- 1School of Medicine, Shanghai University, Shanghai, China
- 2Division of Nephrology, Shanghai Changzheng Hospital, Second Military Medical University, Shanghai, China
Autosomal dominant polycystic kidney disease (ADPKD), the most common monogenic hereditary kidney disease, is the fourth leading cause of end-stage kidney disease worldwide. In recent years, significant progress has been made in delaying ADPKD progression with different kinds of chemical drugs, such as tolvaptan, rapamycin, and somatostatin. Meanwhile, numerous plant-derived compounds have been investigated for their beneficial effects on slowing ADPKD progression. Among them, saikosaponin-d, Ganoderma triterpenes, curcumin, ginkgolide B, steviol, resveratrol, Sparganum stoloniferum Buch.-Ham, Cordyceps sinensis, triptolide, quercitrin, naringin, cardamonin, gambogic acid, and olive leaf extract have been found to retard renal cyst development by inhibiting cell proliferation or promoting cell apoptosis in renal cyst-lining epithelial cells. Metformin, a synthesized compound derived from French lilac or goat’s rue (Galega officinalis), has been proven to retard the progression of ADPKD. This review focuses on the roles and mechanisms of plant-derived compounds in treating ADPKD, which may constitute promising new therapeutics in the future.
1 Introduction
Autosomal dominant polycystic kidney disease (ADPKD) is the most common monogenic hereditary kidney disease and the fourth leading cause of end-stage kidney disease (ESKD) (1). It affects approximately 6 million people worldwide, and approximately 50% of patients develop ESKD after 60 years of age (2). ADPKD often occurs in adults and is characterized by the development of cysts in both kidneys and an increase in total kidney volume (TKV), leading to the destruction of kidney tissue and the eventual development of renal failure, and sufferers can only be sustained by dialysis or kidney transplantation (3). Furthermore, ADPKD is a systemic disease that can cause liver cysts, pancreatic cysts, and intracranial aneurysms in addition to kidney disease, posing a severe risk to human life and health (4). Therefore, it is of great clinical significance to discover new drugs to delay the development of ADPKD.
The pathogenesis of ADPKD involves complex pathophysiological changes; mutations of the PKD1 gene encoding polycystic protein 1 (PC1) and the PKD2 gene encoding polycystic protein 2 (PC2) are the leading causes of ADPKD (5). In addition, ciliary dysfunction, non-antagonistic proliferation of renal tubular cells, impaired polarity of polarized and planar cells, disorder of intracellular Ca2+ levels, and abnormalities of cyclic adenosine monophosphate (cAMP), the mammalian target of rapamycin (mTOR), and other signaling pathways contribute to the formation and enlargement of renal cysts (6). Therefore, many drugs for treating ADPKD are primarily studied by interfering with these genetic and molecular mechanisms responsible for cystic formation.
In recent years, significant progress has been made in delaying ADPKD progression with different drugs, such as tolvaptan, rapamycin, and somatostatin. As the first drug approved by FDA for the treatment of ADPKD, tolvaptan can slow the growth of TKV and estimated glomerular filtration rate (eGFR) loss, but its hydration and potential liver injury indicate the need for further therapeutic interventions (7). In addition, rapamycin, an mTOR inhibitor, and its analogs did not show satisfactory therapeutic effects in clinical studies (8, 9).
Plant-derived compounds are natural organic components, some of which are considered beneficial to health, and most are easily absorbed and metabolized in the body (10). The development of modern science and technology has broadened people’s understanding of phytochemical components. Thousands of plant-derived compounds have been used to treat various diseases, such as cancer, metabolic diseases, and neurodegeneration (11). Meanwhile, numerous plant-derived compounds have also been explored for their beneficial effects on ADPKD progression. Studies have found that several plant-derived compounds, such as saikosaponin-d, Ganoderma triterpenes, curcumin, ginkgolide B, steviol, resveratrol, Sparganum stoloniferum Buch.-Ham, Cordyceps sinensis, triptolide, quercitrin, naringin, cardamonin, gambogic acid, and olive leaf extract, may delay the development of cysts and improve renal function in ADPKD. Moreover, metformin, a synthesized compound derived from the French lilac or goat’s rue (Galega officinalis), has been proven to retard the progression of chronic kidney disease in ADPKD. This review focuses on the mechanisms (Figure 1) of these plant-derived compounds in treating ADPKD, which may constitute promising new therapeutics in the future.
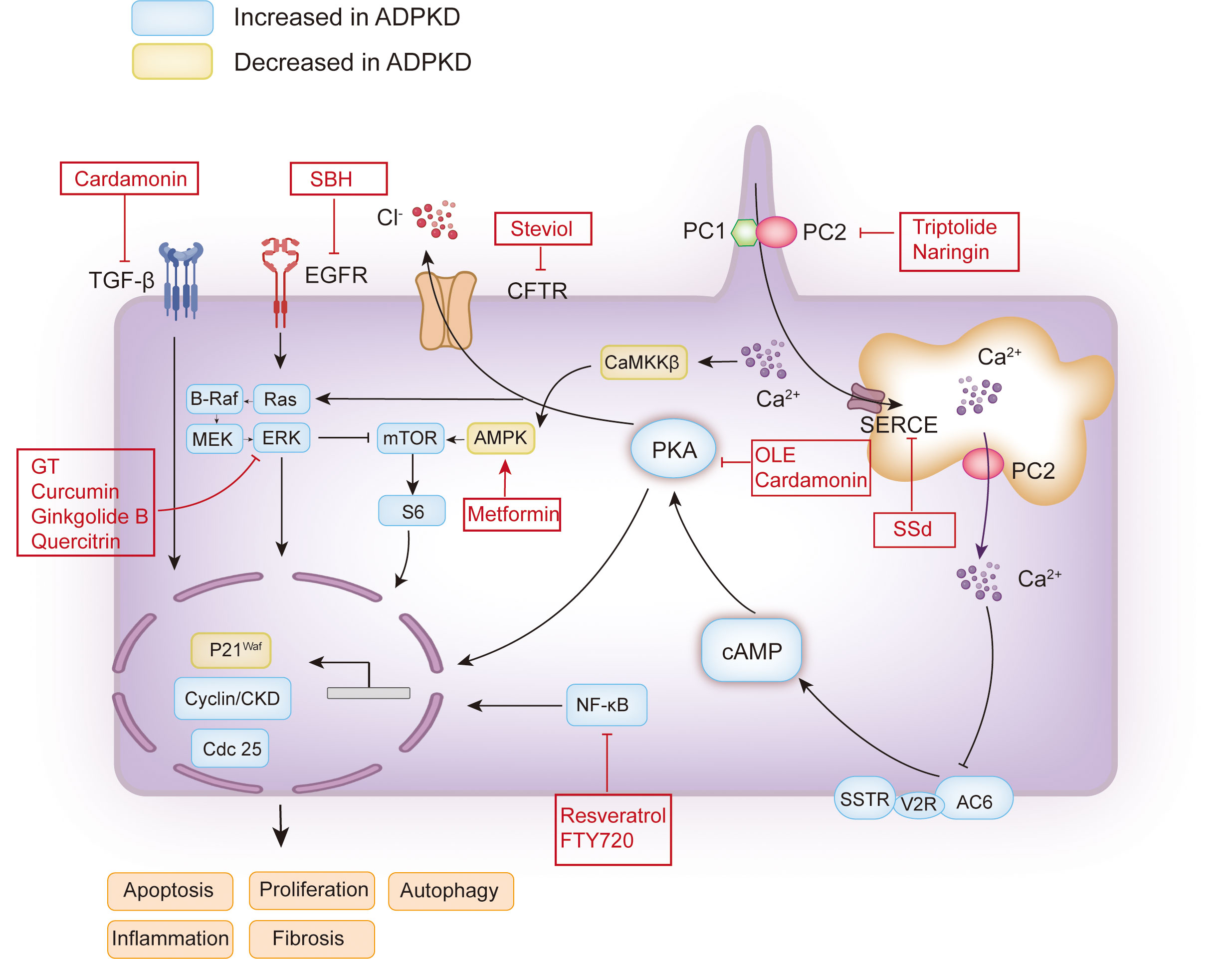
Figure 1 Schematic map of the pathogenesis of ADPKD and the therapeutic targets of plant extracts or plant-derived compounds. The functional site of PC1 and PC2 associated with polycystic kidney disease is on the cilia. The decrease or loss of PC1 or PC2 caused by PKD1 and PKD2 mutations may lead to a decrease in intracellular calcium concentration or an increase in intracellular cAMP. The increase of cAMP will activate PKA, which will activate the mTOR, Ras, and other signaling pathways, and promote cell proliferation. Activated PKA can also promote Cl- to enter the cyst cavity through CFTR, thus promoting the secretion of cyst fluid. In addition, EGFR can promote cell proliferation by activating Ras. Additionally, calcium ions can induce autophagy by activating CaMKK β-AMPK-mTOR. The red boxes contain the candidate plant extracts or plant-derived compounds. PC1, polycystin-1; PC2, polycystin-2; AC6, adenylyl cyclase six; V2R, vasopressin type 2 receptor; SSTR, somatostatin receptor; cAMP, cyclic adenosine monophosphate; PKA, protein kinase A; CFTR, cystic fibrosis transmembrane conductance regulator; EGFR, epidermal growth factor receptor; MEK, mitogen-activated protein kinase; ERK, extracellular-signal-regulated kinase; mTOR, the mammalian target of rapamycin; SERCA, sarcoplasmic/endoplasmic reticulum Ca2+ ATPase; CaMKKβ, Ca2+/CaM-dependent protein kinase β; AMPK, AMP-activated protein kinase; TGF-β, transforming growth factor-β; SSd, saikosaponin-d; GT, Ganoderma triterpenes; SBH, Sparganum stoloniferum Buch.-Ham.
