- 1Division of Cardiovascular Medicine, The University of Tennessee Health Science Center, Memphis, TN, United States
- 2Department of Internal Medicine, Baton Rouge General Medical Center, Baton Rouge, LA, United States
- 3Department of Internal Medicine, University of Texas Rio Grande Valley, McAllen, TX, United States
- 4Department of Cardiology, Memphis Veterans Affairs (VA) Medical Center, Memphis, TN, United States
- 5Nephrology Division, Renal Associates of Baton Rouge, Baton Rouge, LA, United States
Dialysis patients experience 10–20 times higher cardiovascular mortality than the general population. The high burden of both conventional and nontraditional risk factors attributable to loss of renal function can explain higher rates of cardiovascular disease (CVD) morbidity and death among dialysis patients. As renal function declines, uremic toxins accumulate in the blood and disrupt cell function, causing cardiovascular damage. Hemodialysis patients have many cardiovascular complications, including sudden cardiac death. Peritoneal dialysis puts dialysis patients with end-stage renal disease at increased risk of CVD complications and emergency hospitalization. The current standard of care in this population is based on observational data, which has a high potential for bias due to the paucity of dedicated randomized clinical trials. Furthermore, guidelines lack specific guidelines for these patients, often inferring them from non-dialysis patient trials. A crucial step in the prevention and treatment of CVD would be to gain better knowledge of the influence of these predisposing risk factors. This review highlights the current evidence regarding the influence of advanced chronic disease on the cardiovascular system in patients undergoing renal dialysis.
1 Introduction
Over 450,000 individuals are currently on maintenance dialysis in the United States (1). Despite recent advances in the dialysis process, these patients continue to experience high hospitalization rates, poor quality of life, and increased annual mortality above 20% of the general population. Chronic kidney disease (CKD) impacts the cardiovascular system tremendously and is regarded as an independent risk factor (1, 2). Survival rates for patients on dialysis are poor, at 40%, regardless of the mode of dialysis (3, 4). Cardiovascular disease mortality accounts for a significant proportion of all-cause mortality in this population (3, 4). A growing body of evidence endorses that CKD patients are more likely to die from cardiovascular disease-related adverse events than from events related to kidney failure (5, 6). It is estimated that around 80% of hemodialysis patients suffer from at least one form of cardiovascular disease at the time of dialysis initiation, stemming from preexisting cardiovascular risk factors in these patients (7–10). Among these conditions are left ventricular hypertrophy (29%–75%), congestive heart failure (20%–40%), coronary artery disease (22%–39%), atrial fibrillation (11%–27%) and valvular heart disease (24%) (10–17). Traditional and nontraditional risk factors, not limited to hypertension and hyperglycemia, altered bone mineral metabolism, endothelial dysfunction, volume overload, and uremic toxins contribute to the development of CVD in this population (18–22).
Creatinine-based estimated glomerular filtration rate and albuminuria are independent predictors of poor cardiovascular disease outcomes (23). In fact, microalbuminuria in the absence of overt renal dysfunction confers an increased risk of cardiovascular disease by two to fourfold (24). Go et al (25), in one of the most powered studies, evaluated the impact of kidney dysfunction on cardiovascular events, mortality, and hospitalization in an adult population of >1.1 million in northern California. Baseline GFR was estimated from the abbreviated Modification of Diet in Renal Disease (MDRD) equation (26). The authors used the modified National Kidney Foundation classification (27) of chronic kidney disease to longitudinally stratify eGFR among the study subjects. The mean follow-up period was 2.84 years. After adjusting for comorbidities, age, sex, ethnicity and socioeconomic status, the risk of cardiovascular events, mortality and hospitalization increased as the eGFR decreased below 60 ml per minute per 1.73 m2 of body-surface area (25). An important limitation to this study, which is not uncommon, is the exclusion of patients on dialysis and renal transplant recipients. This reiterates the current challenges in managing cardiovascular disease in dialysis and transplant recipients as several landmark and clinical practice changing investigations often exclude these patients. Furthermore, there is heterogeneity in the inherent predisposing factors, distribution, and types of cardiovascular disease manifestations in individual CKD patients. Therefore, the extent to which these traditional interventions can deliver clinical benefits in this population remains uncertain.
Studies comparing cardiovascular outcomes among patients on HD and those on peritoneal dialysis report higher mortality association with PD. The contributing mechanisms are unclear but proposed hypotheses include unpredictable and inadequate fluid removal and higher atherogenic lipid profile leading to higher risk CVD in PD (28–30). A comparison of 3468 HD patients with 933 PD patients in an observational study revealed that PD significantly increased the risk of all-cause mortality including, cardiovascular mortality.
The first part of this review focuses on contemporary evidence regarding the impact of advanced kidney disease on the cardiovascular system in patients undergoing renal dialysis, the predisposing traditional and nontraditional risk factors. The second part reviews the pathophysiologic drivers of several CV diseases and current screening tools and biomarkers.
2 Pathophysiological mechanisms of specific cardiovascular diseases in dialysis
2.1 Left ventricular hypertrophy
Left ventricular hypertrophy (LVH) in dialysis patients is a complex physiologic adaptive response to long-term increase in volume or pressure load (31). It is not an uncommon complication among pre-dialysis patients with CKD (32, 33). LVH is prevalent in up to 75% of CKD patients at initiation of dialysis (34). Concentric (from LV pressure overload) and eccentric (from volume overload) patterns have been reported in literature (11). Prominent predisposing factors comprise of anemia, hypertension, dialysis vintage, volume overload, interdialytic weight gain and bone-mineral metabolism (35–38). Anemia is an established reversible risk factor for LVH among patients on dialysis (37, 39, 40). Anemia in CKD is primarily due to insufficient endogenous erythropoietin production. Furthermore, iron deficiency develops from over consumption associated with accelerated erythropoiesis following exogenous EPO, gastrointestinal bleeding (from uremia and arteriovenous malformations) and unavoidable blood loss associated with HD access cannulation (32). There is a significant link between left ventricular mass (LV) and volume with the degree and duration of anemia in patients with end stage renal disease (ESRD) (31). A decrease in hemoglobin concentration as low as 1 g/dL has been linked to a heightened risk of LV dilatation, systolic dysfunction, heart failure, and mortality (37, 41–43). The interplay of hemodynamic and non-hemodynamic mechanism compensates for anemia in patients with ESRD (31). Enhanced erythropoiesis from increased endogenous erythropoietin production and increased tissue oxygen extraction mediated by 2,3-diphosphoglycerate concentrations are the main non-hemodynamic compensatory mechanisms in anemia (31). Hemodynamic compensatory mechanisms are mediated by increase in preload (via venoconstriction), positive inotropy and chronotropy (via sympathetic mediators) and reduced afterload, to maintain appropriate cardiac output (31). Vasodilatory mechanisms and reduced vascular resistance resulting from tissue hypoxia, increased nitric oxide activity and lower blood viscosity are instrumental to maintaining reduced after load. Increased venous return increases the preload and left ventricular filling, in turn increasing the left ventricular end diastolic and stroke volumes. Enhanced contractility is attributable to increased concentrations of catecholamine and other inotropic factors. Chronic hemoglobin concentration less than 10-11 g/dL has been implicated as the clinical threshold for anemia induced LVH (35). In non-exertional states and hemoglobin concentration >10 g/dL, the non-hemodynamic mechanisms alone sufficiently compensate for anemia without engaging hemodynamic factors (44, 45). In contrast, hemodynamic mechanisms become necessary when hemoglobin concentration falls below <10 g/dL or with exertion (44, 45). As anemia progresses in chronicity, the maladaptive phase ensues as these mechanisms become ultimately overwhelmed resulting in often irreversible cardiomyopathy and left ventricular hypertrophy (46). Additionally, in ESRD patients, sustained increase in cardiac output induces arterial remodeling (intima-media thickening) in the large vessels (aorta and carotids) and interstitial fibrosis contributing further to the maladaptive phase (31, 47, 48).
Hypertension is a known risk factor for LVH in non-dialysis patients (34, 36, 49). It is not unlikely that the aging ventricle is relatively sensitive to hypertrophic effect of systolic blood pressures (36). Increased afterload stemming from increase in peripheral resistance and impedance causes myocardial remodeling, thickening of myofibers and then concentric hypertrophy. This pathological form of LV remodeling is often in concert with interstitial fibrosis (50). A prospective study enrolled fifty-one ESRD patients with no evidence of LVH on baseline echocardiogram at the initiation of dialysis. Mean follow up duration was twenty-nine months. Cases had remarkably higher systolic blood pressure than controls (149.6 [SD, 13.4] mm Hg versus 137.1 [SD, 15.7] mm Hg; P = 0.0091). There was a positive correlation (r = 0.39; P 0.01) between systolic blood pressure and final left ventricular wall thickness (36). Overall, age and systolic blood pressure were independent predictors of development of LVH (36). A study showed a correlation between pre-dialysis systolic blood pressure between LV mass in hemodialysis patients. The authors propose that controlling pre-dialysis BP alongside post dialysis systolic BP is more impactful than maintaining post dialysis SBP in the normal range. This study enrolled very few subjects therefore these claims warrant higher powered studies to confirm its merits.
High levels of phosphorus are independently linked to increased LV mass in dialysis patients (51). The mechanism is still unclear, but studies report an association with vascular calcification as well as arterial stiffness leading to pressure overload on the left ventricle. Therefore, therapeutic interventions to lower phosphorus levels could improve cardiovascular outcomes (51). A multinational blinded randomized controlled trial demonstrated an association between dialysis vintage and left ventricular volume increase even after adjusting for age and gender (35). At baseline, patients with lower dialysis vintage had lower left ventricular volume increase (35).
FGF23 is markedly elevated in dialysis due to the feedback signal of intractable hyperphosphatemia and klotho insufficiency (52). Several studies support its association with LVH in uremic patients. There are theoretical pathogenic pathways for this relationship (52–55). In uremic patients, FGF23 is activated by the renin-angiotensin-aldosterone system which promotes pro-fibrotic interaction between cardiac myocytes and fibroblasts (56). Several other risk factors known to contribute to the pathogenesis of LVH in non-dialysis patients including diabetes have been explored in dialysis patients but was found to be noncontributory. This may be due to co-existence of other factors with more prominent influence such as hypertension, anemia, and volume overload in CKD patients (51).
2.2 Heart failure
Heart failure is a syndrome that comprises structural or functional cardiac dysfunction manifesting as failure of the heart to pump blood and maintain physiological circulation (51). HD patients are at risk of either diastolic or systolic dysfunction or both (51). The development of heart failure is mainly due to cardiomyopathy and ischemic disease. About 36% of ESRD patients requiring dialysis already exhibit clinical signs of HF at initiation of dialysis (57). CHF and chronic kidney disease share several overlapping risk factors and pathomechanisms, including renin–angiotensin–aldosterone system activation. ESRD patients with HF have poor survival facing as high as 25-35% mortality risk (11, 58–60). Hemodialysis is a well-documented independent cardiovascular stressor that can precipitate repetitive HD-induced myocardial stunning, resulting in LV dysfunction, myocardial hibernation, as well as fibrosis, resulting in heart failure (61). Data from the U.S Renal Data System suggests that HD in itself is an independent risk factor for development of de novo heart failure and once diagnosed, the two-year mortality is as high as 51%. Left ventricular hypertrophy is an important precursor of HF in HD patients. It results in an abnormal adaptive myocardial remodeling which is usually progressive and irreversible given that the precipitating factors are persistent in ESRD (62, 63).
The creation of arteriovenous access for HD has cardiovascular implications because of hemodynamic changes. An increase in hemodialysis AV access flow rate as well as right ventricular dilation and left atrial dilation are all risk factors associated with hemodialysis AV access induced heart failure, as are being male, having prior vascular access surgery, and having prior vascular access surgery (64). The risk of AV access induced HF appears unrelated to access type. Dialysis patients with upper arm fistula are at relatively higher risk of HF (due to increased blood flow) compared to those with forearm fistulas (64, 65). There is a temporal pattern to the pathophysiologic changes that occur in AV access induced HF (66, 67). Within days following creation of AV access, there is acute decrease in systemic vascular resistance with an increase in cardiac output through increase in stroke volume and heart rate. Peripheral vascular resistance also drops due to the access resistance phenomenon (68). As blood flow and shear stress increase, in response, the vascular endothelial cells release vasodilatory factors including nitric oxide to normalize shear stress (68). The drop in systemic vascular resistance is accompanied by a fall in systemic blood pressure. It is then the response of the sympathetic nervous system to increase stroke volume and contractility that helps maintain cardiac output in the immediate post access period (68). Persistent increase in cardiac output enhances venous to the right side of the heart which leads to right ventricular dilatation and failure (64). In the weeks to months following AV access placement, there is worsening of right ventricular dilation and dysfunction, left atrial dilatation, left ventricular hypertrophy (LVH) and myocardial remodeling (69–71). Oftentimes, left ventricular hypertrophy continues to worsen despite appropriate management of fluid and hypertension (70).
2.3 Coronary artery disease
Ischemic heart disease is common among patients with ESRD at the initiation of dialysis and these patients have a worse survival rate when compared to those without heart disease (72). This is understandably so as many of the factors that predispose to ischemic heart disease are already in place prior to initiating dialysis therapy (72). The estimated prevalence at the time of dialysis ranges from 15 to 73% in literature (34, 73, 74). In these patients, significant stenotic lesions of at least 75% narrowing of the reference segment abound, with majority (73%) presenting with multivessel disease and calcification. In asymptomatic patients the prevalence is as high as 53.8% (74). The likelihood of having CAD rises proportionally as the glomerular filtration rate (GFR) decreases (75). Acute myocardial infarction (AMI) is more severe and has a poorer short- and long-term prognosis in the presence of uremia (76). The proposed mechanisms of CAD include accelerated atherosclerosis by dialysis therapy and the presence of CAD risk factors prior to dialysis. Supporting this is the finding that incidence of coronary artery events among these patients does not increase in the first year, but mortality shows an upward trend (77, 78). A history of CAD adds crucial predictive information to established risk factors for deteriorating renal impairment in diabetic nephropathy (79).
There appears to be a link between coronary vascular calcification in the pathophysiology of CAD in renal disease patients (80). ESRD patients show calcific atherosclerotic disease in select vasculatures such as the coronary arteries, aorta, abdominal and lower extremity arteries (81). The pattern of calcification can be analyzed by plain radiographs showing distinct characteristics (82, 83). Here, intimal atherosclerosis and calcification exhibit a patchy distribution while medial calcification follows are pipeline-like pattern (83). Renal dialysis patients show both patterns according to studies, developing earlier relative to non ESRD patients who mainly exhibit intimal calcification pattern (80, 84–86). No existing noninvasive imaging technique can provide exact localization of intimal or medial calcification in living patients. Microscopic analysis of vascular samples from surgery or postmortem samples provides insights into this delineation. A postmortem study reviewed the relationship between the stage of kidney dysfunction and the prevalence of coronary artery calcification (80). Results showed that while intimal calcification was higher among ESRD patients, medial calcification was exclusive to this group. Patients on hemodialysis had more pronounced intimal plaque calcification attributable to uremic risk factors. Researchers noted a graded relationship between coronary artery calcium and CKD severity, even after controlling for traditional coronary artery disease risk factors in the Chronic Renal Insufficiency Cohort (CRIC) study (87). Garland et al (88) investigated the impact of coronary artery calcium in CAD development in 125 CKD patients for decline in renal function and discovered that coronary artery calcium score of 100 to 399 has 7.4 increased odds of decline in renal function, and a score >400 correlate with 8.8 increased odds of decline in kidney function at 1 year of follow up. There is a difference in clinical significance between intimal and medial calcification (89). Whereas intimal calcification tends towards plaque fragility, medial calcification seems to lead to vascular stiffness. Possessing predominantly intimal calcification confers a relatively higher risk of mortality than medial calcification (83). Parfrey et al (72) investigated the outcomes and risk factors for ischemic heart disease among a cohort of 432 consecutive dialysis patients. Patients had an echocardiogram at baseline to investigate for the presence of cardiomyopathies and left ventricular hypertrophy. The authors found that ischemic heart disease is common among dialysis patients. De novo ischemic heart disease was independently precipitated by risk factors including older age, hyperglycemia, hypertension, hypoalbuminemia, and underlying cardiomyopathy detected on baseline echocardiography and that mortality was due to subsequent development of congestive of heart failure. In those with de novo ischemic heart disease while on dialysis, shorter time to event (24 months vs 55 months in non-dialysis patients) (72) has been observed.
Hyperhomocysteinemia has been reported as a powerful risk factor for atherosclerosis through the induction of oxidative stress and arterial endothelial damage (90–92). Impaired homocysteine (hcy) metabolism and extremely elevated homocysteine levels are frequently detected in individuals with ESRD (93, 94). There is an inverse relationship between rising hcy levels and decline in renal function. The fundamental etiology of hyperhomocysteinemia in renal impairment is not completely understood but seems to be related to impaired elimination by dysfunctional kidneys. Studies have shown a positive association between high plasma homocysteine levels and vascular intimal-medial wall thickness via promoting smooth muscles cells proliferation and inhibition of endothelial cell growth (95–97).
2.4 Valvular heart disease
CKD patients have higher odds of left sided, aortic stenosis (AS) and mitral regurgitation (MR), not related to age or comorbidities (98). Both classic and uremia-related risk factors contribute to the elevated frequency of vascular/valvular heart disease in renal dialysis disease patients (Figure 1). Advanced age and dialysis vintage are principal factors associated with valvopathy. Pathological dystrophic calcification from deranged calcium-phosphate metabolism in renal dialysis involves the cardiac skeleton and valve leaflets (99, 100). Hyperphosphatemia above 6.5 mg/dL has been linked to increased mortality in ESRD patients from cardiovascular complications (101). Inflammation is thought to be a potent mediator of valvopathy. There is evidence of macrophage and T- lymphocyte deposition in diseased cardiac valves (102, 103). The pathogenesis in mitral valvopathy is via morphological destruction of the mitral valve apparatus including the leaflets, chordae, and limitation in annular motion (99, 100). A retrospective multicenter study investigating the etiological factors associated with valvular heart disease included 98 patients on chronic renal dialysis reported an annual incidence of 15-19 cases per 10,000 dialysis patients (104). The most common etiologies were endocarditis (19%) and calcific valvular heart disease (69%). Calcific valvopathy was more associated with aortic stenosis and relatively lower prevalence (9%) of multivalvular disease (>1 valvular lesion). Endocarditis induced valvopathy showed a predilection towards mitral valve involvement with more than 1 valvular lesion (32%). Aortic stenosis progresses rapidly in ESRD patients resulting in short term irreversible heart failure (104). There is an association between adynamic bone disease and valvular calcification. This is thought to be related to calcitriol and calcium containing phosphate binders (105). Valvular sclerosis predisposes to rapidly progressive valvopathy that involves the chordae tendineae, valve leaflets and cusps (106, 107). Gross inspection of the chordae tendineae reveals an uneven thickening and shortening with the histological appearance of “concentric rings of loose connective tissue surrounding a central dense core.” (107) Regurgitant and stenotic lesions are the most prevalent clinically significant valvular abnormalities identified in dialysis patients. Aortic stenosis is the most prevalent stenotic valvopathy seen in hemodialysis patients with mitral stenosis being the second most common lesion. Tricuspid and pulmonic stenosis are uncommon (108). As aforementioned, stenotic valvopathy are typically associated with annular calcification in the setting of hyperparathyroidism and an increased serum calcium-phosphorus metabolite (108). In non-dialysis patients, bicuspid aortic valve and rheumatic fever are independent risk factors in the accelerated development of aortic stenosis. However, in renal dialysis patients this senescence appears even earlier and accelerated due to uremia (108). Likewise, advanced age is an independent contributor in the development of aortic stenosis in this population (108). Histological examination of stenotic aortic valves exhibits many osteoblastic markers, including osteopontin, osteocalcin, LRP5, osteoprotegerin/RANKL/RANK, BMP-2, and Cbfa1/Runx2 (109–112). In AS, the ratio of RANKL/RANK to osteoprotegerin is skewed against osteoprotegerin, hence favoring calcifying processes (113, 114).
