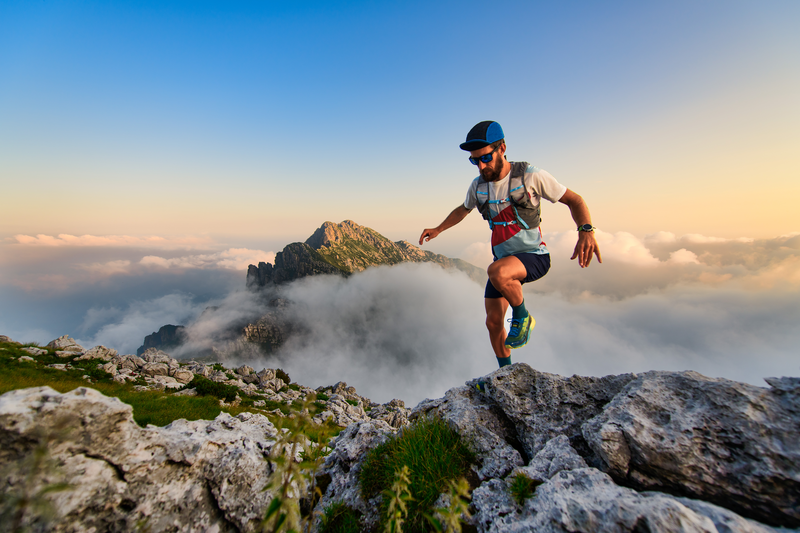
94% of researchers rate our articles as excellent or good
Learn more about the work of our research integrity team to safeguard the quality of each article we publish.
Find out more
METHODS article
Front. Neuroanat. , 18 May 2023
Volume 17 - 2023 | https://doi.org/10.3389/fnana.2023.1176351
This article is part of the Research Topic Methods and Applications in Frontiers in Neuroanatomy View all 8 articles
Stereotaxis is widely used in clinical neurosurgery, neuroradiosurgery, and neuroimaging. Yet, maps of brain structures obtained from post-mortem human brains are not usually presented in known stereotaxic coordinates. Post-mortem brain data given in stereotaxic coordinates would facilitate comparisons with in vivo human neuroimages and would also facilitate intra and inter-experiment comparisons. In this article, we present a crafted instrument for stereotaxic cutting of post-mortem human brain hemispheres. The instrument consists of a transparent methacrylate plate facing a mirror, four legs, and lateral regularly spaced columns permitting the insertion of large knives in-between the columns. This instrument can be built in any laboratory to obtain human brain slabs in the stereotaxic space of Talairach and Tournoux. We explain in detail the procedure for stereotaxic cutting of human brain hemispheres in the coronal plane, as well as the basis for calculating stereotaxic coordinates of histological sections obtained following the stereotaxic cutting protocol.
Stereotaxic (or stereotactic) is a term that refers to procedures “characterized by precise positioning in space; said especially of discrete areas of the brain that control specific functions”; it is usually applied to “types of brain surgery that use a system of three-dimensional coordinates to locate the site to be operated on” (Dorland, 2007). Thus, stereotaxis aims to precisely localize a brain point using three coordinates that are defined as the distance of this point to the three space reference planes (X, Y, and Z). Currently, stereotaxis is regularly used in clinical neurosurgery [including deep brain stimulation, deep electrophysiological recording, and tumor biopsy (Pouratian and Sameer, 2020)], clinical neuroradiosurgery (Sheehan and Lunsford, 2022), and experimental brain research in laboratory animals [e.g., injection of axonal tracers (Joyce et al., 2020; Timbie et al., 2020)].
The history of stereotaxis and its applications to brain research and human neurosurgery has been summarized in several extensive reviews (Gildenberg and Krauss, 2009; Rahman et al., 2009; Rzesnitzek et al., 2019); thus, we briefly mention its most relevant hallmarks. The first stereotaxic apparatus for brain surgery was designed by Horsley and Clarke (1908) with the purpose of producing brain lesions in laboratory animals to study synaptic connections by means of secondary degeneration (Sachs, 1909). In the Horsley and Clarke apparatus, the desired targets in the animal brain were located according to external (skull) fiduciary marks. This led to frequent target errors because the anatomical correlation of brain structures with skull landmarks is poor. Therefore, neurosurgeons could not implement stereotaxic approaches until brain targets could be aimed in relation to landmarks inside the brain itself (Rahman et al., 2009). This was achieved by Spiegel et al. (1947) who used a system of coordinates based on the radiological identification of the ventricles (using pneumoencephalograms) and the pineal gland (identified by its characteristic calcifications in regular roentgenography).
