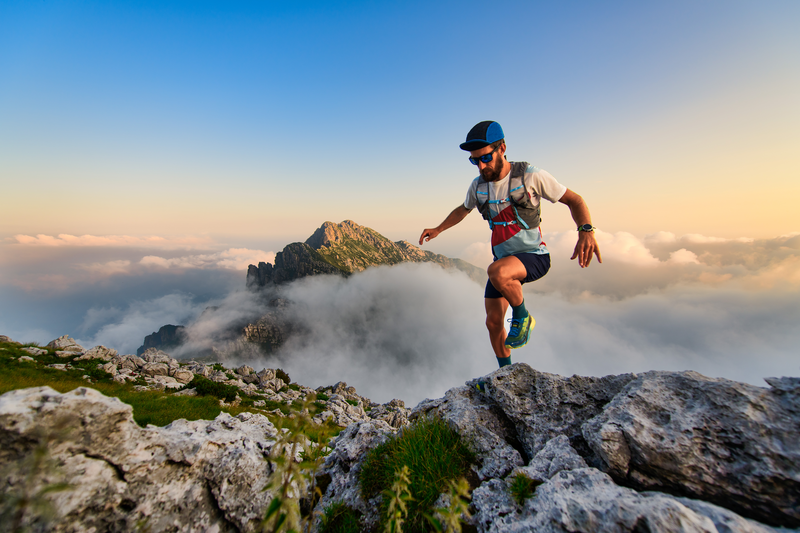
94% of researchers rate our articles as excellent or good
Learn more about the work of our research integrity team to safeguard the quality of each article we publish.
Find out more
REVIEW article
Front. Nat. Prod. , 20 September 2024
Sec. Isolation and Purification
Volume 3 - 2024 | https://doi.org/10.3389/fntpr.2024.1425242
This article is part of the Research Topic Innovative Approaches in Isolation and Purification: Balancing Efficiency and Sustainability View all articles
Seaweeds or marine algae with their three main taxa are recognized as a potential pot for numerous products. This has been imperative to valorize their components at an industrial scale in the context of the sustainability goals of the United Nations (UN). Biorefinery approaches have been attempted for several decades to produce bioactive, biofuels, fine chemicals, and nutritional products from seaweeds. However, with the recent UN goals, climatic changes, and the global economic situation, it is essential to assess all the production processes involved concerning several factors. Numerous steps are performed following algae collection, including optimization of downstream processing (e.g., drying, extraction, and purification), in addition to suitable preservation to maintain products’ integrity till their usage. Moreover, breakthroughs in biotechnologies and the biological sciences are also targeted to achieve the goals of the blue circular bioeconomy which focuses on renewable resources of foods, drug discovery, and energy, while preserving the ocean ecosystem. Hence, the current article provided new insights into the various processes to valorize marine algae in the frame of circular bioeconomy and sustainability concepts.
Circular bioeconomy is an ecosystem-driven economy that strives for sustainable and resource-efficient operations replacing the vast array of non-renewable, fossil-based products now in use by emphasizing the utilization of renewable natural capital and minimizing waste. Hence, this approach should reduce emissions and mitigate climate change (Kardung et al., 2021; Abdelmohsen et al., 2022). Bioeconomy activities have gained a particular interest since the announcement of the United Nations 17 Sustainable Development Goals (SDGs) (Heimann, 2019; Rushdi et al., 2020c). Besides, biorefinery is a relatively novel trend usually used in industrial fields to optimize the use of bioresources to produce bioactive and fine chemicals achieving the goals of zero waste technology, and hence, sustainability and circular bioeconomy (Orejuela-Escobar et al., 2021). This is in line with the concept of the “blue economy” which was coined by Gunter Pauli and refers to the sustainable use of the marine environment for better living standards and economic growth while maintaining the health of the marine ecosystem (Pauli, 2010). Based on information obtained from the Food and Agriculture Organization (FAO), it is estimated that annual food waste amounts to about 1.6 billion metric tonnes, of which 1.3 billion metric tonnes are edible food items, components, or products. Examples include nearly 46% of the fruits, vegetables, roots, and tubers are wasted, while 35% of fish and shellfish and 30% of grains are thrown away which becomes a challenging issue (Lahiri et al., 2023; Salem et al., 2023).
To reduce biowastes and use large volumes of extraction solvents as in classical traditional methods, great developments were achieved in downstream processing, including extraction and purification methods in the last few years. In this context, various modern extraction methods were developed such as microwave-assisted extraction (MAE), ultrasound-assisted extraction (UAE), enzyme-assisted extraction (EAE), and super critical fluid extraction (SFE) (El-Shamy and Farag, 2021). Such an approach could show high potential to valorize diverse agricultural and food by-products produced following processing of, for example, onion and potato (Salem et al., 2023), artichoke, buckthorn (Zayed and Farag, 2020; Fouda et al., 2024), mango (El-Shabasy et al., 2024), citrus fruits (Zayed et al., 2021). The biorefinery approach is not limited to terrestrial plants, but it has been expanded to marine organisms such as marine microalgae (Cheng et al., 2022; Aboubakr et al., 2024) and macroalgae (Álvarez-Viñas et al., 2019). These efforts have resulted in the isolation of value-added compounds with promising health-promoting benefits, including anti-tumor, antioxidant, anti-inflammatory, and others (Zayed and Farag, 2020; Liu et al., 2021; Zayed et al., 2021; Benedetti et al., 2023; Salem et al., 2023). However, macroalgae valorization still needs more investigations for extraction optimization and full recovery of their bioactive metabolites.
Closely connected to this issue, the sustainability of the techniques used to extract and purify bioactive phytochemicals is critical. Today, not only is extraction technique efficiency pursued by extracting phytochemicals with the best possible extraction yield and related bioactivity, but also the development of environmentally friendly extraction processes is chosen over traditional extraction protocols. These discoveries are highly recommended to comply with green chemistry guidelines concerning extraction. Such guidelines encourage innovation using renewable resources, employing other solvents, primarily water, energy consumption decreases with modern technologies, creation of co-products rather than garbage, reducing unit operations and promoting process automation, and aim for non-denatured, biodegradable extracts that are free of pollutants (Chemat et al., 2017).