2 The effect of plant-derived compounds in ADPKD models
2.1 Saikosaponin-d
Saikosaponin-d (SSd) is a significant triterpenoid saponin derived from Bupleurum falcatum L. It has immunomodulatory, anti-inflammatory, antiviral, anti-proliferation, and anti-cancer effects in vivo and in vitro (12–15). Many studies have found that SSd has an anti-tumorigenic effect, while ADPKD is considered a tumor-like disease. There are similarities between ADPKD and tumors in pathophysiology (16). This shows the application potential of SSd in the treatment of ADPKD. In ADPKD, PC1 deficiency may activate the function of sarcoplasmic/endoplasmic reticulum calcium ATPase (SERCA) and inhibit flux across the endoplasmic reticulum membrane (17). Research shows that, as a SERCA inhibitor, SSd induces autophagy via the direct inhibition of SERCA, which in turn upregulates intracellular calcium levels. In 2018, we reported for the first time the role of SSd in ADPKD (18). SSd directly inhibits SERCA to upregulate Ca2+ levels, thereby activating the CaMKKβ-AMPK-mTOR signaling pathway, which subsequently induces autophagy in ADPKD cells (18) (Table 1). However, this study was limited to the cellular level, and more studies are needed further to investigate the therapeutic effect of SSd in ADPKD.
2.2 Ganoderma triterpenes
Ganoderma triterpenes (GTs) are a family of lanostane triterpenes isolated from Ganoderma lucidum, which is used in Chinese traditional medicine. As primary secondary metabolites of G. lucidum, GTs undertake anti-cancer, anti-inflammatory, antioxidative, and hepatoprotective therapeutic activities, among others (39). They have been shown to inhibit cell proliferation and invasion, induce cell apoptosis, and regulate immune response (40). The effect of GTs on regulating multiple signaling pathways shared by ADPKD implies their possible role in modulating cyst development. In 2017, Su et al. (19) confirmed that GTs significantly downregulate the RAS/MAPK signaling pathway and inhibit renal cysts in the embryonic renal cyst model and rapidly progressive ADPKD mouse model. Further studies have shown that GT monomer CBLZ-7 (ethyl ganoderate C2) can downregulate the RAS/MAPK signaling pathway in forskolin-stimulated Madin–Darby canine kidney (MDCK) cells in a dose-dependent manner and inhibit the expansion of cysts (19). Hence, GTs have great potential to be developed as a novel therapeutic agent for treating PKD.
2.3 Curcumin
Curcumin is a yellow- or orange-pigmented substance obtained from the root of the turmeric plant (41). In 1937, scientific research on curcumin in the treatment of diseases was first published, and a study investigating the antibacterial activity of curcumin, published in 1949, achieved exciting results (42). More recently, it has been found that curcumin has anti-inflammatatory (43), immune regulation (44), renoprotective (45), hepatoprotective (46), and hypoglycemic (46) effects. Owing to its easy availability, low cost, and low toxicity, it is expected to be an ideal drug. Gao et al. (2011) (20) explored the mechanism of curcumin in an in vitro renal cyst model. The results showed that curcumin significantly inhibits the formation and enlargement of the MDCK cell cystic model and fetal renal cyst and reduced the expression of signal proteins RAS, B-RAF, p-MEK, p-ERK, c-fos, and Egr-1 in forskolin-treated MDCK cells, while increasing the expression of Raf-1 and NAB2 (20). These data suggest that curcumin may inhibit the development of renal cysts by regulating the Ras/MAPK signaling pathway and has the potential to be developed as a candidate drug for the treatment of PKD. In addition, curcumin has the disadvantage of low bioavailability and can interfere with other drugs.
2.4 Ginkgolide B
Ginkgolide B is a major bioactive component of Ginkgo biloba and undertakes anti-inflammatory (47), anti-allergy, antioxidative, anti-cancer, and neuroprotective (48–50) activities, among others. The increase of intracellular cAMP mainly stimulates the proliferation of renal cystic epithelial cells by activating the MAPK/ERK signaling pathway, which may be related to the regulation of B-Raf and Raf-1 (51, 52). Zhou et al. (21) used an in vitro MDCK cyst model, an embryonic kidney cyst model, and an in vivo PKD mouse model to study the effect of ginkgolide B on cysts. The results showed that ginkgolide B does not induce cytotoxicity and apoptosis in MDCK cells but significantly inhibits the formation and growth of renal cysts (21). Ginkgolide B could downregulate the level of B-Raf in forskolin-treated MDCK cells, but upregulate the level of Raf-1. The opposite regulation of B-Raf and Raf-1 may be the key mechanism that allows ginkgolide B to inhibit cysts (21). Therefore, the RAS/MAPK signaling pathway may be involved in the inhibitory effect of ginkgolide B on the abnormal proliferation of cystic cells.
2.5 Steviol
Stevioside, extracted from Stevia rebuadiana, is widely used as a non-calorie sweetener in food (53). Pharmacokinetic studies have shown that stevioside is first transformed into steviol, the primary metabolite, by intestinal flora, then absorbed by the intestinal tract and distributed to several organs, such as the intestine, liver, and kidneys through blood (54–56). It has been found that the interaction between steviol and renal organic anion transporter helps improve the therapeutic effect of drugs (57, 58). In addition, steviol and its derivative (dihydroisosteviol) inhibit cAMP-activated chlorine secretion by targeting CFTR in human colonic epithelial cell lines (59). The dilatation of the cyst cavity of ADPKD is related to the proliferation of cyst wall epithelial cells and the secretion of fluid. Fluid secretion depends on the chloride channel of the CFTR (60) and the water channels of the parietal membranes of the cyst wall epithelial cells (61). Chloride enters the cystic cavity through CFTR activated by cAMP, accumulates in the cyst cavity, and sucks sodium and water into the cyst cavity through the paracellular pathway, causing the cyst to enlarge (60). Yuajit et al. (2013) (22) studied the inhibitory effect of steviol and its derivatives on the cystic growth of MDCK cells and its mechanism. The results showed that steviol has the most substantial inhibitory effect on the growth of MDCK cysts, which was achieved by inhibiting the activity of the CFTR chloride channel and reducing the expression of CFTR (22). Further study in the PKD1 mouse model showed that steviol at 200mg/kg body weight for 14 days could significantly reduce kidney weight and the cystic index and improve renal function in mice. This effect is achieved in part by activating AMPK, inhibiting the expression of the CFTR chloride channel, and inhibiting the proliferation of renal epithelial cells through the mTOR/S6K pathway (23). Moreover, overexpression of aquaporin 2 (AQP2) was shown to be involved in fluid secretion, leading to cyst enlargement in ADPKD. A study in 2018 found that steviol not only affected the activity of CFTR but inhibited the expression of AQP2 at the transcriptional level and promoted the degradation of AQP2 mediated by proteasomes and lysosomes, resulting in a decrease in water transport to the cyst cavity, thus delaying the growth of cysts (24). Therefore, steviol may be a potential botanical candidate for treating PKD.
