- 1Nephrology Service, Hospital Civil de Guadalajara Fray Antonio Alcalde, Guadalajara, Mexico
- 2University Center for Health Sciences, University of Guadalajara, Guadalajara, Mexico
- 3Nephrology Service, Regional General Hospital 46, Mexican Social Security Institute, Guadalajara, Mexico
Within the multiple communication pathways of the intestine-kidney axis, one of the most important pathways is the interaction between the commensals of the intestinal microbiome, through the production of short-chain fatty acids, and the segments of the nephron. These interactions maintain a perfect environmental balance. During AKI, there are negative repercussions in all organs, and the systemic interconnection is related in part to the intense inflammation and the uremic environment that this syndrome generates. For example, in the intestine, the microbiome is severely affected, with a decrease in benign bacteria that promote anti-inflammatory effects and an increase in negative, pro-inflammatory bacteria. This scenario of intestinal dysbiosis widens the inflammatory loop that favors worsening kidney function and the probability of dying. It is possible that the manipulation of the intestinal microbiome with probiotics, prebiotics and symbiotics is a reasonable therapeutic goal for AKI.
Introduction
The intestinal microbiome is made up of millions of bacteria, viruses, fungi and protozoa that interact in health and disease processes. This dynamic and symbiotic relationship modifies the physiological responses of practically any organ and system of our body (1). Studies such as the Human Microbiome Project (HMP) and the Metagenomics of the Human Intestinal Tract (Meta-HIT) have shown how complex the microbiome is. These studies have also presented ample information regarding the discovery of this fascinating interaction, which has coexisted during evolution of the human being (2, 3).
The intestinal microbiota is made up of 100 trillion microorganisms that can be divided into 500 to 1,000 species of bacteria that are specific to each individual. However, there are certain similarities between people who live in the same communities and between families and those who share aspects of daily life, such as diet. These bacteria change according to physiological needs and aging (4). They are cataloged into groups of Bacteroidetes, Firmicutes, and Actinobacteria (5), which represent >90% of all species.
In nonpathological states, intestinal microorganisms (Firmicutes, Bacteroidetes, Actinobacteria, Verrucomicrobia, Proteobacteria) metabolize the components of the diet through proteases, galactosidases, hexosaminidases, tryptophanases and other enzymes (6). Carbohydrates are subjected to a fermentation process and are used as a source of energy. They contribute to the synthesis of short-chain fatty acids (SCFAs), such as acetate, propionate and butyrate (7). These products have a profound impact on the cells of the renal tubule and on the immune system (6). In addition to being a source of energy, they also serve as signaling molecules (7).
Four SCFA receptors have been described in the kidney (Gpr41, Gpr43, Gpr109a, and Olfr78). Gpr41 and Gpr43 are the most studied, they have important physiological roles in the metabolism of renal cells, renal arteries and the immune system, respectively. Olfr78 receptors that are more expressed in afferent renal arteriole and in large renal vessels, promoting hemodynamic changes. Finally, Gpr109a, is the least studied and its physiological effects are not yet known. All of them have the potential to promote cell proliferation, apoptosis, and histone inhibition (7).
During pathological processes, such as inflammation or chronic diseases, the microbiome suffers alterations in the quality and quantity of its components. The bacteria considered benign decrease, and the pathological bacteria trend to proliferate. This generates dysbiosis, which is an event that has been fully identified in patients with impaired renal function (8).
Acute kidney injury (AKI) is a condition in which kidney function deteriorates rapidly; it is a very common syndrome that occurs in 22% of all hospitalized patients (9). It carries a high mortality rate of approximately 23%, and in severe cases, according to the KDIGO classification (10), it can reach a mortality of up to 45%. The clinical outcomes are even worse in emerging countries (11). By its nature, AKI is a highly inflammatory pathology (12) with systemic involvement that affects all organs with profound repercussions on the functioning of all of them. The most common etiology of AKI is sepsis; sepsis increases the risk of developing AKI by up to 210% (13). Compared to other etiologies, AKI associated with sepsis has a poor clinical course, as it has a mortality rate of 49% in the hospital, 45% during intensive care, 36% at 28 days, and 64% at 3 months (14).