A consequence of the development of human stereotaxic neurosurgery was the elaboration of post-mortem human brain stereotaxic atlases. These atlases were meant to help neurosurgeons to precisely locate brain targets in relation to the described intracerebral references [the features and evolution of human brain stereotaxic atlases have been described in detail by Coffey (2009)]. The most commonly used stereotaxic space in human brain atlases (e.g., Schaltenbrand and Bailey, 1959) relies on a line joining the anterior commissure (AC) and the posterior commissure (PC). This is the criterion followed by Talairach et al. (1957, 1993), Talairach (1967), and Talairach and Tournoux (1988), who set specific guidelines for accurate alignment of brain hemispheres to the AC and PC. Talairach realized that the deep brain structures of interest for stereotaxic neurosurgery [e.g., nucleus VIM -ventral intermediate- in the thalamus (Spiegel et al., 1952)] have a consistent anatomical relation with an intercommissural line passing through the superior edge of the AC and the inferior edge of the PC. Thus, the Talairach space defines three planes: the midsagittal plane, the horizontal commissural plane perpendicular to the midsagittal plane, and a coronal plane passing through the posterior edge of the AC and perpendicular to the other two planes (Talairach and Tournoux, 1988). These precise guidelines for the alignment of the brain using the AC and PC anatomical references followed by Talairach and Tournoux are more specific and reproducible than those of other human atlases that also use AC and PC as main references, like that of Schaltenbrand and Bailey (1959). The latest editions of the Talairach atlas (Talairach and Tournoux, 1988; Talairach et al., 1993) incorporated brain imaging, making its stereotaxic coordinates the most widely used in human brain mapping (Mazoyer, 2008; Cox, 2012; Makris et al., 2017).
Stereotaxic approaches can also be applied to human neuroanatomy by cutting post-mortem human brains within a predetermined stereotaxic space. This approach allows for obtaining thin brain sections that, once processed for molecular markers of interest, can be mapped in known stereotaxic coordinates to facilitate comparison with in vivo brain imaging (e.g., García-Cabezas et al., 2007). In spite of this advantage, the most relevant human neuroanatomical studies of the last decades have been performed in manually cut post-mortem human brains without stereotaxic reference (e.g., Hirai and Jones, 1989). In the present article we describe a stereotaxic apparatus that was developed in our laboratory for cutting post-mortem human brains in the Talairach and Tournoux stereotaxic space. We provide indications to build a similar apparatus in other institutions and exemplify its use to obtain stereotaxic coronal slabs of post-mortem human brains.
As far as we know, there is no commercial human stereotaxic frame for cutting post-mortem brains in stereotaxic planes. Thus, we designed in our laboratory a crafted instrument for stereotaxically cutting human brains in the space of Talairach and Tournoux.
The instrument consists of a transparent methacrylate plate (black arrowhead, Figure 1A) of 30 cm× 25 cm× 1 cm, a mirror (black arrow, Figures 1A, B) of 25 cm× 24.5 cm, four metal legs (green arrowheads, Figures 1A, B) of 2.5 cm× 2.5 cm× 40 cm, 52 metal columns (magenta arrowheads, Figure 1B) of 14 cm height and 0.8 cm in diameter, and a methacrylate base (green arrow, Figure 1A) of 40 cm× 30 cm× 1.5 cm. We describe the spatial relations of all these elements using the cartoons of Figure 2.