2.6 Resveratrol
Resveratrol is a natural polyphenol and occurs abundantly in red grapes, berries, peanuts, and legumes (62). Studies have shown that resveratrol has therapeutic effects on various diseases, such as aging, hypertension, cancer, and kidney diseases (62, 63). It has been found that resveratrol exerts its anti-inflammatory, antioxidative, and anti-proliferative effects by acting on different intracellular targets (63). Inflammation plays an essential role in the pathogenesis of ADPKD (64). Inflammatory factors have been found in the urine and renal cyst fluid of ADPKD patients (64). In addition, inflammatory cells, such as macrophages, accumulate in cystic kidneys and have been shown to promote the growth of renal cysts (64, 65). We reported the role of resveratrol in ADPKD in 2016 (25). In this study, we demonstrated that the anti-inflammatory substance resveratrol reduced the production of monocyte chemotactic protein-1, complement factor B, and tumor necrosis factor-α (TNF-α) and reduced macrophage infiltration in cystic kidneys, delaying the progression of PKD by reducing inflammation in cystic kidneys (25). Notably, resveratrol is also an activator of the SIRT pathway (66), which may have deleterious effects on PKD. However, Zhou et al. (67) found that activation of SIRT1 led to the proliferation of renal epithelial cells through deacetylation and phosphorylation of the retinoblastoma (Rb) protein and dysregulation of cell death through deacetylation of the P53 protein, leading to continued epithelial cell growth and cystic lesion formation. Thus, excessive amounts of resveratrol may cause excessive activation of the SIRT pathway, which promotes vesicle formation and expansion. Further studies are needed to address these critical issues and identify resveratrol’s safe and effective dosage.
2.7 Sparganum stoloniferum Buch.-Ham
Sparganum stoloniferum Buch.-Ham (SBH), also known as Coptis Chinensis, is a commonly used traditional Chinese medicine that is widely used to improve blood circulation and reduce vascular obstruction (27). In a study, we found that SBH could prevent cells at the G0/G1 phase from reaching the G2/M phase, and inhibited the phosphorylation of EGFR, thus inhibiting the proliferation of ADPKD cystic epithelial cells (26). The PKDL gene encodes polycystic protein-L (PCL), which has 50% homology with PC2 (68). PC2 and PCL are non-selective cation channels for the permeability of potassium, sodium, and calcium, which are closely related to the occurrence of renal cysts (69). Li et al. (27) expressed human PCL in Xenopus oocytes and examined the effects of SBH on PCL channel function, using the 2-electrode voltage-clamp technique and radiolabeled 45Ca uptake measurements. The results showed that SBH contained one or more components that inhibited PCL channels, which might be useful for diseases related to abnormal PCL function (27). However, whether SBH also inhibits PC2 channels remains to be determined.
2.8 Cordyceps sinensis
Cordyceps Sinensis (CS) is a unique leafy fungus growing on caterpillars and is regarded as a beneficial herbal medicine in traditional Chinese medicine and is used to treat many diseases, including those that affect the respiratory system, liver, cardiovascular system, as well as hyperlipidemia (70). CS has renal protective effects (71) and has been used to treat several renal diseases, including chronic renal failure, renal transplantation, and acute renal injury (72–74). FTY720 (Fingolimod) is a novel immunomodulatory compound derived from CS and is an effective inhibitor of sphingosine-1-phosphate receptor (S1PR) (75). S1P has been approved by the FDA for the treatment of multiple sclerosis. S1P is an inflammatory regulator that activates the signal transducer and activator of the transcription 3 (STAT3) pathway or directly activates the NF-κB pathway through S1PR1 (76). We found that FTY720 can inhibit the expression of pro-inflammatory cytokines, such as IL-6 and tumor necrosis factor-α(TNF-α), block the activation of inflammatory pathways, such as STAT and NF-κB, and thus inhibit the growth of renal cysts in PKD rats (28).
2.9 Quercitrin
Quercitrin is a kind of plant polyphenol widely found in many kinds of vegetables and fruits (77). It has many pharmacological effects, such as those that are anti-inflammatory, anti-tumorigenic, anti-oxidative, neuroprotective, and anti-aging (78–80). Studies have shown that quercitrin significantly inhibits the growth and proliferation of tumor cells through MAPK/ERK and AKT/mTOR (81, 82), which corresponds to the pathophysiology of ADPKD. Zhu et al. (29) used an MDCK cystic model and a PKD mouse model to study the effect of quercitrin on renal cysts. The results showed that quercitrin significantly inhibits the formation and development of vesicles both in vivo and in vitro in a dose-dependent manner (29). Quercitrin significantly decreases the levels of AKT and ERK in the kidney cells of PKD mice. In addition, E-cadherin is a kind of cell membrane protein that is involved in the maintenance of intercellular adhesion and plane polarity (83, 84) and can be regulated through the regulation of the ERK and AKT signal pathways (85). Zhu et al. showed that the expression of E-cadherin is reduced in PKD mice and is located in proximal tubules (29). Quercitrin reversed E-cadherin expression in proximal tubules of PKD mice on P10. Meanwhile, quercitrin can decrease p-ERK and p-AKT expression, thus inhibiting cystic development (29). Therefore, quercitrin has great development potential as a candidate drug for treating ADPKD.
2.10 Naringin
Naringin is a flavanone compound found in citrus fruits and has anti-inflammatory, antioxidative, and cholesterol-lowering effects (86). In addition, naringin can promote cell cycle arrest and p53-dependent apoptosis to inhibit the growth of tumor cells (87). Waheed et al. (30) studied the effect of naringin on the growth of cysts in PC2 knockout MDCK cells. The results showed that naringin can significantly reduce the viability and inhibit the growth of MDCK cells in a PC2-dependent manner (30). As PC2 participates in intracellular calcium signal transduction, thus affecting the secretion of Cl-, the effect of naringin on Cl- was investigated (30). However, naringin did not reduce the short-circuit current compared with the positive control group (e.g., genistein and apigenin), which inhibited the entry of chloride ions through the basement membrane (30). This suggests that naringin exerts its anti-proliferative effect by activating PC2, but the downstream mechanism does not include liquid secretion (30).
2.11 Cardamonin
Cardamonin is a chalcone compound isolated from Alpinia katsumadai and which has significant anti-inflammatory, anti-proliferative, and immunomodulatory effects (88). Many studies have shown that cardamonin has an obvious curative effect on many diseases, such as arthritis (89), colitis (90), and cancer (91). It has been found that the occurrence of ADPKD is related to the changes in the composition of the extracellular matrix and the thickness of the basement membrane. The excessive production of collagen leads to the deposition of fibroblasts and collagen fibers (92). He et al. (31) studied the role of cardamonin in the treatment of a cyst enlargement MDCK cyst model, an embryonic kidney cyst model, and an orthologous mouse model of ADPKD. The results showed that cardamonin delayed cystic growth and alleviated renal fibrosis by downregulating the MAPK, Wnt, mTOR, and TGF-β signaling pathways (31).
2.12 Gambogic acid
Gambogic acid (GA) is a compound isolated from the brownish orange gamboge resin of the Garcinia hanburyi tree (93) and undertakes anti-proliferative, anti-apoptotic, anti-tumorigenic, anti-angiogenic, and anti-inflammatory activities, among others (93–98). GA has been shown to inhibit the proliferation of melanoma (95), esophageal squamous carcinoma (99), and glioma cells (100). Khunpatee et al. (32) studied the effect of GA on ADPKD in MDCK and PKD1 mutant cells. The results showed that GA inhibits the enlargement of cysts by inhibiting the phosphorylation of the ERK1/2 and mTOR/S6K signaling pathways (32). In addition, GA can significantly improve the phosphorylation activity of AMPK (32).
2.13 Olive leaf extract
Extra virgin olive oil contains a lot of polyphenols, which can lower blood pressure, increase blood flow in coronary arteries, and slow down heart rate (101, 102). Olive leaf extract (OLE) is often used to prevent and treat high blood pressure or as a diuretic or preservative (103). Recent studies have found that OLE can treat a variety of cancers (104–107). Toteda et al. (33) investigated whether OLE can inhibit the cystic growth of ADPKD in vitro. The results showed that OLE could reduce the level of PKA, p-ERK, and cAMP and upregulate the level of p-AKT, thus reducing the growth of cystic cells in vitro (33).