There are no specific treatments for AKI, and to date, only its complications have been treated through kidney replacement therapy. However, this treatment does not radically change the trajectory of these patients. The dire need for a treatment for AKI has been suggested as a priority research area by expert consensus (15). To date, various therapeutic targets have failed to improve renal function and mortality in these patients (16–21).
The relationship between intestinal dysbiosis and AKI is bidirectional, that is, dysbiosis generates AKI plausibly by inflammation and AKI promotes and worsens dysbiosis.
Intestinal Dysbiosis and AKI
Among the many hypotheses about the mechanisms of injury in AKI, the one related to intense systemic inflammation is the best known. AKI directly or indirectly alters the composition of the intestinal microbiome. Then, the permeability of the intestine is dramatically altered by edema, hypoperfusion and ischemia, leading to a “leaky gut” that activates the immune response by bacterial translocation (1). This response generates and amplifies systemic inflammation. During this process, the gut microbiota interacts with sepsis and AKI independently.
In a symbiotic (normal) intestinal environment in which commensal bacteria proliferate and generate SCFAs, the intestinal integrity is strong and predominantly anti-inflammatory. However, in a uremic environment due to AKI or chronic kidney disease (CKD), the intestinal integrity is degenerated. Thus, intestinal dysbiosis occurs with the increased production of uremic toxins, resulting in a decrease in saccharolytic bacteria and proliferation of proteolytic bacteria. There are also reports on bacterial translocation (elevated during AKI) as a consequence of uremia (22). Urea is broken down in the intestine by bacteria that have the enzyme urease and is converted into ammonium and ammonium hydroxide. At the epithelial junctions of the intestine, these products increase transluminal flow and the translocation of bacteria from the intestine to the systemic circulation. Thus, the activation of Th17, Th1 cells, B cells and macrophages, a proinflammatory environment are promoted (6). These events of dysbiosis and increased inflammation are believed to be synergistic between AKI and sepsis, and are referred to as gut-kidney interactions.
In the renal tubule, the microbiota communicates with the ligands FFA3, FFA2 and OR51E2 via SCFAs. This interaction produces a change in cell metabolism (7). It has been observed that the systemic inflammatory response decreases up to 10,000 times the amount of intestinal bacteria considered beneficial, such as Bifidobacterium and Lactobacillus. In contrast, it increases up to 100 times the number of pathogenic bacteria, such as Staphylococcus (23). This translates into a decrease in the generation of SCFAs, assessed by an increase in the fecal pH. The fecal pH of patients with inflammation can reach up to 7.4, while a normal fecal pH is 6.6 in healthy patients (reflecting dysbiosis) (24).
It has also been reported that the number of obligate and facultative anaerobes is related to mortality in critically ill patients (24). Along these lines, in a cohort of 82 soldiers who had suffered AKI during combat, it was established through metabolomics measurement (84 metabolites) that the most important determinants for the severity of their AKI and the requirement for kidney replacement therapy are directly related to the intestinal microbiome by the expression of indoxyl sulfate, indole-3-acetate, hippurate and phenylacetylglycine (25).
Experimental Evidence in Animal Models on the Interaction and Modulation of Dysbiosis and AKI
The evidence obtained through experimental studies that justifies the hypothesis of the interaction between intestinal microbiome, dysbiosis and AKI is extensive (Table 1).
In a mouse model of renal ischemia/reperfusion, the proliferation of bacteria such as Rothia sp., and Staphylococcus sp. was consistent with the severity of AKI and correlated linearly with the elevation in creatinine and urea (33). In rats with microbiota depletion prior to an episode of AKI due to ischemia reperfusion, there was a notable decrease in markers of inflammation and tubular damage after the event. In mice depleted of intestinal bacteria with the use of antibiotics, the percentage of expression of the classic inflammatory markers and renal macrophages did not change despite a reduction in chemokine receptors. Likewise, in mice without intestinal microbiota, the benefit that they had presented was reversed once a fecal transplant was performed (32).