To meet these requirements and increase the “greenness” of these processes even further, biorefinery concepts are being developed, which are based on the use of natural biomass to produce a diverse range of products useful in various production fields (e.g., food, pharmaceutical, or agricultural) while also producing energy to reduce or eliminate any industrial waste (Herrero et al., 2015). The principles, advantages and possible uses of these technologies concerning marine algae phytochemicals are examined. Higher yield, shorter treatment times, and cheaper costs are benefits of new technologies over conventional solvent extraction methods. Additionally, several combinations of innovative extraction techniques and thermolabile compound-friendly technologies are found. Also addressed are the drawbacks and difficulties associated with applying these revolutionary extraction technologies in industry (Herrero et al., 2015). The attainment of the active fraction or pure natural products requires further separation and purification of specific products based on the inherent disparities, whether physical or chemical, that exist among each natural product. Of all the techniques employed, chromatography, particularly column chromatography, stands as the prevailing technique for the isolation of uncontaminated natural compounds from intricate mixtures. Therefore, the current article reviewed firstly the various extraction procedures either traditional or modern green, applied particularly for marine algae-derived bioactive compounds. Afterward, the macroalgae bioactive constituents shall be addressed for optimization of their recovery. Hence, the study may present optimization for isolating specific compounds, particularly from algae, exhibiting potential bioactivities.
A biorefinery may be characterized as a series of processes or a cascade of processes that convert biomass into various products intended for either material or energy applications, as delineated in its fundamental definition (Van Dyne et al., 1999; Abdel-Rahman et al., 2022b). One of the most plentiful resources in the water is marine algae. Contributing 27% of the global yearly harvest of wild marine algae and 72% of the global annual crop of cultivated marine algae, respectively (Zheng L.-X. et al., 2020a). A biorefinery is a facility that uses biomass to create a wide range of products for different markets, including food, feed, materials, and energy. It is distinguished by its high process integration, low waste production, and high flexibility in responding to shifting markets for products and raw materials. Biorefinery has increased significantly in recent decades due to factors such as the growing global population, the scarcity of sustainably useable cultivation areas, and the politically motivated efforts of many nations to transition from a fossil-based economy to a more bio-based economy (Schmidt et al., 2019). We will now direct our attention towards elucidating the various categories of Biorefineries. We will now focus on describing the different kinds of Biorefineries. The traits of the one under study in this conceptual analysis will next be described in greater detail.
Biorefinery Phase I: is exemplified by an ethanol dry mill facility. Such a plant possesses a set processing capacity, utilizes grain as raw algae material, and produces predetermined quantities of carbon dioxide, ethanol, and feed byproducts. Its processing adaptability is essentially non-existent. This type will solely be employed for comparative purposes.
Biorefinery Phase II: The wet milling technology that is used today is one example of this. Despite using grain feedstocks, this technique can produce a range of end products based on contract obligations, costs, and product demand. These goods consist of corn oil, starch, ethanol, high fructose corn syrup, and corn gluten feed and meal. This kind of starts to imply the series of industrial facilities required to balance the current boom-and-bust nature of the algae economy.
Biorefinery Phase III: is the type that is examined. A Phase III Biorefinery can create a wide range of chemicals, fuels, and intermediate or final products. It can also create these products using different kinds of feedstocks and processing techniques for the industrial market.
The key element in adapting to shifts in the supply and demand of feed, food, fiber, and industrial commodities is the flexibility of feedstock utilization. In summary, these algae of the future will be able to:
(1) Accept a variety of agricultural feedstocks.
(2) Employ several kinds of processing techniques.
(3) Coproduce ethanol and a variety of higher-value compounds.
An integrated biorefinery platform with algae bio stimulants is anticipated to attain environmental, technological, and economic sustainability (Cheng et al., 2022). Primary and secondary biorefinery techniques have been developed for processing algae biomass. To separate the biomass components in their original or slightly modified states, such as extracting lipids, proteins, cellulose, minerals, carbohydrates, agar, alginate, and carrageenan, the primary-level biorefining process is utilized. Primary-level biorefinery techniques used in this kind of approach are chemical, mechanical, and water treatment. Secondary biorefinery techniques directly convert complete algae biomass into final or end products, such as bioethanol, biogas, biobutanol, bio-oil, biochar, acids, etc., the second level of biorefining techniques is utilized. Treatments like hydrolysis, fermentation, pyrolysis, anaerobic digestion, and hydrothermal liquefaction are used in this kind of approach. Compared to the secondary-level strategy, the primary-level biorefinery systems have advantages. They yield high-value products with a greater biomass utilization rate, and the extracted products can be further modified, derivatized, and used according to needs. In comparison to conventional marine algae processing, the marine algae biorefining process offers several advantages, including increasing the value of biomass, guaranteeing sustainable biofuel generation, and improving the environment (Baghel, 2023). The cost of producing biomass for a biorefinery can be greatly decreased by cultivating macroalgae in an IMTA system. Additionally, growing marine macroalgae requires the use of sea water, which eliminates the need for significant freshwater resource usage as compared to terrestrial plants and freshwater microalgae. Ulva lactuca, Saccharina latissima, and Mastocarpus stellatus (a red algae that generates carrageenan) are among the marine microalgae that have been successfully cultivated and employed as biofilters in an integrated multi-trophic aquaculture (IMTA) pilot system (Filote et al., 2021a). The biomass of Laminaria digitata collected between late spring and midsummer has been shown to have the greatest sugar concentrations (Alvarado-Morales et al., 2015). For the biomass gathered in July, agar derived from Gracillaria verrucosa had the maximum yield, whereas the algae obtained in May yielded the lowest (Kumar et al., 2013). Alginates derived from wild Sargassum latifolium harvested in the springtime exhibited a greater mannuronic/guluronic acid ratio (M/G) (Fawzy et al., 2017). The biomass of Gracilaria chilensis collected in winter and spring when the protein level is highest, while the summer protein level is lowest (Abreu et al., 2009). Research shows that compared to macroalgae growing in the wild, those grown in an IMTA system have a more consistent protein chemical composition. The number of valuable biomolecules that can be discovered in cultivated macroalgae that differ from those found in wild species is an essential factor that biorefineries should take into account. To effectively generate macroalgae biomass, a few prerequisites need to be addressed: temperature, turbidity, depth, illumination, and nutrients in the water (Aravind et al., 2020).
Algae are a diverse collection of aquatic autotrophs that serve as valuable models for morphology, physiology, and molecular biology research (Rushdi et al., 2022a). About 175,427 species and infraspecific of algae have been identified, which have been classified into macroalgae (commonly known as marine algae) and phytoplankton or microalgae. Diatoms (Bacillariophyta), dinoflagellates (Dinophyta), green and yellow-brown flagellates (Prasinophyta, Prymnesiophyta, Cryptophyta, Chrysophyta, and Rhaphidiophyta), and blue-green microalgae (Cyanophyta) make up most phytoplankton (Jimenez-Lopez et al., 2021; Abdel-Rahman et al., 2022a; Guiry and Guiry, 2024). Macroalgae, on the other hand, are categorized into three major groupings based on the types of pigments, i.e., green algae (Chlorophyta), brown algae (Ochrophyta), and red algae (Rhodophyta), which enable them to reside and survive at various depths (Attia et al., 2024; Guiry and Guiry, 2024).
