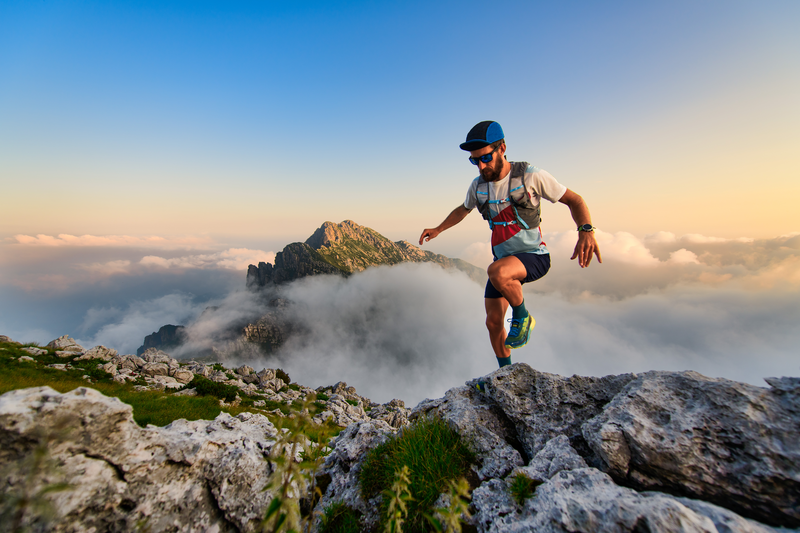
94% of researchers rate our articles as excellent or good
Learn more about the work of our research integrity team to safeguard the quality of each article we publish.
Find out more
EDITORIAL article
Front. Neuroanat. , 27 October 2022
Volume 16 - 2022 | https://doi.org/10.3389/fnana.2022.1060405
This article is part of the Research Topic The Human Brain Multiscale Imaging Challenge View all 5 articles
Editorial on the Research Topic
The human brain multiscale imaging challenge
The combination of tissue preparation techniques (Costantini et al., 2019), advanced optical microscopy (Abdelfattah et al., 2022), and big data analysis is revolutionizing the way of studying the brain anatomy (Ueda et al., 2020). These techniques already allowed mapping of cell distribution or reconstruction of neuronal circuits in whole mouse brains (BRAIN Initiative Cell Census Network, 2021; Silvestri et al., 2021). However, the analysis of the human brain is still in its infancy. Such specimens present specific challenges that need to be solved in comparison to animal models: massive dimension of the specimen (up to several cm3), geometry, variability of post-mortem fixation conditions and storage, presence of blood inside the vasculature, autofluorescence signals from lipofuscin-type pigments, and consistency of cellular labeling. In addition, alteration of antigens, due to fixation and storage conditions, may prevent reliable immunostaining (Weiss et al., 2021; Pesce et al., 2022). Various optical technologies have started to address human brain reconstruction in combination with advanced staining methods or relying on label-free detections (Axer et al., 2016; Wang et al., 2018; Menzel et al., 2020; Costantini et al., 2021a) but much remains to be done. Moreover, the capability of achieving the reconstruction of human brains has also raised the problem of creating new software platforms that enable to manage, analyze, and share TB-sized volumetric images (Tyson and Margrie, 2022). In this collection various methodologies are proposed to perform human brain study from the macro- to the microscale. These studies are summarized in the following paragraphs.
In this study, Scardigli et al. evaluated different tissue transformation approaches to find the best solution to uniformly clear and label all neurons in the human cerebral cortex to perform 3D reconstruction with light-sheet fluorescence microscopy (LSFM) (Hillman et al., 2019). They applied to adult human brain samples various tissue transformation protocols: CLARITY (Chung et al., 2013; Costantini et al., 2015), SWITCH (Murray et al., 2015; Costantini et al., 2021b), SHIELD (Park et al., 2018), and ExM (Expansion Microscopy) (Chen et al., 2015). Then they optimize a specific procedure to obtain homogeneous staining of the samples with high-density epitopes marker such as NeuN (neuronal nuclear antigen). Finally, they performed mesoscopic high-resolution 3D reconstruction of the successfully cleared and immunostained samples with a custom-made LSFM.
