- 1Bioelectronic Neurophysiology and Engineering Laboratory, Department of Neurology, Mayo Clinic, Rochester, MN, United States
- 2Department of Physiology and Biomedical Engineering, Mayo Clinic, Rochester, MN, United States
- 3Department of Neurologic Surgery, Mayo Clinic, Rochester, MN, United States
- 4Departments of Psychiatry and Psychology, Mayo Clinic, Rochester, MN, United States
- 5Department of Radiology, Mayo Clinic, Rochester, MN, United States
- 6Czech Institute of Informatics, Robotics, and Cybernetics, Czech Technical University, Prague, Czechia
The network nature of focal epilepsy is exemplified by mesial temporal lobe epilepsy (mTLE), characterized by focal seizures originating from the mesial temporal neocortex, amygdala, and hippocampus. The mTLE network hypothesis is evident in seizure semiology and interictal comorbidities, both reflecting limbic network dysfunction. The network generating seizures also supports essential physiological functions, including memory, emotion, mood, and sleep. Pathology in the mTLE network often manifests as interictal behavioral disturbances and seizures. The limbic circuit is a vital network, and here we review one of the most common focal epilepsies and its comorbidities. We describe two people with drug resistant mTLE implanted with an investigational device enabling continuous hippocampal local field potential sensing and anterior nucleus of thalamus deep brain stimulation (ANT-DBS) who experienced reversible psychosis during continuous high-frequency stimulation. The mechanism(s) of psychosis remain poorly understood and here we speculate that the anti-epileptic effect of high frequency ANT-DBS may provide insights into the physiology of primary disorders associated with psychosis.
1 Introduction
Epilepsy is a common neurologic disease characterized by recurrent seizures that impact over 60 million people worldwide (Institute of Medicine Committee on the Public Health Dimensions of the Epilepsies et al., 2012; Devinsky et al., 2018). In addition to seizures, the quality of life for people with epilepsy (PWE) can be markedly affected by psychiatric and neurologic comorbidities (Institute of Medicine Committee on the Public Health Dimensions of the Epilepsies et al., 2012). Epilepsy is a network circuit disorder with dysregulation of specific brain networks underlying sporadic seizures and chronic comorbidities (Institute of Medicine Committee on the Public Health Dimensions of the Epilepsies et al., 2012; Spencer, Gerrard, and Zaveri, 2018; Lehnertz, Bröhl, and Wrede, 2023). Anti-seizure medications are the mainstay of epilepsy therapy, but over 1/3 PWE have drug-resistant epilepsy (Kwan et al., 2011) and continue to have seizures despite taking daily medications.
Mesial temporal lobe epilepsy (mTLE) is one of the most common epilepsies. It is defined by focal seizures originating from mesial temporal structures (J. Engel Jr, 2001; Berg, 2008) that comprise part of the limbic circuitry (Papez, 1937; MacLean, 1949; Child and Eduardo, 2013) with seizures involving the amygdala, hippocampus, and parahippocampal neocortex. mTLE (Berg, 2008) is a common limbic circuit disorder with disabling seizures and a high incidence of interictal psychiatric (Nadkarni, Arnedo, and Devinsky, 2007; Kanner, 2009; Kanner, Ribot, and Mazarati, 2018), memory (B. Bell et al., 2011; McAuley et al., 2010), and sleep (MMS) disturbances (Devinsky et al., 2018; Moore et al., 2021; Garg, Charlesworth, and Shukla, 2022). Destructive surgical procedures are proven treatments for drug-resistant mTLE (Wiebe et al., 2001; Engel Jr et al., 2012). Unfortunately, many people with drug-resistant mTLE are poor surgical candidates because the destruction of the circuit responsible for their seizures would negatively impact memory (Engel et al., 2012; M. L; Bell et al., 2009). The same limbic circuitry responsible for mTLE continues to perform vital functions when it is not producing sporadic, disabling seizures. These treatment challenges are more pronounced for people with normal structural imaging (M. L. Bell et al., 2009), bilateral mTLE (Squire, 2009), or high baseline memory performance. This has stimulated interest in non-destructive, reversible options like electrical brain stimulation (Fisher and Ana, 2014).
The human limbic circuit is a complex system involving multiple brain structures and is essential for regulating emotional processing, memory processing, and sleep and alertness. The circuit is generally considered to include the amygdala, hippocampus, anterior nucleus of the thalamus, hypothalamus, cingulate cortex and fornix (Figure 1). The amygdala (AMG) is critical for processing emotions and how memories are influenced by emotion (Paré and Headley, 2023). The HPC is critical for encoding memories in thalamocortical networks including long-term, autobiographical memory (Baker and Zeman, 2017; Lemesle et al., 2021). The anterior nucleus of the thalamus (ANT) is crucial for regulating memory, sleep, and alertness (Szabó et al., 2022). The hypothalamus regulates homeostatic functions. The cingulate cortex plays a role in emotional and cognitive processing and the fornix (FRX) is a significant output tract from the hippocampus.
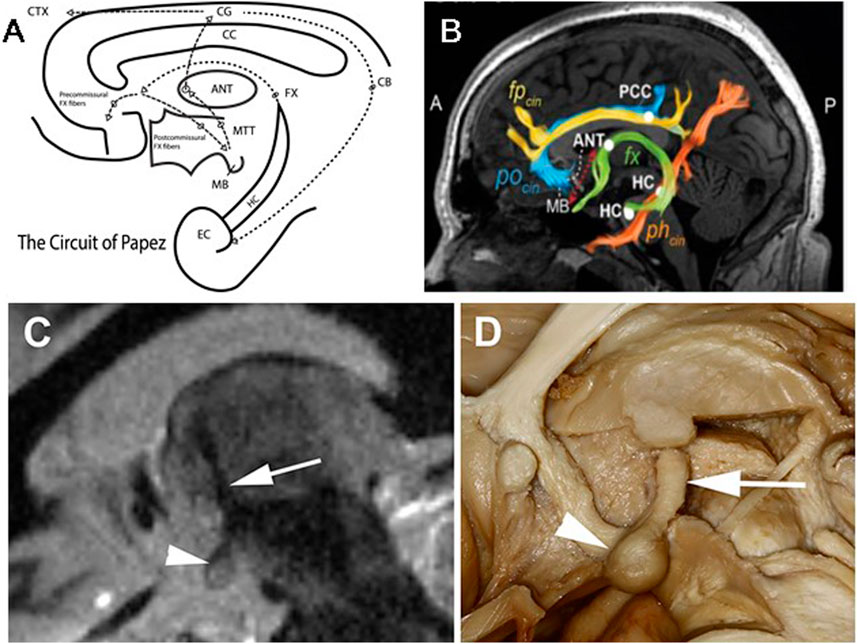
Figure 1. Limbic Circuit of Papez and MacLean: McLean modified Papez version of the limbic circuitry and emphasized the amygdala and septum in addition to hippocampus [20]. (A) Schematic of Limbic Circuit highlighting the closed-loop pathway from the entorhinal cortical (EC) input to hippocampus (HC). The outflow of HC via the Fornix (fx) fibers spans the corpus callosum (CC) and divides into pre- (terminate in Septal Nuclei (SN)) and post- (terminate in the mammillary bodies (MB)) CC commissural fibers. The post-commissural fibers project through the hypothalamus to the MB, and MB fibers project via mammillary thalamic tract (MTT) to the anterior nucleus of the thalamus (ANT). (Note: The MTT is widely used for direct targeting of the ANT for ANT-DBS). The ANT output via anterior thalamocortical fibers to the anterior Cingulate Gyrus and back to EC and HC via the Cingulum. (B) Diffusion tensor imaging (DTI) demonstrating white matter tracks discussed above. (C) Fast gray matter acquisition T1 inversion recovery (FGATIR) MRI sequence visualizing the MB, MTT and ANT. (D) Human brain dissection demonstrating the MB and MTT connecting MB and ANT. (B) modified from Ojeda Valencia, Gabriela, et al. “Signatures of Electrical Stimulation Driven Network Interactions in the Human Limbic System.” Journal of Neuroscience: 43, no. 39 (27 September 2023): 6697–6711. (C,D) modified from Grewal, S., et al. “Fast Gray Matter Acquisition T1 Inversion Recovery MRI to Delineate the Mammillothalamic Tract for Preoperative Direct Targeting of the Anterior Nucleus of the Thalamus for Deep Brain Stimulation in Epilepsy.” Neurosurgical Focus 45, no. 2 (2018).