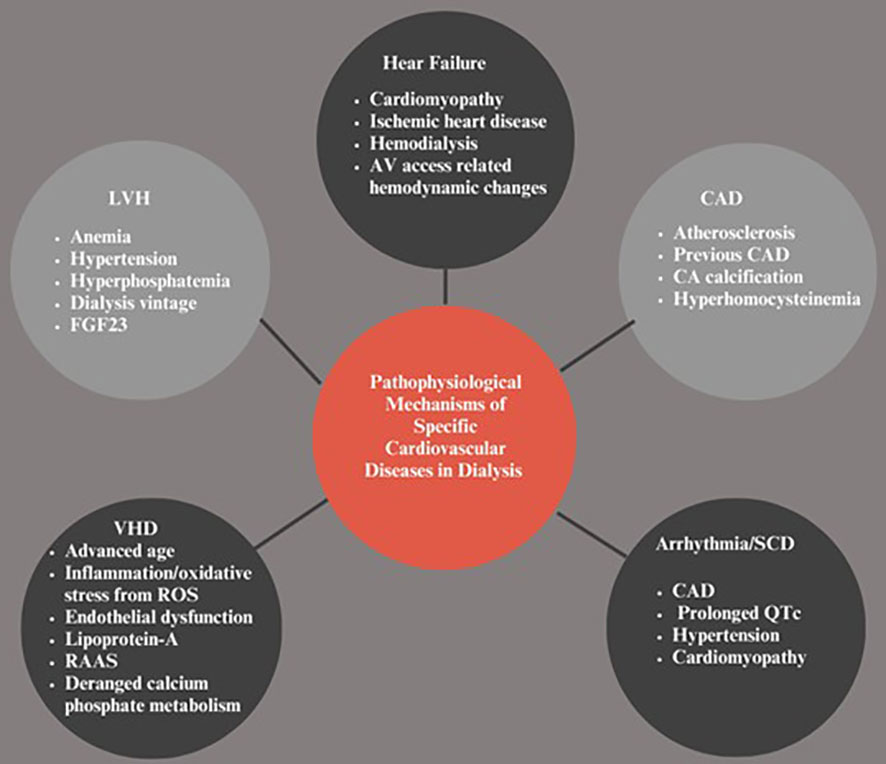
Figure 1 Pathophysiologic.al Mechanisms of Specific Cardiovascular Diseases in Dialysis. LVH, Left ventricular hypertrophy; VHD, Vascular heart disease; SCD, Sudden cardiac death; CAD, Coronary artery disease; CA, Coronary artery; FGF-23, Fibroblast growth factor-23; RAAS, Renin angiotensin-aldosterone system; ROS, Reactive oxygen species.
Endothelial dysfunction is a known contributor to development valvopathy in ESRD. Earlier in stenotic disease, there is subendothelial thickening and alteration of the basement membrane in areas of low shear stress of the valvular leaflets. The shear stress-related endothelial damage inflicted by recurrent turbulent blood flow across the valve is prevalent and pervasive in dialysis patients. In fact, fluid overload, anemia, and shunts across arteriovenous fistulae generate a state of excessive cardiac output, which results in increased flow velocity and turbulence across the valve culminating in valvopathy (102). Lipoprotein-A has been implicated in the pathogenesis of valvopathy in renal dialysis through endothelial barrier disruption, lipid deposition in the leaflets which in turn trigger local inflammation and cellular differentiation processes (115). Furthermore, angiotensin converting enzyme is found in relation to stenotic aortic valves alongside angiotensin II and apolipoprotein B. This highlights the impact of the renin angiotensin aldosterone (RAAS) system in valvopathy via extracellular matrix remodeling and fibrosis processes (116, 117). Imbalance between reactive oxygen species (superoxide and hydrogen peroxide) which are markedly elevated in calcified regions of affected vessels and low activity of the antioxidant mechanisms (superoxide dismutase enzymes) predispose to aortic valvopathy (110).
2.5 Arrhythmias/sudden cardiac death
Most recent epidemiological reports indicate that CVD remains the leading cause of death in CKD with arrhythmias and cardiac arrest accounting for 41.4% of all fatalities with an established cause of death (1). Lethal cardiac arrhythmia requires 3 prominent factors: triggering factors, arrhythmogenic substrates and overactive sympathetic nervous system. Prolonged QTc, hypertension and coronary artery disease are important factors in cardiac arrhythmia. According to DeLima et al. (118) hypertension induces arrhythmia through mechanical stress and inducing ischemia particularly in the context of LVH or myocardial fibrosis. In LVH, myocardial fibrotic bands prevent impulse propagation by precipitating re-entrant ventricular tachyarrhythmia (55). Prolonged QT is prevalent in dialysis patients and is thought to be due to autonomic neuropathy, cardiac myocyte hypertrophy, myocardial calcification, fibrosis and increased ventricular wall tension (119–121). Re-entry phenomenon underlies most life-threatening ventricular arrhythmias (122, 123). Altered and slow conductions are needed to propagate reentrant circuit. The aberrant regions of ventricular myocardium with delayed activation are thought to be the source of the late potential, providing the substrate for re-entry (122, 123).
Fast ventricular rate in the setting of atrial tachyarrhythmia has been hypothesized to decrease ventricular refractoriness, hence increasing susceptibility to ventricular arrhythmias (124). Moreover, atrial fibrillation (AF) can cause short-long-short sequences in the ventricular cycle length, which in turn promotes certain forms of ventricular arrhythmias (125). Overactivation of the sympathetic nervous system has both short-term and long-term proarrhythmogenic effects and contributes to the pathophysiology of arrhythmic fatalities in CKD patients. A potent mediator of sympathetic hyperstimulation is high levels of angiotensin 2 via the renin-angiotensin activation system (126, 127). Released norepinephrine stimulates the afferent renal nerves and chemoreceptors via adenosine release potentiated by MicroRNA -92 upregulation (128). Sympathetic activation influences cellular channel function, increasing repolarization heterogeneity, and contributes to cardiac fibrosis via activating -adrenergic receptors and generating inflammatory responses (129, 130). The modulation of the baroreceptor reflex has also been demonstrated to be altered in dialysis, as well as its relation to vascular calcification and arterial stiffness suggesting a potential anatomical connection between vascular alterations and baroreceptor function (131).
3 Roles of traditional and non-traditional risk factors in the pathogenesis of cardiovascular in end stage renal disease
Cardiovascular risk assessment in dialysis patients optimizes therapeutic measures and reduces cardiovascular morbidity and death. Dialysis patients share most of the risk factors (including hypertension, diabetes mellitus, cigarette smoking, dyslipidemia, obesity) for cardiovascular morbidity and mortality in the general population (Figure 2). Traditional risk variables do not adequately account for the significant cardiovascular risk associated with CKD. There have been several studies exploring the roles of non-traditional risk factors such as microinflammation and vascular dysfunction, oxidative stress, uremic toxins, advanced glycation end products and volume overload. An interplay of these factors leads to the development of cardiovascular disease morbidity and mortality in this population.
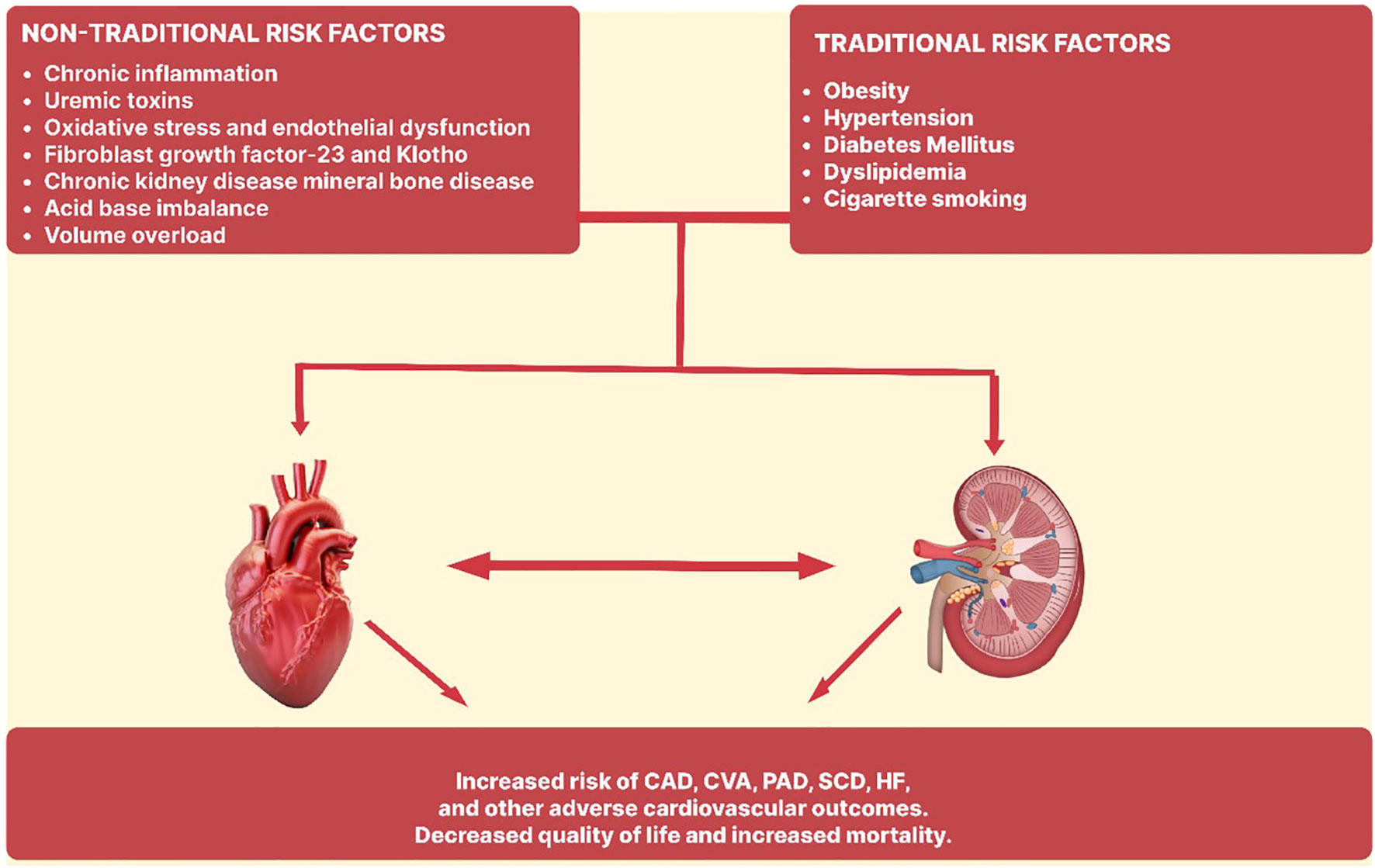
Figure 2 Traditional and non-traditional risk factors in the pathogenesis of cardiovascular disease in end stage renal disease. CAD, Coronary artery disease; CVA, Cerebrovascular accident; PAD, Peripheral artery disease; SCD, Sudden cardiac death; HF, Heart failure.
4 Traditional risk factors
4.1 Diabetes mellitus
As the prevalence of diabetes mellitus (DM) rises worldwide, so does the number of individuals requiring renal replacement treatment due to complications from the disease (62, 132–134). Diabetes increases the risk of cardiovascular disease, notably acute myocardial infarction, and sudden cardiac death (135, 136). Diabetic ESRD patients have a worse 5-year adjusted survival than those with hypertension or glomerulonephritis (137). Uncontrolled DM (HbA1c > 10%) in dialysis patients increases all-cause and cardiac mortality, as well as worse 5-year survival compared to those with better control [HbA1c < 7.5%] (138–140). A target HbA1c of 7% is reasonable for glycemic control in ESRD patients with the goal of avoiding hypoglycemia. A U-shaped association between HbA1c and mortality has been demonstrated, with increased mortality in both high and low HbA1c levels (141, 142). It is important to note that there are some limitations to the use of HbA1c as an indicator of glycemic control in ESRD patients. Protein-energy malnutrition shortened red blood cell life span, anemia and the use of erythropoiesis stimulating agents have been shown to falsely reduce HbA1c. On the other hand, elevated blood urea nitrogen and metabolic acidosis are associated with falsely high HbA1c levels. Other suggested markers of glycemic control in patients with ESRD asides from HbA1c include glycated albumin and fructosamine (143).
4.2 Hypertension
Hypertension is a well-known risk factor for CKD. Studies do not often agree on what constitutes an adequate criterion for identifying hypertension, hence the actual prevalence may never be known with any degree of precision. In a multi-center trial of over 2000 HD patients in which hypertension was defined as the use of antihypertensive medications or weekly average pre-dialysis systolic blood pressure > 150/85 mmHg, the prevalence of hypertension was 86% (144). Similarly, a prevalence of 82% was reported in another HD cohort with the definition of hypertension being the prescription of any antihypertensive medication or inter-dialytic ambulatory blood pressure ≥ 135/85 mmHg (145). Defining blood pressure at ≥140/90 mmHg among patients on peritoneal dialysis, the prevalence of hypertension was found to be between 69 to 88% (146). Due to variations in pre-dialysis and post-dialysis BP readings, ambulatory blood pressure monitoring [ABPM] is the most reliable method to diagnose hypertension in dialysis patients and has the added advantage of being reproducible and able to provide information on circadian variation (147, 148). In comparison to dialysis unit recordings, home BP recordings, including self-measured readings and ABPM and self-measured readings are greater predictors of cardiovascular and all-cause mortality. Therapy with anti-hypertensive medications is associated with a reduction in cardiovascular morbidity and mortality in ESRD patients. A meta-analysis demonstrated that BP lowering with medications reduced the risk of cardiovascular events as well as cardiovascular and all-cause mortality in dialysis patients (149). Similarly, several studies have shown a reduction in cardiovascular morbidity; cardiovascular and all-cause mortality with the use of angiotensin receptor blockers for BP control in ESRD patients (150, 151). Despite these proven benefits, the optimal target BP has not been determined (152).
4.3 Obesity
In the general population, obesity is associated with increased cardiovascular and all-cause mortality; however, a paradoxically inverse association has been observed between body mass index and mortality in hemodialysis patients (153–158). This obesity paradox does not necessarily imply that the mechanisms contributing to obesity associated cardiovascular outcomes in ESRD patients is entirely different from that of the general population, but that this pathway could be obscured by other dominating factors in ESRD (159). Several explanations for the obesity paradox include inflammation and protein calorie wasting, time disparities among competing factors among others (159). A chronic inflammatory state induced by chronic kidney disease leads to proteolysis and appetite suppression in dialysis patients due to the release of cytokines such as interleukin-6 and tumor necrosis factor alpha (160, 161). It is thought that obesity may provide a ‘functional reserve’, potentially diminishing the effect of inflammation and protein energy wasting in patients with a higher body mass index (BMI). Another view is that the competing short-term effects of inflammation and protein-energy wasting (PEW) may outweigh the conventional long-term deleterious effects of obesity in dialysis patients who already have a high mortality (159). ESRD This paradoxical association is less evident in PD patients compared to HD patients. An observational study of PD patients reported lower cardiovascular and all-cause mortality in patients with high BMI/high muscle mass compared to those with normal BMI/high muscle mass. It also demonstrated an increased risk of cardiovascular and all-cause mortality in patients with a high BMI and low muscle mass (162).
4.4 Dyslipidemia
Dyslipidemia is an important risk factor for cardiovascular mortality in the general population with risk scores designed based on lipid levels and other risk factors to determine the need for initiation of interventions to reduce cardiovascular risk. In ESRD patients, dyslipidemia is majorly characterized by normal to low total cholesterol and low-density lipoprotein, high triglycerides, and low high-density lipoprotein (163). The relationship between cardiovascular risk and serum cholesterol levels in patients on dialysis is complex. An inverse relationship has been reported in observational studies between total cholesterol and survival in dialysis patients (164–166). However, when corrected for C-reactive protein, Interleukin-6 and serum albumin, the association between cholesterol and mortality reflects that seen in the general population; suggesting that the inverse relationship reported is probably confounded by chronic inflammation and malnutrition (167). In ESRD patients, it appears that the role of cholesterol in atherosclerosis differs; and a five-fold increase in the prevalence of vascular calcification as well as greater deposition of inflammatory cytokines and more intense intra-plaque hemorrhage have been reported in coronary artery studies (163). In a study comparing the effect of lipid lowering therapy in CKD and non-CKD patients with coronary artery disease, the carotid artery media/intima thickness reduced significantly with atorvastatin therapy during the 2-year observation period in the non-CKD group while this change was not observed in the CKD cohort and it was suggested that the beneficial effect of statins is likely negated by the uremic milieu (168). There is an accumulation of markedly atherogenic lipoproteins including lipoprotein A, oxidized low density lipoprotein (LDL), intermediate-density lipoproteins, small dense LDL and very low-density lipoprotein (VLDL) due to the deficiency of hepatic lipase, lipoprotein lipase and LDL receptor-related protein in ESRD patients (169). This atherogenic lipid profile has been reported to be more prominent in the PD population compared to patients on hemodialysis. In the 2021 European Society of Cardiology (ESC) guidelines for dyslipidemia, severe CKD, with estimated glomerular filtration rate (eGFR) of 30 mL/min/1.73 m2, and moderate CKD, with eGFR of 30–59 mL/min/1.73 m2, are categorized as very high and high cardiovascular (CV) risks, respectively. Similarly, Kidney Disease Improving Global Outcomes (KDIGO) guidelines integrates albuminuria and renal function into CVD risk estimation for CKD patients (170). A validation study in this regard was implemented on a large database with information from 1.8 million patients. The investigators found an increased prevalence of high cardiovascular risk CKD patients (<0.001) in both men and women than was previously determined based on scoring systems excluding these 2 values (171).
4.5 Cigarette smoking
Cigarette smoking is an important cause of preventable death in the United States with ischemic cardiovascular disease contributing a third of these deaths (172). Among dialysis patients, the reported prevalence of smoking is about 15% (173). A systematic review and meta-analysis of smoking and cardiovascular outcomes in dialysis patients showed a significant increase in all-cause mortality among smokers, although no significant increase was seen in cardiovascular events (174). These findings were thought to be due to the fact that smoking may contribute to mortality through non-cardiovascular mechanisms and that the occurrence of cardiovascular events was probably multifactorial in this group of patients and was likely not influenced by smoking alone.
