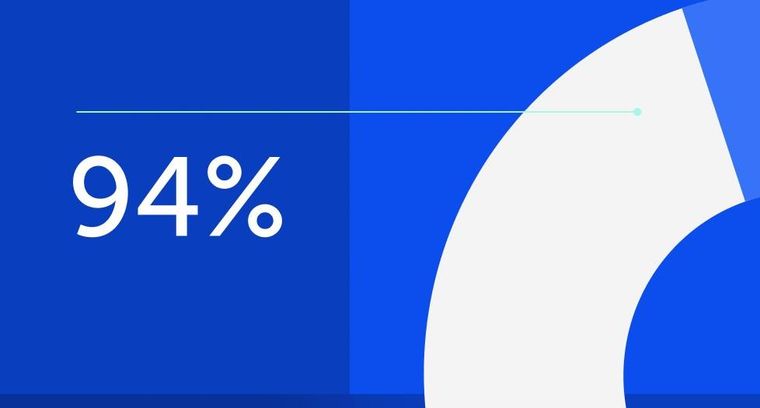
94% of researchers rate our articles as excellent or good
Learn more about the work of our research integrity team to safeguard the quality of each article we publish.
Find out more
CLINICAL TRIAL article
Front. Nephrol., 17 March 2025
Sec. Clinical Research in Nephrology
Volume 5 - 2025 | https://doi.org/10.3389/fneph.2025.1540471
This article is part of the Research TopicAdvancement in Kidney Care: Targeted Delivery and Precision Medicine ApproachesView all articles
Objectives: Kidney injury in patients with lupus nephritis (LN) results in pro-fibrotic biomarker expression, a manifestation also observed with calcineurin inhibitor (CNI) therapy. The second-generation CNI, voclosporin, is approved in the United States and Europe for the treatment of patients with active LN in combination with background immunosuppression, based on successful outcomes from the global phase 2 AURA-LV and phase 3 AURORA 1 studies, which demonstrated the efficacy of voclosporin across diverse racial and ethnic populations, and encompassing multiple biopsy classes of LN, alongside a favorable safety profile. This post hoc analysis examined changes from baseline levels of serum and urinary biomarkers, including pro-fibrotic biomarkers, in a cohort of patients from the parent AURORA 1 study
Methods: Samples were analyzed from a cohort of patients in AURORA 1 treated with voclosporin (23.7 mg twice daily, n=57) or placebo (n=59) in combination with mycophenolate mofetil (MMF) and low-dose glucocorticoids, including in a subgroup of patients that experienced a ≥30% decline from baseline in estimated glomerular filtration rate (voclosporin, n=26; placebo, n=20).
Results: The addition of voclosporin to MMF and low-dose glucocorticoids for the treatment of LN did not result in significant differences in normalized urinary concentrations of KIM-1, TGF-β1, MCP-1, or NGAL, biomarkers indicative of renal fibrosis and kidney damage, when compared to MMF and low-dose glucocorticoids alone.
Conclusion: These findings further support the safety of voclosporin for the treatment of LN in adult patients.
Clinical Trial Registration: ClinicalTrials.gov, identifier NCT03021499; EudraCT, identifier 2016-004045-81.
Lupus nephritis (LN) is one of the most severe clinical manifestations of systemic lupus erythematosus (SLE) and is a major cause of morbidity and mortality in this patient population, occurring in around half of the patients diagnosed with SLE. Furthermore, 40% of patients with LN are thought to experience varying degrees of renal impairment, with 10-30% of patients with LN progressing to chronic kidney failure within 15 years of diagnosis (1–4). The past five decades have witnessed significant advances in treatment options for SLE patients, including the introduction of calcineurin inhibitors (CNIs) to the immunosuppressive regimen, a course of therapy associated with improved prognosis (5, 6). Early studies with first-generation CNIs (cyclosporine A [CsA] and tacrolimus [TAC]) report improved efficacy in LN patients (7–9). Recently, the second-generation CNI, voclosporin, has shown to be a promising alternative to first-generation CNIs in the treatment of LN (10, 11).
Voclosporin, in combination with a background immunosuppressive regimen, is the first approved oral therapy for adult patients with active LN in the United States and Europe based on safety and efficacy outcomes from two pivotal, global, clinical studies, the AURA-LV phase 2 and AURORA 1 phase 3 studies (10, 11). Moreover, an additional two years of safety and efficacy data were reported for the phase 3 AURORA 2 continuation study (12). Outcomes demonstrated improved renal response rates in patients treated with voclosporin, compared to the control arm of placebo, both combined with mycophenolate mofetil (MMF) and low-dose glucocorticoids. Voclosporin demonstrated efficacy across diverse racial and ethnic populations, and multiple biopsy classes of LN. Improved kidney function, indicated by significant decreases in proteinuria with stable estimated glomerular filtration rate (eGFR), without a clinically meaningful impact on serum electrolytes, was accompanied by safety profiles, comparable to patients treated with MMF and low-dose glucocorticoids alone, following three years of treatment (10–12).
CNI immunosuppression derives from reduced T-cell activation via calcineurin inhibition. Distinct from immunosuppression, CNI use is associated with nephrotoxicity, resulting in considerable impact on the clinical management of patients requiring CNI therapy, including kidney and non-kidney solid organ transplantation patients. Classed as acute or chronic, dependent on the reversibility of functional changes, nephrotoxicity observed with long-term administration of CsA and TAC is associated with progressive kidney damage following solid organ transplantation (13–16).