3 The effect and safety of plant-derived compounds in ADPKD patients
3.1 Metformin
Metformin is a synthesized compound derived from goat’s rue (Galega officinalis). As an AMPK activator, metformin has been widely used for treating type 2 diabetes and polycystic ovary syndrome for decades (108). During the development of PKD, AMPK activity decreases. Several studies have shown that AMPK is a potential target for treating ADPKD (34). In preclinical studies, metformin can inhibit renal cysts in ADPKD mice, miniature pig models, and in vitro experiments. Therapeutic AMPK activation can reduce the severity of cystic kidney disease in Pkd-/- animal models by improving mitochondrial biogenesis and reducing tissue inflammation (109). Recent preclinical studies have shown that it may play a role in delaying the development of renal cysts in patients with ADPKD (110). The therapeutic effect of metformin on ADPKD was first proposed by Takiar et al. (34). They found that large doses (300 mg/kg body weight) of metformin can stimulate AMPK, resulting in the inhibition of CFTR and mTOR, thereby inhibiting the secretion and proliferation of Pkd-/- mouse epithelial cells (34). Another study showed that metformin inhibits the formation of renal cysts in Pkd2 morphant zebrafish by activating the AMPK pathway and reducing cell proliferation and autophagy (35). Similarly, in a miniature Pkd-/- pig model, oral metformin can inhibit renal cyst growth and improve renal function (36). However, no beneficial effect of metformin was observed in another Pkd-/- mouse model (111). The reason for this may be that this study injected tamoxifen later causing the disease to progress slowly compared with the study by Takiar et al. Another difference between the two studies may be the method of drug administration. Takiar et al. administered the drug by injection (34), while Leonhard et al. (111) administered it orally. The oral bioavailability of metformin was only 40–60%. This suggests that the animal models and administration methods used in the study will affect metformin’s effectiveness. Furthermore, the tolerance, safety, and preliminary efficacy of metformin in adult patients with ADPKD were evaluated in a phase 2 double-blind placebo-controlled randomized controlled trial (37). In this study, 97 ADPKD patients between the ages of 18 and 60 were randomly assigned to receive a 1:1 administration of metformin and placebo. The results showed that metformin slightly reduces the decrease of GFR in patients with ADPKD, but the effect was not significant (37). Encouragingly, metformin showed good safety and tolerance in this study (37). Hence, the evaluation of efficacy requires a larger trial with sufficient power to detect differences in endpoints. Interestingly, the phase 3 clinical trial (IMPEDE-PKD) of metformin therapy to alleviate renal function decline in ADPKD is expected to be completed in 2026. By then, we should have a clear answer as to whether metformin will be effective in the management of ADPKD.
3.2 Triptolide
Triptolide is a compound derived from the Chinese herbal medicine Tripterygium wilfordii Hook f (TWHf) and exhibits anti-proliferative, immunosuppressive, and anti-inflammatory effects in many diseases (112). Root extracts of TWHf have been used to treat nephrotic syndrome, cancer, lupus, Behçet’s disease, and other diseases throughout history (113, 114). Since triptolide was extracted and isolated from TWHf in 1972, its mechanism of action and clinical efficacy have been extensively investigated, and its inhibitory effect on renal cysts has also become a research hotspot. Several studies have shown the inhibitory effect of triptolide on renal cysts in several premature Pkd1 animal models at embryogenic, neonatal, or neonatal to adult transition stages (38, 115, 116). Research shows that triptolide can regulate the release of Ca2+ through a PC2-dependent mechanism to arrest cyst proliferation (116). In 2018, we reported the effect of triptolide in an adult PKD rat model. Triptolide treatment for 12 weeks delayed the decline of renal function and inhibited renal cysts in adult PKD rats, perhaps through the JAK2/STAT3 pathway (117). In a clinical study, Chen et al. found that albuminuria decreases in patients with ADPKD after 6 months of triptolide treatment, and cyst growth rate and renal dysfunction are significantly improved (118). However, the study also mentions that the use of triptolide in treating ADPKD may cause menstrual disorders in female patients (118), and the water solubility of triptolide is poor (118). Hence, we need more rigorous pharmacological research and well-designed clinical trials to provide evidence-based support for its safety and efficacy.
4 Conclusions and outlook
ADPKD patients show extreme enlargement of both kidneys, which are filled with cystic fluid, and this eventually leads to ESKD (2). At present, the limited treatment options for ADPKD is still a challenge for nephrologists. So far, some plant-derived compounds have been shown to inhibit the activity of renal cysts through a variety of potential mechanisms, which provides new options for ADPKD treatment. However, there are still some problems that need to be considered in the future development of plant-derived compounds. First, some plant-derived compounds inhibit renal cysts in vitro but much less so in vivo or in clinical trials, which may be due to the toxicity or low bioavailability of the drugs. Therefore, more basic and clinical studies are needed to prove the safety and effectiveness of these plant-derived compounds. Second, there are many factors affecting the effectiveness of plant-derived compounds, and good quality control is the key to ensuring their safety and effectiveness. Therefore, a more comprehensive quality control model is needed, which requires pharmacological/biological evaluation in addition to some emerging chemical analysis assessments, such as chromatographic fingerprinting and multi-component quantification (119, 120). Besides, the nephrotoxicity of plant-derived compounds cannot be ignored (121). In addition to aristolochic acid, some other plant-derived compounds, such as Tripterygium regelii Sprague et Takeda, can cause renal tubular damage and inflammatory cell infiltration (122). It is speculated that more than 100 herbs have adverse effects on the kidneys (123). In addition, although many plant-derived compounds have been widely used in clinical environments, the mechanism of most plant-derived compounds is still unclear. A more comprehensive understanding of the specific mechanisms will lead to the discovery of more potent drugs to treat ADPKD. Finally, the application of artificial intelligence technology and the development of bioinformatics may provide new insights for research of plant-derived compounds for treating ADPKD. In addition to those described in this paper, there are some other plant-derived compounds, such as colchicine and emodin, that are associated with the pathophysiology of ADPKD (124, 125).
In conclusion, plant-derived compounds provide a rich resource for the development of drugs in the treatment of ADPKD. Many plant-derived compounds show good application potential for the inhibition of renal cyst growth and may provide promising new therapeutic choices for ADPKD in the future.
Author contributions
All authors listed have made a substantial, direct, and intellectual contribution to the work and approved the manuscript for publication.
Funding
The authors acknowledge the financial support from the National Natural Science Foundation of China (81770670 and 82070705); Medical innovation research project of Shanghai Science and Technology Innovation Plan (22Y11905500); Medical-enterprise integration innovation achievement transformation project (SHDC2022CRD024).
Conflict of interest
The authors declare that the research was conducted in the absence of any commercial or financial relationships that could be construed as a potential conflict of interest.
Publisher’s note
All claims expressed in this article are solely those of the authors and do not necessarily represent those of their affiliated organizations, or those of the publisher, the editors and the reviewers. Any product that may be evaluated in this article, or claim that may be made by its manufacturer, is not guaranteed or endorsed by the publisher.