Menzel et al. developed a method based on visual light scattering (Scattered Light Imaging SLI, introduced in Menzel et al., 2021) to infer fiber orientations, particularly in crossing fiber configurations, in unstained histological brain sections. They used a display with individually controllable light-emitting diodes to measure the full distribution of scattered light behind the sample (scattering pattern) for each image pixel recorded at once, enabling scatterometry measurements of whole brain tissue samples. Finally, they performed SLI scatterometry measurements of a human brain section with 3-μm in-plane resolution, demonstrating that the technique is a powerful approach to gain new insights into the nerve fiber architecture of the human brain and to construct a detailed network model of the brain.
In the presented work, Ellis and Aizenberg aim to obtain information that can accurately predict variations in task activation between individuals from structural imaging. To this end, they trained a convolutional neural network to use structural imaging (T1-weighted, T2-weighted, and diffusion tensor imaging) to predict 47 different functional MRI task activation volumes across seven task domains. These results obtained indicate that indeed structural imaging contains information that is predictive of inter-subject variability in task activation mapping and that cortical folding patterns, as well as microstructural features, could be a key component to linking brain structure to brain function.
The purpose of Zheng et al. is to investigate the application of 3D arterial spin labeling (3D-ASL) (Zhang et al., 2015) for evaluating distal limb ischemic preconditioning (Basalay et al., 2020) to improve acute ischemic stroke (AIS) perfusion. The study is divided in two analysis. The first one is conducted on 40 patients with AIS and 15 healthy individuals in whom diffusion-weighted imaging (DWI), magnetic resonance angiography (MRA), and 3D-ASL, and cerebral infarct volume and cerebral blood flow (CBF) were measured in the area of the infarct lesion. The second part is performed on rats to characterize the cerebral artery occlusion (MCAO) in correlation with limb remote ischemic preconditioning (LRP). From the work on patients the authors found that hypertension and internal carotid atherosclerosis are high-risk factors for ischemic stroke, and CBF values in the infarct area are significantly lower than those in the corresponding regions of the contralateral side. From the second part of the study, they found that LRP reduced cerebral infarct size and improved neurological function in rats after ischemic stroke, while CBF measurements reflected a corresponding improvement in perfusion. The authors concluded that 3D-ASL can be used to evaluate LRP to improve stroke perfusion, and its protective effect may be closely related to LRP-induced vascular regeneration.
Characterizing the human brain cytoarchitecture, myeloarchitecture, and angioarchitecture, remains one of the biggest challenges of neuroscience. Optical imaging is a powerful tool to analyze such networks and their relationships to cellular anatomy at the microscopic level, which is not achievable by traditional imaging techniques such as dMRI and could lead to better understanding of connectivity, brain development and pathology. Addressing the morphomolecular specificities of cellular diversity globally in the human brain at multiple levels of resolution requires a large-scale, convergent efforts from several disciplines. The papers included in the present collection demonstrates that only the combination of knowledge among various fields, methods, and modalities allows describing the complexity of the brain in an integrated and useful manner. This approach reflects the efforts of massive projects such as the BRAIN Initiative Cell Census Network (BICCN), the BRAIN Initiative Cell Atlas Network (BICAN), and the Human Brain Project (HBP). Realizing human brain atlases with a comprehensive structural and molecular characterization at the cellular level will allow to determine the roles of the different cell types in health and disease, explain the functional alterations occurring in specific cell populations and brain regions as part of a given disease process, opening the possibility of deeply understanding brain's functionalities, while providing accessible, permanent high-quality datasets to the scientific community.
All authors listed have made a substantial, direct, and intellectual contribution to the work and approved it for publication.
This project received funding from the European Union's Horizon 2020 Framework Programme for Research and Innovation under the Specific Grant Agreement No. 785907 (Human Brain Project SGA2) and No. 945539 (Human Brain Project SGA3), from the General Hospital Corporation Center of the National Institutes of Health under award number U01 MH117023, and from the Italian Ministry for Education in the framework of Euro-Bioimaging Italian Node (ESFRI research infrastructure). This project has also been made possible in part by Grant No. 2019-198101 from the Chan-Zuckerberg Initiative DAF, an advised fund of the Silicon Valley Community Foundation. Finally, this research was carried out with the contribution from Fondazione CR Firenze.