Kidney fibrosis, in particular “striped fibrosis”, is a well-described yet unique form of nephrotoxicity associated with CNI use (17). Whilst the mechanism is not fully understood, chronic ischemia along the medullary rays of the kidney may contribute to tubular drop-out and fibrosis. Other factors may include direct stimulation of transforming growth factor beta (TGF-β) by renal tubular epithelium (18). A number of pro-fibrotic and pro-inflammatory markers, including kidney injury molecule-1 (KIM-1), neutrophil gelatinase-associated lipocalin (NGAL), monocyte chemoattractant protein-1 (MCP-1) and TGF-β1 are linked to kidney injury and fibrosis in patients with LN and in patients exposed to CNIs (19–21). Thus, it would be beneficial for a CNI to exhibit efficacy whilst limiting treatment-related adverse events (AEs). Electrolyte derangement is another indicator of kidney damage, with hypomagnesemia and hyperkalemia often evident in patients receiving CNI therapy.
Based on AURORA 1, where improved urine protein creatinine ratio (UPCR) was observed without a clinically meaningful impact on serum electrolytes, including magnesium and potassium, the current study reports on a post hoc analysis of biomarker profiles in a sample of AURORA 1 patients with active LN. Pro-fibrotic biomarker expression was evaluated in LN patients treated with voclosporin or placebo, both in combination with MMF and low-dose glucocorticoids. Additionally, to rule out dietary impact on the levels of serum electrolytes, urinary electrolytes were analyzed in both treatment arms.
Details of the AURORA 1 study have been reported previously, however, the inclusion and exclusion criteria for AURORA 1 can also be found in the Supplementary Information (Supplementary Table S1) (11).
Briefly, patients with biopsy-proven (Class III, IV or V ± III/IV) active LN according to American College of Rheumatology (ACR) criteria, proteinuria ≥1.5 g/g (≥2 g/g for pure Class V), and eGFR >45 mL/min/1.73 m2, were eligible to enroll and were randomized to the voclosporin arm (23.7 mg twice daily [BID], n=179) or control arm receiving placebo (n=178) for the study duration (52 weeks). All patients received MMF (target dose 1 g, BID) and low-dose oral glucocorticoids (tapered over 16 weeks to 2.5 mg/day). Given the known hemodynamic effects of CNIs, eGFR was monitored and guidance provided on study drug dose modification for patients experiencing decreases.
Patients provided written informed consent prior to trial-related activities, in accordance with the Declaration of Helsinki and International Council for Harmonisation of Technical Requirements for Pharmaceuticals for Human Use and Good Clinical Practice guidelines. An Institutional Review Board or Independent Ethics Committee at each site approved the informed consent form and protocol. AURORA 1 is registered with ClinicalTrials.gov (NCT03021499) and EudraCT (2016–004045–81).
For this current study, a random sample of 60 patients per treatment arm was selected with approximately 50% treatment responders, 50% non-responders, and all patients that experienced a ≥30% decrease from baseline in eGFR (confirmed based on two consecutive eGFR measures). Treatment responders were defined as those with >50% reduction from baseline in UPCR at Week 52; all patients not achieving at 50% reducing in UPCR, including patients with missing data, were considered non-responders. Samples obtained at baseline and end of treatment visits were analyzed; the number of patients with samples for each analysis is detailed in Supplementary Figure S1.
Biomarker analysis of urine and serum samples was carried out by Myriad RBM (Austin, Texas, USA). Biomarker analysis included several inflammatory cytokines and chemokines (Supplementary Table S2). Change from baseline (CFB) was calculated by subtracting a patient’s baseline from their EoT value, providing an absolute value. All urinary biomarkers were normalized to urinary creatinine to account for changes in urine concentration. All analyses are presented here as Least Square (LS) means.
Urinary electrolyte analysis was performed at Medpace, Inc. (Stirling, UK) and comprised 24-hour urine samples analyzed for creatinine, magnesium, potassium and sodium and the change in each from baseline. As well as data for 24-hour urine electrolyte excretion rates, changes in absolute concentration of each urinary electrolyte were also analyzed.
Statistical analysis of biomarker and electrolyte expression was performed by comparing voclosporin and control treatment arms, using data from an all-patient cohort (which included all responders, non-responders and patients who experienced a ≥30% decrease in eGFR; urine biomarkers n=50; serum biomarkers n=108; electrolytes n=116), a responder cohort (urine biomarkers n=27; serum biomarkers n=50; electrolytes n=56), a non-responder cohort (urine biomarkers n=23; serum biomarkers n=58; electrolytes n=60) and a cohort of all patients who experienced a ≥30% decrease in eGFR in AURORA 1 (urine biomarkers n=21; serum biomarkers n=42; electrolytes n=46). The study was not powered to detect differences between cohorts.
Each parameter was summarized at baseline using a general linear model incorporating a covariate for treatment arm. LS means and standard error (SE) were calculated from this model. End of treatment and CFB data were analyzed using a similar model that included a covariate for the baseline of the applicable parameter. LS means and SE for results within each treatment arm were calculated. This model assessed differences in the CFB to EoT between arms, summarized using the estimate of treatment difference, a SE, and 95% confidence interval (CI).
Of the random sample of 120 patients from AURORA 1, four patients were excluded because they did not have urinary or serum samples. In total, 116 patients (voclosporin arm, n=57; control arm, n=59) were included in the study with 50 patients as part of the urinary biomarker analysis, 108 patients contributing to the serum biomarker analysis and 116 patients with samples for the electrolyte analysis.
