- 1Deutecno Noses S.L., Madrid, Spain
- 2Department of Otorhinolaryngology, Fundación Hospital Alcorcón, Madrid, Spain
- 3Grupo de Neurocomputación Biológica, Departamento de Ingeniería Informática, Escuela Politécnica Superior, Universidad Autónoma de Madrid, Madrid, Spain
- 4Pharmacy Unit, Fundación Hospital Alcorcón, Madrid, Spain
Clinical olfactory tests are used to address hyposmia/anosmia levels in patients with different types of olfactory impairments. Typically, a given test is employed clinically and then replaced by a new one after a certain period of use which can range from days to several months. There is a need to assess control quality of these tests and also for a procedure to quantify their degradation over time. In this paper we propose a protocol to employ low-cost artificial noses for the quantitative characterization of olfactory tests used in clinical studies. In particular, we discuss a preliminary study on the Connecticut Chemosensorial Clinical Research Center Test kit which shows that some odorants, as sensed by an artificial nose, seem to degrade while others are potentiated as the test ages. We also discuss the need to establish a map of correspondence between human and machine olfaction when artificial noses are used to characterize or compare human smell performance in research and clinical studies.
Introduction
The assessment of olfactory function is an essential step for the diagnosis and treatment of olfactory disabilities. In particular, the quantification of the olfaction functional level is necessary to assess the recovery from or the progression of the smell dysfunction. The link between some types of smell impairments and the early detection of neurodegenerative (Albers et al., 2006; Barrios et al., 2007; Doty, 2008) or psychiatric (Atanasova et al., 2008) diseases also emphasizes the need of precise olfactory quantification procedures. Different protocols like the Connecticut Chemosensory Clinical Research Center (CCCRC) test (Cain, 1989), the University of Pennsylvania Smell Identification Test (UPSIT) (Doty et al., 1984), Sniffin' Sticks (Burghart, Wedel, Germany) (Hummel et al., 1997), T&T olfactometry, or the Odor Stick Identification Test for Japanese (OSIT-J) (Zusho, 1983) are employed throughout the world to assess the sense of smell in patients with different cultural backgrounds and disabilities. These tests consist of different odor containers that are presented to a patient to quantify specific odor thresholds, and to evaluate odor identification and discrimination.
A given test sample is typically employed in the clinic and then replaced by a new one after a certain period of use. In many cases the sample is opened, used, and closed again over an extended period of time which can range from days to several months. Thus, there is a need to assess control quality of these test samples and to establish a protocol to quantify their degradation over time.
Artificial noses are devices that use one or multiple sensors to mimic the sense of smell. The sensor reacts to an odorant and generates a signal that can be used for characterization, discrimination, or recognition purposes. The first artificial nose with a tin-oxide sensor was reported in the early 1980s. A wide range of sensor technologies have been developed since them to build many different types of artificial noses: electrical, gravimetric, optical, etc., [for a review of sensor technologies for artificial noses see (Stitzel et al., 2011)]. The applications of artificial noses cover areas such as safety, security, food, and beverage quality control, environmental monitoring, medical diagnostics, etc., (Dymerski et al., 2011; Stitzel et al., 2011).
In the following sections we propose the use of low-cost artificial noses to quantitatively characterize olfactory tests for clinical studies, and we discuss a preliminary study on the CCCRC kit. We also discuss the need to establish a map between human and machine olfaction for research and clinical studies.
Materials and Methods
Connecticut Chemosensorial Clinical Research Center Test
The CCCRC kit was developed by Cain and colleagues in 1988. This test consists of two parts: the butanol threshold test and the identification test. For the purposes of this study we will mainly concentrate on the butanol threshold test. The test was manufactured at Hospital Fundación de Alcorcón, Madrid with the collaboration of the Pharmacy Unit following the guidelines of the original article. The threshold test employs aqueous dilutions of 1-butanol where successive dilutions differ by a factor of three. The highest aqueous concentration is 4%. The number of dilution steps ranges from 0 to 8 depending on testing circumstances. The test solutions were stored in 125 ml polyethylene bottles containing 60 ml of solution which are presented to patients during the test. The bottle closure has a pop-up spout that fits to both nostrils (see Figure 1). To sample a bottle, the person places the spout into both nostrils and then sniffs. There are two common ways to present the stimulus: the ascending method of limits (AMLs) procedure and the single staircase (SS) procedure. In the AML, the odorant and the water are presented sequentially from low to high concentration and the point of transition between no detection and detection is estimated. In the SS method, the concentration of the stimulus is increased following trials in which correct detection occurs. In both methods, the stimulus is presented in order from weak to strong. The SS procedure is more reliable and is used more often for threshold testing. Four correct choices in a row lead to end the test. The concentration at which this occurs marks the threshold.
