- Institute for Molecular Bioscience, The University of Queensland, Brisbane, QLD, Australia
The MATRIX protocol presents a novel miniaturized 24-well plate approach for microbial cultivation profiling, enabling the efficient and parallel assessment of microbial metabolite production. This method integrates a media MATRIX within a microbioreactor to facilitate the creation of diverse growth conditions for microbial cultivation. The 24-well format supports the simultaneous study of multiple microbial strains and conditions, allowing the discovery of novel bioactive compounds. By employing a variety of media compositions and cultivations, MATRIX enhances the understanding of microbial metabolic pathways and interactions. This protocol provides a robust platform for natural product discovery, microbial ecology studies, and drug development, showcasing its potential to uncover new natural products and other valuable bioactives. The MATRIX approach offers a resource-efficient platform for microbial biodiscovery, contributing significantly to natural product research and drug development.
1 Introduction
Microbial-derived natural products have long been a cornerstone of innovation in medicine, agriculture, and biotechnology. These bioactive compounds have provided society with groundbreaking antibiotics, immunosuppressants, antineoplastic agents, and agrochemicals (Pham et al., 2019). However, despite their unparalleled contributions, traditional microbial drug discovery has faced significant hurdles, including the rediscovery of known compounds and the underutilization of the vast biosynthetic potential that is encoded in microbial genomes (Demain and Sanchez, 2009). This has prompted a paradigm shift toward approaches that can efficiently unlock silent or cryptic biosynthetic gene clusters which often remain dormant under standard laboratory conditions.
Advances in genomics have revealed that microbes harbor an untapped reservoir of biosynthetic gene clusters, each with the potential to encode novel chemical scaffolds (Dougherty and Miller, 2006). The challenge lies in activating these silent clusters to uncover new natural products. Cultivation-based strategies have emerged as a promising avenue, providing an experimental framework for eliciting the production of novel metabolites. However, conventional cultivation techniques, relying on Petri dishes and shake flasks, are labor-intensive, resource-heavy, and inefficient for the high-throughput exploration of microbial metabolic diversity.
To address these limitations, we introduce a miniaturized 24-well plate approach to microbial cultivation profiling (MATRIX), a novel system designed to streamline microbial cultivation profiling. By enabling the simultaneous assessment of multiple strains and conditions, this approach offers a scalable and resource-efficient platform for activating and detecting natural product biosynthesis. In our laboratory, we integrated the MATRIX system with modern analytical tools, including ultra-performance liquid chromatography-diode array detection (UPLC-DAD) and ultra-performance liquid chromatography-quadrupole time-of-flight mass spectrometry with tandem mass spectrometry (UPLC-QTOF-MS/MS). Furthermore, the data generated were analyzed using Global Natural Products Social Molecular Networking (GNPS) (Wang et al., 2016) to expedite the discovery of novel bioactive compounds. Here, we present the methodology, its application in microbial biodiscovery, and examples of its success in identifying unique metabolites under tailored cultivation environments.
2 Materials and equipment
Other organic solvents can be used for microbial extraction and chemical analysis. Care should be taken when using all organic solvents as many can be toxic, flammable, and/or act as irritants. All handling should include the use of appropriate personal protective equipment (PPE) (i.e., laboratory coats, gloves, and safety glasses). Solvents should be handled in a well-ventilated laboratory, preferably in a suitably certified and maintained chemical fumehood. Any spills should be cleaned up promptly and waste materials disposed of appropriately in accordance with instructions on relevant material safety datasheets.
3 Methods
3.1 MATRIX equipment
MATRIX employs the Applikon Biotechnology® micro-bioreactor cultivation system, which incorporates deep 24-well microtiter plates. Each plate is equipped with a multilayered cover consisting of an extruded Teflon (0.2 μm) layer, a microfiber layer, and a soft silicone layer, all secured by a perforated stainless-steel cover (Tables 1, 2; Figure 1). This design ensures sterility by preventing cross-contamination between wells and external sources while permitting air exchange. The system supports cultivation profiling across a variety of media compositions, including those with natural or synthetic transcriptional activators, under shaken or static liquid broth (1.5 mL) and solid-phase (2.5 mL) conditions. Following cultivation, in situ solvent extraction (see Table 3) enables the rapid and economical creation of an extract library for qualitative and quantitative chemical analyses (e.g., UPLC-DAD, UPLC-QTOF-MS/MS, and GNPS and biological screenings).
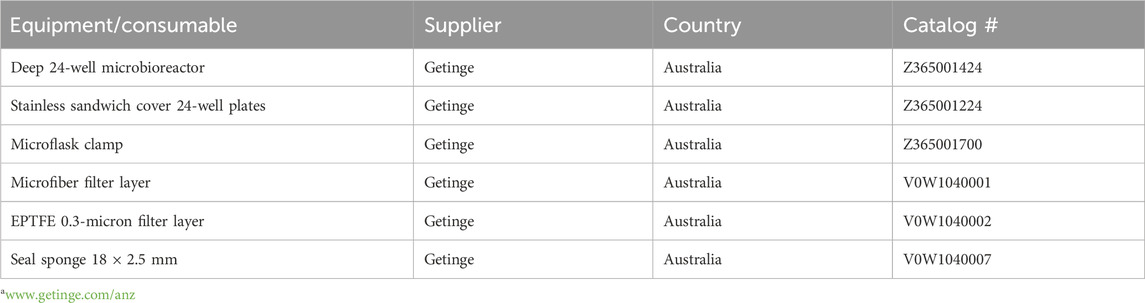
Table 1. List of equipment/consumables (supplier, country, and catalogue #) of the Applikon Micro-Flask system from Getingea.
