- Department of Psychiatry, School of Medicine, Washington University in St. Louis, St. Louis, MO, United States
Sexual behavior is crucial for reproduction in many animals. In many vertebrates, females exhibit sexual behavior only during a brief period surrounding ovulation. Over the decades, studies have identified the roles of ovarian sex hormones, which peak in levels around the time of ovulation, and the critical brain regions involved in the regulation of female sexual behavior. Modern technical innovations have enabled a deeper understanding of the neural circuit mechanisms controlling this behavior. In this review, I summarize our current knowledge and discuss the neural circuit mechanisms by which female sexual behavior occurs in association with the ovulatory phase of their cycle.
Introduction
Sexual behavior is essential to reproduction in many animals and is instinctual that the behavior can be displayed without any prior experience. This instinct suggests that the behavior is genetically hard-wired, with the corresponding neural circuits being generated and established during the developmental phase. In many vertebrates, female sexual behavior is synchronized with the estrous cycle. Females are sexually receptive toward male mounting only during a short period surrounding ovulation (estrus phase) and are not receptive during the other stages of the estrous cycle. For example, female mice are sexually receptive once in every four to five days while female giant pandas are receptive only a few days in a year (Allen, 1922; Lindburg et al., 2001). Despite the variability in the duration of female estrous cycles across species, the concurrence of ovulation and female sexual receptivity is a common trait among many mammals. This synchronization is crucial for efficient reproduction, minimizing the risk of predation and waste of energy. Female sex hormones 17β-estradiol (referred to as estrogen here) and progesterone are released from the ovary and their levels peak at the timing surrounding ovulation. Over the decades, studies revealed that estrogen and progesterone, and their receptors, are essential to female sexual behavior (Ring and Providence, 1944; Powers, 1970; Beach, 1976; Lydon et al., 1995; Rissman et al., 1997; Arnold, 2009). Recent studies employing novel genetic, imaging, and behavioral approaches further characterized how these sex hormones contribute to the behavior by modulating neural circuits in the female brain. In this review, I illustrate the overview of how this alliance between ovulation and female sexual behavior is controlled by neural circuitry in the brain.
Female sexual behavior depends on female steroid hormones
The sex steroid hormones estrogen and progesterone, produced and released by the ovary, are essential for inducing female sexual behavior. These sex hormone levels are closely related to the estrous cycle: they peak around the estrus, the stage that female is sexually receptive and ovulating, while it goes down to undetectable levels at the other stages of the estrous cycle (Deleon et al., 1990; Nelson et al., 1992). Removal of ovaries eliminates these changes in sex hormone levels, and consequently, female sexual receptivity. Subsequent supplementation of estrogen and progesterone rescues the behavior in the ovariectomized (OVX) female, demonstrating that female sexual behavior can be induced by increased levels of sex hormones without actual ovulation. This OVX and hormonal priming regimen is widely used to induce female sexual behavior in experimental animals (Whalen, 1974; Nelson et al., 1992; Lydon et al., 1995; Rissman et al., 1997; Ogawa et al., 1998; Bakker et al., 2002; Kudway and Rissman, 2003; Wu et al., 2009; Xu et al., 2012; Yang et al., 2013).
Estrogen and progesterone bind to their cognate receptors such as estrogen receptor a (ERa or Esr1) and progesterone receptor (PR). These receptors are known as transcription factors that they alter gene expression and protein synthesis. Studies utilizing knockout mice of the receptors revealed that signaling of these sex hormones in the brain is essential for female sexual behavior (Lubahn et al., 1993; Lydon et al., 1995; Rissman et al., 1997). Inhibition of protein synthesis in the brain also suppresses the behavior even when OVX females are supplemented with sex hormones (Rainbow et al., 1980, 1982; Meisel and Pfaff, 1985). Together, these findings suggest that a sequence of steroid hormone signaling, followed by gene expression and protein synthesis in the brain, is essential for inducing female sexual behavior.
A more recent study employing microarray, bulk, and single-nucleus RNA sequencing compared gene expression profiles in four different hypothalamic and limbic regions between OVX and hormonally primed OVX females (Xu et al., 2012; Knoedler et al., 2022). Notably, Knoedler et al. (2022) identified 1,415 genes whose expression changes in four limbic and hypothalamic regions depending on sex hormones. This is the first report to highlight such extensive changes in gene expression profiles due to different hormonal states in females. Which genes are critical for controlling female sexual behavior? Gene ontogeny analysis identified that they include genes in synaptic transmission, steroid hormone-related processes, behavior, peripheral reproductive organ processes, and regulation of gene expression. The expression levels of these hormone-sensitive genes vary across different brain regions. Therefore, to elucidate roles of these identified hormone-sensitive genes, it is critical not only to analyze brain-wide gene knockout animals but also to employ region-specific viral delivery of CRISPR-Cas9 for gene knockout and RNAi for gene knockdown (Musatov et al., 2006).
Key neural circuits controlling female sexual behavior
Many hypothalamic and limbic regions in the brain are found to be involved in female sexual behavior. As key regions, the ventromedial hypothalamus (VMH), medial preoptic area (mPOA), and periaqueductal gray (PAG) were identified decades ago as they are critical to the behavior (Figure 1). The induction of the immediate early gene Fos in these regions during female sexual behavior suggests their activation (Pfaus et al., 1993; Blaustein et al., 1994; Flanagan-Cato and Mcewen, 1995; Yamada and Kawata, 2014). Importantly, histological studies indicate that these regions are rich in expression of sex hormonal receptors such as Esr1 or PR (Wu et al., 2009; Cheong et al., 2015; Kim et al., 2019). Local infusion of sex hormones into these areas modulates female sexual behavior, underscoring their control in association with the estrous cycle (Robert, 1962; Barfield and Chen, 1977; Floody et al., 1986; Rajendren et al., 1991). Early work by Sakuma and Pfaff in the 1970s highlighted the importance of these brain regions in modulating female sexual behavior. This review will summarize the roles of each circuit in detail.
