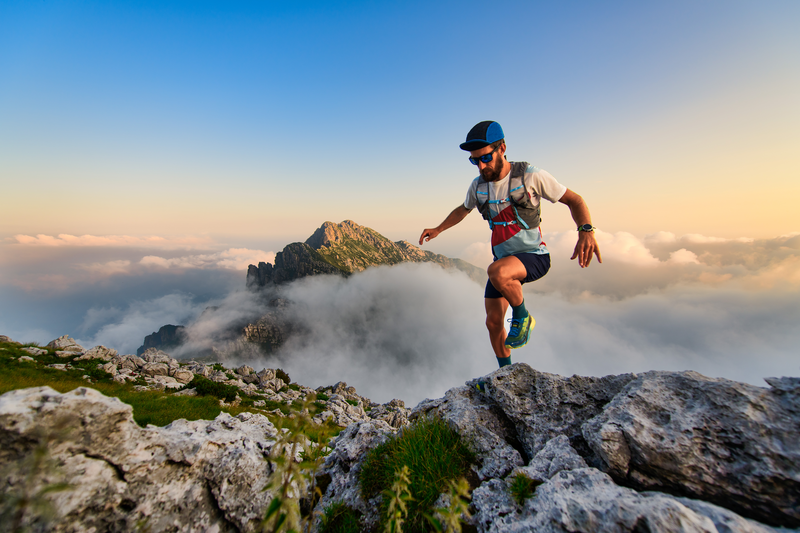
94% of researchers rate our articles as excellent or good
Learn more about the work of our research integrity team to safeguard the quality of each article we publish.
Find out more
ORIGINAL RESEARCH article
Front. Neuroanat. , 08 December 2016
Volume 10 - 2016 | https://doi.org/10.3389/fnana.2016.00118
In vertebrates, γ-aminobutyric acid (GABA) is the main inhibitory transmitter in the central nervous system (CNS) acting through ionotropic (GABAA) and metabotropic (GABAB) receptors. The GABAB receptor produces a slow inhibition since it activates second messenger systems through the binding and activation of guanine nucleotide-binding proteins [G-protein-coupled receptors (GPCRs)]. Lampreys are a key reference to understand molecular evolution in vertebrates. The importance of the GABAB receptor for the modulation of the circuits controlling locomotion and other behaviors has been shown in pharmacological/physiological studies in lampreys. However, there is no data about the sequence of the GABAB subunits or their expression in the CNS of lampreys. Our aim was to identify the sea lamprey GABAB1 and GABAB2 transcripts and study their expression in the CNS of adults. We cloned two partial sequences corresponding to the GABAB1 and GABAB2 cDNAs of the sea lamprey as confirmed by sequence analysis and comparison with known GABAB sequences of other vertebrates. In phylogenetic analyses, the sea lamprey GABAB sequences clustered together with GABABs sequences of vertebrates and emerged as an outgroup to all gnathostome sequences. We observed a broad and overlapping expression of both transcripts in the entire CNS. Expression was mainly observed in neuronal somas of the periventricular regions including the identified reticulospinal cells. No expression was observed in identifiable fibers. Comparison of our results with those reported in other vertebrates indicates that a broad and overlapping expression of the GABAB subunits in the CNS is a conserved character shared by agnathans and gnathostomes.
γ-aminobutyric acid (GABA) is the main inhibitory transmitter in the central nervous system (CNS), and it acts via ionotropic (GABAA) and metabotropic (GABAB) GABA receptors (Pinard et al., 2010; Chalifoux and Carter, 2011). Unlike GABAA receptors that form ion channels, GABAB receptors address second messenger systems through the binding and activation of guanine nucleotide-binding proteins [G-protein-coupled receptors (GPCRs)], producing a slower and more prolonged inhibition than ionotropic GABAA receptors, which results in reduced neuronal excitability (Blein et al., 2000; Bettler et al., 2004; Pinard et al., 2010). GABAB receptors have been identified on both pre- and postsynaptic terminals (Curtis et al., 1968, 1977; Alford and Grillner, 1991). Presynaptic GABAB receptors can exist as either auto-(those that control GABA release) or heteroreceptors (those activated by other neurons) to modulate neurotransmitter release (Bettler et al., 2004). Depending on whether GABAB receptors are activated on excitatory or inhibitory terminals, their effects on the postsynaptic neuron are inhibitory or dis-inhibitory, respectively. Currently, it is accepted that functional GABAB receptors are obligate heterodimers (Kammerer et al., 1999; Kuner et al., 1999; Blein et al., 2000; Villemure et al., 2005; Geng et al., 2013) composed of two receptor subunits, GABAB1 (Kaupmann et al., 1997; Padgett and Slesinger, 2010) and GABAB2 (Kaupmann et al., 1998; White et al., 1998; Kuner et al., 1999). The GABAB1 receptor subunit is responsible for agonist binding (Galvez et al., 2001), whereas the GABAB2 receptor subunit is essential for trafficking of the heterodimer to the cell surface and for signal transduction following agonist activation (Calver et al., 2002) through the activation of G-proteins (Villemure et al., 2005). Interestingly, a study of Maurel et al. (2008) indicates a possible formation of GABAB oligomers, which show decreased G-protein coupling efficiency. Thus, formation of GABAB oligomers may regulate receptor efficacy, making their formation potentially critical to cellular function.
The presence of GABAB receptors in a single-cell species of paramecium has been demonstrated by immunohistochemical methods (Ramoino et al., 2006), which indicates that the GABAB receptor appeared early during evolution. Only a few studies have shown the presence and/or expression of GABAB receptors in invertebrate species. An optogenetic study revealed GABAB receptor expression in motor neurons of Caenorhabditis elegans (Schultheis et al., 2011). Ramoino et al. (2007) demonstrated a GABAergic-like system as well as the expression of GABAB1 and GABAB2 subunits in a marine demosponge, Chondrilla nucula. Expression of GABAB receptors has been reported in olfactory sensory neurons of moths (Pregitzer et al., 2013) and in the entire CNS of cockroaches (Blankenburg et al., 2015), spiders (Panek et al., 2003) and Drosophila melanogaster (Mezler et al., 2001). The expression of GABAB transcripts (GABAB1 and GABAB2) has been also reported in a few jawed vertebrate species (rats: Bischoff et al., 1999; Fritschy et al., 1999; humans: Calver et al., 2000; Berthele et al., 2001; non-human primates: Muñoz et al., 1998, 2001; zebrafish: Tabor et al., 2008; and frogs: Kaeser et al., 2011). These studies reveal a wide distribution of this receptor in the entire CNS of invertebrate and vertebrate species. So far, the expression of the GABAB subunits has not been reported in any jawless vertebrate. It has been suggested that more studies providing a detailed distribution of the GABAB1 and GABAB2 subunits are necessary to understand the roles that these receptors have in CNS neurotransmission (Charles et al., 2001).
The sea lamprey, Petromyzon marinus L., belongs to an ancient lineage of vertebrates, the Agnathans or jawless vertebrates, which occupy a key phylogenetic position at the transition between urochordates and jawed vertebrates. Lampreys are important model vertebrates for studies of nervous system development and evolution, as its genome is a key reference to understand molecular evolution in vertebrates (Smith et al., 2013). Sea lampreys have a complex and long life cycle, which includes a metamorphosis that transforms blind filter-feeder larvae into young adults (post-metamorphic sea lampreys). These descend to the sea to feed as parasites of teleost fishes and then, after 1–2 years, mature adult sea lampreys return to rivers to breed and die (Hardisty and Potter, 1971).
In addition, lampreys have been extensively used to identify the cellular mechanisms involved in the generation and control of vertebrate locomotion (Dubuc et al., 2008; Grillner et al., 2008). Many pharmacological and physiological studies have shown the importance of GABA in the modulation of central pattern generators (CPGs) and sensory inputs in the spinal cord (Tegnér et al., 1993; Parker et al., 1998; Schmitt et al., 2004), and brain circuits that control locomotion, including the paleostriatal pathway and the striatal neurons projecting to the motor centers (Grillner et al., 2008); as well as the specific role of GABAB receptors in these processes (Alford and Grillner, 1991). Lampreys have become an interesting model to understand successful regeneration following spinal cord injury (reviewed in Barreiro-Iglesias, 2012, 2015; Rodicio and Barreiro-Iglesias, 2012). A recent study of our group demonstrated that in lampreys, as in mammals, there is a massive release of glutamate and GABA after a complete spinal injury (Fernández-López et al., 2014). GABA accumulated in the form of halos around some of the descending axons after the injury and statistical analyses showed a correlation between the presence of this halos and a higher survival ability of the identified descending neurons (Fernández-López et al., 2014). This revealed a possible neuroprotective role of GABA following spinal cord injury in lampreys. This type of studies show the importance of increasing our knowledge on the GABAergic system of lampreys.
