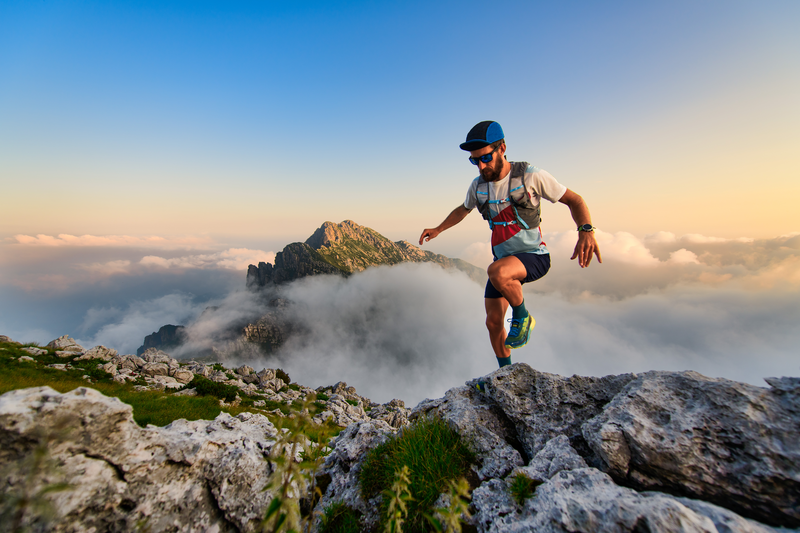
95% of researchers rate our articles as excellent or good
Learn more about the work of our research integrity team to safeguard the quality of each article we publish.
Find out more
OPINION article
Front. Neurol. , 16 March 2012
Sec. Sleep Disorders
Volume 3 - 2012 | https://doi.org/10.3389/fneur.2012.00038
This article is part of the Research Topic Sleep is an auto-regulatory global phenomenon View all 8 articles
Sleep is a complex dynamic phenomenon, which is continuously attracting attention of the scientific fraternity due to its mystifying unanswered nature and enigmatic functional significance. Understanding of the neurophysiological basis of sleep, primarily defined in electrophysiological landscape, has undergone tremendous changes in past years. There has been a paradigm shift in understanding of sleep from passive to active process, notwithstanding the recent autonomous self-regulatory global theory. In 1924, a broad consensus was arrived at on the electroencephalographic correlates of waking and sleep when Hans Berger recorded distinctly different wave patterns during these two states. In 1916, von Economo’s conscientious observation of lesions at midbrain diencephalon junction in patients who died of encephalitis lethargica or sleeping sickness (excessive sleepiness) had set the precedence for looking for localization of brain regions involved in the regulation of sleep (von Economo, 1930). But all along there was the wider belief that sleep, like life itself, may be too complex a phenomenon to be localized in any part of the brain. Later Aserinsky and Kleitman (1953) discovered rapid eye movement (REM) sleep, a classification was introduced for sleep as REM and non-rapid eye movement (NREM) sleep. There has been an apparent paradigm shift in the understanding of the mechanism of sleep regulation with the recent suggestion of autonomous self-regulatory global entity (Kumar, 2010). The complex organizational network of the mammalian brain oscillated through three distinct global activity states: wakefulness, NREM, and REM sleep. The micro-features, and neural network involved in this auto-oscillation of sleep-wakefulness, are highlighted here to give an insight into global dynamics of the prototype sleep.
Single neuronal activity recording during sleep-wakefulness provided an insight into localization of various neurons that altered their firing rates during different stages of sleep. Such neurons specifically showing correlation with sleep-wakefulness have been identified in various parts of the brain including the basal forebrain, preoptic area (POA), hypothalamic area, locus coeruleus, pontine tegmentum (Pace-Schott and Hobson, 2002). In the POA, the medial POA and the ventrolateral POA were recognized and debated for their role in initiation and maintenance of NREM sleep, whereas pontine tegmental areas were identified for playing a significant role in generation of REM sleep. Recent studies brought out the involvement of basal forebrain in the regulation of REM sleep (Srividya et al., 2004; Gulia et al., 2008). It was also proposed that the sleep-wake regulatory network is organized in a hierarchical rostro-caudal manner in the brain (Villablanca, 2004). It is interesting to note that a lesion in any one of these areas never elicited absolute insomnia. A meta-analysis of sleep loss after experimentally induced lesions in the crucial sleep regulating brain regions showed that the encountered sleep loss ranged between 10 and 55%. Similarly, in neurological conditions like a stroke, a complete loss of sleep was rarely ever observed, and sleep was restored within a couple of days, depending on the degree of insult to brain and the associated recovery due to medical intervention. These observations suggest a wide distribution of sleep regulatory networks in both the hemispheres of the brain.