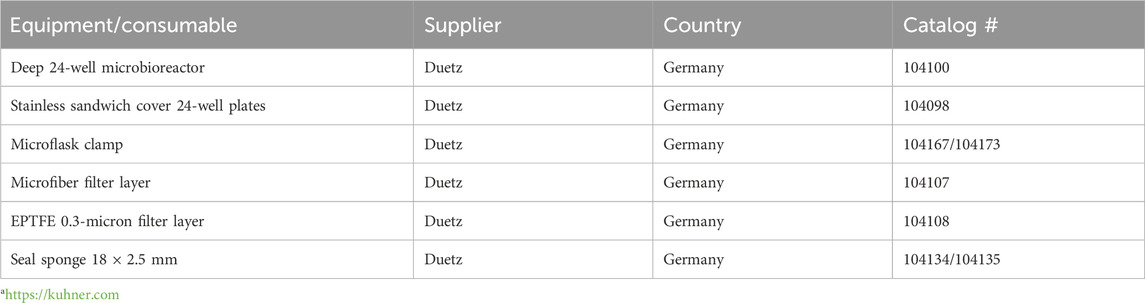
Table 2. List of equipment/consumables (supplier, country, and catalog #) of the Duetz system from Kuhner AGa.
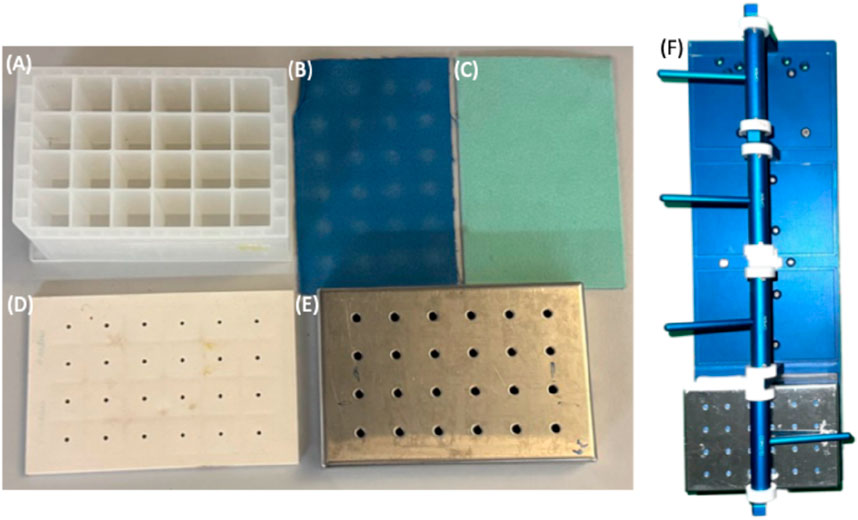
Figure 1. Applikon Biotechnology® micro-bioreactor cultivation system, (A) 24-well plate, (B) extruded Teflon (0.2 μm), (C) microfiber, (D) soft silicone layers, (E) perforated stainless steel cover, and (F) plate holder.
3.2 MATRIX media compositions
MATRIX can accommodate standard and specialist media compositions (for examples, see Tables 4–7). Individual MATRIX plates can be configured as a single media composition inoculated with multiple microbes or as multiple media compositions inoculated with the same microbe, with at least one well per plate per medium set aside as a negative control. All MATRIX plate preparations require the use of sterile handling in a biosafety cabinet with appropriate PPE.
3.3 Preparation and cultivation of MATRIX broth media
Sterile broth media (1.5 mL) is dispensed into the wells of a 24-well sterile plate. Once the media cools to room temperature, an inoculum solution containing the target microbe(s) is added to all wells, except the designated negative control(s) (see Figure 2A). At this stage, optional media additives—such as synthetic or natural chemical elicitors or biosynthetic precursors—can be included. The plate is then covered with a multilayered assembly consisting of an extruded Teflon (0.2 μm) layer, a microfiber layer, a soft silicone layer, and a perforated stainless-steel cover.
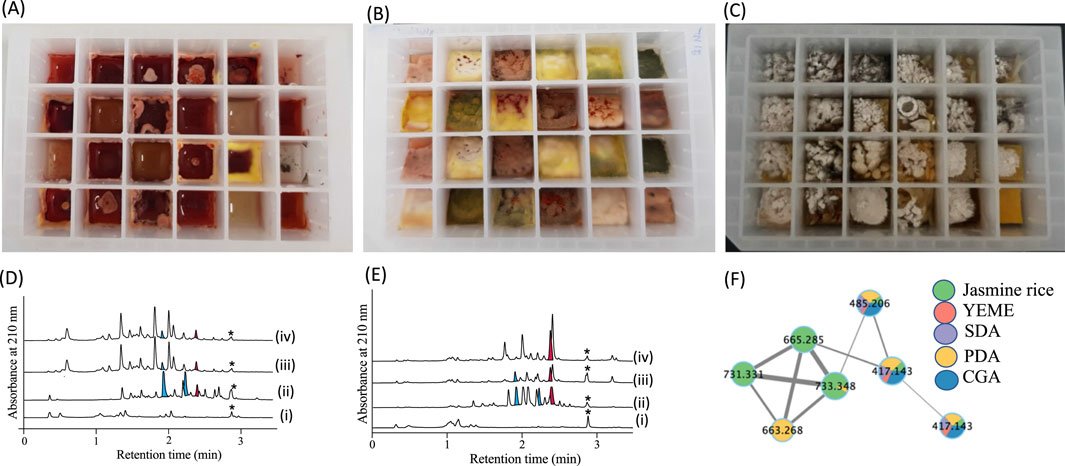
Figure 2. 24-well microbioreactor cultures: (A) broth, (B) agar, and (C) grain. (D, E) UPLC-DAD (210 nm) chromatograms illustrating MATRIX extracts from various media compositions, including (i) uninoculated control, (ii) solid-phase agar culture, (iii) shaken broth, and (vi) static broth. (F) GNPS molecular network of MATRIX data, highlighting different chemical entities across multiple cultivation conditions. *calibrant standard with a known concentration allowing for the accurate determination of unknown concentrations in the sample.
