- 1School of Medicine, Institute of Anatomy “Niko Miljanić”, University of Belgrade, Belgrade, Serbia
- 2Department of Physiology, Faculty of Pharmacy, University of Belgrade, Belgrade, Serbia
- 3Pharmacogenetics Section, Department of Physiology and Pharmacology, Karolinska Institutet, Stockholm, Sweden
- 4Group for Molecular Oncology, Institute for Medical Research, University of Belgrade, Belgrade, Serbia
- 5Department of Hematology, Clinical Center “Dragiša Mišović”, Belgrade, Serbia
Early life adversities leave long-lasting structural and functional consequences on the brain, which may persist later in life. Dopamine is a neurotransmitter that is extremely important in mood and motor control. The aim of this study was to investigate the effect of maternal deprivation during the ninth postnatal day on the volume of dopaminergic nuclei and the number of dopaminergic neurons in adolescence and adulthood. Maternally deprived and control Wistar rats were sacrificed on postnatal day 35 or 60, and the dopaminergic neurons were stained in coronal histological sections of ventral midbrain with the tyrosine hydroxylase antibody. The volume of dopaminergic nuclei and the number of dopaminergic neurons in the substantia nigra (SN) and ventral tegmental area (VTA) were analyzed in three representative coordinates. Maternal deprivation caused weight loss on postnatal day 21 (weaning) and corticosterone blood level elevation on postnatal days 35 and 60 in stressed compared to control rats. In maternally deprived animals, the volumes of SN and VTA were increased compared to the controls. This increase was accompanied by an elevation in the number of dopaminergic neurons in both nuclei. Altogether, based on somatic and corticosterone level measurements, maternal deprivation represents a substantial adversity, and the phenotype it causes in adulthood includes increased volume of the dopaminergic nuclei and number of dopaminergic neurons.
Introduction
Development of the nervous system plays a pivotal role in the establishment of healthy adult brain, while stressful events in critical neurodevelopmental periods may cause abberations and maladaptive phenotypes, such as schizophrenia and other neurocognitive disorders (Murray et al., 1992; Waddington et al., 1997). In support of this, maternal deprivation (MD) has been proposed as an animal model for studies of certain neuropsychiatric disorders, including schizophrenia (Ellenbroek and Cools, 2002). Maternal deprivation is an animal model in which rat pups are suddenly separated from their mothers for a defined period of time (Husum and Mathe, 2002; Aksic et al., 2013). Early traumatic experiences are postulated to cause long-term neural changes which are an integral part of the etiology of numerous psychiatric disorders (Llorente et al., 2012). Ellenbroek and Cools (2002) have shown that early maternal deprivation induces schizophrenia-like phenotypes. In experimental animals, early life stress caused by maternal deprivation of pups during a critical period of development is known as the stress hyporesponsive period (SHRP) (Chocyk et al., 2011). The SHRP for rats occurs between postnatal days 4 and 14, and it is a period of time during which pup–mother interactions, such as licking, grooming, and arched-back nursing, suppress the basal and stress-induced levels of glucocorticoids (de Kloet et al., 2005). One of the main biological functions of the SHRP is to protect the developing brain from fluctuations of glucocorticoid levels (Champagne et al., 2009). Glucocorticoids profoundly affect brain development as they are involved in neurogenesis, gliogenesis, myelination, differentiation, and apoptosis (Almeida et al., 2000; Champagne et al., 2009). Adversities during SHRP can profoundly affect the neuronal functioning later in life, and the timing has been shown to be crucial for the development of various phenotypes. It has been previously shown that early maternal deprivation can disrupt normal salience in adult rats and that this phenotype is dependent on the day of deprivation (Ellenbroek and Cools, 2002). In particular, prepulse inhibition was disrupted when deprivation took place on postnatal day 9 (P9), but it remained intact when the rats were deprived on P13 (Ellenbroek et al., 1998; Ellenbroek and Cools, 2002), meaning that prepulse inhibition was most severely affected when deprivation took place around P6–P9, right in the middle of SHRP (Ellenbroek and Cools, 2002).
The substantia nigra (SN) and the ventral tegmental area (VTA) are the principal dopaminergic nuclei with projections throughout the brain and which control various processes. The enrichment of dopaminergic innervation continues to increase until postnatal day 60 in rats, whereas after that point, the density and topography of the dopaminergic afferents remain relatively constant (Suri et al., 2015). Profound changes in the dopaminergic system morphology and signaling caused by early life stress have been observed in a series of previous findings; in particular, maternal deprivation increased the circulating level of dopamine in the rat brain (Huppertz-Kessler et al., 2012) and increased the number of tyrosine hydroxylase positive neurons in the SN (Chocyk et al., 2011) and VTA (Jahng et al., 2010) later in life. In addition, a short period of maternal deprivation increased the expressions of Drd1 and Drd2 throughout many regions of the adult brain (Ploj et al., 2003; Brenhouse et al., 2013; Li et al., 2013). However, relatively little is known regarding the consequences of early life stress on the dopaminergic system morphology during adolescence and adulthood.
Previous studies have shown an important role for glucocorticoids, primarily dexamethasone, which mimic the effects of stress and which can alter the cytoarchitecture and number of dopaminergic cells in the SN and VTA in adult rats when administrated during the prenatal and early postnatal periods (McArthur et al., 2005). Previous morphometric microscopy studies revealed alterations in the neuronal density of the limbic system and temporal and frontal cortices (Arnold and Trojanowski, 1996). During the first 2 weeks of postnatal development, the dopaminergic neurons are particularly sensitive to adversities (Chocyk et al., 2011); therefore, the aim of this study was to investigate the effect of maternal deprivation conducted on the ninth postnatal day on the volume of dopaminergic nuclei and the number dopaminergic neurons in the dopaminergic nuclei in adolescent and adult rats.
Materials and Methods
Animals and Procedures
Male and four nulliparous female Wistar rats (3 months old) were put together in standard Plexiglass cages with normal embedding (26 × 42 × 15 cm) in a temperature-controlled room (23 ± 1°C). The rats were on a standard 12-h light/dark cycle with lights on from 7:00 a.m. to 7:00 p.m., with freely available water and food. Two weeks later, the males (one per each group) were removed, and pregnant female (one per each group) rats were checked twice daily for a potential delivery. The day of delivery was noted as postnatal day 0 (P0). On P9, two litters were weighed and subjected to the maternal deprivation (MD) procedure according to the previously published protocol (Ellenbroek and Cools, 2000; Roceri et al., 2002; Aksic et al., 2013; Aleksic et al., 2016). Briefly, the mothers were removed from the litter at 10:00 a.m., after which the pups were weighed and returned to their home cage. They remained in their home cage at room temperature for 24 h. This meant that the pups also remained without access to food during this period. The mothers of the control litters (two groups) were very briefly (3 min) removed from their home cages. All litters were later left undisturbed, except for the routine cleaning of the cages twice per week, until P21, when the litters were weaned and classified according to gender; at this point, the animals were weighed. For this experiment, 20 animals were divided into four groups, out of which two groups (five control and five MD rats) were sacrificed on P35, which corresponds to periadolescence. The other two groups (five control and five MD rats) were sacrificed at the period of young adulthood (P60). All efforts were made to minimize animal suffering and reduce the number of animals used in the study. All experimental procedures were in compliance with the EEC Directive (2010/63/EU) on the protection of animals used for experimental and other scientific purposes and were approved by the Ethical Committee for the Use of Laboratory Animals of the School of Medicine, University of Belgrade (approval no. 323-07-01245/2014-05/2).