The biomass produced by marine algae is a great source of oils, proteins, polysaccharides (e.g., fucoidans, laminarin, and alginic acid), and other value-added value compounds present in smaller amounts, such as carotenoids, pigments, antioxidants, sterols, and other unsaponifiable, antimicrobials, or minerals. Microalgae can use residual nutrients and CO2 from flue gas. Furthermore, triacyl glycerides (TAG), a source of long-chain poly unsaturated fatty acids (LC PUFA) such as the omega-3 fatty acids EPA and DHA, may be obtained from microalgae (Catchpole et al., 2010). However, downstream processes, including algal biomass harvesting, drying, extraction and purification of metabolites, may provide a lot of challenges when attempting to extract considerable amounts of, for instance, protein due to their adaption to the marine environment, cell wall composition, and secondary metabolites. The initial stage in isolating the desired phytochemicals from marine algae is harvesting high concentration of microalgae biomass then drying procedures to stabilize the alae biomass followed by effective extraction method (Simon and Helliwell, 1998). In addition, natural matrices are extremely complicated and effective separation techniques are necessary to attain the desired result. The isolated bioactive compounds should be preserved in a suitable form to protect them from external deteriorating factors in addition to improving their bioavailability. The bioactive phytochemicals present in those materials must be selectively removed to be completely exploited (Montañés and Tallon, 2018). Hence, various methods have been reported and may be classified into traditional extraction procedures such as maceration, percolation, and reflux extraction, in addition to some modern or greener extraction methods such as supercritical fluid extraction (SFC), pressurized liquid extraction (PLE), and microwave-assisted extraction (MAE). The latter procedures have been used in phytochemicals extraction and offer benefits providing a number of advantages as lower organic solvent consumption, shorter extraction time, and higher selectivity (Zhang et al., 2018).
The stages of downstream algae production that require the greatest energy are harvesting and drying (Lafarga, 2020). Algal cells are collected and separated from the liquid media during the harvesting process. Algae normally make up 1% (w/v) of the culture media, which means that their concentration requires a lot of energy (Kuzhiumparambil et al., 2022).
Similar to harvesting, drying requires a significant amount of energy to remove the water from the biomass of microalgae (Dixon and Wilken, 2018). The microalgae that are obtained typically have a water content of 85%; the drying procedure is used to reduce this to typically 5% (Fasaei et al., 2018). In order to stabilise microalgal biomass by lowering the rate of microbial and chemical degradation, low water content is required (Biz et al., 2019). A number of techniques for harvesting and drying will be covered in this section.
One method of harvesting microalgae is flocculation, which entails aggregating the particles to increase their size and aid in the separation of microalgae from huge volumes of suspension (Mata et al., 2010), There are several varieties of flocculation, including bio-, electro-, and chemical flocculation.
The basis of this kind of flocculation is the neutralisation of the charge on the microalgae’s surface with the addition of cations, such as poly aluminium chloride and aluminium sulphate, which can successfully offset the negative charge of the microalgae cells. High quantities of these multivalent cations can contaminate algal biomass, which can be hazardous to metabolic processes by impeding algal growth and production and complicating downstream processing. This is considered the drawback of this harvesting method (Chen et al., 2011; Lee et al., 2013).
Electro-flocculation is a well-established method for harvesting micro algal biomass due to its advantages of being simple to use, requiring little chemical input, and leaving no residual anions behind (Xu et al., 2010). This method involves depositing the culture medium into a container that has many metallic electrodes, and then using an electric current to release a small amount of ions into the medium. Algal biomass can agglomerate quickly as a result of this neutralising the negative charge of the cells (Chatsungnoen and Chisti, 2019).
Bio-flocculants may be bacterial byproducts. Bacterial surfactants, proteins, humic compounds, carbohydrates, and nucleic acids make up these bio-flocculants. The removal of metals from water, the flocculation of wastewater, and the harvesting of microalgae are all made possible by the widespread usage of bio-flocculants derived from bacteria like Scenedesmus quadricauda, Botryococcus braunii, and Selenastrum capricornutum. Bacterial bio-flocculants have an issue in that their purification is expensive (Wan et al., 2013).
Flotation harvesting technology has the advantage of reduced initial equipment costs and is especially efficient for very thin algal suspensions. Using this method, fine air bubbles are introduced at the flotation tank’s bottom to facilitate the separation of liquid from solid. As the bubbles cling to the particulate matter, the particles are propelled to the surface by their combined buoyancy. A layer of thickened slurry will form once the particles have floated to the surface, and this can be recovered using a skimming operation. The best dosage of various coagulants should be injected into the algal suspension since the amount of algae biomass that can be recovered by flotation operations is restricted (Bare et al., 1975).
In this technique, algal suspension is forced through the filter medium by a suction pump during the filtering process. Afterwards, the medium is harvested after the algal biomass has been concentrated there. Spirulina platensis and Coelastrum proboscideum are two examples of large-sized microalgae that may be harvested via filtration, which is mostly advantageous for these types of algae. The two primary issues with filtering algae are the tiny volume of fluid flow and the deposited cells’ tendency to clog the medium. To prevent filter clogging, a number of alternative techniques have been developed (Show et al., 2019).
Gravity sedimentation is a solid-liquid separation technique that divides a feed suspension into a higher concentration slurry and an effluent that is essentially clear liquid. It is employed in the extraction of particles from a suspension with a suitable settling velocity. After the thickened sludge underflow is removed from the tank’s bottom, the supernatant, also known as effluent, overflows a weir and is piped back to the treatment plant’s inlet (Show et al., 2019).
Microalgae are separated from the suspension via centrifugation, which uses a stronger centrifugal force than gravity. Feeding suspension into the bowl, it is rotated. The solids in the suspension would spin out faster against the revolving bowl wall the higher the rotation speed. Using a skimming tube, the supernatant is extracted. While centrifugation techniques have many advantages for harvesting algae, their high running costs and tendency to destroy cells generally outweigh their benefits (Show et al., 2019).
It's a drying method that makes use of sun energy, which is abundant and naturally renewable. However, it necessitates lengthy drying durations and vast drying surfaces. Furthermore, the sluggish drying rate that results in biomass degradation, such as microalgae discolouration, is caused by the dehydration and breakdown of the chlorophyll in the microalgae (Brink and Marx, 2013; Fudholi et al., 2014). This makes it challenging to preserve the quality of the final product when utilizing conventional open solar drying methods. When compared to alternative drying methods, it appears to have little effect on certain operations, such as oil extraction, despite these disadvantages (Guldhe et al., 2014).