5 Non-traditional risk factors
5.1 Chronic inflammation
Chronic inflammation has been recognized as an important component of CKD and plays a significantly unique role in its pathophysiology as well as the development of protein-energy wasting, cardiovascular and all-cause mortality. Factors contributing to persistent inflammation in CKD include decreased excretion and increased production of pro-inflammatory cytokines, acidic milieu, recurrent and chronic infections, oxidative stress, altered metabolism of adipose tissue, and intestinal dysbiosis. Inflammation worsens with progression of CKD and in dialysis patients, where access-related infections and complications from bioincompatible materials in the dialysis circuit, microbiological quality of the dialysate and impurities in dialysis water play an additional role (175). Several biomarkers of inflammation including IL-6, IL-1β, IL-1 receptor antagonist, CRP, TNF-α, and fibrinogen were directly associated with albuminuria and inversely associated with the measures of kidney function in the Chronic Renal Insufficiency Cohort (CRIC) study (176). However, IL-6 has been found to predict cardiovascular and all-cause mortality better than other cytokines (177, 178). Current research suggests that disorders of arginine metabolism may contribute to the elevated renal and cardiovascular risk in CKD (179, 180). Patients with mild to moderate CKD have been shown to have higher serum asymmetric dimethylarginine (ADMA) and symmetric dimethyarginine (SDMA) levels which was more perverse in those with ESRD (179, 180). Endogenous methylarginines such as ADMA and SDMA as well as other methylarginines such as L-NG monomethylarginine (LNMMA) have been identified as proinflammatory elements. Serum ADMA is elevated in patients with ESRD and has been associated with endothelial dysfunction and atherosclerosis; and predicts mortality in this population. ADMA inhibits nitric oxide (NO) synthase, reducing NO bioavailability and increasing the expression of IL-6 and TNF-α. Furthermore, an increased risk for CKD progression and atherosclerotic CV events has been reported in CKD patients with elevated plasma SDMA (181, 182) It is to be noted that, most of the epidemiological studies have reported conflicting data, included mostly small sized cohorts and were not uniform in their inclusion of the endogenous methylarginines (183–186). A relatively more inclusive study of the five metabolites found that SDMA had the strongest association with cardiovascular outcomes. The full cardiovascular impact of these methylarginines warrant further studies to validate these assertions.
5.2 Uremic toxins
Uremic toxins have been implicated as risk factors for significant cardiovascular mortality and morbidity in the ESRD population (187). The mechanisms by which they negatively impact the cardiovascular system are incompletely understood but are thought to be multifactorial. Uremic toxins cause cardiovascular damage through their effects on leukocytes, endothelial cells, smooth muscle cells and platelets (188). Several other uremic toxins aside from urea and creatinine have been identified in ESRD patients. These have been divided into small molecules, middle molecules and protein-bound molecules based on their physicochemical properties. Small molecules include urea, creatinine, creatine, uric acid, asymmetrical dimethylarginine, symmetrical dimethylarginine among others. They generally have a molecular weight (MW) ≤ 500 Daltons, are water soluble and not bound to proteins. Middle molecules have a MW of >500 Da and are removed only by hemodialysis employing high-flux membranes with the prototype being B2-microglobulin. The protein-bound molecules generally have a low MW, many are toxic and are difficult to remove via HD. These include hippuric acid, para hydroxy hippuric acid, homocysteine, indole-3-acetic acid, indoxyl sulfate, p-Cresol and retinol binding protein (189).
Under the influence of uremic toxins, leukocytes are less responsive to activation and stimulation, resulting in infections, chronic microinflammations, malnutrition, and atherosclerosis. Uremic states lead to macrophage activation via upregulation of TNF-α and stimulation of nuclear factor-kappa B (NF-κB) which in turn drives vascular damage, including aortic calcification. via oxidative stress and high superoxide anion levels (190–192). Furthermore, they cause disruption of the endothelial glycocalyx leading to increased microvascular permeability and systemic vascular dysfunction. They also decrease tissue plasminogen factor and increase plasminogen activator inhibitor-1 and von Willebrand factor (192). Dysregulation of vascular tone, production of reactive oxygen species, inhibition of nitric oxide production and promotion of cellular senescence are reported detrimental effects of uremic toxins. Consequently, these substances increase the proliferation of vascular smooth muscle cells, resulting in thickening and calcification of the aorta (24, 193). Thrombosis is another cardiovascular manifestation of uremia (194). Patients with uremia have been reported to have enhanced caspase-3 activity which mediates exposure of phosphatidylserine on the outer membrane of platelets and these activated platelets are more prone to Thrombosis (194).
p-Cresol tends to accumulate in CKD patients due to reduced excretion and alterations in the gut microbiome. It is the product of bacterial degradation of tyrosine and phenylalanine in the gut and is absorbed from the gut as its main metabolites, p-cresylsulfate (pCS) and p-cresylglucuronide, two protein-bound small molecules. Elevated plasma concentrations of p-cresol are strongly linked to CV risk in CKD (195, 196). Indoxyl sulfate (IS), on the other hand, is an end-product of tryptophan metabolism that is albumin-bound. It accelerates the progression of CKD and stimulates oxidative stress and inflammation in a concentration dependent pattern (197, 198). IS has been implicated in hemostatic dysfunction, vascular damage, stimulation of tissue factor (TF) in vascular smooth muscle cells and an increase in pro-thrombotic risk in a TF-dependent manner specifically after vascular interventions (199–201).
5.3 Oxidative stress and endothelial dysfunction
Oxidative stress (OS) occurs when the antioxidant defense mechanisms of the body are overwhelmed by pro-oxidant molecules, and this has been found to be a significant driver of cardiovascular morbidity in dialysis patients. In CKD, there is increased production of molecules such as nitric oxide (NO) and reactive oxygen species (ROS) as well as deficient antioxidant defense mechanisms and insufficient clearance of oxidative products (202). Antioxidants, which can be endogenous or dietary supplements are lost during dialysis and leukocytes are activated, both of which result in production of ROS. The buildup of NO and ROS stimulates the activation of inflammatory mediators leading to oxidation and modification of nucleic acids, lipids, proteins, and carbohydrates (203).
Oxidative stress is now recognized as a risk factor for inflammation, atherosclerosis, diabetes mellitus, and CKD progression and in ESRD patients and it has been shown that those on hemodialysis have significantly increased OS compared to those on peritoneal dialysis (204, 205). The dialysis modality, biocompatibility of dialyzer membrane and dialysate, dialysis vintage and type of vascular access all play a part in worsening oxidative stress (206).
5.4 Fibroblast growth factor-23, klotho and CVD
Fibroblast growth factor-23 (FGF23) is a protein secreted by osteocytes and osteoclasts that is involved in phosphate metabolism alongside parathyroid hormone via interaction with FGF receptors. FGF23 regulates the excretion of phosphate and requires the presence of coreceptor a-Klotho to act. FGF23 reduces phosphorus levels by inhibiting the sodium-phosphate co-transporter at the proximal and distal tubules, inhibiting the production of 1,25-hydroxyvitamin D and by suppressing parathyroid hormone (PTH) production in the parathyroid glands, probably in a Klotho-dependent manner (207). FGF23 has been found to be elevated compared to PTH and phosphorus in the early stages of CKD [from stage 2] and alongside Klotho, may play a role in the prediction of CVD in ESRD. Some studies have reported an association between elevated plasma FGF23 concentration and an increased risk of CKD progression, cardiovascular complications, and mortality in ESRD. FGF23 plasma levels in coronary patients have also been associated with a higher risk of death and CV, even after adjustment for confounding factors. Soluble Klotho levels have been found to be reduced in patients with CKD and Klotho deficiency and FGF23 elevation are associated with poor outcomes and complications in CKD (208). They have both been recommended as biomarkers for adverse renal and extrarenal outcomes in patients with CKD. Serum Klotho was independently associated with arterial stiffness in a cross-section of patients with CKD and reduction in klotho worsens vascular calcification and uremic cardiomyopathy.
5.5 Chronic kidney disease mineral bone disease and CVD
Chronic kidney disease mineral bone disease (CKD-MBD) plays a profound role in the development of cardiovascular calcifications, which in turn is associated with cardiovascular morbidity and mortality in patients with CKD. The process of vascular calcification is a complex active regulated process resembling bone osteogenesis and is not just a simple deposition of calcium phosphate crystals (209). It involves a transformation of vascular smooth muscle cells into cells resembling bone progenitor cells with an upregulation of osteochondrogenesis resulting in a loss of contractile competence of the vessel as well as the production of a collagen matrix and the formation of calcium–phosphorus-rich vesicles leading to the mineralization process of the vessel internal surface (210). Cardiovascular calcification can increase the incidence of sudden cardiac death, arrhythmias, stroke and mortality in patients with ESRD; It can lead to ischemic CVD and elevated pulse pressure and pulse wave velocity resulting in a reduction of diastolic coronary perfusion with consequent development of left ventricular hypertrophy [LVH] which is a well-known CV risk factor. Aortic stenosis is a well-documented consequence of vascular calcification which results in an increase in afterload, leading to LVH (211). A meta-analysis demonstrated an increased risk of mortality with increasing severity of calcifications and there was a correlation between the number of calcified valves and the risk of mortality. Cardiovascular calcifications increase the risk of both cardiovascular and all-cause mortality; therefore, the detection of valve calcification is essential for risk stratification in ESRD patients. Computed tomography (CT) is the gold standard for the evaluation of vascular calcification, but plain radiographs are an acceptable cheaper alternative with less radiation exposure, and lateral abdominal radiographs are recommended by KDIGO to detect the presence or absence of vascular calcification in CKD Stage 3–5 patients as a reasonable alternative to CT-based imaging (212). Aside from lateral abdominal radiographs, plain radiographs of the chest, pelvis, both hands and feet can also be used to detect calcifications of the aortic arch, iliac and femoral arteries and the small arteries of the hands and feet respectively.
5.6 Acid-base imbalance and CVD
Acid base imbalance: majorly characterized by metabolic acidosis is common in ESRD patients due to a decreased renal production of bicarbonate and a reduction in the ability of the kidneys to excrete non-volatile acids. Metabolic acidosis has been associated with inflammation, malnutrition and increased mortality (213). In a cohort of ESRD patients on both peritoneal and hemodialysis, the adjusted risk for all-cause mortality in most subgroups with serum bicarbonate <22mEq/L was higher irrespective of the modality of dialysis and a time-averaged serum bicarbonate of <19mEq/L was associated with a higher risk of cardiovascular and all-cause mortality in peritoneal dialysis patients (213). In a cross-sectional study of 52 patients on intermittent HD that was designed to determine the association between low serum bicarbonate and cardiovascular disease; metabolic acidosis was found to be associated with diastolic dysfunction and peripheral vascular disease; although after adjusting for covariates, it was not an independent risk factor for diastolic dysfunction. They also did not find a significant association between acidosis and coronary artery disease or systolic dysfunction (214).
5.7 Volume overload and CVD
Volume overload is quite common in dialysis patients and has been found to be an independent predictor of cardiovascular and all-cause mortality (215, 216). Fluid overload was reported to be an independent predictor of increased cardiovascular and all-cause mortality in a cohort of over 300 continuous ambulatory peritoneal dialysis patients (217). Also, in a study of approximately 35,000 incident HD patients across 26 countries, in whom volume status was evaluated using bio-impedance spectroscopy, baseline fluid overload, and cumulative 1-year fluid overload exposure predicted an increased risk of mortality across all BP categories with the highest risk of mortality in those with fluid overload and systolic BP < 130 mmHg at baseline and at 1 year (218). There is a need to maintain the dry weight of dialysis patients with sodium and volume restriction as well as ultrafiltration during dialysis. In the bid to control volume, ultrafiltration rates should generally not exceed 13 ml/kg/h as a retrospective analysis of about 118,000 HD patients found that ultrafiltration rates > 13 ml/kg/h were associated with higher mortality compared with rates ≤ 13 ml/kg/h (219, 220).
5.8 Hyperhomocysteinemia
Homocysteine is a non-essential amino acid that is involved in the metabolism of methionine. In the methionine pathway, the conversion of homocysteine back to methionine is catalyzed by methyltetrahydrofolate and methylcobalamin; hence deficiencies of folate and vitamin B12 can lead to accumulation of excess homocysteine which is referred to as hyperhomocysteinemia. Hyperhomocysteinemia is thought to be implicated in atherothrombosis, vascular calcification and cardiovascular disease (221). Patients on dialysis are at risk of malnutrition as well as micronutrient deficiencies including folate and vitamin B12 and it has been reported that 85–100% of ESRD patients have hyperhomocysteinemia and it is associated with increased cardiovascular morbidity and mortality in this population (220, 222). A meta-analysis of 7 RCTs performed to assess if homocysteine-lowering with folic acid reduced cardiovascular events in patients with ESRD found a significant reduction in risk, with the greatest benefit seen in patients who had a > 20% decrease in homocysteine levels, a longer duration of therapy and no or partial folic acid fortification (223). On the other hand, another systematic review of 6 randomized control trials (RCTs) found that homocysteine-lowering therapy had little or no effect on cardiovascular or all-cause mortality in patients with ESRD (224).
6 Screening for cardiovascular disease in dialysis patients
6.1 LVH/heart failure
De novo and recurrent heart failure affects morbidity and mortality as well as the capacity to provide appropriate dialysis in most patients on dialysis. The KDIGO recommends echocardiographic assessment of all dialysis patients with clinical indications for heart failure or cardiomyopathy like the methods and indications in the general population (225). In fact, there is a grade B recommendation to perform echocardiogram in all patients at the initiation of dialysis following volume and electrolyte optimization, ideally within 1 to 3 months and then at 3-yearly intervals. Much like the general population, dialysis patients with de novo reduced LV systolic function (EF 40%) should be assessed for coronary artery disease. This may include stress testing and/or coronary angiography. However, given the reduced diagnostic accuracy of stress tests in CKD patients, coronary angiography may be preferred in individuals at high risk for CAD, such as those with diabetic CKD. This is true even for people who have had negative stress imaging tests (225). Echocardiography provides information on cardiac chamber volumes, LV mass, systolic and diastolic function. LVH is defined as having an LV mass index of more than 134 g/m2 of body surface area for men and less than 110 g/m2 for women on echo. ECG is a widely utilized initial screening test for LVH determination in the general population. However, traditional criteria for determining LVH has poor sensitivity in the assessment of dialysis patients. A prospective study investigating echocardiographic LV mass index (LVMI) and ECG-LVH criteria in patients with end-stage renal disease (ESRD) to determine the predictive relevance of ECG-LVH criteria noted a Sokolow Lyon voltage duration product and Cornell voltage duration product ECG criteria as the most predictive measures of LVH and cardiovascular mortality in HD patients (226). MRI offers an alternative means of assessing LV mass in dialysis patients. It has been proposed to perform better in this population due to the potential for echocardiogram to overestimate LV mass because of abnormal LV geometry and volume derangement associated with dialysis status (227).
6.2 Coronary heart disease
Dialysis patients are less likely to exhibit the characteristic signs and symptoms of coronary artery disease (CAD) and to be appropriately identified during an acute coronary syndrome (ACS) (76). Among the reasons for this atypical presentation are chronic elevated troponin levels without acute coronary syndrome, deconditioning and limited physical activity optimally triggering anginal symptoms, diabetes preventing localization of pain, and a low percentage of dialysis patients meeting the ST-T wave abnormality pattern criteria for ACS (76, 228–230). Troponin assays, including Troponins I and T are sensitive in diagnosing and predicting acute coronary events. As aforementioned, these enzymes are chronically elevated in ESRD patients due to several cardiac and noncardiac factors, limiting its specificity (231–233). Notwithstanding, KDIGO recommends the use of troponin T in the risk stratification of ACS with careful consideration of clinical context among renal dialysis patients (225).
Resting 12 lead ECG is a noninvasive means of investigating CAD. ESRD patients are likely to have nonspecific ST and T wave abnormalities at baseline making this diagnostic modality difficult to interpret with a sensitivity and specificity of 35% and 64%, respectively (234). A significant proportion of HD patients are physically unfit or have baseline ECG abnormalities precluding the use of exercise stress test. Pharmacologic stress test becomes an alternative. Coronary artery calcium score (CACS) is a noninvasive screening test that requires exposure to low radiation and no contrast. It is utilized in primary prevention strategies for risk stratifying patients requiring atherosclerotic disease interventions (235–238). Its utility is limited among ESRD patients due to diffuse and pervasive coronary artery calcification. In non ESRD patients, coronary artery calcification is only present in advanced atherosclerotic plaques but diffusely affects arterial media calcification reducing its diagnostic accuracy of CACS. CACS >0 has good negative predictive value and could be applied in the exclusion of obstructive CAD (239, 240). Similarly, the utility of coronary computed tomography angiography (CCTA) in diagnosing obstructed CAD is limited due to high calcification burden and artefacts in the absence of obstruction in ESRD patients (235). Myocardial perfusion scintigraphy (MPS) is a noninvasive nuclear imaging test for ischemic heart disease via administration of a radiotracer and pharmacological stress to assess myocardial viability and perfusion (241). A Cochrane meta-analysis comprising nine studies (582 participants) demonstrated that the pooled sensitivity and specificity for angiographic CAD were 0.74 and 0.70, respectively (242). Left ventricular hypertrophy, altered endothelial function in the absence of epicardial stenosis and triple-visible disease impair the reliability of the scan (235). MPS provides essential but imprecise predictive information on Cardiovascular events in ESRD patients not particularly attributable to its capacity to differentiate severe CAD in this cohort (235).
Percutaneous invasive coronary angiography is regarded as the gold standard for diagnosing coronary artery stenosis. It is invasive, costly and requires ionizing radiation and contrast media (235). Therefore, it should only be utilized after careful consideration of the clinical picture, risks and benefits. The integration of physiological parameters such as fractional flow reserve (FFR) to invasive coronary angiography improves diagnostic accuracy of CAD lesion severity (243). Just 2% of the participants of the study providing this evidence had ESRD limiting its application and generalizability in this group. FFR is an indicator of functional significance of stenotic lesions. Microvascular dysfunction, diffuse CAD, vascular calcification which are rife among dialysis patients may result in gross underestimation of the CAD stenosis severity (244, 245). Although the role of FFR in ESRD patients remains ill-defined, combining both anatomic and physiologic measures provide a superior assessment than either alone (235). Overall, invasive coronary angiography should be reserved for high-risk CAD patients presenting with symptoms and/or strongly positive stress test and would benefit from revascularization.