1.1 Ictal and interictal epileptiform discharges mTLE
The symptoms and signs of TLE seizures have appeared in medical literature throughout history (Temkin, 1994). Hughlings Jackson, in the 19th century, described intellectual or “dreamy state” temporal lobe seizures (Hughlings-Jackson, 1888; Hogan and Kitti, 2003). The seizures in people with mTLE are primarily diurnal, occurring during the daytime (Durazzo et al., 2008; Mivalt et al., 2023).
Interictal epileptiform spikes (IES) and pathological high-frequency oscillations (Worrell and Gotman, 2011) are interictal biomarkers of the epileptogenic brain with ultradian, circadian, and infradian periodicities (Baud et al., 2018; Karoly et al., 2021). Interestingly, the IES are increased in slow-wave sleep but seizures are more likely in wakefulness in mTLE (Kremen et al., 2024).
1.2 Interictal comorbidities of mTLE
It is widely appreciated that mTLE is associated with Memory, Mood, and Sleep (MMS) Epilepsy Comorbidities. There are well-known but poorly understood complex bidirectional interactions between epilepsy, sleep (Grigg-Damberger and Foldvary-Schaefer, 2021; Moore et al., 2021), mood (Bølling-Ladegaard et al., 2023), and memory (Blake et al., 2000).
Verbal and spatial memory deficits are a common problem in mTLE, contributing to one of the major comorbidities affecting quality of life (Milner, 1968; L. R; Squire and Zola-Morgan, 1991; McAuley et al., 2010). Multiple studies show that IES in TLE can impair memory task performance (Kleen et al., 2013; Matsumoto et al., 2013; Horak et al., 2017). Accelerated long-term forgetting describes difficulties that people with epilepsy have in retaining new information over extended periods of time (Blake et al., 2000; Baker and Zeman, 2017; Helmstaedter et al., 2019). Seizures and interictal epileptiform spikes/sharp waves (IES) may interfere with the neural process by which memories are stabilized and stored for long-term use, i.e., the memory consolidation process (Blake et al., 2000). The possibility that mTLE “hijacks” the physiological process of memory and consolidation of engrams underlying the natural progression of mTLE is interesting to consider and suggests that post-ictal disruption of consolidation may be a viable treatment strategy (Beenhakker and Huguenard, 2009; Bower et al., 2015; 2017).
Sleep disruption is common in epilepsy (Moore et al., 2021; Garg, Charlesworth, and Shukla, 2022) and can be exacerbated by thalamic deep brain stimulation (Voges et al., 2015; Szabó et al., 2022; F; Mivalt et al., 2022; Kremen et al., 2024).
People with epilepsy have high rates of psychiatric comorbidity and there is a well-established bidirectional relationship (Kanner, Ribot, and Mazarati, 2018; Bølling-Ladegaard et al., 2023). The lifetime prevalence of major depressive disorder and anxiety-related disorders in epilepsy is 6% and 22%, higher than in the general population (Tellez-Zenteno et al., 2007). The severity of depression and anxiety symptoms can be better predictors of quality of life in epilepsy than seizure frequency (Boylan et al., 2004; Johnson et al., 2004).
Psychosis is a less common but clinically significant phenomenon in mTLE (Nadkarni, Arnedo, and Devinsky, 2007). Psychosis symptoms in mTLE resemble those seen in primary psychiatric disorders such as schizophrenia, including hallucinations (typically auditory but also visual), delusions, and paranoid thoughts. Psychosis is associated with seizures is referred to as “ictal or post-ictal psychosis,” occurring during or closely following a seizure. Interictal psychosis occurs independently of seizure activity (Leutmezer et al., 2003; Hilger et al., 2013).
1.3 Neuromodulatory treatment for drug-resistant mTLE
Early investigations using thalamus deep brain stimulation began with anterior nucleus of thalamus deep brain stimulation (ANT-DBS) (Cooper and Upton, 1985) and centromedian nucleus of thalamus (CMT-DBS) (Velasco et al., 1987). Later controlled trials targeting vagus nerve stimulation (VNS) (E. Ben-Menachem et al., 1999; Ben-Menachem, 2002; R. S; Fisher, 2012), deep brain stimulation targeting the anterior nucleus of the thalamus (ANT-DBS) (R. Fisher et al., 2010; Salanova et al., 2021), and responsive neural stimulation (RNS) (Morrell and RNS System in Epilepsy Study Group, 2011) lead to class-I evidence for drug-resistant mTLE. But patients rarely achieve long-term, >1 year, seizure freedom. The pivotal SANTE (Stimulation of ANT for Epilepsy) trial proved duty cycle, high-frequency stimulation (145 Hz; 1 min on and 5 min off) reduced seizures. However, ANT-DBS with SANTE stimulation parameters rarely yields seizure-free outcomes and can exacerbate mood, memory, and sleep (MMS) comorbidities (Voges et al., 2015; Tröster et al., 2017; Szabó et al., 2022). Despite increased mood and memory complaints in SANTE standard neuropsychological assessments did not detect a change (Tröster et al., 2017).