In lampreys, the distribution of GABA immunoreactive neurons and fibers in the CNS of adults (Meléndez-Ferro et al., 2000; Robertson et al., 2007), their ontogeny (Meléndez-Ferro et al., 2002, 2003; Ruiz et al., 2004), the origin of the descending GABAergic projections to the spinal cord (Valle-Maroto et al., 2011) and the co-localization of GABA with others classic neurotransmitters in the same neuron (Villar-Cerviño et al., 2008, 2010, 2013, 2014; Barreiro-Iglesias et al., 2009a,b) have been widely studied. However, and despite the wide knowledge on the GABAergic system of lampreys, the cloning and molecular characterization of the GABAB receptors subunits of lampreys as well as their pattern of expression in the CNS have not been reported so far.
In the present study, we report the identification and characterization, by means of phylogenetic and sequence analyses, of sea lamprey sequences corresponding to GABAB1 and GABAB2 cDNAs. We also report the pattern of expression of these transcripts in the brain and spinal cord of young (post-metamorphic) and mature (upstream migrating) adults of the sea lamprey. Moreover, we compare the expression pattern of the two GABAB transcripts in the sea lamprey with those of other species, providing an anatomical and genetic basis to further understand the roles that this pre- and post-synaptic receptor may have in mediating inhibitory neurotransmission in the CNS.
Young (n = 4) and mature adults (n = 4) of the sea lamprey, Petromyzon marinus L., were used for the in situ hybridization experiments. Ten larvae were used for RNA extraction. Young adults and larvae were collected from the River Ulla (Galicia, Northwest Spain) and mature upstream migrating adults were obtained from a local commercial supplier. All experiments were approved by the Bioethics Committee of the University of Santiago de Compostela and the Consellería do Medio Rural e do Mar of the Xunta de Galicia (JLPV/IId) and conformed to the European Union and Spanish regulations for the care and handling of animals in research.
Larvae were anesthetized by immersion in 0.1% ethyl 3-aminobenzoate methanesulfonate salt (MS-222; Sigma, St. Louis, MO, USA) and the brain and spinal cord were dissected out under sterile conditions. Total RNA was isolated from these tissues using the TriPure reagent (Roche, Mannhein, Germany). The first-strand cDNA synthesis reaction from total RNA was catalyzed with Superscript III reverse transcriptase (Invitrogen, Waltham, MA, USA) using random primers (hexamers; Invitrogen). For polymerase chain reaction (PCR) cloning, specific oligonucleotide primers, 5′-TGGCACTGGCCCTGAACAAG-3′ forward and 5′- GTTGAGGTTGGGCTGCGAGT-3′ reverse; and 5′- GACAAATCTTGCTCGACGCC-3′ forward and 5′- AAACGTTGCTGAGGACACCA-3′ reverse, were designed based on the sea lamprey GABAB1 and GABAB2 sequences, respectively, annotated in the sea lamprey genome and deposited in the Ensembl database (Smith et al., 20131). The amplified fragments were cloned into pGEM-T vectors (Promega, Madison, WI, USA) and sequenced by GATC Biotech (Cologne, Germany) using Sanger sequencing. Sequence analysis and comparison was done through Basic Local Alignment Search Tool (BLAST) on NCBI and in the SMART website (Schultz et al., 1998; Letunic et al., 20152).
For this analysis, GABAB1 (protein GenBank ID: Canis lupus familiaris: XP_005640226.1; Drosophila melanogaster: AAK13420.1; Danio rerio: CAP09588.1; Bos taurus: AAI46242.1; Homo sapiens: AAH50532.2; Mus musculus: AAH54735.1; Rattus norvegicus: CAE84069.1; Columba livia: XP_013227121.1; Xenopus laevis: ADQ43745.1; Pan troglodytes: XP_009449053.1) and GABAB2 (protein GenBank ID: Canis lupus familiaris: XP_538749.2; Danio rerio: NP_001137515.1; Drosophila melanogaster: AAK13421.1; Columba livia: XP_013227167.1; Homo sapiens: AAH35071.2; Mus musculus: NP_001074610.1; Rattus norvegicus: EDL98850.1; Xenopus laevis: ADQ43746.1; Pan troglodytes: XP_009455264.1; Bos taurus: XP_002689780.1) sequences of representative species were aligned with the sea lamprey GABAB1 and GABAB2 partial deduced protein sequences deposited in the Ensembl database. All sequences were aligned using CLUSTALW and a phylogenetic tree was constructed using the neighbor-joining method with Poisson corrected distances on amino acids and bootstrap analysis (1000 replications) using MEGA 6 software (Tamura et al., 2013). An alternative phylogenetic tree was constructed with the minimum-evolution method with Poisson corrected distances on amino acids and bootstrap analysis (1000 replications) using also the MEGA 6 software.
Templates for in vitro transcription were prepared by PCR amplification as follows. Two 459 base pairs (bp) and 446 bp fragments corresponding to GABAB1 and GABAB2 sequences, respectively, were obtained using the primers mentioned above. In this case, the reverse primers include the sequence of the universal T7 promoter (TAAGCTTTAATACGACTCACTATAGGGAGA). For the generation of sense probes, the sequence of the T7 promoter was included in the forward primers. The identity of the amplified fragments was confirmed by direct sequencing. Digoxigenin (DIG)-labeled riboprobes were synthesized using the amplified fragments as templates and following standard protocols using T7 polymerase (Roche Diagnostics, Germany).
In situ hybridization experiments were performed as previously described for riboprobes against the serotonin 1a receptor (5-ht1a; Cornide-Petronio et al., 2013). Briefly, the brain/rostral spinal cord of young and mature adults were fixed by immersion for 12 h in 4% paraformaldehyde. Then, they were cryoprotected with sucrose 30% and sectioned in a cryostat in the transverse plane. Two parallel series of sections were obtained. The sections of each series were incubated with the GABAB1 or GABAB2 DIG-labeled probes, respectively, at 70°C overnight in hybridization mix and treated with RNAse A (Invitrogen) in the posthybridization washes. Then, the sections were incubated with a sheep anti-DIG antibody conjugated to alkaline phosphatase (1:2000; Roche) overnight. Staining was conducted in BM Purple (Roche) at 37°C until the signal was clearly visible. Finally, the sections were mounted in Mowiol® (Calbiochem; Temecula, CA, USA) and photographed with an Olympus photomicroscope (AX-70; Provis) equipped with a color digital camera (Olympus DP70; Tokyo, Japan). Images were slightly adjusted for brightness and contrast with Adobe Photoshop CS4 to compose the plates. No staining was observed when sense probes were used.
The cloned GABAB1 and GABAB2 cDNAs sequences corresponded to the nucleotides 302–760 for GABAB1 (Figure 1A), and 729–1162 for GABAB2 (Figure 1A′) of the partial cDNA sequences of these receptors annotated in sea lamprey genome of the Ensembl database. The cloned sequences had a 100% similarity with the putative GABAB1 and GABAB2 sequences of the Ensemble database, respectively. The cloned sequences were deposited in the GenBank database (GABAB1: KX655780; GABAB2: KX655781). Due to a high level of similarity between the cloned sequences and those annotated in the Ensembl database, we used the partial, but longer, GABAB1 and GABAB2 protein sequences (Figures 1B,B′, respectively) deduced from the sea lamprey genome sequences ENSPMAG00000006844 (corresponds to GABAB1), which is located in the scaffold GL479777, and ENSPMAG00000004383 (corresponds to GABAB2), which is located in the scaffold GL478877, for the subsequent analyses. Both partial deduced protein sequences contained the “ligand-binding domain of GABAB receptors” and the “seven transmembrane sweet-taste receptor of 3 GCPR” as revealed by the SMART online tool (Figures 1C,C′).
Figure 1. Partial cDNA sequences of the GABAB1 (A) and GABAB2 (A′) subunits from the Ensembl database, with the cloned sequences marked in red. Sequences of the deduced GABAB1 (B) and GABAB2 (B′) proteins with the sequence corresponding to the cloned cDNA sequences highlighted in red. Analyses of the predicted GABAB1 (C) and GABAB2 (C′) proteins in SMART and Basic Local Alignment Search Tool (BLAST).
Percentages of similarity between the sea lamprey GABAB1 and GABAB2 partial deduced protein sequences and those of other species are shown in Table 1. We observed a percentage of similarity of 68% to 73% and 66% to 69% between the sea lamprey partial GABAB1 and GABAB2 deduced protein sequences, respectively, and the corresponding deduced protein sequences of other vertebrate species. As expected, the percentage of similarity of the partial sea lamprey GABAB1 and GABAB2 deduced amino acids sequences with those of the protostome invertebrate D. melanogaster is low (less than 50%). The similarity between the sea lamprey partial GABAB1 and GABAB2 deduced protein sequences is only 33%.
Table 1. Percentage of homology between the partial amino acid sequences of the sea lamprey GABAB subunits and the amino acid sequences of GABAB subunits of different vertebrate and invertebrate species.