One typical technique for extracting water from microalgae is convective drying. Its basic principle is the dehydration that results from distributing the biomass into thin layers and providing convective hot air in an oven or tray drier. This technique can be carried out in temperatures between 20°C and 60°C and between 18 and 48 h (Chen et al., 2015). When compared to fresh biomass, it was found that there are no appreciable changes in the content of fatty acids or oil extraction (Guldhe et al., 2014).
The process of spray drying involves mixing liquid atomization gas with droplets and then drying the liquid droplets. When a liquid droplet sprayed downwards in a vertical tower gets into contact with a hot gas stream, drying is initiated. When producing algae for human consumption, spray drying is a suitable drying technique. In addition to being expensive, the high-pressure atomization method used in the process can break intact cells, even with its great drying efficiency. Spray drying demonstrated a high level of efficiency, removing 10,000 kg of water per hour from microalgae (Show et al., 2019).
Freeze drying is a popular drying technique in food processing due to its ability of freeze drying to retain the majority of the protein in dried microalgal biomass with protein loss around 10% (Desmorieux and Hernandez, 2004). The initial step in this procedure entails freezing the biomass and transferring it to a vacuum chamber. Sublimation of water using heat or radiation is the second phase. Algal components that are heat-and oxygen-sensitive can be treated using this approach. The biggest drawbacks are the high running costs and lengthy drying times (de Farias Neves et al., 2019).
By using this technique, high drying efficiency at comparatively low temperatures can be achieved. It works well with algal materials that are oxidation-sensitive. When drying Spirulina sp., the vacuum shelf drying approach was shown to have a good drying efficiency. Dried biomass with a water content of 4% was produced at 50°C–65°C and a low pressure of 0.06 atm. The primary benefit of the vacuum shelf drying process is that it minimises phycocyanin loss and lipid oxidation while preserving the high quality of algal products. The primary disadvantage is high operating costs in spite of these benefits (Mee-ngern et al., 2014; Larrosa et al., 2017).
Flash drying is used for algae drying by introducing a mixture of dry and wet algae into a hot gas stream. The moisture from the algae is transferred to the gas by means of this heated gas. The method of obtaining the hot gas determines the economical effectiveness (Show et al., 2013).
This drying technique makes use of several circular steel cylinders called hearth incinerators. Algae can be dried in an incinerator by lowering the degree of heat that enters. An alternative kind of incineration drier that guarantees a uniform drying of the algae does so by using a fluidized sand bed as a heat reservoir. The primary drawback is the price (Delrue et al., 2012).
This drying technique combines a compartment dryer with a hot air cross flow. When Spirulina sp. is dried using cross flow air, an efficient approach yields dried biomass with a water content of 4%–8% after 14 h at 62°C. The only thing impeding its utilisation despite its remarkable drying efficiency was the high energy cost (Delrue et al., 2012; Liu et al., 2012).
Microalgae may be dried quickly with microwave drying because of its effective heat distribution. Because of heat produced by water molecules friction against one another and microwave energy absorbed by the water, the microwave frequency utilized in micro algal drying—2.45 GHz—helps to evaporate water. Utilizing this technique with the previously described frequency for drying Chlorella vulgaris, which has a moisture content of 42.5%, demonstrated the great drying efficiency and quick drying periods (Chandrasekaran et al., 2013; Villagracia et al., 2016).
Traditional extraction techniques are always performed with a simple solid-solvent procedure including maceration, heat-assisted extraction, percolation, and Soxhlet extraction. They often involve organic solvents and necessitate a high volume of solvents as well as lengthy extraction periods. According to the extraction principle, extraction processes include solvent extraction, distillation, pressing, and sublimation, where the most common method is solvent extraction (Attia et al., 2022). One of the earliest methods of solid sample preparation is solvent extraction, often known as “solid-liquid extraction (SLE)” but more accurately referred to as “leaching” or “lixiviation” which corresponds to its physical-chemical basis more closely. It assists in separating and eliminating molecules of interest from insoluble high-molecular-weight fractions as well as from other compounds that can obstruct the analysis next steps. Leaching has traditionally been done extensively by maceration, which relies on the proper solvent selection and the use of heat and/or agitation to speed up the mass transfer and improve the solubility of the constituents. Despite the widespread use of maceration, especially for the separation of natural compounds, this is characterized by lengthy extraction procedures with poor yields (Luque de Castro and Priego-Capote, 2010). This technique is used by most of the algae extraction to get active phytochemicals. The major benefit of this approach is that it has simple reasoning, clear operation, set procedures, and minimal instrument and equipment needs. The selection of organic solvents and the evaluation of extraction parameters (e.g., temperature, time, sample pretreatment, etc.), which have a direct impact on the extraction effectiveness and purity of active ingredients, are crucial to the success of organic solvent extraction. Among these, ethanol is the organic solvent frequently utilized in the extraction of brown algae constituents. Recent developments in the extraction of marine phenolics from marine algae have been based on SLE (Zheng et al., 2022).
Maceration is an easy extraction process; it has the disadvantage of taking a long time and having a low extraction efficiency. It has the potential to be employed for the extraction of thermolabile components. Two solvents, methanol, and acetone, as well as four extraction procedures, probe sonication, bath sonication, tissue grinding, and mortar and pestle maceration, were compared in chlorophyll analysis from freshwater green algae. A probe sonicator was more efficient than the other extraction methods in extracting chlorophyll from methanol. Except for the maceration procedure, methanol was the superior solvent. The maceration approach was equally successful for both solvents but significantly less effective than methanol and probe sonicator. The extraction approach appears to be one of the main steps in chlorophyll analysis (Simon and Helliwell, 1998; Rushdi et al., 2022b). To find the best chlorophyll extraction parameters from dried Laminaria saccharina using canola oil maceration. To calculate the sum of chlorophylls, UV-spectroscopy was used. As a solvent, pure acetone was utilized. As a result, it was determined that the ideal maceration settings for the total extraction of chlorophylls are as follows: The particle size of raw materials must not exceed 1 mm, the maceration period must not exceed 10 days, and a single maceration is sufficient. Additionally, it is simple to modify the protocols utilized in this procedure to yield a variety of molecules of interest. This is made feasible by the ability to use various solvents, temperatures, and agitation settings, enhancing the selectivity and efficiency of mass transfer. Unfortunately, separating the chemicals from the matrix requires multiple cycles of filtration or centrifugation (Garcia-Vaquero et al., 2020). However, percolation is a continuous process in which the saturated solvent is constantly replenished by fresh solvent, and hence, it is more efficient than maceration (Zhang et al., 2018). The decoction extract contains a high concentration of water-soluble contaminants. The decoction cannot be used to extract thermolabile or volatile constituents (Zhang et al., 2018).
