- 1Inserm, U1216, Univ. Grenoble Alpes, Grenoble Institut Neurosciences, Grenoble, France
- 2Department of Psychological and Brain Sciences, Krieger School of Arts and Sciences, Johns Hopkins University, Baltimore, MD, United States
Introduction
The zona incerta (ZI), a small and historically overlooked structure located beneath the thalamus, has been increasingly recognized for its role in diverse behavioral processes. Early studies in the 1970s and 1980s explored its role in ingestive behaviors, including drinking and feeding (for review, see Mitrofanis, 2005) (Figure 1A), suggesting its involvement in motivation and survival mechanisms related to food and water intake. The ZI is now known to be a heterogeneous nucleus, divided into four major sectors: rostral (ZIr), dorsal (ZId), ventral (ZIv), and caudal (ZIc) (Mitrofanis, 2005), each with distinct neurochemical profiles. GABAergic cells, which constitute the majority of the ZI's neurons, and parvalbumin positive neurons are mostly concentrated in the ZIv, while the ZId is rich in glutamatergic cells, and the ZIr contains dopaminergic neurons (Mitrofanis, 2005).
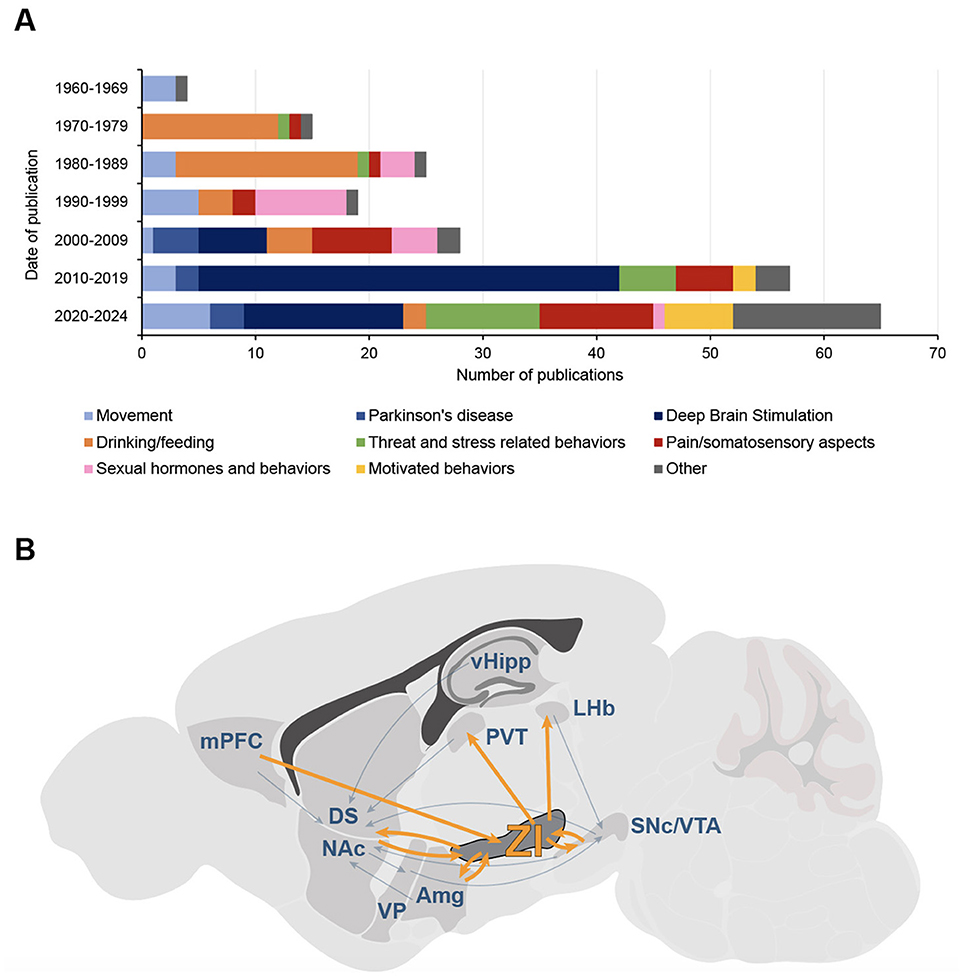
Figure 1. The Zona Incerta interest's evolution, from motor function to addiction? (A) Evolution of functions and topics studied in publications on the zona incerta (ZI) from 1965 to 2024. Data were obtained from a bibliometric analysis of scientific literature on PubMed, including only articles with “zona incerta” in the title; studies primarily focused on tracing, anatomy, cytoarchitecture, or neurodevelopment were excluded, even if they referenced functions (n = 213 articles). Bars represent the number of publications per decade, divided into main functional and thematic categories: motor functions (light blue), Parkinson's disease (PD; medium blue), deep-brain stimulation (DBS; dark blue), drinking and feeding behaviors (orange), threat and stress-related behaviors (green), pain and somatosensory functions (red), sexual hormones and behaviors (pink), motivated and appetitive behaviors (yellow), other functions (gray). While early research emphasized non-motor functions of the ZI, particularly ingestive behaviors, the focus started to shift to motor-related roles in the 1990s with studies on PD, and even more in the 2010s with a tremendous increase of interest on DBS; in the last years, there has been a resurgence of interest in non-motor functions, including motivated behaviors. (B) Position of the zona incerta in the canonical circuits for addiction. The main structures and circuits mediating positive and negative reinforcement in addiction are represented; simplified and adapted from Lüscher and Janak (2021). The zona incerta shares connections with most of these structures, positioning it as a critical node for different aspects of addiction. Amg, amygdala; DS, dorsal striatum; NAc, nucleus accumbens; LHb, lateral habenula; mPFC, medial prefrontal cortex; PVT, paraventricular nucleus of thalamus; SNc, substantia nigra pars compacta; vHipp, ventral hippocampus; VP, ventral pallidum; VTA, ventral tegmental area; ZI, zona incerta.