Tissue Processing
The rats were deeply anesthetized and transcardially perfused, starting with a vascular rinse until the liver was discolored (200 ml of 0.9% saline, perfusion speed of 40 ml/min), followed by a 4% paraformaldehyde solution in 0.1 M phosphate-buffered saline (PBS; 200 ml, 100 ml at 40 ml/min and then 30 ml/min), and finally with the 10% sucrose solution in 0.1 M PBS (200 ml, 30 ml/min). The animals’ brains were extracted and cleared of the meninges and blood vessels and then immersed in 4% paraformaldehyde solution overnight. On the next day, the brains were moved to the 30% sucrose solution for several days until they sunk and then were flash frozen. The brains were cut in a coronal plane into 40-μm-thick sections using a cryostat (Leica Instruments, Nußloch, Germany). Free-floating sections were stained, mounted, and coverslipped with DPX (Sigma) and then examined under an Olympus CH2 (Japan) microscope equipped with a camera.
Immunohistochemistry was performed under the same conditions for all experimental samples, and the volume of the dopaminergic nuclei as well as the number of dopaminergic neurons were quantified based on tyrosine hydroxylase (TH) positively immunostained (TH +) cells in the substantia nigra pars reticulata (SNpr), substantia nigra pars compacta (SNpc), and in the ventral tegmental area (VTA). Brain sections were initially thoroughly rinsed with 0.1 M PBS, pH 7.4. The endogenous peroxidase activity was neutralized with 3% hydrogen peroxide/10% methanol for 15 min, and non-specific binding was prevented by 60 min incubation in 5% normal donkey serum (D9663, Sigma-Aldrich, United States)/0.1 M PBS at room temperature. The sections were further incubated for 48 h at 4°C with primary mouse monoclonal anti-TH antibody (dilution 1:1,000, T2928, Sigma-Aldrich, United States) in blocking solution (0.5% Triton X-100 in 0.1 M PBS) and subsequently for 90 min in donkey anti-mouse IgG-HRP (dilution 1:50, sc-2318, Santa Cruz, United States). Between each immunolabeling step, the sections were washed in fresh 0.1 M PBS (3 × 5 min). Immunoreactive signals were visualized with a diaminobenzidine solution [1% 3,3′-diaminobenzidine (11208, Acros Organics)/0.3% hydrogen peroxide/0.1 M PBS]. All sections were finally mounted on slides, dehydrated in a series of solutions with increasing ethanol concentrations (70, 96%, and 100% ethanol; Zorka Pharma, RS), placed in a clearing agent (xylene; Zorka Pharma, RS), mounted with DPX (Sigma-Aldrich, United States), and coverslipped.
Serum Corticosterone Assay
After the sacrifice of animals on P35 and P60, blood was collected from the rats in both the MD and control groups by cardiac puncture. After the blood was harvested, it was allowed to clot for 1 h at room temperature and centrifuged at 2,000 rpm for 10 min at 4°C to obtain the serum. The serum samples were collected into ice-cooled tubes and stored at −20°C until the corticosterone assay. Corticosterone concentration was measured in 1:10 diluted serum samples using a commercially available enzyme immunoassay (EIA) kit (IDS; EURL Paris, France). Briefly, 100 μl of the diluted serum or corticosterone standards was added to the wells of an antibody-coated microtiter plate. Then, 100 μl of the enzyme conjugate was added to each well of the plate and incubated for 24 h at 4°C. After the plate was washed three times with the wash solution, 200 μl of tetramethylbenzidine (TMB) substrate was added to each well and incubated for 30 min at room temperature. The absorbance was read at 450 nm using a microplate reader within 30 min of adding the stop solution. Each sample was run in duplicate and the concentration of corticosterone was determined by using the calibration curve based on the concentrations of the external standards.
Volumetric Measurements of Dopaminergic Nuclei
All the tissue samples of the control and experimental brains (three sections per rat) were grouped into three defined stereotaxic ranges of the overall SNpr, SNpc, and VTA rostro-caudal dimensions. Namely, the volume of each structure was approximated by the surface area in three 40-μm-thick coronal sections per brain, representing the overall rostro-caudal dimension of SNpr, SNpr, and VTA in three defined stereotaxic ranges (4.60–5.10 mm, 5.20–5.70 mm, and 5.80–6.30 mm caudally from bregma). The whole SNpr, SNpc, and VTA were delineated in three stereotaxic ranges and the area was measured from the micrographs using ImageJ 1.46 software. All measurements were performed on both sides of each brain in a defined stereotaxic range, after which the mean value of the measured sides was used as a result for the statistical analysis of each brain. The volumes of the whole SNpr, SNpc, and VTA were approximated by the surface area in three coordinates according to Cavalieri’s principle (Chocyk et al., 2011), i.e., [Area 1 (left side of the section) + Area 2 (right side of the section)]/2 × 0.04 (thickness of the section/1,000).
Image Acquisition and Quantitative Analysis of Tyrosine Hydroxylase (TH +) Cells
Images were taken using an Olympus CH2 (Japan) microscope and analyzed in ImageJ 1.46 software. Tyrosine hydroxylase-positive cells were counted in stereological sections of the rat brains through the overall rostro-caudal dimension of SNpr, SNpc, and VTA in three defined stereotaxic ranges (4.60–5.10 mm, 5.20–5.70 mm, and 5.80–6.30 mm caudally from bregma). All counts were performed on both sides of each brain in a defined stereotaxic range, after which the mean value of the measured sides was taken for each brain as results for statistical analysis.
Statistical Analysis
All numerical data are presented as group mean values with standard errors of the mean (SEM). All statistical analyses were done in the IBM SPSS 11.0 software using Student’s t test. The differences were considered significant if the p-value was lower than 0.05.
Results
To address whether maternal deprivation affected the animals’ development and growth, the animals were weighed upon separation from their mothers. At weaning, maternal deprivation caused a profound 56% loss of weight in stressed rats compared with the controls (Figure 1A). To address whether maternal deprivation affected the glucocorticoid levels in adolescent and adult animals, the concentration of circulating corticosterone was measured in the blood of the rats sacrificed on postnatal days 35 and 60 (P35 and P60, respectively). Maternally deprived animals exhibited 1.9- and 1.4-fold elevations in blood corticosterone levels on P35 and P60 (Figure 1B), respectively. This implies that the early life adversity caused profound phenotype changes connected with the early development arrest and the elevation of glucocorticoid levels at later stages of life, more so in adolescence than in adulthood.