References
1. Chapman AB, Devuyst O, Eckardt K-U, Gansevoort RT, Harris T, Horie S, et al. Autosomal-dominant polycystic kidney disease (Adpkd): Executive summary from a kidney disease: Improving global outcomes (Kdigo) controversies conference. Kidney Int (2015) 88(1):17–27. doi: 10.1038/ki.2015.59
2. Cornec-Le Gall E, Alam A, Perrone RD. Autosomal dominant polycystic kidney disease. Lancet (2019) 393(10174):919–35. doi: 10.1016/S0140-6736(18)32782-X
3. Harris PC, Torres VE. Polycystic kidney disease. Annu Rev Med (2009) 60:321–37. doi: 10.1146/annurev.med.60.101707.125712
4. Xue C, Zhou C-C, Wu M, Mei C-L. The clinical manifestation and management of autosomal dominant polycystic kidney disease in China. Kidney Dis (Basel) (2016) 2(3):111–9. doi: 10.1159/000449030
5. Torres VE, Harris PC. Progress in the understanding of polycystic kidney disease. Nat Rev Nephrol (2019) 15(2):70–2. doi: 10.1038/s41581-018-0108-1
6. Torres VE, Harris PC. Strategies targeting camp signaling in the treatment of polycystic kidney disease. J Am Soc Nephrol (2014) 25(1):18–32. doi: 10.1681/ASN.2013040398
7. Blair HA. Tolvaptan: A review in autosomal dominant polycystic kidney disease. Drugs (2019) 79(3):303–13. doi: 10.1007/s40265-019-1056-1
8. Capuano I, Buonanno P, Riccio E, Rizzo M, Pisani A. Tolvaptan vs. somatostatin in the treatment of adpkd: A review of the literature. Clin Nephrol (2022) 97(3):131–40. doi: 10.5414/CN110510
9. Li A, Fan S, Xu Y, Meng J, Shen X, Mao J, et al. Rapamycin treatment dose-dependently improves the cystic kidney in a new adpkd mouse model Via the Mtorc1 and cell-Cycle-Associated Cdk1/Cyclin axis. J Cell Mol Med (2017) 21(8):1619–35. doi: 10.1111/jcmm.13091
10. Ramakrishna Y, Goda H, Baliga MS, Munshi AK. Decreasing cariogenic bacteria with a natural, alternative prevention therapy utilizing phytochemistry (Plant extracts). J Clin Pediatr Dent (2011) 36(1):55–63. doi: 10.17796/jcpd.36.1.f485870h90174311
11. Armendáriz-Barragán B, Zafar N, Badri W, Galindo-Rodríguez SA, Kabbaj D, Fessi H, et al. Plant extracts: From encapsulation to application. Expert Opin Drug Delivery (2016) 13(8):1165–75. doi: 10.1080/17425247.2016.1182487
12. Bermejo Benito P, Abad Martínez MJ, Silván Sen AM, Sanz Gómez A, Fernández Matellano L, Sánchez Contreras S, et al. In vivo and in vitro antiinflammatory activity of saikosaponins. Life Sci (1998) 63(13):1147–56. doi: 10.1016/S0024-3205(98)00376-2
13. Cheng P-W, Ng L-T, Chiang L-C, Lin C-C. Antiviral effects of saikosaponins on human coronavirus 229e in vitro. Clin Exp Pharmacol Physiol (2006) 33(7):612–6. doi: 10.1111/j.1440-1681.2006.04415.x
14. Kang SJ, Lee YJ, Kim BM, Kim YJ, Woo HD, Jeon HK, et al. Effect of bupleuri radix extracts on the toxicity of 5-fluorouracil in Hepg2 hepatoma cells and normal human lymphocytes. Basic Clin Pharmacol Toxicol (2008) 103(4):305–13. doi: 10.1111/j.1742-7843.2008.00280.x
15. Li C, Guan X, Xue H, Wang P, Wang M, Gai X. Reversal of p-Glycoprotein-Mediated multidrug resistance is induced by saikosaponin d in breast cancer mcf-7/Adriamycin cells. Pathol Res Pract (2017) 213(7):848–53. doi: 10.1016/j.prp.2017.01.022
16. Ding Y, Chen L, Deng F-M, Melamed J, Fan R, Bonsib S, et al. Localized cystic disease of the kidney: Distinction from cystic neoplasms and hereditary polycystic diseases. Am J Surg Pathol (2013) 37(4):506–13. doi: 10.1097/PAS.0b013e318271eff9
17. Weber KH, Lee EK, Basavanna U, Lindley S, Ziegelstein RC, Germino GG, et al. Heterologous expression of polycystin-1 inhibits endoplasmic reticulum calcium leak in stably transfected mdck cells. Am J Physiol Renal Physiol (2008) 294(6):F1279–F86. doi: 10.1152/ajprenal.00348.2007
18. Shi W, Xu D, Gu J, Xue C, Yang B, Fu L, et al. Saikosaponin-d inhibits proliferation by up-regulating autophagy via the camkkβ-Ampk-Mtor pathway in adpkd cells. Mol Cell Biochem (2018) 449(1-2):219–26. doi: 10.1007/s11010-018-3358-0
19. Su L, Liu L, Jia Y, Lei L, Liu J, Zhu S, et al. Ganoderma triterpenes retard renal cyst development by downregulating Ras/Mapk signaling and promoting cell differentiation. Kidney Int (2017) 92(6):1404–18. doi: 10.1016/j.kint.2017.04.013
20. Gao J, Zhou H, Lei T, Zhou L, Li W, Li X, et al. Curcumin inhibits renal cyst formation and enlargement in vitro by regulating intracellular signaling pathways. Eur J Pharmacol (2011) 654(1):92–9. doi: 10.1016/j.ejphar.2010.12.008
21. Zhou H, Gao J, Zhou L, Li X, Li W, Li X, et al. Ginkgolide b inhibits renal cyst development in in vitro and in vivo cyst models. Am J Physiol Renal Physiol (2012) 302(10):F1234–F42. doi: 10.1152/ajprenal.00356.2011
22. Yuajit C, Homvisasevongsa S, Chatsudthipong L, Soodvilai S, Muanprasat C, Chatsudthipong V. Steviol reduces mdck cyst formation and growth by inhibiting cftr channel activity and promoting proteasome-mediated cftr degradation. PloS One (2013) 8(3):e58871. doi: 10.1371/journal.pone.0058871
23. Yuajit C, Muanprasat C, Gallagher A-R, Fedeles SV, Kittayaruksakul S, Homvisasevongsa S, et al. Steviol retards renal cyst growth through reduction of cftr expression and inhibition of epithelial cell proliferation in a mouse model of polycystic kidney disease. Biochem Pharmacol (2014) 88(3):412–21. doi: 10.1016/j.bcp.2014.01.038
24. Noitem R, Yuajit C, Soodvilai S, Muanprasat C, Chatsudthipong V. Steviol slows renal cyst growth by reducing Aqp2 expression and promoting Aqp2 degradation. BioMed Pharmacother (2018) 101:754–62. doi: 10.1016/j.biopha.2018.02.139
25. Wu M, Gu J, Mei S, Xu D, Jing Y, Yao Q, et al. Resveratrol delays polycystic kidney disease progression through attenuation of nuclear factor Kb-induced inflammation. Nephrol Dial Transplant (2016) 31(11):1826–34. doi: 10.1093/ndt/gfw058
26. Xu CG MC, Ge SY, Sun TM. Effects of traditional Chinese medicine sanleng on proliferation and phosphorylation of epithelial growth factor receptor in cyst lining epithelial cells of polycystic kidney disease. Chin J Nephrol (2002), 18(1).
27. Li F, Dai X-Q, Li Q, Wu Y, Chen X-Z. Inhibition of polycystin-l channel by the Chinese herb sparganum stoloniferum buch.-ham. Can J Physiol Pharmacol (2006) 84(8-9):923–7. doi: 10.1139/y06-040
28. Li X, Wu M, Chen L, Lu J, Li G, Fu L, et al. A sphingosine-1-Phosphate modulator ameliorates polycystic kidney disease in Han:Sprd rats. Am J Nephrol (2020) 51(1):1-10. doi: 10.1159/000502855
29. Zhu Y, Teng T, Wang H, Guo H, Du L, Yang B, et al. Quercetin inhibits renal cyst growth in vitro and via parenteral injection in a polycystic kidney disease mouse model. Food Funct (2018) 9(1):389–96. doi: 10.1039/c7fo01253e
30. Waheed A, Ludtmann MHR, Pakes N, Robery S, Kuspa A, Dinh C, et al. Naringenin inhibits the growth of dictyostelium and mdck-derived cysts in a Trpp2 (Polycystin-2)-Dependent manner. Br J Pharmacol (2014) 171(10):2659–70. doi: 10.1111/bph.12443
31. He J, Zhou H, Meng J, Zhang S, Li X, Wang S, et al. Cardamonin retards progression of autosomal dominant polycystic kidney disease Via inhibiting renal cyst growth and interstitial fibrosis. Pharmacol Res (2020) 155:104751. doi: 10.1016/j.phrs.2020.104751
32. Khunpatee N, Bhukhai K, Chatsudthipong V, Yuajit C. Potential application of gambogic acid for retarding renal cyst progression in polycystic kidney disease. Molecules (2022) 27(12):3837. doi: 10.3390/molecules27123837
33. Toteda G, Vizza D, Lupinacci S, Perri A, Scalise MF, Indiveri C, et al. Olive leaf extract counteracts cell proliferation and cyst growth in an in vitro model of autosomal dominant polycystic kidney disease. Food Funct (2018) 9(11):5925–35. doi: 10.1039/c8fo01481g
34. Takiar V, Nishio S, Seo-Mayer P, King JD, Li H, Zhang L, et al. Activating amp-activated protein kinase (Ampk) slows renal cystogenesis. Proc Natl Acad Sci U.S.A. (2011) 108(6):2462–7. doi: 10.1073/pnas.1011498108
35. Chang M-Y, Ma T-L, Hung C-C, Tian Y-C, Chen Y-C, Yang C-W, et al. Metformin inhibits cyst formation in a zebrafish model of polycystin-2 deficiency. Sci Rep (2017) 7(1):7161. doi: 10.1038/s41598-017-07300-x
36. Lian X, Wu X, Li Z, Zhang Y, Song K, Cai G, et al. The combination of metformin and 2-deoxyglucose significantly inhibits cyst formation in miniature pigs with polycystic kidney disease. Br J Pharmacol (2019) 176(5):711–24. doi: 10.1111/bph.14558
37. Perrone RD, Abebe KZ, Watnick TJ, Althouse AD, Hallows KR, Lalama CM, et al. Primary results of the randomized trial of metformin administration in polycystic kidney disease (Tame pkd). Kidney Int (2021) 100(3):684–96. doi: 10.1016/j.kint.2021.06.013
38. Leuenroth SJ, Bencivenga N, Igarashi P, Somlo S, Crews CM. Triptolide reduces cystogenesis in a model of adpkd. J Am Soc Nephrol (2008) 19(9):1659–62. doi: 10.1681/ASN.2008030259
39. Gill BS, Sharma P, Kumar R, Kumar S. Misconstrued versatility of ganoderma lucidum: A key player in multi-targeted cellular signaling. Tumour Biol (2016) 37(3):2789–804. doi: 10.1007/s13277-015-4709-z
40. Xia Q, Zhang H, Sun X, Zhao H, Wu L, Zhu D, et al. A comprehensive review of the structure elucidation and biological activity of triterpenoids from ganoderma spp. Molecules (2014) 19(11):17478–535. doi: 10.3390/molecules191117478
41. Unlu A, Nayir E, Dogukan Kalenderoglu M, Kirca O, Ozdogan M. Curcumin (Turmeric) and cancer. J BUON (2016) 21(5):1050–60.