In another experimental model, AKI was induced in rats by urosepsis via E. coli inoculation. In this model, the administration of probiotics (Lactobacillus acidophilus and Bifidobacterium) one month before and two months after renal insult significantly attenuated the histological evidence of kidney injury (34).
When comparing sham rats with those that underwent cecal ligation, the administration of Lactobacillus rhamnosus GG and Bifidobacterium longum for 7 days was associated with a 40% decrease in the risk of dying (35).
Another experimental study administered SCFAs to rats after AKI in an ischemia/reperfusion model and found improvement in renal function and tissue damage. They observed a decrease in all inflammatory factors, such as TNF alpha, IL-6, IL-1b and MCP-1 (26).
Another study found that the protection conferred by acetate administration was associated with a decrease in the production of reactive oxygen species, a local decrease in cytokines and chemokines, lower levels of receptor messenger RNA, toll-type 4 and its ligand (biglycan), and lower activation of NF-kB.
Similarly, before the induction of AKI by cisplatin, the administration of lactic acid bacteria (Lactobacillus salivarius) maintained lower levels of creatinine, urea, inflammatory markers, and tissue damage scores (36); additionally, the integrity of the intestinal wall barrier was maintained.
Human Clinical Evidence of Intestinal Dysbiosis in AKI
Studies on intestinal dysbiosis in humans with AKI are very scarce, but some findings suggest that the biology of humans is similar to that of the experimental studies. It may be that the influence of the microbiome on AKI is based on the “Hygiene hypothesis”, which establishes how the microbiome (physiological) induces less inflammation (37). In addition, the same uremia generated with AKI promotes dysbiosis, and urea degrades the intestinal mucosa, which also facilitates this event. As an example, 13 patients with AKI demonstrated increases in the production of D-amino acids (D-serine) produced by SCFAs (abundant in symbiosis). This is an event that could represent a kidney protection mechanism by intestinal bacteria before kidney insult (31). Patients with AKI and sepsis receive broad-spectrum antibiotics, which have a profound impact on the gut microbiota by changing its conformation (38).
In CKD, the quantity of uremic toxins derived from the colon, such as indoxyl sulfate (IS), increases; however, it seems that this situation also occurs in AKI.
In a prospective cohort of 262 patients with AKI, an association was identified between high levels of IS and mortality, increasing this outcome threefold (39). In another cohort of 194 patients in an intensive care unit, improvement in the RIFLE score in AKI was observed in those who had a decrease in these colon-derived toxins (40). Multiple studies have been conducted to assess the effect of symbiosis on IS and p-cresol-sulfate concentrations and other markers in predialysis CKD patients.
Therapeutic Advances
Probiotics are live microorganisms that, when administered in the appropriate amounts, offer benefits to the host. The combination of both prebiotics and probiotics is called symbiotic, and they are usually administered in food supplements (41, 42). Until now, the experimental studies are encouraging since they have been favorable in reducing systemic inflammation, improving renal function parameters and even histological damage (Figure 1). The information derived from studies in patients with CKD supports the rationale for testing this therapeutic target in the context of AKI, a clinical entity that carries high morbidity and mortality. Recently, Rydzewska-Rosołowska et al. (1) conducted a systematic review identifying only 5 human studies (three prospective and two retrospective); however, up until the time of this review, there were no clinical trials with the use of probiotics in AKI (24, 43).