Reflux extraction is more efficient than percolation or maceration and uses less solvent and time. It is incompatible with the extraction of thermolabile natural compounds (Zhang et al., 2018). Normal operating parameters include ambient temperature and atmospheric pressure, though heating is also an option. The effectiveness of reflux extraction is higher than that of maceration and percolation, even though it takes slightly less time and solvent to complete the extraction. Additionally, heating and ambient pressure are the operational conditions (Perez-Vazquez et al., 2023).
The extraction of volatile oil is generally accomplished using hydro distillation (HD) and steam distillation (SD). In HD and SD, certain natural substances decompose. The Oman Gulf’s Chabahar region was used to capture the brown algae Nizamuddinia zanardinii. and GC-MS were used to examine the hydro-distilled essential oil of N. zanardinii. A total of 15 substances were found, and the primary components of these were 7, 10, and 13-hexadecatrienoic acid methyl ester (41.8%), followed by hexadecanoic acid methyl ester (19.3%), n-heneicosane (3.6%), and 7, 10-hexadecadienoic acid methyl ester (3.4%). Additionally, certain terpenes, including -element, trans-farnesene, and -ionone, were discovered for the first time in the oil of N. zanardinii (Firouzi et al., 2013). Using a combined steam distillation and solvent extraction method, the red marine algae Polysiphonia sphaerocarpa was extracted, and various brominated chemicals were discovered using gas chromatography-mass spectrometry. 2,4-dibromo anisole, 2,4,6-tribromoanisole, 3-bromo cresol, 3,5-dibromo-4-hydroxybenzaldehyde, 2-bromophenol, 4-bromophenol, 2,4-dibromo phenol, 2,6-dibromo phenol, and 2,4,6-tribromophenol were among the substances found (Flodin and Whitfield, 2000). It is commonly known that marine algae produce volatile organic compounds (VOCs). The VOC from algae has greatly improved because of the headspace collection of volatiles, solid phase microextraction, and the conventional extraction by hydrodistillation combined with analytical chromatographic techniques (i.e., GC-MS). Hydrocarbons, terpenes, phenols, alcohols, aldehydes, ketones, esters, fatty acids, and chemicals containing halogens or sulfur are the main volatile substances found in marine algae (Gressler et al., 2009).
The non-conventional ones include pressurized liquid extraction, microwave-assisted extraction, ultrasound-assisted extraction, and subcritical CO2 extraction, among others. Shorter extraction periods, lower operating temperatures, less solvent used, and process automation are characteristics of new extraction processes. These methods are also seen to be environmentally benign due to the advantages outlined above. To produce greater yields of the target analyte, certain parameters need to be tuned. Therefore, frequent variables to be optimized in these novel techniques include solvent ratio, extraction solvent, extraction time, pH, temperature, and particle size (Perez-Vazquez et al., 2023).
Pressurized liquid extraction (PLE) as accelerated solvent extraction, enhanced solvent extraction, pressurized fluid extraction, accelerated fluid extraction, and high-pressure solvent extraction. High pressure preserves solvents in a liquid condition above their boiling point, resulting in high lipid solute solubility and diffusion rate in the solvent, as well as high solvent penetration in the matrix (Michalak and Chojnacka, 2014; Sosa-Hernández et al., 2018). When compared to other methods, PLE significantly reduced extraction time and solvent usage while improving repeatability. Also been referred to in the literature as accelerated solvent extraction (ASE), pressurized fluid extraction (PFE), or pressurized hot-solvent extraction (PHSE). When water is used as the extraction solvent, this method is also known as superheated water extraction (SHWE), subcritical water extraction (SWE), or pressurized hot-water extraction (PHWE). The general concepts and instrumental needs are the same in both circumstances because only the solvent is different. In general, PLE methods are faster and use less solvent than traditional extraction techniques like Soxhlet extraction. These properties are supplied by the increased mass-transfer rate, increased analyte solubility, and decreases in solvent viscosity and surface tension that occur under PLE conditions. The dielectric constant (ε) of water, which is a measure of the polarity of the solvent and is considerably reduced when water is heated at high temperatures while remaining in the liquid state, affects the extraction in marine algae (Herrero and Ibáñez, 2015). Six species of marine algae from the Northwest of Spain were studied, including F. vesiculosus, D. dichotoma, C. baccata, H. elongata, U. intestinalis, and U. lactuca, for their lipid composition. To extract high-quality lipids from F. vesiculosus algae, the F. vesiculosus algae can be processed using PLE technology to extract high-quality lipids. It was determined how the extraction conditions affected the yield and fatty acids composition of F. vesiculosus. The lipid profile was not significantly affected by temperature in the investigated range (80°C–160°C), according to PLE optimization. Ethyl acetate improves the extraction of long-chain fatty acids (oleic acid), producing extracts with nearly twice the amount of ethanol and acetone solvents, after testing five different polarity solvents (hexane, ethanol, ethyl acetate, acetone, and ethanol: water (50:50). Although the most polar solvents, such as ethanol and ethanol: water 50:50, produced the best (lowest) ω-6/-ω3 ratios (Otero et al., 2018). Pressurized liquid extraction with solid-phase extraction (PLE-SPE) combination is the foundation of a novel extraction method. The technique was used to extract bioactive phenolic acids (protocatechuic, p-hydroxybenzoic, 2,3-dihydroxybenzoic, chlorogenic, vanillic, caffeic, p-coumaric, salicylic acid), cinnamic acid, and hydroxybenzaldehydes (p-hydroxybenzaldehyde, 3,4-dihydroxy benzaldehyde, vanillin) from in vitro cultures of two fresh freshwater algae (Anabaena doliolum and Spongiochloris spongiosa) and from food products of marine macroalgae Porphyra tenera (nori) and Undaria pinnatifida (wakame). The marine and freshwater algae products had sub-microgram or microgram levels of the phenols listed above per Gram of lyophilized material (Onofrejová et al., 2010).