For patients in the eGFR decrease cohort, median (interquartile range [IQR]) treatment duration was 22.9 (15.79 – 37.43) weeks in the control arm (n=20) and 26.2 (16.71 – 51.14) weeks in the voclosporin arm (n=26). For all other patients, the median (IQR) treatment duration was 52 (50.86, 53.14) weeks in the control arm and 52 (51.14, 53.14) weeks in the voclosporin arm.
Of the 43 urinary analytes assayed, 27 had consistently measurable levels. There were no significant changes from baseline to EoT in markers associated with acute nephrotoxicity or kidney fibrosis (KIM-1, MCP-1, NGAL or latency-associated peptide [LAP] TGF-β) when comparing across treatment arms (Table 1; Supplementary Table S3). This was consistent in responder and non-responder patients (data not shown) as well as in patients who experienced a ≥30% decrease in their eGFR (Supplementary Table S3).
Table 1. Normalized urinary kidney injury and pro-fibrotic biomarker levels in all-patient and eGFR decrease cohorts.
Of the 27 measurable urinary biomarkers, a change in the baseline expression of only three biomarkers was found to differ significantly between treatment arms at EoT in the all-patient and eGFR cohorts. Whilst decreased levels of eotaxin-1, interleukin (IL)-1β and IL-1α were observed at EoT compared to baseline in all voclosporin-treated patients, in contrast, increased expression of all three biomarkers was observed in all placebo-treated patients. A similar effect was seen with eotaxin-1 and IL-1β in patients that experienced a ≥30% decline in their eGFR (Supplementary Table S3). Voclosporin treatment did not impact the CFB expression of any other biomarker when compared to patients in the control arm (Supplementary Table S3). Absolute levels of urinary biomarkers can be found in Supplementary Table S4.
Of the 30 serum biomarkers assessed, 27 had consistently measurable levels and, as with urinary analysis, KIM-1 expression remained unchanged in the serum of any patient cohort (Supplementary Table S5). All-patient cohort analyses identified a significant difference in the CFB expression in macrophage migration inhibitory factor (MIF) in the voclosporin arm, compared to the control arm, with a smaller decrease from baseline observed in voclosporin-treated patients than the decrease seen in the control arm (Supplementary Table S5). In patients who experienced a ≥30% decrease in eGFR, a significantly different change in baseline expression was found only for B lymphocyte chemoattractant (BLC), with an increase from baseline in the voclosporin treatment arm compared to a decrease from baseline in the control arm at EoT (Supplementary Table S5).
Previously published AURORA 1 data reports stable electrolytes throughout the study with no observed evidence of tubular toxicity (11). Mean values for electrolytes were stable throughout the study; importantly, magnesium (Baseline [mean ± SD], 2.093 ± 0.2074 mg/dL; EoT [mean ± SD], 2.043 ± 0.2115 mg/dL) and potassium (Baseline [mean ± SD], 4.089 ± 0.4857 mmol/L; EoT [mean ± SD], 4.183 ± 0.4233 mmol/L) remained within normal limits (magnesium, 1.8 – 2.4 mg/dL; potassium, 3.5 – 5.1 mmol/L). Moreover, there were no significant differences in the fractional excretion (%) of magnesium, sodium, or potassium at EoT observed in this post hoc analysis (Supplementary Table S6).
The current study assessed urine and serum analytes in a sample of placebo- and voclosporin-treated patients with active LN from the AURORA 1 study, including patients who experienced an eGFR decrease ≥30% during the trial. Voclosporin treatment was not associated with the expression of biomarkers indicative of kidney fibrosis or damage (KIM-1, TGF-β, NGAL or MCP-1) and demonstrated favorable electrolyte homeostasis.
Numerous preclinical studies have reported on the expression of pro-fibrotic biomarkers, such as KIM-1, TGF-β, NGAL or MCP-1, in relation to kidney function. For example, MCP-1, which is found in rat renal interstitial fibroblasts and damaged cortical tubules, shows increased expression with interstitial inflammation and fibrosis, alongside another pro-fibrotic marker, TGF-β (22–24). Increased NGAL expression has also been reported in proximal tubule cells in a mouse model of acute kidney injury (AKI) and in a model of drug-induced nephrotoxicity, with the authors suggesting that NGAL may be suitable as a urinary biomarker in ischemic and nephrotoxic renal injury (25).
Clinically, expression of the aforementioned biomarkers may indicate the progression of kidney disease (CKD). Kidney fibrosis is commonly observed in CKD, and TGF-β is a critical pro-fibrotic molecule which up-regulates matrix protein synthesis, inhibits matrix degradation, and alters the epithelial mesenchymal transition (26). KIM-1 is associated with an increased incidence of interstitial fibrosis. Given that KIM-1 is expressed in regenerating proximal tubules, it has been proposed as a useful biomarker for tubular kidney injury (19, 27–31). Further, KIM-1 levels have been found to be significantly higher in patients with SLE and active kidney disease, compared with patients without renal activity, an association also seen with the biomarkers MCP-1 and NGAL (32–34). Additionally, both MCP-1 and NGAL are correlated with renal decline and allograft failure in transplant patients.
Given the considerable overlap in the expression of KIM-1, MCP-1, TGF-b and NGAL in kidney injury, several studies have looked at the concurrent expression of two or more markers as a more accurate indicator of renal function (30, 35). This includes the Renal Activity Index for Lupus (RAIL) which is based on the expression of six urinary biomarkers including NGAL, MCP-1, and KIM-1 (36).