6.3 Valvular heart disease
Echocardiography is the gold standard for the evaluation of valvular anatomy and characterization of degree of valve stenosis and/or regurgitation in ESRD patients according to the updated 2017 kidney disease: Improving Global Outcomes (KDIGO) CKD-mineral bone disease recommendations (246, 247). In assessing valve regurgitation, echocardiography should be done post-dialysis when the patient has their “dry weight” and blood pressure is controlled (247). In addition to screening the heart valves, it is essential to assess the left ventricular morphology considering that a significant proportion of ESRD patients exhibit enlargement, with concentric hypertrophy being the main pattern (248). In CKD patients with severe AS, echocardiographic screening plays a major role in risk stratification and decision making to guide management strategies. There are several clinically relevant echocardiographic parameters such as left ventricular ejection fraction, wall thickness, mass index, estimated filling pressure (E/e’ ratio), left atrial volume index, estimated pulmonary artery systolic pressure (ePASP), and mean transaortic pressure gradient (98, 249). In surveilling patients with established valvular heart disease, it is recommended that patients with mild VHD be re-screened in 12 months interval, moderate VHD in 6-12 months and severe VHD in 3 months (246). The risk of infective endocarditis increases in dialysis patients, and transesophageal echocardiography should be performed if this is suspected (250). Despite advancement in cardiac CT and MRI techniques in the evaluation of several cardiac conditions, their roles in screening VHD especially in CKD patients remain subpar compared to echocardiogram (251). The risk of contrast induced nephropathy makes their use almost prohibitive. Cardiac CT scan quantify valvular and annular calcification and is often performed in CKD patients to evaluate the morphology and size of the aorta and annulus prior to transcatheter valve replacement (246, 250, 252). Furthermore, with cardiac CT, it is possible to determine the degree of AS in both low-flow, low-gradient severe AS with impaired ejection fraction and low-flow, low-gradient severe AS with intact ejection fraction (246, 252). The sensitivity of a chest X-ray for diagnosing VHD in ESRD patients is inadequate (250).
6.4 Arrhythmias
The standard 12 lead electrocardiogram (ECG) is helpful in assessing conduction abnormalities and is often the initial investigation in dialysis patient suspected of cardiac arrythmias. Notably, dialysis related fluid and electrolytes shifts may affect the ECG waveform and likely impair interpretation (253). Tezcan et al. (254) showed a 28% increase in intradialytic P wave duration, which was inversely correlated with potassium and magnesium concentrations at the termination of HD. Berta et al (255). found a correlation between hypokalemia and an increase in QRS length towards the conclusion of HD. Hypokalemia and hypocalcemia have been implicated in intradialytic QTc prolongations. ECGs should be evaluated based on the recording period with reference to HD therapy timing and the patient’s fluid and electrolyte status.
Due to fluid-related alterations in QRS amplitude, post-HD ECGs are likely to indicate left ventricular hypertrophy (253). Variations in QRS duration measurement in relation to HD may affect the evaluation of intraventricular conduction delays which could have management implications. Heart rate variability (HRV) is an indirect noninvasive method for assessing tachograms of continuous ECGs. It analyzes the cardiac autonomic modulation based on the variations in cardiac pacemaker reactivity to autonomic activations (256). Abnormal HRV parameters have been linked to cardiovascular mortality among dialysis patients (257–260). Decreased HRV in CKD patients reflects an aberrant autonomic nervous system response (261). Continuous ECG monitoring via implantable loop recorders may be obtained in dialysis patients vulnerable to substantial conduction abnormalities, including ventricular arrhythmias, or atrial fibrillation or flutter to facilitate early diagnosis and commencement of appropriate management (262). It offers remote monitoring and transmission capabilities. Sudden cardiac death is a major cause of mortality among dialysis patients. Study data from prolonged cardiac rhythm monitoring at the time of death among dialysis patients via implantable loop recorders points to bradycardia and asystole as the most common rhythms degenerating to sudden cardiac death rather than ventricular arrhythmia seen in the general population (262, 263). Echocardiography can assess for structural abnormalities such as valvular dysfunction, left atrial enlargement, and left ventricular hypertrophy, which are not uncommon in dialysis patients and could predispose to arrhythmias (264). Cardiac magnetic resonance imaging is of little prognostic utility, is cumbersome and cost prohibitive relative to various forms of ECG monitoring (264).
7 Use of atherosclerotic cardiovascular disease risk calculator in dialysis patients
Existing data support the use of an atherosclerotic cardiovascular disease (ASCVD) calculator to assess the presence of risk variables for future ASCVD events in asymptomatic adults without CKD (265). Age, sex, race, blood pressure, cholesterol, diabetes mellitus, smoking status, and therapy with antihypertensive drugs, statins, and aspirin are all factors considered by the ASCVD risk calculator. However, the study validation for the use of ASCVD parameters in risk classification did not include dialysis patients. Nonetheless, it is recommended in asymptomatic CKD patients without cardiovascular disease (266).
8 Cardiac biomarkers in dialysis
8.1 Cardiac troponin
Troponin I, and Troponin T are released into the circulation in the event of cardiac myocyte injury and necrosis following ischemia, inflammation, or trauma. High troponin levels are linked to higher mortality in dialysis patients even in those with preserved left ventricular function (267, 268). In non-CKD/ESRD population, elevations in troponins in conjunction with clinical symptom and electrocardiographic (ECG) changes are used to diagnose acute myocardial injury and to determine short- and long-term adverse outcomes. The high prevalence of persistently elevated troponin levels in asymptomatic ESRD patients makes it difficult to interpret cardiac troponin levels. Troponin T relative to I isotype is the most elevated of the two, therefore several assessment assays screen troponin T. It is unclear whether the chronic troponin elevation is linked to impaired kidney clearance or enhanced cardiac release. Several lines of evidence support that increased cardiac release of troponins, rather than decreased clearance, is the primary etiology of elevated basal levels in these patients (269, 270). The study by Diris et al. supports the theory of poor renal clearance (271). The authors demonstrated that troponin T, despite being a large molecule, is degraded before being excreted by the kidneys and can thus still be detected by traditional methods. Subclinical myocardial damage from micro-infarctions and those associated with left ventricular hypertrophy without clinical evidence of acute myocardial ischemia have also been proposed as mechanisms contributing to chronic troponin elevation (272–274). In renal dialysis patients, several studies have proposed different mechanisms by which hemodialysis and ESRD state could contribute to troponin elevation. These include elevated ventricular wall pressure and stretch, coronary microvascular dysfunction, anemia, hypotension, uremia, hemoconcentration, dialysis related cardiac injury, decreased clearance, or binding to dialysis membrane of cardiac troponin fragments (275).
Although there is limited supporting data, it is believed that decreased level of cardiac troponin I (cTnI) among patients on peritoneal dialysis patients compared to hemodialysis patients (276). A small study in critical care patients undergoing continuous renal replacement therapy (CRRT) revealed a decline in cardiac troponin level in the first 5 to10 hours after initiation of CRRT. Therefore, when interpreting serial cardiac troponins in dialysis patients for the diagnosis of acute myocardial infarction (AMI) timing of dialysis should be considered. Hemodialysis enhances troponin clearance therefore assays obtained in the immediate period could lead to falsely low levels. Miller-Hodges et al (233) in their study to risk stratify dialysis patients based on high sensitivity troponin found that in patients being assessed for acute coronary syndrome, high-sensitivity cardiac troponin categorized fewer individuals with renal impairment as low risk, and a majority as high risk, specificity was also reduced for type 1 MI. Several other risk stratification tools including the European Society of Cardiology 0/1-hour algorithm for rapid rule in and rule out in non-ST elevation MI, have been studied but they lacked in sensitivity, specificity, and low utility in the diagnosis of acute coronary syndromes (232, 277).
Use of serial troponin levels are preferred to diagnose acute myocardial infarction in addition to clinical symptoms and suggestive ECG findings in patients with normal kidney function as well as ESRD patients (278). There is no consensus about the level of cardiac troponin T (cTnT) or cTnI elevation needed to diagnose AMI among patients with CKD. Despite baseline elevations in troponin, it remains invaluable in diagnosing acute myocardial ischemia in the right clinical setting. Either cardiac troponin I or T may be used for diagnosis of acute MI. The Fourth Universal Definition of Myocardial Infarction recommends a change in troponin elevation above 20% from baseline or above the 99th percentile of values established in healthy individuals, for the determination of ACS ESRD patients on dialysis when accompanied by clinical symptoms and suggestive ECG findings (279). A persistently elevated troponin level, with no signs of rising or falling patterns, is most likely a sign of chronic myocardial damage, even if substantial (279). Undulating values are more likely related to other types of cardiac ischemia other than type 1 and may as well be suggestive of acute volume overload or CHF.
8.2 B natriuretic peptide
B type Natriuretic peptide (BNP) is produced in the cardiac myocytes, secreted predominantly from the ventricles. Ventricular cells are recruited to secrete BNP in response to high ventricular filling pressure, volume overload, and left ventricular dysfunction in both symptomatic and asymptomatic subjects. BNP has diuretic, natriuretic, and hypotensive effects. It also inhibits the renin-angiotensin system, endothelin secretion, and systemic and renal sympathetic activity. Plasma BNP has a variety of clinical immunoassays, which include rapid point of care assay and central lab assays. A 100 ng/L threshold is set for these assays, but correlation is weak above or below this level. A potential source of error lie in the fact that plasma BNP concentration can vary with the assay used, age, sex, and body mass index. The normal values of BNP and N-terminal pro b-type natriuretic peptide (NT – proBNP) tend to increase with age, and in women than men. On the other hand, they are lower in obese individuals. In normal subjects, plasma concentrations of BNP and NT-proBNP are similar. However, in subjects with LV dysfunction and ESRD, plasma NT-proBNP assays are comparatively higher than BNP. While scaling varies among BNP assays, NT-proBNP assays are standardized. An NT-proBNP level > 900 pg/mL provides equivalent accuracy as a BNP level of > 100 pg/mL for diagnosis of heart failure.
Whether or not subjects have clinically diagnosed HF, Plasma BNP and NT-proBNP concentrations are elevated in ESRD patients. Decreased GFR is directly associated with persistently elevated BNP and NT- proBNP concentrations. BNP is cleared by the receptor-mediated binding and removal, neutral endopeptidase, as well as by passive excretion so, GFR is inversely related to BNP concentrations. As a result, cutoff values for plasma BNP in patients with ESKD are different from those in patients with normal renal function. Elevation in BNP levels may also result from volume expansion or LV hypertrophy commonly found in dialysis patients.
Hemodialysis has been shown to affect serum concentrations of BNP and NT-proBNP. BNP is a smaller peptide and is cleared by low flux membranes whereas NT- proBNP is only cleared by high flux membranes. Consequently, serum concentrations of BNP and NT-proBNP depend on the type of ultrafiltration used during hemodialysis. Mahmood et al (280) The effect of peritoneal dialysis on the clearance of plasma BNP and NT-proBNP remains unclear. Neither BNP nor NT-proBNP is sufficiently accurate for diagnosing HF in patients with ESRD. According to Cheng et al (281), in stable ischemic heart disease, BNP and NT-proBNP display strong and near-identical test performance in ruling out severely reduced LVEF and in prediction of all-cause mortality or heart failure despite significant effects of age, gender, and renal function on levels of both markers.
8.3 Suppression of tumorigenicity-2 (ST2)
Suppression of tumorigenicity-2 (ST2) belongs to the interleukin (IL)-1 receptor family and is released under conditions of myocardial and vascular strain. ST2 consists of 2 isoforms, a transmembrane ligand (ST2 ligand) and a soluble component (sST2). The ST2 system plays an essential role in mediating myocardial and vascular remodeling and fibrosis, early atherosclerosis, and hypertension. Concentrations of sST2 are not useful in diagnosing heart failure, but they have strong prognostic value in acute and chronic CHF (282). The prognostic value of ST2 is not influenced by renal function, suggesting that ST2 may well serve as a surrogate bio marker in patients with renal insufficiency.
8.4 Galectin-3
Galectin-3 is a macrophage lectin product that plays a role in a cascade of events leading to tissue fibrosis which is a hallmark of cardiac remodeling and heart failure. Several studies of plasma GAL-3 as a biomarker in heart failure have been published which shows that GAL-3 was independently predictive of mortality in chronic heart failure patients. Similar to findings in the general population, GAL-3 is an independent predictor of mortality in HD patients (283). Both sST2 and GAL-3 received a class II recommendation in the American College of Cardiology/American Heart Association/Heart failure Society of America HF guidelines for risk prediction in HF.
8.5 Beta 2-microglobulin
Although a few studies have found a link between Beta2- Macroglobulin (B2M) and the risk of cardiovascular disease (CVD) in patients undergoing dialysis, it remains poorly understood at this time. B2M is a low molecular weight protein in the HLA complex that is similar to immunoglobulins. Due to its diminutive size, it is easily filtered at the glomerulus and subsequently metabolized by the proximal tubular cells. Serum levels tend to be higher in renal dysfunction (284). B2M has been identified as an inflammation marker and is toxic to blood vessels by contributing to amyloid formation (285). Further investigations are warranted for B2M in the context of predicting CVD risk.
8.6 Matrix metalloproteinases
Matrix metalloproteinases (MMPs) are a family of Zn2+- and Ca2+-dependent enzymes, which are important in the resorption of extracellular matrices in both normal physiological processes and pathological states. MMPs are one of the two systems that predominate and interact to achieve homeostasis within the vessel wall. They play key roles in the pathogenesis of atherosclerosis and postangioplasty restenosis (286). Nine MMPs have been identified. In patients with CKD and diabetics, serum levels of MMP-2, MMP-8, and MMP-9 were found to be higher, being correlated with serum phosphate (MMP-2), fibroblast growth factor-23 (FGF-23), and proteinuria (MMP-8 and MMP-9), which are both associated with oxidative stress and cardiovascular health. In addition, MMP-2 has been directly linked to vascular calcification, atherosclerotic plaque rupture, and carotid intima-media thickness, making it vital to atherogenesis (287).
8.7 Conclusions and future perspectives
Maintenance hemodialysis patients experience anatomical and functional cardiovascular complications, in addition to the stress related to hemodialysis. Cardiovascular mortality is still pervasive despite advancements in dialysis modalities. Nontraditional risk factors related to uremia are associated with higher cardiovascular morbidity and mortality rates in dialysis patients. The identification of cardiovascular disease risk factors and end organ damage in dialysis patients contributes greatly to the reduction of cardiovascular disease morbidity and mortality. Screening modalities in non-dialysis patients are also employed in cardiovascular assessment in this population. However, interpretation must be undertaken with careful consideration of contemporary guideline recommendations. Further research into how to effectively risk stratify these patients is desperately needed to mitigate cardiovascular complications in this vulnerable demographic.
Author contributions
GE, DK conceptualized the topic. GE, IS, SB, IB-R and DK drafted the manuscript. GE, DK, IB-R critically reviewed the manuscript.
Conflict of interest
The authors declare that the research was conducted in the absence of any commercial or financial relationships that could be construed as a potential conflict of interest.
Publisher’s note
All claims expressed in this article are solely those of the authors and do not necessarily represent those of their affiliated organizations, or those of the publisher, the editors and the reviewers. Any product that may be evaluated in this article, or claim that may be made by its manufacturer, is not guaranteed or endorsed by the publisher.
Glossary
References
1. United States Renal Data System. 2022 USRDS Annual Data Report: Epidemiology of kidney disease in the United States (2022). Available at: https://adr.usrds.org/2022.
2. Cozzolino M, Mangano M, Stucchi A, Ciceri P, Conte F, Galassi A. Cardiovascular disease in dialysis patients. Nephrol Dial Transplant (2018) 33(suppl_3):iii28–34. doi: 10.1093/ndt/gfy174
3. Eknoyan G, Beck GJ, Cheung AK, Daugirdas JT, Greene T, Kusek JW, et al. Effect of dialysis dose and membrane flux in maintenance hemodialysis. N Engl J Med (2002) 347(25):2010–9. doi: 10.1056/NEJMoa021583
4. de Jager DJ, Grootendorst DC, Jager KJ, van Dijk PC, Tomas LM, Ansell D, et al. Cardiovascular and noncardiovascular mortality among patients starting dialysis. Jama (2009) 302(16):1782–9. doi: 10.1001/jama.2009.1488
5. Sarnak MJ, Levey AS, Schoolwerth AC, Coresh J, Culleton B, Hamm LL, et al. Kidney disease as a risk factor for development of cardiovascular disease: a statement from the American Heart Association Councils on Kidney in Cardiovascular Disease, High Blood Pressure Research, Clinical Cardiology, and Epidemiology and Prevention. Hypertension (2003) 42(5):1050–65. doi: 10.1161/01.HYP.0000102971.85504.7c
6. Tonelli M, Wiebe N, Culleton B, House A, Rabbat C, Fok M, et al. Chronic kidney disease and mortality risk: a systematic review. J Am Soc Nephrol (2006) 17(7):2034–47. doi: 10.1681/ASN.2005101085
7. Foley RN, Parfrey PS, Sarnak MJ. Epidemiology of cardiovascular disease in chronic renal disease. J Am Soc Nephrol (1998) 9(12 Suppl):S16–23. doi: 10.1053/ajkd.1998.v32.pm9820470
8. Stack AG, Bloembergen WE. Prevalence and clinical correlates of coronary artery disease among new dialysis patients in the United States: a cross-sectional study. J Am Soc Nephrol (2001) 12(7):1516–23. doi: 10.1681/ASN.V1271516
9. Foley RN, Parfrey PS. Cardiac disease in chronic uremia: clinical outcome and risk factors. Adv Ren Replace Ther (1997) 4(3):234–48. doi: 10.1016/s1073-4449(97)70032-3
10. Cheung AK, Sarnak MJ, Yan G, Berkoben M, Heyka R, Kaufman A, et al. Cardiac diseases in maintenance hemodialysis patients: results of the HEMO Study. Kidney Int (2004) 65(6):2380–9. doi: 10.1111/j.1523-1755.2004.00657.x
11. Parfrey PS, Foley RN, Harnett JD, Kent GM, Murray DC, Barre PE. Outcome and risk factors for left ventricular disorders in chronic uraemia. Nephrol Dial Transplant (1996) 11(7):1277–85. doi: 10.1093/ndt/11.7.1277
12. Harnett JD, Foley RN, Kent GM, Barre PE, Murray D, Parfrey PS. Congestive heart failure in dialysis patients: prevalence, incidence, prognosis and risk factors. Kidney Int (1995) 47(3):884–90. doi: 10.1038/ki.1995.132
13. Genovesi S, Pogliani D, Faini A, Valsecchi MG, Riva A, Stefani F, et al. Prevalence of atrial fibrillation and associated factors in a population of long-term hemodialysis patients. Am J Kidney Dis (2005) 46(5):897–902. doi: 10.1053/j.ajkd.2005.07.044
14. Winkelmayer WC, Patrick AR, Liu J, Brookhart MA, Setoguchi S. The increasing prevalence of atrial fibrillation among hemodialysis patients. J Am Soc Nephrol (2011) 22(2):349–57. doi: 10.1681/ASN.2010050459
15. Wizemann V, Tong L, Satayathum S, Disney A, Akiba T, Fissell RB, et al. Atrial fibrillation in hemodialysis patients: clinical features and associations with anticoagulant therapy. Kidney Int (2010) 77(12):1098–106. doi: 10.1038/ki.2009.477
16. Chertow GM, Levin NW, Beck GJ, Depner TA, Eggers PW, Gassman JJ, et al. In-center hemodialysis six times per week versus three times per week. N Engl J Med (2010) 363(24):2287–300. doi: 10.1056/NEJMoa1001593
17. Middleton RJ, Parfrey PS, Foley RN. Left ventricular hypertrophy in the renal patient. J Am Soc Nephrol (2001) 12(5):1079–84. doi: 10.1681/ASN.V1251079
18. Jankowski J, Floege J, Fliser D, Böhm M, Marx N. Cardiovascular disease in chronic kidney disease: pathophysiological insights and therapeutic options. Circulation (2021) 143(11):1157–72. doi: 10.1161/circulationaha.120.050686
19. Buglioni A, Burnett JC Jr. Pathophysiology and the cardiorenal connection in heart failure. Circulating hormones: biomarkers or mediators. Clin Chim Acta (2015) 443:3–8. doi: 10.1016/j.cca.2014.10.027
20. Onal EM, Sag AA, Sal O, Yerlikaya A, Afsar B, Kanbay M. Erythropoietin mediates brain-vascular-kidney crosstalk and may be a treatment target for pulmonary and resistant essential hypertension. Clin Exp Hypertens (2017) 39(3):197–209. doi: 10.1080/10641963.2016.1246565
21. Tan K, Sethi SK. Biomarkers in cardiorenal syndromes. Trans Res (2014) 164(2):122–34. doi: 10.1016/j.trsl.2014.04.011
22. Agharazii M, St-Louis R, Gautier-Bastien A, Ung RV, Mokas S, Larivière R, et al. Inflammatory cytokines and reactive oxygen species as mediators of chronic kidney disease-related vascular calcification. Am J Hypertens (2015) 28(6):746–55. doi: 10.1093/ajh/hpu225
23. Matsushita K, Coresh J, Sang Y, Chalmers J, Fox C, Guallar E, et al. Estimated glomerular filtration rate and albuminuria for prediction of cardiovascular outcomes: a collaborative meta-analysis of individual participant data. Lancet Diabetes Endocrinol (2015) 3(7):514–25. doi: 10.1016/S2213-8587(15)00040-6
24. Schiffrin EL, Lipman ML, Mann JF. Chronic kidney disease: effects on the cardiovascular system. Circulation (2007) 116(1):85–97. doi: 10.1161/CIRCULATIONAHA.106.678342
25. Go AS, Chertow GM, Fan D, McCulloch CE, Hsu CY. Chronic kidney disease and the risks of death, cardiovascular events, and hospitalization. N Engl J Med (2004) 351(13):1296–305. doi: 10.1056/NEJMoa041031
26. Levey AS, Bosch JP, Lewis JB, Greene T, Rogers N, Roth D. A more accurate method to estimate glomerular filtration rate from serum creatinine: a new prediction equation. Modification of Diet in Renal Disease Study Group. Ann Intern Med (1999) 130(6):461–70. doi: 10.7326/0003-4819-130-6-199903160-00002
27. Foundation NK. K/DOQI clinical practice guidelines for chronic kidney disease: evaluation, classification, and stratification. Am J Kidney Dis (2002) 39(2 Suppl 1):S1–266.