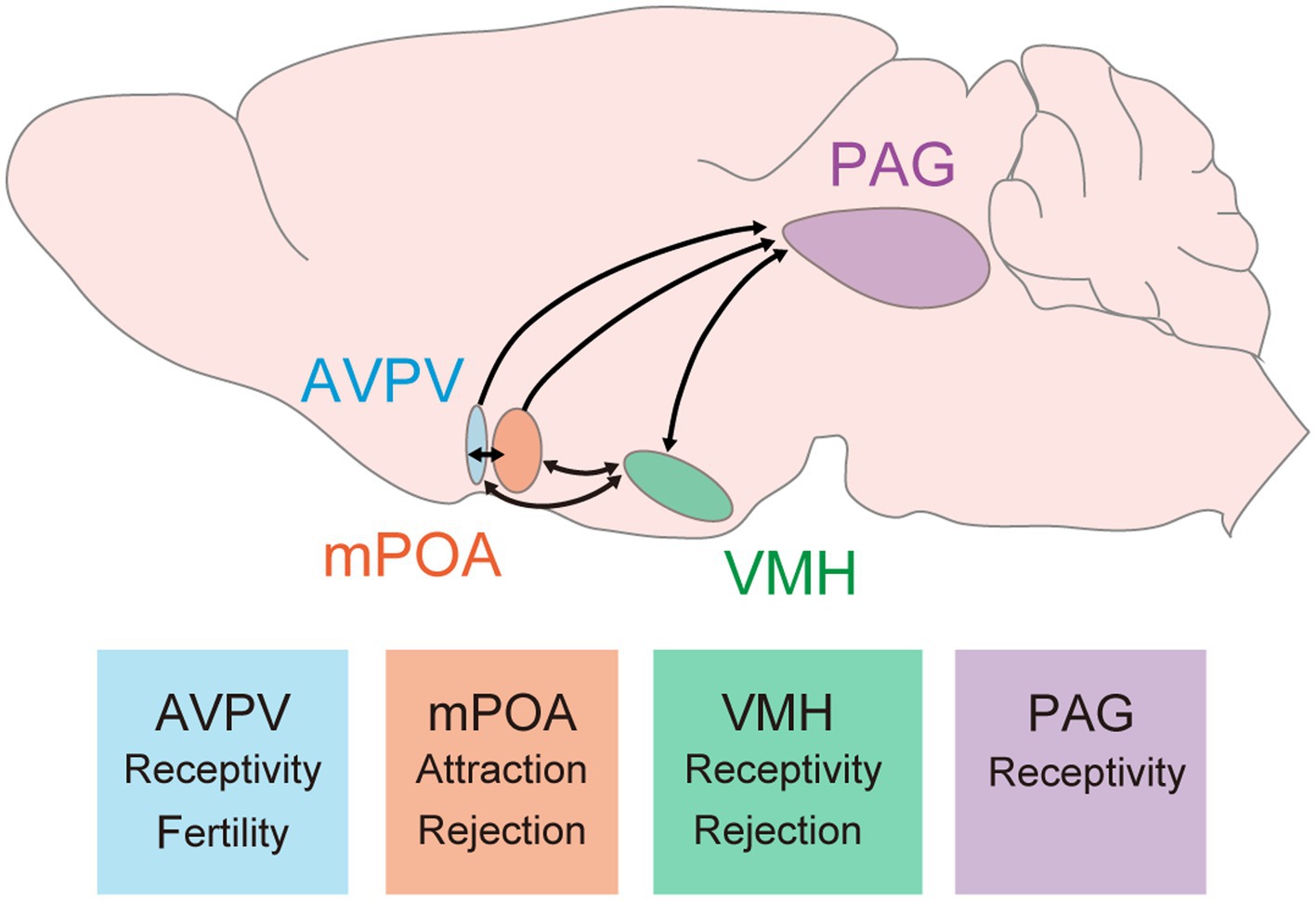
Figure 1. Key brain regions and their roles in female sexual behavior. These hypothalamic and midbrain circuits have been studied and demonstrated their contribution to female sexual behavior. Arrows indicate connections between these regions. It is important to note that reciprocal connections between the PAG and either the AVPV or mPOA have not yet been reported; however, it is possible that such connections exist. Major findings related to female sexual behavior are described at bottom boxes. AVPV, anteroventral periventricular nucleus; mPOA, medial preoptic area; VMH, ventromedial hypothalamus; PAG, periaqueductal gray.