Figure 1. Instrument for stereotaxic cutting post-mortem human brains. (A) Photograph of a side view of the instrument showing its components. (B) Photograph of an overhead view of the instrument, showing its components and the carved lines to align the brain. Components of the instrument as labeled in the figure: Methacrylate plate, black arrowhead; mirror, black arrow; methacrylate base, green arrow; metal legs, green arrowheads; metal columns perpendicular to the methacrylate plate, magenta arrowheads; screws to adjust the vertical position of the mirror, orange arrowheads; long line carved onto the methacrylate, blue arrow; short carved lines onto the methacrylate plate, blue arrowheads.
Figure 2. Sketches of the instrument for stereotaxic cutting post-mortem human brains. (A) Overhead view sketch of the methacrylate plate (black arrowhead) and the metal columns (magenta arrowheads), showing the spatial relations between the metal columns, the carved lines (navy blue solid lines), and the planes created by facing slots between the metal columns (sky-blue and navy blue dashed lines). Note that, for simplicity, only 13 metal columns have been represented on each side in these sketches, instead of the 26 of the real instrument (Figure 1). (B) Side sketch of the methacrylate plate and the metal columns, showing the spatial relations between the elements and one of the planes created by facing slots between the metal columns (sky-blue plane). (C) Side sketch of the complete instrument, including the methacrylate plate (black arrowhead), the metal columns (magenta arrowheads), the mirror (black arrow), and the legs (green arrowheads); with a brain placed on the instrument. (D) Detail of the medial face of the brain hemisphere reflected on the mirror, with the anterior (AC) and posterior (PC) commissures intensified and aligned with the lines carved on the methacrylate plate (drawn in navy blue).
First, there is a horizontal transparent rectangular methacrylate plate to hold the brain hemisphere (black arrowhead, Figures 2A–C) supported by four metal legs (green arrowheads, Figure 2C), like a small table. This transparent plate permits visualization from below of structures leaning onto it. The methacrylate plate presents three carved lines that help orienting the brain hemisphere. A long line is carved along the central portion of the methacrylate plate in parallel to its long axis (navy blue arrow, Figures 1B, 2A). Two additional short lines are carved on the methacrylate plate crossing perpendicularly the long line at approximately 1/3 and 2/3 of its length (navy blue arrowheads, Figures 1B, 2A).
Second, the methacrylate plate holds on a series of 26 vertical metal columns (magenta arrowheads, Figures 2A–C) on each of its long margins. The columns (0.8 cm in diameter) form 90° angles with the methacrylate plate, are aligned in parallel to the long carved line, and are separated by open slots of 0.2 cm. Thus, the distance between the center of two consecutive slots is 1 cm (0.8 cm column diameter + 0.2 cm slot). Each column faces another column on the opposite margin of the methacrylate plate. Imaginary planes pass through the slots between two “face-to-face” pairs of columns on each plate margin (sky-blue dotted lines, Figure 2A; sky-blue plane, Figures 2B, C). These imaginary planes are perpendicular to the long carved line (90° angles in Figures 2A, B), and run parallel to the short carved lines. Thus, the distance between two consecutive imaginary planes is 1 cm. Two of those planes pass along the short carved lines (navy blue dotted line, Figures 2A, B).
Third, the legs of the instrument also support a mirror that is placed beneath the methacrylate plate facing upward (black arrow, Figure 2C). This mirror permits that the structures on the methacrylate plate are reflected and seen by the experimenters while placing and cutting the brain. Thus, the long and short lines carved on the methacrylate plate as well as the medial surface of a brain hemisphere resting on the plate are reflected on the mirror (Figure 2C). This allows for precise alignment of hallmarks in the medial face of the hemisphere (AC and PC) with the carved lines (Figure 2D). The heigh of the mirror can be modified by four adjustable screws, one in each leg (orange arrowheads, Figure 1A).
The slots between the metal columns permit the insertion of a sharp blade through them, as shown by the sky-blue plane in Figures 2B, C. The blades are custom-made, 1.5 mm thick; their cutting edge is of the Scandinavian or V grind type (symmetrical, V shaped, sharped cutting edge). Therefore, the cutting edge of the blade cuts through the central plane of the slot and the distance between two consecutive cutting planes is 1 cm. The orientation of the blade passing through the slots is perpendicular to the methacrylate plate and to the long carved line (90° angles in Figures 2A, B). While stored, the blades are kept cushioned onto polyurethane foam to minimize blunting of the cutting edge. After each use, the knifes are cleaned with running tap water, rinsed in ethanol, and dried with absorbent paper. When necessary, blades are sharpened by a professional knife grinder.