The extraction solvent in supercritical fluid extraction (SFE) is supercritical fluid (SF). SF has a liquid-like solubility and a gas-like diffusivity, and it can dissolve a wide range of phytochemicals. Because of minor pressure and temperature changes, their solvating characteristics altered substantially around their critical points. Because of its appealing properties such as low critical temperature (31°C), selectivity, inertness, low cost, non-toxicity, and capacity to extract thermally labile chemicals, supercritical carbon dioxide (S-CO2) was frequently utilized in SFE. S-CO2’s low polarity makes it perfect for extracting non-polar phytochemicals such as lipids (Michalak and Chojnacka, 2014; Sosa-Hernández et al., 2018). To considerably improve S-CO2 solvating properties, a modifier can be added. Daidzin, Genistin, Daidzein, Ononin, Sissotrin, Genistein, Formononetin, and Biochanin A, isoflavones detected in aqueous methanol extract of (Sargassum muticum, Sargassum vulgare, Hypnea spinella, Porphyra sp., Undaria pinnatifida, Chondrus crispus, and Halopytis incurvus) using Hyphenated technique for the extraction Ultrasound-assisted supercritical fluid extraction followed by fast chromatography with tandem mass spectrometry (Klejdus et al., 2010). To extract valuable phytochemicals from marine algae, innovative extraction techniques such as supercritical fluid extraction, microwave-assisted extraction, ultrasound-assisted extraction, enzyme-assisted extraction, and pressurized liquid extraction have been studied. Based on their concentrations of high added-value metabolites like fucoxanthin and polyphenols, six species of Dictyotales (Dictyopteris polypodioides, Dictyota dichotoma, Dictyota fasciola, Dictyota spiralis, Padina pavonica, and Taonia atomaria) collected from the Tunisian coasts were examined. High-performance liquid chromatography (HPLC) was used to quantitatively assess polyphenols and fucoxanthin, respectively. Therefore, T. atomaria and D. polypodioides had the highest amounts of fucoxanthin (5.53 ± 1.2 and 3.43 ± 1.3 mg/g dry weight, respectively), whereas D. spiralis had the lowest value (0.23 ± 0.1 mg/g dry weight). The highest total phenol content was produced by D. dichotoma and T. atomaria (19.3 ± 0.4 and 15.2 ± 1.1 mg/g dry weight, respectively). The most prevalent species, D. polypodioides, was employed in the second step to extract fucoxanthin using supercritical carbon dioxide (ScCO2). The optimal extraction parameters for fucoxanthin extraction by ScCO2 were 60°C temperature and 50-MPa pressure, which resulted in an extraction yield that ranged from 0.50% ± 0.04% to 1.32% ± 0.02%. The extract’ highest levels of fucoxanthin and polyphenol recovery were 15% and 64%, respectively (Ktari et al., 2021).
Polysaccharides like carrageenan and alginate, pigments like fucoxanthin, chlorophylls, or beta-carotene, and phenolic compounds, among others, have been extracted using this technique from a variety of marine algae. This makes the use of UAE to characterize marine algae as a new supply of metabolites, notably suitable for vegetarian and vegan diets, an effective and sustainable method (Carreira-Casais et al., 2021). Ultrasound-assisted extraction (UAE), often known as ultrasonic extraction or sonication, extracts using ultrasonic wave energy. Ultrasound in the solvent results in cavitation, which increases solute dissolution and diffusion as well as heat transfer, improving extraction efficiency. Another advantage of UAE is that it uses less solvent and energy, and it reduces extraction temperature and time. UAE can be used to extract thermolabile and unstable chemicals (Michalak and Chojnacka, 2014). UAE is extensively used in the extraction of a wide range of natural goods (Vázquez-Rodríguez et al., 2020). This method makes use of ultrasonic waves, which have frequencies between 20 kHz and 10 MHz and are located between audible and microwave ranges. Within the ultrasound spectrum, two areas can be distinguished, power ultrasound (20–100 kHz), which has a high intensity and is used for extraction and processing applications; and signal or diagnostic ultrasound (100–10 MHz), which is used as a clinical diagnostic technique as well as for control and quality assessment (Tiwari, 2015). Water and acidic solutions are the most used solvents in the extraction of carbohydrates from marine algae. In general, the extraction is done at room temperature, and the extraction time (ET) is roughly 1 h, though greater ET has been documented (Chemat et al., 2017). It is vital to remember that the extraction pH has a significant impact on protein extraction and isolation. Ultrasound-assisted extraction was utilized to extract protein from marine algae (Yücetepe et al., 2018). Regarding the extraction of carbohydrates from algae, water, and acidic solutions are the most employed solvents. In general, the extraction is performed at room T, while ET is about 1 h, although longer ET has been reported. Three pretreatment procedures revealed that ultrasound-assisted extraction (UAE) was particularly successful. The impacts of four parameters on extraction efficiency were also studied (ultrasonic power, extraction time, flow rate, and algal concentration, in that order) (Zhao et al., 2013).
One of the most promising methods for extracting high yields of phytochemicals (i.e., polysaccharides, proteins-peptides, lipids, polyphenols, and carotenoids) from macroalgae is microwave-assisted extraction (MAE), which also uses less energy, organic solvents, and waste production than traditional extraction methods (Gomez et al., 2020). Microwaves create heat by interacting with polar chemicals in the marine algae matrix, such as water and some organic components, using the ionic conduction and dipole rotation mechanisms (Michalak and Chojnacka, 2014; Sosa-Hernández et al., 2018). In MAE, heat and mass transfers are in the same direction, resulting in a synergistic effect that speeds up extraction and improves extraction yield. MAE use has numerous benefits, including increased extract yield, reduced thermal degradation, and selective heating of vegetal material. MAE is also classified as a green technique because it uses fewer organic solvents. MAE procedures are classified into two types: solvent-free extraction (often for volatile compounds) and solvent extraction (typically for non-volatile substances) (Rodriguez-Jasso et al., 2011).
Because it can improve mass transfer during extraction by disrupting membrane structures, pulsed electric field extraction greatly boosts extraction yield and decreases extraction time. PEF treatment success is determined by several factors, including field strength, specific energy input, pulse number, and treatment temperature. PEF extraction is a non-thermal approach that reduces thermolabile chemical degradation (Kempkes, 2016). Three Icelandic marine algae aqueous extracts, created by pulsed electric fields (PEF), underwent a screening for possible cosmetic uses. In terms of polyphenol, flavonoid, and carbohydrate content, PEF-produced extracts from U. lactuca, Alaria esculenta, and Palmaria palmata were compared to the conventional hot water extraction. PEF demonstrated outcomes comparable to those of the conventional method while demonstrating various benefits, including its non-thermal nature and quicker extraction time (Castejón et al., 2021).
According to earlier research, using enzymes to extract phytochemicals from terrestrial plants for food and nutraceuticals like EAE has several benefits over conventional extraction techniques. Due to the complex and varied structure and content of marine algae cell walls compared to those of terrestrial plants, it is challenging to apply EAE methods to recover active chemicals from marine algae. Particularly, cellulose and hemicellulose predominate in marine algae walls, making them chemically more complex than those of land plants. The ability of enzymes to hydrolyze marine algae cell wall components, destroying its structural complexity by generating an enzyme-substrate binding complex, and releasing the cell content into the environment is generally what causes EAE for marine algae (Michalak and Chojnacka, 2014; Sosa-Hernández et al., 2018). Marine algae biomass is handled with enzymes as a processing ingredient in the majority of investigations on the enzymatic hydrolysis of marine algae. Therefore, the majority of investigations focus on EAE rather than a particular enzymatic hydrolysis. Carbohydrases and proteases are the two enzymes that are most frequently utilized to extract bioactive chemicals from marine algae. A few of the often used enzymes are xylanase, agarase, Alcalase®, amylase, arabinase, carrageenanase, Celluclast®, cellulase, Flavourzyme®, glucanase, KojizymeTM, Neutrase®, protease, and Ultraflo® (Sanjeewa et al., 2023).