We deeply thank all the authors and reviewers who have participated in this Research Topic.
The authors declare that the research was conducted in the absence of any commercial or financial relationships that could be construed as a potential conflict of interest.
All claims expressed in this article are solely those of the authors and do not necessarily represent those of their affiliated organizations, or those of the publisher, the editors and the reviewers. Any product that may be evaluated in this article, or claim that may be made by its manufacturer, is not guaranteed or endorsed by the publisher.
The content of this work is solely the responsibility of the authors and does not necessarily represent the official views of the National Institutes of Health.
Abdelfattah, A. S., Ahuja, S., Akkin, T., Allu, S. R., Brake, J., Boas, D. A., et al. (2022). Neurophotonic tools for microscopic measurements and manipulation: status report. Neurophotonics 9, 013001. doi: 10.1117/1.NPh.9.S1.013001
Axer, M., Strohmer, S., Grassel, D., Bucker, O., Dohmen, M., Reckfort, J., et al. (2016). Estimating fiber orientation distribution functions in 3D-polarized light imaging. Front. Neuroanat. 10, 40. doi: 10.3389/fnana.2016.00040
Basalay, M. V., Wiart, M., Chauveau, F., Dumot, C., Leon, C., Amaz, C., et al. (2020). Neuroprotection by remote ischemic conditioning in the setting of acute ischemic stroke: a preclinical two-centre study. Sci. Rep. 10, 16874. doi: 10.1038/s41598-020-74046-4
BRAIN Initiative Cell Census Network (2021). A multimodal cell census and atlas of the mammalian primary motor cortex. Nature 598, 86–102. doi: 10.1038/s41586-021-03950-0
Chen, F., Tillberg, P. W., and Boyden, E. S. (2015). Optical imaging. Expansion microscopy. Science 347, 543–548. doi: 10.1126/science.1260088
Chung, K., Wallace, J., Kim, S. Y., Kalyanasundaram, S., Andalman, A. S., Davidson, T. J., et al. (2013). Structural and molecular interrogation of intact biological systems. Nature 497, 332–337. doi: 10.1038/nature12107
Costantini, I., Baria, E., Sorelli, M., Matuschke, F., Giardini, F., Menzel, M., et al. (2021a). Autofluorescence enhancement for label-free imaging of myelinated fibers in mammalian brains. Sci. Rep. 11, 8038. doi: 10.1038/s41598-021-86092-7
Costantini, I., Cicchi, R., Silvestri, L., Vanzi, F., and Pavone, F. S. (2019). In-vivo and ex-vivo optical clearing methods for biological tissues: review. Biomed. Opt. Express 10, 5251–5267. doi: 10.1364/BOE.10.005251
Costantini, I., Ghobril, J. P., Di Giovanna, A. P., Allegra Mascaro, A. L., Silvestri, L., Mullenbroich, M. C., et al. (2015). A versatile clearing agent for multi-modal brain imaging. Sci. Rep. 5, 9808. doi: 10.1038/srep09808
Costantini, I., Mazzamuto, G., Roffilli, M., Laurino, A., Maria Castelli, F., Neri, M., et al. (2021b). Large-scale, cell-resolution volumetric mapping allows layer-specific investigation of human brain cytoarchitecture. Biomed. Opt. Express 12, 3684–3699. doi: 10.1364/BOE.415555
Hillman, E. M. C., Voleti, V., Li, W., and Yu, H. (2019). Light-Sheet microscopy in neuroscience. Annu. Rev. Neurosci. 42, 295–313. doi: 10.1146/annurev-neuro-070918-050357
Menzel, M., Axer, M., De Raedt, H., Costantini, I., Silvestri, L., Pavone, F. S., et al. (2020). Toward a high-resolution reconstruction of 3D nerve fiber architectures and crossings in the brain using light scattering measurements and finite-difference time-domain simulations. Phys. Rev. X 10, 021002. doi: 10.1103/PhysRevX.10.021002