While motor functions of the ZI were identified as early as the 60s (Hyde and Toczek, 1962), the focus really shifted on those motor-related roles in the 1990s, as the ZI became studied in the context of Parkinson's disease (PD) (Shi et al., 2024). The introduction of deep brain stimulation (DBS) as a treatment for PD patients, with the subthalamic nucleus (STN) as a primary target (Benabid et al., 2009), revealed that stimulating the nearby ZI also produced significant improvements in motor symptoms (Voges et al., 2002; Ossowska, 2020). This led to a renewed interest for the ZI, placing it in the spotlight for movement disorder therapies. Consequently, its non-motor functions, particularly the potential reward processes driving the ingestive behaviors previously characterized, received less attention. However, reports of non-motor effects following ZI stimulation in PD patients—especially on mood (Stefurak et al., 2003; Tommasi et al., 2008), or emotion (Burrows et al., 2012)—suggested a broader role for the ZI. Notably, motivational alterations such as apathy (Czernecki, 2005; Ricciardi et al., 2014) drew attention to its potential role in motivational processes.
Today, there is a renewed interest in the link between ZI and behaviors related to reward and motivation. The contemporary approaches to map, monitor and manipulate neural circuits are improving our understanding of the contribution of the different subregions and neuronal populations constituting the ZI to its various functions. Notably, the ZI shares remarkable similarities with the substantia nigra pars compacta (SNc) and the ventral tegmental area (VTA), particularly in terms of neuronal populations and their specific involvements in behavior (Mitrofanis, 2005; Morales and Margolis, 2017). While the VTA, and more recently the SNc, are intensively investigated in the context of motivation-related pathologies, especially addiction, this aspect of the ZI remains largely unexamined. Addiction is a chronic psychiatric disorder defined by a loss of control over specific behaviors such as drug intake, despite negative consequences. It involves recurrent periods of drug seeking, withdrawal and relapse, leading to a spiraling addiction cycle (Koob and Le Moal, 2008; Koob and Volkow, 2010). Recent findings on the ZI raise new questions about its potential involvement in addiction related behaviors. This short essay examines current evidence suggesting the ZI's potential role in psychiatric disorders akin to drug addiction and highlights future research directions.
ZI activity is modulated by drugs of abuse
While the role of ZI in addiction remains underexplored, early pharmacological studies support its responsiveness to drugs of abuse. In situ alcohol injection into the ZI elicits strong neuronal responses (Wayner et al., 1975), and injections of tetrahydropapaveroline, previously used to initiate alcohol consumption in rats when injected into the mesocorticolimbic pathway, also induces alcohol preference and consumption when injected into the ZI (Myers and Privette, 1989). Cocaine intake impacts the ZI as well, increasing Fos expression (Zahm et al., 2010) and reducing neurotensin binding, paralleling effects in mesocorticolimbic pathways (Pilotte et al., 1991). Furthermore, morphine induces tyrosine hydroxylase expression and enhances dopamine (DA) metabolism in the ZI, implicating it in dopaminergic modulation (Molnar et al., 1994). These findings indicate that drugs of abuse directly impact ZI activity, supporting its relevance in addiction research.
ZI dopaminergic neurons mediate motivation
The ZI shares critical features with the VTA and the SNc. A first notable parallel between the three structures is the presence of dopaminergic neurons. Dopaminergic transmission from the VTA and the SNc is instrumental for motivational processes and at the core of addiction disorders. All drugs of abuse trigger DA release in the striatum, particularly in the nucleus accumbens (NAc) (Di Chiara and Imperato, 1988), signaling positive and negative values and valence of biologically relevant stimuli (Bromberg-Martin et al., 2010; Schultz, 1998), but also encoding reward prediction errors (RPE) to guide behavior and learning (Hollerman and Schultz, 1998; Schultz, 1998), making mesolimbic and nigrostriatal DA fundamental for motivated behaviors (Berke, 2018). Alterations in these pathways contribute to drug addiction-like behaviors, from the drug withdrawal symptoms to excessive drug seeking and taking (Keiflin and Janak, 2015; Goutaudier et al., 2023; Giuliano et al., 2019; Koob and Le Moal, 1997). Very few studies have focused on the dopaminergic neurons of the ZI (ZIDA), but recent findings suggest a pivotal role for motivation (Ye et al., 2023), as VTA and SNc dopaminergic neurons.