The GABAB1 and GABAB2 deduced protein sequences annotated in the Ensembl database were aligned with GABAB1 and GABAB2 deduced protein sequences of representative vertebrate and invertebrate species and this alignment was used to construct a phylogenetic tree using the neighbor-joining and the minimum-evolution methods. All vertebrate GABAB1 and GABAB2 sequences, including the sea lamprey sequences, were clustered together in their respective groups in the resulting trees and the sea lamprey sequences were found to emerge as an outgroup to the corresponding gnathostome sequences (Figures 2A,B).
Figure 2. Phylogenetic trees of GABAB protein sequences of different species according the neighbor-joining (A) and the minimum-evolution methods (B). The bootstraps are indicated on a scale of 100 based on 1000 replications. Scale indicates 0.1 amino acids substitutions per locus.
We observed a widespread expression of the GABAB1 and GABAB2 transcripts in neuronal populations in the brain of the adult sea lamprey. No clear and obvious differences were observed in the expression of these transcripts between young and mature adult sea lampreys. In situ hybridization signal appeared as a dotted labeling in the tissue sections.
No clear differences were noted in the expression of the transcripts of the two GABAB subunits (Figure 3). Accordingly, only 1 set of schematic drawings of transverse sections taken from a young post-metamorphic adult showing the expression pattern of both transcripts is presented in Figure 4. Photomicrographs of representative transverse sections of the brain are shown in Figures 3, 5, 6. These photomicrographs were taken from two young postmetamorphic and three upstreaming migrating adults.
Figure 3. Photomicrographs from the sea lamprey brain showing the expression of both GABAB subunits in the same brain regions. (A–D) corresponds to GABAB1 expression and (A′–D′) corresponds to GABAB2 expression. The arrows indicate some small positive dots. Please note in (D,D′) that the astrocytes are located in the central part of the optic nerve. Asterisks indicate the ventricles. Abbreviations as in Figure 4. Scale bars = 50 μm.
Figure 4. Schematic drawings of transverse sections of the adult sea lamprey brain and spinal cord showing the distribution of GABAB transcripts (on the right side), and the main brain and spinal cord regions (on the left side). (A–G) Representative sections of the prosencephalon in a rostro-caudal order. (G,H) Representative sections of the mesencephalon in a rostro-caudal order. Note that section (G) includes rostral mesencephalic and caudal diencephalic regions. (I–L) Representative sections of the rhombencephalon in a rostro-caudal order. (M) Representative section of the spinal cord. The level of sections is indicated in the upper left figure showing a lateral view of the brain. Dots represent the location and an estimate of the relative density of both GABAB transcripts. Correspondence with photomicrographs in other figures is indicated by squared areas. Abbreviations: dC, dorsal cell; DCN, dorsal column nucleus; DG, dorsal gray; DIG, dorsal isthmic gray; DMN, dorsomedial neuropil; DN, dorsal nucleus of the octavolateral area; eC, edge cell; GL, glomerular layer; Ha, habenula; HY, hypothalamus; I1, isthmic Müller cell 1; IGL, inner granular layer; IIIi, intermediate oculomotor subnucleus; IIIl, lateral oculomotor subnucleus; IIIm, medial oculomotor subnucleus; IR, infundibular recess; IsRF, isthmic reticular formation; IVm, trochlear motor nucleus; LP, lateral pallium; LG, lateral gray; M1, Müller cell 1; M2, Müller cell 2; M3, Müller cell 3; MA, mature upstream migrating adult; MCL, mitral cell layer; MLF, medial longitudinal fasciculus; MN, medial nucleus of the octavolateral area; Mn, motor neurons; MP, medial pallium; Mr, mammilar region; MRF, middle rhombencephalic reticular formation; MT, mesencephalic tegmentum; nMLF, nucleus of the medial longitudinal fasciculus; NOMI, intermediate octavomotor nucleus; nTPOC, nucleus of the tract of the postoptic commissure; OB/P, olfactory bulbs/pallium; OLa, octavolateral area; OT, optic tectum; P, pineal organ; pc, posterior commissure; PCP, paracommissural preoptic nucleus; PM, post-metamorphic sea lamprey; PO, preoptic nucleus; POR, preoptic recess; PP, parapineal organ; PR, postoptic recess; PRF, posterior rhombencephalic reticular formation; PT, pretectum; PTh, prethalamus; PtN, posterior tubercular nucleus; PTu, posterior tuberculum; sa + gc, stratum “album” et griseum central; SCO, subcommissural organ; sfgs, stratum fibrosum et griseum superficiale; sgp, stratum griseum periventriculare; ShL, subhippocampal lobe; Sol, nucleus of the solitary tract; SP, septum; ST, striatum; Th, thalamus; TRF, trigeminal reticular formation; TS, torus semicircularis; Vm, trigeminal motor nucleus; VIIm, facial motor nucleus; VN, ventral nucleus of the octavolateral area; Xm, vagal motor nucleus. Scale bars = 50 μm.
Figure 5. Photomicrographs from different regions of the prosencephalon showing expression of both GABAB transcripts. Abbreviations as in Figure 4. (A–I) Photomicrographs of the forebrain regions indicated in Figure 4. (A,D,E,G) GABAB1. (B,C,F,H,I) GABAB2. Arrows indicate small positive dots. Arrowheads indicate accumulations of transcript expression in giant cells. Asterisks indicate the ventricle. Scale bars = 50 μm.
Figure 6. Photomicrographs from different regions of the mesencephalon (A,B), rhombencephalon (C–F) and spinal cord (G,H) showing expression of both GABAB transcripts. Abbreviations as in Figure 4. Arrows indicate small positive dots. The arrowhead indicates accumulations of transcript expression in a giant cell. Asterisks indicate the ventricle. Scale bars = 50 μm.
In the telencephalon, the olfactory bulbs showed numerous dots of positive GABAB1 and GABAB2 expression (Figures 4A, 5A). Most of the expression was observed in cells of the mitral and inner cellular layers. Some expression was also observed in cells localized between the glomeruli. In the medial pallium, GABAB1 and GABAB2 expression was very scarce in cells of the periventricular layer and no expression was observed in migrated cells (Figures 4C, 5B). We observed expression of both transcripts in neurons surrounding the dorsomedial neuropil (Figure 4C). The lateral pallium showed more GABAB1 and GABAB2 expression in the cells of the periventricular layer than in migrated cells (Figures 4B,C, 5C). In the subhippocampal lobe, most of the GABAB1 and GABAB2 expression was observed in the cells of the periventricular layer (Figure 4C). In the subpallium, the cells of the septum showed GABAB1 and GABAB2 positive dots (Figures 4B, 5D). In the striatum, GABAB1 and GABAB2 expression was observed mainly in migrated cells, with less expression being observed in the periventricular layer (Figures 3A,A′, 4C). In contrast, the preoptic nucleus showed wide GABAB1 and GABAB2 expression in periventricular cells, mainly in the intermediate layer of the preoptic nucleus (Figures 3A,A′, 4C).
The hypothalamus showed wide expression of the GABAB1 and GABAB2 transcripts in cells of the periventricular layers, while the migrated cells showed very scarce expression (Figure 5E). Many GABAB1 and GABAB2 positive dots were observed in the nucleus of the postoptic commissure, the paracommissural preoptic nucleus, the dorsal and ventral hypothalamus and the mammillary nucleus (Figures 4D–F, 5E). The pattern of expression observed in the hypothalamus was also observed in the prethalamus and thalamus (Figures 4D–F, 5F,G). GABAB1 and GABAB2 expression was also observed in the pineal and parapineal organs (Figure 4C). The habenula showed GABAB1 and GABAB2 expression, with some differences between the left and right sides (Figures 3B,B′, 4D,E). In the right habenula, which is bigger than the left one, GABAB1 and GABAB2 positive dots were more numerous than in the left habenula, although positive dots in cells of the left habenula were larger. The pretectum showed expression of both transcripts in cells of the periventricular layer and scarce expression in migrated neurons (Figure 4F). In the paratubercular nucleus, very few cells showed expression of the transcripts (Figure 4F). In contrast, a large number of GABAB1 and GABAB2 positive dots were observed in the nucleus of the medial longitudinal fascicle including the first and second Müller cells (Figures 4G, 5I).
In the mesencephalon, the optic tectum showed GABAB1 and GABAB2 positive dots with most of them distributed throughout the cells of the stratum griseum periventriculare, but also in cells of the stratum “album” et griseum central (Figures 3C,C′, 4H). The torus semicircularis showed numerous positive dots. The positive dots in cells of the torus were larger than those in the optic tectum (Figure 4H). The migrated neurons of both areas showed very little expression of the transcripts. There was strong GABAB1 and GABAB2 expression in the tegmentum and the mesencephalic reticular area (including the third Müller cell) and the motor neurons of the oculomotor nuclei showed a large amount of GABAB1 and GABAB2 expression in their somas (Figure 4H).