Relatively little is known about ANT-DBS parameter optimization (R. S. Fisher, 2023; Lundstrom and Gregg, 2023; McIntyre et al., 2006) and assessment of MMS comorbidities have largely relied on sparse data collected at clinic visits. Seizure counts have relied on patient diaries that are known to be inaccurate (Elger, 2014; Morrell, 2014; Osorio, 2014; Elger and Hoppe, 2018). Advances in MRI imaging have significantly improved the targeting for ANT-DBS (Figure 1) (Grewal et al., 2018; Järvenpää et al., 2020). In summary, optimal ANT-DBS parameters for mTLE remain unknown. Emerging device technologies are overcoming the gaps and providing dense behavioral tracking and accurate seizure diaries (Figure 2) (V. Kremen et al., 2018; Sladky et al., 2022; F; Mivalt et al., 2022; Kremen et al., 2018; Filip Mivalt, Sladky, et al., 2023; Gregg et al., 2020) to help optimize ANT-DBS parameters.
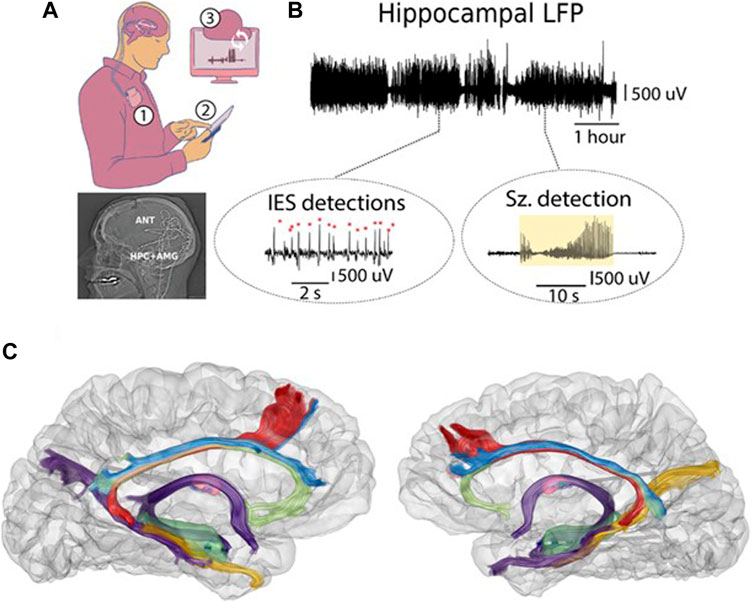
Figure 2. Human limbic circuit electrophysiology. Continuous LFP streaming was used to track mesial temporal lobe and ANT electrophysiology in people with mTLE living in their natural home environment. (A) Synchronized bidirectional communications between patients, implantable neural sensing-stimulation devices, mobile devices, and cloud computing infrastructure enable monitoring electrophysiology. Top) Implanted neural sensing and stimulation device in subclavicular pocket (1). The implanted devices have bidirectional communication with the mobile device (2) and cloud environment (3). Bottom) Lateral x-ray post-implant showing the 4 leads implanted in bilateral ANT and AMG-HPC. (B) Top) Continuous LFP over multiple hours. Bottom) Circles highlight automated IES and seizure detections on mobile devices (tablet computers). (C) Co-registration of CT and MRI-DTI demonstrating the posterior approach to the AMG-HPC and ANT for brain sensing and stimulation electrodes.
2 Case report of two patients undergoing continuous high-frequency ANT-DBS
Here, we describe two subjects implanted with an investigational device (Medtronic Plc. Summit RC + S™) with continuous hippocampal local field potential (LFP) sensing, enabling accurate seizure diaries (Sladky et al., 2022; V; Kremen et al., 2018). The patients each had over 1 year of weekly contact with the research and clinical teams to assess device connectivity, signal fidelity, seizure frequency, and clinical status. This close monitoring yielded the following case narratives which are high-level summaries of both in-person and remote clinical assessments and patient self-reported symptoms. Although neuropsychological testing was performed at fixed, pre-determined intervals during the study, additional quantitative assessments were not performed at the time of the patients’ psychotic symptoms. Both patients developed reversible psychosis with continuous high-frequency ANT-DBS. The psychosis resolved with the transition to duty cycle stimulation (1 min on and 5 min off) as used in the SANTE trial.
2.1 Patient 1
A 57-year-old, ambidextrous female presented with a 40-year history of epilepsy, depression, and anxiety. At 9 years old, she had a significant head trauma associated with a brief loss of consciousness. No imaging was performed at the time, and she made a complete recovery. She did well in school, but at age 14 years she began having sporadic episodes of déjà vu lasting less than a minute and without loss of awareness. The spells became more intense in college, with intense fear, depersonalization, and false memories lasting 1–2 min in duration. By age 21, the spells commonly progressed to generalized tonic-clonic convulsions. She was started on anti-seizure medications that eliminated the convulsive seizures, but she continued to have seizures with loss of awareness despite multiple anti-seizure medications (ASMs) trials.
She reported a long-standing history of anxiety, stating she awakened each day with fear about having a seizure. She had a history of depression accompanied by low mood, anhedonia, decreased appetite, tearfulness, and insomnia. She had no prior history of mania, hypomania, or psychosis.
She underwent a comprehensive evaluation for drug-resistant epilepsy.
1) Laboratory studies were unremarkable, including an autoimmune epilepsy panel.
2) MRI was unremarkable except for subtle indistinct bilateral hippocampal internal architecture and possible left amygdala enlargement.
3) EMU: Video-EEG monitoring showed frequent bilateral temporal intermittent rhythmic delta activity and independent, left greater than right, temporal epileptiform sharp waves. Focal impaired aware seizures (FIAS), originating from both the left and right temporal head regions, were recorded.
4) Stereo EEG: Four left hippocampal onset FIAS and two right hippocampal onset FIAS were recorded.
5) Presentation at epilepsy surgery conference: Consensus recommendation for neuromodulation with bilateral mesial temporal RNS, ANT-DBS, or investigational study.
6) The patient was consented for the FDA-IDE (https://clinicaltrials.gov/study/NCT03946618) and underwent placement of Medtronic RC + S™ device with 4 leads (bilateral anterior nucleus of thalamus and bilateral amygdala-hippocampus).
As part of the FDA-IDE protocol, she was admitted to hospital epilepsy monitoring unit (EMU) for neurostimulator programming prior to initiation of long-term ambulatory stimulation. She tolerated multiple trial stimulation paradigms and did report a decrease in seizures with ANT 2 Hz stimulation, but continued COVID-19-related anxiety affected her appetite and mood, and continued irritation and stable dysphoria. A subsequent trial of high-frequency ANT-DBS (continuous 145 Hz, 90 PW, 3–5 mA) significantly exacerbated her COVID-19-related anxiety, anorexia, and dysphoria. Within a month of initiating continuous high-frequency ANT-DBS, she reported increasing anxiety and worsening insomnia and mood. She continued to lose weight. A trial of mirtazapine for her anxiety, insomnia, and decreased appetite was not helpful. She subsequently reported “daymares” associated with dissociative symptoms and a sense of inability to distinguish dreams from reality. Transition to duty cycle HF ANT-DBS (SANTE parameters) quickly improved her symptoms of insomnia, anxiety, daymares, dissociation, and difficulty distinguishing between dreaming and reality.