Specifically, ZIDA are overactive during energy deficit, promoting motivation for food-seeking and maintaining food-seeking behavior, partly through projections to the paraventrical nucleus of the thalamus (PVT) (Ye et al., 2023). Moreover, ZIDA also seem to be involved in other appetitive behaviors such as self grooming (Jiang et al., 2024). Increased grooming has been observed during abstinence, reflecting sociability impairments in drug abuse models (Lalanne et al., 2017; Homberg et al., 2002) or as a stereotypical behavior induced by psychostimulants and exacerbated during drug-sensitization (Kalueff et al., 2016). Excessive grooming is also a marker of compulsivity in rodent models of obsessive-compulsive disorders (Mondragón-González et al., 2024). This is consistent with recent connectivity data from both human and non-human primates that have highlighted the ZI as a potential target for treating obsessive-compulsive disorders (ICDs) (Haber et al., 2023).
ZI GABA neurons activation favor appetitive behavior and novelty seeking
In addition to their well-studied dopaminergic neurons, the VTA and SNc also contain substantial GABAergic populations, another feature in common with the ZI. Similar to VTA GABAergic neurons (Zhou et al., 2022; Lowes et al., 2021), GABAergic neurons of the ZI (ZIGABA) regulate reward motivation and appetitive behaviors, and project to the NAc (Wang et al., 2020). Their optogenetic stimulation has been shown to provoke binge-like eating in mice, while their ablation decreases food intake in the long-term, with a reduction of body weight as a result (Zhang and Van Den Pol, 2017). Other types of motivated behaviors have been shown to involve ZIGABA, for example hunting behavior (Zhao et al., 2019), but also novelty seeking (Monosov et al., 2022; Ogasawara et al., 2022), a trait of particular interest in the field of drug addiction, as it is an endophenotype of vulnerability, predicting the transition from controlled to compulsive cocaine use (Belin and Deroche-Gamonet, 2012). Optogenetic activation of ZIGABA has been shown to favor exploration of new objects and conspecifics (Ahmadlou et al., 2021). As NAc-projecting VTA GABAergic neurons undergo significant activity changes and synaptic plasticity following drug exposure (Friend et al., 2021; Williams et al., 2018), and contribute to drug motivation (Ting-A-Kee et al., 2013; Elum et al., 2024; Merkel et al., 2024), one can hypothesize that neuroadaptations within the ZIGABA-NAc circuit may similarly underlie various aspects of drug-seeking behavior, notably through disinhibition and exploratory behavior toward novel elements in the environment.
The ZI regulates negative affect
Collectively, the recent studies exposed above highlight the role of the ZI in motivated behaviors, and point out a potential contribution for the positive reinforcement that drives the early phases of the addiction cycle and eventually leads to compulsive drug seeking and taking (Koob and Le Moal, 1997). The later phases of this cycle are marked by periods of abstinence during which individuals experience dysphoria, apathy, anxiety, depression and pain. These withdrawal symptoms likely contribute to craving, compulsive drug use and relapse, through a negative reinforcement process whereby drug is sought and consumed to alleviate the negative affect experienced (Koob and Le Moal, 1997; Robbins et al., 2024). Beyond its role in motivation, the ZI has been shown to be involved in behaviors reminiscent of this negative state, as anxiety (Li et al., 2021), and pain (Li et al., 2023; Lu et al., 2021). Moreover, the ZI is also implicated in aversive learning (Zhou et al., 2021), partly through connections between ZIDA and the amygdala (Zhang et al., 2022), and through thalamic and periaqueductal gray modulation by ZIGABA (Chou et al., 2018; Venkataraman et al., 2021). Thus, the multifaceted functions of the ZI posit it as a potential significant contributor to both positive and negative reinforcement in addiction.
Integrating the ZI in canonical circuits of addiction
Taken together, all these findings indicate that the ZI may contribute to various aspects of addiction, from withdrawal-related negative symptoms to compulsive drug-seeking, a role that may become increasingly evident when positioning the ZI within the canonical addiction circuit (Lüscher and Janak, 2021) (Figure 1B). In addition to its structural similarities with the VTA and SNc, the ZI shares connections to these two major structures of addiction circuits. It is bidirectionally connected to the VTA and, to a lesser extent, the SNc and projects to the NAc, making it ideally situated to modulate dopaminergic transmission between the midbrain and the striatum (Arena et al., 2024). As mentioned above, the ZI also projects to the PVT (Ye et al., 2023), a key player in motivational circuits that receives inputs from the prefrontal cortex and lateral hypothalamus (Iglesias and Flagel, 2021). The PVT projects to the NAc and has been implicated in drug addiction, driving drug-seeking behavior and withdrawal symptoms (Zhou and Zhu, 2019). While the VTA and SNc do not provide dopaminergic input to the PVT (Li et al., 2014), the ZI could play this modulatory role, linking homeostatic and reward-driven processes, and modulating the activity of the NAc both directly and indirectly. This aligns with the observed decrease in striatal DA metabolism after ZI electrolytic lesion in rats (Walker et al., 2010). Finally, the ZI is connected to several other nuclei involved in positive and negative reinforcement circuits for addiction, including the prefrontal cortex, amygdala, and lateral habenula (Arena et al., 2024) (Figure 1B). Thus, the ZI is strategically positioned to modulate numerous canonical circuits implicated in addiction processes.
