- 1Human Performance Laboratory, Department of Sport and Exercise Sciences, Barry University, Miami Shores, FL, United States
- 2Keck Laboratory for Network Physiology, Department of Physics, Boston University, Boston, MA, United States
- 3Clinical Muscle Biology Laboratory, Department of Biology, Baylor University, Waco, TX, United States
Introduction: High-intensity interval exercise (HIIE) is deemed effective for cardiovascular and autonomic nervous system (ANS) health-related benefits, while ANS disturbance increases the risk for cardiovascular disease (CVD). Postprandial lipemia and acute-partial sleep deprivation (APSD) are considered as CVD risk factors due to their respective changes in ANS. Exercising in the morning hours after APSD and have a high-fat breakfast afterwards may alter the interactions of the cardiovascular, autonomic regulation, and postprandial lipemic systems threatening individuals’ health. This study examined postprandial network interactions between autonomic regulation through heart rate variability (HRV) and lipemia via low-density lipoprotein (LDL) cholesterol in response to APSD and HIIE.
Methods: Fifteen apparently healthy and habitually good sleepers (age 31 ± 5.2 SD yrs) completed an acute bout of an isocaloric HIIE (in form of 3:2 work-to-rest ratio at 90 and 40% of VO2 reserve) after both a reference sleep (RSX) and 3–3.5 h of acute-partial sleep deprivation (SSX) conditions. HRV time and frequency domains and LDL were evaluated in six and seven time points surrounding sleep and exercise, respectively. To identify postprandial network interactions, we constructed one correlation analysis and one physiological network for each experimental condition. To quantify the interactions within the physiological networks, we also computed the number of links (i.e., number of significant correlations).
Results: We observed an irruption of negative links (i.e., negative correlations) between HRV and LDL in the SSX physiological network compared to RSX. Discussion: We recognize that a correlation analysis does not constitute a true network analysis due to the absence of analysis of a time series of the original examined physiological variables. Nonetheless, the presence of negative links in SSX reflected the impact of sleep deprivation on the autonomic regulation and lipemia and, thus, revealed the inability of HIIE to remain cardioprotective under APSD. These findings underlie the need to further investigate the effects of APSD and HIIE on the interactions among physiological systems.
1 Introduction
A plethora of data suggest the cardioprotective effects of exercise due to its impact either on reducing the associated to cardiovascular disease risk factors (e.g., hypertension, lipidemia, diabetes, and insulin resistance, obesity) or having a direct effect on processes and functions of different physiological systems (e.g., atherosclerotic process and cardiovascular function) (Powers et al., 2002; Phrommintikul et al., 2022). Moreover, exercise presents cardioprotective effects due to autonomic nervous system (ANS) adjustments on heart rate variability (HRV) and baroreflex sensitivity (Grant et al., 2012; Subramanian et al., 2019). High-intensity interval exercise (HIIE), exercise performed in brief, successive intervals consisting of a period of high-intensity (e.g., >80% of peak oxygen consumption) followed by lower-intensity recovery periods, is proposed as alternative time-efficient exercise method with wide health related benefits during both fasting and postprandial states (Gibala, 2007; Wisloff et al., 2007; Tjonna et al., 2008; Tyldum et al., 2009; Gibala et al., 2012; Weston et al., 2014; Bond et al., 2015; Sawyer et al., 2016; Ramírez-Vélez et al., 2018; Tucker et al., 2018). The protective cardiometabolic effect of HIIE is postulated due to greater induced antioxidant status both during and after HIIE (Harris et al., 2008; Di Francescomarino et al., 2009; Fisher-Wellman and Bloomer, 2009; Tyldum et al., 2009; Gabriel et al., 2012). On top of that, HIIE has a significantly greater impact on ANS (Gibala et al., 2012; Bhati and Moiz, 2017), with HIIE work to rest (W:R) ratio of 1:2 to be proposed as highly effective for cardiovascular and autonomic related health benefits (Heydari et al., 2013; Ramírez-Vélez et al., 2016; Ramirez-Velez et al., 2020). It has been shown that ANS disturbance due to exercise, presented as increased sympathetic tone and parasympathetic withdrawal leads to a decreased HRV, which in turn increases the risk for cardiovascular disease (CVD) (Besnier et al., 2017). HIIE affects simultaneously the interactions among physiological systems and organs where the strength of these interactions may represent different physiological states and pathological conditions. Such pathological conditions may be manifested due to failure of the system to perform various coupling and feedback interactions under an integrated physiological system with linear and non-linear characteristics (Bashan et al., 2012; Ivanov and Bartsch, 2014; Bartsch et al., 2015).
Postprandial lipemia is an independent risk factor for CVD (Hyson et al., 2003; Cromwell et al., 2007). Postprandial increased concentration of low-density (LDL) lipoprotein cholesterol induces a heightened inflammatory state in the vascular wall that is highly susceptible to oxidative changes (Littlefield and Grandjean, 2015) promoting vascular endothelial dysfunction (Wallace et al., 2010). In apparently healthy men, LDL cholesterol had inverse relation to HRV (Kupari et al., 1993; Christensen et al., 1999), which is a marker of ANS activation and linked to CVD (Thayer et al., 2010; Chung et al., 2020).
Short sleep duration has been linked to increased risks of morbidity and mortality (Hale et al., 2020; Krittanawong et al., 2020). Acute partial sleep deprivation (APSD), of less than 5 h of sleep, is associated to CVD (Tobaldini et al., 2017; Liew and Aung, 2021). This association to CVD is attributed to changes in autonomic nervous system (ANS) (Miller and Cappuccio, 2013; Tobaldini et al., 2014; Wright et al., 2015; Tobaldini et al., 2017; American Sleep Association, 2018; Seravalle et al., 2018; Liu and Chen, 2019; Oliver et al., 2020). Under APSD such detrimental health effects may represent the breakdown of dynamic network interactions among organs systems and metabolic processes, such as ANS and postprandial lipemia and their failure to ensure a healthy vital status (Bartsch et al., 2015; Ivanov et al., 2016; Balague et al., 2020; Lehnertz et al., 2020). It seems that after sleep deprivation the pronounced HR and HRV reduction reflect the inability of the cardiovascular system to respond and adapt to such a trigger (Zhong et al., 2005; Sauvet et al., 2010). When there is failure in the interaction and coordination between the parasympathetic activity (PA) withdrawal and decreased total HRV (i.e., decreased high frequency, increased low frequency, increased low frequency/high frequency ratio) then sleep deprivation may pose as a risk factor for CVD (Zhong et al., 2005; Tobaldini et al., 2014; Johnston et al., 2020).
It is possible that APSD prior to exercise to mask the intended health benefits of HIIE (Gibala, 2007; Wisloff et al., 2007; Harris et al., 2008; Tjonna et al., 2008; Di Francescomarino et al., 2009; Fisher-Wellman and Bloomer, 2009; Tyldum et al., 2009; Gabriel et al., 2012; Gibala et al., 2012; Heydari et al., 2013; Weston et al., 2014; Bond et al., 2015; Ramírez-Vélez et al., 2016; Sawyer et al., 2016; Ramírez-Vélez et al., 2018; Tucker et al., 2018; Ramirez-Velez et al., 2020), especially for those who are regular good sleepers particularly as it is related to postprandial HRV and cardiometabolic health (Christensen et al., 1999; Gielen and Hambrecht, 2005; Mestek et al., 2006; Mestek et al., 2008; Gielen et al., 2010; Gielen et al., 2011; Chung et al., 2020). Research questions related to the immediate HRV responses after acute HIIE following reference sleep and APSD are still under investigation. Previous work from our lab has examined such research questions (Papadakis et al., 2020; Papadakis et al., 2021a; Papadakis et al., 2021b) under the traditional Exercise Physiology approach, which focuses on a single physiological system by reducing complex multicomponent systems on their respective parts (Machamer et al., 2000; Bechtel and Richardson, 2010; Balague et al., 2020). We reported that the expected HRV disturbance as a response to an acute HIIE was not influenced by APSD (Papadakis et al., 2021a), HIIE after APSD was still cardioprotective for the postprandial endothelial function (Papadakis et al., 2020), and lastly that fasted HIIE and performance were not affected by sleep conditions (Papadakis et al., 2021b).
The human organism though, is comprised by multicomponent physiological systems that operate through non-linear feedback mechanisms at several spatio-temporal scales generating complex dynamics that continuously adapt to various intrinsic and extrinsic stimuli (Bashan et al., 2012; Ivanov and Bartsch, 2014; Bartsch et al., 2015). Accordingly, exercising in the morning hours after APSD may impact negatively interactions among physiological systems (e.g., cardiovascular and ANS) and, therefore, generate a differentiated network of physiological interactions compared to exercising after a full night sleep. It is possible, that many people after exercise, may consume a typical American high-fat convenience breakfast (Bourland and Vogt, 2009; Lang et al., 2014), a behavior that adds another level in the complex interactions between the aforementioned physiological systems. Such behavior, may disrupt the network interactions among the cardiovascular, autonomic regulation, and postprandial lipemic systems ultimately jeopardizing their health.
Therefore, this paper attempts to revisit our previous work (Papadakis et al., 2020; Papadakis et al., 2021a; Papadakis et al., 2021b), through the prism of Network Physiology of Exercise (NPE) (Balague et al., 2020), a new branch of the interdisciplinary field of Network Physiology (Ivanov et al., 2016; Ivanov, 2021; Ivanov et al., 2021). NPE addresses the fundamental question of how physiological systems coordinate and synchronize their dynamics as a network to optimize organism function, and how these network interactions change in response to exercise and training. NPE utilizes novel methods and approaches in Network Theory, Nonlinear Dynamics, Computational and Statistical Physics, and Biomedical Informatics to represent localized integrated organ systems and their interactions across various scales with the respective nodes (examined variables) and edges/links (respective interactions) in their dynamic network (Bartsch et al., 2014; Ivanov and Bartsch, 2014; Bartsch et al., 2015; Ivanov et al., 2016; Ivanov et al., 2017; Balague et al., 2020; Lehnertz et al., 2020; Meyer, 2020; Rizzo et al., 2020). Accordingly, we aimed to examine the postprandial network interactions between autonomic regulation through HRV and lipemia through low-density lipoprotein (LDL) cholesterol in response to APSD and HIIE.