28. Enia G, Mallamaci F, Benedetto FA, Panuccio V, Parlongo S, Cutrupi S, et al. Long-term CAPD patients are volume expanded and display more severe left ventricular hypertrophy than haemodialysis patients. Nephrol Dial Transplant (2001) 16(7):1459–64. doi: 10.1093/ndt/16.7.1459
29. Margetts PJ, Churchill DN. Acquired ultrafiltration dysfunction in peritoneal dialysis patients. J Am Soc Nephrol (2002) 13(11):2787–94. doi: 10.1681/asn.v13112787
30. Lui YW, Geras K, Block KT, Parente M, Hood J, Recht MP. How to implement AI in the clinical enterprise: opportunities and lessons learned. J Am Coll Radiol (2020) 17(11):1394–7. doi: 10.1016/j.jacr.2020.09.039
31. Metivier F, Marchais SJ, Guerin AP, Pannier B, London GM. Pathophysiology of anaemia: focus on the heart and blood vessels. Nephrol Dial Transplant (2000) 15 Suppl 3:14–8. doi: 10.1093/oxfordjournals.ndt.a027970
32. Meena O, Meghwal H, Meena R, Gurjar S. A study on correlation of anemia with left ventricular hypertrophy in chronic kidney disease patients: a cross sectional study from Southern Rajasthan. Int J Adv Med (2022) 9:796. doi: 10.18203/2349-3933.ijam20221706
33. Jesuorobo DE, Odia JO, Uchenna DI. Left ventricular hypertrophy and its correlates in chronic kidney disease patients in a Nigerian tertiary hospital. Int J Internal Med (2012) 1:11–6.
34. Foley RN, Parfrey PS, Harnett JD, Kent GM, Martin CJ, Murray DC, et al. Clinical and echocardiographic disease in patients starting end-stage renal disease therapy. Kidney Int (1995) 47(1):186–92. doi: 10.1038/ki.1995.22
35. Foley RN, Curtis BM, Randell EW, Parfrey PS. Left ventricular hypertrophy in new hemodialysis patients without symptomatic cardiac disease. Clin J Am Soc Nephrol (2010) 5(5):805–13. doi: 10.2215/cjn.07761109
36. Harnett JD, Kent GM, Barre PE, Taylor R, Parfrey PS. Risk factors for the development of left ventricular hypertrophy in a prospectively followed cohort of dialysis patients. J Am Soc Nephrol (1994) 4(7):1486–90. doi: 10.1681/asn.v471486
37. Foley RN, Parfrey PS, Harnett JD, Kent GM, Murray DC, Barre PE. The impact of anemia on cardiomyopathy, morbidity, and and mortality in end-stage renal disease. Am J Kidney Dis (1996) 28(1):53–61. doi: 10.1016/s0272-6386(96)90130-4
38. Foley RN, Parfrey PS, Harnett JD, Kent GM, Murray DC, Barre PE. Impact of hypertension on cardiomyopathy, morbidity and mortality in end-stage renal disease. Kidney Int (1996) 49(5):1379–85. doi: 10.1038/ki.1996.194
39. Weiner DE, Tighiouart H, Vlagopoulos PT, Griffith JL, Salem DN, Levey AS, et al. Effects of anemia and left ventricular hypertrophy on cardiovascular disease in patients with chronic kidney disease. J Am Soc Nephrol (2005) 16(6):1803–10. doi: 10.1681/asn.2004070597
40. Levin A, Thompson CR, Ethier J, Carlisle EJ, Tobe S, Mendelssohn D, et al. Left ventricular mass index increase in early renal disease: impact of decline in hemoglobin. Am J Kidney Dis (1999) 34(1):125–34. doi: 10.1016/s0272-6386(99)70118-6
41. London GM, Fabiani F, Marchais SJ, de Vernejoul MC, Guerin AP, Safar ME, et al. Uremic cardiomyopathy: an inadequate left ventricular hypertrophy. Kidney Int (1987) 31(4):973–80. doi: 10.1038/ki.1987.94
42. London GM, Parfrey PS. Cardiac disease in chronic uremia: pathogenesis. Adv Ren Replace Ther (1997) 4(3):194–211. doi: 10.1016/s1073-4449(97)70029-3
43. Silberberg JS, Rahal DP, Patton DR, Sniderman AD. Role of anemia in the pathogenesis of left ventricular hypertrophy in end-stage renal disease. Am J Cardiol (1989) 64(3):222–4. doi: 10.1016/0002-9149(89)90462-1
44. Oski FA, Marshall BE, Cohen PJ, Sugerman HJ, Miller LD. The role of the left-shifted or right-shifted oxygen-hemoglobin equilibrium curve. Ann Intern Med (1971) 74(1):44–6. doi: 10.7326/0003-4819-74-1-44
45. Varat MA, Adolph RJ, Fowler NO. Cardiovascular effects of anemia. Am Heart J (1972) 83(3):415–26. doi: 10.1016/0002-8703(72)90445-0
46. Katz AM. Cardiomyopathy of overload. A major determinant of prognosis in congestive heart failure. N Engl J Med (1990) 322(2):100–10. doi: 10.1056/nejm199001113220206
47. Ritz E. Left ventricular hypertrophy in renal disease: beyond preload and afterload. Kidney Int (2009) 8:771–3. doi: 10.1038/ki.2009.35
48. London GM, Guerin AP, Marchais SJ, Pannier B, Safar ME, Day M, et al. Cardiac and arterial interactions in end-stage renal disease. Kidney Int Aug (1996) 50(2):600–8. doi: 10.1038/ki.1996.355
49. Greaves SC, Gamble GD, Collins JF, Whalley GA, Sharpe DN. Determinants of left ventricular hypertrophy and systolic dysfunction in chronic renal failure. Am J Kidney Dis (1994) 24(5):768–76. doi: 10.1016/s0272-6386(12)80670-6
50. Amann K, Rychlík I, Miltenberger-Milteny G, Ritz E. Left ventricular hypertrophy in renal failure. Kidney Int Suppl (1998) 68:S78–85. doi: 10.1046/j.1523-1755.1998.06818.x
51. Han BG, Lee JY, Choi SO, Yang JW, Kim JS. Relative overhydration is independently associated with left ventricular hypertrophy in dialysis naïve patients with stage 5 chronic kidney disease. Sci Rep (2020) 10(1):15924. doi: 10.1038/s41598-020-73038-8
52. Gutiérrez OM, Mannstadt M, Isakova T, Rauh-Hain JA, Tamez H, Shah A, et al. Fibroblast growth factor 23 and mortality among patients undergoing hemodialysis. N Engl J Med (2008) 359(6):584–92. doi: 10.1056/NEJMoa0706130
53. Tanaka S, Fujita S, Kizawa S, Morita H, Ishizaka N. Association between FGF23, α-Klotho, and Cardiac AbnorMalities among Patients with Various Chronic Kidney Disease Stages. PloS One (2016) 11(7):e0156860. doi: 10.1371/journal.pone.0156860
54. Jovanovich A, Ix JH, Gottdiener J, McFann K, Katz R, Kestenbaum B, et al. Fibroblast growth factor 23, left ventricular mass, and left ventricular hypertrophy in community-dwelling older adults. Atherosclerosis (2013) 231(1):114–9. doi: 10.1016/j.atherosclerosis.2013.09.002
55. Canziani ME, Tomiyama C, Higa A, Draibe SA, Carvalho AB. Fibroblast growth factor 23 in chronic kidney disease: bridging the gap between bone mineral metabolism and left ventricular hypertrophy. Blood Purif (2011) 31(1-3):26–32. doi: 10.1159/000321368
56. Leifheit-Nestler M, Kirchhoff F, Nespor J, Richter B, Soetje B, Klintschar M, et al. Fibroblast growth factor 23 is induced by an activated renin-angiotensin-aldosterone system in cardiac myocytes and promotes the pro-fibrotic crosstalk between cardiac myocytes and fibroblasts. Nephrol Dial Transplant (2018) 33(10):1722–34. doi: 10.1093/ndt/gfy006
57. Schreiber BD. Congestive heart failure in patients with chronic kidney disease and on dialysis. Am J Med Sci (2003) 325(4):179–93. doi: 10.1097/00000441-200304000-00004
58. Stack AG, Molony DA, Rahman NS, Dosekun A, Murthy B. Impact of dialysis modality on survival of new ESRD patients with congestive heart failure in the United States. Kidney Int (2003) 64(3):1071–9. doi: 10.1046/j.1523-1755.2003.00165.x
59. Trespalacios FC, Taylor AJ, Agodoa LY, Bakris GL, Abbott KC. Heart failure as a cause for hospitalization in chronic dialysis patients. Am J Kidney Dis (2003) 41(6):1267–77. doi: 10.1016/s0272-6386(03)00359-7
60. Yamada S, Ishii H, Takahashi H, Aoyama T, Morita Y, Kasuga H, et al. Prognostic value of reduced left ventricular ejection fraction at start of hemodialysis therapy on cardiovascular and all-cause mortality in end-stage renal disease patients. Clin J Am Soc Nephrol (2010) 5(10):1793–8. doi: 10.2215/cjn.00050110
61. Burton JO, Jefferies HJ, Selby NM, McIntyre CW. Hemodialysis-induced repetitive myocardial injury results in global and segmental reduction in systolic cardiac function. Clin J Am Soc Nephrol (2009) 4(12):1925–31. doi: 10.2215/cjn.04470709
62. Collins AJ, Foley RN, Chavers B, Gilbertson D, Herzog C, Johansen K, et al. ‘United States Renal Data System 2011 Annual Data Report: Atlas of chronic kidney disease & end-stage renal disease in the United States. Am J Kidney Dis (2012) 1 Suppl 1:A7, e1–420.
63. Glassock RJ, Pecoits-Filho R, Barberato SH. Left ventricular mass in chronic kidney disease and ESRD. Clin J Am Soc Nephrol (2009) 4 Suppl 1:S79–91. doi: 10.2215/cjn.04860709
64. Reddy YNV, Obokata M, Dean PG, Melenovsky V, Nath KA, Borlaug BA. Long-term cardiovascular changes following creation of arteriovenous fistula in patients with end stage renal disease. Eur Heart J (2017) 38(24):1913–23. doi: 10.1093/eurheartj/ehx045
65. Caroli A, Manini S, Antiga L, Passera K, Ene-Iordache B, Rota S, et al. Validation of a patient-specific hemodynamic computational model for surgical planning of vascular access in hemodialysis patients. Kidney Int (2013) 84(6):1237–45. doi: 10.1038/ki.2013.188
66. Basile C, Lomonte C, Vernaglione L, Casucci F, Antonelli M, Losurdo N. The relationship between the flow of arteriovenous fistula and cardiac output in haemodialysis patients. Nephrol Dial Transplant (2008) 23(1):282–7. doi: 10.1093/ndt/gfm549
67. Wijnen E, Keuter XH, Planken NR, van der Sande FM, Tordoir JH, Leunissen KM, et al. The relation between vascular access flow and different types of vascular access with systemic hemodynamics in hemodialysis patients. Artif Organs (2005) 29(12):960–4. doi: 10.1111/j.1525-1594.2005.00165.x
68. Korsheed S, Eldehni MT, John SG, Fluck RJ, McIntyre CW. Effects of arteriovenous fistula formation on arterial stiffness and cardiovascular performance and function. Nephrol Dial Transplant (2011) 26(10):3296–302. doi: 10.1093/ndt/gfq851
69. London GM. Left ventricular alterations and end-stage renal disease. Nephrol Dial Transplant (2002) 17 Suppl 1:29–36. doi: 10.1093/ndt/17.suppl_1.29
70. Banerjee D, Ma JZ, Collins AJ, Herzog CA. Long-term survival of incident hemodialysis patients who are hospitalized for congestive heart failure, pulmonary edema, or fluid overload. Clin J Am Soc Nephrol (2007) 2(6):1186–90. doi: 10.2215/cjn.01110307
71. Ori Y, Korzets A, Katz M, Erman A, Weinstein T, Malachi T, et al. The contribution of an arteriovenous access for hemodialysis to left ventricular hypertrophy. Am J Kidney Dis (2002) 40(4):745–52. doi: 10.1053/ajkd.2002.35685
72. Parfrey PS, Foley RN, Harnett JD, Kent GM, Murray D, Barre PE. Outcome and risk factors of ischemic heart disease in chronic uremia. Kidney Int (1996) 49(5):1428–34. doi: 10.1038/ki.1996.201
73. Barrett BJ, Parfrey PS, Morgan J, Barré P, Fine A, Goldstein MB, et al. Prediction of early death in end-stage renal disease patients starting dialysis. Am J Kidney Dis (1997) 29(2):214–22. doi: 10.1016/s0272-6386(97)90032-9
74. Joki N, Hase H, Nakamura R, Yamaguchi T. Onset of coronary artery disease prior to initiation of haemodialysis in patients with end-stage renal disease. Nephrol Dial Transplant (1997) 12(4):718–23. doi: 10.1093/ndt/12.4.718
75. Manjunath G, Tighiouart H, Ibrahim H, MacLeod B, Salem DN, Griffith JL, et al. Level of kidney function as a risk factor for atherosclerotic cardiovascular outcomes in the community. J Am Coll Cardiol (2003) 41(1):47–55. doi: 10.1016/s0735-1097(02)02663-3
76. Herzog CA, Littrell K, Arko C, Frederick PD, Blaney M. Clinical characteristics of dialysis patients with acute myocardial infarction in the United States: a collaborative project of the United States Renal Data System and the National Registry of Myocardial Infarction. Circulation (2007) 116(13):1465–72. doi: 10.1161/circulationaha.107.696765
77. Ritz E, Deppisch R, Stier E, Hänsch G. Atherogenesis and cardiac death: are they related to dialysis procedure and biocompatibility? Nephrol Dial Transplant (1994) 9 Suppl 2:165–72.