Discussion
Overall, while clear evidence is still emerging, the ZI's connectivity with addiction-related circuits, including dopaminergic pathways, and its role in motivation, emotion, and interoception, provide strong theoretical support for its role in addiction. The presence of ZIDA potentially recapitulating to a certain extent some feature of VTA/SNc dopaminergic neurons is obviously a strong argument underpinning this opinion (Jiang et al., 2024). However, the precise involvement of ZIDA is discussed, as others found no alteration of motivated behaviors when manipulating this cell population, and highlighted a role limited to prehensile movement (Garau et al., 2023). This is in stark contrast with the place preference induced by ZIDA optogenetic activation (Jiang et al., 2024), for instance. Furthermore, while the involvement of ZIGABA in motivation seems to be more widely accepted, the clear distinction between ZI GABA and DA transmission must however be put into perspective, as it seems that ZIDA are not purely dopaminergic but also co-express GABA neurotransmission markers (Negishi et al., 2020). Beyond these considerations, several key questions remain unanswered: Does drug intake induce DA release from the ZI? Does ZIDA encode RPE as well? Do neuronal adaptations in the ZI drive drug intake, escalation, withdrawal symptoms, or compulsive seeking? Investigating these questions could clarify the ZI's role in addiction and its potential therapeutic relevance.
The ZI's potential role at the intersection between PD and drug addiction offers intriguing perspectives, not only for understanding its broader involvement in motor and psychiatric disorders, but also for its clinical relevance. ICDs, such as gambling, hypersexuality, or compulsive eating, are prevalent in PD (Houeto et al., 2016; Leclercq and Corvol, 2024), and resume most of the psychobiological mechanisms observed in drug addiction (Vassileva and Conrod, 2019). They are largely attributed to DA replacement therapy (Houeto et al., 2016), but their pathological underpinnings are still not fully characterized (Prange and Thobois, 2024), and might also involve ZIDA. Interestingly, L-DOPA enhances ZI neuronal activity in parkinsonian rats (Cole et al., 1993; Bastide et al., 2014), supporting this hypothesis.
ICDs are globally well alleviated by STN-DBS in patients with PD (Scherrer et al., 2020). Given the ZI's anatomical proximity to the STN and reports of compulsive behavior suppression following ZI-DBS (Mallet et al., 2002), this therapeutic benefit might partly involve modulation of ZI activity, particularly ZIDA. As DBS is increasingly applied to neurological disorders, including addiction (Vorspan et al., 2023; Pelloux and Baunez, 2013), and considering the clinical similarities between ICDs and drug addiction (Potenza, 2014), ZI-DBS may emerge as a potential therapeutic avenue for addictions. However, behavioral side effects associated with ZI-DBS (Ossowska, 2020) point out the need for major investigations to delineate ZI sectors and circuits potentially implicated in addiction and optimize therapeutic strategies.
Author contributions
MW: Conceptualization, Writing – original draft, Writing – review & editing. RM: Writing – original draft, Writing – review & editing. SC: Conceptualization, Writing – review & editing, Validation. YV: Conceptualization, Funding acquisition, Methodology, Supervision, Validation, Writing – original draft, Writing – review & editing.
Funding
The author(s) declare financial support was received for the research, authorship, and/or publication of this article. The processing charges are paid with the IReSP INCa grant # RPT23018CCA.
Acknowledgments
We thank Sabrina Boulet for constructive and artistic comments on the manuscript.
Conflict of interest
The authors declare that the research was conducted in the absence of any commercial or financial relationships that could be construed as a potential conflict of interest.
Generative AI statement
The author(s) declare that no Gen AI was used in the creation of this manuscript.
Publisher's note
All claims expressed in this article are solely those of the authors and do not necessarily represent those of their affiliated organizations, or those of the publisher, the editors and the reviewers. Any product that may be evaluated in this article, or claim that may be made by its manufacturer, is not guaranteed or endorsed by the publisher.