2 Materials and Methods
2.1 Study Design and Participants
As stated, this paper is revisiting data collected as part of a bigger project that involved parameters related to sleep, exercise, and cardiovascular function and outcomes of these investigations presented in detail elsewhere (Papadakis et al., 2020; Papadakis et al., 2021a; Papadakis et al., 2021b). Briefly, a within-subject randomized crossover experimental design with three 3) experimental conditions (i.e., a reference sleep—no exercise “control condition” (RS) in which a standardized test meal was ingested in the morning after 9–9.5 h of time-in-bed in which at least 8 h of sleep was attained; a “reference sleep and high-intensity interval exercise condition” (RSX), similar to RS condition in terms of the meal and the obtained sleep time with the exception of a high-intensity interval exercise with 3:2 intervals at 90 and 40% of VO2 reserve that averaged 70% of VO2 reserve and expended 500 kcals of energy, and; a “short and disrupted sleep and high-intensity interval exercise—acute partial sleep deprivation condition” (SSX), similar to RSX in terms of the meal and the performed exercise with the exception of the sleep time that was regulated to 3–3.5 h of time-in-bed limited to no more than 3.5 h of sleep) was employed to answer the research questions as depicted earlier (Figure 1). All experimental conditions began after 48 h of controlling activities of daily living, medication use, standardized diet to what the individuals consumed during the first experimental condition, and supplementation of any kind with a minimum 72-h and maximum 2 weeks washout period between each condition. Thirty healthy males (25–55 years) with normal and overweight body mass index (BMI) met the following inclusion criteria: 1) being recreationally physically active, but not engaging in training for long-distance endurance events, 2) non-smokers, 3) not taking any medications known to alter blood pressure, lipidemic and glucose profile, and 4) not taking any medications known to alter sleep. Participants had to be “good” sleepers as indicated by a score of ≤5 on the Pittsburgh Sleep Quality Index (PSQI) (Buysse et al., 1989). Study was approved by the Institutional Review Board and performed in agreement to the Declaration of Helsinki with all participants having read and signed an informed consent form prior to participation.
Experimental conditions completed on two consecutive days and began after 48 h of physical inactivity, no medication use, and the consumption of a diet standardized to what the individual consumed during the first intervention and free from supplementation of any kind. Conditions involved one pre-sleep standard meal consumed in the evening of the first experimental day, six hear rate variability (HRV) recordings, and a morning standard meal surrounding the sleep and exercise interventions. Conditions included: 1) a reference sleep—no exercise “control condition” (RS) in which a standardized test meal was ingested in the morning after 9–9.5 h of time-in-bed in which at least 8 h of sleep was attained) a “reference sleep and high-intensity interval exercise condition” (RSX) in which the test meal was ingested in the morning after reference sleep and after a session of high-intensity interval exercise (3:2 intervals at 90 and 40% of VO2 reserve that average 70% of VO2 reserve) to expend 500 kcals of energy, and; 3) a “short and disrupted sleep and high-intensity interval exercise condition—acute partial sleep deprivation” (SSX) in which an experimental test meal was ingested in the morning after 3–3.5 h of time-in-bed limited to no more than 3.5 h of sleep and after a session of high-intensity interval exercise to expend 500 kcals of energy. Participants arrived at 7p.m. at the laboratory and stayed around 8:30p.m. During this time blood was collect (indicated by the syringe) and an HRV measurement was taken before the evening meal. We discharged participants from the lab accounting for commuting time and bed-preparation time so at 9p.m. all to be in bed. Participants stayed in their homes until the awake time that it was set at 6:00a.m. During this time only data from Sensewear were collected to verify the sleep duration. Participants had to be at the lab at 7:00a.m. the next day. Between 7 and 9a.m., another blood draw was performed and a HRV measurement was taken around 7:00a.m., followed by the exercise condition and another blood draw immediate post-exercise. Around 8:30–9:00a.m. the morning meal was provided followed by another blood draw and a HRV measurement. Every 2 hours post-exercise a blood draw was performed and an HRV measurement was taken until round 3:00p.m.
2.2 Pre-Experimental Conditions
2.2.1 Body Composition and Cardiovascular Fitness
Preliminary measurement of participants’ body composition via dual-energy X-ray absorptiometry (DXA) (Discovery DXA™, Hologic®, Bedford, MA) was performed. After that, an individualized maximal graded exercise test using a modified ramped treadmill protocol to determine participants’ cardiovascular fitness (VO2) via collection of respiratory gases (TrueOne 2400™, ParvoMedics®, Sandy, UT) was executed. Results of this test were used to calculate the experimental exercise intensities as illustrated in Figure 1 (i.e., 3:2 intervals at 90 and 40% of VO2 reserve that average 70% of VO2 reserve; VO2 reserve was calculated as (VO2 max—VO2 rest) X % intensity + VO2 rest; with VO2 rest to be 3.5 ml/kg/min) (American College of Sports Medicine, 2013), and also to familiarize participants with exercise intervals to ascertain their comfort during the experimental conditions.
2.2.2 Sleep and Physical Activity Monitor—Diet Records
On top of using the PSQI scale to identify the “regular good sleepers”, participants’ sleep was monitored by the Sense Wear armband (Sense Wear™, Body Media®, Pittsburgh, PA), which is a validated method to assess both sleep and physical activity parameters (Almeida et al., 2011; Van Wouwe et al., 2011; Sharif and Bahammam, 2013; Soric et al., 2013; Shin et al., 2015). Participants had to wear the monitor on their non-dominant arm for 23 h/day each day, for 1 week prior to experimental conditions and for 2 days leading up to experimental conditions. Information from the monitor was used to characterize participants’ sleep duration, sleep consistency, sedentary time, levels of physical activity and to ensure the homogeneity of study’s sample in terms of sleep and physical activity patterns. Moreover, participants’ diet was controlled as they were asked 2 days prior and during the experimental conditions to maintain their typical dietary habits and consume food that were easily reproducible. Dietary intake and macronutrient composition was analyzed using the ChooseMyPlate® (U.S. Department of Agriculture, Washington, DC). Ensuring a stable diet, sleep, and physical activity patterns-habits was paramount to reduce their respective influence on changes in the dependent variables.
2.3 Experimental Conditions
2.3.1 Standard Evening Meal
The standardized consumed evening meal of day 1 (∼805 kcal) was turkey and cheese sandwich on whole grain bread, a medium banana, a 150 g cup of Greek yogurt, and a 24 oz Gatorade® drink. It was the last meal that all participants had from 7p.m. until 9p.m., before they went to bed and until the following morning. Participants remained at the lab until they returned to their residence to go to sleep.
2.3.2 Sleep
Participants completed all the sleep elements of the study at their residence in order to eliminate any disturbances that may occurred if they slept in an unfamiliar laboratory place. Per research design, the RS and RSX conditions allowed for 9.5 h of time-in-bed in the hopes that at least ≥8 h of sleep would have achieved. In the SSX, the research design allowed for 3.5 h of time-in-bed limiting the sleep to ≤3 h. Researchers instructed participants to return to their residence once they left the lab, as all the experimental conditions were calculated to allow enough time for commute and sleep preparation/hygiene routine. No other food was allowed, no watching television neither engaging in computer activities were allowed as participants were preparing for sleep. Participants had also to record both the time that they entered the bed and the time they woke up.
2.3.3 High-Intensity Interval Exercise
High-intensity interval exercise sessions were performed on Trackmaster® TMX 428CP treadmill. Following the research design, sessions began at least 10–12 h after the evening meal and completed 1 h before the test meal. After a 5-min warmup at 2 mph and 0% grade, the HIIE sessions were completed in 3-min running intervals at 90% of VO2 reserve separated by 2-min intervals of jogging/walking at 40% of VO2 reserve until 500 kcal were expended. The average intensity of all HIIE sessions was equated to 70% of VO2 reserve. The applied HIIE protocol of 3:2 min work to rest ratio was a modified one from previous studies (Kaikkonen et al., 2008; Matsuo et al., 2014; Osuka et al., 2017; Ito, 2019).
2.3.4 Standard Morning Meal
A standard commercially available meal was provided to participants 60 min after completing the HIIE sessions of day 2. The meal included a Jimmy Dean® sausage, egg, and cheese biscuit (∼410 kcals; 29 g fat; 26 g carbohydrate; 11.5 g protein); a Jimmy Dean® fully-cooked pork sausage patty (∼270 kcals; 24 g fat; 2 g carbohydrate; 10.5 g protein); a Little Debbie® honey bun (∼483 kcals; 27 g fat; 55 g carbohydrate; 5.5 g protein), and; a cup of whole milk (∼146 kcals; 8 g fat; 11 g carbohydrate; 7.5 g protein). The test meal had a total of 1,309 kcals (88 g fat; 94 g carbohydrate; 35 g protein). For the RS condition of day 2, the meal was provided 60 min after their arrival at the lab and matched their respective HIIE sessions.
2.3.5 Cardiac Autonomic Regulation—Heart Rate Variability
A standardized HRV protocol and methodology for circadian influence on cardiac autonomic assessment was followed as previously described (Malik, 1996; Sammito and Böckelmann, 2016; Riganello et al., 2019; Johnston et al., 2020). Participants after being in supine position for 10 min in a quiet and temperature-controlled environment (∼21–24°C and 40–60% relative humidity), heart rate (R-R intervals) using a Polar belt (FT1™, Polar Wearlink® Lake Success, NY) was recorded for 5 min at a sampling rate of 1,000 Hz. We used this heart rate recording to calculate the R-R interval and obtain the related heart rate variability indices as previously described (Achten and Jeukendrup, 2003; Engström et al., 2012; Buchheit, 2014). Cardiac autonomic modulation through HRV was assessed the night before (D1), the morning of the next day (D2), 0, 2, 4, and 6-h post-exercise (PE) (Figure 1). We followed previously published guidelines to reduce anxiety of measurement and control for recording errors (Malik, 1996; Sammito and Böckelmann, 2016; Johnston et al., 2020). CardioMood® smartphone application for iPhone was used to process the recorded data as described (Flatt and Esco, 2015; Markov et al., 2016; Baevsky and Chernikova, 2017; Perrotta et al., 2017). A total of five HRV parameters and time intervals were selected based on the literature (Malik, 1996; Trimmel et al., 2015; Abreu et al., 2019; Johnston et al., 2020). Specifically, 1) the standard deviation of RR interval (SDNN; i.e., marker of the sympathovagal balance influenced by the sympathetic activity) and 2) the root mean square of successive normal RR interval differences (RMSSD; i.e., marker of parasympathetic activity) were examined from the time domain of HRV indices. In addition, 3) the high frequency power (0.15–0.40 Hz) (HF; i.e., marker of parasympathetic activity), 4) total power (0–0.4 Hz) (TP; i.e., marker of the sympathovagal balance influenced by the sympathetic activity), and 5) low frequency power (0.04–0.15 Hz) (LF; i.e., that reflects sympathovagal balance, baroreceptor reflex activity or neither) (Akselrod et al., 1981; McCraty et al., 2001; Rahman et al., 2011; Martelli et al., 2014) were examined from the frequency domain of the HRV indices.
2.3.6 Blood Collection and Biochemical Analysis
Blood samples were drawn using universal procedures (WHO, 2010) as follows: on the evening before rest/sleep (D1); on the following morning just prior to exercise (D2); following exercise immediate post-exercise (IPE) and immediately prior to eating a standard test meal (0h-), and; again at two (2h-), four (4h-), and 6 h post-exercise (6h-PE) after eating the test meal (Figure 1). All assays were performed in duplicate on first thaw of the samples after being stored at -80°C. All blood samples were analyzed for lipemia related variables (e.g., triglycerides, low-density lipoprotein cholesterol, high-density lipoprotein cholesterol, total cholesterol) using commercial ELISA kits (Wako Pure Diagnostics® Richmond, VA). Standard curves for all assays were developed to determine the concentrations in the study samples. All blood variables were corrected for plasma volume shifts known to occur with exercise. All assays for each subject were run on the same day with the same reagent batch to minimize intra- and inter-variability and keep high the internal quality control of our laboratory analysis.