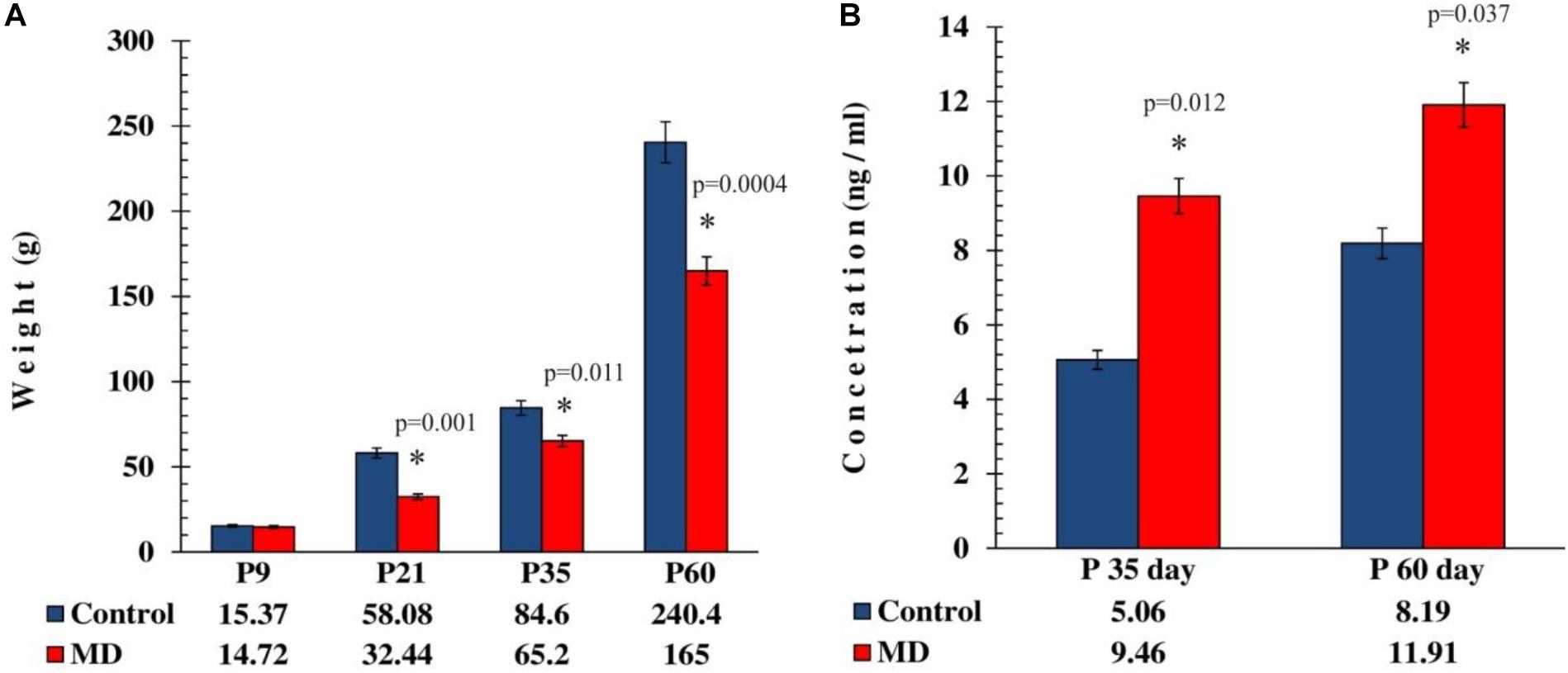
Figure 1. Effect of maternal deprivation on the animals’ weight at weaning age and on the corticosterone levels in adolescence and adulthood. All results are presented as the mean values ± SEM. Asterisk indicates significant between-group differences of the mean values (Student’s t-test for two independent groups, p < 0.05). (A) The body weight reduction in maternally deprived animals at weaning period, P35 and P60, was reduced compared with the controls (p < 0.05), while on P9 there was no statistically significant difference (p > 0.05). (B) The corticosterone levels were elevated in maternally deprived rats compared with the controls in adolescence (p < 0.05) and adulthood (p < 0.05).
To address whether maternal deprivation affected the volume and composition of dopaminergic nuclei in adolescence and adulthood, histological slides containing coronal sections of ventral midbrain prepared from postmortem rat brains sacrificed on P35 and P60 were analyzed. The volume of the outlined nuclei was assessed for volumetric changes approximated by the surface area in three coordinates, whereas the number of tyrosine hydroxylase-positive (TH +) neurons was used to assess the changes in the number of dopaminergic neurons. On P35, 1.7-, 3. 1-, and 2.8-fold increases in the volume and 1.7-, 1.6-, and 1.6-fold increases in the number of TH + neurons were observed in SNpr, SNpc, and VTA, respectively (Figures 2A–F and Tables 1, 2). These increases were observed along the whole anteroposterior axis of the analyzed nuclei. On P60, 1.2-, 1.3-, and 1.7-fold increases in the volume of dopaminergic nuclei and 1.7-, 2.3-, and 2.6-fold increases in the number of TH + neurons were observed in SNpr, SNpc, and VTA, respectively (Figures 3A–F and Tables 1–3). These increases were observed along the whole anteroposterior axis of the analyzed nuclei. Altogether, early life adversity leads to a long-lasting pronounced increase in the volume of dopaminergic nuclei and the number of dopaminergic neurons among all major dopaminergic nuclei.
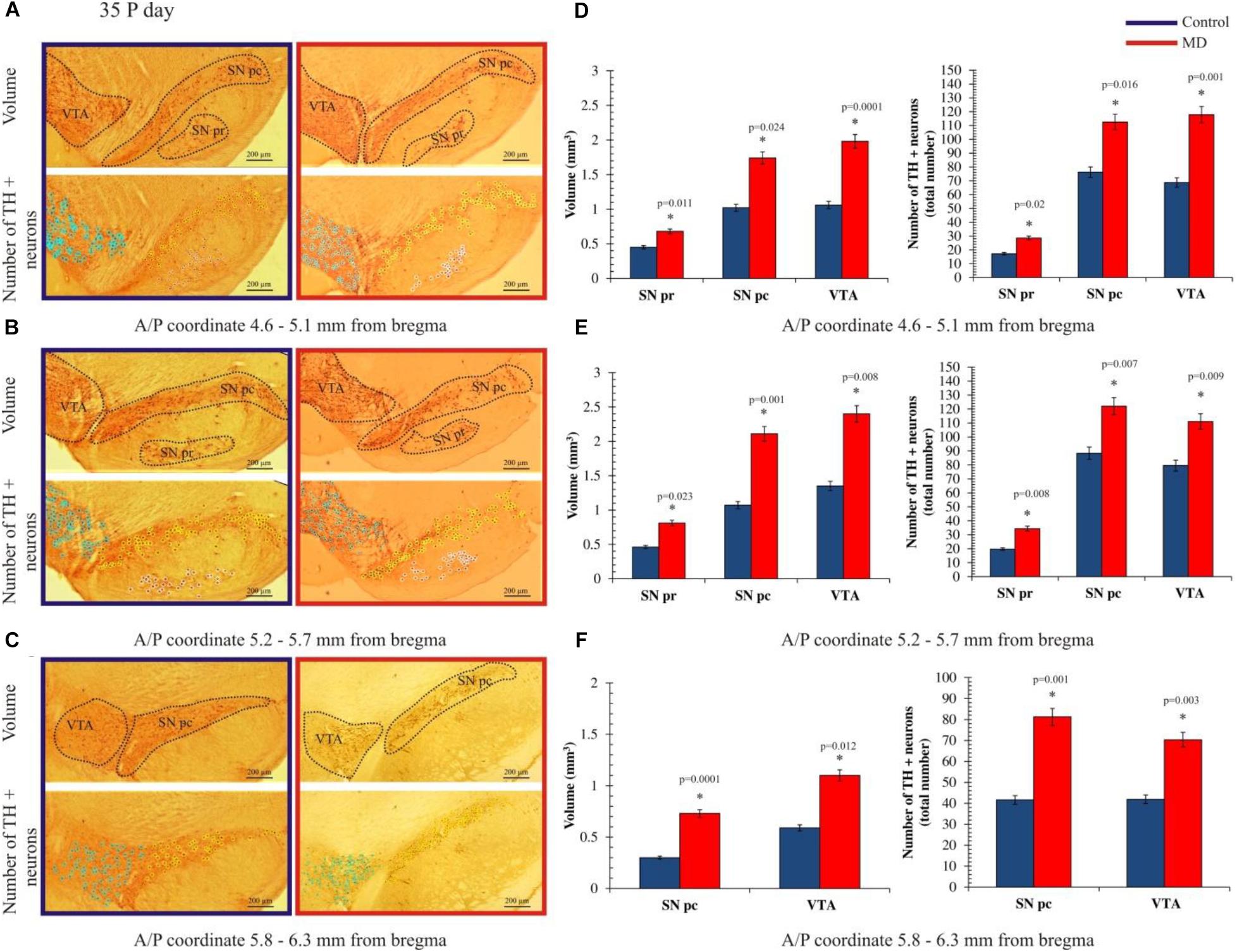