References
Ahmadlou, M., Houba, J. H. W., van Vierbergen, J. F. M., Giannouli, M., Gimenez, G.-A., van Weeghel, C., et al. (2021). A cell type–specific cortico-subcortical brain circuit for investigatory and novelty-seeking behavior. Science 372:eabe9681. doi: 10.1126/science.abe9681
Arena, G., Londei, F., Ceccarelli, F., Ferrucci, L., Borra, E., and Genovesio, A. (2024). Disentangling the identity of the zona incerta: a review of the known connections and latest implications. Ageing Res. Rev. 93:102140. doi: 10.1016/j.arr.2023.102140
Bastide, M. F., Dovero, S., Charron, G., Porras, G., Gross, C. E., Fernagut, P.-O., et al. (2014). Immediate-early gene expression in structures outside the basal ganglia is associated to l-DOPA-induced dyskinesia. Neurobiol. Dis. 62, 179–192. doi: 10.1016/j.nbd.2013.09.020
Belin, D., and Deroche-Gamonet, V. (2012). Responses to novelty and vulnerability to cocaine addiction: contribution of a multi-symptomatic animal model. Cold Spring Harb. Perspect. Med. 2:a011940. doi: 10.1101/cshperspect.a011940
Benabid, A. L., Chabardes, S., Mitrofanis, J., and Pollak, P. (2009). Deep brain stimulation of the subthalamic nucleus for the treatment of Parkinson's disease. Lancet Neurol. 8, 67–81. doi: 10.1016/S1474-4422(08)70291-6
Berke, J. D. (2018). What does dopamine mean? Nat. Neurosci. 21, 787–793. doi: 10.1038/s41593-018-0152-y
Bromberg-Martin, E. S., Matsumoto, M., and Hikosaka, O. (2010). Dopamine in motivational control: rewarding, aversive, and alerting. Neuron 68, 815–834. doi: 10.1016/j.neuron.2010.11.022
Burrows, A. M., Ravin, P. D., Novak, P., Peters, M. L. B., Dessureau, B., Swearer, J., et al. (2012). Limbic and motor function comparison of deep brain stimulation of the zona incerta and subthalamic nucleus. Neurosurgery 70, 125–130; discussion 130–131. doi: 10.1227/NEU.0b013e318232fdac
Chou, X.-L., Wang, X., Zhang, Z.-G., Shen, L., Zingg, B., Huang, J., et al. (2018). Inhibitory gain modulation of defense behaviors by zona incerta. Nat. Commun. 9:1151. doi: 10.1038/s41467-018-03581-6
Cole, D. G., Growdon, J. H., and DiFiglia, M. (1993). Levodopa induction of Fos immunoreactivity in rat brain following partial and complete lesions of the substantia nigra. Exp. Neurol. 120, 223–232. doi: 10.1006/exnr.1993.1057
Czernecki, V. (2005). Does bilateral stimulation of the subthalamic nucleus aggravate apathy in Parkinson's disease? J. Neurol. Neurosurg. Psychiatry 76, 775–779. doi: 10.1136/jnnp.2003.033258
Di Chiara, G., and Imperato, A. (1988). Drugs abused by humans preferentially increase synaptic dopamine concentrations in the mesolimbic system of freely moving rats. Proc. Natl. Acad. Sci. USA. 85, 5274–5278. doi: 10.1073/pnas.85.14.5274
Elum, J. E., Szelenyi, E. R., Juarez, B., Murry, A. D., Loginov, G., Zamorano, C. A., et al. (2024). Distinct dynamics and intrinsic properties in ventral tegmental area populations mediate reward association and motivation. Cell Rep. 43:114668. doi: 10.1016/j.celrep.2024.114668
Friend, L. N., Wu, B., and Edwards, J. G. (2021). Acute cocaine exposure occludes long-term depression in ventral tegmental area GABA neurons. Neurochem. Int. 145:105002. doi: 10.1016/j.neuint.2021.105002
Garau, C., Hayes, J., Chiacchierini, G., McCutcheon, J. E., and Apergis-Schoute, J. (2023). Involvement of A13 dopaminergic neurons in prehensile movements but not reward in the rat. Curr. Biol. 33, 4786–4797.e4. doi: 10.1016/j.cub.2023.09.044
Giuliano, C., Belin, D., and Everitt, B. J. (2019). Compulsive alcohol seeking results from a failure to disengage dorsolateral striatal control over behavior. J. Neurosci. 39, 1744–1754. doi: 10.1523/JNEUROSCI.2615-18.2018
Goutaudier, R., Joly, F., Mallet, D., Bartolomucci, M., Guicherd, D., Carcenac, C., et al. (2023). Hypodopaminergic state of the nigrostriatal pathway drives compulsive alcohol use. Mol. Psychiatry 28, 463–474. doi: 10.1038/s41380-022-01848-5
Haber, S. N., Lehman, J., Maffei, C., and Yendiki, A. (2023). The rostral zona incerta: a subcortical integrative hub and potential deep brain stimulation target for obsessive-compulsive disorder. Biol. Psychiatry 93, 1010–1022. doi: 10.1016/j.biopsych.2023.01.006
Hollerman, J. R., and Schultz, W. (1998). Dopamine neurons report an error in the temporal prediction of reward during learning. Nat. Neurosci. 1, 304–309. doi: 10.1038/1124