We exemplify the use of the instrument for human brain stereotaxic cutting using one post-mortem human brain obtained from the Program of Body Donation for Teaching and Research of the School of Medicine of Autónoma University of Madrid; the use of this brain for the present study was approved by the Ethics Committee for Research of Autónoma University of Madrid (Authorization CEI-104-2011).
After fixation, either by immersion or perfusion, the post-mortem human brain is cut through the midline to separate the hemispheres. Brain hemispheres can be blocked together with the brainstem and cerebellum or the brainstem and cerebellum can be initially separated from the cerebrum by cutting through the interpeduncular fossa and the superior edge of the lamina quadrigemina. The two hemispheres are separated with a sagittal cut passing through the corpus callosum and the plane between the mammillary bodies, the frontal lobes, and the occipital lobes. This cutting is made from ventral to dorsal to have full view and control of the plane of cutting: one experimenter holds and stabilizes the brain with his/her hands on the dorsal surface of the hemispheres, exposing the ventral surface; then, another experimenter cuts between the hemispheres. Separating the two hemispheres is necessary because the AC and PC can only be identified in the sectioned midsagittal plane; AC and PC are the intracerebral references for stereotaxic blocking according to Talairach and Tournoux (1988). The medial face of one brain hemisphere is placed on the methacrylate plate facing the mirror (Figure 3A). Then, several hallmarks on the medial surface of the hemisphere are aligned with the carved lines on the methacrylate plate with the help of the mirror (Figures 2D, 3B, C). Specifically: the superior edge of the AC and the inferior edge of the PC are aligned with the long carved line on the methacrylate plate; also, the posterior edge of the AC is aligned with one of the two short carved lines (Figures 2D, 3C). Following these alignments, the hemisphere is oriented according to the stereotaxic space of Talairach and Tournoux (1988).
Figure 3. Steps for cutting post-mortem human brains in the stereotaxic instrument. (A,B) Brain hemisphere placed in the stereotaxic instrument, with the superior edge of the anterior commissure (AC) and the inferior edge of the posterior commissure (PC) aligned with the long line carved on the methacrylate plate [long blue line in panels (B,C)], and the posterior edge of the AC aligned with one the short lines carved on the methacrylate plate [short blue line in panels (B,C)]. (C) Detail of the AC and PC alignments. (D) A first blade is inserted in the slot between the metal columns corresponding with the position of the short carved line on the methacrylate plate, aligned with the posterior edge of the AC, which corresponds to coronal plane 0 of Talairach and Tournoux (1988). (E) A second blade is passed through the next slot toward the occipital pole, to obtain the coronal slab –1. (F) Superior view of coronal slab –1, in between the two blades. (G) The first blade is removed from the first slot and is introduced in the next slot toward the occipital pole, to obtain the coronal slab –2. Note the presence of coronal slab –1 in front of the blade (black arrowheads).
Once the hemisphere is aligned, a sharp blade is passed through the slot between the metal columns that corresponds with the short carved line aligned with the posterior edge of the AC (Figure 3D). This plane of cutting corresponds to coronal plane 0 of Talairach and Tournoux (1988). Then, another blade is passed through the next slot toward the occipital pole of the hemisphere (Figure 3E) to obtain a 1 cm thick coronal brain slab (Figure 3F); this slab is number −1 and its posterior surface is 1 cm from plane 0. The next step consists in removing the first blade and passing it through the next slot toward the occipital pole to obtain another 1 cm thick coronal brain slab (Figure 3G), whose anterior surface and posterior surfaces are 1 and 2 cm from plane 0, respectively. These procedures are repeated until the occipital pole is reached. The slabs cut posterior to the AC are numbered with negative values (Figure 4A).