Over and above the effect seen with kidney injury, the use of traditional CNIs such as CsA and TAC is also known to result in pro-fibrotic marker expression. Studies in rats treated with CsA or TAC have demonstrated renal function decline and interstitial fibrosis accompanied by increased expression of TGF-β, NGAL, MCP-1 and KIM-1, attributed to CNI-induced nephrotoxicity (37–43). Future studies in preclinical animal models could provide insight into the mechanistic differentiation of voclosporin from CsA and TAC. There is also evidence of both CsA and TAC increasing TGF-β production in the kidneys of transplant patients, correlated with diffuse interstitial fibrosis and graft atherosclerosis (44–50). Fibrogenic effects in patients undergoing kidney transplantation with increased serum levels of TAC and proximal tubule injury are also accompanied by increased levels of KIM-1 (19).
The lack of significant changes between treatment arms in urinary or serum levels of any of the biomarkers shown to be associated with kidney injury or fibrosis suggests no clinically meaningful bearing on the biomarkers analyzed, regardless of treatment outcome. Furthermore, the lack of biomarker expression seen in patients treated with voclosporin, MMF and low-dose glucocorticoids also suggests a concurrent lack of acute tubular injury in these patients.
Across the entire patient cohort in this post hoc analysis, the change in expression from baseline to EoT of a very small proportion of biomarkers were found to be significantly altered in the urine (eotaxin-1, IL-1α and IL-1β) and serum (MIF and BCL) of voclosporin-treated patients, however, the clinical relevance of these changes is, as yet, undetermined. Previous studies have reported increased eotaxin levels in the plasma of LN patients and SLE patients with organ damage and IL-1β and IL-1 levels are increased in the kidneys of mice with LN (51). Although IL-1α in LN has received limited attention it has been reported to contribute to glomerulonephritis in mice (52). Macrophage migration inhibitory factor has been shown to play a role in kidney dysfunction with possible therapeutic potential in LN patients (53, 54). B lymphocyte chemoattractant may also play a role in SLE and LN pathogenesis, with higher serum levels in patients with SLE, further increased in patients with LN (55). Given that calcineurin is activated by increased intracellular Ca2+ concentrations and plays an essential role in many signalling pathways, inhibition by voclosporin will affect many pathways and is likely responsible for the changes observed in other biomarkers.
The stable mean levels of blood pressure and electrolytes observed throughout AURORA 1 is suggestive of a lack of tubular toxicity. These findings were also consistent with results from the phase 2 AURA-LV study, the phase 3 AURORA 2 study, and further confirmed by an integrated data analysis from combined phase 2 AURA-LV and phase 3 AURORA 1 clinical studies (10–12, 56). In contrast, use of traditional CNIs is known to lead to electrolyte and metabolic abnormalities. Indeed, CsA- and TAC-induced immunosuppression has been shown to impact electrolyte levels in kidney transplant patients (57, 58). However, it is possible that dietary supplementation of electrolytes may correct serum electrolyte imbalances. The post hoc analysis presented here demonstrates, once again, that voclosporin treatment does not significantly impact serum or urine magnesium, potassium, or sodium levels confirming no impact of dietary supplementation on the previous findings in serum concentrations of electrolytes for this patient cohort. Voclosporin treatment reduced urinary magnesium 24-hour excretion in patients who experienced an eGFR decrease ≥30%, however, as previously mentioned, LS mean levels remained within normal limits. Hypomagnesemia, via renal magnesium wasting, is common in CsA- and TAC-treated patients, presumably due to effects on reabsorption. Hypomagnesemia is also implicated as a significant contributor to nephrotoxicity associated with CsA and TAC and, notably, features on the labeling for both CNIs (15, 58–60). Previously reported study data from AURORA 1 confirmed no unexpected safety signals, unanticipated AEs, or evidence of chronic nephrotoxicity (11).
The analysis presented here includes a small subgroup of AURORA 1 patients treated for up to 52 weeks and results may not be generalizable to the overall population. No trends indicating nephrotoxicity emerged, however, nephrotoxicity associated with traditional CNIs is observed following long-term administration. The 52-week study duration of AURORA 1 limits the ability of these findings to be extrapolated to chronic or long-term exposure effects. Notably, the AURORA 2 clinical trial provides follow up data on up to 3 years of treatment exposure with voclosporin in a larger cohort, demonstrating sustained efficacy and safety, whilst maintaining electrolyte homeostasis (12). Histopathological evaluation after 18 months of treatment in a small subset of patients from AURORA 2 confirmed exposure to study treatment was not associated with nephrotoxicity (12).
In conclusion, voclosporin treatment in LN patients does not induce common indicators of nephrotoxicity such as pro-fibrotic markers or electrolyte imbalance. These data support a much-improved biomarker profile for voclosporin over traditional CNIs.
The datasets presented in this article are not readily available due to patient privacy reasons. Requests to access the datasets should be directed to TFJlaGF1bWVAYXVyaW5pYXBoYXJtYS5jb20=.
Detailed information on study conduct and sites has previously been published (11). AURORA 1 was conducted in accordance with local legislation and institutional requirements. AURORA 1 was approved by an independent Data and Safety Monitoring Board who monitored the safety of study participants and efficacy of treatment. An Institutional Review Board or Independent Ethics Committee at each participating site reviewed and approved the informed consent form and protocol. The participants provided their written informed consent to participate in this study. The trial is registered with ClinicalTrials.gov (NCT03021499) and with EudraCT (2016-004045-81).