Figure 2. Effect of maternal deprivation on the volume of dopaminergic nuclei and the number of dopaminergic neurons in adolescence (postnatal day 35, P35). Representative examples of six sections are presented for (A) 4.6–5.1 mm from bregma; (B) 5.2–5.7 mm from bregma; and (C) 5.8–6.3 mm from bregma. The upper pictures depict surface areas, and these are marked with dashed lines encircling the dopaminergic structures, i.e., ventral tegmental area (VTA), substantia nigra pars compacta (SNpc), and substantia nigra pars reticulate (SNpr). The lower pictures depict the quantification of the number of tyrosine hydroxylase-positive (TH +) neurons, where TH + neurons belonging to the VTA, SNpc, and SNpr are shown are purple, yellow, and white circles, respectively. Bar graphs depict the comparisons between the maternally deprived and control rats for (D) 4.6–5.1 mm from bregma; (E) 5.2–5.7 mm from bregma; and (F) 5.8–6.3 mm from bregma. Volumes of the dopaminergic nuclei are presented on the left, while the total numbers of the TH + neurons per nucleus are represented on the right. The results are presented as the mean values ± SEM. Asterisk indicates significant between-group differences of the mean values (Student’s t-test for two independent groups, p < 0.05). ∗Increases in the volume of the dopaminergic nuclei and the number of TH + neurons in the maternally deprived (MD) group were present in all analyzed sections. Anterior/posterior (A/P) 4.60–5.10 mm caudally from bregma, there were 1.5- (p < 0.05), 1.7- (p < 0.05), and 1.9-fold (p < 0.001) increases in the volume of the dopaminergic nuclei and the 1.6- (p < 0.05), 1.5- (p < 0.05), and 1.7-fold (p < 0.05) increases in the number of TH + neurons in the SNpr, SNpc, and VTA. A/P 5.20–5.70 mm caudally from bregma, there were 1.8- (p < 0.05), 2.0- (p < 0.05), and 1.8-fold (p < 0.05) increases in the volume of the dopaminergic nuclei and 1.7- (p < 0.05), 1.4- (p < 0.05), and 1.4-fold (p < 0.05) increases in the number of TH + neurons in the SNpr, SNpc and VTA. A/P 5.80–6.30 mm caudally from bregma, there were 2.4- (p < 0.001) and 1.9-fold (p < 0.05) increases in the volume of the dopaminergic nuclei and 1.9- (p < 0.05) and 1.7-fold (p < 0.05) increases in the number of TH + neurons in the SNpr, SNpc, and VTA.
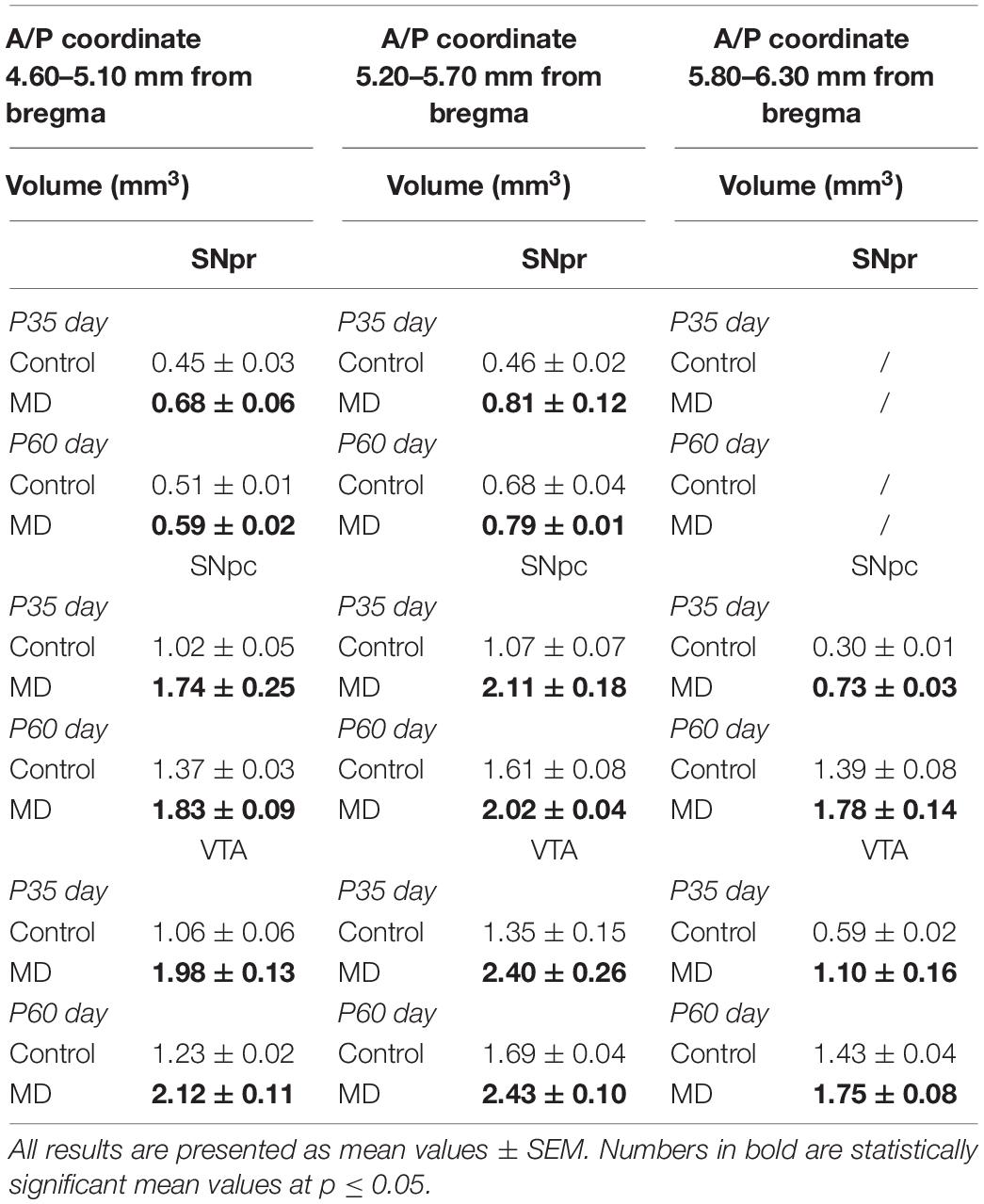
Table 1. Volumes of the substantia nigra pars reticulata (SNpr), substantia nigra pars compacta (SNpc), and ventral tegmental area (VTA) in adolescence (postnatal day 35, P35) and adulthood (postnatal day 60, P60).