To ensure that the cover is secure, the sealed plate is placed in an Applikon Biotechnology® plate holder, where clamping pressure is applied. The plate is then incubated at the desired temperature, with or without shaking, for a period appropriate to the study. Additives can be introduced into individual sealed wells during cultivation through the perforated cover if necessary. For static broth cultures, the plate holder is not required; it may be replaced with a strong elastic band to tightly secure the cover, which also facilitates efficient stacking in incubators. Incubation parameters, including duration, temperature, and shaking speed (e.g., 10–14 days at 27 °C and 190 rpm), can be adjusted to meet experimental needs.
3.4 Preparation and cultivation of MATRIX solid media
Sterile agar media (2.5 mL) is dispensed into each well of a 24-well sterile plate. The plate is then tilted at 40° and allowed to cool to room temperature, forming solid slants. An inoculum solution containing the target microbe(s) is applied to all wells except the designated negative controls (see Figure 2B). At this stage, optional media additives can be included either before sealing the plate for incubation or later during the cultivation process by injection through the perforated stainless-steel cover.
If additives are to be introduced during incubation, it is recommended that the slants be configured in a defined orientation to ensure that the top edge remains identifiable under the cover, allowing precise additive delivery. Since solid-phase plates are not shaken, the Applikon Biotechnology® plate holder is unnecessary and may be substituted with a strong elastic band to secure the cover tightly. This also enables more efficient stacking of plates in incubators. Plates can be incubated under the required conditions of temperature and duration (e.g., 10–14 days at 27 °C).
3.5 Preparation and cultivation of MATRIX grain media
A 24-well plate is filled with approximately 1 g of grain per well, followed by the addition of 1.5 mL of yeast extract peptone solution in distilled water to each well. The plate is then sealed with the appropriate covers, wrapped in aluminum foil, and sterilized by autoclaving. Once sterilized, the plate is unsealed, and an inoculum containing the target microbe(s) is added to all wells, except for the designated negative controls (Figure 2C).
As with other setups, media additives can be included either before sealing the plate for incubation or introduced during cultivation through the perforated stainless-steel cover. Since solid-phase plates do not require shaking, the Applikon Biotechnology® plate holder is unnecessary and can be replaced with a strong elastic band to secure the cover. This also facilitates space-efficient stacking in incubators. Plates can be incubated under the required conditions of temperature and duration (e.g., 10–14 days at 27 °C).
3.6 Preparation of MATRIX crude extracts
Following incubation, wells in a MATRIX experiment are extracted individually in situ using an organic solvent. This can be done simultaneously across all wells or staggered at specific intervals for time-course studies. For such studies, it is strongly recommended that each plate be dedicated to a single microbial strain to minimize the risk of cross-contamination caused by repeated unsealing and resealing (see Box 1). In these cases, it is preferable to transfer the contents of the target well(s) for separate extraction, avoiding solvent exposure to the remaining cultures.
Box 1 Matrix plates unsealing and resealing.
When unsealing and resealing any MATRIX plate, take care that the covers are replaced in their original orientation to avoid cross contamination between wells.
The extraction method differs slightly between broth and solid-phase cultures. For broth cultures, the plate is unsealed, an aliquot of organic solvent (e.g., ethyl acetate) is added to each well, and the plate is resealed. Gentle shaking at room temperature allows the solvent to extract the metabolites. The organic phase is then carefully removed, filtered through a 0.45 PTFE μm filter, and concentrated under a dry nitrogen stream at 40 °C before being re-dissolved in a solvent suitable for subsequent analysis (see Box 2). For solid-phase cultures, the protocol is similar but includes an additional step—chopping the agar with a sterile spatula prior to addition of the organic solvent.
Box 2 Matrix chemical analysis calibrant.
As MATRIX extracts will be subjected to quantitative comparative chemical analysis, it is essential to include a calibrant standard of a known and common concentration across all extracts. This can be achieved by adding a suitable calibrant to the organic solvent used to reconstitute extracts. Ideally, a calibrant should:
• Be readily available at high purity at low cost;
• Be chemically stable and non-volatile;
• Be soluble in solvents used for chemical analysis;
• Have a chromatographic elution profile that does not overlap with other analytes (i.e., non-polar and late/last eluting on a reverse phase UPLC/HPLC analysis);
• Be compatible with chemical analysis detection systems (i.e., for UV-vis or DAD, possess a prominent chromophore easily detected at low concentration).
An example of a suitable calibrant is Fmoc phenylalanine.
3.7 Integrated UPLC and GNPS-based analysis of MATRIX extracts
Chemical analysis of MATRIX extracts can be performed using UPLC systems equipped with autosamplers, diode array detectors (DAD), binary/quaternary pumps capable of gradient elution, and analytical columns suited to resolving analytes across a wide polarity range (see Box 3). In our laboratory, MATRIX chemical analysis is routinely conducted on an Agilent 1290 Infinity UPLC system fitted with a Zorbax SB-C8 RRHD 1.8 μm, 2.1 × 50 mm column. The system employs gradient elution at 0.417 mL/min over 2.5 min from 90% H₂O/MeCN to MeCN with an isocratic 0.01% TFA/MeCN modifier. Pre-prepared extracts diluted in filtered HPLC-grade methanol are loaded into the autosampler for sequential injection (1 μL per sample at 5 mg/mL conc.). Acquired data are exported in. CSV format and processed using GraphPad Prism (version 10.2.0). For visualization, data are typically displayed as UPLC-DAD chromatograms at 210 nm grouped by media type (e.g., uninoculated controls, static broth, shaken broth, and solid agar cultivations) (Figures 2D, E). In cases where UPLC-DAD includes an additional mass detector, peaks can be annotated with corresponding mass-to-charge (m/z) values.