Figure 4. Coronal slabs from a human post-mortem brain obtained stereotaxically. (A) Coronal slabs obtained from the post-mortem human right hemisphere shown in Figure 3 using the stereotaxic instrument. Slabs are placed on the surgical drape on their anterior surface, thus their posterior surface is shown. Slabs are ordered from the frontal pole (S6, left upper corner) to the occipital pole (S –10, right lower corner). Slabs anterior/frontal to the coronal plane 0 are numbered with positive numbers (S1, S2… S6), while slabs posterior/occipital to the coronal plane 0 are numbered with negative numbers (S–1, S–2… S–10). (B) Posterior surface of coronal slab 1 (S1). (C) Anterior surface of coronal slab –1 (S –1). Note that the plane between panels (B,C) is the coronal anteroposterior plane 0. AC, anterior commissure; Amy, amygdala; Ca, caudate; cc, corpus callosum, ci, internal capsule; Cl, claustrum; GP, globus pallidus; Pu, putamen.
After the posterior part of the hemisphere is cut, one blade is passed again through the slot corresponding to coronal plane 0. The same procedures described for cutting the part of the hemisphere posterior to the AC are repeated until the frontal pole is reached. Special care must be paid when cutting through the most anterior portion of the temporal pole that is not attached to the insula and the posterior orbitofrontal cortex because it does not hold with the reminder of the temporal lobe and can be squeezed by the blade. The slabs cut anterior to the AC are numbered with positive values (Figure 4A).
The instrument presented in this article is designed for stereotaxic coronal cutting of human brain hemispheres. However, cutting brains in the horizontal plane could be done with a similar instrument following analogous alignments: the long carved line on the methacrylate plate should run parallel to the planes of cutting through the slots, instead of perpendicular to them.
The coronal slabs obtained from a post-mortem human brain hemisphere using the instrument and methods described in the preceding sections are shown in Figure 4A. Stereotaxic cutting as described in the present article yields coronal brain slabs oriented in the space of Talairach and Tournoux. Also, each and every slab, with the exception of those corresponding to the frontal and occipital poles and the loose part of the temporal pole, have the same thickness: 1 cm.
The stereotaxic slabs can be postfixed if fixation was not complete. Then, whole coronal slabs, or parts of them containing a region of interest (like the thalamus), that we name blocks, can be cryoprotected and cut on a freezing microtome to collect all the sections in consecutive series as described (García-Cabezas et al., 2007). Coronal plane 0 of the Talairach and Tournoux space is between the posterior surface of slab 1 (Figure 4B) and the anterior surface of slab −1 (Figure 4C). In these surfaces, AC is clearly visible (Figures 4B, C). Thus, the anterior surface of the first thin section obtained from microtome sectioning blocks from slab −1, starting on the anterior block face, at 50 μm thickness, corresponds to coronal plane 0 and its posterior surface corresponds to coronal plane −50 μm.
We exemplify next the calculation of stereotaxic coordinates of a given histological section obtained from a stereotaxically cut post-mortem human brain. First, we select the brain slab with the region of interest and the block containing this region is separated from the rest of the slab (e.g., the posterior hippocampal formation at the level of the splenium of the corpus callosum; Figure 5A). Then, the block is cryoprotected and is freeze-sectioned in a freezing microtome, collecting the consecutive sections in the desired number of series. For example, to collect 10 series, each of the 10 first cut sections will be the first section in each of the ten series, which are collected separately. Then, section number 11 is section 2 of series 1 (and can be collected together with section 1 of the same series or on a separate well); section number 12 is section 2 of series 2, section number 13 is section 2 of series 3… and so on until section number 20, which is section 2 of series 10. Then, the procedure is repeated with section number 3 of each series (from cut section 21 to cut section 30). This serial sectioning is continued until the posterior face of the block is reached.
Figure 5. Example of human brain histological processing in known stereotaxic coordinates of the Talairach and Tournoux space. (A) Macroscopic picture of the posterior surface of slab –5 from a right human hemisphere. The region containing the posterior hippocampus has been dissected in a block for microtome sectioning and histological processing. The slab is soaked in an ethylene-glycol cryoprotective solution; thereby the glitter throughout (some prominent glitter has been toned down to improve visualization). (B) Microphotograph from a posterior view of a coronal section from the block shown in A processed for acetylcholinesterase, in an anteroposterior (AP) level near the posterior surface of the slab. Note that a precise stereotaxic AP coordinate is given, as well as the position of the section in the slab. Topographical references, such as sulci (red arrowheads) and ventricle angles (black arrowheads) have been indicated to facilitate comparison between the two photographs. LV, lateral ventricle; or, optic radiation; pHp, posterior hippocampus; sp, splenium of corpus callosum; t, tapetum.