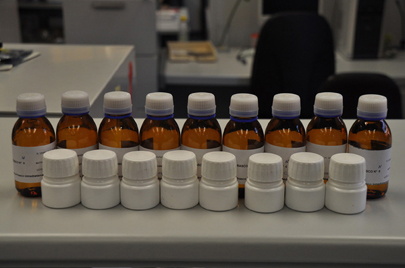
Figure 1. The Connecticut Chemosensorial Clinical Research Center Test used in this study. The brown bottles contain the butanol threshold test, while the white plastic jars contain the identification test.
Recently, Toledano and colleagues have described a short olfactory test based on CCCRC that predicts how severe is the smell loss (Toledano et al., 2009). This short test consists on determining the lowest concentration of butanol that the patient can detect four times in a row. Patients detecting beyond the butanol dilution number 3 have a high probability of normal olfaction.
The participant receives the identification test after the threshold test. The identification kit is composed of ten 180 ml opaque plastic jars containing 5 g of the substance in sachet-like packets of stimuli. Based on the performance of anosmic patients, we can ascertain that seven stimuli appeal almost exclusively to the sense of smell (baby powder, chocolate, cinnamon, coffee, mothballs, peanut butter, and bar soap) and one appeals to the trigeminal sense as well (Vicks). These eight items are presented in the same order to both nostrils. When presented with an item, the patient chooses from a 20-item list. The list contains the names of the eight test products and 13 distractors. In addition to the names on the list, responses of “no sensation” and “do not know” are permitted. The examiner provides corrective feedback whenever the participant makes an error. If the participant exhibits some evidence of function, but nevertheless makes mistakes, the examiner presents missed items for a second time. A correct answer upon a second presentation cancels a previous error. This allows the patient to rectify mistakes and thereby decreases the possibility of cognitive errors. In such cases, the first trial with an item serves as training. The score for the test comprises the number of olfactory items out of seven correctly identified and a notation regarding the ability to perceive trigeminal stimulation.
The outcome of the threshold and the identification tests is combined into a composite score, an average of the two tests. As previously defined (Toledano et al., 2003), a score of 5 points or more indicates normosmia, a score between 2 and 4.5 points indicates reduced olfactory function in terms of hyposmia, and a score of less than 2 points indicates functional anosmia.
To categorize progression of olfactory function, a subjective improvement and a normal score in butanol, threshold and composite, respectively, are regarded as a clinically significant improvement of olfactory function. Its correlation with previously established tests of olfactory function (e.g., the 12-item Cross-Cultural Smell Identification Test [CC-SIT, a subtest of the UPSIT] and the “Sniffin' Sticks” test) has been demonstrated in various studies (Kobal et al., 2000; Toledano et al., 2007).
Artificial Noses for Quality Control of Olfactory Tests
During the last decades artificial nose technology has provided many successful examples of industrial applications [for recent reviews see (Dymerski et al., 2011; Stitzel et al., 2011)]. As this technology becomes cheaper and more accessible, the possibilities of potential use in medical and clinical studies have also increased. In this section we discuss a preliminary study on the use of a low-cost, portable artificial nose to quantitatively characterize the CCCRC test.
For the study reported in this paper we used an odor analysis platform capable of managing up to 16 samples with on/off control electrovalves and three auxiliary devices such as pumps, heaters, and/or mixers (see Figure 2). Although the platform can be equipped with a wide variety of sensor arrays, we aimed to study the performance of inexpensive sensors that could be used in future widely distributed control quality devices. Thus, we selected the TGS2600-B00 (Figaro Engineering Inc.) chemoresistive sensor. This small sensor also provides a good combination of high sensitivity to low concentrations of odorants, long life, low power consumption, and robustness. The sensor is comprised of a metal oxide semiconductor layer formed on an alumina substrate of a sensing chip together with an integrated heater. In the presence of an odorant, the sensor's conductivity increases as a function of the concentration. A simple electrical circuit converts the change in conductivity to an output signal that corresponds to the odorant. The signal generated by the sensor is not amplified, it is only conditioned with an LMC6484 amplifier in voltage follower mode and sent to the Analog-to-Digital (A/D) converter module to be acquired and stored in a computer. The voltage range of this signal is 0–5 V.