42. Zhou H, Beevers CS, Huang S. The targets of curcumin. Curr Drug Targets (2011) 12(3):332–47. doi: 10.2174/138945011794815356
43. Zhang N, Li H, Jia J, He M. Anti-inflammatory effect of curcumin on mast cell-mediated allergic responses in ovalbumin-induced allergic rhinitis mouse. Cell Immunol (2015) 298(1-2):88–95. doi: 10.1016/j.cellimm.2015.09.010
44. Jantan I, Bukhari SNA, Lajis NH, Abas F, Wai LK, Jasamai M. Effects of diarylpentanoid analogues of curcumin on chemiluminescence and chemotactic activities of phagocytes. J Pharm Pharmacol (2012) 64(3):404–12. doi: 10.1111/j.2042-7158.2011.01423.x
45. Trujillo J, Chirino YI, Molina-Jijón E, Andérica-Romero AC, Tapia E, Pedraza-Chaverrí J. Renoprotective effect of the antioxidant curcumin: Recent findings. Redox Biol (2013) 1:448–56. doi: 10.1016/j.redox.2013.09.003
46. Kiso Y, Suzuki Y, Watanabe N, Oshima Y, Hikino H. Antihepatotoxic principles of curcuma longa rhizomes. Planta Med (1983) 49(3):185–7. doi: 10.1055/s-2007-969845
47. Impey S, Obrietan K, Wong ST, Poser S, Yano S, Wayman G, et al. Cross talk between erk and pka is required for Ca2+ stimulation of creb-dependent transcription and erk nuclear translocation. Neuron (1998) 21(4):869–83. doi: 10.1016/S0896-6273(00)80602-9
48. Huang SH, Duke RK, Chebib M, Sasaki K, Wada K, Johnston GAR. Ginkgolides, diterpene trilactones of ginkgo biloba, as antagonists at recombinant Alpha1beta2gamma2l gabaa receptors. Eur J Pharmacol (2004) 494(2-3):131–8. doi: 10.1016/j.ejphar.2004.04.051
49. Jin G-H, Huang Z, Tan X-F, Tian M-L, Zhang X-H, Qin J-B, et al. Effects of ginkgolide on the development of nos and ache positive neurons in the embryonic basal forebrain. Cell Biol Int (2006) 30(6):500–4. doi: 10.1016/j.cellbi.2005.12.012
50. Wang S-J, Chen H-H, Ginkgolide B. A constituent of ginkgo biloba, facilitates glutamate exocytosis from rat hippocampal nerve terminals. Eur J Pharmacol (2005) 514(2-3):141–9. doi: 10.1016/j.ejphar.2005.03.027
51. Yamaguchi T, Hempson SJ, Reif GA, Hedge A-M, Wallace DP. Calcium restores a normal proliferation phenotype in human polycystic kidney disease epithelial cells. J Am Soc Nephrol (2006) 17(1):178–87. doi: 10.1681/ASN.2005060645
52. Yamaguchi T, Nagao S, Wallace DP, Belibi FA, Cowley BD, Pelling JC, et al. Cyclic amp activates b-raf and erk in cyst epithelial cells from autosomal-dominant polycystic kidneys. Kidney Int (2003) 63(6):1983–94. doi: 10.1046/j.1523-1755.2003.00023.x
53. Chatsudthipong V, Muanprasat C. Stevioside and related compounds: Therapeutic benefits beyond sweetness. Pharmacol Ther (2009) 121(1):41–54. doi: 10.1016/j.pharmthera.2008.09.007
54. Cardoso VN, Barbosa MF, Muramoto E, Mesquita CH, Almeida MA. Pharmacokinetic studies of 131i-stevioside and its metabolites. Nucl Med Biol (1996) 23(1):97-100. doi: 10.1016/0969-8051(95)02072-1
55. Gardana C, Simonetti P, Canzi E, Zanchi R, Pietta P. Metabolism of stevioside and rebaudioside a from stevia rebaudiana extracts by human microflora. J Agric Food Chem (2003) 51(22):6618–22. doi: 10.1021/jf0303619
57. Chatsudthipong V, Jutabha P. Effect of steviol on para-aminohippurate transport by isolated perfused rabbit renal proximal tubule. J Pharmacol Exp Ther (2001) 298(3):1120–7.
58. Srimaroeng C, Jutabha P, Pritchard JB, Endou H, Chatsudthipong V. Interactions of stevioside and steviol with renal organic anion transporters in S2 cells and mouse renal cortical slices. Pharm Res (2005) 22(6):858–66. doi: 10.1007/s11095-005-4580-5
59. Pariwat P, Homvisasevongsa S, Muanprasat C, Chatsudthipong V. A natural plant-derived dihydroisosteviol prevents cholera toxin-induced intestinal fluid secretion. J Pharmacol Exp Ther (2008) 324(2):798–805. doi: 10.1124/jpet.107.129288
60. Sullivan LP, Wallace DP, Grantham JJ. Epithelial transport in polycystic kidney disease. Physiol Rev (1998) 78(4):1165–91. doi: 10.1152/physrev.1998.78.4.1165
61. Terryn S, Ho A, Beauwens R, Devuyst O. Fluid transport and cystogenesis in autosomal dominant polycystic kidney disease. Biochim Biophys Acta (2011) 1812(10):1314–21. doi: 10.1016/j.bbadis.2011.01.011
62. Mukherjee S, Dudley JI, Das DK. Dose-dependency of resveratrol in providing health benefits. Dose Response (2010) 8(4):478–500. doi: 10.2203/dose-response.09-015.Mukherjee
63. Kitada M, Koya D. Renal protective effects of resveratrol. Oxid Med Cell Longev (2013) 2013:568093. doi: 10.1155/2013/568093
64. Ta MHT, Harris DCH, Rangan GK. Role of interstitial inflammation in the pathogenesis of polycystic kidney disease. Nephrol (Carlton) (2013) 18(5):317–30. doi: 10.1111/nep.12045
65. Karihaloo A, Koraishy F, Huen SC, Lee Y, Merrick D, Caplan MJ, et al. Macrophages promote cyst growth in polycystic kidney disease. J Am Soc Nephrol (2011) 22(10):1809–14. doi: 10.1681/ASN.2011010084
66. Moradi H, Vaziri ND. Effect of resveratrol on progression of polycystic kidney disease: A case of cautious optimism. Nephrol Dial Transplant (2016) 31(11):1755–8. doi: 10.1093/ndt/gfw097
67. Zhou X, Fan LX, Sweeney WE, Denu JM, Avner ED, Li X. Sirtuin 1 inhibition delays cyst formation in autosomal-dominant polycystic kidney disease. J Clin Invest (2013) 123(7):3084–98. doi: 10.1172/JCI64401
68. Wu G, Hayashi T, Park JH, Dixit M, Reynolds DM, Li L, et al. Identification of Pkd2l, a human Pkd2-related gene: Tissue-specific expression and mapping to chromosome 10q25. Genomics (1998) 54(3):564–8. doi: 10.1006/geno.1998.5618
69. Murakami M, Ohba T, Xu F, Shida S, Satoh E, Ono K, et al. Genomic organization and functional analysis of murine Pkd2l1. J Biol Chem (2005) 280(7):5626–35. doi: 10.1074/jbc.M411496200
70. Zhang HW, Lin ZX, Tung YS, Kwan TH, Mok CK, Leung C, et al. Cordyceps sinensis (a traditional Chinese medicine) for treating chronic kidney disease. Cochrane Database Syst Rev (2014) 12):CD008353. doi: 10.1002/14651858.CD008353.pub2
71. Zhu JS, Halpern GM, Jones K. The scientific rediscovery of a precious ancient Chinese herbal regimen: Cordyceps sinensis: Part ii. J Altern Complement Med (1998) 4(4):429–57. doi: 10.1089/acm.1998.4.429
72. Cicha I, Regler M, Urschel K, Goppelt-Struebe M, Daniel WG, Garlichs CD. Resveratrol inhibits monocytic cell chemotaxis to mcp-1 and prevents spontaneous endothelial cell migration through rho kinase-dependent mechanism. J Atheroscler Thromb (2011) 18(12):1031–42. doi: 10.5551/jat.8136