Box 3 Matrix extract UPLC-DAD analysis.
Pros - Chemical analysis of MATRIX extracts using UPLC-DAD is especially useful for probing the natural product potential of a microbe, detecting new analogs of a target chemical class, and/or additional chemical classes, as well as using relative levels of production to determine optimal culture conditions. UPLC-DAD analysis is rapid, cost-effective, and highly sensitive, with individual analyses requiring only a few μg and a few minutes.
Cons – While UPLC-DAD can disclose chromophores that reveal and compare the diversity of the chemistry within and between extracts, and even assign likely structure classes based on diagnostic chromophores, it typically does not provide sufficient information to identify molecular structures. Additionally, not all molecules have distinctive UV chromophores.
In parallel, Global Natural Products Social (GNPS) molecular networking enhances the annotation and analysis of MS/MS data derived from MATRIX extracts (Box 4). UPLC-DAD analytes are diluted 50-fold and reanalyzed on an Agilent UPLC-QTOF system equipped with a Zorbax SB-C8 1.8 μm, 2.1 × 50 mm column. Gradient elution is performed at 0.417 mL/min over 2.5 min, from 90% H₂O/MeCN to 100% MeCN, with an isocratic 0.1% formic acid modifier and a collision energy of 35 eV. Data are converted from Agilent MassHunter files to mzXML format using MSConvert software and uploaded to the GNPS platform for molecular network analysis (Aron et al., 2020; Wang et al., 2016). The GNPS workflow applies a spectral clustering algorithm with a cosine similarity score threshold of 0.5 and a minimum of six matched peaks (Wang et al., 2016). The resulting molecular networks are visualized in Cytoscape (version 3.9.1) (Shannon et al., 2003), using ball-and-stick layouts where nodes represent parent masses and edge thickness reflects cosine similarity (Figure 2F). Additionally, group abundances can be represented as pie charts indicating MS signal intensity per microbe and culture condition.
Box 4 Matrix extract UPLC-QTOF-GNPS analysis.
Pros - chemical analysis of MATRIX extracts using GNPS can fast-track the dereplication of new, over-known, and rare from common metabolites. This process is further improved when coupled with high resolution mass measurements to determine molecular formula.
Cons – the exceptional sensitivity of GNPS molecular networks will allow the detection of minor metabolites that cannot be isolated. Equally, the conditions under which some molecules ionize varies, to the degree that some may go undetected under standardized conditions. Finally, as not all molecules cluster into the molecular families that dominate GNPS molecular networks, these (singletons) are more easily overlooked.
This integrated approach leverages both chromatographic and mass spectrometric techniques to comprehensively analyze MATRIX extracts, facilitating the discovery and characterization of bioactive compounds.
3.8 Biological screening of MATRIX extracts
The biological screening of MATRIX extracts enables the rapid identification and characterization of bioactive compounds. For instance, when specific chemicals or chemical classes are produced exclusively under certain culture conditions—and at varying levels—the corresponding extracts can be evaluated for unique bioactivity and potency. Comparative analysis of these extracts helps pinpoint the compounds most likely responsible for the observed biological effects. Furthermore, when MATRIX extracts are supplemented with detailed chemical and biological annotations, they provide valuable insights into the cultivation conditions that are most effective for optimizing the production of these bioactive molecules.
4 MATRIX cultivations • timing ∼2–10 days
1. Prepare media according to the recipe (see examples listed in Tables 5–7).
Δ CRITICAL STEP If using solidified agar, the agar can be heated in the microwave for a maximum of four times.
! CAUTION If you are using glass bottles, do not heat the bottle more than 60 °C as the glass may explode.
? TROUBLESHOOTING (see Table 8)
2. Prepare the seed culture. Broth seed culture: sub-sample a single colony of the bacteria or fungi using a sterile loop into an 80 mL broth in a 250-mL flask and incubate it at 27 °C for 10 d at 190 rpm. Agar seed culture: sub-sample a single colony of the bacteria or fungi using a sterile loop into agar in a Petri dish and incubate at 27 °C for 10 d.
? TROUBLESHOOTING (see Table 8)
3. For each microbe, three microbioreactor plates are needed: (1) liquid static, (2) liquid shaken, and (3) solid static, to generate a total of 33 extracts (11 media in 3 different phases). Two microbes can be grown on one plate.
Δ CRITICAL STEP Do not cultivate bacteria and fungi together in the same microbioreactor. Fungi can grow and sporulate faster than bacteria and can contaminate the bacterial wells.
4. For liquid cultivation: add media (1.5 mL) into each of the microbioreactor wells. For solid cultivation: tilt the microbioreactor plate 40°, then add molten agar media (2.5 mL) into the wells and let them set before inoculation (add any media in well #12 for a negative control).
Δ CRITICAL STEP After dissolving the agar, use a serological pipette to transfer 2.5 mL of hot agar into each well of the micro-bioreactor, then tilt the plate 40° and leave the agar to solidify, which will create a slant.
5. Inoculate each well (except the control well) with bacteria or fungi using a sterile loop to transfer mycelia/spores from a seed culture broth (see step 2 above).
Δ CRITICAL STEP Use a 10 μL loop as it has a broader surface area that will enable the homogenous transfer of spores into the well. You need to discard the loops into a beaker that has 80% EtOH (if you use a 250 mL beaker, add 15 mL of 80% EtOH). Leave the loops for at least 30 min before disposing of them into an autoclaving bin.