Subsequent trials of low frequency (2 Hz continuous 3 mA, 200 μs PW) were associated with decreased frequency and severity of seizures as well as subjective and clinically assessed improved mood and anxiety. She reports improvement in her sleep, although she believes her dreams have been disrupted. The patient continues to maintain stable mood, weight, and sleep (Kremen et al., 2024).
2.2 Patient 2
A 41-year-old, right-handed female with a long-standing history of epilepsy, major depression, and anxiety. She had no risk factors for epilepsy. At the age of 37 years old, she had an unprovoked generalized tonic clonic (GTC) from sleep. In the subsequent months, she developed FIAS with staring, behavioral arrest, and unresponsiveness occurring as often as weekly. With ASMs, she no longer had GTC but continued to have weekly FIAS. She also received a trial of immunotherapy after elevated GAD65 was identified in CSF and serum without benefit. She had a VNS implanted that decreased seizures by approximately 25%, but despite ASM and VNS optimization, she continued to have multiple FIAS each month.
Her mood symptoms began about 5–6 years before her initial seizure. Her anxiety revolved around concerns about her children, the future, her health, and seizures. Her depression was often accompanied by insomnia, decreased appetite, tearfulness, and hopelessness. She had no prior symptoms consistent with hypomania, mania, or psychosis although she had a history of a 5-day episode of hyperactive delirium during her sEEG monitoring which resolved with discontinuation of anticholinergic medications. Her neuropsychology testing showed deficits in delayed memory for both verbal and visual information, referable to bilateral mesial temporal dysfunction.
1) Laboratory studies were remarkable for elevated GAD-65 in serum and CSF.
2) MRI showed an increased T2 signal in the left hippocampus but with normal volumetric measurements.
3) EMU: Video-EEG monitoring showed bilateral independent, left greater than right, temporal epileptiform sharp waves. FIAS seizures originating from the left and right temporal head regions were recorded.
4) Stereo EEG: A single left hippocampal onset seizure was recorded. The evaluation was complicated by a period of agitation and psychosis requiring Haldol.
5) Presentation at epilepsy surgery conference: Consensus recommendation for neuromodulation with RNS or possibly ANT-DBS, but given the findings during sEEG, ANT stimulation was considered a higher risk of side effects. She was a possible candidate for left mesial temporal destructive procedures with laser interstitial thermal therapy (LITT) (Youngerman et al., 2023) or left anterior temporal lobectomy with amygdala-hippocampectomy (Wiebe et al., 2001). However, given the normal hippocampal volume and left-brain dominance the likely impact on verbal memory was not acceptable to the patient.
6) The patient was consented for FDA-IDE (https://clinicaltrials.gov/study/NCT03946618) and underwent placement of Medtronic RC + S™ device with 4 leads (bilateral anterior nucleus of thalamus and bilateral amygdala-hippocampus).
One month later as part of the FDA-IDE protocol, she was admitted for neurostimulator programming prior to initiation of long-term therapeutic ANT-DBS. With continuous 145 Hz stimulation, 4 mA, 90 µs cycling, the patient had an approximately 1-h episode with elevated respiratory rate, dyspnea, disorientation, and visual hallucinations. She became anxious and described a visual hallucination of being in a long room with many beds. She was considerably agitated and concerned about her family. She repeatedly called her husband, who helped calm her, but she continued to perseverate with concerns about her children. There was no change in the scalp EEG or the ANT and HPC recordings during the event, and this was different from her typical seizure semiology. Her stimulation was adjusted to continuous 2 Hz with the same current, and she rapidly improved. Later in the trial she also received duty-cycle high frequency ANT-DBS (SANTE parameters) without side effects.
With ANT-DBS, her seizure frequency decreased 90% from baseline and 50% from VNS, and she reported decreased seizure severity with both low and high frequency duty cycle ANT-DBS. She continues to report substantially improved functional status, and no worsening of depressive or anxiety symptoms throughout continued responsive ANT-DBS stimulation. No other episodes of psychosis reported. Each of her mood, memory, and sleep symptoms improved with extended time without seizures.
3 Discussion
High-frequency duty cycle ANT-DBS is a proven therapy for mTLE (R. Fisher et al., 2010). The precise neurobiological mechanisms for the effectiveness in reducing seizures remain poorly understood. Similarly, the mechanism(s) that underlie psychosis in TLE are not well understood (Nadkarni, Arnedo, and Devinsky, 2007). Here we were able to rule out the patients’ habitual mTLE seizures with continuous hippocampal LFP streaming (Figure 2).
New-onset psychiatric issues are not uncommon following ANT-DBS, with depression being the most common (R. Fisher et al., 2010; Salanova et al., 2015; Tröster et al., 2017). Interestingly, an early study even reported improvement in seizures and psychosis with ANT-DBS (Upton et al., 1985). In a study by Järvenpää et al. (Järvenpää et al., 2018), 2 of 22 patients (9%) developed psychosis following ANT-DBS that resolved with programming changes. Notably, there were no group-level declines in mood or memory status following ANT-DBS in the open-label follow-up of the SANTE cohort (Tröster et al., 2017).
The precise neurobiological mechanisms that underlie psychosis in TLE are not fully understood. Hypotheses include neurotransmitter imbalance, especially serotonin and dopamine, inflammatory cytokines, and genetic predispositions. High-frequency ANT-DBS desynchronizes HPC LFP activity (Stypulkowski et al., 2014; Yu et al., 2018) and fragments sleep (Voges et al., 2015; Szabó et al., 2022; Kremen et al., 2024) that could contribute to psychosis. Disorders of neural synchrony have been proposed as a mechanistic model for schizophrenia (Uhlhaas and Singer 2010). Additionally, the effect of ANT-DBS on sleep may be related to its impact on surrounding thalamic nuclei. In particular, HF stimulation of central laminar thalamus has been shown to promote arousal and would likely disrupt sleep (Redinbaugh et al., 2020). In the future, it may be possible to further dissect the underlying mechanism of ANT-DBS related psychosis.
In the current study, limiting high-frequency ANT-DBS to duty cycle paradigms (1 min on and 5 min off) appeared to be better tolerated. In the setting of electrical brain stimulation, it is essential to recognize psychiatric side effects early, adjust stimulation parameters, and consider pharmacologic interventions such as antipsychotic medications (de Toffol et al., 2018). It is often difficult to rule out the role of seizure activity in the development of psychosis in people with TLE. However, in this report with continuous LFP tracking and seizure detection we were able to demonstrate that seizures did not explain their psychosis.