The components in the extract from the preceding procedures are complicated and contain a variety of natural products that must be separated and purified further to achieve the active fraction or pure natural products. The separation is determined by the physical or chemical differences between each natural product. Chromatography, particularly column chromatography, is the most common method for isolating pure natural compounds from a complicated mixture. Separation techniques shall be discussed in the following subsections.
Because of the simplicity, high capacity, and low cost of adsorbents such as silica gel and macroporous resins, adsorption column chromatography is frequently employed for the separation of metabolites, particularly in the first separation step. The separation is based on differences in the adsorption affinities of the natural products for the adsorbent surfaces. The choice of adsorbents (stationary phase) and mobile phase is critical for achieving effective separation of metabolites, maximizing target compound recovery, and avoiding irreversible adsorption of target compounds onto the adsorbents. In phytochemical research, silica gel is the most employed adsorbent. It was estimated that silica gel was used in about 90% of phytochemical separation (preparative scale). Silica gel is a silanol-containing polar absorbent. The silica gel holds molecules in place via hydrogen bonding and dipole-dipole interactions. As a result, metabolites last longer in silica gel columns than nonpolar ones. Certain polar metabolite compounds may experience irreversible chemisorption at times. Adsorption is reduced when silica gel is deactivated by adding water before usage or by using a mobile phase containing water. When separating alkaloids on silica gel, severe tailing may occur; however, the addition of a small amount of ammonia or organic amines such as triethylamine may minimize the tailing. Caulerpa taxifolia extract was used to extract an active inhibitor called caulerpenyne, which was then purified using ethyl acetate extraction and subsequent chromatography on silica gel columns (Bitou et al., 1999; Rushdi et al., 2020a). Sulfoquinovosyl diacylglycerol (SQDG) has been isolated from the red algae Osmundaria obtusiloba that grows on the coast of southeast Brazil. Chloroform/methanol was used to extract the acetone-insoluble material, and the glycolipid-enriched lipid fraction was separated on a silica gel column before being eluted with acetone, chloroform, and finally methanol (De Souza et al., 2012; Rushdi et al., 2020b). A new sterol, 24-R-stigmasta-4,25-diene-3,6-diol, was isolated from the green algae Codium divaricatum Holmes, a traditional Chinese medicine that is effective against cancer, along with three other sterols: clerosterol, loliolide, and 24-R-3-O-d-glucopyranosyl-stigmasta-5,25-diene. Si-gel CC (160–200 and 300–400 mesh) was used for column chromatography (He et al., 2010). Utilizing preparative circular chromatography (Chromatotron) on silica gel in an N2 environment with n-hexane/acetone 8:2 as the mobile phase, carotenoids such as peridinin and β -carotene were extracted from Gonyaulax polyedra (Pinto et al., 2000). An easy-column method that requires minimal reagent to separate aromatic hydrocarbons, particularly polycyclic aromatic hydrocarbon compounds (PAHs), from crude oils. To successfully separate PAHs into sub-fractions, it was necessary to identify the most effective and dependable column chromatography approach. Investigators discovered that a successful column chromatography separation depends on the type of column that is used. Analysis showed that the silica-alumina (1:1) column is selected to be the best among the two columns (alumina and silica-alumina 1:1) for separating PAHs into various sub-fractions when using alumina and silica-alumina at a ratio of 1:1 for the separation of the aromatic fraction (Rushdi et al., 2021; Konan et al., 2022). Alumina (aluminum oxide) is a strong polar adsorbent that is utilized in the separation of natural compounds, particularly alkaloids. Adsorption on alumina differs from that on silica gel due to the strong positive field of Al3+ and the basic sites in alumina affecting readily polarized molecules. Because alumina can promote dehydration, breakdown, or isomerization during separation, its use in natural product separation has declined dramatically in recent years (Zhang and Su, 2000). Polyamides used in chromatography have both acryl and amide groups in their structures. Depending on the mobile phase composition, hydrophobic and/or hydrogen bond interaction will occur in polyamide column chromatography. When polar solvents, such as aqueous solvents, are employed as the mobile phase, polyamides serve as the non-polar stationary phase, and the chromatography behaves similarly to reversed-phase chromatography. Polyamides, on the other hand, operate as the polar stationary phase, and the chromatographic behavior is identical to that of normal phase chromatography. Polyamide column chromatography is a well-established method for separating natural polyphenols such as anthraquinones, phenolic acids, and flavonoids, the mechanisms of which are attributed to the creation of hydrogen bonds between polyamide absorbents, mobile phase, and target metabolites. The chromatographic behavior of polyphenols on polyamide columns, including phenolic acids and flavonoids, the polyamide was discovered to operate as a hydrogen bond acceptor, and the number of phenolic hydroxyls and their locations in the molecule controlled the adsorption strength. Polyamide column chromatography was used to enhance the total saponins of Kuqingcha, Adsorptive macroporous resins are polymer adsorbents with macroporous structures but no ion exchange groups that can absorb practically any natural substance selectively. Because of their features, such as strong adsorptive capacity, cheap cost, fast regeneration, and easy scale-up, they have been frequently utilized as a standalone system or as part of a pretreatment process for eliminating contaminants or enriching target compounds. Electrostatic forces, hydrogen bonding, complex formation, and size-sieving processes between the resins and the natural products in solution are among the adsorptive mechanisms of adsorptive macroporous resins. The main elements influencing resin capacity are surface area, pore diameter, and polarity. Silver nitrate is another useful solid support in the separation of natural products. Natural products containing electrons reversibly interact with silver ions to form polar complexes. The greater the number of double bonds or aromaticity of the natural product, the stronger the complexation forms.
Conventional column chromatography takes a long time. Therefore, this issue is solved by flash chromatography, which forces the mobile phase through the column at a medium pressure of roughly 10 psi of nitrogen or air. Poor separation efficacy was the outcome of the rapid mobile phase rate (Kondeti et al., 2014) Therefore, flash chromatography uses significantly smaller silica gel particles (230–400 mesh) (40–60 μm) in a short glass column with a large inner diameter in order to increase the quality of column separation (Roge et al., 2011).
These days, a number of automated flash purification systems include automated fraction collectors and microcomputer-controlled detectors in addition to prepacked cartridges with various silica weights and particle sizes through which solvent is delivered by high efficiency solvent pumps. A dual column flash chromatography method was also used in several investigations (Surve et al., 2023).
