Khan M, Rattan R, Mangalam A, Makkar RS, De Meester C, et al. Loss of AMPK exacerbates experimental autoimmune encephalomyelitis disease severity. Biochem Biophys Res Commun (2009) 386:16–20. doi:10.1016/j.bbrc.2009.05.106
15. Culmsee C, Monnig J, Kemp BE, Mattson MP. AMP-activated protein kinase is highly expressed in neurons in the developing rat brain and promotes neuronal survival following glucose deprivation. J Mol Neurosci (2001) 17:45–58. doi:10.1385/JMN:17:1:45
16. Neurath KM, Keough MP, Mikkelsen T, Claffey KP. AMP-dependent protein kinase alpha 2 isoform promotes hypoxia-induced VEGF expression in human glioblastoma. Glia (2006) 53:733–43. doi:10.1002/glia.20326
17. Huang X, Fan R, Lu Y, Yu C, Xu X, Zhang X, et al. Regulatory effect of AMP-activated protein kinase on pulmonary hypertension induced by chronic hypoxia in rats: in vivo and in vitro studies. Mol Biol Rep (2014) 41(6):4031–41. doi:10.1007/s11033-014-3272-9
18. Abi-Saab WM, Maggs DG, Jones T, Jacob R, Srihari V, Thompson J, et al. Striking differences in glucose and lactate levels between brain extracellular fluid and plasma in conscious human subjects: effects of hyperglycemia and hypoglycemia. J Cereb Blood Flow Metab (2002) 22:271–9. doi:10.1097/00004647-200203000-00004
19. Prins ML. Cerebral metabolic adaptation and ketone metabolism after brain injury. J Cereb Blood Flow Metab (2008) 28:1–16. doi:10.1038/sj.jcbfm.9600543
20. Katerelos M, Mudge SJ, Stapleton D, Auwardt RB, Fraser SA, Chen CG, et al. 5-Aminoimidazole-4-carboxamide ribonucleoside and AMP-activated protein kinase inhibit signalling through NF-kappaB. Immunol Cell Biol (2010) 88:754–60. doi:10.1038/icb.2010.44
21. Jiang P, Gan M, Ebrahim AS, Castanedes-Casey M, Dickson DW, Yen SH. Adenosine monophosphate-activated protein kinase overactivation leads to accumulation of alpha-synuclein oligomers and decrease of neurites. Neurobiol Aging (2013) 34:1504–15. doi:10.1016/j.neurobiolaging.2012.11.001
22. Giri S, Nath N, Smith B, Viollet B, Singh AK, Singh I. 5-Aminoimidazole-4-carboxamide-1-beta-4-ribofuranoside inhibits proinflammatory response in glial cells: a possible role of AMP-activated protein kinase. J Neurosci (2004) 24:479–87. doi:10.1523/JNEUROSCI.4288-03.2004
Keywords: fetus, neuroinflammation, EEG, microglia, neurons, metabolism, sheep
Citation: Frasch MG (2014) Putative role of AMPK in fetal adaptive brain shut-down: linking metabolism and inflammation in the brain. Front. Neurol. 5:150. doi: 10.3389/fneur.2014.00150
Received: 20 June 2014; Accepted: 25 July 2014;
Published online: 11 August 2014.
Edited by:
Gavin John Clowry, Newcastle University, UKReviewed by:
Irina Burd, Johns Hopkins University School of Medicine, USACopyright: © 2014 Frasch. This is an open-access article distributed under the terms of the Creative Commons Attribution License (CC BY). The use, distribution or reproduction in other forums is permitted, provided the original author(s) or licensor are credited and that the original publication in this journal is cited, in accordance with accepted academic practice. No use, distribution or reproduction is permitted which does not comply with these terms.
*Correspondence:bWcuZnJhc2NoQHVtb250cmVhbC5jYQ==
Disclaimer: All claims expressed in this article are solely those of the authors and do not necessarily represent those of their affiliated organizations, or those of the publisher, the editors and the reviewers. Any product that may be evaluated in this article or claim that may be made by its manufacturer is not guaranteed or endorsed by the publisher.
Research integrity at Frontiers
Learn more about the work of our research integrity team to safeguard the quality of each article we publish.