In conclusion, ANT-DBS in people with mTLE is not uncommonly associated with exacerbation, or even new onset of psychiatric symptoms (Tröster et al., 2017). It is critical to quickly recognize the development of new symptoms or exacerbation of prior symptoms. Fortunately, here the symptoms were responsive to programming changes in these two patients.
Data availability statement
The original contributions presented in the study are included in the article/supplementary material, further inquiries can be directed to the corresponding authors.
Ethics statement
Written informed consent was obtained from the individual(s) for the publication of any potentially identifiable images or data included in this article.
Author contributions
LW: Writing–original draft, Writing–review and editing. SW: Writing–original draft, Writing–review and editing. IB: Writing–original draft, Writing–review and editing. JB: Writing–original draft, Writing–review and editing. DH: Writing–original draft, Writing–review and editing. PC: Writing–original draft, Writing–review and editing. SM: Writing–original draft, Writing–review and editing. JV: Writing–original draft, Writing–review and editing. KM: Writing–original draft, Writing–review and editing. VK: Writing–original draft, Writing–review and editing. GW: Writing–original draft, Writing–review and editing.
Funding
The author(s) declare that financial support was received for the research, authorship, and/or publication of this article. This work was supported by National Institutes of Health Grants UH2 and 3 NS095495, R01 NS112144 and U24 NS113637. The implanted devices were donated by Medtronic as part of the National Institutes of Health Brain Initiative.
Acknowledgments
We thank Cindy Nelson, Andrea Victoria, and Starr Guzman from Mayo Clinic for patient coordination; Abbey Becker, Dave Linde, and Scott Stanslaski from Medtronic for providing engineering support for the investigational RC+STM devices and the OpenMind team for providing community expertise and resources (https://openmind-consortium.github.io/).
Conflict of interest
Unrelated to this research GW and JVG. are inventors of intellectual property developed at Mayo Clinic and licensed to Cadence Neuroscience Inc. Mayo Clinic has received research support and consulting fees on behalf of GW from Cadence Neuroscience and Medtronic. GW is on the scientific advisory boards of LivaNova Inc., NeuroPace Inc., and Cadence Neuroscience Inc. PC has received research grant support from Neuronetics, NeoSync, and Pfizer; grant in kind (equipment support) from Assurex, MagVenture, and Neuronetics; and served as a consultant for Engrail Therapeutics, Myriad Neuroscience, Procter and Gamble, and Sunovion.
The remaining authors declare that the research was conducted in the absence of any commercial or financial relationships that could be construed as a potential conflict of interest.
Publisher’s note
All claims expressed in this article are solely those of the authors and do not necessarily represent those of their affiliated organizations, or those of the publisher, the editors and the reviewers. Any product that may be evaluated in this article, or claim that may be made by its manufacturer, is not guaranteed or endorsed by the publisher.
References
Baker, J., and Zeman, A. (2017). “Accelerated long-term forgetting in epilepsy—and beyond,” in Cognitive neuroscience of memory consolidation. Studies in neuroscience, psychology and behavioral economics. Editors N. Axmacher, and B. Rasch (Cham: Springer International Publishing), 401–417.
Baud, M. O., Kleen, J. K., Mirro, E. A., Andrechak, J. C., King-Stephens, D., Chang, E. F., et al. (2018). Multi-day Rhythms modulate seizure risk in epilepsy. Nat. Commun. 9 (1), 88. doi:10.1038/s41467-017-02577-y
Beenhakker, M. P., and Huguenard, J. R. (2009). Neurons that fire together also conspire together: is normal sleep circuitry hijacked to generate epilepsy? Neuron 62 (5), 612–632. doi:10.1016/j.neuron.2009.05.015
Bell, B., Lin, J. J., Seidenberg, M., and Hermann, B. (2011). The neurobiology of cognitive disorders in temporal lobe epilepsy. Nat. Rev. Neurol. 7 (3), 154–164. doi:10.1038/nrneurol.2011.3
Bell, M. L., Rao, S., So, E. L., Trenerry, M., Kazemi, N., Matt Stead, S., et al. (2009). Epilepsy surgery outcomes in temporal lobe epilepsy with a normal MRI. Epilepsia 50 (9), 2053–2060. doi:10.1111/j.1528-1167.2009.02079.x
Ben-Menachem, E. (2002). Vagus-nerve stimulation for the treatment of epilepsy. Lancet Neurol. 1 (8), 477–482. doi:10.1016/s1474-4422(02)00220-x
Ben-Menachem, E., Hellström, K., Waldton, C., and Augustinsson, L. E. (1999). Evaluation of refractory epilepsy treated with vagus nerve stimulation for up to 5 years. Neurology 52 (6), 1265–1267. doi:10.1212/wnl.52.6.1265
Berg, A. T. (2008). The natural history of mesial temporal lobe epilepsy. Curr. Opin. Neurology 21 (2), 173–178. doi:10.1097/WCO.0b013e3282f36ccd
Blake, R. V., Wroe, S. J., Breen, E. K., and McCarthy, R. A. (2000). Accelerated forgetting in patients with epilepsy: evidence for an impairment in memory consolidation. Brain A J. Neurology 123 (3), 472–483. doi:10.1093/brain/123.3.472
Bølling-Ladegaard, E., Dreier, J. W., Kessing, L. V., Budtz-Jørgensen, E., Lolk, K., and Christensen, J. (2023). Directionality of the association between epilepsy and depression: a nationwide register-based cohort study. Neurology 100 (9), e932–e942. doi:10.1212/WNL.0000000000201542
Bower, M. R., Kucewicz, M. T., St Louis, E. K., Meyer, F. B., Marsh, W. R., Stead, M., et al. (2017). Reactivation of seizure-related changes to interictal spike shape and synchrony during postseizure sleep in patients. Epilepsia 58 (1), 94–104. doi:10.1111/epi.13614