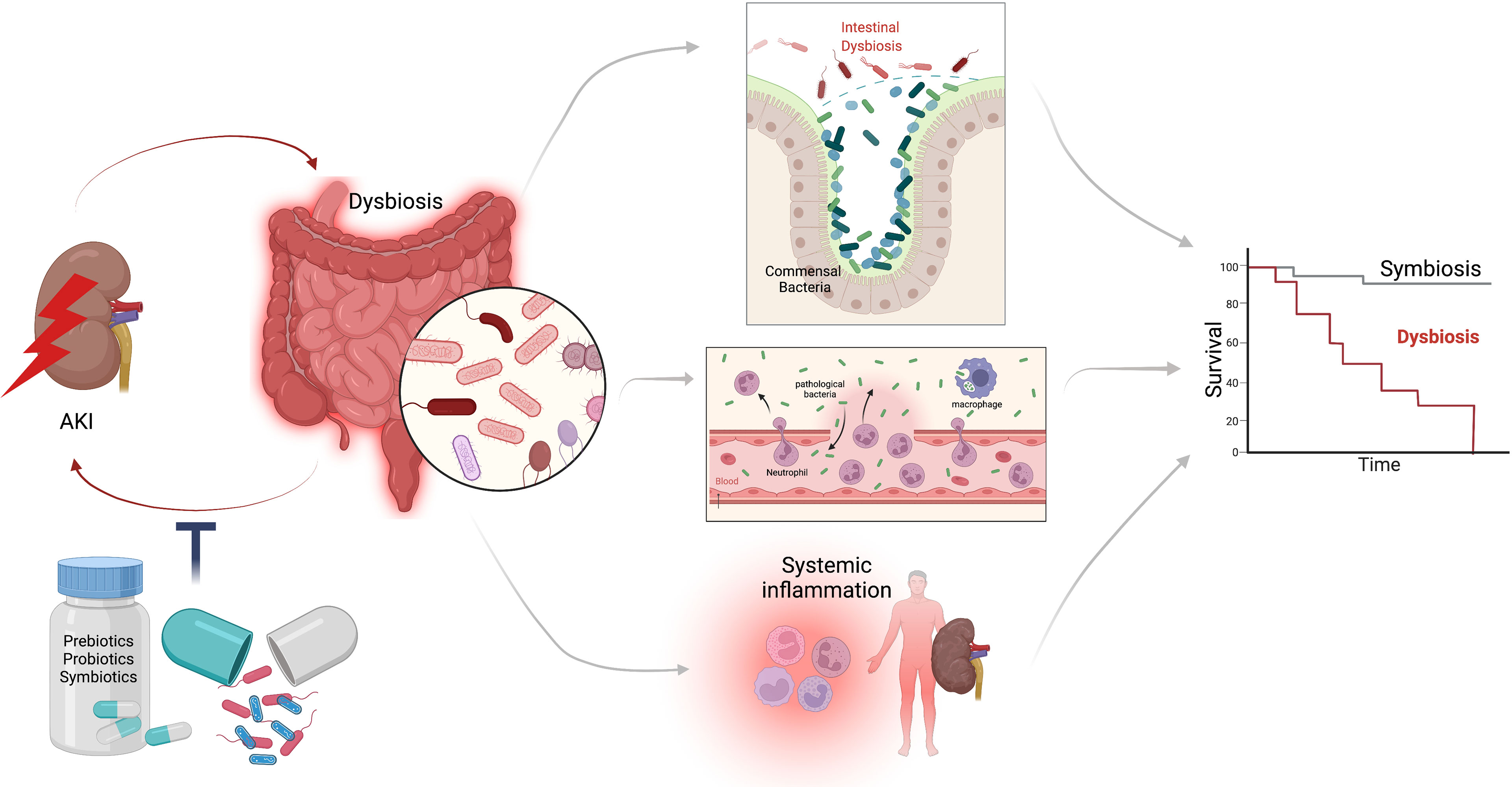
Figure 1 Interaction between AKI and intestinal dysbiosis. During AKI multiple mechanisms lead to alterations in the conformation of the intestinal microbiome, promoting the proliferation of pathogenic bacteria and the decrease of beneficial bacteria, an event known as dysbiosis; which generates rupture of the intestinal barrier, bacterial translocation, and systemic inflammation; further worsening kidney function, preventing its recovery. Dysbiosis-AKI loop has been associated with worse survival. It is possible that the manipulation of the intestinal microbiome with prebiotics, probiotics and symbiotics can attenuate dysbiosis and thus improve the clinical outcomes in AKI patients.