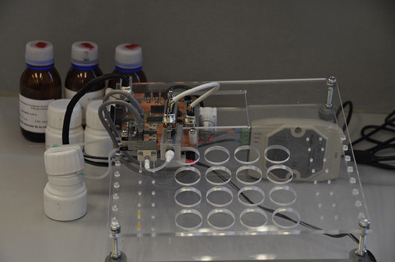
Figure 2. Analysis platform used in this study with the TGS2600-B00 (Figaro Engineering Inc.) chemoresistive sensor.
From the control point of view, the main component used in this platform is an 8-bit PIC18F2455 microcontroller (Microchip Technology Inc.). This microcontroller is ideal for low-cost, low power (nano-Watt), and connectivity applications. The firmware was programmed with the MPLAB IDE development environment provided by the same company. The computer application software was developed with IDE Dev-C++ (GNU license) using Windows APIs. The equipment is connected to a PC via serial bus, by a proprietary protocol based on EIA-485. The transfer rate used in our study was 100 samples per second. For this application we used the rotary vane pump 01-K G-LC (Rietschle Thomas Company) in continuous vacuum mode (−20 mbar max.) running at 80% of its nominal voltage. All the instrumentation used in this study is characterized by its compactness and low price, which are important factors for a wide dissemination of quality control in olfactory tests.
Since the goal of our experiments is to assess the degradation of well-known odorants, we used the peak of the sensor signal to characterize the dilutions/odorants in a comparative manner (the peak corresponding to an aged olfactory test vs. the peak corresponding to a new test). The maximal separability between signals occurs typically at the peak. This is a simple and straightforward measurement that does not require further processing of the signal.
The performance of the TGS2600-B00 sensor has a moderate ambient temperature/humidity dependence as described in the manufacturer's worksheet (http://www.figarosensor.com/products/2600pdf.pdf). All experiments reported here were performed at a room temperature between 23°C and 24°C and a humidity of 30–35%. The acquisition of the signal was performed with the following protocol: the sensor was exposed to room air with no heating (OV) for 5 s, followed by 100 s at maximum heating (4.8 V) to clean the sensor (heater resistance is 83 Ω). After this cleaning phase, we took 5 s of absorption of the odorant (4.8 V for heating, this duration was chosen to avoid saturation), and finally 100 s of desorption (room air again at maximum heating). Five consecutive measurements of all odorants were taken following this protocol to avoid history dependent effects.
Results
The quality control assessment of a clinical olfactory test can use the quantitative comparison of the artificial nose characterization of a brand new test and that of a test that has been used for a certain period of time. Here, we propose a simple quality analysis that consists on the quantitative assessment by the artificial nose of a dilution in the test as compared with the corresponding next dilution of a new test. A given olfactory kit fails the quality test when the response of the artificial nose for a dilution is close to the response obtained for the next dilution in the new test, which contains a lower concentration of the odorant.
As mentioned above, a short version of the CCCRC kit consists in the presentation of dilution number 3. Patients who cannot smell this dilution have a very high probability of olfactory dysfunction (Toledano et al., 2009). To illustrate and test the proposed quality protocol with the artificial nose, we will use dilutions number 2, 3, and 4 of the CCCRC threshold kit. Dilution 2 has a higher concentration of butanol than dilution 3, while dilution 4 has a lower concentration.
Figure 3 shows the response of the artificial nose to these dilutions corresponding to a one year old test (labeled as O for old) and to a new test (labeled as N). This figure illustrates that, after one year, the sensor signal that corresponds to the old dilution number 2 (2O) differs only slightly from the corresponding to the new dilution (2N) and remains far from the signal of the new dilution number 3 (3N). However, the signal corresponding to the old dilution number 3 (3O) is nearly as close to the signal corresponding to new dilution number 4 (4N) as to the new dilution number 3 (3N). Thus, as sensed by the artificial nose, dilution 3O could correspond to the next dilution (lower concentration) of a new test. In this situation, a quality control criteria based in the relative distance between the peaks of the signals would result in discarding the old test kit.