Bower, M. R., Stead, M., Bower, R. S., Kucewicz, M. T., Sulc, V., Cimbalnik, J., et al. (2015). Evidence for consolidation of neuronal assemblies after seizures in humans. J. Neurosci. 35 (3), 999–1010. doi:10.1523/JNEUROSCI.3019-14.2015
Boylan, L. S., Flint, L. A., Labovitz, D. L., Jackson, S. C., Starner, K., and Devinsky, O. (2004). Depression but not seizure frequency predicts quality of life in treatment-resistant epilepsy. Neurology 62 (2), 258–261. doi:10.1212/01.wnl.0000103282.62353.85
Child, N. D., and Eduardo, E. B. (2013). Anterior nucleus of the thalamus: functional organization and clinical implications. Neurology 81 (21), 1869–1876. doi:10.1212/01.wnl.0000436078.95856.56
Cooper, I. S., and Upton, A. R. M. (1985). Therapeutic implications of modulation of metabolism and functional activity of cerebral cortex by chronic stimulation of cerebellum and thalamus. Biol. Psychiatry 20, 811–813. doi:10.1016/0006-3223(85)90164-7
De Toffol, B., Trimble, M., Hesdorffer, D. C., Taylor, L., Sachdev, P., Clancy, M., et al. (2018). Pharmacotherapy in patients with epilepsy and psychosis. Epilepsy and Behav. 88, 54–60. doi:10.1016/j.yebeh.2018.09.001
Devinsky, O., Vezzani, A., O’Brien, T. J., Jette, N., Scheffer, I. E., Curtis, M. de, et al. (2018). Epilepsy. Nat. Rev. Dis. Prim. 4 (1), 18024. doi:10.1038/nrdp.2018.24
Durazzo, T. S., Spencer, S. S., Duckrow, R. B., Novotny, E. J., Spencer, D. D., and Zaveri, H. P. (2008). Temporal distributions of seizure occurrence from various epileptogenic regions. Neurology 70 (15), 1265–1271. doi:10.1212/01.wnl.0000308938.84918.3f
Elger, C. E. (2014). Epilepsy: lost in translation. Lancet Neurol. 13 (9), 862–863. doi:10.1016/S1474-4422(14)70125-5
Elger, C. E., and Hoppe, C. (2018). Diagnostic challenges in epilepsy: seizure under-reporting and seizure detection. Lancet Neurol. 17 (3), 279–288. doi:10.1016/S1474-4422(18)30038-3
Engel, J. (2001). Mesial temporal lobe epilepsy: what have we learned? Neurosci. A Rev. J. Bringing Neurobiol. Neurology Psychiatry 7 (4), 340–352. doi:10.1177/107385840100700410
Engel, J., Michael, P. M. D., Wiebe, S., Langfitt, J. T., Stern, J. M., Dewar, S., et al. (2012). Early surgical therapy for drug-resistant temporal lobe epilepsy: a randomized trial. JAMA J. Am. Med. Assoc. 307 (9), 922–930. doi:10.1001/jama.2012.220
Fisher, R., Salanova, V., Witt, T., Worth, R., Henry, T., Gross, R., et al. (2010). Electrical stimulation of the anterior nucleus of thalamus for treatment of refractory epilepsy. Epilepsia 51 (5), 899–908. doi:10.1111/j.1528-1167.2010.02536.x
Fisher, R. S. (2012). Therapeutic devices for epilepsy. Ann. Neurology 71 (2), 157–168. doi:10.1002/ana.22621
Fisher, R. S. (2023). “Deep brain stimulation (DBS) of thalamus for epilepsy,” in Neurostimulation for epilepsy: advances, applications and opportunities. Editor V. Rao (Academic Press), 133–159. (US).
Fisher, R. S., and Ana, L. V. (2014). Electrical brain stimulation for epilepsy. Nat. Rev. Neurol. 10 (5), 261–270. doi:10.1038/nrneurol.2014.59
Garg, D., Charlesworth, L., and Shukla, G. (2022). Sleep and temporal lobe epilepsy – associations, mechanisms and treatment implications. Front. Hum. Neurosci. 16, 849899. doi:10.3389/fnhum.2022.849899
Gregg, N., Nasseri, M., Kremen, V., Patterson, E., Sturges, B., Denison, T., et al. (2020). “Circadian and multiday seizure periodicities, and seizure clusters in canine epilepsy.” brain communications 2(1): fcaa008. G, divyani, laurel Charlesworth, and garima Shukla. 2022. “Sleep and temporal lobe epilepsy – associations, mechanisms and treatment implications.”. Front. Hum. Neurosci. 16. doi:10.3389/fnhum.2022.849899
Grewal, S. S., Middlebrooks, E. H., Kaufmann, T. J., Stead, M., Lundstrom, B. N., Worrell, G. A., et al. (2018). Fast gray matter acquisition T1 inversion recovery MRI to delineate the mammillothalamic tract for preoperative direct targeting of the anterior nucleus of the thalamus for deep brain stimulation in epilepsy. Neurosurg. Focus. 45 (2). doi:10.3171/2018.4.FOCUS18147
Grigg-Damberger, M., and Foldvary-Schaefer, N. (2021). Bidirectional relationships of sleep and epilepsy in adults with epilepsy. Epilepsy and Behav. E&B 116 (107735), 107735. doi:10.1016/j.yebeh.2020.107735
Helmstaedter, C., Winter, B., Melzer, N., Lohmann, H., and Witt, J.-A. (2019). Accelerated long-term forgetting in focal epilepsies with special consideration given to patients with diagnosed and suspected limbic encephalitis. Cortex; a J. Devoted Study Nerv. Syst. Behav. 110 (January), 58–68. doi:10.1016/j.cortex.2018.01.003
Hilger, E., Zimprich, F., Jung, R., Pataraia, E., Baumgartner, C., and Bonelli, S. (2013). Postictal psychosis in temporal lobe epilepsy: a case-control study. Eur. J. Neurology Official J. Eur. Fed. Neurological Soc. 20 (6), 955–961. doi:10.1111/ene.12125
Hogan, R. E., and Kitti, K. (2003). The ‘dreamy state’: john hughlings-jackson’s ideas of epilepsy and consciousness. Am. J. Psychiatry 160 (10), 1740–1747. doi:10.1176/appi.ajp.160.10.1740
Horak, P. C., Meisenhelter, S., Song, Y., Testorf, M. E., Kahana, M. J., Viles, W. D., et al. (2017). Interictal epileptiform discharges impair word recall in multiple brain areas. Epilepsia 58 (3), 373–380. doi:10.1111/epi.13633
Hughlings-Jackson, J. (1888). On a Particular Variety of Epilepsy (‘Intellectual Aura’), One Case With Symptoms of Organic Brain Disease. Brain A J. Neurology 11 (2), 179–207. doi:10.1093/brain/11.2.179
Institute of Medicine (US) Committee on the Public Health Dimensions of the Epilepsies (2012). in Epilepsy across the spectrum: promoting health and understanding. Editors M. J. England, C. T. Liverman, A. M. Schultz, and L. M. Strawbridge (Washington, DC: National Academies Press US).