In the alar plate of the rhombencephalon, the dorsal isthmic gray showed expression of both transcripts (Figures 4I, 6A). In the octavolateral nuclei, there was GABAB1 and GABAB2 expression, mainly in cells of the periventricular layer (Figures 4J,K, 6B,C). The octavomotor nuclei showed GABAB1 and GABAB2 positive dots (Figures 4K, 6C). Numerous GABAB1 and GABAB2 positive dots were observed in cells of the periventricular layer of the solitary tract (Figures 4L, 6D) and dorsal column nuclei. Dorsal cells of the caudal rhombencephalon (primary medullary and spinal nucleus of the trigeminus) also showed GABAB1 and GABAB2 expression (Figures 6B,C). In the basal plate of the rhombencephalon, the soma of the cells of the visceromotor nuclei (V, VII, IX and X) showed many GABAB1 and GABAB2 positive dots (Figures 4J–L, 6E). In addition, wide GABAB1 and GABAB2 expression was observed in all rhombencephalic reticular nuclei (isthmic, trigeminal, middle and posterior reticular nuclei), including the giant Müller and Mauthner cells (Figures 4I–L, 6F).
In the rostral spinal cord of the adult sea lamprey, most neuronal types showed GABAB1 and GABAB2 expression (Figures 4M, 6G,H). Dorsal cells showed large amounts of GABAB1 and GABAB2 positive dots in their soma (Figures 4M, 6G). We also observed some expression of the transcripts in the edge cells (Figure 4M). GABAB1 and GABAB2 expression was observed in interneurons of different sizes located in the dorsal and lateral regions (Figures 4M, 6G). Expression of both transcripts was observed in the somas of motor neurons (Figure 6G). Cerebrospinal fluid-contacting (CSF-c) cells showed numerous GABAB1 and GABAB2 positive dots, even in their apical dendrites (Figure 6H).
The glial cells of the brain of the sea lamprey are almost exclusively ependymal cells, although astrocytes could be identified in the optic nerve. No expression of any of the two transcripts was observed in brain ependymocytes (examples in Figures 5B,C) or in the subcommissural organ (Figures 4F, 5H). The astrocytes of the optic nerve, which are easily identified because of the absence of neurons in the optic nerve, did not show expression of the transcripts (Figures 3D,D′).
Here, we report for the first time the identification and characterization of the GABAB1 and GABAB2 cDNA sequences and the distribution of the GABAB transcripts in the CNS of the adult sea lamprey.
In our BLAST searches of the sea lamprey genome database, we found 1 GABAB1 gene and 1 GABAB2 gene, whose sequences were confirmed after PCR amplification and cloning of the respective cDNAs. The presence of only a single gene of each GABAB subunit is also the case in mammals, birds and amphibians. Two paralogous copies of the GABAB1 subunit gene have been found in zebrafish (Klee et al., 2012), which is probably due to the additional whole-genome duplication that occurred in the actinopterygian lineage (Taylor et al., 2001). In the phylogenetic trees, the GABAB1 and GABAB2 partial amino acid sequences of the sea lamprey were located at the base of the vertebrate branches clustering the GABAB1 and GABAB2 sequences, appearing as sister members, respectively, of the GABAB1 and GABAB2 sequences of gnathostomes. Each of the subunits is grouped in an independent cluster (Figure 2). The location of the sea lamprey GABAB1 and GABAB2 sequences at the base of the vertebrate branches of the phylogenetic tree and its higher similarity with the vertebrate sequences than with those of Drosophila are in agreement with the phylogenetic position of lampreys and confirmed the GABAB identity of the sea lamprey sequences identified in our study.
It is currently accepted that a functional GABAB receptor consists of a heterodimer of GABAB1 and GABAB2 subunits (Jones et al., 1998; Kaupmann et al., 1998; White et al., 1998; Bettler et al., 2004). GABAB1 binds to GABA, while GABAB2 is needed to transmit the signal, because G-protein coupling is mediated via GABAB2 (Margeta-Mitrovic et al., 2000; Calver et al., 2001; Galvez et al., 2001; Pagano et al., 2001; Geng et al., 2013). Because of this, it seems clear that the co-expression of GABAB1 and GABAB2 mRNAs is necessary to form a functional GABAB receptor. In our study, we observed an overlapping expression of the GABAB1 and GABAB2 mRNAs in all brain regions and in the spinal cord of the adult sea lamprey when using consecutive brain sections. Co-localization of both subunits in the same single cells of lampreys is clear in the giant individually identifiable reticulospinal neurons like the Mauthner and Müller neurons. This is also the case in D. melanogaster, where no differences were observed between the expressions of GABAB subunits in in situ hybridization assays (Mezler et al., 2001). In zebrafish, a recent study using qPCR methods has shown that the two b1 and the b2 subunits are all expressed in the same brain regions, with the b1b and b2 being more represented than the b1a in some regions and in the brain as a whole (Cocco et al., 2016). Kuner et al. (1999) observed by analyzing serial rat brain sections, that GABAB1 and GABAB2 transcripts are widely expressed and that they also show considerable overlap in most regions of the brain, although in some regions the expression of the GABAB1 transcript was enriched. This suggests that a wide and highly overlapping expression of the GABAB1 and GABAB2 subunits is an ancestral and conserved character of vertebrates and invertebrates.
In the mouse brain, an association between the GABAB2 subunit and M2 muscarinic receptors has been shown which appears to enhance muscarinic signaling (Boyer et al., 2009). In lampreys, pharmacological treatments combined with electrophysiological studies have shown a role for muscarinic receptors in the modulation of the trigeminal-reticular pathway (Le Ray et al., 2004) and in the activation of reticulospinal neurons (Smetana et al., 2007). Moreover, the presence of cells immunoreactive for muscarinic receptors has been shown in the region of the posterior rhombencephalic reticular nucleus of lampreys (Smetana et al., 2007), a region that shows the expression of the GABAB2 transcript (present results). Whether the modulation of muscarinic receptors by the GABAB2 subunit also occurs in lampreys needs further investigation. This would show us whether this is an ancestral characteristic of vertebrates.
All brain regions and the spinal cord showed a broad expression of both GABAB transcripts in the adult sea lamprey, which is in concordance with previous reports in invertebrates (e.g., D. melanogaster (Mezler et al., 2001), cockroaches (Blankenburg et al., 2015) or spiders (Panek et al., 2003)) and in jawed vertebrates (e.g., humans (Calver et al., 2000; Berthele et al., 2001), non-human primates (Muñoz et al., 1998; Nürnberger and Schöniger, 2001), rats (Bowery et al., 1987; Bischoff et al., 1999; Clark et al., 2000), birds (Veenman et al., 1994), frogs (Kaeser et al., 2011) and zebrafish (Tabor et al., 2008; Cocco et al., 2016)). Positive in situ signal in sea lamprey brain sections had a granular appearance probably due to low expression of these mRNAs in each single cell of the sea lamprey. Previous studies looking at the expression of different neurotransmitter receptors in lampreys have shown that the in situ hybridization signals appeared as a dotted labeling in sections of the CNS: serotonin receptor 1A (Cornide-Petronio et al., 2013, 2014), dopamine receptor D2 (Robertson et al., 2012; Fernández-López et al., 2015), and dopamine receptor D4 (Pérez-Fernández et al., 2016); suggesting that low expression levels are a common feature of different metabotropic neurotransmitter receptors.
The broad expression of these transcripts in the CNS suggests that this receptor is extensively used in the modulation of brain circuits in lampreys. The expression of both GABAB transcripts in non-GABAergic cells, which can be identified by their size and/or location, such as the mitral cells of the olfactory bulbs, the giant reticulospinal neurons, the spinal motoneurons, the motoneurons of the visceromotor rhombencephalic nuclei, the primary sensory cells of the rhombencephalon and spinal cord or the edge cells of the spinal cord indicate that the GABAB receptor plays a role in the modulation of the activity of non-GABAergic cells in the sea lamprey brain. The expression of GABAB transcripts observed here is in agreement with the GABAergic modulation of spinal motoneurons and interneurons mediated by the GABAB receptor as reported in previous pharmacological and electrophysiological studies (Alford and Grillner, 1991; Alford et al., 1991; Matsushima et al., 1993; Schmitt et al., 2004). Edge cells in the spinal cord are richly innervated by GABAergic fibers (Fernández-López et al., 2012) and recent work has shown that they are modulated by GABA (Svensson et al., 2013). Our results suggest that GABA could act trough the GABAB receptor in the edge cells. The present results also support the idea of the role of the GABAB receptor in the modulation of the lamprey respiratory network (Bongianni et al., 2006; Cinelli et al., 2014). Also, in agreement with our expression results, a modulatory action of GABA onto the pathway from the lateral columns to reticulospinal neurons has been suggested to be mediated by the GABAB receptor (Vinay et al., 1998). These reticulospinal inputs are also regulated by peptidergic transmitters (Parker, 2000). Our study extends the number of neuronal populations known to be modulated through GABAB signaling and opens the opportunity to conduct functional studies on the role of GABA and the GABAB receptor in other circuits.