73. Wang Y, Yin H, Lv X, Wang Y, Gao H, Wang M. Protection of chronic renal failure by a polysaccharide from cordyceps sinensis. Fitoterapia (2010) 81(5):397–402. doi: 10.1016/j.fitote.2009.11.008
74. Shahed AR, Kim SI, Shoskes DA. Down-regulation of apoptotic and inflammatory genes by cordyceps sinensis extract in rat kidney following Ischemia/Reperfusion. Transplant Proc (2001) 33(6):2986–7. doi: 10.1016/S0041-1345(01)02282-5
75. Fujita T, Inoue K, Yamamoto S, Ikumoto T, Sasaki S, Toyama R, et al. Fungal metabolites. part 11. A potent immunosuppressive activity found in isaria sinclairii metabolite. J Antibiot (Tokyo) (1994) 47(2):208–15. doi: 10.7164/antibiotics.47.208
76. Liang J, Nagahashi M, Kim EY, Harikumar KB, Yamada A, Huang W-C, et al. Sphingosine-1-Phosphate links persistent Stat3 activation, chronic intestinal inflammation, and development of colitis-associated cancer. Cancer Cell (2013) 23(1):107–20. doi: 10.1016/j.ccr.2012.11.013
77. Morris ME, Zhang S. Flavonoid-drug interactions: Effects of flavonoids on abc transporters. Life Sci (2006) 78(18):2116–30. doi: 10.1016/j.lfs.2005.12.003
78. Russo M, Spagnuolo C, Tedesco I, Bilotto S, Russo GL. The flavonoid quercetin in disease prevention and therapy: Facts and fancies. Biochem Pharmacol (2012) 83(1):6-15. doi: 10.1016/j.bcp.2011.08.010
79. Cai X, Fang Z, Dou J, Yu A, Zhai G. Bioavailability of quercetin: Problems and promises. Curr Med Chem (2013) 20(20):2572–82. doi: 10.2174/09298673113209990120
80. Dajas F. Life or death: Neuroprotective and anticancer effects of quercetin. J Ethnopharmacol (2012) 143(2):383–96. doi: 10.1016/j.jep.2012.07.005
81. Santoso NG, Cebotaru L, Guggino WB. Polycystin-1, 2, and Stim1 interact with Ip(3)R to modulate er Ca release through the Pi3k/Akt pathway. Cell Physiol Biochem (2011) 27(6):715–26. doi: 10.1159/000330080
82. de Stephanis L, Bonon A, Varani K, Lanza G, Gafà R, Pinton P, et al. Double inhibition of camp and mtor signalling may potentiate the reduction of cell growth in adpkd cells. Clin Exp Nephrol (2017) 21(2):203–11. doi: 10.1007/s10157-016-1289-1
83. Jia L, Liu F, Hansen SH, Ter Beest MBA, Zegers MMP. Distinct roles of cadherin-6 and e-cadherin in tubulogenesis and lumen formation. Mol Biol Cell (2011) 22(12):2031–41. doi: 10.1091/mbc.E11-01-0038
84. Nagaoka T, Inutsuka A, Begum K, Bin hafiz Km, Kishi M. Vangl2 regulates e-cadherin in epithelial cells. Sci Rep (2014) 4:6940. doi: 10.1038/srep06940
85. Lee WH, Choong LY, Jin TH, Mon NN, Chong S, Liew CS, et al. Trpv4 plays a role in breast cancer cell migration Via Ca-dependent activation of akt and downregulation of e-cadherin cell cortex protein. Oncogenesis (2017) 6(5):e338. doi: 10.1038/oncsis.2017.39
86. Zeng W, Jin L, Zhang F, Zhang C, Liang W. Naringenin as a potential immunomodulator in therapeutics. Pharmacol Res (2018) 135:122–6. doi: 10.1016/j.phrs.2018.08.002
87. Meiyanto E, Hermawan A. Anindyajati. natural products for cancer-targeted therapy: Citrus flavonoids as potent chemopreventive agents. Asian Pac J Cancer Prev (2012) 13(2):427–36. doi: 10.7314/apjcp.2012.13.2.427
88. Gonçalves LM, Valente IM, Rodrigues JA. An overview on cardamonin. J Med Food (2014) 17(6):633–40. doi: 10.1089/jmf.2013.0061
89. Voon F-L, Sulaiman MR, Akhtar MN, Idris MF, Akira A, Perimal EK, et al. Cardamonin (2',4'-Dihydroxy-6'-Methoxychalcone) isolated from boesenbergia rotunda (L.) mansf. inhibits cfa-induced rheumatoid arthritis in rats. Eur J Pharmacol (2017) 794:127–34. doi: 10.1016/j.ejphar.2016.11.009
90. Wang K, Lv Q, Miao Y-M, Qiao S-M, Dai Y, Wei Z-F. Cardamonin, a natural flavone, alleviates inflammatory bowel disease by the inhibition of Nlrp3 inflammasome activation Via an Ahr/Nrf2/Nqo1 pathway. Biochem Pharmacol (2018) 155:494–509. doi: 10.1016/j.bcp.2018.07.039
91. Jin J, Qiu S, Wang P, Liang X, Huang F, Wu H, et al. Cardamonin inhibits breast cancer growth by repressing hif-1α-Dependent metabolic reprogramming. J Exp Clin Cancer Res (2019) 38(1):377. doi: 10.1186/s13046-019-1351-4
92. Norman J. Fibrosis and progression of autosomal dominant polycystic kidney disease (Adpkd). Biochim Biophys Acta (2011) 1812(10):1327–36. doi: 10.1016/j.bbadis.2011.06.012
93. Liu Y, Chen Y, Lin L, Li H. Gambogic acid as a candidate for cancer therapy: A review. Int J Nanomedicine (2020) 15:10385–99. doi: 10.2147/IJN.S277645
94. Wang T, Du J, Kong D, Yang G, Zhou Q, You F, et al. Gambogic acid inhibits proliferation and induces apoptosis of human acute T−Cell leukemia cells by inducing autophagy and downregulating B−Catenin signaling pathway: Mechanisms underlying the effect of gambogic acid on T−All cells. Oncol Rep (2020) 44(4):1747–57. doi: 10.3892/or.2020.7726
95. Li C-Y, Wang Q, Wang X-M, Li G-X, Shen S, Wei X-L. Gambogic acid exhibits anti-metastatic activity on malignant melanoma mainly through inhibition of Pi3k/Akt and erk signaling pathways. Eur J Pharmacol (2019) 864:172719. doi: 10.1016/j.ejphar.2019.172719
96. Yi T, Yi Z, Cho S-G, Luo J, Pandey MK, Aggarwal BB, et al. Gambogic acid inhibits angiogenesis and prostate tumor growth by suppressing vascular endothelial growth factor receptor 2 signaling. Cancer Res (2008) 68(6):1843–50. doi: 10.1158/0008-5472.CAN-07-5944
97. Wang QL, Yang DZ, Lv C. Anti−Inflammatory effects of gambogic acid in murine Collagen−Induced arthritis through Pi3k/Akt signaling pathway. Mol Med Rep (2018) 17(3):4791–6. doi: 10.3892/mmr.2018.8389
98. Zhao T, Wang H-J, Zhao W-W, Sun Y-L, Hu L-K. Gambogic acid improves non-small cell lung cancer progression by inhibition of mtor signaling pathway. Kaohsiung J Med Sci (2017) 33(11):543–9. doi: 10.1016/j.kjms.2017.06.013
99. Yu J, Wang W, Yao W, Yang Z, Gao P, Liu M, et al. Gambogic acid affects escc progression through regulation of Pi3k/Akt/Mtor signal pathway. J Cancer (2020) 11(19):5568–77. doi: 10.7150/jca.41115
100. He X-y, Liu X-j, Chen X, Bian L-g, Zhao W-g, Shen J-k, et al. Gambogic acid induces egfr degradation and Akt/Mtorc1 inhibition through ampk dependent-Lrig1 upregulation in cultured U87 glioma cells. Biochem Biophys Res Commun (2013) 435(3):397–402. doi: 10.1016/j.bbrc.2013.04.099
101. Khayyal MT, el-Ghazaly MA, Abdallah DM, Nassar NN, Okpanyi SN, Kreuter M-H. Blood pressure lowering effect of an olive leaf extract (Olea europaea) in l-name induced hypertension in rats. Arzneimittelforschung (2002) 52(11):797–802. doi: 10.1055/s-0031-1299970