Menzel, M., Reuter, J. A., Grassel, D., Huwer, M., Schlomer, P., Amunts, K., et al. (2021). Scattered light imaging: resolving the substructure of nerve fiber crossings in whole brain sections with micrometer resolution. Neuroimage 233, 117952. doi: 10.1016/j.neuroimage.2021.117952
Murray, E., Cho, J. H., Goodwin, D., Ku, T., Swaney, J., Kim, S. Y., et al. (2015). Simple, scalable proteomic imaging for high-dimensional profiling of intact systems. Cell 163, 1500–1514. doi: 10.1016/j.cell.2015.11.025
Park, Y. G., Sohn, C. H., Chen, R., Mccue, M., Yun, D. H., Drummond, G. T., et al. (2018). Protection of tissue physicochemical properties using polyfunctional crosslinkers. Nat. Biotechnol. doi: 10.1038/nbt.4281
Pesce, L., Scardigli, M., Gavryusev, V., Laurino, A., Mazzamuto, G., Brady, N., et al. (2022). 3D molecular phenotyping of cleared human brain tissues with light-sheet fluorescence microscopy. Commun. Biol. 5, 447. doi: 10.1038/s42003-022-03390-0
Silvestri, L., Mullenbroich, M. C., Costantini, I., Di Giovanna, A. P., Mazzamuto, G., Franceschini, A., et al. (2021). Universal autofocus for quantitative volumetric microscopy of whole mouse brains. Nat. Methods 18, 953–958. doi: 10.1038/s41592-021-01208-1
Tyson, A. L., and Margrie, T. W. (2022). Mesoscale microscopy and image analysis tools for understanding the brain. Prog. Biophys. Mol. Biol. 168, 81–93. doi: 10.1016/j.pbiomolbio.2021.06.013
Ueda, H. R., Erturk, A., Chung, K., Gradinaru, V., Chedotal, A., Tomancak, P., et al. (2020). Tissue clearing and its applications in neuroscience. Nat. Rev. Neurosci. 21, 61–79. doi: 10.1038/s41583-019-0250-1
Wang, H., Magnain, C., Wang, R., Dubb, J., Varjabedian, A., Tirrell, L. S., et al. (2018). as-PSOCT: Volumetric microscopic imaging of human brain architecture and connectivity. Neuroimage 165, 56–68. doi: 10.1016/j.neuroimage.2017.10.012
Weiss, K. R., Voigt, F. F., Shepherd, D. P., and Huisken, J. (2021). Tutorial: practical considerations for tissue clearing and imaging. Nat. Protoc. 16, 2732–2748. doi: 10.1038/s41596-021-00502-8
Keywords: human brain, imaging, multiscale, big data, tissue clearing, cellular atlas
Citation: Costantini I, Axer M, Magnain C and Hof PR (2022) Editorial: The human brain multiscale imaging challenge. Front. Neuroanat. 16:1060405. doi: 10.3389/fnana.2022.1060405
Received: 03 October 2022; Accepted: 14 October 2022;
Published: 27 October 2022.
Edited and reviewed by: Javier DeFelipe, Polytechnic University of Madrid, Spain
Copyright © 2022 Costantini, Axer, Magnain and Hof. This is an open-access article distributed under the terms of the Creative Commons Attribution License (CC BY). The use, distribution or reproduction in other forums is permitted, provided the original author(s) and the copyright owner(s) are credited and that the original publication in this journal is cited, in accordance with accepted academic practice. No use, distribution or reproduction is permitted which does not comply with these terms.
*Correspondence: Irene Costantini, Y29zdGFudGluaUBsZW5zLnVuaWZpLml0
Disclaimer: All claims expressed in this article are solely those of the authors and do not necessarily represent those of their affiliated organizations, or those of the publisher, the editors and the reviewers. Any product that may be evaluated in this article or claim that may be made by its manufacturer is not guaranteed or endorsed by the publisher.
Research integrity at Frontiers
Learn more about the work of our research integrity team to safeguard the quality of each article we publish.