The broad expression of the GABAB transcripts together with the broad distribution of GABAergic cells in the brain and spinal cord (Meléndez-Ferro et al., 2001; Robertson et al., 2007) suggests that the sea lamprey GABAB receptor could modulate the activity of GABAergic cells. The expression of the GABAB transcripts in periglomerular cells of the olfactory bulbs, which are mainly GABAergic (Meléndez-Ferro et al., 2001), or in the CSF-c cells of the spinal cord, which are mainly GABAergic as well (Rodicio et al., 2008; Villar-Cerviño et al., 2008; Fernández-López et al., 2012; Jalalvand et al., 2014), could potentially support this hypothesis. Results from pharmacological and electrophysiological studies have shown that inhibitory premotor interneurons respond to the application of GABAB agonists (Alford and Grillner, 1991; Alford et al., 1991; Matsushima et al., 1993). Unfortunately, the different requirements of fixative for in situ hybridization and GABA immunohistochemistry precluded us from providing a definitive demonstration of the presence of GABAB receptors in GABAergic cells of the sea lamprey. The present results show expression of the GABAB transcripts in the dendrites of CSF-c cells of the spinal cord. Currently, some functions have been proposed for CSF-c neurons in controlling the composition of the CSF and releasing substances into the ventricular system (Vígh et al., 2004; Jalalvand et al., 2016). The GABAB receptor in these cells could play a role for detecting GABA in the CSF. The detection of serotonin from the CSF has been also proposed for the 5-HT1A receptor due its expression in CSF-c dendrites (Cornide-Petronio et al., 2013). As stated above, the GABAB receptor could modulate CSF-c cells acting as an autoreceptor and/or as a heteroreceptor trough synapses from other GABAergic cells.
Our results show a lack of expression of the GABAB transcripts in ependymocytes along the lamprey brain. The ependymal cells are the main glial type present in the brain of the sea lamprey, while astrocytes are only associated to some nervous tracts. However, the spinal cord shows both types of glial cells (Retzius, 1893). In contrast to jawed vertebrates, oligodendrocytes are not present in lampreys. The glial cells of lampreys do not display immunoreactivity to glial fibrillary acid protein (GFAP), but they express cytokeratins (Merrick et al., 1995). Few works have been done to study the expression of GABAB1 and GABAB2 mRNAs and/or GABAB1 and GABAB2 subunits in the ependymal layer of vertebrates. A pharmacological study of Corns et al. (2013) reported that only the GABAA, and not GABAB, receptor mediates the GABAergic responses in mammalian ependymal cells surrounding the central canal. More studies in other groups of vertebrates are necessary to determine the evolution of this character due to the large evolution distance between mammals and lampreys. In addition, the cells of the subcommissural organ, a special type of ependymal cells, also lack the GABAB receptor both in lampreys, and in other vertebrates like teleosts, frogs and mammals (Jiménez et al., 2000; Saha et al., 2000; Nürnberger and Schöniger, 2001). Studies in these vertebrates have shown that the GABAergic responses in the subcommissural organ are also mediated by GABAA receptors.
No expression of the GABAB transcripts was observed in astrocytes of the optic nerve although previous studies have reported the presence of GABAB subunits in astrocytes, of rodents (Charles et al., 2003; Oka et al., 2006; Luyt et al., 2007; Beenhakker and Huguenard, 2010), and the expression of GABAB1 and GABAB2 mRNAs in human astrocytes (Lee et al., 2011). Again, more studies are necessary in other groups of vertebrates to determine whether the lack of GABAB expression in astrocytes is the ancestral condition or a derived character.
In this study we show for the first time the distribution of the GABAB transcripts in the CNS of the sea lamprey. The location of the lamprey GABAB1 and GABAB2 sequences in the phylogenetic trees and their average of similarity with those of other species are in agreement with the phylogenetic position of lamprey and confirms their identity. A wide and overlapping expression of both GABAB transcripts was observed in neurons of the brain and spinal cord of the sea lamprey. In contrast, no expression of the transcripts was observed in the ependymal layer. The broad expression of the GABAB transcripts in the neuronal populations of the CNS and their absence in ependymocytes is in agreement with that observed in other vertebrates.
DR-S, BF-L, AB-I and MCR contributed to the acquisition of experimental data, data analysis/interpretation and drafting of the manuscript. DS-C contributed to the acquisition of experimental data and data analysis/interpretation; AB-I and MCR contributed to the concept/design of the study. All authors have approved the final manuscript.
This work was supported by grants from the Spanish Ministry of Science and Innovation-FEDER (BFU2010-17174), Spanish Ministry of Economy and Competitiveness-FEDER (BFU2014-56300-P) and Xunta de Galicia (GPC2014/030). ABI was supported by a grant from the Xunta de Galicia (2016-PG008).
The authors declare that the research was conducted in the absence of any commercial or financial relationships that could be construed as a potential conflict of interest.
We thank the Consellería de Medio Ambiente (Xunta de Galicia) for the permission to capture the lampreys used in the study.
Alford, S., Christenson, J., and Grillner, S. (1991). Presynaptic GABAA and GABAB receptor-mediated phasic modulation in axons of spinal motor interneurons. Eur. J. Neurosci. 3, 107–117. doi: 10.1111/j.1460-9568.1991.tb00071.x
Alford, S., and Grillner, S. (1991). The involvement of GABAB receptors and coupled G-proteins in spinal GABAergic presynaptic inhibition. J. Neurosci. 11, 3718–3726.
Barreiro-Iglesias, A. (2012). ‘Evorego’: studying regeneration to understand evolution, the case of the serotonergic system. Brain Behav. Evol. 79, 1–3. doi: 10.1159/000334187
Barreiro-Iglesias, A. (2015). “Bad regenerators” die after spinal cord injury: insights from lampreys. Neural Regen. Res. 10, 25–27. doi: 10.4103/1673-5374.150642
Barreiro-Iglesias, A., Cornide-Petronio, M. E., Anadón, R., and Rodicio, M. C. (2009a). Serotonin and GABA are colocalized in restricted groups of neurons in the larval sea lamprey brain: insights into the early evolution of neurotransmitter colocalization in vertebrates. J. Anat. 215, 435–443. doi: 10.1111/j.1469-7580.2009.01119.x
Barreiro-Iglesias, A., Villar-Cerviño, V., Anadón, R., and Rodicio, M. C. (2009b). Dopamine and gamma-aminobutyric acid are colocalized in restricted groups of neurons in the sea lamprey brain: insights into the early evolution of neurotransmitter colocalization in vertebrates. J. Anat. 215, 601–610. doi: 10.1111/j.1469-7580.2009.01159.x
Beenhakker, M. P., and Huguenard, J. R. (2010). Astrocytes as gatekeepers of GABAB receptor function. J. Neurosci. 30, 15262–15276. doi: 10.1523/JNEUROSCI.3243-10.2010
Berthele, A., Platzer, S., Weis, S., Conrad, B., and Tölle, T. R. (2001). Expression of GABAB1 and GABAB2 mRNA in the human brain. Neuroreport 12, 3269–3275. doi: 10.1097/00001756-200110290-00025
Bettler, B., Kaupmann, K., Mosbacher, J., and Gassmann, M. (2004). Molecular structure and physiological functions of GABAB receptors. Physiol. Rev. 84, 835–867. doi: 10.1152/physrev.00036.2003
Bischoff, S., Leonhard, S., Reymann, N., Schuler, V., Shigemoto, R., Kaupmann, K., et al. (1999). Spatial distribution of GABABR1 receptor mRNA and binding sites in the rat brain. J. Comp. Neurol. 412, 1–16. doi: 10.1002/(SICI)1096-9861(19990913)412:1<1::AID-CNE1>3.0.CO;2-D
Blankenburg, S., Balfanz, S., Hayashi, Y., Shigenobu, S., Miura, T., Baumann, O., et al. (2015). Cockroach GABAB receptor subtypes: molecular characterization, pharmacological properties and tissue distribution. Neuropharmacology 88, 134–144. doi: 10.1016/j.neuropharm.2014.08.022
Blein, S., Hawrot, E., and Barlow, P. (2000). The metabotropic GABA receptor: molecular insights and their functional consequences. Cell. Mol. Life Sc. 57, 635–650. doi: 10.1007/pl00000725
Bongianni, F., Mutolo, D., Nardone, F., and Pantaleo, T. (2006). GABAergic and glycinergic inhibitory mechanisms in the lamprey respiratory control. Brain Res. 1090, 134–145. doi: 10.1016/j.brainres.2006.03.056
Bowery, N. G., Hudson, A. L., and Price, G. W. (1987). GABAA and GABAB receptor site distribution in the rat central nervous system. Neuroscience 20, 365–383. doi: 10.1016/0306-4522(87)90098-4
Boyer, S. B., Clancy, S. M., Terunuma, M., Revilla-Sánchez, R., Thomas, S. M., Moss, S. J., et al. (2009). Direct interaction of GABAB receptors with M2 muscarinic receptors enhances muscarinic signaling. J. Neurosci. 29, 15796–15809. doi: 10.1523/JNEUROSCI.4103-09.2009
Calver, A. R., Davies, C. H., and Pangalos, M. (2002). GABAB receptors: from monogamy to promiscuity. Neurosignals 11, 299–314. doi: 10.1159/000068257
Calver, A. R., Medhurst, A. D., Robbins, M. J., Charles, K. J., Evans, M. L., Harrison, D. C., et al. (2000). The expression of GABAB1 and GABAB2 receptor subunits in the cNS differs from that in peripheral tissues. Neuroscience 100, 155–170. doi: 10.1016/s0306-4522(00)00262-1
Calver, A. R., Robbins, M. J., Cosio, C., Rice, S. Q., Babbs, A. J., Hirst, W. D., et al. (2001). The C-terminal domains of the GABAb receptor subunits mediate intracellular trafficking but are not required for receptor signaling. J. Neurosci. 21, 1203–1210.