Järvenpää, S., Rosti-Otajärvi, E., Rainesalo, S., Laukkanen, L., Lehtimäki, K., and Peltola, J. (2018). Executive functions may predict outcome in deep brain stimulation of anterior nucleus of thalamus for treatment of refractory epilepsy. Front. Neurology 9, 324. doi:10.3389/fneur.2018.00324
Järvenpää, S., Rosti-Otajärvi, E., Lehtimäki, K., Rainesalo, S., Möttönen, T., and Peltola, J. (2020). Improving the effectiveness of ANT DBS therapy for epilepsy with optimal current targeting. Epilepsia Open 5, 406–417. doi:10.1002/epi4.12407
Johnson, E. K., Jones, J. E., Seidenberg, M., and Hermann, B. P. (2004). The relative impact of anxiety, depression, and clinical seizure features on health-related quality of life in epilepsy. Epilepsia 45 (5), 544–550. doi:10.1111/j.0013-9580.2004.47003.x
Kanner, A. M. (2009). Psychiatric issues in epilepsy: the complex relation of mood, anxiety disorders, and epilepsy. Epilepsy and Behav. E&B 15 (1), 83–87. doi:10.1016/j.yebeh.2009.02.034
Kanner, A. M., Ribot, R., and Mazarati, A. (2018). Bidirectional relations among common psychiatric and neurologic comorbidities and epilepsy: do they have an impact on the course of the seizure disorder? Epilepsia Open 3 (S2), 210–219. doi:10.1002/epi4.12278
Karoly, P. J., Rao, V. R., Gregg, N. M., Worrell, G. A., Bernard, C., Cook, M. J., et al. (2021). Cycles in epilepsy. Nat. Rev. Neurol. 17 (5), 267–284. doi:10.1038/s41582-021-00464-1
Kleen, J. K., Scott, R. C., Holmes, G. L., Roberts, D. W., Rundle, M. M., Testorf, M., et al. (2013). Hippocampal interictal epileptiform activity disrupts cognition in humans. Neurology 81 (1), 18–24. doi:10.1212/WNL.0b013e318297ee50
Kremen, V., Brinkmann, B. H., Kim, I., Guragain, H., Nasseri, M., Magee, A. L., et al. (2018). Integrating brain implants with local and distributed computing devices: a next generation epilepsy management system. IEEE J. Transl. Eng. Health Med. 6, 2500112. doi:10.1109/JTEHM.2018.2869398
Kremen, V., Sladky, V., Mivalt, F., Gregg, N. M., Balzekas, I., Marks, V., et al. (2024). A platform for brain network sensing and stimulation with quantitative behavioral tracking: application to limbic circuit epilepsy. medRxiv, 02 (09), 24302358. doi:10.1101/2024.02.09.24302358
Kwan, P., Schachter, S. C., and Brodie, M. J. (2011). Drug-resistant epilepsy. New England J. Med. 365 (10), 919–926. doi:10.1056/NEJMra1004418
Lehnertz, K., Bröhl, T., and Wrede, R. V. (2023). Epileptic-network-based prediction and control of seizures in humans. Neurobiol. Dis. 181, 106098. doi:10.1016/j.nbd.2023.106098
Lemesle, B., Barbeau, E. J., Rigal, E. M., Denuelle, M., Valton, L., and Pariente, Jérémie (2021). Hidden objective memory deficits behind subjective memory complaints in patients with temporal lobe epilepsy. Neurology 98 (8), e818–e828. doi:10.1212/WNL.0000000000013212
Leutmezer, F., Podreka, I., Asenbaum, S., Pietrzyk, U., Lucht, H., and Back, Claude (2003). Postictal psychosis in temporal lobe epilepsy. Epilepsia 44 (4), 582–590. doi:10.1046/j.1528-1157.2003.32802.x
Lundstrom, B., and Gregg, N. (2023). What should we expect for real-world outcomes of deep brain stimulation of the anterior nucleus of the thalamus for epilepsy. Neurology 100 (18), 845–846. doi:10.1212/WNL.0000000000201455
MacLean (1949). Psychosomatic disease and the visceral brain; recent developments bearing on the Papez theory of emotion. Psychosom. Med. 11 (6), 338–353. doi:10.1097/00006842-194911000-00003
Matsumoto, J. Y., Stead, M., Kucewicz, M. T., Matsumoto, A. J., Peters, P. A., Brinkmann, B. H., et al. (2013). Network oscillations modulate interictal epileptiform spike rate during human memory. Brain A J. Neurology 136 (Pt 8), 2444–2456. doi:10.1093/brain/awt159
McAuley, J. W., Elliott, J. O., Patankar, S., Hart, S., Long, L., Layne Moore, J., et al. (2010). Comparing patients’ and practitioners’ views on epilepsy concerns: a call to address memory concerns. Epilepsy and Behav. E&B 19 (4), 580–583. doi:10.1016/j.yebeh.2010.09.001
McIntyre, D. C., Kelly, M. E., and Staines, W. A. (2006). “Preface: material-specific and generalized memory loss.” In: C. G. Wasterlain, and D. M. Treiman (eds) Status epilepticus Mech. Manag. MIT Press Camb. MA, pp229–238.
Milner, B. (1998). “Preface: material-specific and generalized memory loss.” Neuropsychologia 6 (3): 175–179. doi:10.1016/0028-3932(68)90017-1
Mivalt, F., Kremen, V., Sladky, V., Balzekas, I., Nejedly, P., Gregg, N. M., et al. (2022). Electrical brain stimulation and continuous behavioral state tracking in ambulatory humans. J. Neural Eng. 19 (1), 016019. doi:10.1088/1741-2552/ac4bfd
Mivalt, F., Kremen, V., Sladky, V., Cui, J., Gregg, N. M., Balzekas, I., et al. (2023). Impedance Rhythms in human limbic system. J. Neurosci. Official J. Soc. Neurosci. 43 (39), 6653–6666. doi:10.1523/JNEUROSCI.0241-23.2023
Moore, J. L., Carvalho, D. Z., St Louis, E. K., and Bazil, C. (2021). Sleep and epilepsy: a focused review of pathophysiology, clinical syndromes, Co-morbidities, and therapy. Neurother. J. Am. Soc. Exp. Neurother. 18 (1), 170–180. doi:10.1007/s13311-021-01021-w
Morrell, M. J.RNS System in Epilepsy Study Group (2011). Responsive cortical stimulation for the treatment of medically intractable partial epilepsy. Neurology 77 (13), 1295–1304. doi:10.1212/WNL.0b013e3182302056
Morrell, M. J. (2014). In response: the RNS System multicenter randomized double-blinded controlled trial of responsive cortical stimulation for adjunctive treatment of intractable partial epilepsy: knowledge and insights gained. Epilepsia 55, 1470–1471. doi:10.1111/epi.12736
Nadkarni, S., Arnedo, V., and Devinsky, O. (2007). Psychosis in epilepsy patients. Epilepsia 48 (Suppl. 9), 17–19. doi:10.1111/j.1528-1167.2007.01394.x
Osorio, I. (2014). The NeuroPace trial: missing knowledge and insights. Epilepsia 55 (9), 1469–1470. doi:10.1111/epi.12701