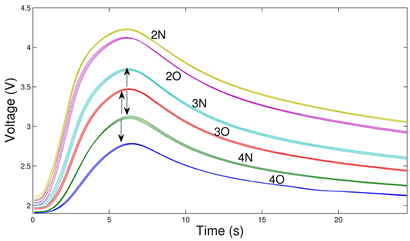
Figure 3. Response of the artificial nose to three dilutions of the CCCRC kit for two different sets: a kit that has been used for over one year (dilutions labeled as 2O, 3O, and 4O) and a new one (dilutions labeled as 2N, 3N, and 4N). For each dilution we show the mean of five measurements (middle trace) together with the standard deviation (upper and lower traces). Note that the artificial nose signal for dilution 3O is nearly as close to 3N as to 4N. A quality control criteria based in the relative distance between the peaks of the signals would result in discarding the old test kit.
For each dilution, the maximum peak is reached a little after the stimulation is stopped. We remind that the protocol that we follow uses five consecutive measurements of the same odorant to avoid history dependent effects between different odorants. Note that we use the same desorption period for all of them and odorants that reach a higher maximum also start from a slightly higher baseline in this protocol.
We have also addressed the analysis with the artificial nose of the identification kit that the subject receives after the threshold kit in the CCCRC test. The purpose of this second kit is the evaluation of the ability to identify an odor. Thus, the analysis of the artificial nose in this case is oriented to determine the stability of the odorant over time. If the odorant changes significantly over the lifespan of the kit (typically one year), this could result in the lack of identification or in a wrong identification of the odorant by the subject. Figure 4 shows the result of this analysis for two odorants (coffee and peanut butter). The aged tests (used clinically for more than one year) show a larger intensity as sensed by the artificial nose. The odorants in this identification kit are hidden under a gauze pad. The increase in signal amplitude observed in these aged jars is probably due to a degenerative chemical reaction or the presence of bacteria in the sample and in the pad because of the prolonged use of the kit. The rest of the odorants in the identification kit present a normal degradation as sensed by the electronic nose.
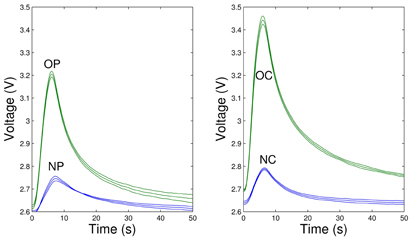
Figure 4. Response of the artificial nose to two samples (peanut butter—left panel and coffee—right panel) of the identification part of the CCCRC kit. For each sample we show the mean of five measurements (middle trace) together with the standard deviation (upper and lower traces). Note that, as sensed by the artificial nose, the old peanut butter (OP) and coffee jars (OC) have higher odor intensity than the ones that contain a new test (NP and NC, respectively).
Discussion
The precise quantification of olfactory perception is an essential step for the evaluation, diagnosis, and treatment of smell disorders and contributes to the success of a corresponding therapy. In the last decades several olfactory tests have been proposed to quantify odor thresholds and to evaluate human odor identification and discrimination. In this paper we have argued that olfactory tests could benefit from quality control performed by artificial noses to assess and guarantee their validity over their lifespan.
We have proposed a protocol to test the quality of a threshold clinical kit by comparing the response of the artificial nose to a given dilution of the test with the immediate next dilution of a new test. We have validated this protocol with the CCCRC threshold kit using an inexpensive chemoresistive sensor, which is a requirement for the successful dissemination of a quality control device for clinical purposes. The protocol does not need a complex processing of the sensor signal such as the use of classification or clustering algorithms required in many applications with artificial noses (Hierlemann and Gutierrez-Osuna, 2008; Muezzinoglu et al., 2010). As illustrated in Figures 3 and 4, the response of the sensor to the odorants in the CCCRC kit is highly reproducible. The comparative nature of the protocol (the results of the artificial nose on an old test are always compared with those of a new test) reduces the dependence of the results on sensor drift and environmental conditions such as temperature and humidity.