The VMH, especially the ventrolateral part of the VMH (VMHvl), is well characterized its role in female sexual behavior. Single-unit recordings of neuronal firing in awake mice and primates reveal increased firing frequency in VMHvl neurons during conspecific male investigation or upon receiving male mounting and intromission (Aou et al., 1988; Nomoto and Lima, 2015). Electrical stimulation of the VMHvl enhances, while lesions suppress, female sexual receptivity, indicating this region’s necessity and sufficiency (Pfaff and Sakuma, 1979a,b). Moreover, sex hormones play crucial roles in this modulation. Local hormone infusion in the VMHvl elevates female sexual behavior (Barfield and Chen, 1977; Rajendren et al., 1991). Histological studies demonstrate that expressions of sex hormonal receptors, such as Esr1 or PR, are dense in the VMHvl (Xu et al., 2012; Yang et al., 2013). Approximately 50% of VMHvl neurons are Esr1+, with these neurons becoming active during male–female interactions (Hashikawa et al., 2017). Knockdown of Esr1 gene in the VMHvl via RNAi decreases female sexual receptivity (Musatov et al., 2006), suggesting that Esr1 signaling in the VMHvl is necessary to the behavior. Recent studies have further delineated the neuronal populations in control. Almost all PR+ VMHvl neurons co-express Esr1, with about 60% of Esr1+ VMHvl neurons being PR+ (Yang et al., 2013). These PR+ VMHvl neurons, when active during male mounting and lordosis behavior, are crucial for driving female sexual behavior, as shown by fiber photometry imaging (Inoue et al., 2019). Genetically targeted ablation or acute chemogenetic inhibition of these PR+ VMHvl neurons reduces female sexual receptivity, even in hormonally primed OVX females (Yang et al., 2013; Inoue et al., 2019). These findings indicate that PR+ VMHvl neurons are essential to drive female sexual behavior. Single-nucleus RNA sequencing from Esr1+ VMHvl neurons identified a subset expressing the cholecystokinin-a receptor (Cckar) within total 27 of clusters (Figure 2), significant only in hormonally primed OVX females and not in males or OVX females, aligning with earlier microarray and in situ hybridization studies (Xu et al., 2012; Knoedler et al., 2022). This suggests the critical role of Cckar+ VMHvl neurons in female sexual behavior. Fiber photometry imaging revealed that Cckar+ neurons are active during mating, like PR+ VMHvl neurons (Yin et al., 2022). Further, behavioral studies with acute inhibition of Cckar+ VMHvl neurons, conducted by two different groups, found that these neurons are crucial to female sexual receptivity (Knoedler et al., 2022; Yin et al., 2022). Thus, Cckar+ VMHvl neurons are the subset of Esr1+ PR+ VMHvl neurons that control female sexual behavior. Gene knockout of Cckar abolishes female sexual receptivity (Xu et al., 2012), however, whether Cckar gene expression within these neurons is necessary remains to be elucidated. Considering the increased Cckar expression in OVX primed females, it is possible that both firing activity and gene expression in Cckar+ VMHvl neurons control the behavior in a coordinated manner. These Cckar+ VMHvl neurons exist mostly in the center to posterior VMHvl. More recent study indicates that anterior PR+ VMHvl neurons can drive rejection towards male mounts (Gutierrez-Castellanos et al., 2023). The detailed molecular identity of these anterior PR+ VMHvl neurons and connection between Cckar+ VMHvl neurons to regulate sexual receptivity and rejection are interesting topics to be revealed in future studies.
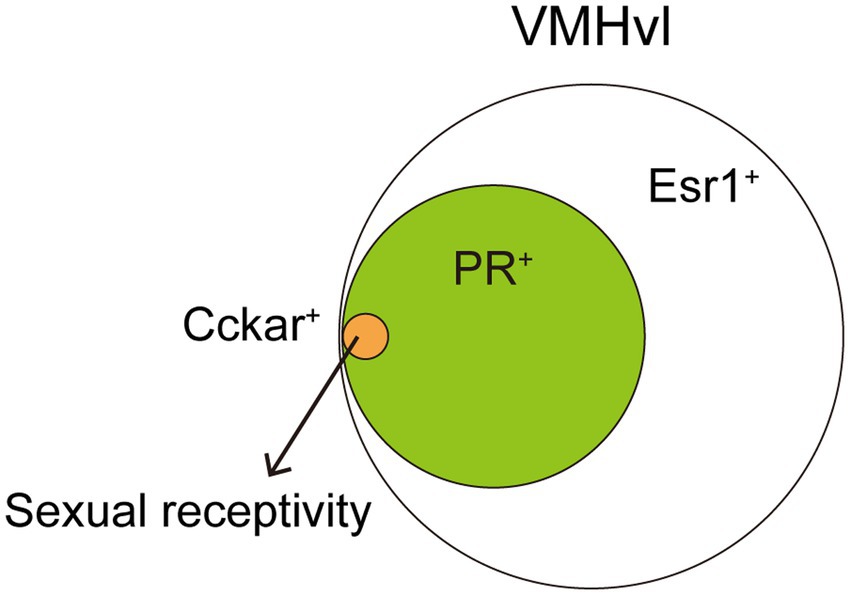
Figure 2. A small subset of VMHvl neurons can drive female sexual behavior. About 60% of the VMHvl neurons expressing Esr1 are also PR+. Further, Cckar+ population is one of 27 clusters identified in Esr1+ VMHvl neurons. Such a small subset of neurons is critical to drive female sexual behavior. Cckar+ VMHvl neurons, as well as PR+ VMHvl neurons, project to the AVPV and this connectivity change depending on ovarian hormone levels.
The PAG is one of the major downstream circuits receiving inputs from VMHvl. The PAG has been shown to increase lordosis upon electrical stimulation and decrease behavior upon lesioning, highlighting its role in modulating female sexual responsiveness (Sakuma and Pfaff, 1979a,b). Electrophysiological recordings from the PAG of anesthetized rats found that PAG neurons response to VMH and mPOA stimulation, suggesting that it works as the downstream of these neural circuits (Sakuma and Pfaff, 1980). The complexity and size of the PAG make it challenging to focus on specific subregions, which may explain the scarcity of in vivo electrophysiological recordings or calcium imaging studies specifically addressing female sexual behavior. Recent advances in spatial transcriptomics have identified distinct neural populations within the lateral PAG that are activated in response to female sexual behavior, as indicated by the co-expression of immediate early genes (Vaughn et al., 2022). This finding aligns with previous research demonstrating Fos expression in the lateral PAG following female sexual behavior (Yamada and Kawata, 2014). Dissecting the roles of these molecularly defined subpopulations within the PAG could provide deeper insights into their specific contributions to female sexual behavior. Given its position in the midbrain and proximity to motor output circuits, the PAG is considered a pivotal output center for the modulation of female sexual behavior. Further elucidation of the projections from PAG subpopulations to the downstream brainstem is crucial for a comprehensive understanding of the neural circuits that govern female sexual behavior.