Consequently, anteroposterior coordinates of any histological section from stereotaxically cut human brains can be calculated using several parameters: the section thickness, the series number, the section number, and the total number of series collected (Figures 5, 6). While cutting in the microtome, each section is labeled with a series and a section number. Because the section and series numbers are used later to calculate AP levels, we provide a formula to calculate these levels using section and series numbers, considering that the slabs are cut from anterior to posterior. The following equations provide the AP level of the anterior surface of any section (Figure 6):
Figure 6. Graphic representation of AP level calculations of histological sections. (A) Schematic representation of slabs obtained from a right human hemisphere (Figures 3, 4). (B) Schematic representation of typical serial sectioning of a posterior slab (slab –7) with AP levels indicated in relevant positions: sections are 50 μm thick and 10 series are collected. Note than in this case, sections between the same series would be 500 μm (0.5 mm) apart. A random section as example is highlighted in yellow and AP calculations following the equation provided in the article is shown. (C) Typical serial sectioning of an anterior slab (slab 3) with AP levels indicated in relevant positions, and AP level calculated for a random section (highlighted in yellow).
For slabs anterior to AP 0: [Slab# × 10 mm]–[(section# −1) × (section thickness × number of series)]–[(series#–1) × section thickness].
For slabs posterior to AP 0: [(Slab# + 1) × 10 mm]–[(section# −1) × (section thickness × number of series)]–[(series#–1) × section thickness].
Most articles on post-mortem human brain anatomy use thin brain sections obtained from manually cut brains (e.g., Hirai and Jones, 1989; Zilles et al., 1995). Actually, stereotaxic cutting of post-mortem human brains in the Talairach and Tournoux space (García-Cabezas et al., 2007; Pérez-Santos, 2021) is quite uncommon in contemporary human neuroanatomy. Nonetheless, earlier procedures for stereotaxic cutting of post-mortem human hemispheres have been described (Schaltenbrand and Bailey, 1959; Insausti et al., 1995). These procedures do not conform to the Talairach and Tournoux criteria, which is the most widely used in current neuroimaging. In line with this, the resourceful procedure described by Insausti et al. (1995) does not permit precise calculation of the stereotaxic coronal planes of actual brain slices, although it permits cutting human hemispheres consistently.
Precise stereotaxic cutting of post-mortem human brains is commendable because it provides slabs and blocks of human brain tissue than can be cut in histological thin sections of known position along the (anterior-posterior) axis perpendicular to the (coronal) plane of cutting. Thus, the coordinates in the space of Talairach and Tournoux on a given thin section can be known. This is useful, for example, to obtain maps of specific innervation (e.g., dopaminergic axons) in deep brain gray matter structures, like the thalamus (García-Cabezas et al., 2007). These maps can be easily collated with MR images obtained in the Talairach and Tournoux space, and permit extrapolation of post-mortem results to in vivo imaging.
The device described and illustrated in this article is proposed to neuroscientists working with post-mortem human brains, so that brain slices conform to the Talairach and Tournoux space, and data can be offered in this space. This approach should be particularly useful to compare experimental post-mortem data from human brains with in vivo neuroimaging data, as well as to make accurate comparisons of data from different laboratories/studies.
The original contributions presented in the study are included in the article, further inquiries can be directed to the corresponding author.
CC developed the original conception and design of the stereotaxic device and read and corrected the manuscript. MG-C and IP-S wrote the first draft of the manuscript. All authors commented on previous versions of the manuscript, read, and approved the final manuscript.