Human olfaction and machine olfaction have different mechanisms to sense smell, and thus the quantitative description of an odorant can also be very different for human and artificial noses (Burl et al., 2001; Schiffman et al., 2002; Lewis, 2004; Haddad et al., 2008, 2010). When artificial noses are used to characterize or compare human smell performance, the signals obtained with these devices need to be calibrated and matched to what is considered a normal perception by a human. In the context of clinical olfactory tests where the smell of odorants takes place under controlled conditions, the quantitative description of an odor by an artificial nose can be compared to an established reference to assess the quality of the test. The results shown in this paper indicate that this is possible, and that an inexpensive chemoresistive sensor can be used to characterize the threshold part of the Connecticut Chemosensorial Clinical Research Center Test. We have also reported that some odorants of the identification part of the CCCRC seem to be potentiated as sensed by the artificial nose while others tend to degrade as the test grows older. The analysis of these results suggests that the conditions to keep the odorants in the identification test could be improved.
Further efforts to build a map between human and machine olfaction can largely contribute to the assessment of odor perception and to develop novel protocols for odor impairment diagnosis and treatment. Artificial noses can be used to assess the intensity, steadiness, or temporal evolution of an odorant acting as a stimulus in a perception experiment. These experiments can be carried out with a standard feedback from the self-assessment of smell perception by the subject or through a more quantitative protocol that takes into account levels of brain activity in EEG (Lorig, 2000) and/or fMRI setups (Lombion et al., 2009) (which can largely contribute to a quantitative map between human and machine olfaction). In all cases, the assessment of odor temporal structure and intensity by artificial noses will improve the quantification and the standardization criteria in these protocols. In addition, artificial noses can also be used in closed-loop experiments with olfactometers and other stimulation devices to implement neural activity-dependent stimulation protocols.
Conflict of Interest Statement
The authors declare that the research was conducted in the absence of any commercial or financial relationships that could be construed as a potential conflict of interest.
Acknowledgments
This work was supported by MICINN BFU2009-08473, TIN-2010-19607, and IPT-2011-0727-020000.
References
Albers, M. W., Tabert, M. H., and Devanand, D. P. (2006). Olfactory dysfunction as a predictor of neurodegenerative disease. Curr. Neurol. Neurosci. Rep. 6, 379–386.
Atanasova, B., Graux, J., Hage, W. E., Hommet, C., Camus, V., and Belzung, C. (2008). Olfaction: a potential cognitive marker of psychiatric disorders. Neurosci. Biobehav. Rev. 32, 1315–1325.
Barrios, F. A., Gonzalez, L., Favila, R., Alonso, M. E., Salgado, P. M., Diaz, R., and Fernandez-Ruiz, J. (2007). Olfaction and neurodegeneration in HD. Neuroreport 18, 73–76.
Burl, M. C., Doleman, B. J., Schaffer, A., and Lewis, N. S. (2001). Assessing the ability to predict human percepts of odor quality from the detector responses of a conducting polymer composite-based electronic nose. Sens. Actuators B Chem. 72, 149–159.
Cain, W. S. (1989). Testing olfaction in a clinical setting. Ear Nose Throat J. 68, 316, 316–322, 328.
Doty, R. L. (2008). The olfactory vector hypothesis of neurodegenerative disease: is it viable? Ann. Neurol. 63, 7–15.
Doty, R. L., Shaman, P., and Dann, M. (1984). Development of the University of Pennsylvania Smell Identification Test: a standardized microencapsulated test of olfactory function. Physiol. Behav. 32, 489–502.
Dymerski, T. M., Chmiel, T. M., and Wardencki, W. (2011). The olfactory vector hypothesis of neurodegenerative disease: is it viable? Rev. Sci. Instrum. 82, 111101.
Haddad, R., Carmel, L., Sobel, N., and Harel, D. (2008). Predicting the receptive range of olfactory receptors. PLoS Comput. Biol. 4, e18. Public Library of Science. doi: 10.1371/journal.pcbi.0040018
Haddad, R., Medhanie, A., Roth, Y., Harel, D., and Sobel, N. (2010). Predicting odor pleasantness with an electronic nose. PLoS Comput. Biol. 6, e1000740. Public Library of Science. doi: 10.1371/journal.pcbi.1000740
Hierlemann, A., and Gutierrez-Osuna, R. (2008). Higher-order chemical sensing. Chem. Rev. 108, 563–613.