There are instances of uni-hemispheric sleep in some animals in which slow-wave sleep, characterized by slow-wave electroencephalography (EEG) activity, in one hemisphere is accompanied by a low voltage EEG (characteristic of wakefulness) in the contra-lateral hemisphere. This pattern switches over periodically, between the two hemispheres (Mukhametov et al., 1977; Lyamin et al., 2008). Amongst the mammalian species, uni-hemispheric sleep is observed in aquatic species of the orders Cetacea and pinnipedia (dolphins and seals). A few migratory avian species also display such phenomenon (Rattenborg, 2006). The activity versus rest (sleep) framework is germane to the simplest single cellular organisms and the highly evolved species on the earth. It perhaps may be nature’s solicitous blueprint to provide alternate pathways in brain so that the crucial function like sleep may be least compromised even in most extreme circumstances. That is possible only if there are multiple regulatory areas which process different inputs from the environment and are capable of some autonomous control on sleep.
In homeotherms, sleep shares a close interrelationship with other crucial functions like thermoregulation for eliciting homeostatic responses in the body. The sleep disruption alters the body temperature, which in turn affects sleep. It has been demonstrated that the destruction of peripheral warm receptors during very early development produces long-term neuronal changes. The animals in this study showed a persistent increase in body temperature when they became adults (Gulia et al., 2005). Besides, animals spent more time in NREM sleep when exposed to high ambient temperature suggesting that the sleep regulatory circuit worked in close synchrony with the central thermoregulatory mechanism. This may provide a key adaptive mechanism that favors energy conservation for optimal survival in homeotherms. In higher primates, sleep might also serve as an integrator and co-ordinator of various physiological functions like emotionality, attention, learning, memory, etc. Various neural networks in different areas of brain organize and consolidate sleep-wakefulness in a very subtle way, depending upon internal and external inputs.
Sleep is traditionally assessed by monitoring the field potentials from the scalp as an indicator of neuronal activity in different regions of the cortex. Though the cortical EEG is a useful tool for classification of sleep, it is only a gross indicator of neuronal activity. Cortical electrical activity during NREM sleep is dominated by slow-wave oscillatory activity. Though there are growing evidences to prove that slow-wave oscillations can be generated autonomously by neocortical tissue (as these are preserved even after extensive thalamectomy), they become more regular and organized only through a thalamo-cortical feedback loop (Steriade, 2003; Fucke et al., 2011). The individual neurons are also shown to oscillate between activity and rest, and this dynamic potential entropy could be influenced by the various inputs. The sleep-like functional states may be localized even in small neuronal collections like cortical columns (Rector et al., 2005; Millman et al., 2010). It was even suggested that the cortical columns have intrinsic property which might operate even in the absence of sensory inputs in both in vivo and in vitro conditions.
Neocortical neurons display two distinct activity states, i.e., up states and down states. In slow-wave sleep, when there is a relative absence of sensory inputs, the cortex displays the spontaneous slow oscillatory pattern referred to as up and down states, and the cell ensembles fluctuate between states of sustained activity and silent epochs (Reig and Sanchez-Vives, 2007; Peyrache et al., 2010; Moore et al., 2011). It is also suggested that the full manifestation of this fundamental sleep oscillation is dependent not only on (predominant synaptically based) cortical oscillator, but it also requires a dynamic interaction with other cardinal thalamic oscillators (Crunelli and Hughes, 2010). More recently, it was suggested that activity waves during slow-wave sleep do not occur spontaneously at random locations within the network, but follow preferred synaptic pathways on a spatial scale measured through the scalp EEG.
Slow-wave sleep is detected as large-amplitude, low-frequency waves in electroencephalographic recordings at macroscale. At the cellular level, firing patterns in large neuronal populations is synchronized to the slow oscillation in specific cerebral regions. It is hypothesized that these slow oscillations during sleep might facilitate neuronal interactions in the brain and that the alteration in cortical synaptic strength can account for the changes in slow-wave activity (Esser et al., 2007; Dang-Vu et al., 2008). An evaluation of functional connectivity during slow-wave sleep is projected as a promising technique for comparing functional neural networks between healthy state and mental illness (Langheim et al., 2011). In extreme conditions like fatal familial insomnia, there may be severe disintegration of neural networks controlling sleep-wakefulness due to degeneration in thalamus (Provini et al., 2008).