Papez, J. W. (1937). A proposed mechanism of emotion. Archives Neurology Psychiatry 38 (4), 725. doi:10.1001/archneurpsyc.1937.02260220069003
Paré, D., and Headley, D. (2023). The amygdala mediates the facilitating influence of emotions on memory through multiple interacting mechanisms. Neurobiol. Stress 24, 100529. doi:10.1016/j.ynstr.2023.100529
Redinbaugh, M. J., Phillips, J. M., Kambi, N. A., Mohanta, S., Andryk, S., Dooley, G. L., et al. (2020). Thalamus modulates consciousness via layer-specific control of cortex. Neuron 106 (1), 66–75. doi:10.1016/j.neuron.2020.01.005
Salanova, V., Sperling, M. R., Gross, R. E., Irwin, C. P., Vollhaber, J. A., Giftakis, J. E., et al. (2021). The SANTÉ study at 10 years of follow-up: effectiveness, safety, and sudden unexpected death in epilepsy. Epilepsia 62 (6), 1306–1317. doi:10.1111/epi.16895
Salanova, V., Witt, T., Worth, R., Henry, T. R., Gross, R. E., Nazzaro, J. M., et al. (2015). Long-term efficacy and safety of thalamic stimulation for drug-resistant partial epilepsy. Neurology 84 (10), 1017–1025. doi:10.1212/WNL.0000000000001334
Sladky, V., Nejedly, P., Mivalt, F., Brinkmann, B. H., Kim, I., St. Louis, E. K., et al. (2022). Distributed brain Co-processor for tracking spikes, seizures and behaviour during electrical brain stimulation. Brain Commun. 4 (3), fcac115. doi:10.1093/braincomms/fcac115
Spencer, D. D., Gerrard, J. L., and Zaveri, H. P. (2018). The roles of surgery and technology in understanding focal epilepsy and its comorbidities. Lancet Neurol. 17, 373–382. doi:10.1016/S1474-4422(18)30031-0
Squire, L. R. (2009). The legacy of patient H.M. For neuroscience. Neuron 61 (1), 6–9. doi:10.1016/j.neuron.2008.12.023
Squire, L. R., and Zola-Morgan, S. (1991). The medial temporal lobe memory system. Sci. (New York, N.Y.) 253 (5026), 1380–1386. doi:10.1126/science.1896849
Stypulkowski, P. H., Stanslaski, S. R., Jensen, R. M., Denison, T. J., and Giftakis, J. E. (2014). Brain stimulation for epilepsy--local and remote modulation of network excitability. Brain Stimul. 7 (3), 350–358. doi:10.1016/j.brs.2014.02.002
Szabó, J. P., Fabó, D., Pető, N., Sákovics, A., and Bódizs, R. (2022). Role of anterior thalamic circuitry during sleep. Epilepsy Res. 186 (106999), 106999. doi:10.1016/j.eplepsyres.2022.106999
Tellez-Zenteno, J. F., Patten, S. B., Jetté, N., Williams, J., and Wiebe, S. (2007). Psychiatric comorbidity in epilepsy: a population-based analysis. Epilepsia 48 (12), 2336–2344. doi:10.1111/j.1528-1167.2007.01222.x
Temkin, O. (1994). The falling sickness: a history of epilepsy from the Greeks to the beginnings of modern neurology (softshell books). 2nd ed. Baltimore, MD: Johns Hopkins University Press.
Tröster, A. I., Meador, K. J., Irwin, C. P., and Fisher, R. S.SANTE Study Group (2017). Memory and mood outcomes after anterior thalamic stimulation for refractory partial epilepsy. Seizure 45, 133–141. doi:10.1016/j.seizure.2016.12.014
Upton, A. R., Cooper, I. S., Springman, M., and Amin, I. (1985). Suppression of seizures and psychosis of limbic system origin by chronic stimulation of anterior nucleus of the thalamus. Int. J. Neurology 19-20, 223–230.
Velasco, F., Velasco, M., Ogarrio, C., and Fanghanel, G. (1987). Electrical stimulation of the centromedian thalamic nucleus in the treatment of convulsive seizures: a preliminary report. Epilepsia 28, 421–430. doi:10.1111/j.1528-1157.1987.tb03668.x
Voges, B. R., Schmitt, F. C., Hamel, W., House, P. M., Kluge, C., Moll, C. K. E., et al. (2015). Deep brain stimulation of anterior nucleus thalami disrupts sleep in epilepsy patients. Epilepsia 56 (8), e99–e103. doi:10.1111/epi.13045
Wiebe, S., Blume, W. T., Girvin, J. P., and Eliasziw, M.Effectiveness and Efficiency of Surgery for Temporal Lobe Epilepsy Study Group (2001). A randomized, controlled trial of surgery for temporal-lobe epilepsy. New England J. Med. 345 (5), 311–318. doi:10.1056/NEJM200108023450501
Worrell, G., and Gotman, J. (2011). High-frequency oscillations and other electrophysiological biomarkers of epilepsy: clinical studies. Biomarkers Med. 5 (5), 557–566. doi:10.2217/bmm.11.74
Youngerman, B. E., Banu, M. A., Khan, F., McKhann, G. M., Schevon, C. A., Jagid, J. R., et al. (2023). Long-term outcomes of mesial temporal laser interstitial thermal therapy for drug-resistant epilepsy and subsequent surgery for seizure recurrence: a multi-centre cohort study. J. Neurology, Neurosurg. Psychiatry 94 (11), 879–886. doi:10.1136/jnnp-2022-330979
Keywords: limbic network, Epilepsy, seizure, psychosis, ANT-DBS
Citation: Wheeler L, Worrell SE, Balzekas I, Bilderbeek J, Hermes D, Croarkin P, Messina S, Van Gompel J, Miller KJ, Kremen V and Worrell GA (2024) Case report: Bridging limbic network epilepsy with psychiatric, memory, and sleep comorbidities: case illustrations of reversible psychosis symptoms during continuous, high-frequency ANT-DBS. Front. Netw. Physiol. 4:1426743. doi: 10.3389/fnetp.2024.1426743
Received: 02 May 2024; Accepted: 16 July 2024;
Published: 08 August 2024.
Edited by:
Klaus Lehnertz, University of Bonn, GermanyReviewed by:
Andreas Schulze-Bonhage, University of Freiburg Medical Center, GermanyPål Gunnar Larsson, Oslo University Hospital, Norway
Copyright © 2024 Wheeler, Worrell, Balzekas, Bilderbeek, Hermes, Croarkin, Messina, Van Gompel, Miller, Kremen and Worrell. This is an open-access article distributed under the terms of the Creative Commons Attribution License (CC BY). The use, distribution or reproduction in other forums is permitted, provided the original author(s) and the copyright owner(s) are credited and that the original publication in this journal is cited, in accordance with accepted academic practice. No use, distribution or reproduction is permitted which does not comply with these terms.
*Correspondence: Lydia Wheeler, d2hlZWxlci5seWRpYUBtYXlvLmVkdQ==; Gregory A. Worrell, d29ycmVsbC5ncmVnb3J5QG1heW8uZWR1