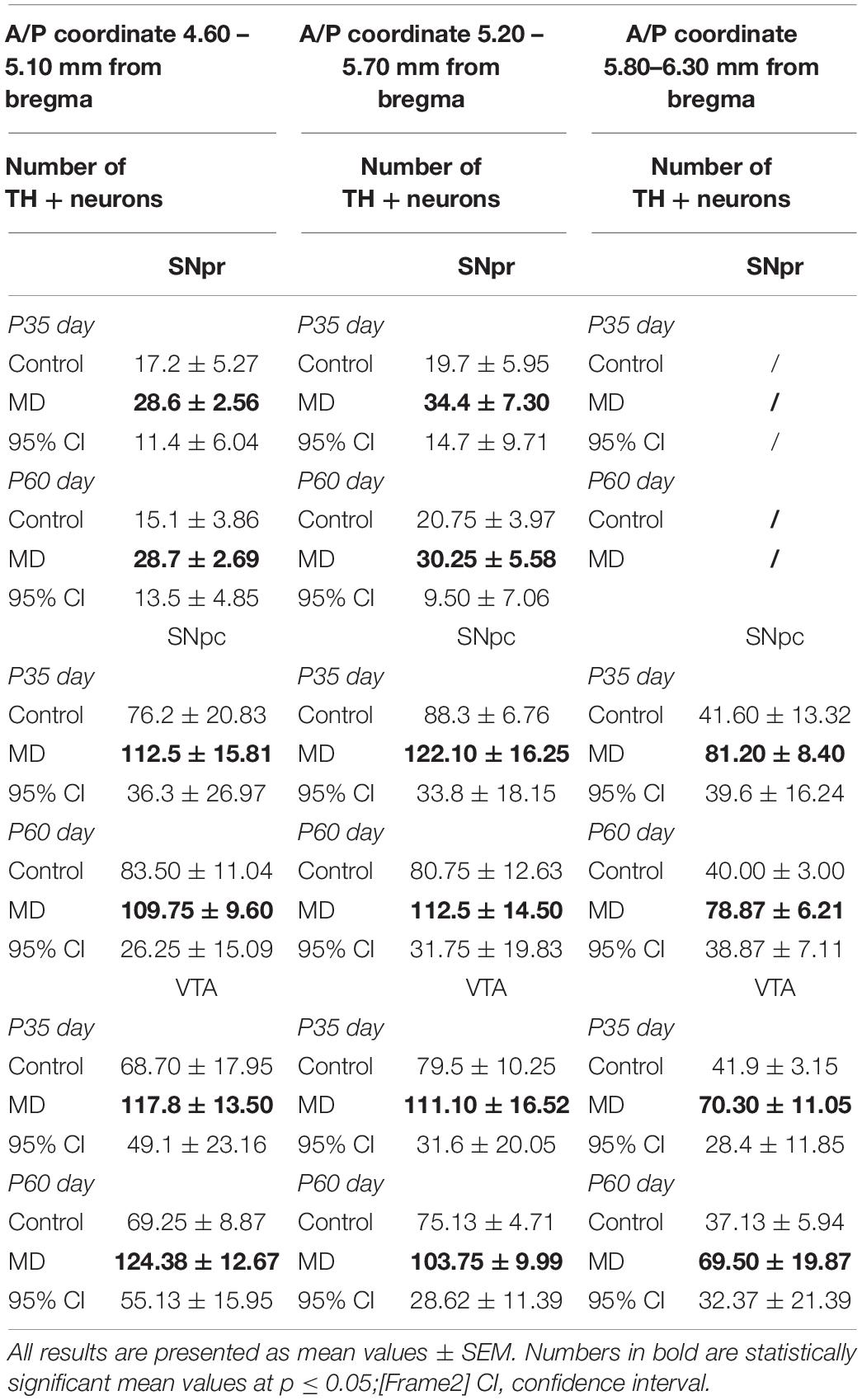
Table 2. Number of TH + neurons in the substantia nigra pars reticulata (SNpr), substantia nigra pars compacta (SNpc), and ventral tegmental area (VTA) in adolescence (postnatal day 35, P35) and adulthood (postnatal day 60, P60).
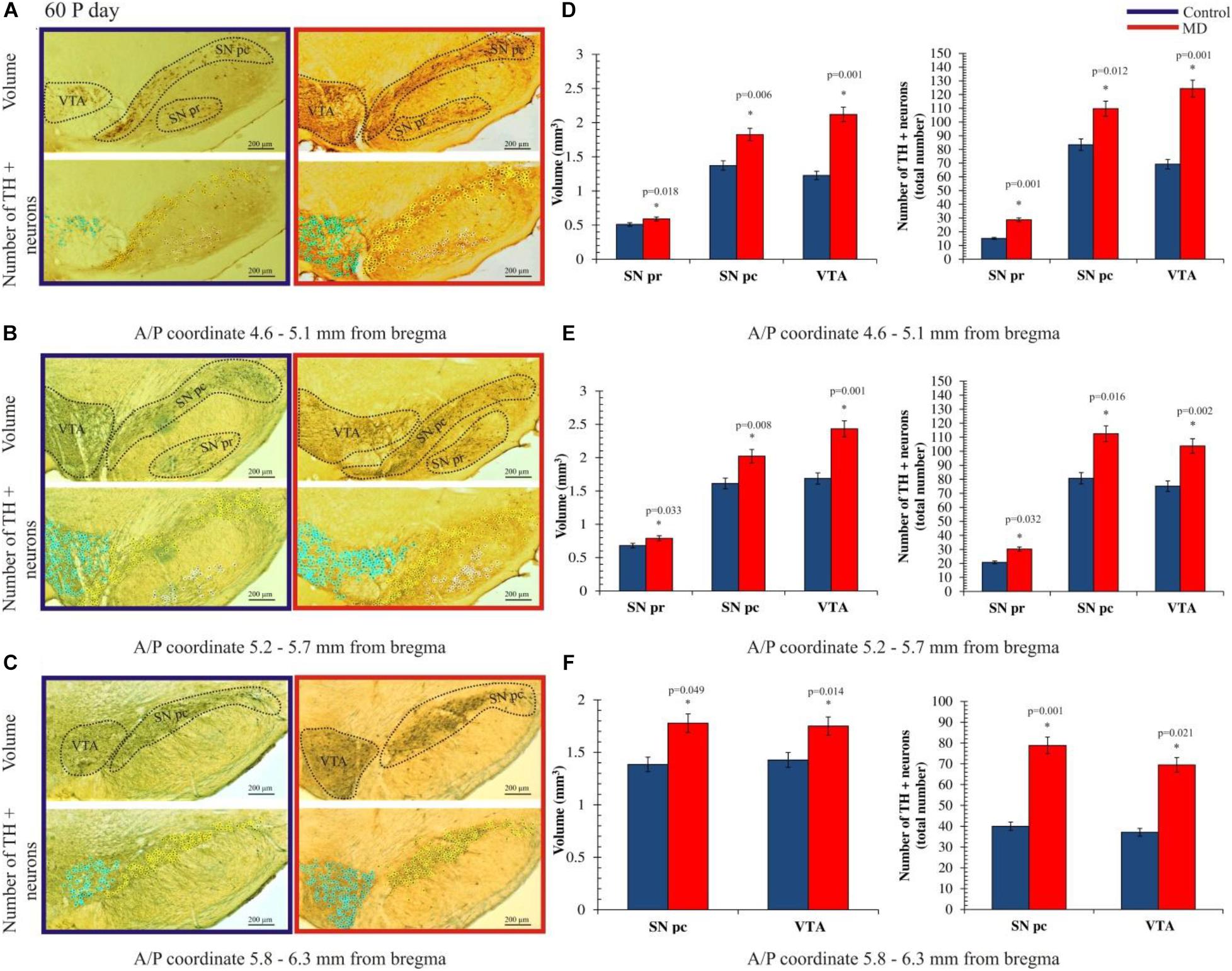
Figure 3. Effect of maternal deprivation on the volume of dopaminergic nuclei and the number of dopaminergic neurons in adulthood (postnatal day 60, P60). Representative examples of six sections are presented for (A) 4.6–5.1 mm from bregma; (B) 5.2–5.7 mm from bregma; and (C) 5.8–6.3 mm from bregma. The upper pictures depict surface areas, and these are marked with dashed lines encircling the dopaminergic structures i.e., ventral tegmental area (VTA), substantia nigra pars compacta (SNpc), and substantia nigra pars reticulate (SNpr). The lower pictures depict the quantification of the number of tyrosine hydroxylase-positive (TH +) neurons, where TH + neurons belonging to the VTA, SNpc, and SNpr are shown as purple, yellow, and white circles, respectively. Bar graphs depict the comparisons between the maternally deprived and control rats for (D) 4.6–5.1 mm from bregma; (E) 5.2–5.7 mm from bregma; and (F) 5.8–6.3 mm from bregma. Volumes of the dopaminergic nuclei are presented on the left, while the total numbers of the TH + neurons per nucleus are represented on the right. The results are presented as the mean values ± SEM. Asterisk indicates significant between-group differences of the mean values (Student’s t-test for two independent groups, p < 0.05). ∗Increases of the volume of the dopaminergic nuclei and the number of TH + neurons in the maternally deprived (MD) group were present in all analyzed sections. Anterior/posterior (A/P) 4.60–5.10 mm caudally from bregma, the increases in the volume of the dopaminergic nuclei were 1.2- (p < 0.05), 1.3- (p < 0.05), and 1.7-fold (p < 0.05), respectively, and those in the number of TH + neurons in the SNpr, SNpc, and VTA were 1.9- (p < 0.05), 1.3- (p < 0.05), and 1.8-fold (p < 0.05), respectively. A/P 5.20–5.70 mm caudally from bregma, increases in the volume of the dopaminergic nuclei were 1.2- (p < 0.05), 1.3- (p < 0.05), and 1.4-fold (p < 0.05), respectively, and those in the number of TH + neurons in the SNpr, SNpc, and VTA were 1.4- (p < 0.05), 1.4- (p < 0.05), and 1.4-fold (p < 0.05), respectively. A/P 5.80–6.30 mm caudally from bregma, there were 1.3- (p < 0.05) and 1.2-fold (p < 0.05) increases in the volume of the dopaminergic nuclei and 1.9- (p < 0.05) and 1.9-fold (p < 0.05) increases in the number of TH + neurons in the SNpc and VTA, respectively.