CC and IP-S were the recipients of grants from the Chair in Neuroscience UAM-Fundación Tatiana Pérez de Guzmán el Bueno, Spain. MG-C was the recipient of a Beatriz Galindo senior research position in the School of Medicine at Universidad Autónoma de Madrid (BEAGAL18/00098) and of a Grant for I+D Projects to the Beatriz Galindo Program Researchers at Universidad Autónoma de Madrid (SI2/PBG/2020-00014) from the Madrid Government (Comunidad de Madrid-Spain) under the Multiannual Agreement with Universidad Autónoma de Madrid in the line of action encouraging young research doctors, in the context of the V PRICIT (Regional Program of Research and Technological Innovation).
We gratefully acknowledge brain donors of the Program of Body Donation for Teaching and Research of the School of Medicine of Autónoma University of Madrid (UAM). We give special thanks to the personnel at the warehouse of the UAM Interdepartmental Research Service (Sidi), where the stereotaxic device was built.
The authors declare that the research was conducted in the absence of any commercial or financial relationships that could be construed as a potential conflict of interest.
All claims expressed in this article are solely those of the authors and do not necessarily represent those of their affiliated organizations, or those of the publisher, the editors and the reviewers. Any product that may be evaluated in this article, or claim that may be made by its manufacturer, is not guaranteed or endorsed by the publisher.
Coffey, R. J. (2009). “Printed Stereotactic Atlases, Review,” in Textbook of stereotactic and functional neurosurgery, eds A. M. Lozano, P. L. Gildenberg, and R. R. Tasker (Heidelberg: Springer), 347–371. doi: 10.1007/978-3-540-69960-6_25
Cox, R. W. (2012). AFNI: What a long strange trip it’s been. Neuroimage 62, 743–747. doi: 10.1016/j.neuroimage.2011.08.056
García-Cabezas, M. Á., Rico, B., Sánchez-González, M. Á., and Cavada, C. (2007). Distribution of the dopamine innervation in the macaque and human thalamus. Neuroimage 34, 965–984. doi: 10.1016/j.neuroimage.2006.07.032
Gildenberg, P. L., and Krauss, J. K. (2009). “History of Stereotactic Surgery,” in Textbook of stereotactic and functional neurosurgery, eds A. M. Lozano, P. L. Gildenberg, and R. R. Tasker (Heidelberg: Springer Berlin), 1–33. doi: 10.1007/978-3-540-69960-6_1
Hirai, T., and Jones, E. G. (1989). A new parcellation of the human thalamus on the basis of histochemical staining. Brain Res. Brain Res. Rev. 14, 1–34. doi: 10.1016/0165-0173(89)90007-6
Horsley, V., and Clarke, R. H. (1908). The structure and functions of the cerebellum examined by a new method. Brain 31, 45–124. doi: 10.1093/brain/31.1.45
Insausti, R., Tunon, T., Sobreviela, T., Insausti, A. M., and Gonzalo, L. M. (1995). The human entorhinal cortex: A cytoarchitectonic analysis. J. Comp. Neurol. 355, 171–198. doi: 10.1002/cne.903550203
Joyce, M. K. P., García-Cabezas, M. Á., John, Y. J., and Barbas, H. (2020). Serial prefrontal pathways are positioned to balance cognition and emotion in primates. J. Neurosci. 40, 8306–8328. doi: 10.1523/JNEUROSCI.0860-20.2020
Makris, N., Zhu, A., Papadimitriou, G. M., Mouradian, P., Ng, I., Scaccianoce, E., et al. (2017). Mapping temporo-parietal and temporo-occipital cortico-cortical connections of the human middle longitudinal fascicle in subject-specific, probabilistic, and stereotaxic Talairach spaces. Brain Imaging Behav. 11, 1258–1277. doi: 10.1007/s11682-016-9589-3
Mazoyer, B. (2008). In memoriam: Jean Talairach (1911-2007): A life in stereotaxy. Hum. Brain Mapp. 29, 250–252. doi: 10.1002/hbm.20473
Pérez-Santos, I. (2021). Noradrenaline in the primate thalamus: Axon ad receptor distributions in macaques and humans. PhD thesis. Madrid: Autónoma University of Madrid.