78. Collins AJ, Foley RN, Chavers B, Gilbertson D, Herzog C, Ishani A, et al. US renal data system 2013 annual data report. Am J Kidney Dis (2014) 63(1 Suppl):A7. doi: 10.1053/j.ajkd.2013.11.001
79. Sabe MA, Claggett B, Burdmann EA, Desai AS, Ivanovich P, Kewalramani R, et al. Coronary artery disease is a predictor of progression to dialysis in patients with chronic kidney disease, type 2 diabetes mellitus, and anemia: an analysis of the trial to reduce cardiovascular events with aranesp therapy (TREAT). J Am Heart Assoc (2016) 5(4). doi: 10.1161/jaha.115.002850
80. Nakamura S, Ishibashi-Ueda H, Niizuma S, Yoshihara F, Horio T, Kawano Y. Coronary calcification in patients with chronic kidney disease and coronary artery disease. Clin J Am Soc Nephrol (2009) 4(12):1892–900. doi: 10.2215/cjn.04320709
81. Drüeke TB. Arterial intima and media calcification: distinct entities with different pathogenesis or all the same? Clin J Am Soc Nephrol (2008) 3(6):1583–4. doi: 10.2215/cjn.03250708
82. Bellasi A, Raggi P. Techniques and technologies to assess vascular calcification. Semin Dial (2007) 20(2):129–33. doi: 10.1111/j.1525-139X.2007.00259.x
83. London GM, Guérin AP, Marchais SJ, Métivier F, Pannier B, Adda H. Arterial media calcification in end-stage renal disease: impact on all-cause and cardiovascular mortality. Nephrol Dial Transplant (2003) 18(9):1731–40. doi: 10.1093/ndt/gfg414
84. McCullough PA, Agrawal V, Danielewicz E, Abela GS. Accelerated atherosclerotic calcification and Monckeberg’s sclerosis: a continuum of advanced vascular pathology in chronic kidney disease. Clin J Am Soc Nephrol (2008) 3(6):1585–98. doi: 10.2215/cjn.01930408
85. Amann K. Media calcification and intima calcification are distinct entities in chronic kidney disease. Clin J Am Soc Nephrol (2008) 3(6):1599–605. doi: 10.2215/cjn.02120508
86. McCullough PA, SOman S. Cardiovascular calcification in patients with chronic renal failure: are we on target with this risk factor? Kidney Int Suppl (2004) 90):S18–24. doi: 10.1111/j.1523-1755.2004.09008.x
87. Budoff MJ, Rader DJ, Reilly MP, Mohler ER 3rd, Lash J, Yang W, et al. Relationship of estimated GFR and coronary artery calcification in the CRIC (Chronic Renal Insufficiency Cohort) Study. Am J Kidney Dis (2011) 58(4):519–26. doi: 10.1053/j.ajkd.2011.04.024
88. Garland JS, Holden RM, Hopman WM, Gill SS, Nolan RL, Morton AR. Body mass index, coronary artery calcification, and kidney function decline in stage 3 to 5 chronic kidney disease patients. J Ren Nutr (2013) 23(1):4–11. doi: 10.1053/j.jrn.2011.12.008
89. Johnson RC, Leopold JA, Loscalzo J. Vascular calcification: pathobiological mechanisms and clinical implications. Circ Res (2006) 99(10):1044–59. doi: 10.1161/01.res.0000249379.55535.21
90. Bostom AG, Culleton BF. Hyperhomocysteinemia in chronic renal disease. J Am Soc Nephrol (1999) 10(4):891–900. doi: 10.1681/asn.v104891
91. Bostom AG, Silbershatz H, Rosenberg IH, Selhub J, D'Agostino RB, Wolf PA, et al. Nonfasting plasma total homocysteine levels and all-cause and cardiovascular disease mortality in elderly Framingham men and women. Arch Intern Med (1999) 159(10):1077–80. doi: 10.1001/archinte.159.10.1077
92. Moustapha A, Naso A, Nahlawi M, Gupta A, Arheart KL, Jacobsen DW, et al. Prospective study of hyperhomocysteinemia as an adverse cardiovascular risk factor in end-stage renal disease. Circulation (1998) 97(2):138–41. doi: 10.1161/01.cir.97.2.138
93. Friedman AN, Bostom AG, Selhub J, Levey AS, Rosenberg IH. The kidney and homocysteine metabolism. J Am Soc Nephrol (2001) 12(10):2181–9. doi: 10.1681/asn.v12102181
94. Foley RN, Parfrey PS, Sarnak MJ. Clinical epidemiology of cardiovascular disease in chronic renal disease. Am J Kidney Dis (1998) 32(5 Suppl 3):S112–9. doi: 10.1053/ajkd.1998.v32.pm9820470
95. Bots ML, Launer LJ, Lindemans J, Hofman A, Grobbee DE. Homocysteine, atherosclerosis and prevalent cardiovascular disease in the elderly: The Rotterdam Study. J Intern Med (1997) 242(4):339–47. doi: 10.1046/j.1365-2796.1997.00239.x
96. McQuillan BM, Beilby JP, Nidorf M, Thompson PL, Hung J. Hyperhomocysteinemia but not the C677T mutation of methylenetetrahydrofolate reductase is an independent risk determinant of carotid wall thickening. The Perth Carotid Ultrasound Disease Assessment Study (CUDAS). Circulation (1999) 99(18):2383–8. doi: 10.1161/01.cir.99.18.2383
97. Voutilainen S, Alfthan G, Nyyssönen K, Salonen R, Salonen JT. Association between elevated plasma total homocysteine and increased common carotid artery wall thickness. Ann Med (1998) 30(3):300–6. doi: 10.3109/07853899809005859
98. Samad Z, Sivak JA, Phelan M, Schulte PJ, Patel U, Velazquez EJ. Prevalence and outcomes of left-sided valvular heart disease associated with chronic kidney disease. J Am Heart Assoc (2017) 6(10). doi: 10.1161/jaha.117.006044
99. Raggi P, Boulay A, Chasan-Taber S, Amin N, Dillon M, Burke SK, et al. Cardiac calcification in adult hemodialysis patients. A link between end-stage renal disease and cardiovascular disease? J Am Coll Cardiol (2002) 39(4):695–701. doi: 10.1016/s0735-1097(01)01781-8
100. Freeman RV, Otto CM. Spectrum of calcific aortic valve disease: pathogenesis, disease progression, and treatment strategies. Circulation (2005) 111(24):3316–26. doi: 10.1161/circulationaha.104.486738
101. Ganesh SK, Stack AG, Levin NW, Hulbert-Shearon T, Port FK. Association of elevated serum PO(4), Ca x PO(4) product, and parathyroid hormone with cardiac mortality risk in chronic hemodialysis patients. J Am Soc Nephrol (2001) 12(10):2131–8. doi: 10.1681/asn.v12102131
102. Otto CM, Kuusisto J, Reichenbach DD, Gown AM, O’Brien KD. Characterization of the early lesion of ‘degenerative’ valvular aortic stenosis. Histological and immunohistochemical studies. Circulation (1994) 90(2):844–53. doi: 10.1161/01.cir.90.2.844
103. Wang AYM, Woo J, Wang M, Sea MMM, Ip R, Li PKT, et al. Association of inflammation and malnutrition with cardiac valve calcification in continuous ambulatory peritoneal dialysis patients. J Am Soc Nephrol (2001) 12(9):1927–36. doi: 10.1681/asn.v1291927
104. Baglin A, Hanslik T, Vaillant JN, Boulard JC, Moulonguet-Doleris L, Prinseau J. Severe valvular heart disease in patients on chronic dialysis. A five-year multicenter French survey. Ann Med Interne (Paris) (1997) 148(8):521–6.
105. Salusky IB, Goodman WG. Cardiovascular calcification in end-stage renal disease. Nephrol Dial Transplant (2002) 17(2):336–9. doi: 10.1093/ndt/17.2.336
106. Otto CM, Lind BK, Kitzman DW, Gersh BJ, Siscovick DS. Association of aortic-valve sclerosis with cardiovascular mortality and morbidity in the elderly. N Engl J Med (1999) 341(3):142–7. doi: 10.1056/nejm199907153410302
107. D’Cruz I, Panetta F, Cohen H, Glick G. Submitral calcification or sclerosis in elderly patients: M mode and two dimensional echocardiography in “mitral anulus calcification”. Am J Cardiol (1979) 44(1):31–8. doi: 10.1016/0002-9149(79)90247-9
108. Straumann E, Meyer B, Misteli M, Blumberg A, Jenzer HR. Aortic and mitral valve disease in patients with end stage renal failure on long-term haemodialysis. Br Heart J (1992) 67(3):236–9. doi: 10.1136/hrt.67.3.236
109. Shuvy M, Abedat S, Beeri R, Danenberg HD, Planer D, Ben-Dov IZ, et al. Uraemic hyperparathyroidism causes a reversible inflammatory process of aortic valve calcification in rats. Cardiovasc Res (2008) 79(3):492–9. doi: 10.1093/cvr/cvn088
110. Miller JD, Chu Y, Brooks RM, Richenbacher WE, Peña-Silva R, Heistad DD. Dysregulation of antioxidant mechanisms contributes to increased oxidative stress in calcific aortic valvular stenosis in humans. J Am Coll Cardiol (2008) 52(10):843–50. doi: 10.1016/j.jacc.2008.05.043
111. Rajamannan NM, Subramaniam M, Rickard D, Stock SR, Donovan J, Springett M, et al. Human aortic valve calcification is associated with an osteoblast phenotype. Circulation (2003) 107(17):2181–4. doi: 10.1161/01.cir.0000070591.21548.69
112. Pohjolainen V, Taskinen P, Soini Y, Rysä J, Ilves M, Juvonen T, et al. Noncollagenous bone matrix proteins as a part of calcific aortic valve disease regulation. Hum Pathol (2008) 39(11):1695–701. doi: 10.1016/j.humpath.2008.04.015
113. Bennett BJ, Scatena M, Kirk EA, Rattazzi M, Varon RM, Averill M, et al. Osteoprotegerin inactivation accelerates advanced atherosclerotic lesion progression and calcification in older ApoE-/- mice. Arterioscler Thromb Vasc Biol (2006) 26(9):2117–24. doi: 10.1161/01.ATV.0000236428.91125.e6
114. Steinmetz M, Skowasch D, Wernert N, Welsch U, Preusse CJ, Welz A, et al. Differential profile of the OPG/RANKL/RANK-system in degenerative aortic native and bioprosthetic valves. J Heart Valve Dis (2008) 17(2):187–93.
115. Hopewell JC, Haynes R, Baigent C. The role of lipoprotein (a) in chronic kidney disease. J Lipid Res (2018) 59(4):577–85. doi: 10.1194/jlr.R083626
116. Caira FC, Stock SR, Gleason TG, McGee EC, Huang J, Bonow RO, et al. Human degenerative valve disease is associated with up-regulation of low-density lipoprotein receptor-related protein 5 receptor-mediated bone formation. J Am Coll Cardiol (2006) 47(8):1707–12. doi: 10.1016/j.jacc.2006.02.040
117. O’Brien KD, Shavelle DM, Caulfield MT, McDonald TO, Olin-Lewis K, Otto CM, et al. Association of angiotensin-converting enzyme with low-density lipoprotein in aortic valvular lesions and in human plasma. Circulation (2002) 106(17):2224–30. doi: 10.1161/01.cir.0000035655.45453.d2
118. de Lima JJ, Vieira ML, Lopes HF, Gruppi CJ, Medeiros CJ, Ianhez LE, et al. Blood pressure and the risk of complex arrhythmia in renal insufficiency, hemodialysis, and renal transplant patients. Am J Hypertens (1999) 12(2 Pt 1):204–8. doi: 10.1016/s0895-7061(98)00232-5
119. Jassal SV, Coulshed SJ, Douglas JF, Stout RW. Autonomic neuropathy predisposing to arrhythmias in hemodialysis patients. Am J Kidney Dis (1997) 30(2):219–23. doi: 10.1016/s0272-6386(97)90055-x
120. Suzuki R, Tsumura K, Inoue T, Kishimoto H, Morii H. QT interval prolongation in the patients receiving maintenance hemodialysis. Clin Nephrol (1998) 49(4):240–4.
121. Abe S, Yoshizawa M, Nakanishi N, Yazawa T, Yokota K, Honda M, et al. Electrocardiographic abnorMalities in patients receiving hemodialysis. Am Heart J (1996) 131(6):1137–44. doi: 10.1016/s0002-8703(96)90088-5
122. Meier P, Vogt P, Blanc E. Ventricular arrhythmias and sudden cardiac death in end-stage renal disease patients on chronic hemodialysis. Nephron (2001) 3:199–214. doi: 10.1159/000045917
123. Bozbas H, Atar I, Yildirir A, Ozgul A, Uyar M, Ozdemir N, et al. Prevalence and predictors of arrhythmia in end stage renal disease patients on hemodialysis. Ren Fail (2007) 29(3):331–9. doi: 10.1080/08860220701191237
124. Denes P, Wu D, Dhingra R, Pietras RJ, Rosen KM. The effects of cycle length on cardiac refractory periods in man. Circulation (1974) 49(1):32–41. doi: 10.1161/01.cir.49.1.32
125. Grönefeld GC, Mauss O, Li YG, Klingenheben T, Hohnloser SH. Association between atrial fibrillation and appropriate implantable cardioverter defibrillator therapy: results from a prospective study. J Cardiovasc Electrophysiol (2000) 11(11):1208–14. doi: 10.1046/j.1540-8167.2000.01208.x
126. Kaur J, Young BE, Fadel PJ. Sympathetic overactivity in chronic kidney disease: consequences and mechanisms. Int J Mol Sci (2017) 18(8). doi: 10.3390/ijms18081682
127. Shang F, Wang SC, Hsu CY, Miao Y, Martin M, Yin Y, et al. MicroRNA-92a mediates endothelial dysfunction in CKD. J Am Soc Nephrol (2017) 28(11):3251–61. doi: 10.1681/asn.2016111215
128. Young CN, Fisher JP, Gallagher KM, Whaley-Connell A, Chaudhary K, Victor RG, et al. Inhibition of nitric oxide synthase evokes central sympatho-excitation in healthy humans. J Physiol (2009) 587(Pt 20):4977–86. doi: 10.1113/jphysiol.2009.177204
129. Vaseghi M, Lux RL, Mahajan A, Shivkumar K. Sympathetic stimulation increases dispersion of repolarization in humans with myocardial infarction. Am J Physiol Heart Circ Physiol (2012) 302(9):H1838–46. doi: 10.1152/ajpheart.01106.2011
130. Levick SP, Murray DB, Janicki JS, Brower GL. Sympathetic nervous system modulation of inflammation and remodeling in the hypertensive heart. Hypertension (2010) 55(2):270–6. doi: 10.1161/hypertensionaha.109.142042
131. Chesterton LJ, Sigrist MK, Bennett T, Taal MW, McIntyre CW. Reduced baroreflex sensitivity is associated with increased vascular calcification and arterial stiffness. Nephrol Dial Transplant (2005) 20(6):1140–7. doi: 10.1093/ndt/gfh808
132. Bello AK, Johnson DW, Feehally J, Harris D, Jindal K, Lunney M, et al. Global Kidney Health Atlas (GKHA): design and methods. Kidney Int Supplements (2017) 7(2):145–53. doi: 10.1016/j.kisu.2017.08.001
133. Bello AK, Levin A, Tonelli M, Okpechi IG, Feehally J, Harris D, et al. Assessment of global kidney health care status. Jama (2017) 317(18):1864–81. doi: 10.1001/jama.2017.4046
134. Locatelli F, Pozzoni P, Del Vecchio L. Renal replacement therapy in patients with diabetes and end-stage renal disease. J Am Soc Nephrol (2004) 15 Suppl 1:S25–9. doi: 10.1097/01.asn.0000093239.32602.04
135. Le Feuvre C, Borentain M, Beygui F, Helft G, Batisse JP, Metzger JP. Comparison of short- and long-term outcomes of coronary angioplasty in patients with and without diabetes mellitus and with and without hemodialysis. Am J Cardiol (2003) 92(6):721–5. doi: 10.1016/s0002-9149(03)00838-5
136. Trespalacios FC, Taylor AJ, Agodoa LY, Abbott KC. Incident acute coronary syndromes in chronic dialysis patients in the United States. Kidney Int (2002) 62(5):1799–805. doi: 10.1046/j.1523-1755.2002.00638.x
137. Saran R, Li Y, Robinson B, Ayanian J, Balkrishnan R, Bragg-Gresham J, et al. US renal data system 2014 annual data report: epidemiology of kidney disease in the United States. Am J Kidney Dis (2015) 66(1 Suppl 1):Svii, S1–305. doi: 10.1053/j.ajkd.2015.05.001
138. Kalantar-Zadeh K, Kopple JD, Regidor DL, Jing J, Shinaberger CS, Aronovitz J, et al. A1C and survival in maintenance hemodialysis patients. Diabetes Care (2007) 30(5):1049–55. doi: 10.2337/dc06-2127
139. Williams ME, Lacson E Jr., Wang W, Lazarus JM, Hakim R. Glycemic control and extended hemodialysis survival in patients with diabetes mellitus: comparative results of traditional and time-dependent Cox model analyses. Clin J Am Soc Nephrol (2010) 5(9):1595–601. doi: 10.2215/cjn.09301209
140. Zoungas S, Kerr PG, Lui M, Teede HJ. The impact of glycaemic control on outcomes in patients with end-stage renal disease and type 2 diabetes. Nephrol (Carlton) (2008) 13(2):124–7. doi: 10.1111/j.1440-1797.2007.00901.x
141. Ramirez SP, McCullough KP, Thumma JR, Nelson RG, Morgenstern H, Gillespie BW, et al. Hemoglobin A(1c) levels and mortality in the diabetic hemodialysis population: findings from the Dialysis Outcomes and Practice Patterns Study (DOPPS). Diabetes Care (2012) 35(12):2527–32. doi: 10.2337/dc12-0573
142. Ricks J, Molnar MZ, Kovesdy CP, Shah A, Nissenson AR, Williams M, et al. Glycemic control and cardiovascular mortality in hemodialysis patients with diabetes: a 6-year cohort study. Diabetes (2012) 61(3):708–15. doi: 10.2337/db11-1015
143. Rhee CM, Leung AM, Kovesdy CP, Lynch KE, Brent GA, Kalantar-Zadeh K. Updates on the management of diabetes in dialysis patients. Semin Dial (2014) 27(2):135–45. doi: 10.1111/sdi.12198
144. Agarwal R, Nissenson AR, Batlle D, Coyne DW, Trout JR, Warnock DG. Prevalence, treatment, and control of hypertension in chronic hemodialysis patients in the United States. Am J Med (2003) 115(4):291–7. doi: 10.1016/s0002-9343(03)00366-8
145. Agarwal R. Epidemiology of interdialytic ambulatory hypertension and the role of volume excess. Am J Nephrol (2011) 34(4):381–90. doi: 10.1159/000331067
146. Cocchi R, Degli Esposti E, Fabbri A, Lucatello A, Sturani A, Quarello F, et al. Prevalence of hypertension in patients on peritoneal dialysis: results of an Italian multicentre study. Nephrol Dial Transplant (1999) 14(6):1536–40. doi: 10.1093/ndt/14.6.1536
147. Sankaranarayanan N, Santos SF, Peixoto AJ. Blood pressure measurement in dialysis patients. Adv Chronic Kidney Dis (2004) 11(2):134–42. doi: 10.1053/j.arrt.2004.01.005
148. Peixoto AJ, Santos SF, Mendes RB, Crowley ST, Maldonado R, Orias M, et al. Reproducibility of ambulatory blood pressure monitoring in hemodialysis patients. Am J Kidney Dis (2000) 36(5):983–90. doi: 10.1053/ajkd.2000.19100
149. Heerspink HJ, Ninomiya T, Zoungas S, de Zeeuw D, Grobbee DE, Jardine MJ, et al. Effect of lowering blood pressure on cardiovascular events and mortality in patients on dialysis: a systematic review and meta-analysis of randomised controlled trials. Lancet (2009) 373(9668):1009–15. doi: 10.1016/s0140-6736(09)60212-9
150. Takahashi A, Takase H, Toriyama T, Sugiura T, Kurita Y, Ueda R, et al. Candesartan, an angiotensin II type-1 receptor blocker, reduces cardiovascular events in patients on chronic haemodialysis–a randomized study. Nephrol Dial Transplant (2006) 21(9):2507–12. doi: 10.1093/ndt/gfl293
151. Suzuki H, Kanno Y, Sugahara S, Ikeda N, Shoda J, Takenaka T, et al. Effect of angiotensin receptor blockers on cardiovascular events in patients undergoing hemodialysis: an open-label randomized controlled trial. Am J Kidney Dis (2008) 52(3):501–6. doi: 10.1053/j.ajkd.2008.04.031
152. Roehm B, Weiner DE. Blood pressure targets and kidney and cardiovascular disease: same data but discordant guidelines. Curr Opin Nephrol Hypertens (2019) 28(3):245–50. doi: 10.1097/mnh.0000000000000492
153. Fleischmann E, Teal N, Dudley J, May W, Bower JD, Salahudeen AK. Influence of excess weight on mortality and hospital stay in 1346 hemodialysis patients. Kidney Int (1999) 55(4):1560–7. doi: 10.1046/j.1523-1755.1999.00389.x
154. Ricks J, Molnar MZ, Kovesdy CP, Kopple JD, Norris KC, Mehrotra R, et al. Racial and ethnic differences in the association of body mass index and survival in maintenance hemodialysis patients. Am J Kidney Dis (2011) 58(4):574–82. doi: 10.1053/j.ajkd.2011.03.023
155. Kalantar-Zadeh K, Kopple JD, Kilpatrick RD, McAllister CJ, Shinaberger CS, Gjertson DW, et al. Association of morbid obesity and weight change over time with cardiovascular survival in hemodialysis population. Am J Kidney Dis (2005) 46(3):489–500. doi: 10.1053/j.ajkd.2005.05.020
156. Kalantar-Zadeh K, Kuwae N, Wu DY, Shantouf RS, Fouque D, Anker SD, et al. Associations of body fat and its changes over time with quality of life and prospective mortality in hemodialysis patients. Am J Clin Nutr (2006) 83(2):202–10. doi: 10.1093/ajcn/83.2.202
157. Johansen KL, Young B, Kaysen GA, Chertow GM. Association of body size with outcomes among patients beginning dialysis. Am J Clin Nutr (2004) 80(2):324–32. doi: 10.1093/ajcn/80.2.324
158. Badve SV, Paul SK, Klein K, Clayton PA, Hawley CM, Brown FG, et al. The association between body mass index and mortality in incident dialysis patients. PloS One (2014) 9(12):e114897. doi: 10.1371/journal.pone.0114897
159. Park J, Ahmadi SF, Streja E, Molnar MZ, Flegal KM, Gillen D, et al. Obesity paradox in end-stage kidney disease patients. Prog Cardiovasc Dis (2014) 56(4):415–25. doi: 10.1016/j.pcad.2013.10.005
160. Qureshi AR, Alvestrand A, Divino-Filho JC, Gutierrez A, Heimbürger O, Lindholm B, et al. Inflammation, malnutrition, and cardiac disease as predictors of mortality in hemodialysis patients. J Am Soc Nephrol (2002) 13 Suppl 1:S28–36. doi: 10.1681/ASN.V13suppl_1s28
161. Kalantar-Zadeh K, Ikizler TA, Block G, Avram MM, Kopple JD. Malnutrition-inflammation complex syndrome in dialysis patients: causes and consequences. Am J Kidney Dis (2003) 42(5):864–81. doi: 10.1016/j.ajkd.2003.07.016
162. Ramkumar N, Pappas LM, Beddhu S. Effect of body size and body composition on survival in peritoneal dialysis patients. Perit Dial Int (2005) 25(5):461–9. doi: 10.1177/089686080502500510
163. Ritz E, Wanner C. Lipid abnorMalities and cardiovascular risk in renal disease. J Am Soc Nephrol (2008) 19(6):1065–70. doi: 10.1681/asn.2007101128
164. Lowrie EG, Lew NL. Death risk in hemodialysis patients: the predictive value of commonly measured variables and an evaluation of death rate differences between facilities. Am J Kidney Dis (1990) 15(5):458–82. doi: 10.1016/s0272-6386(12)70364-5
165. Cheung AK, Sarnak MJ, Yan G, Dwyer JT, Heyka RJ, Rocco MV, et al. Atherosclerotic cardiovascular disease risks in chronic hemodialysis patients. Kidney Int (2000) 58(1):353–62. doi: 10.1046/j.1523-1755.2000.00173.x
166. Iseki K, Yamazato M, Tozawa M, Takishita S. Hypocholesterolemia is a significant predictor of death in a cohort of chronic hemodialysis patients. Kidney Int (2002) 61(5):1887–93. doi: 10.1046/j.1523-1755.2002.00324.x
167. Liu Y, Coresh J, Eustace JA, Longenecker JC, Jaar B, Fink NE, et al. Association between cholesterol level and mortality in dialysis patients: role of inflammation and malnutrition. Jama (2004) 291(4):451–9. doi: 10.1001/jama.291.4.451
168. Fathi R, Isbel N, Short L, Haluska B, Johnson D, Marwick TH. The effect of long-term aggressive lipid lowering on ischemic and atherosclerotic burden in patients with chronic kidney disease. Am J Kidney Dis (2004) 43(1):45–52. doi: 10.1053/j.ajkd.2003.09.012
169. Qunibi WY. Dyslipidemia in dialysis patients. Semin Dialysis (2015) 28(4):345–53. doi: 10.1111/sdi.12375
170. Mach F, Baigent C, Catapano AL, Koskinas KC, Casula M, Badimon L, et al. 2019 ESC/EAS Guidelines for the management of dyslipidaemias: lipid modification to reduce cardiovascular risk: The Task Force for the management of dyslipidaemias of the European Society of Cardiology (ESC) and European Atherosclerosis Society (EAS). Eur Heart J (2019) 41(1):111–88. doi: 10.1093/eurheartj/ehz455
171. Cebrian A, Escobar C, Aranda U, Palacios B, Capel M, Sicras A, et al. The 2021 European Society of Cardiology Cardiovascular Disease Prevention Guidelines: adding albuminuria to the SCORE scale increases the prevalence of very high/high cardiovascular risk among patients with chronic kidney disease. Clin Kidney J (2022) 15(6):1204–8. doi: 10.1093/ckj/sfac019
172. Smoking-attributable mortality, years of potential life lost, and productivity losses–United States, 2000-2004. MMWR Morb Mortal Wkly Rep (2008) 57(45):1226–8.