Homberg, J. R., van den Akker, M., Raasø, H. S., Wardeh, G., Binnekade, R., Schoffelmeer, A. N. M., et al. (2002). Enhanced motivation to self-administer cocaine is predicted by self-grooming behaviour and relates to dopamine release in the rat medial prefrontal cortex and amygdala. Eur. J. Neurosci. 15, 1542–1550. doi: 10.1046/j.1460-9568.2002.01976.x
Houeto, J.-L., Magnard, R., Dalley, J. W., Belin, D., and Carnicella, S. (2016). Trait impulsivity and anhedonia: two gateways for the development of impulse control disorders in Parkinson's disease? Front. Psychiatry 7:91. doi: 10.3389/fpsyt.2016.00091
Hyde, J. E., and Toczek, S. (1962). Functional relation of interstitial nucleus to rotatory movements evoked from zona incerta stimulation. J. Neurophysiol. 25, 455–466. doi: 10.1152/jn.1962.25.4.455
Iglesias, A. G., and Flagel, S. B. (2021). The paraventricular thalamus as a critical node of motivated behavior via the hypothalamic-thalamic-striatal circuit. Front. Integr. Neurosci. 15:706713. doi: 10.3389/fnint.2021.706713
Jiang, Z., He, M., Young, C., Cai, J., Xu, Y., Jiang, Y., et al. (2024). Dopaminergic neurons in zona incerta drives appetitive self-grooming. Adv. Sci. 11:2308974. doi: 10.1002/advs.202308974
Kalueff, A. V., Stewart, A. M., Song, C., Berridge, K. C., Graybiel, A. M., and Fentress, J. C. (2016). Neurobiology of rodent self-grooming and its value for translational neuroscience. Nat. Rev. Neurosci. 17, 45–59. doi: 10.1038/nrn.2015.8
Keiflin, R., and Janak, P. H. (2015). Dopamine prediction errors in reward learning and addiction: from theory to neural circuitry. Neuron 88, 247–263. doi: 10.1016/j.neuron.2015.08.037
Koob, G. F., and Le Moal, M. (1997). Drug abuse: hedonic homeostatic dysregulation. Science 278, 52–58. doi: 10.1126/science.278.5335.52
Koob, G. F., and Le Moal, M. (2008). Addiction and the brain antireward system. Annu. Rev. Psychol. 59, 29–53. doi: 10.1146/annurev.psych.59.103006.093548
Koob, G. F., and Volkow, N. D. (2010). Neurocircuitry of addiction. Neuropsychopharmacology 35, 217–238. doi: 10.1038/npp.2009.110
Lalanne, L., Ayranci, G., Filliol, D., Gavériaux-Ruff, C., Befort, K., Kieffer, B. L., et al. (2017). Kappa opioid receptor antagonism and chronic antidepressant treatment have beneficial activities on social interactions and grooming deficits during heroin abstinence. Addict. Biol. 22, 1010–1021. doi: 10.1111/adb.12392
Leclercq, V., and Corvol, J.-C. (2024). Impulse control disorder: review on clinical, pharmacologic, and genetic risk factors. Rev. Neurol. 180, 1071–1077. doi: 10.1016/j.neurol.2024.07.001
Li, J., Peng, S., Zhang, Y., Ge, J., Gao, S., Zhu, Y., et al. (2023). Glutamatergic neurons in the zona incerta modulate pain and itch behaviors in mice. Mol. Neurobiol. 60, 5866–5877. doi: 10.1007/s12035-023-03431-7
Li, S., Shi, Y., and Kirouac, G. J. (2014). The hypothalamus and periaqueductal gray are the sources of dopamine fibers in the paraventricular nucleus of the thalamus in the rat. Front. Neuroanat. 8:136. doi: 10.3389/fnana.2014.00136
Li, Z., Rizzi, G., and Tan, K. R. (2021). Zona incerta subpopulations differentially encode and modulate anxiety. Sci. Adv. 7:eabf6709. doi: 10.1126/sciadv.abf6709
Lowes, D. C., Chamberlin, L. A., Kretsge, L. N., Holt, E. S., Abbas, A. I., Park, A. J., et al. (2021). Ventral tegmental area GABA neurons mediate stress-induced blunted reward-seeking in mice. Nat. Commun. 12:3539. doi: 10.1038/s41467-021-23906-2
Lu, C. W., Harper, D. E., Askari, A., Willsey, M. S., Vu, P. P., Schrepf, A. D., et al. (2021). Stimulation of zona incerta selectively modulates pain in humans. Sci. Rep. 11:8924. doi: 10.1038/s41598-021-87873-w
Lüscher, C., and Janak, P. H. (2021). Consolidating the circuit model for addiction. Annu. Rev. Neurosci. 44, 173–195. doi: 10.1146/annurev-neuro-092920-123905
Mallet, L., Mesnage, V., Houeto, J.-L., Pelissolo, A., Yelnik, J., Behar, C., et al. (2002). Compulsions, Parkinson's disease, and stimulation. Lancet Lond. Engl. 360, 1302–1304. doi: 10.1016/S0140-6736(02)11339-0
Merkel, R., Hernandez, N., Weir, V., Zhang, Y., Rich, M. T., Crist, R. C., et al. (2024). An endogenous GLP-1 circuit engages VTA GABA neurons to regulate mesolimbic dopamine neurons and attenuate cocaine seeking. bioRxiv [Preprint]. doi: 10.1101/2024.06.20.599574
Mitrofanis, J. (2005). Some certainty for the “zone of uncertainty”? Exploring the function of the zona incerta. Neuroscience 130, 1–15. doi: 10.1016/j.neuroscience.2004.08.017