Even though sleep patterns are largely organized by homeostatic, circadian, and allostatic drives in many studied species including human, modifications in the sleep-wake switch reflect an adaptive ability through evolution, partly due to habitat and ecological variables (Siegel, 2009; Phillips et al., 2010; Saper et al., 2010). Proverbial statement that “Sleep is of the brain, by the brain, and for the brain” identifies sleep in a broader outlook (Hobson, 2005). Whilst most functional systems of the body show some degree of auto-regulation, it is clearly observed in the brain for sleep regulation also. There are growing evidences to show that sleep might be regulated at a local level in different regions of the brain and it is projected as a fundamental property of neuronal networks, dependent on prior activity in each network (Krueger et al., 2008; Vassalli and Dijk, 2009; Vyazovskiy et al., 2011a). The sleep pressure imposes a basic physiological constraint on brain function. It is shown that when the animals are kept awake for a long time, brief episodes of slow-wave appeared in the cortical neurons even during waking state (Vyazovskiy et al., 2011b). These episodic slow-waves are similar to slow-wave activities observed during the sleep, and the incidence of these waves increased in different areas of the cortex with the increased time spent in forced waking. It is reasonable to believe that appearance of such peculiar local sleep at micro-level can emerge as a consequence of prolonged waking. These waking experience-induced local network changes are believed to be sensed by the sleep homeostatic process for regional network stabilization.
The concept of local sleep in a construct of individual cortical column microcircuits appears promising in understanding the deficits in cognition under the various circumstances of sleep loss. Nevertheless, the term “local sleep” fails to qualify classical physiological characteristics involved in defining sleep. Local sleep in isolated single neurons may be too brief and inadequate to qualify the criterion for sleep. It may be more appropriate to describe local features of networks similar to sleep rather than designating local sleep as a sub-type of sleep. Sleep is a complex neurological state that constitutes various key physiological and behavioral characteristics and follows a specific pattern for its consolidation. Global sleep, inevitably representing the coherence patterns in a larger network, provides a kaleidoscope of entire brain activity. However, the description of sleep as a global phenomenon fails to explain various conditions like parasomnia where co-existence of various local dissociated states is prevalent. It might be appropriate to study thoroughly the various aspects of the criteria employed in defining the prototype sleep with latest emerging knowledge and concepts. It would be beneficial to look into the properties of the billions of connected and unconnected neurons and glial cells to understand the functional correlates of prototype sleep.
The author acknowledges the Department of Science and Technology, India (SR/WOS-A/LS-59/2008) for the support.
Aserinsky, E., and Kleitman, N. (1953). Regularly occurring periods of eye motility, and concomitant phenomena, during sleep. Science 118, 273–274.
Crunelli, V., and Hughes, S. W. (2010). The slow (<1 Hz) rhythm of non-REM sleep: a dialogue between three cardinal oscillators. Nat. Neurosci. 13, 9–17.
Dang-Vu, T. T., Schabus, M., Desseilles, M., Albouy, G., Boly, M., Darsaud, A., Gais, S., Rauchs, G., Sterpenich, V., Vandewalle, G., Carrier, J., Moonen, G., Balteau, E., Degueldre, C., Luxen, A., Phillips, C., and Maquet, P. (2008). Spontaneous neural activity during human slow wave sleep. Proc. Natl. Acad. Sci. U.S.A. 105, 15160–15165.
Esser, S. K., Hill, S. L., and Tononi, G. (2007). Sleep homeostasis and cortical synchronization: I. Modeling the effects of synaptic strength on sleep slow waves. Sleep 30, 1617–1630.
Fucke, T., Suchanek, D., Nawrot, M. P., Seamari, Y., Heck, D. H., Aertsen, A., and Boucsein, C. (2011). Stereotypic spatio-temporal activity patterns during slow-wave activity in the neocortex. J. Neurophysiol. 106, 3035–3044.
Gulia, K. K., Jodo, E., Kawauchi, A., Miki, T., Kayama, Y., Mallick, H. N., and Koyama, Y. (2008). The septal area, site for the central regulation of penile erection during waking and rapid eye movement sleep in rats: a stimulation study. Neuroscience 156. 1064–1073.
Gulia, K. K., Mallick, H. N., and Kumar, V. M. (2005). Ambient temperature related sleep changes in rats neonatally treated with capsaicin. Physiol. Behav. 85, 414–418.
Krueger, J. M., Rector, D. M., Roy, S., Van Dongen, H. P., Belenky, G., and Panksepp, J. (2008). Sleep as a fundamental property of neuronal assemblies. Nat. Rev. Neurosci. 9, 910–919.
Kumar, V. M. (2010). Sleep is neither a passive nor an active phenomenon. Sleep Biol. Rhythms 8, 163–169.