? TROUBLESHOOTING (see Table 8)
6. Put the sandwich cover and stainless-steel cover on the microbioreactor plates and incubate the plates at 26 °C (for liquid shaken, put the plate onto an orbital shaker at 190 rpm) for 10 days.
Δ CRITICAL STEP To set the sandwich cover, first lay the Teflon (blue layer), microfiber (green layer), and then the silicon layer (yellow layer). Put all three layers into the stainless cover with the silicone layer on top (i.e., facing the researcher). Pack the stainless cover with the layers into an autoclaving bag and steam-sterilize at 121°C for 15 min. The microbioreactor can be covered with aluminum foil, added to the autoclaving paper bag, and steam-autoclaved at 121°C for 15 min. After autoclaving, open the bags in a biosafety cabinet. Be careful to avoid touching the microbioreactor wells to prevent contamination. The layers under the stainless steel lid must be replaced with new ones every 10–20 cultures. Gloves must be worn at all times.
? TROUBLESHOOTING (see Table 8)
7. At the end of the incubation period, add EtOAc (2 mL) using polypropylene pipette tips (e.g., Eppendorf tips) into each of the microbioreactor wells (for solid media, chop the agar first using a sterile spatula into approx. 1 cm3 pieces), cover the plate with parafilm, and put it into an orbital shaker (190 rpm) at room temperature to extract the soluble organic material from the cultivation broth.
Δ CRITICAL STEP Cover the plate with parafilm as this will prevent ethyl acetate from evaporating into the silicone layer, which can cause it to swell. Sealing the stainless-steel lid with sticky tape is crucial to prevent the organic solvent from evaporating. Never use a polystyrene pipette (e.g., serological pipette) as EtOAc can dissolve the plastic, causing a dry plastic film to form upon drying of the crude extract under N2. The microbioreactor must be cleaned using water and soap. A brush can be used to clean the grooves of any fungal/bacterial cells. After cleaning, the plate must be rinsed with water and left to air-dry for 1 day. Never use harsh chemicals such as acetone to clean the wells as they can destroy the polypropylene of the plate.
8. Pipette the EtOAc layer using a glass Pasteur pipette into pre-weighed 2 mL vials, remove the solvent under N2 stream at 40 °C, and then weigh the resulting extracts.
Δ CRITICAL STEP The organic extract will need to be centrifuged at 13,000 rpm for 3 min, and the supernatant removed into a clean 2 mL vial. This will prevent any insoluble substances from blocking the HPLC system. It is crucial that the nitrogen needles are inside the 2 mL vials and that the heating block is set at 40 °C. In addition, ensure that the nitrogen flow is adjusted.
♦PAUSE POINT The dried extract can be stored in a −20 °C freezer until further use.
9. Dissolve the crude extracts in MeOH (30–100) μL and analyze the secondary metabolite production.
Δ CRITICAL STEP Ensure that all material is soluble; otherwise, repeat the centrifugation step.
? TROUBLESHOOTING (see Table 8)
5 Analysis of crude extracts generated by MATRIX cultivation
10. UHPLC-DAD analysis: inject 1 μL of crude extract generated from the MATRIX cultivation into UHPLC-DAD employing a UPLC C8 column (2.1 × 50 mm, 1.8 μm), running a 2.50 min gradient elution at 0.417 mL/min from 90% H2O/MeCN to 100% MeCN, followed by a 1 min hold at 100% MeCN, inclusive of an isocratic 0.01% trifluoroacetic acid (TFA) modifier, with UV detection at 210 and 254 nm.
11. HPLC-DAD-ESIMS analysis: inject 10 μL of crude extract generated from the MATRIX cultivation into HPLC-DAD-ESI(±)MS employing an analytical C8 column (3.0 × 150 mm, 2.7 μm), running a 8.75 min gradient elution at 0.81 mL/min from 90% H2O/MeCN to 100% MeCN, followed by a 3.25 min hold at 100% MeCN, inclusive of an isocratic 0.1% formic acid (HCO2H) modifier, with UV detection at 210 and 254 nm and MS detection in both ESI positive and negative modes.
12. UHPLC-QTOF analysis: inject 1 μL of crude extract generated from the MATRIX cultivation into a UHPLC-QTOF instrument equipped with a C8 UHPLC column (2.1 mm × 50 mm, 1.8 μm), running 2.50 min gradient elution at 0.417 mL/min from 90% H2O/MeCN to 100% MeCN, inclusive of 0.1% formic acid. Perform MS/MS analysis on the same instrument for ions detected in the full scan at an intensity above 1000 counts at 10 scans/s, with an isolation width of 4 ∼m/z using a fixed collision energy and a maximum of three selected precursors per cycle.
Δ CRITICIAL STEP Dilute the crude extract to 0.1 mg/mL.
6 MATRIX plate/cover cleaning and recycling
13. Remove any remaining solid media or cultures from each well using a sterile spatula or pipette.
Δ CRITICIAL STEP Cleaning the 24-well MBR is a critical step in maintaining optimal experimental conditions and ensuring reliable results.
14. Soak the MBR in a mild detergent solution to break down residual organic matter.
Δ CRITICIAL STEP Do not wash the layers or the stainless-steel cover. Gentle scrubbing for the MBR with a soft brush can help dislodge any adhered material.