The role of the medial preoptic area (mPOA) in female sexual behavior is complex and subject to ongoing debate. Electrical stimulation or lesion of the mPOA change the behavior facilitatory and inhibitory directions, indicating its nuanced role in modulating the behavior (Moss et al., 1974; Bast et al., 1987; Takeo et al., 1993). Studies utilizing single-unit recording and fiber photometry imaging techniques have observed that neurons within the mPOA are particularly active during instances when a female receives male mounting, with their activity diminishing as the male dismounts (Aou et al., 1988; Sakuma, 1994; Kato and Sakuma, 2000; Wei et al., 2018). Consistent with these findings, recent fiber photometry imaging of the activity of hormone-sensitive Esr1+ mPOA neurons revealed that they are similarly active when a female receives male mounting (Wei et al., 2018). A two-photon calcium imaging study demonstrates the role of Neurotensin+ mPOA neurons (84% of them are Esr1+) in the initial stages of sexual behavior. These neurons activate when a female encounters male pheromones, and optogenetic suppression of their activity results in a decreased attraction towards males, indicating a role in the pre-copulatory phase of sexual behavior (McHenry et al., 2017). These findings suggest the critical roles that genetically identified neuronal populations in the mPOA play in orchestrating female sexual behavior. However, causal relationships of these populations in copulation remained elusive. Recently, Ishii et al. (2023) discovered that a subset of GABAergic neurons in the mPOA reacts to the completion of mating, specifically when a female receives male ejaculation. These neurons were identified and labeled separately from those activated by pre-mating attractive behavior, allowing for targeted investigation into their respective roles in modulating female sexual behavior. Chemogenetic activation of the mating completion-activated neurons, but not those activated by attractive behavior, led to a reduction in female sexual receptivity. This finding suggests the presence of an mPOA neuronal subpopulation capable of suppressing sexual behavior, highlighting a complex regulatory mechanism within the mPOA. Further complexity will be revealed in the nature of Esr1+ mPOA neurons, the majority of which are GABAergic, suggesting an interplay between estrogen signaling and inhibitory neurotransmission within this brain region (Wei et al., 2018; Knoedler et al., 2022). RNA sequencing studies have identified diverse clusters of neurons within the mPOA, pointing to a rich mosaic of functional and phenotypic heterogeneity (Moffitt et al., 2018; Knoedler et al., 2022). Future studies into these subpopulations will unravel the detailed mechanisms by which the mPOA influences both the attraction phase and the copulatory process in female sexual behavior, offering deeper insights into the neural underpinnings of these complex behaviors.
Another hypothalamic region which is important for female sexual behavior is the anteroventral periventricular nucleus (AVPV). This region is also rich in expression of estrogen and progesterone receptors (Xu et al., 2012; Yang et al., 2013). Recent research has shown that ablation of a subset of AVPV neurons, kisspeptin+ AVPV neurons, leads to a reduction in female sexual receptivity, whereas optogenetic activation of these neurons enhances the behavior (Hellier et al., 2018). PR+ VMHvl neurons exhibit structural plasticity depending on sex hormones (Inoue et al., 2019). Labeling projections of PR+ VMHvl neurons with virally-encoded mCherry fused to the synaptic vesicle synaptophysin (Syp:mCherry) revealed that there is a dramatic, 3-fold increase of presynaptic termini in the AVPV in OVX primed and natural estrus females. Most of PR+ VMHvl neurons are glutamatergic. The amplitude of optogenetically evoked EPSCs in AVPV neurons, following ChR2-assisted stimulation of PR+ VMHvl neuronal axon termini, is larger in OVX primed females compared to OVX females. Subsequent experiments utilizing an optogenetic approach have emphasized the pivotal role of this PR+ VMHvl to AVPV circuit in controlling female sexual receptivity. These findings suggest that sex hormones enhance excitatory synaptic transmission through an increase in glutamatergic projections within PR+ VMHvl to AVPV circuit. Ovarian hormones induce plastic changes in many brain regions (Inoue, 2022). This study represents the first to link hormone-induced plasticity with behavioral alterations throughout the estrous cycle, offering insights into the neural mechanisms underpinning female sexual behavior. As described above, Cckar+ VMHvl neurons are a subset of PR+ VMHvl neurons critical to regulate female sexual behavior. Because almost all of PR+ VMHvl neurons are glutamatergic, Cckar+ VMHvl neurons are also glutamatergic. These Cckar+ VMHvl neurons also project to the AVPV, mPOA, and PAG. Retrograde labeling of AVPV-projecting neurons combined with cell body labeling of Cckar+ VMHvl neurons revealed that over 70% of AVPV-projecting VMHvl neurons are Cckar+. In addition, the same as PR+ VMHvl neurons, Cckar+ VMHvl neurons also increase their presynaptic termini, labeled with Syp:mCherry, in OVX primed females, while Cckar-VMHvl neurons do not (Knoedler et al., 2022). Thus, Cckar+ VMHvl neurons exhibit preferential projection to the AVPV and structural plasticity, suggesting that the Cckar+ projection within PR+ VMHvl to AVPV circuit plays a crucial role in controlling female sexual behavior.
As the VMH, mPOA, AVPV, and PAG are interconnected to each other, how information processing is achieved in these neural circuits is an important future question to understand the whole picture of the neural circuit mechanisms controlling female sexual behavior.
Discussion
Classic studies have identified the pivotal roles of sex hormones and specific brain regions in regulating female sexual behavior. Recent advancements, including transcriptomic analyses and the viral delivery of transgenes to molecularly identified neuronal populations, have enhanced our understanding of how these populations govern behavior. Future research should aim to further elucidate the mechanisms through which sex hormone-mediated regulation of neural circuit functions and behaviors occurs. A critical avenue of investigation involves deciphering the molecular underpinnings of hormone-dependent neural plasticity. Estrogen signaling is important for the structural plasticity in many brain regions including PR+ VMHvl neurons. Utilizing data from recent transcriptomic analyses will be essential in identifying the genes responsible for such structural plasticity. Intriguingly, male PR+ VMHvl neurons do not exhibit the same increase in presynaptic termini as their female counterparts, while the most of PR+ VMHvl neurons express Esr1 in both sexes (Inoue et al., 2019). It is likely that the ability of PR+ VMHvl neurons to rewire the circuit in adults is developmentally hard-wired into the female brain. Such sexually dimorphic structural plasticity could be a result of difference in gene expression downstream of Esr1. Examining differences in Esr1-mediated gene expression between sexes could shed light on the mechanisms behind this sexually dimorphic structural plasticity. Further studies demonstrated that VMHvl neurons change their firing activity across the estrous cycle, and this may also result in cyclic change in female sexual behavior across the estrous cycle (Yin et al., 2022). Identifying the genes whose expression changes in response to hormonal fluctuations within these populations could provide insights into the nature of these plastic changes.