Molnar, J., He, J. R., and Barraclough, C. A. (1994). Effect of morphine on hypothalamic tyrosine hydroxylase mRNA levels in dopaminergic neurons and on preoptic DOPAC levels measured by microdialysis. Brain Res. Mol. Brain Res. 22, 97–106. doi: 10.1016/0169-328x(94)90036-1
Mondragón-González, S. L., Schreiweis, C., and Burguière, E. (2024). Closed-loop recruitment of striatal interneurons prevents compulsive-like grooming behaviors. Nat. Neurosci. 27, 1148–1156. doi: 10.1038/s41593-024-01633-3
Monosov, I. E., Ogasawara, T., Haber, S. N., Heimel, J. A., and Ahmadlou, M. (2022). The zona incerta in control of novelty seeking and investigation across species. Curr. Opin. Neurobiol. 77:102650. doi: 10.1016/j.conb.2022.102650
Morales, M., and Margolis, E. B. (2017). Ventral tegmental area: cellular heterogeneity, connectivity and behaviour. Nat. Rev. Neurosci. 18, 73–85. doi: 10.1038/nrn.2016.165
Myers, R. D., and Privette, T. H. (1989). A neuroanatomical substrate for alcohol drinking: identification of tetrahydropapaveroline (THP)-reactive sites in the rat brain. Brain Res. Bull. 22, 899–911. doi: 10.1016/0361-9230(89)90035-x
Negishi, K., Payant, M. A., Schumacker, K. S., Wittmann, G., Butler, R. M., Lechan, R. M., et al. (2020). Distributions of hypothalamic neuron populations co-expressing tyrosine hydroxylase and the vesicular GABA transporter in the mouse. J. Comp. Neurol. 528, 1833–1855. doi: 10.1002/cne.24857
Ogasawara, T., Sogukpinar, F., Zhang, K., Feng, Y.-Y., Pai, J., Jezzini, A., et al. (2022). A primate temporal cortex-zona incerta pathway for novelty seeking. Nat. Neurosci. 25, 50–60. doi: 10.1038/s41593-021-00950-1
Ossowska, K. (2020). Zona incerta as a therapeutic target in Parkinson's disease. J. Neurol. 267, 591–606. doi: 10.1007/s00415-019-09486-8
Pelloux, Y., and Baunez, C. (2013). Deep brain stimulation for addiction: why the subthalamic nucleus should be favored. Curr. Opin. Neurobiol. 23, 713–720. doi: 10.1016/j.conb.2013.02.016
Pilotte, N. S., Mitchell, W. M., Sharpe, L. G., De Souza, E. B., and Dax, E. M. (1991). Chronic cocaine administration and withdrawal of cocaine modify neurotensin binding in rat brain. Synap. N. Y. N 9, 111–120. doi: 10.1002/syn.890090205
Potenza, M. N. (2014). Non-substance addictive behaviors in the context of DSM-5. Addict. Behav. 39, 1–2. doi: 10.1016/j.addbeh.2013.09.004
Prange, S., and Thobois, S. (2024). Imaging of impulse control disorders in Parkinson's disease. Rev. Neurol. 180, 1078–1086. doi: 10.1016/j.neurol.2024.09.004
Ricciardi, L., Morgante, L., Epifanio, A., Zibetti, M., Lanotte, M., Lopiano, L., et al. (2014). Stimulation of the subthalamic area modulating movement and behavior. Parkinsonism Relat. Disord. 20, 1298–1300. doi: 10.1016/j.parkreldis.2014.07.013
Robbins, T. W., Banca, P., and Belin, D. (2024). From compulsivity to compulsion: the neural basis of compulsive disorders. Nat. Rev. Neurosci. 25, 313–333. doi: 10.1038/s41583-024-00807-z
Scherrer, S., Smith, A. H., Gowatsky, J., Palmese, C. A., Jimenez-Shahed, J., Kopell, B. H., et al. (2020). Impulsivity and compulsivity after subthalamic deep brain stimulation for Parkinson's disease. Front. Behav. Neurosci. 14:47. doi: 10.3389/fnbeh.2020.00047
Schultz, W. (1998). Predictive reward signal of dopamine neurons. J. Neurophysiol. 80, 1–27. doi: 10.1152/jn.1998.80.1.1
Shi, Y., Zhang, J., Xiu, M., Xie, R., Liu, Y., Xie, J., et al. (2024). The zona incerta system: involvement in Parkinson's disease. Exp. Neurol. 382:114992. doi: 10.1016/j.expneurol.2024.114992
Stefurak, T., Mikulis, D., Mayberg, H., Lang, A. E., Hevenor, S., Pahapill, P., et al. (2003). Deep brain stimulation for Parkinson's disease dissociates mood and motor circuits: a functional MRI case study. Mov. Disord. 18, 1508–1516. doi: 10.1002/mds.10593
Ting-A-Kee, R., Vargas-Perez, H., Mabey, J. K., Shin, S. I., Steffensen, S. C., and van der Kooy, D. (2013). Ventral tegmental area GABA neurons and opiate motivation. Psychopharmacology 227, 697–709. doi: 10.1007/s00213-013-3002-3
Tommasi, G., Lanotte, M., Albert, U., Zibetti, M., Castelli, L., Maina, G., et al. (2008). Transient acute depressive state induced by subthalamic region stimulation. J. Neurol. Sci. 273, 135–138. doi: 10.1016/j.jns.2008.06.012
Vassileva, J., and Conrod, P. J. (2019). Impulsivities and addictions: a multidimensional integrative framework informing assessment and interventions for substance use disorders. Philos. Trans. R. Soc. Lond. B. Biol. Sci. 374:20180137. doi: 10.1098/rstb.2018.0137