15. Rinsing with deionized water is essential to remove detergent residues.
16. Turn the MBR upside down and leave it to air-dry.
Δ CRITICIAL STEP After cleaning, the plate must be rinsed with water and left to air-dry for 1 day. Never use harsh chemicals such as acetone to clean the wells as they can destroy the polypropylene of the plate. Care should be taken to ensure that all parts are completely air-dried before reassembly or storage to prevent the growth of unwanted microorganisms. Regular cleaning practices not only preserve the integrity of the MBR but also contribute to the reliability and reproducibility of subsequent experiments. Recycling MBR plates involves a series of steps to ensure that the materials are properly processed and repurposed. First, any residual cultures or media should be carefully removed from the plates. Second, the stainless-steel lids and the microfiber/silicone layers must be free from any contaminants or adhered materials. The entire system can be used for 20–50 experiments and can be changed when needed.
• TIMING
Steps 1–2: 2 weeks per isolate.
Steps 3–6: 2 weeks per isolate.
Step 7: 1 h to 24 h.
Step 8: 2–3 h.
Steps 9–12: The timing of these steps is dependent on the chosen method, column, and the number of generated crude extracts.
7 Anticipated results
Below are examples from our group publications that illustrate the application of the described protocols for media MATRIX for bacterial and fungal cultures.
7.1 Example 1
7.1.1 Identifying oxandrastins as new antibacterial meroterpenes from an Australian mud-dauber wasp nest-associated fungus, Penicillium sp. CMB-MD14
This study describes our bioassay-guided investigations into the secondary metabolism of a mud-dauber wasp nest-associated fungus, Penicillium sp. CMB-MD14, which led to the discovery and structure elucidation of four new meroterpenoids, oxandrastins A–D (1–4), the two known andrastins C (5) and F (6), and a new meroterpenoid from the Austalides family, isoaustalide F (7) (Figure 3) (Elbanna et al., 2021).
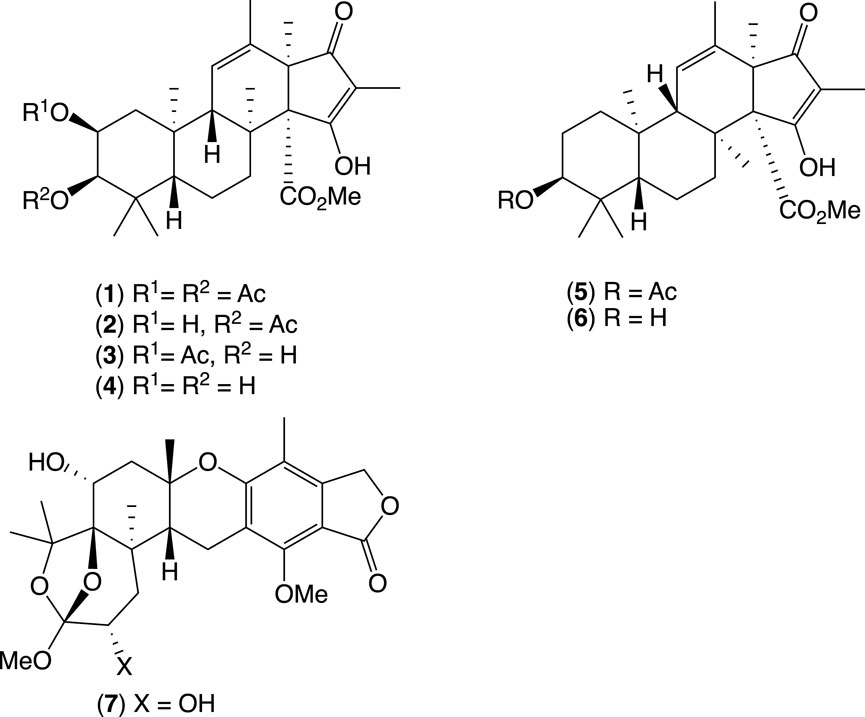
Figure 3. New oxandrastins A–D, andrastins C and F, and new isoaustalide F from mud-dauber wasp nest-associated fungus, Penicillium sp. CMB-MD14.
Briefly, the ethyl acetate extract of CMB-MD14 showed promising antibacterial activity against vancomycin-resistant enterococci (VRE). A bioassay-guided chemical investigation of a scaled-up ISP-2 agar cultivation of CMB-MD14 identified the new anti-VRE meroterpene, oxandrastin A (1). To better explore this pharmacophore, a media optimization strategy was employed, with a scaled-up rice cultivation of CMB-MD14 yielding the three new analogs 2–4, the two known 5 and 6, and a new austalide analog, isoaustalide F (7).
7.2 Example 2
7.2.1 Identifying glenthenamines A–F: enamine pyranonaphthoquinones from the Australian pasture plant-derived Streptomyces sp. CMB-PB042
This study describes a chemical investigation into the secondary metabolites of Streptomyces sp. CMB-PB042, isolated from Australian sheep station pasture plant. This led to the discovery of the rare enamine naphthopyranoquinones BE-54238 A (8) and BE-54238B (9), together with four new analogs, glenthenamines B–D (11–13) and F (15), and two handling artifacts, glenthenamines A (10) and E (14) (Figure 4) (Wu et al., 2022). In this study, we employed a media MATRIX cultivation approach to find the best media for the production of new metabolites from CMB-PB042.