BFP: Conceptualization, Formal Analysis, Methodology, Data curation, Writing – review & editing. JAT: Conceptualization, Data curation, Formal Analysis, Writing – review & editing. JR: Conceptualization, Data curation, Formal Analysis, Methodology, Writing – review & editing. LMR: Conceptualization, Data curation, Formal Analysis, Methodology, Project administration, Validation, Visualization, Writing – original draft, Writing – review & editing. JLC: Conceptualization, Data curation, Formal Analysis, Methodology, Project administration, Investigation, Validation, Writing – original draft, Writing – review & editing. RBH: Conceptualization, Data curation, Formal Analysis, Methodology, Investigation, Validation, Writing – original draft, Writing – review & editing.
The author(s) declare that financial support was received for the research and/or publication of this article. The study and publication were funded by Aurinia Pharmaceuticals Inc.
C. R. Parikh contributed to the initial planning of the study. The manuscript was drafted by Kara McNair (MediComm Partners, London, UK) and managed by Vanessa Birardi (Aurinia Pharmaceuticals Inc., Rockville, MD, USA). We also thank Matt Truman, study statistician.
JAT reports research grants from Aurinia Pharmaceuticals Inc., JR was on the advisory board and a consultant for Aurinia Pharmaceuticals Inc. LMR is an employee and shareholder of Aurinia Pharmaceuticals Inc., JLC and RBH are former employees of Aurinia Pharmaceuticals Inc. RBH is a consultant and shareholder of Aurinia Pharmaceuticals Inc.
The remaining author (BFP) declares that the research was conducted in the absence of any commercial or financial relationships that could be constructed as a potential conflict of interest.
The authors declare that this study received funding from Aurinia Pharmaceuticals Inc. The funder had the following involvement in the study: designed, ran, and funded the AURORA 1 clinical trial; provided samples and funded this substudy; and employed LMR, JLC and RBH.
The author(s) declare that no Generative AI was used in the creation of this manuscript.
All claims expressed in this article are solely those of the authors and do not necessarily represent those of their affiliated organizations, or those of the publisher, the editors and the reviewers. Any product that may be evaluated in this article, or claim that may be made by its manufacturer, is not guaranteed or endorsed by the publisher.
The Supplementary Material for this article can be found online at: https://www.frontiersin.org/articles/10.3389/fneph.2025.1540471/full#supplementary-material
1. Davidson A. What is damaging the kidney in lupus nephritis? Nat Rev Rheumatol. (2016) 12:143–53. doi: 10.1038/nrrheum.2015.159
2. Fanouriakis A, Kostopoulou M, Cheema K, Anders HJ, Aringer M, Bajema I, et al. 2019 Update of the Joint European League Against Rheumatism and European Renal Association-European Dialysis and Transplant Association (EULAR/ERA-EDTA) recommendations for the management of lupus nephritis. Ann Rheum Dis. (2020) 79:713–23. doi: 10.1136/annrheumdis-2020-216924
3. Rovin BH, Adler SG, Barratt J, Bridoux F, Burdge KA, Chan TM, et al. KDIGO 2021 clinical practice guideline for the management of glomerular diseases. Kidney Int. (2021) 100:S1–S276. doi: 10.1016/j.kint.2021.05.021
4. Parikh SV, Almaani S, Brodsky S, Rovin BH. Update on lupus nephritis: core curriculum 2020. Am J Kidney Dis. (2020) 76:265–81. doi: 10.1053/j.ajkd.2019.10.017
5. Tektonidou MG, Dasgupta A, Ward MM. Risk of end-stage renal disease in patients with lupus nephritis, 1971–2015: A systematic review and bayesian meta-analysis. Arthritis Rheumatol. (2016) 68:1432–41. doi: 10.1002/art.39594
6. Mok CC. Calcineurin inhibitors in systemic lupus erythematosus. Best Pract Res Clin Rheumatol. (2017) 31:429–38. doi: 10.1016/j.berh.2017.09.010
7. Szeto CC, Kwan BC, Lai FM, Tam LS, Li EK, Chow KM, et al. Tacrolimus for the treatment of systemic lupus erythematosus with pure class V nephritis. Rheumatol (Oxford). (2008) 47:1678–81. doi: 10.1093/rheumatology/ken335
8. Zavada J, Pesickova S, Rysava R, Olejarova M, Horák P, Hrncír Z, et al. Cyclosporine A or intravenous cyclophosphamide for lupus nephritis: the Cyclofa-Lune study. Lupus. (2010) 19:1281–9. doi: 10.1177/0961203310371155
9. Miyasaka N, Kawai S, Hashimoto H. Efficacy and safety of tacrolimus for lupus nephritis: a placebo-controlled double-blind multicenter study. Mod Rheumatol. (2009) 19:606–15. doi: 10.3109/s10165-009-0218-5
10. Rovin BH, Solomons N, Pendergraft WF 3rd, Dooley MA, Tumlin J, Romero-Diaz J, et al. A randomized, controlled double-blind study comparing the efficacy and safety of dose-ranging voclosporin with placebo in achieving remission in patients with active lupus nephritis. Kidney Int. (2019) 95:219–31. doi: 10.1016/j.kint.2018.08.025
11. Rovin BH, Teng YKO, Ginzler EM, Arriens C, Caster DJ, Romero-Diaz J, et al. Efficacy and safety of voclosporin versus placebo for lupus nephritis (AURORA 1): a double-blind, randomised, multicentre, placebo-controlled, phase 3 trial. Lancet. (2021) 397:2070–80. doi: 10.1016/S0140-6736(21)00578-X