Chalifoux, J. R., and Carter, A. G. (2011). GABAB receptor modulation of synaptic function. Curr. Opin. Neurobiol. 21, 339–344. doi: 10.1016/j.conb.2011.02.004
Charles, K. J., Deuchars, J., Davies, C. H., and Pangalos, M. N. (2003). GABAB receptor subunit expression in glia. Mol. Cell. Neurosci. 24, 214–223. doi: 10.1016/S1044-7431(03)00162-3
Charles, K. J., Evans, M. L., Robbins, M. J., Calver, A. R., Leslie, R. A., and Pangalos, M. N. (2001). Comparative immunohistochemical localisation of GABAB1a, GABAB1b and GABAB2 subunits in rat brain, spinal cord and dorsal root ganglion. Neuroscience 106, 447–467. doi: 10.1016/s0306-4522(01)00296-2
Cinelli, E., Mutolo, D., Robertson, B., Grillner, S., Contini, M., Pantaleo, T., et al. (2014). GABAergic and glycinergic inputs modulate rhythmogenic mechanisms in the lamprey respiratory network. J. Physiol. 592, 1823–1838. doi: 10.1113/jphysiol.2013.268086
Clark, J. A., Mezey, E., Lam, A. S., and Bonner, T. I. (2000). Distribution of the GABAB receptor subunit gb2 in rat CNS. Brain Res. 860, 41–52. doi: 10.1016/s0006-8993(00)01958-2
Cocco, A., Carolina Rönnberg, A. M., Jin, Z., André, G. I., Vossen, L. E., Bhandage, A. K., et al. (2016). Characterization of the γ-aminobutyric acid signaling system in the zebrafish (Danio rerio Hamilton) central nervous system by reverse transcription-quantitative polymerase chain reaction. Neuroscience doi: 10.1016/j.neuroscience.2016.07.018 [Epub ahead of print].
Cornide-Petronio, M. E., Anadón, R., Barreiro-Iglesias, A., and Rodicio, M. C. (2013). Serotonin 1A receptor (5-HT1A) of the sea lamprey: cDNA cloning and expression in the central nervous system. Brain Struct. Funct. 218, 1317–1335. doi: 10.1007/s00429-012-0461-y
Cornide-Petronio, M. E., Fernández-López, B., Barreiro-Iglesias, A., and Rodicio, M. C. (2014). Traumatic injury induces changes in the expression of the serotonin 1A receptor in the spinal cord of lampreys. Neuropharmacology 77, 369–378. doi: 10.1016/j.neuropharm.2013.10.017
Corns, L. F., Deuchars, J., and Deuchars, S. A. (2013). GABAergic responses of mammalian ependymal cells in the central canal neurogenic niche of the postnatal spinal cord. Neurosci. Lett. 553, 57–62. doi: 10.1016/j.neulet.2013.07.007
Curtis, D. R., Hösli, L., and Johnston, G. A. (1968). Inhibitory effect of glycine and similar amino acids on spinal neurons. Helv. Physiol. Pharmacol. Acta 26, 205–206.
Curtis, D. R., Lodge, D., and Brand, S. J. (1977). GABA and spinal afferent terminal excitability in the cat. Brain Res. 130, 360–363. doi: 10.1016/0006-8993(77)90283-9
Dubuc, R., Brocard, F., Antri, M., Fénelon, K., Gariépy, J. F., Smetana, R., et al. (2008). Initiation of locomotion in lampreys. Brain Res. Rev. 57, 172–182. doi: 10.1016/j.brainresrev.2007.07.016
Fernández-López, B., Romaus-Sanjurjo, D., Cornide-Petronio, M. E., Gómez-Fernández, S., Barreiro-Iglesias, A., and Rodicio, M. C. (2015). Full anatomical recovery of the dopaminergic system after a complete spinal cord injury in lampreys. Neural Plast. 2015:350750. doi: 10.1155/2015/350750
Fernández-López, B., Valle-Maroto, S. M., Barreiro-Iglesias, A., and Rodicio, M. C. (2014). Neuronal release and successfull astrocyte uptake of aminoacidergic neurotransmitters after spinal cord injury in lampreys. Glia 62, 1254–1269. doi: 10.1002/glia.22678
Fernández-López, B., Villar-Cerviño, V., Valle-Maroto, S. M., Barreiro-Iglesias, A., Anadón, R., and Rodicio, M. C. (2012). The glutamatergic neurons in the spinal cord of the sea lamprey: an in situ hybridization and immunohistochemical study. PLoS One 7:e47898. doi: 10.1371/journal.pone.0047898
Fritschy, J. M., Meskenaite, V., Weinmann, O., Honer, M., Benke, D., and Mohler, H. (1999). GABAB-receptor splice variants GB1a and GB1b in rat brain: developmental regulation, cellular distribution and extrasynaptic localization. Eur. J. Neurosci. 11, 761–768. doi: 10.1046/j.1460-9568.1999.00481.x
Galvez, T., Duthey, B., Kniazeff, J., Blahos, J., Rovelli, G., Bettler, B., et al. (2001). Allosteric interactions between GB1 and GB2 subunits are required for optimal GABAB receptor function. EMBO J. 20, 2152–2159. doi: 10.1093/emboj/20.9.2152
Geng, Y., Bush, M., Mosyak, L., Wang, F., and Fan, Q. R. (2013). Structural mechanism of ligand activation in human GABAB receptor. Nature 504, 254–259. doi: 10.1038/nature12725
Grillner, S., Wallén, P., Saitoh, K., Kozlov, A., and Robertson, B. (2008). Neural bases of goal-directed locomotion in vertebrates–an overview. Brain Res. Rev. 57, 2–12. doi: 10.1016/j.brainresrev.2007.06.027
Hardisty, M. W., and Potter, I. C. (1971). “The general biology of adult lampreys,” in The Biology of Lampreys, (Vol. 1), eds M. W. Hardisty and I. C. Potter (London: Academic Press), 127–206.