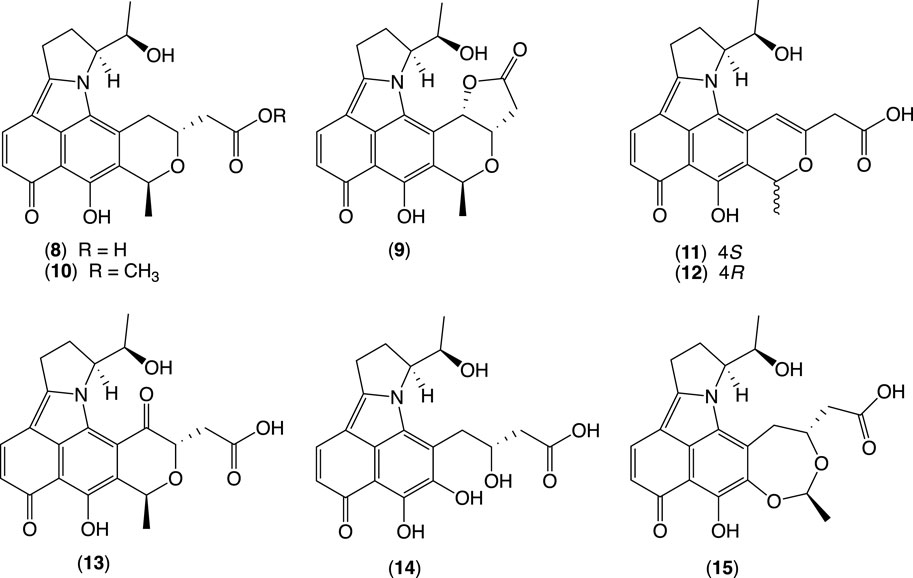
Figure 4. BE-54238A, BE-54238B, and new glenthenamines A–F from the Australian pasture plant-derived Streptomyces sp. CMB-PB042.
Briefly, CMB-PB042 was cultured in a microbioreactor format under 12 different media in solid phase and static and shaken broths (36 conditions). After 7 days, individual cultivations were extracted with EtOAc (2 mL) and concentrated to dryness under N2 at 40 °C. Individual extracts were redissolved in 100 μL MeOH, and aliquots (1 μL) were subjected to UPLC-DAD analysis (Zorbax SB-C8 1.8 μm, 2.1 × 50 mm column, 0.417 mL/min gradient elution over 2.52 min from 90% H2O/MeCN to 100% MeCN, followed by an isocratic elution for 0.83 min with MeCN, with an isocratic 0.01% TFA modifier).
This analysis confirmed that a combination of ISP2 and M2 solid-phase cultivations provides optimal coverage of the target metabolites. Further fractionation of the large-scale ISP2 solid-phase cultivation extract yielded 8–12 and 14–15, while fractionation of a scaled-up M2 solid phase cultivation yielded the additional analogue 13.
7.3 Example 3
7.3.1 The identification of new chrysosporazines from Aspergillus sp. CMB-F661
This study describes our investigations into the gastrointestinal tract of Australian mullet fish, revealing the production of phenylpropanoid piperazine alkaloids (chrysosporazines) (Agampodi Dewa et al., 2022; Elbanna et al., 2019; Mohamed et al., 2020). We integrated a comprehensive analysis, including cultivating the fungal strains under different media conditions/compositions using microbioreactor 24-well plate (MATRIX), followed by UPLC-DAD and UPLC-QTOF-MS/MS chemical profiling. This analysis showed that Aspergillus sp. CMB-F661 can produce two new major chrysosporazines, 20–21 and, potentially, two new minor azachrysosporazines, T1 (22) and U1 (23) (Figure 5).
To guide chemical investigations into CMB-F661, a media MATRIX cultivation profiling approach was applied to better understand and optimize conditions to produce chrysosporazines. This entailed multiple cultivations of CMB-F661 in a 24-well microbioreactor format using ×11 different culture media across both static and shaken broths (1.5 mL) and solid agar (1 g). Each microbioreactor well cultivation was, in turn, extracted in situ with EtOAc (2 mL) to provide extracts (×33) that were subjected to UPLC-DAD (210 nm) analysis to reveal variable levels of chrysosporazine production. UPLC analysis of all media MATRIX extracts confirmed and quantified these observations. Based on these analyses, the PDA solid phase was selected for scale-up cultivation of CMB-F661 as it was deemed capable of producing two new major chrysosporazines, 16–17, and potentially two new minor azachrysosporazines, 18–19.
8 Discussion
The MATRIX protocol introduces a highly efficient approach to microbial cultivation profiling that can significantly enhance natural product discovery. By miniaturizing the cultivation process to a 24-well plate format, MATRIX reduces the material, labor, and infrastructure costs traditionally associated with microbial cultivation. This approach permits the simultaneous cultivation of multiple strains under diverse conditions, making it highly versatile for exploring secondary metabolite production across a range of microbes and media compositions.
The MATRIX method’s ability to integrate various culture conditions (e.g., solid vs. liquid, static vs. shaken) within a single protocol is instrumental in activating silent biosynthetic gene clusters (BGCs). This flexibility is crucial, as environmental factors heavily influence gene expression, with specific conditions often necessary to stimulate the production of unique secondary metabolites. Consequently, MATRIX provides an adaptable platform for probing the metabolic potential of microbes, especially those with previously unexplored or dormant pathways.
Case studies within this work illustrate the MATRIX system’s efficacy in identifying novel compounds under unique growth conditions. For instance, the isolation of oxandrastins demonstrates how MATRIX facilitates the discovery of diverse chemical entities, while glenthenamines and chrysosporazines highlight its potential to activate otherwise cryptic BGCs. These findings underscore the utility of MATRIX in accelerating biodiscovery efforts, potentially filling the gap left by traditional methods that often fail to elicit the full metabolic capacity of microbial strains.
Moreover, the MATRIX platform’s compatibility with high-throughput chemical analysis methods such as UPLC-DAD and GNPS-based molecular networking further streamlines the process of compound identification and dereplication. By coupling chemical profiling with bioactivity assays, MATRIX allows for the rapid pinpointing of bioactive compounds, facilitating targeted isolation and reducing the time for identifying potential leads in drug discovery.
In conclusion, the MATRIX protocol offers a robust, cost-effective, and scalable solution for microbial cultivation profiling that promises to be a valuable tool in the ongoing search for new bioactive compounds. By enabling the discovery of novel metabolites with potential applications in medicine, agriculture, and biotechnology, MATRIX represents a meaningful advance in microbial biodiscovery.