Conclusions and Perspective
The intestinal dysbiosis that is generated during AKI deteriorates the clinical course and amplifies kidney damage through multiple pathways. Dysbiosis has systemic involvement, and inflammation seems to be the most involved pathway of damage. Modifying clinical outcomes in AKI through modulation of the microbiome is biologically plausible, and we could manipulate that environment. Probiotics are a good option; probiotics are live microorganisms that, when administered in the appropriate amounts, offer benefits to the host. Furthermore, despite years of clinical research, no treatment has been discovered that truly modifies the clinical course of AKI. We are running a randomized, double-blinded, placebo-controlled clinical trial (Clinical Trails registry: NCT03877081), in which we will evaluate the effect of the administration of prebiotics, probiotics and symbiotics for 7 days in patients with AKI induced by sepsis. We are waiting for their results, which may elucidate the fascinating association between the gut microbiota and AKI.
Author Contributions
JSCI, LYVG and AMGG contribute equally to elaborate this mini-review. All authors contributed to the article and approved the submitted version.
Conflict of Interest
The authors declare that the research was conducted in the absence of any commercial or financial relationships that could be construed as a potential conflict of interest.
Publisher’s Note
All claims expressed in this article are solely those of the authors and do not necessarily represent those of their affiliated organizations, or those of the publisher, the editors and the reviewers. Any product that may be evaluated in this article, or claim that may be made by its manufacturer, is not guaranteed or endorsed by the publisher.
References
1. Rydzewska-Rosołowska A, Sroka N, Kakareko K, Rosołowski M, Zbroch E, Hryszko T. The Links Between Microbiome and Uremic Toxins in Acute Kidney Injury: Beyond Gut Feeling-A Systematic Review. Toxins (Basel) (2020) 12(12):788. doi: 10.3390/toxins12120788
2. Qin J, Li R, Raes J, Arumugam M, Burgdorf KS, Manichanh C, et al. A Human Gut Microbial Gene Catalogue Established by Metagenomic Sequencing. Nature (2010) 464(7285):59–65. doi: 10.1038/nature08821
3. Gevers D, Knight R, Petrosino JF, Huang K, McGuire AL, Birren BW, et al. The Human Microbiome Project: A Community Resource for the Healthy Human Microbiome. PloS Biol (2012) 10(8):e1001377. doi: 10.1371/journal.pbio.1001377
4. Xu J, Gordon JI. Honor Thy Symbionts. Proc Natl Acad Sci USA (2003) 100(18):10452–9. doi: 10.1073/pnas.1734063100
5. Tremaroli V, Backhed F. Functional Interactions Between the Gut Microbiota and Host Metabolism. Nature (2012) 489(7415):242–9. doi: 10.1038/nature11552
6. Ramezani A, Raj DS. The Gut Microbiome, Kidney Disease, and Targeted Interventions. J Am Soc Nephrol (2014) 25(4):657–70. doi: 10.1681/ASN.2013080905
7. Pluznick JL. Gut Microbiota in Renal Physiology: Focus on Short-Chain Fatty Acids and Their Receptors. Kidney Int (2016) 90(6):1191–8. doi: 10.1016/j.kint.2016.06.033