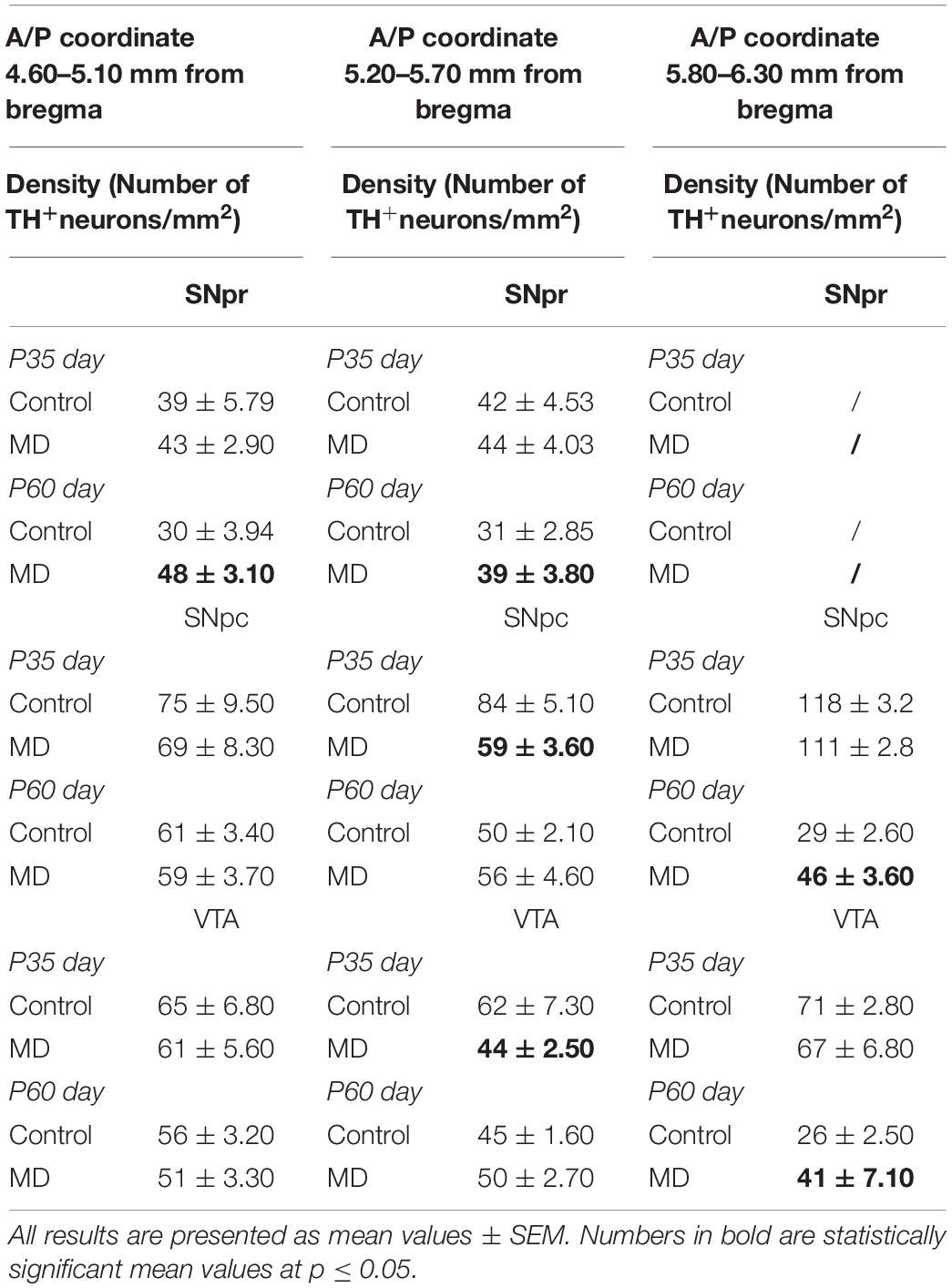
Table 3. Density (number of TH + neurons per square millimeter) of the substantia nigra pars reticulata (SNpr), substantia nigra pars compacta (SNpc), and ventral tegmental area (VTA) in adolescence (postnatal day 35, P35) and adulthood (postnatal day 60, P60).
Discussion
The present study was focused on the effects of early life stress caused by maternal deprivation on the volume of dopaminergic nuclei and the number of dopaminergic cells within these nuclei. The key findings of this report are that both adolescent and adult brains are affected by early life adversity, which is accompanied by an elevation of corticosterone levels. Also, our study supports the findings of Hancock and Grant, who showed that after maternal separation in different periods of development, food intake dropped (Hancock and Grant, 2009). In particular, maternal deprivation increases the volumes of all dopaminergic nuclei and the total number of dopaminergic neurons therein in adolescence and adulthood.
Postnatal separation of pups from their mother caused an aberrant development of the dopaminergic system, which is postulated to play a role in the pathology of psychiatric diseases (Malnic et al., 1971). By restricting mother–pup contact, which also includes food deprivation at this stage, maternal deprivation disrupted the SHRP adaptation response and led to the abnormal functioning of the hypothalamic–pituitary–adrenal (HPA) axis and enhanced stress response (Champagne et al., 2009). Previous studies have shown that perinatal glucocorticoid treatment can permanently alter the basal and stress-induced levels of circulating glucocorticoids in experimental animals, and it is documented that endogenous adrenal steroids augment the activity of dopamine in the striatum (McArthur et al., 2005). Body weight loss in rats also corresponded with elevations of hypothalamic corticotropin-releasing hormone (CRH) messenger RNA (mRNA) expression (Kawaguchi et al., 2005) and increases in circulating adrenocorticotropic hormone (ACTH) (Wong et al., 1993) and corticosterone (Burden et al., 1993). The maternal deprivation method used in this study is different from that used in the majority of other studies reported in the literature, but the obtained results are in concordance with the outcome of a study conducted by Chocyk et al. (2011). They have shown a transient increase in the number of TH + neurons in SNpr in adolescent female rats as well as an increase in the number of TH + neurons in the VTA in adult female rats, with no changes in the volume of the examined structures. Depending on the stage development of the brain, there are several possible explanations for the increased number of TH-positive neurons in SN and VTA. Due to the dependence of the perikaryal TH protein levels on axonal transport rate, it is possible that TH is not present in the cell body or is present in large quantities (Nestler, 1992). Constitutive and sustained changes in the expression of TH + neurons in the midbrain are possible because, in the SN and VTA, Nurr1 and Pitx3 transcription factor expressions occur during the pre- and postnatal periods of brain development and are responsible for the survival of dopaminergic neurons (Ojeda et al., 2003; Korotkova et al., 2005; Katunar et al., 2009, 2010). There is also a possibility that the difference is caused by the TH expression from cells which are different from typical dopaminergic cells in SN and VTA (Ugrumov, 2009). Our results have shown an increase in the density of TH + neurons on P60 in both the examined stereotaxic range of SNpr as well as SNpc and VTA in the anterior/posterior (A/P) coordinate 5.80–6.30 mm from bregma. These results are in correlation with the study conducted on female Wistar rats obtained by Chocyk et al. (Chocyk et al., 2011) which showed that MD increased the number and density of TH + neurons in the SNpr, but not in the VTA, during adolescence. The results obtained in our study are similar to the study demonstrated by McArthur et al. (McArthur et al., 2007), which (in pharmacological terms) can imitate stress effects. De Souza et al. (de Souza et al., 2018) have shown that early life stress induced during the dark phase of the luminosity cycle increased the gene expressions of the dopaminergic receptors Drda and Drd2a. This effect was observed only in male rats.