173. Longenecker JC, Coresh J, Klag MJ, Levey AS, Martin AA, Fink NE, et al. Validation of comorbid conditions on the end-stage renal disease medical evidence report: the CHOICE study. Choices for Healthy Outcomes in Caring for ESRD. J Am Soc Nephrol (2000) 11(3):520–9. doi: 10.1681/asn.v113520
174. Liebman SE, Lamontagne SP, Huang LS, Messing S, Bushinsky DA. Smoking in dialysis patients: a systematic review and meta-analysis of mortality and cardiovascular morbidity. Am J Kidney Dis (2011) 58(2):257–65. doi: 10.1053/j.ajkd.2011.03.025
175. Akchurin OM, Kaskel F. Update on inflammation in chronic kidney disease. Blood Purif (2015) 39(1-3):84–92. doi: 10.1159/000368940
176. Gupta J, Mitra N, Kanetsky PA, Devaney J, Wing MR, Reilly M, et al. Association between albuminuria, kidney function, and inflammatory biomarker profile in CKD in CRIC. Clin J Am Soc Nephrol (2012) 7(12):1938–46. doi: 10.2215/cjn.03500412
177. Honda H, Qureshi AR, Heimbürger O, Barany P, Wang K, Pecoits-Filho R, et al. Serum albumin, C-reactive protein, interleukin 6, and fetuin a as predictors of malnutrition, cardiovascular disease, and mortality in patients with ESRD. Am J Kidney Dis (2006) 47(1):139–48. doi: 10.1053/j.ajkd.2005.09.014
178. Tripepi G, Mallamaci F, Zoccali C. Inflammation markers, adhesion molecules, and all-cause and cardiovascular mortality in patients with ESRD: searching for the best risk marker by multivariate modeling. J Am Soc Nephrol (2005) 16 Suppl 1:S83–8. doi: 10.1681/asn.2004110972
179. Shafi T, Hostetter TH, Meyer TW, Hwang S, Hai X, Melamed ML, et al. Serum asymmetric and symmetric dimethylarginine and morbidity and mortality in hemodialysis patients. Am J Kidney Dis (2017) 70(1):48–58. doi: 10.1053/j.ajkd.2016.10.033
180. Schlesinger S, Sonntag SR, Lieb W, Maas R. Asymmetric and symmetric dimethylarginine as risk markers for total mortality and cardiovascular outcomes: A systematic review and meta-analysis of prospective studies. PloS One (2016) 11(11):e0165811. doi: 10.1371/journal.pone.0165811
181. Pedraza-Chaverri J, Sánchez-Lozada LG, Osorio-Alonso H, Tapia E, Scholze A. New pathogenic concepts and therapeutic approaches to oxidative stress in chronic kidney disease. Oxid Med Cell Longev (2016) 2016:6043601. doi: 10.1155/2016/6043601
182. Emrich IE, Zawada AM, Martens-Lobenhoffer J, Fliser D, Wagenpfeil S, Heine GH, et al. Symmetric dimethylarginine (SDMA) outperforms asymmetric dimethylarginine (ADMA) and other methylarginines as predictor of renal and cardiovascular outcome in non-dialysis chronic kidney disease. Clin Res Cardiol (2018) 107(3):201–13. doi: 10.1007/s00392-017-1172-4
183. Busch M, Fleck C, Wolf G, Stein G. Asymmetrical (ADMA) and symmetrical dimethylarginine (SDMA) as potential risk factors for cardiovascular and renal outcome in chronic kidney disease - possible candidates for paradoxical epidemiology? Amino Acids (2006) 30(3):225–32. doi: 10.1007/s00726-005-0268-8
184. Tripepi G, Mattace Raso F, Sijbrands E, Seck MS, Maas R, Boger R, et al. Inflammation and asymmetric dimethylarginine for predicting death and cardiovascular events in ESRD patients. Clin J Am Soc Nephrol (2011) 6(7):1714–21. doi: 10.2215/cjn.11291210
185. Drew DA, Tighiouart H, Scott T, Kantor A, Fan L, Artusi C, et al. Asymmetric dimethylarginine, race, and mortality in hemodialysis patients. Clin J Am Soc Nephrol (2014) 9(8):1426–33. doi: 10.2215/cjn.00770114
186. Aucella F, Maas R, Vigilante M, Tripepi G, Schwedhelm E, Margaglione M, et al. Methylarginines and mortality in patients with end stage renal disease: a prospective cohort study. Atherosclerosis (2009) 207(2):541–5. doi: 10.1016/j.atherosclerosis.2009.05.011
187. Vanholder R, Baurmeister U, Brunet P, Cohen G, Glorieux G, Jankowski J. A bench to bedside view of uremic toxins. J Am Soc Nephrol (2008) 19(5):863–70. doi: 10.1681/asn.2007121377
188. Moradi H, Sica DA, Kalantar-Zadeh K. Cardiovascular burden associated with uremic toxins in patients with chronic kidney disease. Am J Nephrol (2013) 38(2):136–48. doi: 10.1159/000351758
189. Vanholder R, De Smet R, Glorieux G, Argilés A, Baurmeister U, Brunet P, et al. Review on uremic toxins: classification, concentration, and interindividual variability. Kidney Int (2003) 63(5):1934–43. doi: 10.1046/j.1523-1755.2003.00924.x
190. Liabeuf S, Barreto DV, Barreto FC, Meert N, Glorieux G, Schepers E, et al. Free p-cresylsulphate is a predictor of mortality in patients at different stages of chronic kidney disease. Nephrol Dial Transplant (2010) 25(4):1183–91. doi: 10.1093/ndt/gfp592
191. Ito S, Osaka M, Higuchi Y, Nishijima F, Ishii H, Yoshida M. Indoxyl sulfate induces leukocyte-endothelial interactions through up-regulation of E-selectin. J Biol Chem (2010) 285(50):38869–75. doi: 10.1074/jbc.M110.166686
192. Schepers E, Glorieux G, Dou L, Cerini C, Gayrard N, Louvet L, et al. Guanidino compounds as cause of cardiovascular damage in chronic kidney disease: an in vitro evaluation. Blood Purif (2010) 30(4):277–87. doi: 10.1159/000320765
193. Moe SM, Chen NX. Pathophysiology of vascular calcification in chronic kidney disease. Circ Res (2004) 95(6):560–7. doi: 10.1161/01.res.0000141775.67189.98
194. Bonomini M, Dottori S, Amoroso L, Arduini A, Sirolli V. Increased platelet phosphatidylserine exposure and caspase activation in chronic uremia. J Thromb Haemost (2004) 2(8):1275–81. doi: 10.1111/j.1538-7836.2004.00837.x
195. Meijers BK, Bammens B, De Moor B, Verbeke K, Vanrenterghem Y, Evenepoel P. Free p-cresol is associated with cardiovascular disease in hemodialysis patients. Kidney Int (2008) 73(10):1174–80. doi: 10.1038/ki.2008.31
196. Meijers BK, Claes K, Bammens B, de Loor H, Viaene L, Verbeke K, et al. p-Cresol and cardiovascular risk in mild-to-moderate kidney disease. Clin J Am Soc Nephrol (2010) 5(7):1182–9. doi: 10.2215/cjn.07971109
198. Barisione C, Ghigliotti G, Canepa M, Balbi M, Brunelli C, Ameri P. Indoxyl sulfate: a candidate target for the prevention and treatment of cardiovascular disease in chronic kidney disease. Curr Drug Targets (2015) 16(4):366–72. doi: 10.2174/1389450116666141230114500
199. Shivanna S, Kolandaivelu K, Shashar M, Belghasim M, Al-Rabadi L, Balcells M, et al. The aryl hydrocarbon receptor is a critical regulator of tissue factor stability and an antithrombotic target in uremia. J Am Soc Nephrol (2016) 27(1):189–201. doi: 10.1681/asn.2014121241
200. Chitalia VC, Shivanna S, Martorell J, Balcells M, Bosch I, Kolandaivelu K, et al. Uremic serum and solutes increase post-vascular interventional thrombotic risk through altered stability of smooth muscle cell tissue factor. Circulation (2013) 127(3):365–76. doi: 10.1161/circulationaha.112.118174
201. Kamiński TW, Pawlak K, Karbowska M, Myśliwiec M, Pawlak D. Indoxyl sulfate – the uremic toxin linking hemostatic system disturbances with the prevalence of cardiovascular disease in patients with chronic kidney disease. Antioxidants (Basel) (2021) 10(6):936. doi: 10.3390/antiox10060936
202. Locatelli F, Canaud B, Eckardt KU, Stenvinkel P, Wanner C, Zoccali C. Oxidative stress in end-stage renal disease: an emerging threat to patient outcome. Nephrol Dial Transplant (2003) 18(7):1272–80. doi: 10.1093/ndt/gfg074
203. Pavlakou P, Liakopoulos V, Eleftheriadis T, Mitsis M, Dounousi E. Oxidative stress and acute kidney injury in critical illness: pathophysiologic mechanisms-biomarkers-interventions, and future perspectives. Oxid Med Cell Longev (2017) 2017:6193694. doi: 10.1155/2017/6193694
204. Duni A, Liakopoulos V, RapsOmanikis KP, Dounousi E. Chronic kidney disease and disproportionally increased cardiovascular damage: does oxidative stress explain the burden? Oxid Med Cell Longev (2017) 2017:9036450. doi: 10.1155/2017/9036450
205. Liakopoulos V, Roumeliotis S, Gorny X, Eleftheriadis T, Mertens PR. Oxidative stress in patients undergoing peritoneal dialysis: A current review of the literature. Oxid Med Cell Longev (2017) 2017:3494867. doi: 10.1155/2017/3494867
206. Liakopoulos V, Roumeliotis S, Gorny X, Dounousi E, Mertens PR. Oxidative stress in hemodialysis patients: A review of the literature. Oxid Med Cell Longev (2017) 2017:3081856. doi: 10.1155/2017/3081856
207. Cozzolino M, Galassi A, Pivari F, Ciceri P, Conte F. The cardiovascular burden in end-stage renal disease. Contrib Nephrol (2017) 191:44–57. doi: 10.1159/000479250
208. Cozzolino M, Mazzaferro S. The fibroblast growth factor 23: a new player in the field of cardiovascular, bone and renal disease. Curr Vasc Pharmacol (2010) 8(3):404–11. doi: 10.2174/157016110791112313
209. Vervloet MG, Sezer S, Massy ZA, Johansson L, Cozzolino M, Fouque D. The role of phosphate in kidney disease. Nat Rev Nephrol (2017) 13(1):27–38. doi: 10.1038/nrneph.2016.164
210. Vervloet M, Cozzolino M. Vascular calcification in chronic kidney disease: different bricks in the wall? Kidney Int (2017) 91(4):808–17. doi: 10.1016/j.kint.2016.09.024
211. Bover J, Evenepoel P, Ureña-Torres P, Vervloet MG, Brandenburg V, Mazzaferro S, et al. Pro: cardiovascular calcifications are clinically relevant. Nephrol Dial Transplant (2015) 30(3):345–51. doi: 10.1093/ndt/gfv020
212. Ketteler M, Block GA, Evenepoel P, Fukagawa M, Herzog CA, McCann L, et al. Executive summary of the 2017 KDIGO Chronic Kidney Disease-Mineral and Bone Disorder (CKD-MBD) Guideline Update: what’s changed and why it matters. Kidney Int (2017) 92(1):26–36. doi: 10.1016/j.kint.2017.04.006
213. Vashistha T, Kalantar-Zadeh K, Molnar MZ, Torlén K, Mehrotra R. Dialysis modality and correction of uremic metabolic acidosis: relationship with all-cause and cause-specific mortality. Clin J Am Soc Nephrol (2013) 8(2):254–64. doi: 10.2215/cjn.05780612
214. Raikou VD, Kyriaki D. Association between low serum bicarbonate concentrations and cardiovascular disease in patients in the end-stage of renal disease. Diseases (2016) 4(4). doi: 10.3390/diseases4040036
215. Paniagua R, Ventura MD, Avila-Díaz M, Hinojosa-Heredia H, Méndez-Durán A, Cueto-Manzano A, et al. NT-proBNP, fluid volume overload and dialysis modality are independent predictors of mortality in ESRD patients. Nephrol Dial Transplant (2010) 25(2):551–7. doi: 10.1093/ndt/gfp395
216. Wizemann V, Wabel P, Chamney P, Zaluska W, Moissl U, Rode C, et al. The mortality risk of overhydration in haemodialysis patients. Nephrol Dial Transplant (2009) 24(5):1574–9. doi: 10.1093/ndt/gfn707
217. Guo Q, Lin J, Li J, Yi C, Mao H, Yang X, et al. The effect of fluid overload on clinical outcome in southern chinese patients undergoing continuous ambulatory peritoneal dialysis. Perit Dial Int (2015) 35(7):691–702. doi: 10.3747/pdi.2014.00008
218. Zoccali C, Moissl U, Chazot C, Mallamaci F, Tripepi G, Arkossy O, et al. Chronic fluid overload and mortality in ESRD. J Am Soc Nephrol (2017) 28(8):2491–7. doi: 10.1681/asn.2016121341
219. Assimon MM, Wenger JB, Wang L, Flythe JE. Ultrafiltration rate and mortality in maintenance hemodialysis patients. Am J Kidney Dis (2016) 68(6):911–22. doi: 10.1053/j.ajkd.2016.06.020
220. Wu CC, Zheng CM, Lin YF, Lo L, Liao MT, Lu KC. Role of homocysteine in end-stage renal disease. Clin Biochem (2012) 45(16-17):1286–94. doi: 10.1016/j.clinbiochem.2012.05.031
221. Bostom AG, Carpenter MA, Kusek JW, Levey AS, Hunsicker L, Pfeffer MA, et al. Homocysteine-lowering and cardiovascular disease outcomes in kidney transplant recipients: primary results from the Folic Acid for Vascular Outcome Reduction in Transplantation trial. Circulation (2011) 123(16):1763–70. doi: 10.1161/circulationaha.110.000588
222. Jardine MJ, Kang A, Zoungas S, Navaneethan SD, Ninomiya T, Nigwekar SU, et al. The effect of folic acid based homocysteine lowering on cardiovascular events in people with kidney disease: systematic review and meta-analysis. Bmj (2012) 344:e3533. doi: 10.1136/bmj.e3533
223. Qin X, Huo Y, Langman CB, Hou F, Chen Y, Matossian D, et al. Folic acid therapy and cardiovascular disease in ESRD or advanced chronic kidney disease: a meta-analysis. Clin J Am Soc Nephrol (2011) 6(3):482–8. doi: 10.2215/cjn.05310610
224. Nigwekar SU, Kang A, Zoungas S, Cass A, Gallagher MP, Kulshrestha S, et al. Interventions for lowering plasma homocysteine levels in dialysis patients. Cochrane Database Syst Rev (2016) 2016(5):Cd004683. doi: 10.1002/14651858.CD004683.pub4
225. K/DOQI clinical practice guidelines for cardiovascular disease in dialysis patients. Am J Kidney Dis (2005) 45(4 Suppl 3):S1–153.