8. Ramezani A, Massy ZA, Meijers B, Evenepoel P, Vanholder R, Raj DS. Role of the Gut Microbiome in Uremia: A Potential Therapeutic Target. Am J Kidney Dis (2016) 67(3):483–98. doi: 10.1053/j.ajkd.2015.09.027
9. Chávez-Iñiguez JS, Madero M. Global Perspectives in Acute Kidney Injury: Mexico. Kidney (2022) 360:1–3. doi: 10.34067/KID.0006592021
10. Kellum JA, Lameire N, Aspelin P, Barsoum RS, Burdmann EA, Goldstein SL, et al. KDIGO Clinical Practice Guideline for Acute Kidney Injury. Kidney Int Suppl (2012) 2(1):1–138. doi: 10.1038/kisup.2012.1
11. Komaru Y, Inokuchi R, Iwagami M, Matsuura R, Hamasaki Y, Nangaku M, et al. Correlation Between the Incidence and Attributable Mortality Fraction of Acute Kidney Injury: A Systematic Review. Blood Purif (2020) 49(4):386–93. doi: 10.1159/000505568
12. Peerapornratana S, Manrique-Caballero CL, Gómez H, Kellum JA. Acute Kidney Injury From Sepsis: Current Concepts, Epidemiology, Pathophysiology, Prevention and Treatment. Kidney Int (2019) 96(5):1083–99. doi: 10.1016/j.kint.2019.05.026
13. Poston JT, Koyner JL. Sepsis Associated Acute Kidney Injury. BMJ (2019) 364:k4891. doi: 10.1136/bmj.k4891
14. Liu J, Xie H, Ye Z, Li F, Wang L. Rates, Predictors, and Mortality of Sepsis-Associated Acute Kidney Injury: A Systematic Review and Meta-Analysis. BMC Nephrol (2020) 21(1):318. doi: 10.1186/s12882-020-01974-8
15. Chawla LS, Bellomo R, Bihorac A, Goldstein SL, Siew ED, Bagshaw SM, et al. Acute Kidney Disease and Renal Recovery: Consensus Report of the Acute Disease Quality Initiative (ADQI) 16 Workgroup. Nat Rev Nephrol (2017) 13(4):241–57. doi: 10.1038/nrneph.2017.2
16. Chávez-Iñiguez JS, Poo JL, Ibarra-Estrada M, García-Benavides L, Navarro-Blackaller G, Cervantes-Sánchez C, et al. Effect of Prolonged-Release Pirfenidone on Renal Function in Septic Acute Kidney Injury Patients: A Double-Blind Placebo-Controlled Clinical Trial. Int J Nephrol (2021) 2021:8833278. doi: 10.1155/2021/8833278
17. Pickkers P, Mehta RL, Murray PT, Joannidis M, Molitoris BA, Kellum JA, et al. Effect of Human Recombinant Alkaline Phosphatase on 7-Day Creatinine Clearance in Patients With Sepsis-Associated Acute Kidney Injury: A Randomized Clinical Trial. JAMA (2018) 320(19):1998–2009. doi: 10.1001/jama.2018.14283
18. Wang W, Bansal S, Falk S, Ljubanovic D, Schrier R. Ghrelin Protects Mice Against Endotoxemia-Induced Acute Kidney Injury. Am J Physiol Renal Physiol (2009) 297:F1032–7. doi: 10.1152/ajprenal.00044.2009
19. Simon F, Giudici R, Scheuerle A, Groger M, Asfar P, Vogt JA, et al. Comparison of Cardiac, Hepatic, and Renal Effects of Arginine Vasopressin and Noradrenaline During Porcine Fecal Peritonitis: A Randomized Controlled Trial. Crit Care (2009) 13:R113. doi: 10.1186/cc7959
20. Lee HT, Kim M, Joo JD, Gallos G, Chen JF, Emala CW. A3 Adenosine Receptor Activation Decreases Mortality and Renal and Hepatic Injury in Murine Septic Peritonitis. Am J Physiol Regul Integr Comp Physiol (2006) 291:R959–69. doi: 10.1152/ajpregu.00034.2006
21. Bahlmann FH, Fliser D. Erythropoietin and Renoprotection. Curr Opin Nephrol Hypertens (2009) 18:15–20. doi: 10.1097/MNH.0b013e32831a9dde