Characterizing the entire neural circuits controlling female sexual behavior in the estrous cycle-dependent manner is another important direction. The major outputs of VMH neurons are to the AVPV, mPOA, and PAG. The VMH to PAG circuit has been considered essential for initiating female sexual behavior, largely because both regions contribute to the behavior and the PAG is more directly linked to motor control functions. However, a recent study has highlighted the significance of the PR+ VMHvl to AVPV circuit in female sexual behavior (Inoue et al., 2019). The AVPV is traditionally recognized for its role in fertility and the regulation of the estrous cycle itself. The involvement of AVPV neurons in modulating female sexual behavior has only recently been uncovered, indicating that previous studies may have overlooked the importance of the VMH to AVPV circuit (Hellier et al., 2018). The interconnected nature of the VMH, mPOA, and AVPV, along with the PAG’s receipt of outputs from these hypothalamic regions, underscores the complexity of the neural networks involved. A comprehensive examination of how information is processed within these interconnected circuits to facilitate female sexual behavior is necessary for a full understanding of the neural mechanisms at play. While not the focus of this review, it is important to note that olfactory cues play a crucial role in driving female sexual behavior (Fraser and Shah, 2014). VMH receives inputs from circuits engaged in pheromonal information processing such as the medial amygdala (MeA) and bed nucleus stria terminalis (BNST) (Lo et al., 2019). Research has shown that the MeA, but not the BNST, is involved in pheromone-induced female sexual behavior (Ishii et al., 2017). The application of recent technical advancements, like multi-fiber photometry, allows for the simultaneous recording of activity across various brain regions (Kim et al., 2016; Guo et al., 2023). This, combined with epistasis behavioral experiments that can either suppress or activate specific nodes within the circuits, offers to dissect the full scope of neural circuits and their relationships in controlling female sexual behavior.
Lastly, exploring beyond the neural circuits that control female sexual behavior to include those governing rejection and mating completion behaviors is essential for a comprehensive understanding of reproductive strategy. Rejection behavior serves to avoid non-productive mating, optimizing energy efficiency and minimizing predation risk. During diestrus, when circulating ovarian hormone levels are low, females actively reject male mounts. This raises the question: Is rejection behavior merely a suppressed form of sexual behavior, or does it involve distinct neural circuits specifically for rejection? As described in the previous section, a recent study of anterior VMHvl controlling rejection suggests the latter possibility (Gutierrez-Castellanos et al., 2023). Another finding from different group also indicates that BNST is important for pheromone-induced rejection behavior (Osakada et al., 2018). One possibility is that the neural circuitry for female sexual behavior and rejection operates in a reciprocal inhibitory manner, modulated by ovarian hormone levels, to produce varied behaviors throughout the estrous cycle. Understanding the intricate connections between these neural circuits is crucial for elucidating how these diverse behaviors are orchestrated across the estrous cycle. Mating completion, characterized by a female receiving male ejaculation, triggers a state of satiation that diminishes sexual behavior (Zhou et al., 2023). Similar to rejection, mating completion leads to behaviors that avoid further male interaction, such as decreased attraction and reduced likelihood of copulation. However, mating completion should be seen as a state leading to gestation rather than an isolated behavior, suggesting that the neural circuits involved in mating completion may differ from or be upstream of those controlling rejection. Investigating the activity dynamics of neural populations responsible for rejection during mating completion, and vice versa, could offer valuable insights into the similarities and differences between these behaviors and states. As described, a subpopulation of mPOA neurons has been identified to encode mating completion (Ishii et al., 2023). Interestingly, another subpopulation of mPOA neurons, Galanin+ mPOA neurons, undergo significant plastic changes during pregnancy, contributing to parenting behavior post-delivery (Ammari et al., 2023). Analyzing the overlap or interconnectivity between neurons involved in mating completion and Galanin+ mPOA neurons could provide deeper understanding into the post-mating completion processes essential for successful reproduction. This comprehensive approach to studying the neural underpinnings of female sexual behavior, along with rejection and mating completion, is pivotal in unraveling the complex interplay of behaviors associated with reproduction.
Females, including women, exhibit various behavioral changes throughout their cycle. The dramatic fluctuations in rodent female sexual behavior across the estrous cycle, influenced by ovarian hormone levels, serve as an excellent model for exploring how ovarian hormones modulate neural circuits and, consequently, behaviors. Insights gained from such research can significantly contribute to our understanding of the etiology of women’s psychiatric disorders, including premenstrual dysphoric disorder, postpartum depression, and symptoms associated with menopausal syndrome. By studying these hormonal modulations and their effects on behavior, we can deepen our understanding of these complex conditions and advance towards more effective treatments.
Author contributions
SI: Writing – original draft, Writing – review & editing.
Funding
The author(s) declare that financial support was received for the research, authorship, and/or publication of this article. This work was supported by the Gift from McDonnell Center for Systems Neuroscience.
Acknowledgments
The author thanks Masatoshi Inoue for their valuable input to the manuscript.
Conflict of interest
The author declares that the research was conducted in the absence of any commercial or financial relationships that could be construed as a potential conflict of interest.
Publisher’s note
All claims expressed in this article are solely those of the authors and do not necessarily represent those of their affiliated organizations, or those of the publisher, the editors and the reviewers. Any product that may be evaluated in this article, or claim that may be made by its manufacturer, is not guaranteed or endorsed by the publisher.