12. Saxena A, Ginzler EM, Gibson K, Satirapoj B, Santillán AEZ, Levchenko O, et al. Safety and efficacy of long-term voclosporin treatment for lupus nephritis in the phase 3 AURORA 2 clinical trial. Arthritis Rheumatol. (2024) 76:59–67. doi: 10.1002/art.42657
13. Naesens M, Kuypers DR, Sarwal M. Calcineurin inhibitor nephrotoxicity. Clin J Am Soc Nephrol. (2009) 4:481–508. 10.2215/CJN.04800908
14. Nankivell BJ, Borrows RJ, Fung CL, O’Connell PJ, Allen RD, Chapman JR. The natural history of chronic allograft nephropathy. N Engl J Med. (2003) 349:2326–33. doi: 10.1056/NEJMoa020009
15. Farouk SS, Rein JL. The many faces of calcineurin inhibitor toxicity-what the FK? Adv Chronic Kidney Dis. (2020) 27:56–66. doi: 10.1053/j.ackd.2019.08.006
16. Hošková L, Málek I, Kopkan L, Kautzner J. Pathophysiological mechanisms of calcineurin inhibitor-induced nephrotoxicity and arterial hypertension. Physiol Res. (2017) 66:167–80. doi: 10.33549/physiolres
17. Nankivell BJ, P’Ng CH, O’Connell PJ, Chapman JR. Calcineurin inhibitor nephrotoxicity through the lens of longitudinal histology: comparison of cyclosporine and tacrolimus eras. Transplantation. (2016) 100:1723–31. doi: 10.1097/TP.0000000000001243
18. Akool el S, Doller A, Babelova A, Tsalastra W, Moreth K, Schaefer L, et al. Molecular mechanisms of TGF beta receptor-triggered signaling cascades rapidly induced by the calcineurin inhibitors cyclosporin A and FK506. J Immunol. (2008) 181:2831–45. doi: 10.4049/jimmunol.181.4.2831
19. Cosner D, Zeng X, Zhang PL. Proximal tubular injury in medullary rays is an early sign of acute tacrolimus nephrotoxicity. J Transplant. (2015) 2015:142521. doi: 10.1155/2015/142521
20. Susianti H, Wijaya JW, Rastini A, Handono K, Gunawan A, Kalim H. Urinary neutrophil gelatinase-associated lipocalin to monitor lupus nephritis disease activity. biomark Insights. (2015) 10:81–7. doi: 10.4137/BMI.S27625
21. Khanna A, Plummer M, Bromberek C, Bresnahan B, Hariharan S. Expression of TGF-beta and fibrogenic genes in transplant recipients with tacrolimus and cyclosporine nephrotoxicity. Kidney Int. (2002) 62:2257–63. doi: 10.1046/j.1523-1755.2002.00668.x
22. Eddy AA, Giachelli CM. Renal expression of genes that promote interstitial inflammation and fibrosis in rats with protein-overload proteinuria. Kidney Int. (1995) 47:1546–57. doi: 10.1038/ki.1995.218
23. González-Cuadrado S, Bustos C, Ruiz-Ortega M, Ortiz A, Guijarro C, Plaza JJ, et al. Expression of leucocyte chemoattractants by interstitial renal fibroblasts: up-regulation by drugs associated with interstitial fibrosis. Clin Exp Immunol. (1996) 106:518–22. doi: 10.1046/j.1365-2249.1996.d01-864.x
24. Tesch GH, Schwarting A, Kinoshita K, Lan HY, Rollins BJ, Kelley VR. Monocyte chemoattractant protein-1 promotes macrophage-mediated tubular injury, but not glomerular injury, in nephrotoxic serum nephritis. J Clin Invest. (1999) 103:73–80. doi: 10.1172/JCI4876
25. Mishra J, Ma Q, Prada A, Mitsnefes M, Zahedi K, Yang J, et al. Identification of neutrophil gelatinase-associated lipocalin as a novel early urinary biomarker for ischemic renal injury. J Am Soc Nephrol. (2003) 14:2534–43. doi: 10.1097/01.asn.0000088027.54400.c6
26. Frangogiannis N. Transforming growth factor-β in tissue fibrosis. J Exp Med. (2020) 217:e20190103. doi: 10.1084/jem.20190103
27. Han WK, Bailly V, Abichandani R, Thadhani R, Bonventre JV. Kidney Injury Molecule-1 (KIM-1): a novel biomarker for human renal proximal tubule injury. Kidney Int. (2002) 62:237–44. doi: 10.1046/j.1523-1755.2002.00433.x
28. van Timmeren MM, Vaidya VS, van Ree RM, Oterdoom LH, de Vries AP, Gans RO, et al. High urinary excretion of kidney injury molecule-1 is an independent predictor of graft loss in renal transplant recipients. Transplantation. (2007) 84:1625–30. doi: 10.1097/01.tp.0000295982.78039.ef
29. Nogare AL, Veronese FV, Carpio VN, Montenegro RM, Pedroso JA, Pegas KL, et al. Kidney injury molecule-1 expression in human kidney transplants with interstitial fibrosis and tubular atrophy. BMC Nephrol. (2015) 16:19. doi: 10.1186/s12882-015-0011-y
30. Shinke H, Masuda S, Togashi Y, Ikemi Y, Ozawa A, Sato T, et al. Urinary kidney injury molecule-1 and monocyte chemotactic protein-1 are noninvasive biomarkers of cisplatin-induced nephrotoxicity in lung cancer patients. Cancer Chemother Pharmacol. (2015) 76:989–96. doi: 10.1007/s00280-015-2880-y
31. Wajda J, Dumnicka P, Kolber W, Sporek M, Maziarz B, Ceranowicz P, et al. The marker of tubular injury, kidney injury molecule-1 (KIM-1), in acute kidney injury complicating acute pancreatitis: A preliminary study. J Clin Med. (2020) 9(5):1463. doi: 10.3390/jcm9051463
32. Ghobrial EE, El Hamshary AA, Mohamed AG, Abd El Raheim YA, Talaat AA. Urinary monocyte chemoattractant protein-1 as a biomarker of lupus nephritis activity in children. Saudi J Kidney Dis Transpl. (2015) 26:507–15.