Data availability statement
The raw data supporting the conclusions of this article will be made available by the authors, without undue reservation.
Author contributions
ZK: conceptualization, investigation, methodology, project administration, writing–original draft, writing–review and editing. TS: investigation, methodology, writing-original draft. AS: writing–review and editing. RJC: conceptualization, writing–review and editing.
Funding
The authors declare that financial support was received for the research, authorship, and/or publication of this article. ZK acknowledges support through an ARC Future Fellowship (FT 230100468). ST thanks the University of Queensland for an International Postgraduate Scholarship.
Acknowledgments
We express our acknowledgement to numerous former colleagues who played a crucial role in our studies on 24 well MBR and MATRIX.
Conflict of interest
The authors declare that the research was conducted in the absence of any commercial or financial relationships that could be construed as a potential conflict of interest.
Generative AI statement
The authors declare that no Generative AI was used in the creation of this manuscript.
Publisher’s note
All claims expressed in this article are solely those of the authors and do not necessarily represent those of their affiliated organizations, or those of the publisher, the editors and the reviewers. Any product that may be evaluated in this article, or claim that may be made by its manufacturer, is not guaranteed or endorsed by the publisher.
References
Agampodi Dewa, A., Khalil, Z. G., Elbanna, A. H., and Capon, R. J. (2022). Chrysosporazines revisited: regioisomeric phenylpropanoid piperazine P-glycoprotein inhibitors from Australian marine fish-derived fungi. Molecules 27 (10), 3172. doi:10.3390/molecules27103172
Aron, A. T., Gentry, E. C., McPhail, K. L., Nothias, L. F., Nothias-Esposito, M., Bouslimani, A., et al. (2020). Reproducible molecular networking of untargeted mass spectrometry data using GNPS. Nat. Protoc. 15 (6), 1954–1991. doi:10.1038/s41596-020-0317-5
Demain, A. L., and Sanchez, S. (2009). Microbial drug discovery: 80 years of progress. J. Antibiot. (Tokyo) 62 (1), 5–16. doi:10.1038/ja.2008.16
Dougherty, T. J., and Miller, P. F. (2006). Microbial genomics and drug discovery: exploring innovative routes of drug discovery in the postgenomic era. IDrugs 9 (6), 420–422. Available at: https://www.ncbi.nlm.nih.gov/pubmed/16752312.
Elbanna, A. H., Khalil, Z. G., Bernhardt, P. V., and Capon, R. J. (2019). Chrysosporazines A-E: P-glycoprotein inhibitory piperazines from an Australian marine fish gastrointestinal tract-derived fungus, chrysosporium sp. CMB-F214. Org. Lett. 21 (19), 8097–8100. doi:10.1021/acs.orglett.9b03094
Elbanna, A. H., Khalil, Z. G., and Capon, R. J. (2021). Oxandrastins: antibacterial meroterpenes from an Australian mud dauber wasp nest-associated fungus, Penicillium sp. CMB-MD14. Molecules 26 (23), 7144. doi:10.3390/molecules26237144
Mohamed, O. G., Salim, A. A., Khalil, Z. G., Elbanna, A. H., Bernhardt, P. V., and Capon, R. J. (2020). Chrysosporazines F-M: P-glycoprotein inhibitory phenylpropanoid piperazines from an Australian marine fish derived fungus, chrysosporium sp. CMB-F294. J. Nat. Prod. 83 (2), 497–504. doi:10.1021/acs.jnatprod.9b01181
Pham, J. V., Yilma, M. A., Feliz, A., Majid, M. T., Maffetone, N., Walker, J. R., et al. (2019). A review of the microbial production of bioactive natural products and biologics. Front. Microbiol. 10, 1404. doi:10.3389/fmicb.2019.01404
Shannon, P., Markiel, A., Ozier, O., Baliga, N. S., Wang, J. T., Ramage, D., et al. (2003). Cytoscape: a software environment for integrated models of biomolecular interaction networks. Genome Res. 13 (11), 2498–2504. doi:10.1101/gr.1239303
Wang, M., Carver, J. J., Phelan, V. V., Sanchez, L. M., Garg, N., Peng, Y., et al. (2016). Sharing and community curation of mass spectrometry data with global natural products social molecular networking. Nat. Biotechnol. 34 (8), 828–837. doi:10.1038/nbt.3597
Keywords: matrix methodology, microbial cultivation profiling, natural product discovery, bioactive compounds, biosynthetic gene clusters, high-throughput screening, secondary metabolites, GNPS molecular networking
Citation: Khalil ZG, Sritharan T, Salim AA and Capon RJ (2025) MATRIX: a platform for cultivation profiling and bioactive discovery in natural product research. Front. Nat. Produc. 3:1532543. doi: 10.3389/fntpr.2024.1532543
Received: 22 November 2024; Accepted: 18 December 2024;
Published: 24 January 2025.
Edited by:
Tsong-Long Hwang, Chang Gung University of Science and Technology, TaiwanReviewed by:
Afif Felix Monteiro, University of São Paulo, BrazilWeaam Ebrahim, Mansoura University, Egypt
Copyright © 2025 Khalil, Sritharan, Salim and Capon. This is an open-access article distributed under the terms of the Creative Commons Attribution License (CC BY). The use, distribution or reproduction in other forums is permitted, provided the original author(s) and the copyright owner(s) are credited and that the original publication in this journal is cited, in accordance with accepted academic practice. No use, distribution or reproduction is permitted which does not comply with these terms.
*Correspondence: Zeinab G. Khalil, ei5raGFsaWxAdXEuZWR1LmF1; Robert J. Capon, ci5jYXBvbkB1cS5lZHUuYXU=