References
Allen, E. (1922). The oestrous cycle in the mouse. Am. J. Anat. 30, 297–371. doi: 10.1002/AJA.1000300303
Ammari, R., Monaca, F., Cao, M., Nassar, E., Wai, P., Grosso, N. A. D., et al. (2023). Hormone-mediated neural remodeling orchestrates parenting onset during pregnancy. Science 382, 76–81. doi: 10.1126/science.adi0576
Aou, S., Oomura, Y., and Yoshimatsu, H. (1988). Neuron activity of the ventromedial hypothalamus and the medial preoptic area of the female monkey during sexual behavior. Brain Res. 455, 65–71. doi: 10.1016/0006-8993(88)90115-1
Arnold, A. P. (2009). The organizational-activational hypothesis as the foundation for a unified theory of sexual differentiation of all mammalian tissues. Horm. Behav. 55, 570–578. doi: 10.1016/j.yhbeh.2009.03.011
Bakker, J., Honda, S.-I., Harada, N., and Balthazart, J. (2002). The aromatase knock-out mouse provides new evidence that estradiol is required during development in the female for the expression of sociosexual behaviors in adulthood. J. Neurosci. 22, 9104–9112. doi: 10.1523/JNEUROSCI.22-20-09104.2002
Barfield, R. J., and Chen, J. J. (1977). Activation of estrous behavior in ovariectomized rats by intracerebral implants of estradiol benzoate. Endocrinology 101, 1716–1725. doi: 10.1210/endo-101-6-1716
Bast, J. D., Hunts, C., Renner, K. J., Morris, R. K., Quadagno, D. M., Hunts, C., et al. (1987). Lesions in the preoptic area suppressed sexual receptivity in ovariectomized rats with estrogen implants in the ventromedial hypothalamus. Brain Res. Bull. 18, 153–158. doi: 10.1016/0361-9230(87)90184-5
Beach, F. A. (1976). Sexual attractivity, proceptivity, and receptivity in female mammals. Horm. Behav. 7, 105–138. doi: 10.1016/0018-506x(76)90008-8
Blaustein, J. D., Tetel, M. J., Ricciardi, K. H. N., Delville, Y., and Turcotte, J. C. (1994). Hypothalamic ovarian steroid hormone-sensitive neurons involved in female sexual behavior. Psychoneuroendocrinology 19, 505–516. doi: 10.1016/0306-4530(94)90036-1
Cheong, R. Y., Czieselsky, K., Porteous, R., and Herbison, A. (2015.) Expression of ESR1 in Glutamatergic and GABAergic Neurons Is Essential for Normal Puberty Onset, Estrogen Feedback, and Fertility in Female Mice. J Neurosci. 35: 14533–14543. doi: 10.1523/JNEUROSCI.1776-15.2015
Deleon, D. D., Zelinski-Wooten, M. B., and Barkley, M. S. (1990). Hormonal basis of variation in oestrous cyclicity in selected strains of mice. J. Reprod. Fertil. 89, 117–126. doi: 10.1530/jrf.0.0890117
Flanagan-Cato, L. M., and Mcewen, B. S. (1995). Pattern of Fos and Jun expression in the female rat forebrain after sexual behavior. Brain Res. 673, 53–60. doi: 10.1016/0006-8993(94)01395-x
Floody, O. R., Lisk, R. D., and Vomachka, A. J. (1986). Facilitation of lordosis by estradiol in the mesencephalic central gray. Physiol. Behav. 37, 587–595. doi: 10.1016/0031-9384(86)90291-x
Fraser, E. J., and Shah, N. M. (2014). Complex chemosensory control of female reproductive behaviors. PLoS One 9:e90368. doi: 10.1371/journal.pone.0090368
Guo, Z., Yin, L., Diaz, V., Dai, B., Osakada, T., Lischinsky, J. E., et al. (2023). Neural dynamics in the limbic system during male social behaviors. Neuron 111, 3288–3306.e4. doi: 10.1016/j.neuron.2023.07.011
Gutierrez-Castellanos, N., Husain, B. F. A., Dias, I. C., Nomoto, K., Duarte, M. A., Ferreira, L., et al., (2023) A hypothalamic node for the cyclical control of female sexual rejection. bioRxiv. Available at: https://doi.org/10.1101/2023.01.30.526259. [Epub ahead of preprint]
Hashikawa, K., Hashikawa, Y., Tremblay, R., Zhang, J., Feng, J. E., Sabol, A., et al. (2017). Esr1+ cells in the ventromedial hypothalamus control female aggression. Nat. Neurosci. 20, 1580–1590. doi: 10.1038/nn.4644
Hellier, V., Brock, O., Candlish, M., Desroziers, E., Aoki, M., Mayer, C., et al. (2018). Female sexual behavior in mice is controlled by kisspeptin neurons. Nat. Commun. 9:400. doi: 10.1038/s41467-017-02797-2
Inoue, S. (2022). Neural basis for estrous cycle-dependent control of female behaviors. Neurosci. Res. 176, 1–8. doi: 10.1016/j.neures.2021.07.001
Inoue, S., Yang, R., Tantry, A., Davis, C., Yang, T., Knoedler, J. R., et al. (2019). Periodic remodeling in a neural circuit governs timing of female sexual behavior. Cell 179, 1393–1408.e16. doi: 10.1016/j.cell.2019.10.025
Ishii, K. K., Hashikawa, K., Chea, J., Yin, S., Fox, R. E., Kan, S., et al. (2023) Post-mating inhibition of female sexual drive via heterogeneous neuronal ensembles in the medial preoptic area. bioRxiv. Available at: https://doi.org/10.1101/2023.09.08.556711. [Epub ahead of preprint]
Ishii, K. K., Osakada, T., Mori, H., Miyasaka, N., Yoshihara, Y., Miyamichi, K., et al. (2017). A labeled-line neural circuit for pheromone-mediated sexual behaviors in mice. Neuron 95, 123–137.e8. doi: 10.1016/j.neuron.2017.05.038
Kato, A., and Sakuma, Y. (2000). Neuronal activity in female rat preoptic area associated with sexually motivated behavior. Brain Res. 862, 90–102. doi: 10.1016/s0006-8993(00)02076-x