2.4 Data Analyses
As noted earlier, the dataset for this manuscript was based on previous work and all the related statistical analyses are described in detail elsewhere (Papadakis et al., 2020; Papadakis et al., 2021a; Papadakis et al., 2021b). We used LDL cholesterol, a marker of cardiovascular risk, due its important clinical significance in cardiovascular disease (Cromwell et al., 2007; Trejo-Gutierrez and Fletcher, 2007), exercise (Durstine et al., 2002), and HRV interactions (Kupari et al., 1993; Christensen et al., 1999; Thayer et al., 2010; Chung et al., 2020; Vijayabaskaran et al., 2022). Since we wanted to investigate the postprandial network interactions between autonomic regulation via HRV and lipemia via LDL under APSD and after HIIE, we constructed one correlation analysis and one physiological network only for the experimental conditions of RSX and SSX. The RSX network was defined as the healthy network. We used two groups of parameters: 1) the five HRV parameters (SDNN, RMSSD, HF, TP, and LF) assessed in six different occasions (D1, D2, 0, 2, 4 and 6-h post-exercise), and 2) LDL obtained in seven different moments (D1, D2, IPE, 0, 2, 4, and 6-post-exercise) (total of 37 parameters).
To obtain the correlation analysis (Figure 2A), the Pearson correlation coefficient was used to calculate the correlations between all possible pairs of the aforementioned HRV and LDL parameters, including inter-HRV/LDL (between HRV and LDL), intra-HRV (within HRV) and intra-LDL (within LD) parameters. To visualize the information provided by the correlation analysis, we next mapped the previously obtained correlation analysis into one physiological network (Figure 2B). This graphical approach is essential to identify patterns in the postprandial network structure and to track the differences in network characteristics for the different experimental conditions. The physiological network was constructed utilizing only the statistically significant correlations in the correlation analysis. The physiological network was comprised by two sub-networks: the HRV and the LDL sub-networks, where color nodes (30 for HRV and seven for LDL) represent the different HRV and LPL parameters, and the network links correspond to the correlation analysis elements reflecting the coupling strength between a given pair of parameters. Links strength is marked by line color and width and are divided into six types: strong positive links (Pearson coefficients >0.8), intermediate positive links (0.6 < Pearson coefficients <0.8), weak positive links (0.4 < Pearson coefficients <0.6); weak negative links (−0.4 > Pearson coefficients > −0.6); intermediate negative links (−0.6 > Pearson coefficients > −0.8), and strong negative links (Pearson coefficients < −0.8). With the aim of quantifying the interactions within the physiological network, we computed the number of links (i.e., number of significant correlations; Figure 3). Specifically, we calculated 1) the total number of links in the entire physiological network, 2) the number of links between the HRV and LDL sub-networks (inter-HRV/LDL); 3) the number of links within the HRV sub-network (intra-HRV); and 4) the number of links within the LDL sub-network (intra-LDL). Correlation matrices and physiological networks were processed and obtained by means of Matlab R2016b (Mathworks, Natik, MA, United States). The visualization framework used in our results is based on previous studies analyzing network interactions among physiological systems during different physiological states (Bashan et al., 2012; Bartsch et al., 2015; Lin et al., 2020; Prats-Puig et al., 2020).
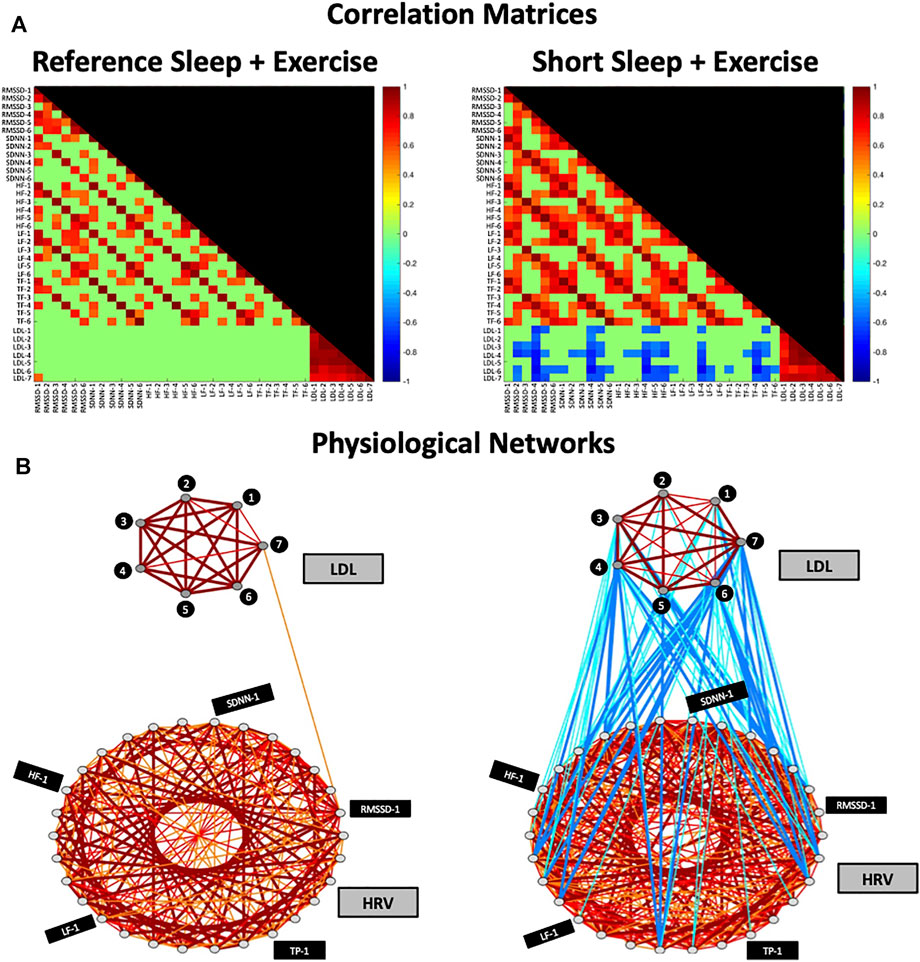
FIGURE 2. Correlation Matrices and Physiological Networks representing postprandial network interactions for Reference Sleep + Exercise (RSX) and Short Sleep + Exercise (SSX). (A) Matrix elements in the correlation matrix represent pairwise coupling strength between each possible pair of HRV and LDL parameters (Pearson correlation coefficient; see Methods). Non-significant correlations are represented in green. Color code is shown in vertical color bars. (B) Nodes in the physiological network represent the different HRV and LDL parameters, and the network links correspond to the correlation matrix elements, reflecting the coupling strength between HRV and LDL parameters. Links strength is marked by line color and width and are divided into six types: strong positive links (Pearson coefficients >0.8), intermediate positive links (0.6 < Pearson coefficients < 0.8), weak positive links (0.4 < Pearson coefficients < 0.6); weak negative links (−0.4 > Pearson coefficients > −0.6); intermediate negative links (−0.6 > Pearson coefficients > −0.8), and strong negative links (Pearson coefficients < −0.8). HRV, heart rate variability; LDL, Low-density lipoprotein; HF-1, High-frequency power at time point -1; SDNN-1, standard deviation of RR interval at time point-1; RMSSD-1, the root mean square of successive normal RR interval differences at time point-1; TP-1, total power at time point-1; LF-1, low-frequency at time point-1.
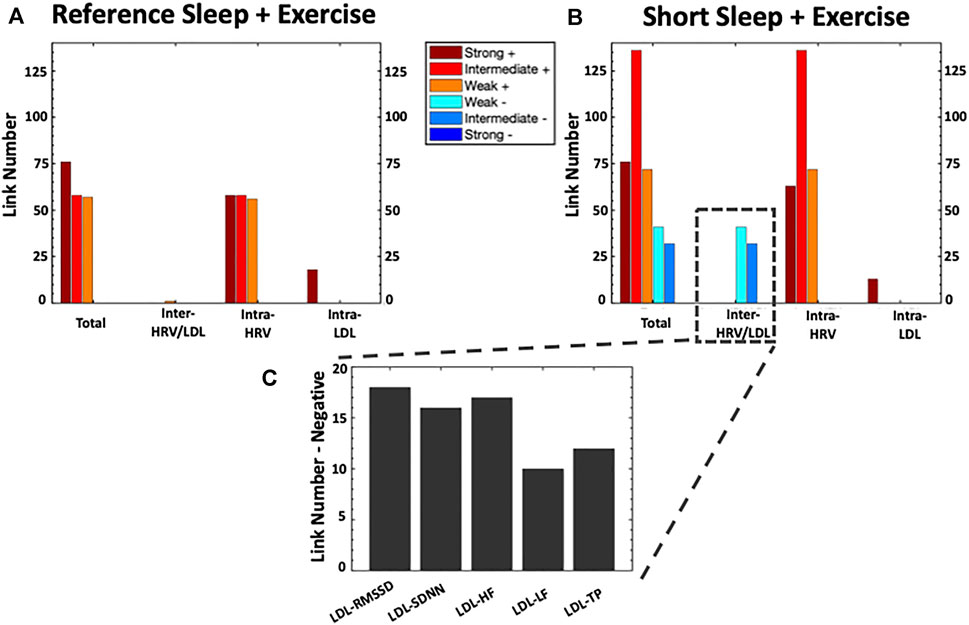
FIGURE 3. Bar Charts Panel representing the number of links (i.e., significant correlations) within each physiological network for Reference Sleep + Exercise (RSX) and Short Sleep + Exercise (SSX). The heigh of the bars in (A) and (B) indicate 1) the total number of links in the entire physiological network, 2) the number of links between the HRV and LDL sub-networks (inter-HRV/LDL); (iii) the number of links within the HRV sub-network (intra-HRV); and (iv) the number of links within the LDL sub-network (intra-LDL). Panel (C) shows the details for the number of inter-HRV/LDL negative links. HRV, heart rate variability; LDL, Low-density lipoprotein; HF, High-frequency power; SDNN, standard deviation of RR interval; RMSSD, the root mean square of successive normal RR interval differences; TP, total power; LF: low-frequency.
3 Results
From the 30 individuals who signed consent participation forms, only 15 participants were able to adhere to study’s requirements and/or completed the study. Baseline screening of anthropometric and physiological characteristics are presented on Table 1. No differences at p = 0.05 were observed between conditions for the pre-experimental collected data of sleep, diet, and physical activity.
Experimental data for sleep and physical activity are presented in Table 2.
Experimental data for exercise are presented in Table 3. Percentage of coefficients of variation (CV%) for LDL cholesterol were calculated for RSX and SSX for all seven timepoints. The CV% for RSX was 9.8% and for the SSX was 10.5%, respectively.