Pouratian, N., and Sameer, A. S. (2020). Stereotactic and functional neurosurgery: Principles and applications. Cham: Springer Nature Swizerland. doi: 10.1007/978-3-030-34906-6
Rahman, M., Murad, G. J., and Mocco, J. (2009). Early history of the stereotactic apparatus in neurosurgery. Neurosurg. Focus 27:E12. doi: 10.3171/2009.7.FOCUS09118
Rzesnitzek, L., Hariz, M., and Krauss, J. K. (2019). The origins of human functional stereotaxis: A reappraisal. Stereotact. Funct. Neurosurg. 97, 49–54. doi: 10.1159/000496157
Sachs, E. (1909). On the structure and functional relations of the optic thalamus. Brain 32, 95–186. doi: 10.1093/brain/32.2.95
Schaltenbrand, G., and Bailey, P. (1959). Introduction to stereotaxis with an atlas of the human brain. Stuttgart: Georg Thieme Verlag.
Sheehan, J. P., and Lunsford, L. D. (2022). Intracranial stereotactic radiosurgery. Boca Raton, FL: CRC Press.
Spiegel, E. A., Wycis, H. T., and Freed, H. (1952). Stereoencephalotomy in thalamotomy and related procedures. J. Am. Med. Assoc. 148, 446–451. doi: 10.1001/jama.1952.02930060028009
Spiegel, E. A., Wycis, H. T., Marks, M., and Lee, A. J. (1947). Stereotaxic apparatus for operations on the human brain. Science 106, 349–350. doi: 10.1126/science.106.2754.349
Talairach, J. (1967). Atlas d’anatomie steìreìotaxique du teìlenceìphale, études anatomo-radiologiques. Paris: Masson et Cie.
Talairach, J., David, M., Tournoux, P., Corredor, H., and Kvasina, T. (1957). Atlas d’anatomie stéréotaxique. repérage radiologique indirect des noyaux gris centraux des régions mésencephalo-sous-optique et hypothalamique de l’homme. Paris: Masson & Cie.
Talairach, J., and Tournoux, P. (1988). Co-planar stereotaxic atlas of the human brain. New York, NY: Thieme.
Talairach, J., Tournoux, P., and Missir, O. (1993). Referentially oriented cerebral MRI Anatomy: An atlas of stereotaxic anatomical correlations for gray and white matter. Stuttgart, NY: Thieme Medical Publishers.
Timbie, C., García-Cabezas, M. Á., Zikopoulos, B., and Barbas, H. (2020). Organization of primate amygdalar-thalamic pathways for emotions. PLoS Biol. 18:e3000639. doi: 10.1371/journal.pbio.3000639
Keywords: stereotaxis, stereotaxic instrument, Talairach, human neuroanatomy, intercommissural plane
Citation: García-Cabezas MÁ, Pérez-Santos I and Cavada C (2023) Stereotaxic cutting of post-mortem human brains for neuroanatomical studies. Front. Neuroanat. 17:1176351. doi: 10.3389/fnana.2023.1176351
Received: 28 February 2023; Accepted: 24 April 2023;
Published: 18 May 2023.
Edited by:
Clement Ricard, Université Paris Cité, FranceReviewed by:
Carlos De La Rosa-Prieto, University of Castilla-La Mancha, SpainCopyright © 2023 García-Cabezas, Pérez-Santos and Cavada. This is an open-access article distributed under the terms of the Creative Commons Attribution License (CC BY). The use, distribution or reproduction in other forums is permitted, provided the original author(s) and the copyright owner(s) are credited and that the original publication in this journal is cited, in accordance with accepted academic practice. No use, distribution or reproduction is permitted which does not comply with these terms.
*Correspondence: Carmen Cavada, Y2FybWVuLmNhdmFkYUB1YW0uZXM=
†These authors have contributed equally to this work and share first authorship
Disclaimer: All claims expressed in this article are solely those of the authors and do not necessarily represent those of their affiliated organizations, or those of the publisher, the editors and the reviewers. Any product that may be evaluated in this article or claim that may be made by its manufacturer is not guaranteed or endorsed by the publisher.
Research integrity at Frontiers
Learn more about the work of our research integrity team to safeguard the quality of each article we publish.