Summing up, dopamine receptor expression is strongly regulated by aversive early life experiences. Actually, these effects may have a sex-specific developmental trajectory. It is known that MD disturbs the action of the HPA axis and affects the levels of circulating glucocorticoids (GCs) (de Kloet et al., 2005). GCs, acting via glucocorticoid and mineralocorticoid receptors and are widely expressed in the brain, affect the transcription of specific genes or exert many non-genomic effects. GCs have a substantial role in the regulation of brain development and maturation. Early life stress-induced imbalance in GC levels may program brain functions, including dopamine neurotransmission, for later life (de Kloet et al., 2005).
Finally, we cannot completely exclude the possibility that an increase in the total number of TH-positive neurons occurs due to neurogenesis at a certain point during ontogenesis, even though this concept is very disputable (Shan et al., 2006; Zhao and Janson Lang, 2009).
Conclusion
This is the first study to provide evidence that early life stress caused by MD conducted on P9 in male rats leads to alterations in the morphological and biochemical parameters, including the body weight of rats, volume of the dopaminergic nuclei, number of TH + neurons in three different stereotaxic ranges through the overall rostro-caudal dimension, and the density of TH + neurons in the same ranges. The observed changes may facilitate the understanding of how early life stress may increase the tendency for neurological disorders, schizophrenia, and attention deficit hyperactivity disorder (ADHD). These disorders are known to be associated with irregular functions in dopaminergic neurotransmission, which may be mediated by aberrant maturation, which may include volumetric changes in the dopaminergic nuclei as well as alterations in the number and density of dopaminergic neurons.
Data Availability Statement
All datasets presented in this study are included in the article/supplementary material.
Ethics Statement
All experimental procedures were in compliance with EEC Directive (2010/63/EU) on the protection of animals used for experimental and other scientific purposes, and were approved by the Ethical Committee for the Use of Laboratory Animals of the School of Medicine, University of Belgrade (Approval No. 323-07-01245/2014-05/2).
Author Contributions
All authors listed have made a substantial, direct and intellectual contribution to the work, and approved it for publication.
Funding
A 2018-S4 grant was awarded to MJ by the non-governmental organization Centar za Razvoj Liderstva and company Phillip Morris doo Serbia. Phillip Morris doo Serbia sponsored the competitive grant application call in Serbia “Pokreni se za Nauku.” The grant obtained by MJ financed the procurement of the TH antibody used in this study. This study was supported by the Ministry for Science and Environmental Protection of the Republic of Serbia (Grant Nos. III41020 and ON175058).
Conflict of Interest
The authors declare that the research was conducted in the absence of any commercial or financial relationships that could be construed as a potential conflict of interest.
References
Aksic, M., Radonjic, N. V., Aleksic, D., Jevtic, G., Markovic, B., Petronijevic, N., et al. (2013). Long-term effects of the maternal deprivation on the volume and number of neurons in the rat neocortex and hippocampus. Acta Neurobiol. Exp. 73, 394–403.
Aleksic, D., Aksic, M., Radonjic, N. V., Jovanovic, A., Markovic, B., Petronijevic, N., et al. (2016). Long-term effects of maternal deprivation on the volume, number and size of neurons in the amygdala and nucleus accumbens of rats. Psychiatr. Danubina 28, 211–219.
Almeida, O. F., Conde, G. L., Crochemore, C., Demeneix, B. A., Fischer, D., Hassan, A. H., et al. (2000). Subtle shifts in the ratio between pro- and antiapoptotic molecules after activation of corticosteroid receptors decide neuronal fate. FASEB J. 14, 779–790. doi: 10.1096/fasebj.14.5.779
Arnold, S. E., and Trojanowski, J. Q. (1996). Recent advances in defining the neuropathology of schizophrenia. Acta Neuropathol. 92, 217–231. doi: 10.1007/s004010050512
Brenhouse, H. C., Lukkes, J. L., and Andersen, S. L. (2013). Early life adversity alters the developmental profiles of addiction-related prefrontal cortex circuitry. Brain Sci. 3, 143–158. doi: 10.3390/brainsci3010143
Burden, V. R., White, B. D., Dean, R. G., and Martin, R. J. (1993). Activity of the hypothalamic-pituitary-adrenal axis is elevated in rats with activity-based anorexia. J. Nutr. 123, 1217–1225. doi: 10.1093/jn/123.7.1217
Champagne, D. L., de Kloet, E. R., and Joels, M. (2009). Fundamental aspects of the impact of glucocorticoids on the (immature) brain. Semin. Fetal Neonatal Med. 14, 136–142. doi: 10.1016/j.siny.2008.11.006
Chocyk, A., Przyborowska, A., Dudys, D., Majcher, I., Mackowiak, M., and Wedzony, K. (2011). The impact of maternal separation on the number of tyrosine hydroxylase-expressing midbrain neurons during different stages of ontogenesis. Neuroscience 182, 43–61. doi: 10.1016/j.neuroscience.2011.03.008
de Kloet, E. R., Sibug, R. M., Helmerhorst, F. M., and Schmidt, M. V. (2005). Stress, genes and the mechanism of programming the brain for later life. Neuroscience Biobehav. Rev. 29, 271–281. doi: 10.1016/j.neubiorev.2004.10.008
de Souza, J. A., da Silva, M. C., de Matos, R. J. B., do Amaral Almeida, L. C., Beltrao, L. C., de Souza, F. L., et al. (2018). Pre-weaning maternal separation increases eating later in life in male and female offspring, but increases brainstem dopamine receptor 1a and 2a only in males. Appetite 123, 114–119. doi: 10.1016/j.appet.2017.12.004
Ellenbroek, B. A., and Cools, A. R. (2000). The long-term effects of maternal deprivation depend on the genetic background. Neuropsychopharmacology 23, 99–106. doi: 10.1016/s0893-133x(00)00088-9
Ellenbroek, B. A., and Cools, A. R. (2002). Early maternal deprivation and prepulse inhibition: the role of the postdeprivation environment. Pharmacol. Biochem. Behav. 73, 177–184. doi: 10.1016/s0091-3057(02)00794-3
Ellenbroek, B. A., van den Kroonenberg, P. T., and Cools, A. R. (1998). The effects of an early stressful life event on sensorimotor gating in adult rats. Schizophrenia Res. 30, 251–260. doi: 10.1016/s0920-9964(97)00149-7
Hancock, S., and Grant, V. (2009). Early maternal separation increases symptoms of activity-based anorexia in male and female rats. J. Exp. Psychol. Anim. Behav. Process. 35, 394–406. doi: 10.1037/a0014736
Huppertz-Kessler, C. J., Poeschl, J., Hertel, R., Unsicker, K., and Schenkel, J. (2012). Effects of a new postnatal stress model on monoaminergic neurotransmitters in rat brains. Brain Dev. 34, 274–279. doi: 10.1016/j.braindev.2011.07.008
Husum, H., and Mathe, A. A. (2002). Early life stress changes concentrations of neuropeptide Y and corticotropin-releasing hormone in adult rat brain. Lithium treatment modifies these changes. Neuropsychopharmacology 27, 756–764. doi: 10.1016/s0893-133x(02)00363-9
Jahng, J. W., Ryu, V., Yoo, S. B., Noh, S. J., Kim, J. Y., and Lee, J. H. (2010). Mesolimbic dopaminergic activity responding to acute stress is blunted in adolescent rats that experienced neonatal maternal separation. Neuroscience 171, 144–152. doi: 10.1016/j.neuroscience.2010.08.063
Katunar, M. R., Saez, T., Brusco, A., and Antonelli, M. C. (2009). Immunocytochemical expression of dopamine-related transcription factors Pitx3 and Nurr1 in prenatally stressed adult rats. J. Neurosci. Res. 87, 1014–1022. doi: 10.1002/jnr.21911
Katunar, M. R., Saez, T., Brusco, A., and Antonelli, M. C. (2010). Ontogenetic expression of dopamine-related transcription factors and tyrosine hydroxylase in prenatally stressed rats. Neurotoxicity Res. 18, 69–81. doi: 10.1007/s12640-009-9132-z
Kawaguchi, M., Scott, K. A., Moran, T. H., and Bi, S. (2005). Dorsomedial hypothalamic corticotropin-releasing factor mediation of exercise-induced anorexia. Am. J. Physiol. Regul. Integr. Comp. Physiol. 288, R1800–R1805.