Figure 2 shows the correlation matrices and physiological networks representing postprandial network interactions for RSX and SSX conditions. Matrix elements in the correlation analysis represent pairwise coupling strength between each possible pair of HRV and LDL parameters. Nodes in the physiological network represent the different HRV and LDL parameters, and the network links correspond to the correlation analysis elements, reflecting the coupling strength between HRV and LDL parameters. We observed a clearly differentiated network of postprandial interactions between RSX and SSX due to the irruption of negative links in the SSX network.
As depicted in Figure 3, the total number of links in the physiological network increased by 86.9% under SSX compared to RSX (356 vs 191 links) due to 1) the manifestation of weak and intermediate negative inter-HRV/LDL links, and 2) an increase of positive intra-HRV links.
No inter-HRV/LDL links were observed between the HRV and LDL sub-networks in RSX. However, a remarkable incursion of weak and intermediate negative links was observed between the HRV and LDL sub-networks in SSX. These negative association were present for all HRV parameters, with higher number of links between LDL and RMSSD, SDNN and HF (see Figure 2C).
Regarding the intra-HRV and intra-LDL links, the HRV sub-network was characterized by an increased number of intermediate positive links in SSX (136%) compared to RSX. No remarkable differences were observed between RSX and SSX for intra-LDL links in the LDL sub-network.
4 Discussion
This study investigated postprandial network interactions between autonomic regulation through HRV, and lipemia through low-density lipoprotein (LDL) cholesterol in response to APSD and after HIIE. We reported an irruption of inter-HRV/LDL negative links in the physiological network of SSX compared to RSX, which a priori we defined as the healthy network. The presence of weak and intermediate negative links between the HRV and LDL sub-networks in SSX reflected the impact of sleep deprivation on the autonomic regulation and lipemia. Further, increased connectivity was noted within the HRV sub-network in SSX, with no differences documented for the LDL sub-network. These findings revealed the inability of HIIE to remain cardioprotective under APSD state, and underlie the need to further investigate the effects of APSD and HIIE on the interactions among physiological systems.
The presence of negative links between HRV and LDL for SSX is supported by evidence indicating that impaired balance of the ANS may be the mechanistic explanation linking APSD to CVD (Zhong et al., 2005; Tobaldini et al., 2014). Literature indicates that APSD increases the sympathoadrenal influence, has a greater impact on metabolic and cardiovascular functions that result to increased catecholamine levels, dampened glucose metabolism, increased heart rate (HR) and blood pressure (BP) (Gottlieb et al., 2006; Hall et al., 2008; Meerlo et al., 2008). In addition, it alters the hypothalamic-pituitary-adrenal (HPA) axis activity towards to a higher glucocorticoid release and subsequent systemic inflammatory and oxidative stress response (Meerlo et al., 2008). Therefore, it is apparent that physiologically speaking, APSD has detrimental health effects, which may be observed after very short-term exposure (Alvarez and Ayas, 2004; Gangwisch et al., 2006; Atkinson and Davenne, 2007; Knutson et al., 2007; Buxton and Marcelli, 2010; Centers for Disease and Prevention, 2011; Dettoni et al., 2012; Wu et al., 2012; Calvin et al., 2014). Moreover, acute exercise induces changes in autonomic tone and disturbs the ANS (Thompson et al., 2007; Fukuda et al., 2015), with the HIIE to disturb even more the ANS, and possible to contributing to an unhealthy cardiometabolic status, even after a single episode of HIIE (Besnier et al., 2017).
Considering RSX as the reference healthy and functional network, the HRV sub-network for SSX was characterized by an increment of intermediate positive links, reflecting an increased connectivity due to a reduced sleep time. As previously described (Balague et al., 2020) both underexpressed (weak) and overexpressed network connectivity could reflect unfunctional/pathological states. More specifically, overexpressed/excessive connectivity as observed within the HRV sub-network for SSX, could be associated with a transitory underexpression of coupling network connectivity (i.e., imbalance: some processes are overexpressed and others underexpressed). An example of such imbalance is the rigidity and reduction of diversity potential provoked by exercise-induced fatigue (Vazquez et al., 2016; Vazquez et al., 2020). Similarly, some pathological conditions (e.g., neuro-muscular disorders) could increase the density and/or strength of interactions among certain nodes, pushing the system toward a rigid order which, in turn, could reduce its adaptability to environmental constraints (Ivanov et al., 1998; Ivanov et al., 2001; Stergiou et al., 2006; Stergiou and Decker, 2011). The results of this study support the notion of characterizing a healthy network based on the number of network links.
Several sleep protocols have examined the sleep deprivation effects on healthy individuals in respect to cardiovascular changes and autonomic control via HRV (Tobaldini et al., 2014; Tobaldini et al., 2017). Some reported no differences in HRV with just 4 h sleep (Muenter et al., 2000), while other showed an increase in sympathetic activity (SA) as demonstrated in total decreased HRV, increased low-frequency (LF), and decreased high-frequency (HF) compared to control of 8 h sleep (Dettoni et al., 2012). Our results are in agreement with those that showed an impact of short sleep duration on autonomic regulation (Dettoni et al., 2012) as we reported negative associations for all the HRV parameters and lipemia.
It is important though to mention that studies that examine sleep, HRV, exercise and cardiometabolic health due to differences in the employed research designs, settings, examined variables, sleep durations, sample characteristics etc., yield heterogenous results that are difficult to compared and provide clear and comprehensive outcomes (Malik, 1996; Elsenbruch et al., 1999; Kaikkonen et al., 2008; Al Haddad et al., 2009; Dettoni et al., 2012; Myllymaki et al., 2012; Uchida et al., 2012; Heydari et al., 2013; Tobaldini et al., 2013; Oda and Shirakawa, 2014; Michael et al., 2017; Tobaldini et al., 2017; van Leeuwen et al., 2018; Abreu et al., 2019; Barroso et al., 2019; Costa et al., 2019; Schneider et al., 2019; Vitale et al., 2019). Therefore, research questions related to the immediate HRV responses after acute HIIE following reference sleep and APSD are still under investigation. Previous work from our lab has examined such research questions (Papadakis et al., 2020; Papadakis et al., 2021a; Papadakis et al., 2021b) using the traditional reductionistic approach focusing on a single physiological system and investigated the mechanistic interactions with other single systems by reducing complex multicomponent systems on their respective parts (Machamer et al., 2000; Bechtel and Richardson, 2010; Balague et al., 2020). We reported that HIIE was cardioprotective and APSD did not influence the HRV (Papadakis et al., 2021a), neither the postprandial endothelial function (Papadakis et al., 2020), nor the exercise performance (Papadakis et al., 2021b).
Investigating though the same research questions under the Network Physiology of Exercise perspective we showed that APSD and HIIE had an impact on the HRV and LDL. It seems though that our previous investigations were not able to capture the synchronization and integration among autonomic nervous and cardiometabolic systems. Recent work from Ivanov and Bartsch, (2014) and Ivanov et al., (2017) highlighted the fact that physiological states emerge due to specific network organization, topology, and their respective network of dynamic interactions. Moreover, such network of dynamic interactions is moving past the concepts of interconnectivity across of physiological systems and the statistical inference of static associations that govern physiological states (Sieck, 2017; Head, 2020). As such, our previous analyses (Papadakis et al., 2020; Papadakis et al., 2021a; Papadakis et al., 2021b), failed to provide a comprehensive understanding of the dynamic interaction of the involved physiological systems and their subsystems to generate dynamic integrated response at the organism level (Balague et al., 2020). The findings of this study reinforce previous works suggesting that the commonly utilized physiological parameters (e.g., VO2max) provide little information on the nature of the dynamic interactions among physiological systems and their common role in an integrated network. Coordinative variables, such as cardio-respiratory coordination or other psychophysiological parameters, can detect qualitative changes related to the coordinated activity among physiological systems, and their changes under exercise-related constraints (Balagué et al., 2013; Esquius et al., 2019; Garcia-Retortillo et al., 2019).
A major limitation of this study is that it cannot be considered as a true network analysis, as no time series of physiological variables were recorded and analyzed. Note that to capture interactions among physiological systems, time series analysis and the detection of coordinative variables would be the most appropriate strategy. This study was not initially conceived to investigate postprandial network interactions between autonomic regulation and lipemia, but to mimic real life settings between APSD and HIIE under the traditional framework of Exercise Physiology. The applied correlation analysis does not have the power to identify dynamic interactions between the investigated physiological systems. Therefore, this study has inherited all the limitations of the traditional Exercise Physiology framework, that is, the tacit assumption that results obtained by a sample can be generalized to a population level based on the representative observed changes of a “typical” (i.e., average) individual. This assumption though can be true only if the system is ergodic and its evolution in time is stationary and the structure of the interindividual multivariate dynamics is the same across all individuals (Balague et al., 2020). Moreover, since this is reanalysis of data collected for another purpose, it carries the limitations of our previous investigations (e.g., absence of APSD and no HIIE, only apparently healthy men who were good sleepers, environmental stress and factors outside of controlled laboratory settings, indirect method of measuring the cardiac autonomic activity, time of day and chronotype of our sample) (Papadakis et al., 2020; Papadakis et al., 2021a; Papadakis et al., 2021b). At the same time though, this study’s strength is the application of the NPE approach to examine a research question with controversial results when examined through the traditional Exercise Physiology framework. This study is providing preliminary evidence on the sensitivity of the NPE approach to capture interactions among different physiological systems. In this line, further research utilizing time series of physiological variables (Garcia-Retortillo et al., 2020) is needed to investigate the effects of APSD and HIIE on postprandial network interactions.
5 Conclusion
The human organism is composed of various integrated networks and sub-networks of interconnected organs, systems, and functions, a disruption or failure of one system can trigger a cascade of failures that can be manifested as a disease state (Ivanov and Bartsch, 2014; Goldman et al., 2015; Liu et al., 2015; Ivanov et al., 2017; Sieck, 2017; Liu and Chen, 2019; Balague et al., 2020; Barajas-Martinez et al., 2020; Corkey and Deeney, 2020). We investigated postprandial network interactions between autonomic regulation through HRV, and lipemia through LDL cholesterol in response to APSD and HIIE. We observed an increase of inter-HRV/LDL negative links in the SSX physiological network compared to RSX. These results reflected the impact of sleep deprivation on the autonomic regulation and lipemia and, revealed the inability of HIIE to remain cardioprotective under APSD.
5.1 Resource Identification Initiative
To take part in the Resource Identification Initiative, please use the corresponding catalog number and RRID in your current manuscript. For more information about the project and for steps on how to search for an RRID, please click here.
Data Availability Statement
The data analyzed in this study is subject to the following licenses/restrictions No restrictions applied to the dataset. Requests to access these datasets should be directed to enBhcGFkYWtpc0BiYXJyeS5lZHU=.
Ethics Statement
The studies involving human participants were reviewed and approved by Baylor University. The patients/participants provided their written informed consent to participate in this study.