33. Cantaluppi V, Dellepiane S, Tamagnone M, Medica D, Figliolini F, Messina M, et al. Neutrophil gelatinase associated lipocalin is an early and accurate biomarker of graft function and tissue regeneration in kidney transplantation from extended criteria donors. PloS One. (2015) 10:e0129279. doi: 10.1371/journal.pone.0129279
34. Nozaki Y, Shiga T, Ashida C, Tomita D, Itami T, Kishimoto K, et al. U-KIM-1 as a predictor of treatment response in lupus nephritis. Lupus. (2023) 32:54–62. doi: 10.1177/09612033221135871
35. Ding Y, Nie LM, Pang Y, Wu WJ, Tan Y, Yu F, et al. Composite urinary biomarkers to predict pathological tubulointerstitial lesions in lupus nephritis. Lupus. (2018) 27:1778–89. doi: 10.1177/0961203318788167
36. Brunner HI, Bennett MR, Abulaban K, Klein-Gitelman MS, O’Neil KM, Tucker L, et al. Development of a novel renal activity index of lupus nephritis in children and young adults. Arthritis Care Res (Hoboken). (2016) 68:1003–11. doi: 10.1002/acr.22762
37. Carlos CP, Sonehara NM, Oliani SM, Burdmann EA. Predictive usefulness of urinary biomarkers for the identification of cyclosporine A-induced nephrotoxicity in a rat model. PloS One. (2014) 9:e103660. doi: 10.1371/journal.pone.0103660
38. Fu R, Tajima S, Shigematsu T, Zhang M, Tsuchimoto A, Egashira N, et al. Establishment of an experimental rat model of tacrolimus-induced kidney injury accompanied by interstitial fibrosis. Toxicol Lett. (2021) 341:43–50. doi: 10.1016/j.toxlet.2021.01.020
39. Kędzierska K, Sindrewicz K, Sporniak-Tutak K, Gołembiewska E, Zair L, Sieńko J, et al. Does immunosuppressive therapy affect markers of kidney damage? Ann Transplant. (2016) 21:137–44. doi: 10.12659/aot.895275
40. Tsuchimoto A, Shinke H, Uesugi M, Kikuchi M, Hashimoto E, Sato T, et al. Urinary neutrophil gelatinase-associated lipocalin: a useful biomarker for tacrolimus-induced acute kidney injury in liver transplant patients. PloS One. (2014) 9:e110527. doi: 10.1371/journal.pone.0110527
41. Stypmann J, Fobker M, Rosing K, Engelen M, Gunia S, Dell’Aquila AM, et al. Neutrophil gelatinase-associated lipocalin (NGAL) in heart transplant recipients after conversion to everolimus therapy. J Cardiol. (2015) 66:347–52. doi: 10.1016/j.jjcc.2014.12.010
42. Gacka E, Życzkowski M, Bogacki R, Paradysz A, Hyla-Klekot L. The usefulness of determining neutrophil gelatinase-associated lipocalin concentration excreted in the urine in the evaluation of cyclosporine A nephrotoxicity in children with nephrotic syndrome. Dis Markers. (2016) 2016:6872149. doi: 10.1155/2016/6872149
43. Chancharoenthana W, Leelahavanichkul A, Wattanatorn S, Avihingsanon Y, Praditpornsilpa K, Eiam-Ong S, et al. Alteration of urinary neutrophil gelatinase-associated lipocalin as a predictor of tacrolimus-induced chronic renal allograft fibrosis in tacrolimus dose adjustments following kidney transplantation. PloS One. (2018) 13:e0209708. doi: 10.1371/journal.pone.0209708
44. Mohamed MA, Robertson H, Booth TA, Balupuri S, Gerstenkorn C, Kirby JA, et al. Active TGF-beta1 expression in kidney transplantation: a comparative study of cyclosporin-A (CyA) and tacrolimus (FK506). Transpl Int. (2000) 13 Suppl 1:S295–8. doi: 10.1007/s001470050346
45. Mohamed MA, Robertson H, Booth TA, Balupuri S, Kirby JA, Talbot D. TGF-beta expression in renal transplant biopsies: a comparative study between cyclosporin-A and tacrolimus. Transplantation. (2000) 69:1002–5. doi: 10.1097/00007890-200003150-00059.