Hummel, T., Sekinger, B., Wolf, S. R., Pauli, E., and Kobal, G. (1997). “Sniffin” sticks': olfactory performance assessed by the combined testing of odor identification, odor discrimination and olfactory threshold. Chem. Senses 22, 39–52.
Kobal, G., Klimek, L., Wolfensberger, M., Gudziol, H., Temmel, A., Owen, C. M., Seeber, H., Pauli, E., and Hummel, T. (2000). Multicenter investigation of 1,036 subjects using a standardized method for the assessment of olfactory function combining tests of odor identification, odor discrimination, and olfactory thresholds. Eur. Arch. Otorhinolaryngol. 257, 205–211.
Lewis, N. S. (2004). Comparisons between mammalian and artificial olfaction based on arrays of carbon black-polymer composite vapor detectors. Acc. Chem. Res. 37, 663–672.
Lombion, S., Comte, A., Tatu, L., Brand, G., Moulin, T., and Millot, J. L. (2009). Patterns of cerebral activation during olfactory and trigeminal stimulations. Hum. Brain Mapp. 30, 821–828.
Lorig, T. S. (2000). The application of electroencephalographic techniques to the study of human olfaction: a review and tutorial. Int. J. Psychophysiol. 36, 91–104.
Muezzinoglu, M. K., Vergara, A., Huerta, R., and Rabinovich, M. I. (2010). A sensor conditioning principle for odor identification. Sen. Actuators B Chem. 146, 472–476.
Schiffman, S. S., Gutierrez-Osuna, R., and Nagle, H. T. (2002). “Measuring odor intensity with e-noses and other sensor types,” in Proceedings of the 9th International Symposium on Olfaction and Electronic Nose, (Rome), 68–72.
Stitzel, S. E., Aernecke, M. J., and Walt, D. R. (2011). Artificial noses. Annu. Rev. Biomed. Eng. 13, 1–25.
Toledano, A., González, E., Onrubia, T. J., Herráiz, C., Mate, M. A., García, M., Navarro, M., Plaza, G., Aparicio, J. M., de los Santos, G., and Galindo, N. (2003). The Connecticut Chemosensorial Clinical Research Center olfaction test: values in healthy volunteers. Acta. Otorrinolaringol. Esp. 54, 678–685.
Toledano, A., González, E., Rodríguez, G., and Galindo, N. (2007). The validity of CCCRC test in patients with nasal polyposis. Rhinology 45, 54–58.
Toledano, A., Ruiz, C., Navas, C., Herráiz, C., González, E., Rodríguez, G., and Galindo, A. N. (2009). Development of a short olfactory test based on the Connecticut Test (CCCRC). Rhinology 47, 465–469.
Keywords: electronic noses, quality control of clinical olfactory tests, map of human and machine olfaction, olfaction, anosmia, olfactory dysfunction, artificial noses
Citation: Yáñez DJ, Toledano A, Serrano E, Martín de Rosales AM, Rodríguez FB and Varona P (2012) Characterization of a clinical olfactory test with an artificial nose. Front. Neuroeng. 5:1. doi: 10.3389/fneng.2012.00001
Received: 30 September 2011; Paper pending published: 20 October 2011;
Accepted: 17 January 2012; Published online: 02 February 2012.
Edited by:
Ramón Huerta, University of California, USAReviewed by:
Alberto Ferrus, Cajal Institute (CSIC), SpainRoberto F. Galán, Case Western Reserve University, USA
Copyright: © 2012 Yáñez, Toledano, Serrano, Martín de Rosales, Rodríguez and Varona. This is an open-access article distributed under the terms of the Creative Commons Attribution Non Commercial License, which permits non-commercial use, distribution, and reproduction in other forums, provided the original authors and source are credited.
*Correspondence: Pablo Varona, Grupo de Neurocomputación Biológica, Departamento de Ingeniería Informática, Escuela Politécnica Superior, Universidad Autónoma de Madrid, C/Francisco Tomás y Valiente, 11, 28049 Madrid, Spain. e-mail: pablo.varona@uam.es