Author Contributions
All authors listed have made a substantial, direct, and intellectual contribution to the work and approved it for publication.
Conflict of Interest
The authors declare that the research was conducted in the absence of any commercial or financial relationships that could be construed as a potential conflict of interest.
Publisher’s Note
All claims expressed in this article are solely those of the authors and do not necessarily represent those of their affiliated organizations, or those of the publisher, the editors and the reviewers. Any product that may be evaluated in this article, or claim that may be made by its manufacturer, is not guaranteed or endorsed by the publisher.
Acknowledgments
The authors would like to thank Dr. Peter Grandjean for his mentorship regarding the original project, from its inception until its completion. We thank all participants who generously contributed to this study. Authors would like also to express their gratitude to Dr. Natalia Balague for her valuable feedback regarding this manuscript.
References
Abreu, R. M. D., Rehder-Santos, P., Simões, R. P., and Catai, A. M. (2019). Can High-Intensity Interval Training Change Cardiac Autonomic Control? A Systematic Review. Braz. J. Phys. Ther. 23 (4), 279–289. doi:10.1016/j.bjpt.2018.09.010
Achten, J., and Jeukendrup, A. E. (2003). Heart Rate Monitoring: Applications and Limitations. Sports Med. 33 (7), 517–538. doi:10.2165/00007256-200333070-00004
Akselrod, S., Gordon, D., Ubel, F. A., Shannon, D. C., Berger, A. C., and Cohen, R. J. (1981). Power Spectrum Analysis of Heart Rate Fluctuation: a Quantitative Probe of Beat-To-Beat Cardiovascular Control. Science 213 (4504), 220–222. doi:10.1126/science.6166045
Al Haddad, H., Laursen, P. B., Ahmaidi, S., and Buchheit, M. (2009). Nocturnal Heart Rate Variability Following Supramaximal Intermittent Exercise. Int. J. Sports Physiol. Perform. 4 (4), 435–447. doi:10.1123/ijspp.4.4.435
Almeida, G. J. M., Wasko, M. C. M., Jeong, K., Moore, C. G., and Piva, S. R. (2011). Physical Activity Measured by the SenseWear Armband in Women with Rheumatoid Arthritis. Phys. Ther. 91 (9), 1367–1376. doi:10.2522/ptj.20100291
Alvarez, G. G., and Ayas, N. T. (2004). The Impact of Daily Sleep Duration on Health: A Review of the Literature. Prog. Cardiovasc. Nurs. 19 (2), 56–59. doi:10.1111/j.0889-7204.2004.02422.x
American College of Sports Medicine (2013). ACSM's Guidelines for Exercise Testing and Prescription. Philadelphia, PA: Lippincott Williams & Wilkins.
American Sleep Association (2018). Sleep Deprivation - Latest Research & Treatments | American Sleep Assoc [Online]. Available: https://www.sleepassociation.org/sleep-disorders/sleep-deprivation/ (Accessed February 5, 2022).
Atkinson, G., and Davenne, D. (2007). Relationships between Sleep, Physical Activity and Human Health. Physiol. Behav. 90 (2-3), 229–235. doi:10.1016/j.physbeh.2006.09.015
Baevsky, R. М., and Chernikova, A. G. (2017). Heart Rate Variability Analysis: Physiological Foundations and Main Methods. Cardiometry 10, 66–76. doi:10.12710/cardiometry.2017.10.6676
Balagué, N., Aragonés, D., Hristovski, R., García-Retortillo, S., and Tenenbaum, G. (2013). Attention Focus Emerges Spontaneously during Progressive and Maximal Exercise. Revista de Psicología Del. Deporte 23 (1), 57–63.
Balagué, N., Hristovski, R., Almarcha, M., Garcia-Retortillo, S., and Ivanov, P. C. (2020). Network Physiology of Exercise: Vision and Perspectives. Front. Physiol. 11 (1607), 611550. doi:10.3389/fphys.2020.611550
Barajas-Martínez, A., Ibarra-Coronado, E., Sierra-Vargas, M. P., Cruz-Bautista, I., Almeda-Valdes, P., Aguilar-Salinas, C. A., et al. (2020). Physiological Network from Anthropometric and Blood Test Biomarkers. Front. Physiol. 11 (1791), 612598. doi:10.3389/fphys.2020.612598
Barroso, R., Silva-Filho, A. C., Dias, C. J., Soares, N., Mostarda, A., Azoubel, L. A., et al. (2019). Effect of Exercise Training in Heart Rate Variability, Anxiety, Depression, and Sleep Quality in Kidney Recipients: A Preliminary Study. J. Health Psychol. 24 (3), 299–308. doi:10.1177/1359105316676329
Bartsch, R. P., Liu, K. K., Ma, Q. D., and Ivanov, P. C. (2014). Three Independent Forms of Cardio-Respiratory Coupling: Transitions across Sleep Stages. Comput. Cardiol. (2010) 41, 781–784.
Bartsch, R. P., Liu, K. K. L., Bashan, A., and Ivanov, P. C. (2015). Network Physiology: How Organ Systems Dynamically Interact. PLoS One 10 (11), e0142143. doi:10.1371/journal.pone.0142143
Bashan, A., Bartsch, R. P., Kantelhardt, J. W., Havlin, S., and Ivanov, P. C. (2012). Network Physiology Reveals Relations between Network Topology and Physiological Function. Nat. Commun. 3 (1), 702. doi:10.1038/ncomms1705
Bechtel, W., and Richardson, R. C. (2010). Discovering Complexity: Decomposition and Localization as Strategies in Scientific Research. Cambridge, MA: MIT press.
Besnier, F., Labrunée, M., Pathak, A., Pavy-Le Traon, A., Galès, C., Sénard, J.-M., et al. (2017). Exercise Training-Induced Modification in Autonomic Nervous System: An Update for Cardiac Patients. Ann. Phys. Rehabil. Med. 60 (1), 27–35. doi:10.1016/j.rehab.2016.07.002
Bhati, P., and Moiz, J. A. (2017). High-Intensity Interval Training and Cardiac Autonomic Modulation. Saudi J. Sports Med. 17 (3), 129–134. doi:10.4103/sjsm.sjsm_2_17
Bond, B., Gates, P. E., Jackman, S. R., Corless, L. M., Williams, C. A., and Barker, A. R. (2015). Exercise Intensity and the protection from Postprandial Vascular Dysfunction in Adolescents. Am. J. Physiology-Heart Circulatory Physiol. 308 (11), H1443–H1450. doi:10.1152/ajpheart.00074.2015
Bourland, C. T., and Vogt, G. L. (2009). “Breakfast Foods,” in The Astronaut's Cookbook (New York, NY: Springer New York), 28–36. doi:10.1007/978-1-4419-0624-3_2
Buchheit, M. (2014). Monitoring Training Status with HR Measures: Do All Roads Lead to Rome? Front. Physiol. 5, 73. doi:10.3389/fphys.2014.00073
Buxton, O. M., and Marcelli, E. (2010). Short and Long Sleep Are Positively Associated with Obesity, Diabetes, Hypertension, and Cardiovascular Disease Among Adults in the United States. Soc. Sci. Med. 71 (5), 1027–1036. doi:10.1016/j.socscimed.2010.05.041
Buysse, D. J., Reynolds, C. F., Monk, T. H., Berman, S. R., and Kupfer, D. J. (1989). The Pittsburgh Sleep Quality Index: A New Instrument for Psychiatric Practice and Research. Psychiatry Res. 28 (2), 193–213. doi:10.1016/0165-1781(89)90047-4
Calvin, A. D., Covassin, N., Kremers, W. K., Adachi, T., Macedo, P., Albuquerque, F. N., et al. (2014). Experimental Sleep Restriction Causes Endothelial Dysfunction in Healthy Humans. J. Am. Heart Assoc. 3 (6), e001143. doi:10.1161/JAHA.114.001143
Centers for Disease and Prevention (2011). Effect of Short Sleep Duration on Daily activities-United States, 2005-2008. MMWR Morb Mortal Wkly Rep. 60 (8), 239–242. doi:10.2307/23319838
Christensen, J. H., Toft, E., Christensen, M. S., and Schmidt, E. B. (1999). Heart Rate Variability and Plasma Lipids in Men with and without Ischaemic Heart Disease. Atherosclerosis 145 (1), 181–186. doi:10.1016/s0021-9150(99)00052-0
Chung, H., Oh, Y. H., Moon, J. H., Kim, H. J., and Kong, M. H. (2020). Risk of Metabolic and Cardiovascular Risk Factors in Individuals with Autonomic Imbalance Measured by Heart Rate Variability. Korean J. Health Promot. 20 (2), 41–48. doi:10.15384/kjhp.2020.20.2.41
Corkey, B. E., and Deeney, J. T. (2020). The Redox Communication Network as a Regulator of Metabolism. Front. Physiol. 11 (1310), 567796. doi:10.3389/fphys.2020.567796
Costa, J., Figueiredo, P., Nakamura, F., Rago, V., Rebelo, A., and Brito, J. (2019). Intra-Individual Variability of Sleep and Nocturnal Cardiac Autonomic Activity in Elite Female Soccer Players during an International Tournament. PLoS One 14 (9), e0218635. doi:10.1371/journal.pone.0218635
Cromwell, W. C., Otvos, J. D., Keyes, M. J., Pencina, M. J., Sullivan, L., Vasan, R. S., et al. (2007). LDL Particle Number and Risk of Future Cardiovascular Disease in the Framingham Offspring Study-Implications for LDL Management. J. Clin. Lipidol. 1 (6), 583–592. doi:10.1016/j.jacl.2007.10.001
Dettoni, J. L., Consolim-Colombo, F. M., Drager, L. F., Rubira, M. C., Cavasin de Souza, S. B. P., Irigoyen, M. C., et al. (2012). Cardiovascular Effects of Partial Sleep Deprivation in Healthy Volunteers. J. Appl. Physiol. 113 (2), 232–236. doi:10.1152/japplphysiol.01604.2011
Di Francescomarino, S., Sciartilli, A., Di Valerio, V., Di Baldassarre, A., and Gallina, S. (2009). The Effect of Physical Exercise on Endothelial Function. Sports Med. 39 (10), 797–812. doi:10.2165/11317750-000000000-00000
Durstine, J. L., Grandjean, P. W., Cox, C. A., and Thompson, P. D. (2002). Lipids, Lipoproteins, and Exercise. J. Cardiopulmonary Rehabil. 22 (6), 385–398. doi:10.1097/00008483-200211000-00002