22. Zhang J, Ankawi G, Sun J, Digvijay K, Yin Y, Rosner MH, et al. Gut-Kidney Crosstalk in Septic Acute Kidney Injury. Crit Care (2018) 22(1):117. doi: 10.1186/s13054-018-2040-y
23. Shimizu K, Ogura H, Hamasaki T, Goto M, Tasaki O, Asahara T, et al. Altered Gut Flora are Associated With Septic Complications and Death in Critically Ill Patients With Systemic Inflammatory Response Syndrome. Dig Dis Sci (2011) 56(4):1171–7. doi: 10.1007/s10620-010-1418-8
24. Shimizu K, Ogura H, Asahara T, Nomoto K, Morotomi M, Tasaki O, et al. Probiotic/synbiotic Therapy for Treating Critically Ill Patients From a Gut Microbiota Perspective. Dig Dis Sci (2013) 58(1):23–32. doi: 10.1007/s10620-012-2334-x
25. Gisewhite S, Stewart IJ, Beilman G, Lusczek E. Urinary Metabolites Predict Mortality or Need for Renal Replacement Therapy After Combat Injury. Crit Care (2021) 25(1):119. doi: 10.1186/s13054-021-03544-2
26. Andrade-Oliveira V, Amano MT, Correa-Costa M, Castoldi A, Felizardo RJ, de Almeida DC, et al. Gut Bacteria Products Prevent AKI Induced by Ischemia-Reperfusion. J Am Soc Nephrol (2015) 26(8):1877–88. doi: 10.1681/ASN.2014030288
27. Park J, Goergen CJ, HogenEsch H, Kim CH. Chronically Elevated Levels of Short-Chain Fatty Acids Induce T Cell-Mediated Ureteritis and Hydronephrosis. J Immunol (2016) 196(5):2388–400. doi: 10.4049/jimmunol.1502046
28. Sun X, Zhang B, Hong X, Zhang X, Kong X. Histone Deacetylase Inhibitor, Sodium Butyrate, Attenuates Gentamicin-Induced Nephrotoxicity by Increasing Prohibitin Protein Expression in Rats. Eur J Pharmacol (2013) 707(1-3):147–54. doi: 10.1016/j.ejphar.2013.03.018
29. Machado RA, Constantino Lde S, Tomasi CD, Rojas HA, Vuolo FS, Vitto MF, et al. Sodium Butyrate Decreases the Activation of NF-κb Reducing Inflammation and Oxidative Damage in the Kidney of Rats Subjected to Contrast-Induced Nephropathy. Nephrol Dial Transplant (2012) 27(8):3136–40. doi: 10.1093/ndt/gfr807
30. Jang HR, Gandolfo MT, Ko GJ, Satpute S, Racusen L, Rabb H. Early Exposure to Germs Modifies Kidney Damage and Inflammation After Experimental Ischemia-Reperfusion Injury. American Journal of Physiology. Renal Physiol (2009) 297(5):F1457–65. doi: 10.1152/ajprenal.90769.2008
31. Nakade Y, Iwata Y, Furuichi K, Mita M, Hamase K, Konno R, et al. Gut Microbiota-Derived D-Serine Protects Against Acute Kidney Injury. JCI Insight (2018) 3(20):e97957. doi: 10.1172/jci.insight.97957
32. Emal D, Rampanelli E, Stroo I, Butter LM, Teske GJ, Claessen N, et al. Depletion of Gut Microbiota Protects Against Renal Ischemia-Reperfusion Injury. J Am Soc Nephrol (2017) 28(5):1450–61. doi: 10.1681/ASN.2016030255
33. Andrianova NV, Popkov VA, Klimenko NS, Tyakht AV, Baydakova GV, Frolova OY, et al. Microbiome-Metabolome Signature of Acute Kidney Injury. Metabolites (2020) 10(4):142. doi: 10.3390/metabo10040142
34. Sabetkish N, Sabetkish S, Mohseni MJ, Kajbafzadeh AM. Prevention of Renal Scarring in Acute Pyelonephritis by Probiotic Therapy: An Experimental Study. Probiotics Antimicrob Proteins (2019) 11(1):158–64. doi: 10.1007/s12602-017-9363-x