Kim, C. K., Yang, S. J., Pichamoorthy, N., Young, N. P., Kauvar, I., Jennings, J. H., et al. (2016). Simultaneous fast measurement of circuit dynamics at multiple sites across the mammalian brain. Nat. Methods 13, 325–328. doi: 10.1038/nmeth.3770
Kim, D. W., Yao, Z, Graybuck, L. T., Kim, T. K., Nguyen, T. N., Smith, K. A., et al. (2019). Multimodal Analysis of Cell Types in a Hypothalamic Node Controlling Social Behavior. Cell. 179:713–728. doi: 10.1016/j.cell.2019.09.020
Knoedler, J. R., Inoue, S., Bayless, D. W., Yang, T., Tantry, A., Davis, C. H., et al. (2022). A functional cellular framework for sex and estrous cycle-dependent gene expression and behavior. Cell 185, 654–671.e22. doi: 10.1016/j.cell.2021.12.031
Kudway, A. E., and Rissman, E. F. (2003). Double oestrogen receptor a and b knockout mice reveal differences in neural oestrogen-mediated progestin receptor induction and female sexual behaviour. J. Neuroendocrinol. 15, 978–983. doi: 10.1046/j.1365-2826.2003.01089.x
Lindburg, D. G., Czekala, N. M., and Swaisgood, R. R. (2001). Hormonal and behavioral relationships during estrus in the giant panda. Zoo Biol. 20, 537–543. doi: 10.1002/zoo.10027
Lo, L., Yao, S., Kim, D. W., Cetin, A., Harris, J., Zeng, H., et al. (2019). Connectional architecture of a mouse hypothalamic circuit node controlling social behavior. Proc. Natl. Acad. Sci. USA. 116: 7503–7512. doi: 10.1073/pnas.1817503116
Lubahn, D. B., Moyer, J. S., Golding, T. S., Couse, J. F., Korach, K. S., and Smithies, O. (1993). Alteration of reproductive function but not prenatal sexual development after insertional disruption of the mouse estrogen receptor gene. Proc. Natl. Acad. Sci. U.S.A. 90, 11162–11166. doi: 10.1073/pnas.90.23.11162
Lydon, J. P., DeMayo, F. J., Funk, C. R., Mani, S. K., Hughes, A. R., Montgomery, C. A., et al. (1995). Mice lacking progesterone receptor exhibit pleiotropic reproductive abnormalities. Genes Dev. 9, 2266–2278. doi: 10.1101/gad.9.18.2266
McHenry, J. A., Otis, J. M., Rossi, M. A., Robinson, J. E., Kosyk, O., Miller, N. W., et al. (2017). Hormonal gain control of a medial preoptic area social reward circuit. Nat. Neurosci. 20, 449–458. doi: 10.1038/nn.4487
Meisel, R. L., and Pfaff, D. W. (1985). Specificity and neural sites of action of anisomycin in the reduction or facilitation of female sexual behavior in rats. Horm. Behav. 19, 237–251. doi: 10.1016/0018-506x(85)90024-8
Moffitt, J. R., Bambah-Mukku, D., Eichhorn, S. W., Vaughn, E., Shekhar, K., Perez, J. D., et al. (2018). Molecular, spatial and functional single-cell profiling of the hypothalamic preoptic region. Science 362:eaau5324. doi: 10.1126/science.aau5324
Moss, R. L., Paloutzian, R. F., and Law, O. T. (1974). Electrical stimulation of forebrain structures and its effect on copulatory as well as stimulus-bound behavior in ovariectomized hormone-primed rats. Physiol. Behav. 12, 997–1004. doi: 10.1016/0031-9384(74)90147-4
Musatov, S., Chen, W., Pfaff, D. W., Kaplitt, M. G., Ogawa, S., and Sciences, B. (2006). RNAi-mediated silencing of estrogen receptor in the ventromedial nucleus of hypothalamus abolishes female sexual behaviors. Proc. Natl. Acad. Sci. U.S.A. 103, 10456–10460. doi: 10.1073/pnas.0603045103
Nelson, J. F., Feliciot, L. S., Osterburg, H. H., and Finch, C. E. (1992). Differential contributions of ovarian and extraovarian factors to age-related reductions in plasma estradiol and progesterone during the estrous cycle of C57BL/6J mice. Endocrinology 130, 805–810. doi: 10.1210/endo.130.2.1733727
Nomoto, K., and Lima, S. Q. (2015). Enhanced male-evoked responses in the ventromedial hypothalamus of sexually receptive female mice. Curr. Biol. 25, 589–594. doi: 10.1016/j.cub.2014.12.048
Ogawa, S., Eng, V., Taylor, J., Lubahn, D. B., Korach, K. S., and Pfaff, D. W. (1998). Roles of estrogen receptor-gene expression in reproduction-related behaviors in female mice. Endocrinology 139, 5070–5081. doi: 10.1210/endo.139.12.6357
Osakada, T., Ishii, K. K., Mori, H., Eguchi, R., Ferrero, D. M., Yoshihara, Y., et al. (2018). Sexual rejection via a vomeronasal receptor-triggered limbic circuit. Nat. Commun. 9:4463. doi: 10.1038/s41467-018-07003-5
Pfaff, D. W., and Sakuma, Y. (1979a). Facilitation of the lordosis reflex of female rats from the ventromedial nucleus of the hypothalamus. J. Physiol. 288, 189–202. doi: 10.1113/jphysiol.1979.sp012690
Pfaff, D. W., and Sakuma, Y. (1979b). Deficit in the lordosis reflex of female rats caused by lesions in the ventromedial nucleus of the hypothalamus. J. Physiol. 288, 203–210. doi: 10.1113/jphysiol.1979.sp012691
Pfaus, J. G., Kleopoulos, S. P., Mobbs, C., Gibbs, R. B., and Pfaff, D. W. (1993). Sexual stimulation activates c-fos within estrogen-concentrating regions of the female rat forebrain. Brain Res. 624, 253–267. doi: 10.1016/0006-8993(93)90085-2