Venkataraman, A., Hunter, S. C., Dhinojwala, M., Ghebrezadik, D., Guo, J., Inoue, K., et al. (2021). Incerto-thalamic modulation of fear via GABA and dopamine. Neuropsychopharmacology 46, 1658–1668. doi: 10.1038/s41386-021-01006-5
Voges, J., Volkmann, J., Allert, N., Lehrke, R., Koulousakis, A., Freund, H.-J., et al. (2002). Bilateral high-frequency stimulation in the subthalamic nucleus for the treatment of Parkinson disease: correlation of therapeutic effect with anatomical electrode position. J. Neurosurg. 96, 269–279. doi: 10.3171/jns.2002.96.2.0269
Vorspan, F., Domenech, P., Grabli, D., Yelnik, J., Delavest, M., Dauré, C., et al. (2023). A single case report of STN-DBS for severe crack-cocaine dependence: double-blind ON vs. SHAM randomized controlled assessment. Front. Psychiatry 14:1146492. doi: 10.3389/fpsyt.2023.1146492
Walker, R. H., Davies, G., Koch, R. J., Haack, A. K., Moore, C., and Meshul, C. K. (2010). Effects of zona incerta lesions on striatal neurochemistry and behavioral asymmetry in 6-hydroxydopamine-lesioned rats. J. Neurosci. Res. 88, 2964–2975. doi: 10.1002/jnr.22446
Wang, Q., Zhang, X., Leng, H., Luan, X., Guo, F., Sun, X., et al. (2020). Zona incerta projection neurons and GABAergic and GLP-1 mechanisms in the nucleus accumbens are involved in the control of gastric function and food intake. Neuropeptides 80:102018. doi: 10.1016/j.npep.2020.102018
Wayner, M. J., Ono, T., and Nolley, D. (1975). Effects of ethyl alcohol on central neurons. Pharmacol. Biochem. Behav. 3, 499–506. doi: 10.1016/0091-3057(75)90062-3
Williams, S. B., Yorgason, J. T., Nelson, A. C., Lewis, N., Nufer, T. M., Edwards, J. G., et al. (2018). Glutamate transmission to ventral tegmental area GABA neurons is altered by acute and chronic ethanol. Alcohol. Clin. Exp. Res. 42, 2186–2195. doi: 10.1111/acer.13883
Ye, Q., Nunez, J., and Zhang, X. (2023). Zona incerta dopamine neurons encode motivational vigor in food seeking. Sci. Adv. 9:eadi5326. doi: 10.1126/sciadv.adi5326
Zahm, D. S., Becker, M. L., Freiman, A. J., Strauch, S., Degarmo, B., Geisler, S., et al. (2010). Fos after single and repeated self-administration of cocaine and saline in the rat: emphasis on the Basal forebrain and recalibration of expression. Neuropsychopharmacology 35, 445–463. doi: 10.1038/npp.2009.149
Zhang, L., Zhang, P., Qi, G., Cai, H., Li, T., Li, M., et al. (2022). A zona incerta-basomedial amygdala circuit modulates aversive expectation in emotional stress-induced aversive learning deficits. Front. Cell. Neurosci. 16:910699. doi: 10.3389/fncel.2022.910699
Zhang, X., and Van Den Pol, A. N. (2017). Rapid binge-like eating and body weight gain driven by zona incerta GABA neuron activation. Science 356, 853–859. doi: 10.1126/science.aam7100
Zhao, Z., Chen, Z., Xiang, X., Hu, M., Xie, H., Jia, X., et al. (2019). Zona incerta GABAergic neurons integrate prey-related sensory signals and induce an appetitive drive to promote hunting. Nat. Neurosci. 22, 921–932. doi: 10.1038/s41593-019-0404-5
Zhou, H., Xiang, W., and Huang, M. (2021). Inactivation of zona incerta blocks social conditioned place aversion and modulates post-traumatic stress disorder-like behaviors in mice. Front. Behav. Neurosci. 15:743484. doi: 10.3389/fnbeh.2021.743484
Zhou, K., and Zhu, Y. (2019). The paraventricular thalamic nucleus: a key hub of neural circuits underlying drug addiction. Pharmacol. Res. 142, 70–76. doi: 10.1016/j.phrs.2019.02.014
Keywords: zona incerta (ZI), addiction, Parkinson's disease, dopamine, deep brain stimulation
Citation: Wilt M, Magnard R, Carnicella S and Vachez YM (2025) Zona incerta: from Parkinson's disease to addiction. Front. Neural Circuits 19:1537449. doi: 10.3389/fncir.2025.1537449
Received: 30 November 2024; Accepted: 27 January 2025;
Published: 06 February 2025.
Edited by:
Wen-Jie Song, Kumamoto University Hospital, JapanReviewed by:
Kazuya Saitoh, Yamagata Prefectural Yonezawa Nutrition University, JapanCopyright © 2025 Wilt, Magnard, Carnicella and Vachez. This is an open-access article distributed under the terms of the Creative Commons Attribution License (CC BY). The use, distribution or reproduction in other forums is permitted, provided the original author(s) and the copyright owner(s) are credited and that the original publication in this journal is cited, in accordance with accepted academic practice. No use, distribution or reproduction is permitted which does not comply with these terms.
*Correspondence: Yvan M. Vachez, eXZhbi52YWNoZXpAaW5zZXJtLmZy