226. Kim SJ, Oh HJ, Yoo DE, Shin DH, Lee MJ, Kim HR, et al. Electrocardiographic left ventricular hypertrophy and outcome in hemodialysis patients. PloS One (2012) 7(4):e35534. doi: 10.1371/journal.pone.0035534
227. Harnett JD, Murphy B, Collingwood P, Purchase L, Kent G, Parfrey PS. The reliability and validity of echocardiographic measurement of left ventricular mass index in hemodialysis patients. Nephron (1993) 65(2):212–4. doi: 10.1159/000187476
228. Kahn MR, Fallahi A, Kim MC, Esquitin R, Robbins MJ. Coronary artery disease in a large renal transplant population: implications for management. Am J Transplant (2011) 11(12):2665–74. doi: 10.1111/j.1600-6143.2011.03734.x
229. Tentori F, Elder SJ, Thumma J, Pisoni RL, Bommer J, Fissell RB, et al. Physical exercise among participants in the Dialysis Outcomes and Practice Patterns Study (DOPPS): correlates and associated outcomes. Nephrol Dial Transplant (2010) 25(9):3050–62. doi: 10.1093/ndt/gfq138
230. McLaurin MD, Apple FS, Voss EM, Herzog CA, Sharkey SW. Cardiac troponin I, cardiac troponin T, and creatine kinase MB in dialysis patients without ischemic heart disease: evidence of cardiac troponin T expression in skeletal muscle. Clin Chem (1997) 43(6 Pt 1):976–82. doi: 10.1093/clinchem/43.6.976
231. deFilippi C, Seliger SL, Kelley W, Duh SH, Hise M, Christenson RH, et al. Interpreting cardiac troponin results from high-sensitivity assays in chronic kidney disease without acute coronary syndrome. Clin Chem (2012) 58(9):1342–51. doi: 10.1373/clinchem.2012.185322
232. Gunsolus I, Sandoval Y, Smith SW, Sexter A, Schulz K, Herzog CA, et al. Renal dysfunction influences the diagnostic and prognostic performance of high-sensitivity cardiac troponin I. J Am Soc Nephrol (2018) 29(2):636–43. doi: 10.1681/asn.2017030341
233. Miller-Hodges E, Anand A, Shah ASV, Chapman AR, Gallacher P, Lee KK, et al. High-sensitivity cardiac troponin and the risk stratification of patients with renal impairment presenting with suspected acute coronary syndrome. Circulation (2018) 137(5):425–35. doi: 10.1161/circulationaha.117.030320
234. Sharma R, Pellerin D, Gaze DC, Gregson H, Streather CP, Collinson PO, et al. Dobutamine stress echocardiography and the resting but not exercise electrocardiograph predict severe coronary artery disease in renal transplant candidates. Nephrol Dial Transplant (2005) 20(10):2207–14. doi: 10.1093/ndt/gfi005
235. Poli FE, Gulsin GS, McCann GP, Burton JO, Graham-Brown MP. The assessment of coronary artery disease in patients with end-stage renal disease. Clin Kidney J (2019) 12(5):721–34. doi: 10.1093/ckj/sfz088
236. Ohtake T, Ishioka K, Honda K, Oka M, Maesato K, Mano T, et al. Impact of coronary artery calcification in hemodialysis patients: Risk factors and associations with prognosis. Hemodial Int (2010) 14(2):218–25. doi: 10.1111/j.1542-4758.2009.00423.x
237. Matsuoka M, Iseki K, Tamashiro M, Fujimoto N, Higa N, Touma T, et al. Impact of high coronary artery calcification score (CACS) on survival in patients on chronic hemodialysis. Clin Exp Nephrol (2004) 8(1):54–8. doi: 10.1007/s10157-003-0260-0
238. Shantouf RS, Budoff MJ, Ahmadi N, Ghaffari A, Flores F, Gopal A, et al. Total and individual coronary artery calcium scores as independent predictors of mortality in hemodialysis patients. Am J Nephrol (2010) 31(5):419–25. doi: 10.1159/000294405
239. Haydar AA, Hujairi NM, Covic AA, Pereira D, Rubens M, Goldsmith DJ. Coronary artery calcification is related to coronary atherosclerosis in chronic renal disease patients: a study comparing EBCT-generated coronary artery calcium scores and coronary angiography. Nephrol Dial Transplant (2004) 19(9):2307–12. doi: 10.1093/ndt/gfh120
240. Mao J, Karthikeyan V, Poopat C, Song T, Pantelic M, Chattahi J, et al. Coronary computed tomography angiography in dialysis patients undergoing pre-renal transplantation cardiac risk stratification. Cardiol J (2010) 17(4):349–61.
241. Simonsen JA, Mickley H, Johansen A, Hess S, Thomassen A, Gerke O, et al. Outcome of revascularisation in stable coronary artery disease without ischaemia: a Danish registry-based follow-up study. BMJ Open (2017) 7(8):e016169. doi: 10.1136/bmjopen-2017-016169
242. Wang LW, Fahim MA, Hayen A, Mitchell RL, Baines L, Lord S, et al. Cardiac testing for coronary artery disease in potential kidney transplant recipients. Cochrane Database Syst Rev (2011) 2011(12):Cd008691. doi: 10.1002/14651858.CD008691.pub2
243. Patel MR, Calhoon JH, Dehmer GJ, Grantham JA, Maddox TM, Maron DJ, et al. ACC/AATS/AHA/ASE/ASNC/SCAI/SCCT/STS 2017 appropriate use criteria for coronary revascularization in patients with stable ischemic heart disease: A report of the american college of cardiology appropriate use criteria task force, american association for thoracic surgery, american heart association, american society of echocardiography, american society of nuclear cardiology, society for cardiovascular angiography and interventions, society of cardiovascular computed tomography, and society of thoracic surgeons. J Am Coll Cardiol (2017) 69(17):2212–41. doi: 10.1016/j.jacc.2017.02.001
244. Hirose K, Chikamori T, Hida S, Tanaka N, Yamashita J, Igarashi Y, et al. Application of pressure-derived myocardial fractional flow reserve in chronic hemodialysis patients. J Cardiol (2018) 71(1):52–8. doi: 10.1016/j.jjcc.2017.05.007
245. Matsuo A, Fujita H, Ueoka A, Maruyama N, Shimoda Y, Kishita E, et al. Importance of measuring the fractional flow reserve in patients receiving hemodialysis. Cardiovasc Interv Ther (2011) 26(3):215–21. doi: 10.1007/s12928-011-0061-4
246. Marwick TH, Amann K, Bangalore S, Cavalcante JL, Charytan DM, Craig JC, et al. Chronic kidney disease and valvular heart disease: conclusions from a Kidney Disease: Improving Global Outcomes (KDIGO) Controversies Conference. Kidney Int (2019) 96(4):836–49. doi: 10.1016/j.kint.2019.06.025
247. KDIGO 2017 clinical practice guideline update for the diagnosis, evaluation, prevention, and treatment of chronic kidney disease-mineral and bone disorder (CKD-MBD). Kidney Int Suppl (2017) 7(1):1–59. doi: 10.1016/j.kisu.2017.04.001
248. Matsuo H, Dohi K, Machida H, Takeuchi H, Aoki T, Nishimura H, et al. Echocardiographic assessment of cardiac structural and functional abnorMalities in patients with end-stage renal disease receiving chronic hemodialysis. Circ J Jan 25 (2018) 82(2):586–95. doi: 10.1253/circj.CJ-17-0393
249. Takada T, Jujo K, Konami Y, Otsuki H, Tanaka K, Saito C, et al. Impact of Transcatheter Aortic Valve Implantation on Kidney Function. Arq Bras Cardiol (2019) 113(6):1104–11. doi: 10.36660/abc.20180356
250. Brandenburg VM, Schuh A, Kramann R. Valvular calcification in chronic kidney disease. Adv Chronic Kidney Dis (2019) 26(6):464–71. doi: 10.1053/j.ackd.2019.10.004
251. Dohi K. Echocardiographic assessment of cardiac structure and function in chronic renal disease. J Echocardiogr (2019) 17(3):115–22. doi: 10.1007/s12574-019-00436-x
252. Baumgartner H, Falk V, Bax JJ, De Bonis M, Hamm C, Holm PJ, et al. 2017 ESC/EACTS guidelines for the management of valvular heart disease. Rev Esp Cardiol (Engl Ed) (2018) 71(2):110. doi: 10.1016/j.rec.2017.12.013
253. Poulikakos D, Malik M. Challenges of ECG monitoring and ECG interpretation in dialysis units. J Electrocardiol (2016) 49(6):855–9. doi: 10.1016/j.jelectrocard.2016.07.019
254. Tezcan UK, Amasyali B, Can I, Aytemir K, Köse S, Yavuz I, et al. Increased P wave dispersion and maximum P wave duration after hemodialysis. Ann Noninvasive Electrocardiol (2004) 9(1):34–8. doi: 10.1111/j.1542-474x.2004.91529.x
255. Berta E, Erdei A, Cseke B, Gazdag A, Paragh G, Balla J, et al. Evaluation of the metabolic changes during hemodialysis by signal averaged ECG. Pharmazie (2012) 67(5):380–3.
256. Poulikakos D, Hnatkova K, Skampardoni S, Green D, Kalra P, Malik M. Sudden cardiac death in dialysis: arrhythmic mechanisms and the value of non-invasive electrophysiology. Front Physiol (2019) 10:144. doi: 10.3389/fphys.2019.00144
257. Hayano J, Takahashi H, Toriyama T, Mukai S, Okada A, Sakata S, et al. Prognostic value of heart rate variability during long-term follow-up in chronic haemodialysis patients with end-stage renal disease. Nephrol Dial Transplant (1999) 14(6):1480–8. doi: 10.1093/ndt/14.6.1480
258. Fukuta H, Hayano J, Ishihara S, Sakata S, Mukai S, Ohte N, et al. Prognostic value of heart rate variability in patients with end-stage renal disease on chronic haemodialysis. Nephrol Dial Transplant (2003) 18(2):318–25. doi: 10.1093/ndt/18.2.318
259. Oikawa K, Ishihara R, Maeda T, Yamaguchi K, Koike A, Kawaguchi H, et al. Prognostic value of heart rate variability in patients with renal failure on hemodialysis. Int J Cardiol (2009) 131(3):370–7. doi: 10.1016/j.ijcard.2007.10.033
260. Chen SC, Huang JC, Tsai YC, Hsiu-Chin Mai RN, Jui-Hsin Chen RN, Kuo PL, et al. Heart rate variability change before and after hemodialysis is associated with overall and cardiovascular mortality in hemodialysis. Sci Rep (2016) 6:20597. doi: 10.1038/srep20597
261. Huang YT, Chang YM, Chen IL, Yang CL, Leu SC, Su HL, et al. Heart rate variability during hemodialysis is an indicator for long-term vascular access survival in uremic patients. PloS One (2017) 12(3):e0172212. doi: 10.1371/journal.pone.0172212
262. Sacher F, Jesel L, Borni-Duval C, De Precigout V, Lavainne F, Bourdenx JP, et al. Cardiac rhythm disturbances in hemodialysis patients: early detection using an implantable loop recorder and correlation with biological and dialysis parameters. JACC Clin Electrophysiol (2018) 4(3):397–408. doi: 10.1016/j.jacep.2017.08.002
263. Wong MCG, Kalman JM, Pedagogos E, Toussaint N, Vohra JK, Sparks PB, et al. Bradycardia and asystole is the predominant mechanism of sudden cardiac death in patients with chronic kidney disease. J Am Coll Cardiol (2015) 65(12):1263–5. doi: 10.1016/j.jacc.2014.12.049
264. Liu YW, Su CT, Song EJ, Tsai WC, Li YH, Tsai LM, et al. The role of echocardiographic study in patients with chronic kidney disease. J Formos Med Assoc (2015) 114(9):797–805. doi: 10.1016/j.jfma.2015.06.009
265. Arnett DK, Blumenthal RS, Albert MA, Buroker AB, Goldberger ZD, Hahn EJ, et al. 2019 ACC/AHA guideline on the primary prevention of cardiovascular disease: executive summary: A report of the american college of cardiology/american heart association task force on clinical practice guidelines. Circulation (2019) 140(11):e563–95. doi: 10.1161/CIR.0000000000000677
266. Jain N, McAdams M, Hedayati SS. Screening for cardiovascular disease in CKD: PRO. Kidney360 (2022) 3(11):1831–5. doi: 10.34067/kid.0005012021
267. Snaedal S, Bárány P, Lund SH, Qureshi AR, Heimbürger O, Stenvinkel P, et al. High-sensitivity troponins in dialysis patients: variation and prognostic value. Clin Kidney J (2021) 14(7):1789–97. doi: 10.1093/ckj/sfaa215
268. Apple FS, Murakami MM, Pearce LA, Herzog CA. Predictive value of cardiac troponin I and T for subsequent death in end-stage renal disease. Circulation (2002) 106(23):2941–5. doi: 10.1161/01.cir.0000041254.30637.34
269. Mingels AM, Cardinaels EP, Broers NJ, van Sleeuwen A, Streng AS, van Dieijen-Visser MP, et al. Cardiac Troponin T: Smaller Molecules in Patients with End-Stage Renal Disease than after Onset of Acute Myocardial Infarction. Clin Chem (2017) 63(3):683–90. doi: 10.1373/clinchem.2016.261644
270. Kanderian AS, Francis GS. Cardiac troponins and chronic kidney disease. Kidney Int (2006) 69(7):1112–4. doi: 10.1038/sj.ki.5000174
271. Diris JH, Hackeng CM, KoOman JP, Pinto YM, Hermens WT, van Dieijen-Visser MP. Impaired renal clearance explains elevated troponin T fragments in hemodialysis patients. Circulation (2004) 109(1):23–5. doi: 10.1161/01.cir.0000109483.45211.8f
272. Freda BJ, Tang WH, Van Lente F, Peacock WF, Francis GS. Cardiac troponins in renal insufficiency: review and clinical implications. J Am Coll Cardiol (2002) 40(12):2065–71. doi: 10.1016/s0735-1097(02)02608-6
273. Francis GS, Tang WH. Cardiac troponins in renal insufficiency and other non-ischemic cardiac conditions. Prog Cardiovasc Dis (2004) 47(3):196–206. doi: 10.1016/j.pcad.2004.07.005
274. Mallamaci F, Zoccali C, Parlongo S, Tripepi G, Benedetto FA, Cutrupi S, et al. Troponin is related to left ventricular mass and predicts all-cause and cardiovascular mortality in hemodialysis patients. Am J Kidney Dis (2002) 40(1):68–75. doi: 10.1053/ajkd.2002.33914
275. Januzzi JL Jr., Filippatos G, Nieminen M, Gheorghiade M. Troponin elevation in patients with heart failure: on behalf of the third Universal Definition of Myocardial Infarction Global Task Force: Heart Failure Section. Eur Heart J (2012) 33(18):2265–71. doi: 10.1093/eurheartj/ehs191
276. Al-Hweish A, Sultan SS, Mogazi K, Elsammak MY. Plasma myeloperoxidase, NT-proBNP, and troponin-I in patients on CAPD compared with those on regular hemodialysis. Hemodial Int (2010) 14(3):308–15. doi: 10.1111/j.1542-4758.2010.00455.x
277. Twerenbold R, Badertscher P, Boeddinghaus J, Nestelberger T, Wildi K, Puelacher C, et al. 0/1-hour triage algorithm for myocardial infarction in patients with renal dysfunction. Circulation (2018) 137(5):436–51. doi: 10.1161/circulationaha.117.028901
278. Stacy SR, Suarez-Cuervo C, Berger Z, Wilson LM, Yeh HC, Bass EB, et al. Role of troponin in patients with chronic kidney disease and suspected acute coronary syndrome: a systematic review. Ann Intern Med (2014) 161(7):502–12. doi: 10.7326/m14-0746
279. Thygesen K, Alpert JS, Jaffe AS, Chaitman BR, Bax JJ, Morrow DA, et al. Fourth universal definition of myocardial infarction (2018). J Am Coll Cardiol (2018) 72(18):2231–64. doi: 10.1016/j.jacc.2018.08.1038
280. Mahmood U, Johnson DW, Fahim MA. Cardiac biomarkers in dialysis. AIMS Genet (2017) 4(1):1–20. doi: 10.3934/genet.2017.1.1
281. Cheng YJ, Yao FJ, Liu LJ, Tang K, Lin XX, Li WJ, et al. B-type natriuretic peptide and prognosis of end-stage renal disease: a meta-analysis. PloS One (2013) 8(11):e79302. doi: 10.1371/journal.pone.0079302
282. Ibrahim NE, Januzzi JL. Established and emerging roles of biomarkers in heart failure. Circ Res (2018) 123(5):614–29. doi: 10.1161/CIRCRESAHA.118.312706
283. Hogas S, Schiller A, Voroneanu L, Constantinescu D, Timar R, Cianga P, et al. Predictive value for galectin 3 and cardiotrophin 1 in hemodialysis patients. Angiology (2016) 67(9):854–9. doi: 10.1177/0003319715623397
284. Bernier GM. beta 2-Microglobulin: structure, function and significance. Vox Sang (1980) 38(6):323–7. doi: 10.1111/j.1423-0410.1980.tb04500.x
285. Matsushita K, Sang Y, Ballew SH, Astor BC, Hoogeveen RC, Solomon SD, et al. Cardiac and kidney markers for cardiovascular prediction in individuals with chronic kidney disease. Arteriosclerosis Thrombosis Vasc Biol (2014) 34(8):1770–7. doi: 10.1161/atvbaha.114.303465
286. Dollery CM, Mcewan JR, Henney AM. Matrix metalloproteinases and cardiovascular disease. Circ Res (1995) 77(5):863–8. doi: 10.1161/01.res.77.5.863
Keywords: chronic kidney disease, hemodialysis, left ventricular hypertrophy, uremic toxins, beta 2-microglobulin, galectin-3, suppression of tumorigenicity-2 (ST2)
Citation: Echefu G, Stowe I, Burka S, Basu-Ray I and Kumbala D (2023) Pathophysiological concepts and screening of cardiovascular disease in dialysis patients. Front. Nephrol. 3:1198560. doi: 10.3389/fneph.2023.1198560
Received: 01 April 2023; Accepted: 10 August 2023;
Published: 29 September 2023.
Edited by:
Marijke Dekker, Maasstad Ziekenhuis, NetherlandsReviewed by:
Yuri Battaglia, University of Verona, ItalyKhaled Mohamed Amin Elzorkany, King Faisal University, Saudi Arabia
Copyright © 2023 Echefu, Stowe, Burka, Basu-Ray and Kumbala. This is an open-access article distributed under the terms of the Creative Commons Attribution License (CC BY). The use, distribution or reproduction in other forums is permitted, provided the original author(s) and the copyright owner(s) are credited and that the original publication in this journal is cited, in accordance with accepted academic practice. No use, distribution or reproduction is permitted which does not comply with these terms.
*Correspondence: Gift Echefu, Z2VjaGVmdUB1dGhzYy5lZHU=