Jalalvand, E., Robertson, B., Wallén, P., and Grillner, S. (2016). Ciliated neurons lining the central canal sense both fluid movement and pH through ASIC3. Nat. Commun. 7:10002. doi: 10.1038/ncomms10002
Jalalvand, E., Robertson, B., Wallén, P., Hill, R. H., and Grillner, S. (2014). Laterally projecting cerebrospinal fluid-contacting cells in the lamprey spinal cord are of two distinct types. J. Comp. Neurol. 522, 1753–1768. doi: 10.1002/cne.23542
Jiménez, A. J., Aller, M. I., Martín, R., Pérez-Martín, M., Pérez-Fígares, J. M., Fernández-López, A., et al. (2000). The subcommissural organ of the frog Rana perezi is innervated by nerve fibres containing GABA. Cell Tissue Res. 299, 253–262. doi: 10.1007/s004410050023
Jones, K. A., Borowsky, B., Tamm, J. A., Craig, D. A., Durkin, M. M., Dai, M., et al. (1998). GABAB receptors function as a heteromeric assembly of the subunits GABABR1 and GABABR2. Nature 396, 674–679. doi: 10.1038/25348
Kaeser, G. E., Rabe, B. A., and Saha, M. S. (2011). Cloning and characterization of GABAA α subunits and GABAB subunits in Xenopus laevisduring development. Dev. Dyn. 240, 862–873. doi: 10.1002/dvdy.22580
Kammerer, R. A., Frank, S., Schulthess, T., Landwehr, R., Lustig, A., and Engel, J. (1999). Heterodimerization of a functional GABAB receptor is mediated by parallel coiled-coil α-helices. Biochemistry. 38, 3263–13269. doi: 10.1021/bi991018t
Kaupmann, K., Huggel, K., Heid, J., Flor, P. J., Bischoff, S., Mickel, S. J., et al. (1997). Expression cloning of GABAB receptors uncovers similarity to metabotropic glutamate receptors. Nature 386, 239–246. doi: 10.1038/386239a0
Kaupmann, K., Malitschek, B., Schuler, V., Heid, J., Froestl, W., Beck, P., et al. (1998). GABAB-receptor subtypes assemble into functional heteromeric complexes. Nature 396, 683–687. doi: 10.1038/25360
Klee, E. W., Schneider, H., Clark, K. J., Cousin, M. A., Ebbert, J. O., Hooten, W. M., et al. (2012). Zebrafish: a model for the study of addiction genetics. Hum. Genet. 131, 977–1008. doi: 10.1007/s00439-011-1128-0
Kuner, R., Köhr, G., Grünewald, S., Eisenhardt, G., Bach, A., and Kornau, H. C. (1999). Role of heteromer formation in GABAB receptor function. Science 283, 74–77. doi: 10.1126/science.283.5398.74
Lee, M., Schwab, C., and McGeer, P. L. (2011). Astrocytes are GABAergic cells that modulate microglial activity. Glia 59, 152–165. doi: 10.1002/glia.21087
Le Ray, D., Brocard, F., and Dubuc, R. (2004). Muscarinic modulation of the trigemino-reticular pathway in lampreys. J. Neurophysiol. 92, 926–938. doi: 10.1152/jn.01025.2003
Letunic, I., Doerks, T., and Bork, P. (2015). SMART: recent updates, new developments and status in 2015. Nucleic Acids Res. 43, D257–D260. doi: 10.1093/nar/gku949
Luyt, K., Slade, T. P., Dorward, J. J., Durant, C. F., Wu, Y., Shigemoto, R., et al. (2007). Developing oligodendrocytes express functional GABAB receptors that stimulate cell proliferation and migration. J. Neurochem. 100, 822–840. doi: 10.1111/j.1471-4159.2006.04255.x
Margeta-Mitrovic, M., Jan, Y. N., and Jan, L. Y. (2000). A trafficking checkpoint controls GABAB receptor heterodimerization. Neuron 27, 97–106. doi: 10.1016/s0896-6273(00)00012-x
Matsushima, T., Tegnér, J., Hill, R. H., and Grillner, S. (1993). GABAB receptor activation causes a depression of low- and high-voltage-activated Ca2+ currents, postinhibitory rebound, and postspike afterhyperpolarization in lamprey neurons. J. Neurophysiol. 70, 2606–2619.
Maurel, D., Comps-Agrar, L., Brock, C., Rives, M. L., Bourrier, E., Ayoub, M. A., et al. (2008). Cell-surface protein-protein interaction analysis with time-resolved FRET and snap-tag technologies: application to GPCR oligomerization. Nat. Methods 5, 561–567. doi: 10.1038/nmeth.1213
Meléndez-Ferro, M., Pérez-Costas, E., González, M. J., Pombal, M. A., Anadón, R., and Rodicio, M. C. (2000). GABA-immunoreactive internuclear neurons in the ocular motor system of lampreys. Brain Res. 855, 150–157. doi: 10.1016/s0006-8993(99)02402-6
Meléndez-Ferro, M., Pérez-Costas, E., Rodríguez-Muñoz, R., Gómez-López, M. P., Anadón, R., and Rodicio, M. C. (2001). GABA immunoreactivity in the olfactory bulbs of the adult sea lamprey Petromyzon marinus L. Brain Res. 893, 253–260. doi: 10.1016/s0006-8993(00)03316-3
Meléndez-Ferro, M., Pérez-Costas, E., Villar-Cheda, B., Abalo, X. M., Rodríguez-Muñoz, R., Rodicio, M. C., et al. (2002). Ontogeny of gamma-aminobutyric acid-immunoreactive neuronal populations in the forebrain and midbrain of the sea lamprey. J. Comp. Neurol. 446, 360–376. doi: 10.1002/cne.10209
Meléndez-Ferro, M., Pérez-Costas, E., Villar-Cheda, B., Rodríguez-Muñoz, R., Anadón, R., and Rodicio, M. C. (2003). Ontogeny of gamma-aminobutyric acid-immunoreactive neurons in the rhombencephalon and spinal cord of the sea lamprey. J. Comp. Neurol. 464, 17–35. doi: 10.1002/cne.10773
Merrick, S. E., Pleasure, S. J., Lurie, D. I., Pijak, D. S., Selzer, M. E., and Lee, V. M. (1995). Glial cells of the lamprey nervous system contain keratin-like proteins. J. Comp. Neurol. 355, 199–210. doi: 10.1002/cne.903550204
Mezler, M., Müller, T., and Raming, K. (2001). Cloning and functional expression of GABAB receptors from Drosophila. Eur. J. Neurosci. 13, 477–486. doi: 10.1046/j.1460-9568.2001.01410.x
Muñoz, A., DeFelipe, J., and Jones, E. G. (2001). Patterns of GABABR1a,b receptor gene expression in monkey and human visual cortex. Cereb. Cortex 11, 104–113. doi: 10.1093/cercor/11.2.104
Muñoz, A., Huntsman, M. M., and Jones, E. G. (1998). GABAB receptor gene expression in monkey thalamus. J. Comp. Neurol. 394, 118–126. doi: 10.1002/(SICI)1096-9861(19980427)394:1<118::AID-CNE9>3.0.CO;2-3
Nürnberger, F., and Schöniger, S. (2001). Presence and functional significance of neuropeptide and neurotransmitter receptors in subcommissural organ cells. Microsc. Res. Tech. 52, 534–540. doi: 10.1002/1097-0029(20010301)52:5<534::aid-jemt1038>3.0.co;2-r
Oka, M., Wada, M., Wu, Q., Yamamoto, A., and Fujita, T. (2006). Functional expression of metabotropic GABAB receptors in primary cultures of astrocytes from rat cerebral cortex. Biochem. Biophys. Res. Commun. 341, 874–881. doi: 10.1016/j.bbrc.2006.01.039
Padgett, C. L., and Slesinger, P. A. (2010). GABAB receptor coupling to G-proteins and ion channels. Adv. Pharmacol. 58, 123–147. doi: 10.1016/s1054-3589(10)58006-2
Pagano, A., Rovelli, G., Mosbacher, J., Lohmann, T., Duthey, B., Stauffer, D., et al. (2001). C-terminal interaction is essential for surface trafficking but not for heteromeric assembly of GABAB receptors. J. Neurosci. 21, 1189–1202.
Panek, I., Meisner, S., and Torkkeli, P. H. (2003). Distribution and function of GABAB receptors in spider peripheral mechanosensilla. J. Neurophysiol. 90, 2571–2580. doi: 10.1152/jn.00321.2003
Parker, D. (2000). Presynaptic and interactive peptidergic modulation of reticulospinal synaptic inputs in the lamprey. J. Neurophysiol. 83, 2497–2507.
Parker, D., Söderberg, C., Zotova, E., Shupliakov, O., Langel, U., Bartfai, T., et al. (1998). Co-localized neuropeptide Y and GABA have complementary presynaptic effects on sensory synaptic transmission. Eur. J. Neurosci. 10, 2856–2870. doi: 10.1111/j.1460-9568.1998.00295.x
Pérez-Fernández, J., Megías, M., and Pombal, M. A. (2016). Expression of a novel D4 dopamine receptor in the lamprey brain. evolutionary considerations about dopamine receptors. Front. Neuroanat. 9:165. doi: 10.3389/fnana.2015.00165
Pinard, A., Seddik, R., and Bettler, B. (2010). GABAB receptors: physiological functions and mechanisms of diversity. Adv. Pharmacol. 58, 231–255. doi: 10.1016/s1054-3589(10)58010-4
Pregitzer, P., Schultze, A., Raming, K., Breer, H., and Krieger, J. (2013). Expression of a GABAB–receptor in olfactory sensory neurons of sensilla trichodea on the male antenna of the moth Heliothis virescens. Int. J. Biol. Sci. 9, 707–715. doi: 10.7150/ijbs.6674
Ramoino, P., Gallus, L., Beltrame, F., Diaspro, A., Fato, M., Rubini, P., et al. (2006). Endocytosis of GABAB receptors modulates membrane excitability in the single-celled organism Paramecium. J. Cell Sci. 119, 2056–2064. doi: 10.1242/jcs.02931
Ramoino, P., Gallus, L., Paluzzi, S., Raiteri, L., Diaspro, A., Fato, M., et al. (2007). The GABAergic-like system in the marine demosponge Chondrilla nucula. Microsc. Res. Tech. 70, 944–951. doi: 10.1002/jemt.20499
Robertson, B., Auclair, F., Ménard, A., Grillner, S., and Dubuc, R. (2007). GABA distribution in lamprey is phylogenetically conserved. J. Comp. Neurol. 503, 47–63. doi: 10.1002/cne.21348
Robertson, B., Huerta-Ocampo, I., Ericsson, J., Stephenson-Jones, M., Pérez-Fernández, J., Bolam, J. P., et al. (2012). The dopamine D2 receptor gene in lamprey, its expression in the striatum and cellular effects of D2 receptor activation. PLoS One 7:e35642. doi: 10.1371/journal.pone.0035642
Rodicio, M. C., and Barreiro-Iglesias, A. (2012). Lampreys as an animal model in regeneration studies after spinal cord injury. Rev. Neurol. 55, 157–166.