Powers, J. B. (1970). Hormonal control of sexual receptivity during the estrous cycle of the rat. Physiol. Behav. 5, 831–835. doi: 10.1016/0031-9384(70)90167-8
Rainbow, T. C., Davis, P. G., and Mcewen, B. S. (1980). Anisomycin inhibits the activation of sexual behavior by estradiol and progesterone. Brain Res. 194, 548–555. doi: 10.1016/0006-8993(80)91240-8
Rainbow, T. C., Mcginnis, M. Y., Davis, P. G., and Mcewen, B. S. (1982). Application of anisomycin to the lateral ventromedial nucleus of the hypothalamus inhibits the activation of sexual behavior by estradiol and progesterone. Brain Res. 233, 417–423. doi: 10.1016/0006-8993(82)91217-3
Rajendren, G., Dudley, C. A., and Moss, R. L. (1991). Role of the ventromedial nucleus of hypothalamus in the male-induced enhancement of lordosis in female rats. Physiol. Behav. 50, 705–710. doi: 10.1016/0031-9384(91)90006-a
Ring, J. R., and Providence, R. I. (1944). The estrogen-progesterone induction of sexual receptivity in the spayed female mouse. Endocrinology 34, 269–275. doi: 10.1210/endo-34-4-269
Rissman, E. F., Early, A. H., Taylor, J. A., Korach, K. S., and Lubahn, D. B. (1997). Estrogen receptors are essential for female sexual receptivity. Endocrinology 138, 507–510. doi: 10.1210/endo.138.1.4985
Robert, D. (1962). Diencephalic placement of estradiol and sexual receptivity in the female rat. Am. J. Phys. 203, 493–496. doi: 10.1152/ajplegacy.1962.203.3.493
Sakuma, Y. (1994). Estrogen-induced changes in the neural impulse flow from the female rat preoptic region. Horm. Behav. 28, 438–444. doi: 10.1006/hbeh.1994.1041
Sakuma, Y., and Pfaff, D. W. (1979a). Facilitation of female reproductive behavior from mesensephalic central gray in the rat. Am. J. Phys. 237, R278–R284. doi: 10.1152/ajpregu.1979.237.5.R278
Sakuma, Y., and Pfaff, D. W. (1979b). Mesencephdic mechanisms for integration of female reproductive behavior in the rat. Am. J. Phys. 237, R285–R290. doi: 10.1152/ajpregu.1979.237.5.R285
Sakuma, Y., and Pfaff, D. W. (1980). Covergent effects of lordosis-relevant somatosensory and hypothalamic influences on central gray cells in the rat mesencephalon. Exp. Neurol. 70, 269–281. doi: 10.1016/0014-4886(80)90026-6
Takeo, T., Chiba, Y., and Sakuma, Y. (1993). Suppression of the lordosis reflex of female rats by efferents of the medial preoptic area. Physiol. Behav. 53, 831–838. doi: 10.1016/0031-9384(93)90258-h
Vaughn, E., Eichhorn, S., Jung, W., Zhuang, X., and Dulac, C.. (2022). Three-dimensional interrogation of cell types and instinctive behavior in the periaqueductal gray. bioRxiv. Available at: https://doi.org/10.1101/2022.06.27.497769. [Epub ahead of preprint]
Wei, Y. C., Wang, S. R., Jiao, Z. L., Zhang, W., Lin, J. K., Li, X. Y., et al. (2018). Medial preoptic area in mice is capable of mediating sexually dimorphic behaviors regardless of gender. Nat. Commun. 9:279. doi: 10.1038/s41467-017-02648-0
Whalen, R. E. (1974). Estrogen-progesterone induction of mating in female rats. Horm. Behav. 5, 157–162. doi: 10.1016/0018-506x(74)90040-3
Wu, M., Manoli, D. S., Fraser, E. J., Coats, J. K., Tollkuhn, J., Honda, S. I., et al. (2009). Estrogen masculinizes neural pathways and sex-specific behaviors. Cell 139, 61–72. doi: 10.1016/j.cell.2009.07.036
Xu, X., Coats, J. K., Yang, C. F., Wang, A., Ahmed, O. M., Alvarado, M., et al. (2012). Modular genetic control of sexually dimorphic behaviors. Cell 148, 596–607. doi: 10.1016/j.cell.2011.12.018
Yamada, S., and Kawata, M. (2014). Identification of neural cells activated by mating stimulus in the periaqueductal gray in female rats. Front. Neurosci. 8:421. doi: 10.3389/fnins.2014.00421
Yang, C. F., Chiang, M. C., Gray, D. C., Prabhakaran, M., Alvarado, M., Juntti, S. A., et al. (2013). Sexually dimorphic neurons in the ventromedial hypothalamus govern mating in both sexes and aggression in males. Cell 153, 896–909. doi: 10.1016/j.cell.2013.04.017
Yin, L., Hashikawa, K., Hashikawa, Y., Osakada, T., Lischinsky, J. E., Diaz, V., et al. (2022). VMHvllCckar cells dynamically control female sexual behaviors over the reproductive cycle. Neuron 110, 3000–3017.e8. doi: 10.1016/j.neuron.2022.06.026
Keywords: social behavior, ovarian hormones, female, sexual behavior, hypothalamus
Citation: Inoue S (2024) Hormonal and circuit mechanisms controlling female sexual behavior. Front. Neural Circuits. 18:1409349. doi: 10.3389/fncir.2024.1409349
Edited by:
Kensaku Mori, RIKEN, JapanReviewed by:
Yoshitaka Oka, The University of Tokyo, JapanCopyright © 2024 Inoue. This is an open-access article distributed under the terms of the Creative Commons Attribution License (CC BY). The use, distribution or reproduction in other forums is permitted, provided the original author(s) and the copyright owner(s) are credited and that the original publication in this journal is cited, in accordance with accepted academic practice. No use, distribution or reproduction is permitted which does not comply with these terms.
*Correspondence: Sayaka Inoue, c2F5YWthQHd1c3RsLmVkdQ==