Elsenbruch, S., Harnish, M. J., and Orr, W. C. (1999). Heart Rate Variability during Waking and Sleep in Healthy Males and Females. Sleep 22 (8), 1067–1071. doi:10.1093/sleep/22.8.1067
Engström, E., Ottosson, E., Wohlfart, B., Grundström, N., and Wisén, A. (2012). Comparison of Heart Rate Measured by Polar RS400 and ECG, Validity and Repeatability. Adv. Physiother. 14 (3), 115–122. doi:10.3109/14038196.2012.694118
Esquius, L., Garcia-Retortillo, S., Balagué, N., Hristovski, R., and Javierre, C. (2019). Physiological- and Performance-Related Effects of Acute Olive Oil Supplementation at Moderate Exercise Intensity. J. Int. Soc. Sports Nutr. 16 (1), 12. doi:10.1186/s12970-019-0279-6
Fisher-Wellman, K., and Bloomer, R. J. (2009). Acute Exercise and Oxidative Stress: A 30 Year History. Dyn. Med. 8, 1. doi:10.1186/1476-5918-8-1
Flatt, A. A., and Esco, M. R. (2015). Smartphone-Derived Heart-Rate Variability and Training Load in a Women's Soccer Team. Int. J. Sports Physiol. Perform. 10 (8), 994–1000. doi:10.1123/ijspp.2014-0556
Fukuda, K., Kanazawa, H., Aizawa, Y., Ardell, J. L., and Shivkumar, K. (2015). Cardiac Innervation and Sudden Cardiac Death. Circ. Res. 116 (12), 2005–2019. doi:10.1161/CIRCRESAHA.116.304679
Gabriel, B., Ratkevicius, A., Gray, P., Frenneaux, M. P., and Gray, S. R. (2012). High-Intensity Exercise Attenuates Postprandial Lipaemia and Markers of Oxidative Stress. Clin. Sci. (Lond) 123 (5), 313–321. doi:10.1042/CS20110600
Gangwisch, J. E., Heymsfield, S. B., Boden-Albala, B., Buijs, R. M., Kreier, F., Pickering, T. G., et al. (2006). Short Sleep Duration as a Risk Factor for Hypertension: Analyses of the First National Health and Nutrition Examination Survey. Hypertension 47 (5), 833–839. doi:10.1161/01.HYP.0000217362.34748.e0
Garcia-Retortillo, S., Javierre, C., Hristovski, R., Ventura, J. L., and Balagué, N. (2019). Principal Component Analysis as a Novel Approach for Cardiorespiratory Exercise Testing Evaluation. Physiol. Meas. 40 (8), 084002. doi:10.1088/1361-6579/ab2ca0
Garcia-Retortillo, S., Rizzo, R., Wang, J. W. J. L., Sitges, C., and Ivanov, P. C. (2020). Universal Spectral Profile and Dynamic Evolution of Muscle Activation: A Hallmark of Muscle Type and Physiological State. J. Appl. Physiol. 129 (3), 419–441. doi:10.1152/japplphysiol.00385.2020
Gibala, M. J. (2007). High-Intensity Interval Training: A Time-Efficient Strategy for Health Promotion? Curr. Sports Med. Rep. 6 (4), 211–213. doi:10.1007/s11932-007-0033-8
Gibala, M. J., Little, J. P., Macdonald, M. J., and Hawley, J. A. (2012). Physiological Adaptations to Low-Volume, High-Intensity Interval Training in Health and Disease. J. Physiol. 590 (5), 1077–1084. doi:10.1113/jphysiol.2011.224725
Gielen, S., and Hambrecht, R. (2005). Treatment Strategies in Endothelial Dysfunction: Physical Exercise Versus Pharmacological Therapy. Eur. J. Cardiovasc. Prev. Rehabil. 12 (4), 318–320. doi:10.1097/01.hjr.0000174826.72022.c4
Gielen, S., Sandri, M., Erbs, S., and Adams, V. (2011). Exercise-Induced Modulation of Endothelial Nitric Oxide Production. Curr. Pharm. Biotechnol. 12 (9), 1375–1384. doi:10.2174/138920111798281063
Gielen, S., Schuler, G., and Adams, V. (2010). Cardiovascular Effects of Exercise Training: Molecular Mechanisms. Circulation 122 (12), 1221–1238. doi:10.1161/CIRCULATIONAHA.110.939959
Goldman, A. W., Burmeister, Y., Cesnulevicius, K., Herbert, M., Kane, M., Lescheid, D., et al. (2015). Bioregulatory Systems Medicine: An Innovative Approach to Integrating the Science of Molecular Networks, Inflammation, and Systems Biology with the Patient's Autoregulatory Capacity? Front. Physiol. 6, 225. doi:10.3389/fphys.2015.00225
Gottlieb, D. J., Redline, S., Nieto, F. J., Baldwin, C. M., Newman, A. B., Resnick, H. E., et al. (2006). Association of Usual Sleep Duration with Hypertension: The Sleep Heart Health Study. Sleep 29 (8), 1009–1014. doi:10.1093/sleep/29.8.1009
Grant, C. C., Viljoen, M., Janse van Rensburg, D. C., and Wood, P. S. (2012). Heart Rate Variability Assessment of the Effect of Physical Training on Autonomic Cardiac Control. Ann. Noninvasive Electrocardiol. 17 (3), 219–229. doi:10.1111/j.1542-474X.2012.00511.x
Hale, L., Troxel, W., and Buysse, D. J. (2020). Sleep Health: An Opportunity for Public Health to Address Health Equity. Annu. Rev. Public Health 41, 81–99. doi:10.1146/annurev-publhealth-040119-094412
Hall, M. H., Muldoon, M. F., Jennings, J. R., Buysse, D. J., Flory, J. D., and Manuck, S. B. (2008). Self-Reported Sleep Duration Is Associated with the Metabolic Syndrome in Midlife Adults. Sleep 31 (5), 635–643. doi:10.1093/sleep/31.5.635
Harris, R. A., Padilla, J., Hanlon, K. P., Rink, L. D., and Wallace, J. P. (2008). The Flow-Mediated Dilation Response to Acute Exercise in Overweight Active and Inactive Men. Obesity (Silver Spring) 16 (3), 578–584. doi:10.1038/oby.2007.87
Head, G. A. (2020). Integrative Physiology: Update to the Grand Challenge 2020. Front. Physiol. 11, 489. doi:10.3389/fphys.2020.00489
Heydari, M., Boutcher, Y. N., and Boutcher, S. H. (2013). High-Intensity Intermittent Exercise and Cardiovascular and Autonomic Function. Clin. Auton. Res. 23 (1), 57–65. doi:10.1007/s10286-012-0179-1
Hyson, D., Rutledge, J. C., and Berglund, L. (2003). Postprandial Lipemia and Cardiovascular Disease. Curr. Atheroscler. Rep. 5 (6), 437–444. doi:10.1007/s11883-003-0033-y
Ito, S. (2019). High-Intensity Interval Training for Health Benefits and Care of Cardiac Diseases - the Key to an Efficient Exercise Protocol. World J. Cardiol. 11 (7), 171–188. doi:10.4330/wjc.v11.i7.171
Ivanov, P. C., and Bartsch, R. P. (2014). “Network Physiology: Mapping Interactions between Networks of Physiologic Networks,” in Networks of Networks: The Last Frontier of Complexity (Cham: Springer), 203–222. doi:10.1007/978-3-319-03518-5_10
Ivanov, P. C., Liu, K. K. L., and Bartsch, R. P. (2016). Focus on the Emerging New fields of Network Physiology and Network Medicine. New J. Phys. 18 (10), 100201. doi:10.1088/1367-2630/18/10/100201
Ivanov, P. C., Liu, K. K., Lin, A., and Bartsch, R. P. (2017). “Network Physiology: From Neural Plasticity to Organ Network Interactions,” in Emergent Complexity from Nonlinearity, in Physics, Engineering and the Life Sciences (Cham: Springer), 145–165. doi:10.1007/978-3-319-47810-4_12
Ivanov, P. C., Nunes Amaral, L. A., Goldberger, A. L., and Stanley, H. E. (1998). Stochastic Feedback and the Regulation of Biological Rhythms. Europhys. Lett. 43 (4), 363–368. doi:10.1209/epl/i1998-00366-3
Ivanov, P. C., Nunes Amaral, L. A., Goldberger, A. L., Havlin, S., Rosenblum, M. G., Stanley, H. E., et al. (2001). From 1/f Noise to Multifractal Cascades in Heartbeat Dynamics. Chaos 11 (3), 641–652. doi:10.1063/1.1395631
Ivanov, P. C. (2021). The New Field of Network Physiology: Building the Human Physiolome. Front. Netw. Physiol. 1, 711778. doi:10.3389/fnetp.2021.711778
Ivanov, P. C., Wang, J. W., Zhang, X., and Chen, B. (2021). “The New Frontier of Network Physiology: Emerging Physiologic States in Health and Disease from Integrated Organ Network Interactions,” in 2019-20 MATRIX Annals (Cham: Springer), 237–254. doi:10.1007/978-3-030-62497-2_12
Johnston, B. W., Barrett-Jolley, R., Krige, A., and Welters, I. D. (2020). Heart Rate Variability: Measurement and Emerging Use in Critical Care Medicine. J. Intensive Care Soc. 21 (2), 148–157. doi:10.1177/1751143719853744
Kaikkonen, P., Rusko, H., and Martinmäki, K. (2008). Post-Exercise Heart Rate Variability of Endurance Athletes after Different High-Intensity Exercise Interventions. Scand. J. Med. Sci. Sports 18 (4), 511–519. doi:10.1111/j.1600-0838.2007.00728.x
Knutson, K. L., Spiegel, K., Penev, P., and Van Cauter, E. (2007). The Metabolic Consequences of Sleep Deprivation. Sleep Med. Rev. 11 (3), 163–178. doi:10.1016/j.smrv.2007.01.002
Krittanawong, C., Kumar, A., Wang, Z., Jneid, H., Baber, U., Mehran, R., et al. (2020). Sleep Duration and Cardiovascular Health in a Representative Community Population (From NHANES, 2005 to 2016). Am. J. Cardiol. 127, 149–155. doi:10.1016/j.amjcard.2020.04.012
Kupari, M., Virolainen, J., Koskinen, P., and Tikkanen, M. J. (1993). Short-Term Heart Rate Variability and Factors Modifying the Risk of Coronary Artery Disease in a Population Sample. Am. J. Cardiol. 72 (12), 897–903. doi:10.1016/0002-9149(93)91103-o
Lang, J. M., Eisen, J. A., and Zivkovic, A. M. (2014). The Microbes We Eat: Abundance and Taxonomy of Microbes Consumed in a Day's worth of Meals for Three Diet Types. PeerJ 2, e659. doi:10.7717/peerj.659
Lehnertz, K., Bröhl, T., and Rings, T. (2020). The Human Organism as an Integrated Interaction Network: Recent Conceptual and Methodological Challenges. Front. Physiol. 11 (1694), 598694. doi:10.3389/fphys.2020.598694
Liew, S. C., and Aung, T. (2021). Sleep Deprivation and its Association with Diseases- a Review. Sleep Med. 77, 192–204. doi:10.1016/j.sleep.2020.07.048