102. Zarzuelo A, Duarte J, Jiménez J, González M, Utrilla MP. Vasodilator effect of olive leaf. Planta Med (1991) 57(5):417–9. doi: 10.1055/s-2006-960138
103. Gorzynik-Debicka M, Przychodzen P, Cappello F, Kuban-Jankowska A, Marino Gammazza A, Knap N, et al. Potential health benefits of olive oil and plant polyphenols. Int J Mol Sci (2018) 19(3):686. doi: 10.3390/ijms19030686
104. Goldsmith CD, Vuong QV, Sadeqzadeh E, Stathopoulos CE, Roach PD, Scarlett CJ. Phytochemical properties and anti-proliferative activity of olea europaea l. leaf extracts against pancreatic cancer cells. Molecules (2015) 20(7):12992–3004. doi: 10.3390/molecules200712992
105. Samet I, Han J, Jlaiel L, Sayadi S, Isoda H. Olive (Olea europaea) leaf extract induces apoptosis and Monocyte/Macrophage differentiation in human chronic myelogenous leukemia K562 cells: Insight into the underlying mechanism. Oxid Med Cell Longev (2014) 2014:927619. doi: 10.1155/2014/927619
106. Barrajón-Catalán E, Taamalli A, Quirantes-Piné R, Roldan-Segura C, Arráez-Román D, Segura-Carretero A, et al. Differential metabolomic analysis of the potential antiproliferative mechanism of olive leaf extract on the jimt-1 breast cancer cell line. J Pharm BioMed Anal (2015) 105:156–62. doi: 10.1016/j.jpba.2014.11.048
107. Acquaviva R, Di Giacomo C, Sorrenti V, Galvano F, Santangelo R, Cardile V, et al. Antiproliferative effect of oleuropein in prostate cell lines. Int J Oncol (2012) 41(1):31–8. doi: 10.3892/ijo.2012.1428
108. Ben Sahra I, Laurent K, Giuliano S, Larbret F, Ponzio G, Gounon P, et al. Targeting cancer cell metabolism: The combination of metformin and 2-deoxyglucose induces P53-dependent apoptosis in prostate cancer cells. Cancer Res (2010) 70(6):2465–75. doi: 10.1158/0008-5472.CAN-09-2782
109. Song X, Tsakiridis E, Steinberg GR, Pei Y. Targeting amp-activated protein kinase (Ampk) for treatment of autosomal dominant polycystic kidney disease. Cell Signal (2020) 73:109704. doi: 10.1016/j.cellsig.2020.109704
110. Ong ACM, Gansevoort RT. Tameing adpkd with metformin: Safe and effective? Kidney Int (2021) 100(3):513–5. doi: 10.1016/j.kint.2021.07.021
111. Leonhard WN, Song X, Kanhai AA, Iliuta I-A, Bozovic A, Steinberg GR, et al. Salsalate, but not metformin or canagliflozin, slows kidney cyst growth in an adult-onset mouse model of polycystic kidney disease. EBioMedicine (2019) 47:436–45. doi: 10.1016/j.ebiom.2019.08.041
112. Noel P, Von Hoff DD, Saluja AK, Velagapudi M, Borazanci E, Han H. Triptolide and its derivatives as cancer therapies. Trends Pharmacol Sci (2019) 40(5):327–41. doi: 10.1016/j.tips.2019.03.002
113. Li X-J, Jiang Z-Z, Zhang L-y. Triptolide: Progress on research in pharmacodynamics and toxicology. J Ethnopharmacol (2014) 155(1):67–79. doi: 10.1016/j.jep.2014.06.006
114. Zhong Y, Deng Y, Chen Y, Chuang PY, Cijiang He J. Therapeutic use of traditional Chinese herbal medications for chronic kidney diseases. Kidney Int (2013) 84(6):1108–18. doi: 10.1038/ki.2013.276
115. Leuenroth SJ, Bencivenga N, Chahboune H, Hyder F, Crews CM. Triptolide reduces cyst formation in a neonatal to adult transition Pkd1 model of adpkd. Nephrol Dial Transplant (2010) 25(7):2187–94. doi: 10.1093/ndt/gfp777
116. Leuenroth SJ, Okuhara D, Shotwell JD, Markowitz GS, Yu Z, Somlo S, et al. Triptolide is a traditional Chinese medicine-derived inhibitor of polycystic kidney disease. Proc Natl Acad Sci U.S.A. (2007) 104(11):4389–94. doi: 10.1073/pnas.0700499104
117. Jing Y, Wu M, Zhang D, Chen D, Yang M, Mei S, et al. Triptolide delays disease progression in an adult rat model of polycystic kidney disease through the Jak2-Stat3 pathway. Am J Physiol Renal Physiol (2018) 315(3):F479–F86. doi: 10.1152/ajprenal.00329.2017
118. Chen D, Ma Y, Wang X, Yu S, Li L, Dai B, et al. Triptolide-containing formulation in patients with autosomal dominant polycystic kidney disease and proteinuria: An uncontrolled trial. Am J Kidney Dis (2014) 63(6):1070–2. doi: 10.1053/j.ajkd.2014.01.418
119. Li SP, Zhao J, Yang B. Strategies for quality control of Chinese medicines. J Pharm BioMed Anal (2011) 55(4):802–9. doi: 10.1016/j.jpba.2010.12.011
120. Liang Y, Xie P, Chau F. Chromatographic fingerprinting and related chemometric techniques for quality control of traditional Chinese medicines. J Sep Sci (2010) 33(3):410–21. doi: 10.1002/jssc.200900653
121. Pranjali Borkar VY, Tiwari RR, Samarth RM. A systematic review of potential candidates of herbal medicine in treatment of chronic kidney disease. Phytomedicine Plus (2022) 2(4). doi: 10.1016/j.phyplu.2022.100361
122. Wang S, Liu YC, Wang HY, Li JJ, Zhou ZZ. Clinical observation on the nephrotoxicity of t. regelii sprague. et takeda. China J Chin Mater (1996).
123. Xu X, Zhu R, Ying J, Zhao M, Wu X, Cao G, et al. Nephrotoxicity of herbal medicine and its prevention. Front Pharmacol (2020) 11:569551. doi: 10.3389/fphar.2020.569551
124. Solak Y, Atalay H, Polat I, Biyik Z. Colchicine treatment in autosomal dominant polycystic kidney disease: Many points in common. Med Hypotheses (2010) 74(2):314–7. doi: 10.1016/j.mehy.2009.08.041
Keywords: polycystic kidney disease, plant-derived compounds, herbal medicine, treatment, mechanism
Citation: Zhang J, Chen J, Xu J, Xue C and Mao Z (2023) Plant-derived compounds for treating autosomal dominant polycystic kidney disease. Front. Nephrol. 3:1071441. doi: 10.3389/fneph.2023.1071441
Received: 16 October 2022; Accepted: 12 January 2023;
Published: 03 February 2023.
Edited by:
Elisabetta Versino, University of Turin, ItalyReviewed by:
Veena Puri, Panjab University, IndiaRavindra M. Samartha, Bhopal Memorial Hospital & Research Centre, India
Copyright © 2023 Zhang, Chen, Xu, Xue and Mao. This is an open-access article distributed under the terms of the Creative Commons Attribution License (CC BY). The use, distribution or reproduction in other forums is permitted, provided the original author(s) and the copyright owner(s) are credited and that the original publication in this journal is cited, in accordance with accepted academic practice. No use, distribution or reproduction is permitted which does not comply with these terms.
*Correspondence: Zhiguo Mao, bWFvemhpZ3VvOTNAMTI2LmNvbQ==; Cheng Xue, Y2hlbmd4aWExNTY4QDEyNi5jb20=
†These authors have contributed equally to this work