Korotkova, T. M., Ponomarenko, A. A., Haas, H. L., and Sergeeva, O. A. (2005). Differential expression of the homeobox gene Pitx3 in midbrain dopaminergic neurons. Eur. J. Neurosci. 22, 1287–1293. doi: 10.1111/j.1460-9568.2005.04327.x
Li, M., Xue, X., Shao, S., Shao, F., and Wang, W. (2013). Cognitive, emotional and neurochemical effects of repeated maternal separation in adolescent rats. Brain Res. 1518, 82–90. doi: 10.1016/j.brainres.2013.04.026
Llorente, R., Villa, P., Marco, E. M., and Viveros, M. P. (2012). Analyzing the effects of a single episode of neonatal maternal deprivation on metabolite profiles in rat brain: a proton nuclear magnetic resonance spectroscopy study. Neuroscience 201, 12–19. doi: 10.1016/j.neuroscience.2011.11.033
Malnic, G., De Mello Aires, M., and Giebisch, G. (1971). Potassium transport across renal distal tubules during acid-base disturbances. Am. J. Physiol. 221, 1192–1208. doi: 10.1152/ajplegacy.1971.221.4.1192
McArthur, S., McHale, E., Dalley, J. W., Buckingham, J. C., and Gillies, G. E. (2005). Altered mesencephalic dopaminergic populations in adulthood as a consequence of brief perinatal glucocorticoid exposure. J. Neuroendocrinol. 17, 475–482. doi: 10.1111/j.1365-2826.2005.01331.x
McArthur, S., McHale, E., and Gillies, G. E. (2007). The size and distribution of midbrain dopaminergic populations are permanently altered by perinatal glucocorticoid exposure in a sex- region- and time-specific manner. Neuropsychopharmacology 32, 1462–1476. doi: 10.1038/sj.npp.1301277
Murray, R. M., O’Callaghan, E., Castle, D. J., and Lewis, S. W. (1992). A neurodevelopmental approach to the classification of schizophrenia. Schizophrenia Bull. 18, 319–332. doi: 10.1093/schbul/18.2.319
Ojeda, V., Fuentealba, J. A., Galleguillos, D., and Andres, M. E. (2003). Rapid increase of Nurr1 expression in the substantia nigra after 6-hydroxydopamine lesion in the striatum of the rat. J. Neurosci. Res. 73, 686–697. doi: 10.1002/jnr.10705
Ploj, K., Roman, E., and Nylander, I. (2003). Long-term effects of maternal separation on ethanol intake and brain opioid and dopamine receptors in male Wistar rats. Neuroscience 121, 787–799. doi: 10.1016/s0306-4522(03)00499-8
Roceri, M., Hendriks, W., Racagni, G., Ellenbroek, B. A., and Riva, M. A. (2002). Early maternal deprivation reduces the expression of BDNF and NMDA receptor subunits in rat hippocampus. Mol. Psychiatry 7, 609–616. doi: 10.1038/sj.mp.4001036
Shan, X., Chi, L., Bishop, M., Luo, C., Lien, L., Zhang, Z., et al. (2006). Enhanced de novo neurogenesis and dopaminergic neurogenesis in the substantia nigra of 1-methyl-4-phenyl-1,2,3,6-tetrahydropyridine-induced Parkinson’s disease-like mice. Stem Cells 24, 1280–1287. doi: 10.1634/stemcells.2005-0487
Suri, D., Teixeira, C. M., Cagliostro, M. K., Mahadevia, D., and Ansorge, M. S. (2015). Monoamine-sensitive developmental periods impacting adult emotional and cognitive behaviors. Neuropsychopharmacology 40, 88–112. doi: 10.1038/npp.2014.231
Ugrumov, M. V. (2009). Non-dopaminergic neurons partly expressing dopaminergic phenotype: distribution in the brain, development and functional significance. J. Chem. Neuroanat. 38, 241–256. doi: 10.1016/j.jchemneu.2009.08.004
Waddington, J. L., Scully, P. J., and Youssef, H. A. (1997). Developmental trajectory and disease progression in schizophrenia: the conundrum, and insights from a 12-year prospective study in the Monaghan 101. Schizophrenia Res. 23, 107–118. doi: 10.1016/s0920-9964(96)00111-9
Wong, M. L., Licinio, J., Gold, P. W., and Glowa, J. (1993). Activity-induced anorexia in rats does not affect hypothalamic neuropeptide gene expression chronically. Int. J. Eating Disord. 13, 399–405. doi: 10.1002/1098-108x(199305)13:4<399::aid-eat2260130408>3.0.co;2-j
Keywords: early life stress, dopamine, substantia nigra, ventral tegmental area, glucocorticoids
Citation: Kapor S, Aksić M, Puškaš L, Jukić M, Poleksić J, Milosavljević F, Bjelica S and Filipovic B (2020) Long-Term Effects of Maternal Deprivation on the Volume of Dopaminergic Nuclei and Number of Dopaminergic Neurons in Substantia Nigra and Ventral Tegmental Area in Rats. Front. Neuroanat. 14:578900. doi: 10.3389/fnana.2020.578900
Received: 01 July 2020; Accepted: 15 September 2020;
Published: 23 October 2020.
Edited by:
Marcello Rosa, Monash University, AustraliaReviewed by:
Nafiseh Atapour, Monash University, AustraliaMasoumeh Nozari, Kerman Medical University, Iran
Jennifer Kaufling, Université de Strasbourg, France
Copyright © 2020 Kapor, Aksić, Puškaš, Jukić, Poleksić, Milosavljević, Bjelica and Filipović. This is an open-access article distributed under the terms of the Creative Commons Attribution License (CC BY). The use, distribution or reproduction in other forums is permitted, provided the original author(s) and the copyright owner(s) are credited and that the original publication in this journal is cited, in accordance with accepted academic practice. No use, distribution or reproduction is permitted which does not comply with these terms.
*Correspondence: Slobodan Kapor, a2Fwb3JiZ0BnbWFpbC5jb20=