Langheim, F. J., Murphy, M., Riedner, B. A., and Tononi, G. (2011). Functional connectivity in slow-wave sleep: identification of synchronous cortical activity during wakefulness and sleep using time series analysis of electroencephalographic data. J. Sleep Res. 20, 496–505.
Lyamin, O. I., Manger, P. R., Ridgway, S. H., Mukhametov, L. M., and Siegel, J. M. (2008). Cetacean sleep: an unusual form of mammalian sleep. Neurosci. Biobehav. Rev. 32, 1451–1484.
Millman, D., Mihalas, S., Kirkwood, A., and Niebur, E. (2010). Self-organized criticality occurs in non-conservative neuronal networks during up states. Nat. Phys. 6, 801–805.
Moore, A. R., Zhou, W. L., Jakovcevski, I., Zecevic, N., and Antic, S. D. (2011). Spontaneous electrical activity in the human fetal cortex in vitro. J. Neurosci. 31, 2391–2398.
Mukhametov, L. M., Supin, A. Y., and Polyakova, I. G. (1977). Interhemispheric asymmetry of the electroencephalographic sleep patterns in dolphins. Brain Res. 134, 581–584.
Pace-Schott, E. F., and Hobson, J. A. (2002). The neurobiology of sleep: genetics, cellular physiology and subcortical networks. Nat. Rev. Neurosci. 3, 591–605.
Peyrache, A., Benchenane, K., Khamassi, M., Wiener, S. I., and Battaglia, F. P. (2010). Sequential reinstatement of neocortical activity during slow oscillations depends on cells’ global activity. Front. Syst. Neurosci. 3:18. doi: 10.3389/neuro.06.018.2009
Phillips, A. J., Robinson, P. A., Kedziora, D. J., and Abeysuriya, R. G. (2010). Mammalian sleep dynamics: how diverse features arise from a common physiological framework. PLoS Comput. Biol. 6, e1000826. doi: 10.1371/journal.pcbi.1000826
Provini, F., Cortelli, P., Montagna, P., Gambetti, P., and Lugaresi, E. (2008). Fatal insomnia and agrypnia excitata: sleep and the limbic system. Rev. Neurol. (Paris) 164, 692–700.
Rector, D. M., Topchiy, I. A., Carter, K. M., and Rojas, M. J. (2005). Local functional state differences between rat cortical columns. Brain Res. 1047, 45–55.
Reig, R., and Sanchez-Vives, M. V. (2007). Synaptic transmission and plasticity in an active cortical network. PLoS ONE 2, e670. doi: 10.1371/journal.pone.0000670
Saper, C. B., Fuller, P. M., Pedersen, N. P., Lu, J., and Scammell, T. E. (2010). Sleep state switching. Neuron 68, 1023–1042.
Siegel, J. M. (2009). Sleep viewed as a state of adaptive inactivity. Nat. Rev. Neurosci. 10, 747–753.
Srividya, R., Mallick, H. N., and Kumar, V. M. (2004). Sleep changes produced by destruction of medial septal neurons in rats. Neuroreport 15, 1831–1835.
Vassalli, A., and Dijk, D. J. (2009). Sleep function: current questions and new approaches. Eur. J. Neurosci. 29, 1830–1841.
Villablanca, J. R. (2004). Counterpointing the functional role of the forebrain and of the brainstem in the control of the sleep-waking system. J. Sleep Res. 13, 179–208.
Vyazovskiy, V. V., Cirelli, C., and Tononi, G. (2011a). Electrophysiological correlates of sleep homeostasis in freely behaving rats. Prog. Brain Res. 193, 17–38.
Citation: Gulia KK (2012) Dynamism in activity of the neural networks in brain is the basis of sleep-wakefulness oscillations. Front. Neur. 3:38. doi: 10.3389/fneur.2012.00038
Received: 24 February 2012; Accepted: 27 February 2012;
Published online: 16 March 2012.
Copyright: © 2012 Gulia. This is an open-access article distributed under the terms of the Creative Commons Attribution Non Commercial License, which permits non-commercial use, distribution, and reproduction in other forums, provided the original authors and source are credited.
*Correspondence:a2tndWxpYUBzY3RpbXN0LmFjLmlu;a2tndWxpYWtAaG90bWFpbC5jb20=
Disclaimer: All claims expressed in this article are solely those of the authors and do not necessarily represent those of their affiliated organizations, or those of the publisher, the editors and the reviewers. Any product that may be evaluated in this article or claim that may be made by its manufacturer is not guaranteed or endorsed by the publisher.
Research integrity at Frontiers
Learn more about the work of our research integrity team to safeguard the quality of each article we publish.