Lin, A., Liu, K. K. L., Bartsch, R. P., and Ivanov, P. C. (2020). Dynamic Network Interactions Among Distinct Brain Rhythms as a Hallmark of Physiologic State and Function. Commun. Biol. 3 (1), 197. doi:10.1038/s42003-020-0878-4
Littlefield, L. A., and Grandjean, P. W. (2015). Exercise Intensity and Postprandial Lipemia (La intensidad del ejercicio y la lipemia postprandial). Retos 27 (27), 206–212. doi:10.47197/retos.v0i27.34380
Liu, H., and Chen, A. (2019). Roles of Sleep Deprivation in Cardiovascular Dysfunctions. Life Sci. 219, 231–237. doi:10.1016/j.lfs.2019.01.006
Liu, K. K. L., Bartsch, R. P., Ma, Q. D. Y., and Ivanov, P. C. (2015). Major Component Analysis of Dynamic Networks of Physiologic Organ Interactions. J. Phys. Conf. Ser. 640, 012013. doi:10.1088/1742-6596/640/1/012013
Machamer, P., Darden, L., and Craver, C. F. (2000). Thinking about Mechanisms. Philos. Sci. 67 (1), 1–25. doi:10.1086/392759
Malik, M. (1996). Heart Rate Variability: Standards of Measurement, Physiological Interpretation, and Clinical Use: Task Force of the European Society of Cardiology and the North American Society for Pacing and Electrophysiology. Ann. Noninv Electrocard 1 (2), 151–181. doi:10.1111/j.1542-474x.1996.tb00275.x
Markov, A., Solonin, I., and Bojko, E. (2016). Heart Rate Variability in Workers of Various Professions in Contrasting Seasons of the Year. Int. J. Occup. Med. Environ. Health 29 (5), 793–800. doi:10.13075/ijomeh.1896.00276
Martelli, D., Silvani, A., McAllen, R. M., May, C. N., and Ramchandra, R. (2014). The Low Frequency Power of Heart Rate Variability Is Neither a Measure of Cardiac Sympathetic Tone Nor of Baroreflex Sensitivity. Am. J. Physiology-Heart Circulatory Physiol. 307 (7), H1005–H1012. doi:10.1152/ajpheart.00361.2014
Matsuo, T., Saotome, K., Seino, S., Shimojo, N., Matsushita, A., Iemitsu, M., et al. (2014). Effects of a Low-Volume Aerobic-Type Interval Exercise on VO2max and Cardiac Mass. Med. Sci. Sports Exerc. 46 (1), 42–50. doi:10.1249/mss.0b013e3182a38da8
McCraty, R., Atkinson, M., Tomasino, D., and Stuppy, W. P. (2001). Analysis of Twenty-Four Hour Heart Rate Variability in Patients with Panic Disorder. Biol. Psychol. 56 (2), 131–150. doi:10.1016/s0301-0511(01)00074-6
Meerlo, P., Sgoifo, A., and Suchecki, D. (2008). Restricted and Disrupted Sleep: Effects on Autonomic Function, Neuroendocrine Stress Systems and Stress Responsivity. Sleep Med. Rev. 12 (3), 197–210. doi:10.1016/j.smrv.2007.07.007
Mestek, M. L., Garner, J. C., Plaisance, E. P., Taylor, J. K., Alhassan, S., and Grandjean, P. W. (2006). Blood Lipid Responses after Continuous and Accumulated Aerobic Exercise. Int. J. Sport Nutr. Exerc. Metab. 16 (3), 245–254. doi:10.1123/ijsnem.16.3.245
Mestek, M. L., Plaisance, E. P., Ratcliff, L. A., Taylor, J. K., Wee, S.-O., and Grandjean, P. W. (2008). Aerobic Exercise and Postprandial Lipemia in Men with the Metabolic Syndrome. Med. Sci. Sports Exerc. 40 (12), 2105–2111. doi:10.1249/MSS.0b013e3181822ebd
Meyer, R. (2020). The Non-Mechanistic Option: Defending Dynamical Explanations. Br. J. Philos. Sci. 71 (3), 959–985. doi:10.1093/bjps/axy034
Michael, S., Graham, K. S., and Davis, G. M. (2017). Cardiac Autonomic Responses during Exercise and Post-Exercise Recovery Using Heart Rate Variability and Systolic Time Intervals-A Review. Front. Physiol. 8, 301. doi:10.3389/fphys.2017.00301
Miller, M. A., and Cappuccio, F. P. (2013). Biomarkers of Cardiovascular Risk in Sleep-Deprived People. J. Hum. Hypertens. 27 (10), 583–588. doi:10.1038/jhh.2013.27
Muenter, N. K., Watenpaugh, D. E., Wasmund, W. L., Wasmund, S. L., Maxwell, S. A., and Smith, M. L. (2000). Effect of Sleep Restriction on Orthostatic Cardiovascular Control in Humans. J. Appl. Physiol. 88 (3), 966–972. doi:10.1152/jappl.2000.88.3.966
Myllymaki, T., Rusko, H., Syvaoja, H., Juuti, T., Kinnunen, M. L., and Kyrolainen, H. (2012). Effects of Exercise Intensity and Duration on Nocturnal Heart Rate Variability and Sleep Quality. Eur. J. Appl. Physiol. 112 (3), 801–809. doi:10.1007/s00421-011-2034-9
Oda, S., and Shirakawa, K. (2014). Sleep Onset Is Disrupted Following Pre-Sleep Exercise that Causes Large Physiological Excitement at Bedtime. Eur. J. Appl. Physiol. 114 (9), 1789–1799. doi:10.1007/s00421-014-2873-2
Oliver, M. D., Baldwin, D. R., and Datta, S. (2020). The Relationship between Sleep and Autonomic Health. J. Am. Coll. Health 68 (5), 550–556. doi:10.1080/07448481.2019.1583652
Osuka, Y., Matsubara, M., Hamasaki, A., Hiramatsu, Y., Ohshima, H., and Tanaka, K. (2017). Development of Low-Volume, High-Intensity, Aerobic-Type Interval Training for Elderly Japanese Men: A Feasibility Study. Eur. Rev. Aging Phys. Act 14 (1), 14. doi:10.1186/s11556-017-0184-4
Papadakis, Z., Forsse, J. S., and Peterson, M. N. (2020). Acute Partial Sleep Deprivation and High-Intensity Interval Exercise Effects on Postprandial Endothelial Function. Eur. J. Appl. Physiol. 120 (11), 2431–2444. doi:10.1007/s00421-020-04468-5
Papadakis, Z., Forsse, J. S., and Peterson, M. N. (2021a). Effects of High-Intensity Interval Exercise and Acute Partial Sleep Deprivation on Cardiac Autonomic Modulation. Res. Q. Exerc. Sport 92 (4), 824–842. doi:10.1080/02701367.2020.1788206
Papadakis, Z., Forsse, J. S., and Stamatis, A. (2021b). High-Intensity Interval Exercise Performance and Short-Term Metabolic Responses to Overnight-Fasted Acute-Partial Sleep Deprivation. Int. J. Environ. Res. Public Health 18 (7), 3655. doi:10.3390/ijerph18073655
Perrotta, A. S., Jeklin, A. T., Hives, B. A., Meanwell, L. E., and Warburton, D. E. R. (2017). Validity of the Elite HRV Smartphone Application for Examining Heart Rate Variability in a Field-Based Setting. J. Strength Cond Res. 31 (8), 2296–2302. doi:10.1519/JSC.0000000000001841
Phrommintikul, A., Chattipakorn, S. C., and Chattipakorn, N. (2022). Exercise and Cardioprotection: A "HIP" Side of HIPK2 in the Heart. EBioMedicine 75, 103766. doi:10.1016/j.ebiom.2021.103766
Powers, S. K., Lennon, S. L., Quindry, J., and Mehta, J. L. (2002). Exercise and Cardioprotection. Curr. Opin. Cardiol. 17 (5), 495–502. doi:10.1097/00001573-200209000-00009
Prats-Puig, A., García-Retortillo, S., Puig-Parnau, M., Vasileva, F., Font-Lladó, R., Xargay-Torrent, S., et al. (2020). DNA Methylation Reorganization of Skeletal Muscle-Specific Genes in Response to Gestational Obesity. Front. Physiol. 11, 938. doi:10.3389/fphys.2020.00938
Rahman, F., Pechnik, S., Gross, D., Sewell, L., and Goldstein, D. S. (2011). Low Frequency Power of Heart Rate Variability Reflects Baroreflex Function, Not Cardiac Sympathetic Innervation. Clin. Auton. Res. 21 (3), 133–141. doi:10.1007/s10286-010-0098-y
Ramírez-Vélez, R., Hernandez, A., Castro, K., Tordecilla-Sanders, A., González-Ruíz, K., Correa-Bautista, J. E., et al. (2016). High Intensity Interval-Vs Resistance or Combined-Training for Improving Cardiometabolic Health in Overweight Adults (Cardiometabolic HIIT-RT Study): Study Protocol for a Randomised Controlled Trial. Trials 17 (1), 298. doi:10.1186/s13063-016-1422-1
Ramírez-Vélez, R., Correa Rodriguez, M., Tordecilla-Sanders, A., Izquierdo, M., Correa-Bautista, J., Álvarez, C., et al. (2018). Exercise and Postprandial Lipaemia: Effects on Vascular Health in Inactive Adults. Lipids Health Dis. 17, 69. doi:10.1186/s12944-018-0719-3
Ramírez-Vélez, R., Tordecilla-Sanders, A., Téllez-T, L. A., Camelo-Prieto, D., Hernández-Quiñonez, P. A., Correa-Bautista, J. E., et al. (2020). Effect of Moderate- Versus High-Intensity Interval Exercise Training on Heart Rate Variability Parameters in Inactive Latin-American Adults: A Randomized Clinical Trial. J. Strength Cond Res. 34 (12), 3403–3415. doi:10.1519/JSC.0000000000001833
Riganello, F., Prada, V., Soddu, A., di Perri, C., and Sannita, W. G. (2019). Circadian Rhythms and Measures of CNS/Autonomic Interaction. Int. J. Environ. Res. Public Health 16 (13), 2336. doi:10.3390/ijerph16132336
Rizzo, R., Zhang, X., Wang, J. W. J. L., Lombardi, F., and Ivanov, P. C. (2020). Network Physiology of Cortico-Muscular Interactions. Front. Physiol. 11, 558070. doi:10.3389/fphys.2020.558070
Sammito, S., and Böckelmann, I. (2016). Factors Influencing Heart Rate Variability. Int. Cardiovasc. Forum J. 6, 18–22. doi:10.17987/icfj.v6i0.242
Sauvet, F., Leftheriotis, G., Gomez-Merino, D., Langrume, C., Drogou, C., Van Beers, P., et al. (2010). Effect of Acute Sleep Deprivation on Vascular Function in Healthy Subjects. J. Appl. Physiol. 108 (1), 68–75. doi:10.1152/japplphysiol.00851.2009
Sawyer, B. J., Tucker, W. J., Bhammar, D. M., Ryder, J. R., Sweazea, K. L., and Gaesser, G. A. (2016). Effects of High-Intensity Interval Training and Moderate-Intensity Continuous Training on Endothelial Function and Cardiometabolic Risk Markers in Obese Adults. J. Appl. Physiol. 121 (1), 279–288. doi:10.1152/japplphysiol.00024.2016