35. Khailova L, Frank DN, Dominguez JA, Wischmeyer PE. Probiotic Administration Reduces Mortality and Improves Intestinal Epithelial Homeostasis in Experimental Sepsis. Anesthesiology (2013) 119(1):166–77. doi: 10.1097/ALN.0b013e318291c2fc
36. Lee TH, Park D, Kim YJ, Lee I, Kim S, Oh CT, et al. Lactobacillus Salivarius BP121 Prevents Cisplatin‐Induced Acute Kidney Injury by Inhibition of Uremic Toxins Such as Indoxyl Sulfate and P‐Cresol Sulfate via Alleviating Dysbiosis. Int J Mol Med (2020) 45(4):1130–40. doi: 10.3892/ijmm.2020.4495
37. Martinez FD. The Coming-of-Age of the Hygiene Hypothesis. Respir Res (2001) 2(3):129–32. doi: 10.1186/rr48
38. Ferrer M, Méndez-García C, Rojo D, Barbas C, Moya A. Antibiotic Use and Microbiome Function. Biochem Pharmacol (2017) 134:114–26. doi: 10.1016/j.bcp.2016.09.007
39. Wang W, Hao G, Pan Y, Ma S, Yang T, Shi P, et al. Serum Indoxyl Sulfate is Associated With Mortality in Hospital-Acquired Acute Kidney Injury: A Prospective Cohort Study. BMC Nephrol (2019) 20(1):57. doi: 10.1186/s12882-019-1238-9
40. Veldeman L, Vanmassenhove J, Van Biesen W, Massy ZA, Liabeuf S, Glorieux G, et al. Evolution of Protein-Bound Uremic Toxins Indoxyl Sulphate and P-Cresyl Sulphate in Acute Kidney Injury. Int Urol Nephrol (2019) 51(2):293–302. doi: 10.1007/s11255-018-2056-x
41. Guida B, German R, Trio R, Russo D, Memoli B, Grumetto L, et al. Effect of Short-Term Synbiotic Treatment on Plasma P-Cresol Levels in Patients With Chronic Renal Failure: A Randomized Clinical Trial. Nutr Metab Cardiovasc Dis (2014) 24:1043–9. doi: 10.1016/j.numecd.2014.04.007
42. Rossi M, Johnson DW, Morrison M, Pascoe EM, Coombes JS, Forbes JM, et al. Synbiotics Easing Renal Failure by Improving Gut Microbiology (SYNERGY): A Randomized Trial. Clin J Am Soc Nephrol (2016) 11:223–31. doi: 10.2215/CJN.05240515
Keywords: AKI, acute kidney injury, dysbiosis, intestinal microbiota, inflammation, prebiotics, probiotic
Citation: Chávez-Iñiguez JS, Villegas-Gutiérrez LY and Gallardo-González AM (2022) Acute Kidney Injury and Intestinal Dysbiosis. Front. Nephrol. 2:916151. doi: 10.3389/fneph.2022.916151
Received: 08 April 2022; Accepted: 07 June 2022;
Published: 07 July 2022.
Edited by:
Jose Ernesto Belizario, University of São Paulo, BrazilReviewed by:
Yvelynne Kelly, Tallaght University Hospital, IrelandMichael Thomsen, The University of Sydney, Australia
Copyright © 2022 Chávez-Iñiguez, Villegas-Gutiérrez and Gallardo-González. This is an open-access article distributed under the terms of the Creative Commons Attribution License (CC BY). The use, distribution or reproduction in other forums is permitted, provided the original author(s) and the copyright owner(s) are credited and that the original publication in this journal is cited, in accordance with accepted academic practice. No use, distribution or reproduction is permitted which does not comply with these terms.
*Correspondence: Jonathan Samuel Chávez-Iñiguez, am9uYXJjaGlfMTBAaG90bWFpbC5jb20=; https://orcid.org/0000-0003-2786-6667