46. Matl I, Viklický O, Voska L, Lodererová A, Vítko S. The effect of different immunosuppressive regimens on TGF-beta1 expression in kidney transplant patients. Transpl Int. (2005) 18:668–71. doi: 10.1111/j.1432-2277.2005.00115.x
47. Citterio F, Pozzetto U, Romagnoli J, Tondolo E, Silvestri P, Nanni G, et al. Plasma levels of transforming growth factor-beta1 in renal transplant recipients receiving different immunosuppressive regimens. Transplant Proc. (2004) 36:698–9. doi: 10.1016/j.transproceed.2004.03.014
48. Khanna A, Plummer M, Bromberek C, Bresnahan B, Hariharan S. Expression of TGF-β and fibrogenic genes in transplant recipients with tacrolimus and cyclosporine nephrotoxicity. Kidney Int. (2002) 62:2257–63. doi: 10.1046/j.1523-1755.2002.00668.x
49. Ozdemir BH, Ozdemir FN, Demirhan B, Haberal M. TGF-beta1 expression in renal allograft rejection and cyclosporine A toxicity. Transplantation. (2005) 80:1681–5. doi: 10.1097/01.tp.0000185303.92981.d6
50. Roos-van-Groningen MC, Scholten EM, Lelieveld PM, Rowshani AT, Baelde HJ, Bajema IM, et al. Molecular comparison of calcineurin inhibitor-induced fibrogenic responses in protocol renal transplant biopsies. J Am Soc Nephrol. (2006) 17:881–8. doi: 10.1681/ASN.2005080891
51. Boswell JM, Yui MA, Burt DW, Kelley VE. Increased tumor necrosis factor and IL-1 beta gene expression in the kidneys of mice with lupus nephritis. J Immunol. (1988) 141:3050–4. doi: 10.4049/jimmunol.141.9.3050
52. Timoshanko JR, Kitching AR, Iwakura Y, Holdsworth SR, Tipping PG. Contributions of IL-1beta and IL-1alpha to crescentic glomerulonephritis in mice. J Am Soc Nephrol. (2004) 15:910–8. doi: 10.1097/01.asn.0000115704.86897.f4
53. Bilsborrow JB, Doherty E, Tilstam PV, Bucala R. Macrophage migration inhibitory factor (MIF) as a therapeutic target for rheumatoid arthritis and systemic lupus erythematosus. Expert Opin Ther Targets. (2019) 23:733–44. doi: 10.1080/14728222.2019.1656718
54. Gamez-Nava JI, Diaz-Rizo V, Perez-Guerrero EE, Muñoz-Valle JF, Saldaña-Cruz AM, Fajardo-Robledo NS, et al. Assessment of serum macrophage migration inhibitory factor (MIF), adiponectin, and other adipokines as potential markers of proteinuria and renal dysfunction in lupus nephritis: a cross-sectional study. biomark Res. (2020) 8:55. doi: 10.1186/s40364-020-00236-x
55. Lee HT, Shiao YM, Wu TH, Chen WS, Hsu YH, Tsai SF, et al. Serum BLC/CXCL13 concentrations and renal expression of CXCL13/CXCR5 in patients with systemic lupus erythematosus and lupus nephritis. J Rheumatol. (2010) 37:45–52. doi: 10.3899/jrheum.090450
56. Arriens C, Teng YKO, Ginzler EM, Parikh SV, Askanase AD, Saxena A, et al. Update on the efficacy and safety profile of voclosporin: an integrated analysis of clinical trials in lupus nephritis. Arthritis Care Res. (2022) 75(7):1399–408. doi: 10.1002/acr.25007
57. Kamel KS, Ethier JH, Quaggin S, Levin A, Albert S, Carlisle EJ, et al. Studies to determine the basis for hyperkalemia in recipients of a renal transplant who are treated with cyclosporine. J Am Soc Nephrol. (1992) 2(8):1279–84. doi: 10.1681/ASN.V281279
58. Nawaz SH, Zafar MN, Naqvi SA, Rizvi SA. Impact of cyclosporin immunosuppression on serum magnesium and its fractional excretion in renal transplant recipients. J Pak Med Assoc. (2005) 55(3):98–100.
59. Mazzola BL, Vannini SD, Truttmann AC, von Vigier RO, Wermuth B, Ferrari P, et al. Long-term calcineurin inhibition and magnesium balance after renal transplantation. Transpl Int. (2003) 16(2):76–81. doi: 10.1007/s00147-002-0479-9
Keywords: lupus nephritis, voclosporin, biomarker, nephrotoxicity, calcineurin inhibitor
Citation: Palmer BF, Tumlin JA, Radhakrishnan J, Rehaume LM, Cross JL and Huizinga RB (2025) The kidney injury biomarker profile of patients with lupus nephritis remains unchanged with the second-generation calcineurin inhibitor voclosporin. Front. Nephrol. 5:1540471. doi: 10.3389/fneph.2025.1540471
Received: 05 December 2024; Accepted: 10 February 2025;
Published: 17 March 2025.
Edited by:
Manuel Alfredo Podestà, Harvard Medical School, United StatesReviewed by:
Gianmarco Sabiu, University of Milan, ItalyCopyright © 2025 Palmer, Tumlin, Radhakrishnan, Rehaume, Cross and Huizinga. This is an open-access article distributed under the terms of the Creative Commons Attribution License (CC BY). The use, distribution or reproduction in other forums is permitted, provided the original author(s) and the copyright owner(s) are credited and that the original publication in this journal is cited, in accordance with accepted academic practice. No use, distribution or reproduction is permitted which does not comply with these terms.
*Correspondence: Biff F. Palmer, YmlmZi5wYWxtZXJAdHR1aHNjLmVkdQ==
†Present addresses: Biff F. Palmer, Departments of Internal Medicine and Medical Education, Texas Tech University Health Science Center, El Paso, TX, United States
Robert B. Huizinga, Reformation Consulting Services, North Saanich, BC, Canada
Disclaimer: All claims expressed in this article are solely those of the authors and do not necessarily represent those of their affiliated organizations, or those of the publisher, the editors and the reviewers. Any product that may be evaluated in this article or claim that may be made by its manufacturer is not guaranteed or endorsed by the publisher.
Research integrity at Frontiers
Learn more about the work of our research integrity team to safeguard the quality of each article we publish.