Schneider, C., Wiewelhove, T., Raeder, C., Flatt, A. A., Hoos, O., Hottenrott, L., et al. (2019). Heart Rate Variability Monitoring during Strength and High-Intensity Interval Training Overload Microcycles. Front. Physiol. 10 (582), 582. doi:10.3389/fphys.2019.00582
Seravalle, G., Mancia, G., and Grassi, G. (2018). Sympathetic Nervous System, Sleep, and Hypertension. Curr. Hypertens. Rep. 20 (9), 74. doi:10.1007/s11906-018-0874-y
Sharif, M. M., and Bahammam, A. S. (2013). Sleep Estimation Using BodyMedia's SenseWear Armband in Patients with Obstructive Sleep Apnea. Ann. Thorac. Med. 8 (1), 53–57. doi:10.4103/1817-1737.105720
Shin, M., Swan, P., and Chow, C. M. (2015). The Validity of Actiwatch2 and SenseWear Armband Compared against Polysomnography at Different Ambient Temperature Conditions. Sleep Sci. 8 (1), 9–15. doi:10.1016/j.slsci.2015.02.003
Sieck, G. C. (2017). Physiology in Perspective: The Importance of Integrative Physiology. Physiology 32 (3), 180–181. doi:10.1152/physiol.00009.2017
Soric, M., Turkalj, M., Kucic, D., Marusic, I., Plavec, D., and Misigoj-Durakovic, M. (2013). Validation of a Multi-Sensor Activity Monitor for Assessing Sleep in Children and Adolescents. Sleep Med. 14 (2), 201–205. doi:10.1016/j.sleep.2012.11.003
Stergiou, N., and Decker, L. M. (2011). Human Movement Variability, Nonlinear Dynamics, and Pathology: Is There a Connection? Hum. Move. Sci. 30 (5), 869–888. doi:10.1016/j.humov.2011.06.002
Stergiou, N., Harbourne, R. T., and Cavanaugh, J. T. (2006). Optimal Movement Variability: A New Theoretical Perspective for Neurologic Physical Therapy. J. Neurol. Phys. Ther. 30 (3), 120–129. doi:10.1097/01.npt.0000281949.48193.d9
Subramanian, S. K., Sharma, V. K., Arunachalam, V., Rajendran, R., and Gaur, A. (2019). Comparison of Baroreflex Sensitivity and Cardiac Autonomic Function between Adolescent Athlete and Non-Athlete Boys - A Cross-Sectional Study. Front. Physiol. 10, 1043. doi:10.3389/fphys.2019.01043
Thayer, J. F., Yamamoto, S. S., and Brosschot, J. F. (2010). The Relationship of Autonomic Imbalance, Heart Rate Variability and Cardiovascular Disease Risk Factors. Int. J. Cardiol. 141 (2), 122–131. doi:10.1016/j.ijcard.2009.09.543
Thompson, P. D., Franklin, B. A., Balady, G. J., Blair, S. N., Corrado, D., Estes, N. A., et al. (2007). Exercise and Acute Cardiovascular Events Placing the Risks into Perspective: A Scientific Statement from the American Heart Association Council on Nutrition, Physical Activity, and Metabolism and the Council on Clinical Cardiology. Circulation 115 (17), 2358–2368. doi:10.1161/CIRCULATIONAHA.107.181485
Tjønna, A. E., Lee, S. J., Rognmo, Ø., Stølen, T. O., Bye, A., Haram, P. M., et al. (2008). Aerobic Interval Training versus Continuous Moderate Exercise as a Treatment for the Metabolic Syndrome: A Pilot Study. Circulation 118 (4), 346–354. doi:10.1161/CIRCULATIONAHA.108.772822
Tobaldini, E., Costantino, G., Solbiati, M., Cogliati, C., Kara, T., Nobili, L., et al. (2017). Sleep, Sleep Deprivation, Autonomic Nervous System and Cardiovascular Diseases. Neurosci. Biobehav Rev. 74 (Pt B), 321–329. doi:10.1016/j.neubiorev.2016.07.004
Tobaldini, E., Nobili, L., Strada, S., Casali, K. R., Braghiroli, A., and Montano, N. (2013). Heart Rate Variability in normal and Pathological Sleep. Front. Physiol. 4, 294. doi:10.3389/fphys.2013.00294
Tobaldini, E., Pecis, M., and Montano, N. (2014). Effects of Acute and Chronic Sleep Deprivation on Cardiovascular Regulation. Arch. Ital. Biol. 152 (2-3), 103–110. doi:10.12871/000298292014235
Trejo-Gutierrez, J. F., and Fletcher, G. (2007). Impact of Exercise on Blood Lipids and Lipoproteins. J. Clin. Lipidol. 1 (3), 175–181. doi:10.1016/j.jacl.2007.05.006
Trimmel, K., Sacha, J., and Huikuri, H. V. (2015). Heart Rate Variability: Clinical Applications and Interaction between HRV and Heart Rate. Lausanne, Switzerland: Frontiers Media SA.
Tucker, W. J., Sawyer, B. J., Jarrett, C. L., Bhammar, D. M., Ryder, J. R., Angadi, S. S., et al. (2018). High-Intensity Interval Exercise Attenuates but Does Not Eliminate Endothelial Dysfunction after a Fast Food Meal. Am. J. Physiol. Heart Circ. Physiol. 314 (2), H188–H194. doi:10.1152/ajpheart.00384.2017
Tyldum, G. A., Schjerve, I. E., Tjønna, A. E., Kirkeby-Garstad, I., Stølen, T. O., Richardson, R. S., et al. (2009). Endothelial Dysfunction Induced by Post-Prandial Lipemia: Complete protection Afforded by High-Intensity Aerobic Interval Exercise. J. Am. Coll. Cardiol. 53 (2), 200–206. doi:10.1016/j.jacc.2008.09.033
Uchida, S., Shioda, K., Morita, Y., Kubota, C., Ganeko, M., and Takeda, N. (2012). Exercise and Sleep - Review and Future Directions. J. Phys. Fitness Sports Med. 1 (2), 317–324. doi:10.7600/jpfsm.1.317
van Leeuwen, W. M. A., Sallinen, M., Virkkala, J., Lindholm, H., Hirvonen, A., Hublin, C., et al. (2018). Physiological and Autonomic Stress Responses after Prolonged Sleep Restriction and Subsequent Recovery Sleep in Healthy Young Men. Sleep Biol. Rhythms 16 (1), 45–54. doi:10.1007/s41105-017-0122-x
Van Wouwe, N. C., Valk, P. J. L., and Veenstra, B. J. (2011). Sleep Monitoring: A Comparison between Three Wearable Instruments. Mil. Med. 176 (7), 811–816. doi:10.7205/milmed-d-10-00389
Vázquez, P., Hristovski, R., and Balagué, N. (2016). The Path to Exhaustion: Time-Variability Properties of Coordinative Variables during Continuous Exercise. Front. Physiol. 7 (37), 37. doi:10.3389/fphys.2016.00037
Vázquez, P., Petelczyc, M., Hristovski, R., and Balagué, N. (2020). Interlimb Coordination: A New Order Parameter and a Marker of Fatigue during Quasi-Isometric Exercise? Front. Physiol. 11 (1728), 612709. doi:10.3389/fphys.2020.612709
Vijayabaskaran, S., B., D., Damodaran, V., and Nagarajan, S. (2022). Association between Lipid Levels and Short-Term Heart Rate Variability (HRV) in Type 2 Diabetes. Egypt. Acad. J. Biol. Sci. C, Physiol. Mol. Biol. 14 (1), 21–30. doi:10.21608/eajbsc.2022.213921
Vitale, J. A., Bonato, M., La Torre, A. L., and Banfi, G. (2019). Heart Rate Variability in Sport Performance: Do Time of Day and Chronotype Play A Role? J. Clin. Med. 8 (5), 723. doi:10.3390/jcm8050723
Wallace, J. P., Johnson, B., Padilla, J., and Mather, K. (2010). Postprandial Lipaemia, Oxidative Stress and Endothelial Function: A Review. Int. J. Clin. Pract. 64 (3), 389–403. doi:10.1111/j.1742-1241.2009.02146.x
Weston, K. S., Wisløff, U., and Coombes, J. S. (2014). High-intensity Interval Training in Patients with Lifestyle-Induced Cardiometabolic Disease: a Systematic Review and Meta-Analysis. Br. J. Sports Med. 48 (16), 1227–1234. doi:10.1136/bjsports-2013-092576
WHO (2010). WHO Guidelines on Drawing Blood: Best Practices in Phlebotomy. Geneva, Switzerland: World Health Organization.
Wisløff, U., Støylen, A., Loennechen, J. P., Bruvold, M., Rognmo, Ø., Haram, P. M., et al. (2007). Superior Cardiovascular Effect of Aerobic Interval Training Versus Moderate Continuous Training in Heart Failure Patients: A Randomized Study. Circulation 115 (24), 3086–3094. doi:10.1161/CIRCULATIONAHA.106.675041
Wright, K. P., Drake, A. L., Frey, D. J., Fleshner, M., Desouza, C. A., Gronfier, C., et al. (2015). Influence of Sleep Deprivation and Circadian Misalignment on Cortisol, Inflammatory Markers, and Cytokine Balance. Brain Behav. Immun. 47, 24–34. doi:10.1016/j.bbi.2015.01.004
Wu, M.-C., Yang, Y.-C., Wu, J.-S., Wang, R.-H., Lu, F.-H., and Chang, C.-J. (2012). Short Sleep Duration Associated with a Higher Prevalence of Metabolic Syndrome in an Apparently Healthy Population. Prev. Med. 55 (4), 305–309. doi:10.1016/j.ypmed.2012.07.013
Keywords: network physiology of exercise, HIIE, postprandial lipemia, heart-rate variability, cardiovascular disease
Citation: Papadakis Z, Garcia-Retortillo S and Koutakis P (2022) Effects of Acute Partial Sleep Deprivation and High-Intensity Interval Exercise on Postprandial Network Interactions. Front. Netw. Physiol. 2:869787. doi: 10.3389/fnetp.2022.869787
Received: 05 February 2022; Accepted: 29 March 2022;
Published: 13 April 2022.
Edited by:
Ginés Viscor, University of Barcelona, SpainReviewed by:
Pablo Vázquez, Generalitat de CatalunyaAthanasios Jamurtas, University of Thessaly, Greece
Copyright © 2022 Papadakis, Garcia-Retortillo and Koutakis. This is an open-access article distributed under the terms of the Creative Commons Attribution License (CC BY). The use, distribution or reproduction in other forums is permitted, provided the original author(s) and the copyright owner(s) are credited and that the original publication in this journal is cited, in accordance with accepted academic practice. No use, distribution or reproduction is permitted which does not comply with these terms.
*Correspondence: Zacharias Papadakis, enBhcGFkYWtpc0BiYXJyeS5lZHU=