Rodicio, M. C., Villar-Cerviño, V., Barreiro-Iglesias, A., and Anadón, R. (2008). Colocalization of dopamine and GABA in spinal cord neurones in the sea lamprey. Brain Res. Bull. 76, 45–49. doi: 10.1016/j.brainresbull.2007.10.062
Ruiz, Y., Pombal, M. A., and Megías, M. (2004). Development of GABA-immunoreactive cells in the spinal cord of the sea lamprey, P. marinus. J. Comp. Neurol. 470, 151–163. doi: 10.1002/cne.11032
Saha, S. G., Jain, M. R., and Subhedar, N. (2000). Subcommissural organ-Reissner’s fiber complex of the teleost Clarias batrachus responds to GABA treatment. Brain Res. 852, 335–343. doi: 10.1016/s0006-8993(99)02144-7
Schmitt, D. E., Hill, R. H., and Grillner, S. (2004). The spinal GABAergic system is a strong modulator of burst frequency in the lamprey locomotor network. J. Neurophysiol. 92, 2357–2367. doi: 10.1152/jn.00233.2004
Schultheis, C., Brauner, M., Liewald, J. F., and Gottschalk, A. (2011). Optogenetic analysis of GABAB receptor signaling in Caenorhabditis elegans motor neurons. J. Neurophysiol. 106, 817–827. doi: 10.1152/jn.00578.2010
Schultz, J., Milpetz, F., Bork, P., and Ponting, C. P. (1998). SMART, a simple modular architecture research tool: identification of signaling domains. Proc. Natl. Acad. Sci. U S A 95, 5857–5864. doi: 10.1073/pnas.95.11.5857
Smetana, R. W., Alford, S., and Dubuc, R. (2007). Muscarinic receptor activation elicits sustained, recurring depolarizations in reticulospinal neurons. J. Neurophysiol. 97, 3181–3192. doi: 10.1152/jn.00954.2006
Smith, J. J., Kuraku, S., Holt, C., Sauka-Spengler, T., Jiang, N., Campbell, M. S., et al. (2013). Sequencing of the sea lamprey (Petromyzon marinus) genome provides insights into vertebrate evolution. Nat. Genet. 45, 415–421, 421e1–421e2. doi: 10.1038/ng.2568
Svensson, E., Kim, O., and Parker, D. (2013). Altered GABA and somatostatin modulation of proprioceptive feedback after spinal cord injury in lamprey. Neuroscience 235, 109–118. doi: 10.1016/j.neuroscience.2013.01.017
Tabor, R., Yaksi, E., and Friedrich, R. W. (2008). Multiple functions of GABAA and GABAB receptors during pattern processing in the zebrafish olfactory bulb. Eur. J. Neurosci. 28, 117–127. doi: 10.1111/j.1460-9568.2008.06316.x
Tamura, K., Stecher, G., Peterson, D., Filipski, A., and Kumar, S. (2013). MEGA6: molecular evolutionary genetics analysis version 6.0. Mol. Biol. Evol. 30, 2725–2729. doi: 10.1093/molbev/mst197
Taylor, J. S., Van de Peer, Y., Braasch, I., and Meyer, A. (2001). Comparative genomics provides evidence for an ancient genome duplication event in fish. Philos. Trans. R. Soc. Lond. B Biol. Sci. 356, 1661–1679. doi: 10.1098/rstb.2001.0975
Tegnér, J., Matsushima, T., el Manira, A., and Grillner, S. (1993). The spinal GABA system modulates burst frequency and intersegmental coordination in the lamprey: differential effects of GABAA and GABAB receptors. J. Neurophysiol. 69, 647–657.
Valle-Maroto, S. M., Fernández-López, B., Villar-Cerviño, V., Barreiro-Iglesias, A., Anadón, R., and Rodicio, M. C. (2011). Inhibitory descending rhombencephalic projections in larval sea lamprey. Neuroscience 194, 1–10. doi: 10.1016/j.neuroscience.2011.08.017
Veenman, C. L., Albin, R. L., Richfield, E. K., and Reiner, A. (1994). Distributions of GABAA, GABAB and benzodiazepine receptors in the forebrain and midbrain of pigeons. J. Comp. Neurol. 344, 161–189. doi: 10.1002/cne.903440202
Vígh, B., Manzano e Silva, M. J., Frank, C. L., Vincze, C., Czirok, S. J., Szabó, A., et al. (2004). The system of cerebrospinal fluid-contacting neurons. Its supposed role in the nonsynaptic signal transmission of the brain. Histol. Histopathol. 19, 607–628.
Villar-Cerviño, V., Barreiro-Iglesias, A., Anadón, R., and Rodicio, M. C. (2008). Distribution of glycine immunoreactivity in the brain of adult sea lamprey (Petromyzon marinus). Comparison with γ-aminobutyric acid. J. Comp. Neurol. 507, 1441–1463. doi: 10.1002/cne.21695
Villar-Cerviño, V., Barreiro-Iglesias, A., Fernández-López, B., Mazan, S., Rodicio, M. C., and Anadón, R. (2013). Glutamatergic neuronal populations in the brainstem of the sea lamprey, Petromyzon marinus: an in situ hybridization and immunocytochemical study. J. Comp. Neurol. 521, 522–557. doi: 10.1002/cne.23189
Villar-Cerviño, V., Barreiro-Iglesias, A., Rodicio, M. C., and Anadón, R. (2010). D-serine is distributed in neurons in the brain of the sea lamprey. J. Comp. Neurol. 518, 1688–1710. doi: 10.1002/cne.22296
Villar-Cerviño, V., Fernández-López, B., Rodicio, M. C., and Anadón, R. (2014). Aspartate-containing neurons of the brainstem and rostral spinal cord of the sea lamprey Petromyzon marinus: distribution and comparison with γ-aminobutyric acid. J. Comp. Neurol. 522, 1209–1231. doi: 10.1002/cne.23493
Villemure, J. F., Adam, L., Bevan, N. J., Gearing, K., Chénier, S., and Bouvier, M. (2005). Subcellular distribution of GABAB receptor homo- and hetero-dimers. Biochem. J. 388, 47–55. doi: 10.1042/bj20041435
Vinay, L., Bongianni, F., Ohta, Y., Grillner, S., and Dubuc, R. (1998). Spinal inputs from lateral columns to reticulospinal neurons in lampreys. Brain Res. 808, 279–293. doi: 10.1016/s0006-8993(98)00835-x
Keywords: GABA, GABAB1, GABAB2, agnathans, receptor heterodimerization
Citation: Romaus-Sanjurjo D, Fernández-López B, Sobrido-Cameán D, Barreiro-Iglesias A and Rodicio MC (2016) Cloning of the GABAB Receptor Subunits B1 and B2 and their Expression in the Central Nervous System of the Adult Sea Lamprey. Front. Neuroanat. 10:118. doi: 10.3389/fnana.2016.00118
Received: 12 August 2016; Accepted: 22 November 2016;
Published: 08 December 2016.
Edited by:
Agustín González, Complutense University of Madrid, SpainReviewed by:
David Parker, University of Cambridge, UKCopyright © 2016 Romaus-Sanjurjo, Fernández-López, Sobrido-Cameán, Barreiro-Iglesias and Rodicio. This is an open-access article distributed under the terms of the Creative Commons Attribution License (CC BY). The use, distribution and reproduction in other forums is permitted, provided the original author(s) or licensor are credited and that the original publication in this journal is cited, in accordance with accepted academic practice. No use, distribution or reproduction is permitted which does not comply with these terms.
*Correspondence: Antón Barreiro-Iglesias, YW50b24uYmFycmVpcm9AdXNjLmVz
María Celina Rodicio, bWNlbGluYS5yb2RpY2lvQHVzYy5lcw==
Disclaimer: All claims expressed in this article are solely those of the authors and do not necessarily represent those of their affiliated organizations, or those of the publisher, the editors and the reviewers. Any product that may be evaluated in this article or claim that may be made by its manufacturer is not guaranteed or endorsed by the publisher.
Research integrity at Frontiers
Learn more about the work of our research integrity team to safeguard the quality of each article we publish.