Several studies have reported the use of flash chromatography to isolate various bioactive compounds from microalgae. For example, high purified lutein was isolated from Chlorella regularis using hexane as the first eluent, which yielded a mixture of α,β carotenes. The eluent was then changed to hexane-acetone-chloroform (7:2:1 v/v) to isolate lutein with a 60% yield (Shibata et al., 2004).
Another study employed gradient elution of petroleum ether and acetone at a flowrate of 8.0 mL/min to purify the carotenoids content of Asteracys quadricellulare microalgae using automated flash chromatography. The findings indicated the presence of β-carotene, lutein, canthaxanthin, and astaxanthin at concentrations of 47, 15.5, 14, and 28.7 μg/mg dry weight biomass, respectively (Singh et al., 2019).
In addition to isolating carotenoids, flash chromatography can also separate other beneficial substances including omega 6 polyunsaturated fatty acids. Due to the high gamma linolenic acid content of Spirulina sp., a study was conducted to assess the viability of using flash chromatography to separate methyl gamma linolenate from Spirulina platensis. According to the findings, an Isolera automated high performance flash chromatographic system using a hexane-acetone (6:4) solvent solution was able to isolate methyl gamma linolenate with a yield of 71% w/w (S et al., 2015).
A separation material that combines adsorption and screening is macroporous resin, commonly referred to as large grid adsorbent. A nonionic polymer with stable characteristics is macroporous resin. It does not interact with inorganic salts, strongly ionic chemicals, or low molecular weight molecules, and it is insoluble in alkalis and organic solvents. The separation, extraction, and purification of natural goods now employ it extensively. Leyton et al. (2017) assessed the adsorption capability of six resins (HP-20, SP-850, XAD-7, XAD-16N, XAD-4, and XAD-2) to purify the phlorotannins. Phlorotannins that had been purified with XAD-16N showed the best efficiency (42%), under the same adsorption duration. The active components in Macrocystis pyrifera might be isolated using a macroporous adsorption resin called HP-20 as an adsorbent and a methanol-water system as an elution agent (Zheng et al., 2022).
In thin layer chromatography (TLC), a uniform thin layer is created by coating glass plates, plastic, or aluminum substrates with a suitable stationary phase, such as silica gel, diatomaceous earth, alumina, or microcrystalline cellulose, and uniformly grinding an adhesive. The retardation factor (Rf) is compared with the Rf of the reference substance solution after the capillary spotting has been unfolded in an organic solvent to identify the active metabolites, check for impurities, and ascertain the quantity. This technique is quick, sensitive, and simple to use. For better separation and purification of brown algae polyphenols, thin-layer chromatography can be combined with column chromatography and the macroporous adsorption resin technique (Zheng et al., 2022). The chlorophylls and carotenoids of green algae and brown algae were separated using thin layers of specially produced sucrose. Pigments separated included chlorophylls a, b, and c, pheophytins a and b, carotenes, lutein, violaxanthin, neoxanthin, diatoxanthin, diadinoxanthin, dinoxanthin, fucoxanthin, neo fucoxanthin, peridinin, neo peridinin, and other minor metabolites. On this adsorbent, there was no pigment degradation, and after two-dimensional chromatography, recoveries of 1–2 g of various colors averaged 95%. Thin layers of cellulose were used to separate chlorophyll degradation products that could not be separated on sucrose plates, whereas quantitative thin layers of polyethylene were used to separate chlorophyll c derivatives and thin layers of quantitative thin layers of alumina-magnesium oxide to separate carotene isomers. The techniques are quick and easy, yet because of the sensitivity (Jeffrey, 1968).
A large inner diameter, high load separation column is used in preparative high-performance liquid chromatography (Prep-HPLC), which allows for highly effective extraction and purification of raw materials. Its basic operation is to use a high-pressure infusion pump to convert the liquid used as the mobile phase into the column. To accomplish the goal of separating each component, the mobile phase and fixed phase’s composition and affinities are distinct, allowing them to flow out of the stationary phase sequentially (López et al., 2011). The isolation of monomeric phlorotannins can be accomplished using the octyl decyl silane (C18) column as the stationary phase, elution with methanol-water, 230 nm UV detection, and continual technique improvement (Kim J. et al., 2016b).
Hexadeca-4Z,7Z,10Z,13Z-tetraenoic acid, which has been linked to decreased cisplatin efficacy, is abundant in the Undaria pinnatifida algae. Undaria pinnatifida was employed as the raw material for its extraction to produce an extract of these −3 polyunsaturated fatty acids that were high in fatty acids for use as an analytical standard. A two-step extraction procedure using 3-aminopropyl silica, and a normal-phase elution mode was devised. It starts with a liquid-liquid extraction and is followed by a solid-phase extraction. The simultaneous comprehensive group selective fatty acids profiling in an untargeted manner and the quantitative analysis of the targeted fatty acid were made possible by ultra-high performance liquid chromatography with electrospray ionization tandem mass spectrometry method. High-resolution product ion spectra were used to identify hexadeca-4Z,7Z,10Z,13Z-tetraenoic acid (Schlotterbeck et al., 2018).
Partition chromatography (PC) is based on the idea of liquid-liquid extraction, which is based on the relative solubility of two separate immiscible liquids. As the stationary phase, one liquid phase was coated on a solid matrix (silica gel, carbon, cellulose, etc.) while another liquid phase was used as the mobile phase. Because of the disadvantages of an easily removed stationary phase and unrepeatable findings, this type of PC is rarely utilized any more. These limitations are overcome by the bonded phase, in which the liquid stationary phase is chemically attached to the inert support employed as the stationary phase. Commercially accessible alkyl (C8 and C18), aryl, cyano, and amino-substituted silanes are frequently utilized as bound phases in the separation of a wide range of materials. Diphlorethohydroxycarmalol (DPHC), one of the principal components of Ishige okamurae, is recognized for its antiviral and anti-inflammatory properties. To successfully separate significant amounts of antioxidant chemicals from marine algae, preparative centrifugal partition chromatography (CPC) in conjunction with 2,2′-azino-bis (3-ethylbenzothiazoline-6-sulphonic acid) (ABTS+) online HPLC was used. By using ABTS+ online HPLC and preparative CPC systems, two major antioxidant chemicals, DPHC and octaphlorethol A (OPA), respectively, were verified and extracted from the ethyl acetate (EtOAc) fraction of I. okamurae. Both liquid chromatography with diode array detection and electrospray ionization mass spectrometry (LC-DAD-ESI/MS) and ABTS+ online HPLC systems were used to validate the presence of DPHC and OPA in the EtOAc fraction of I. okamurae: DPHC (39 mg) and OPA (23 mg) (Kim H.-H. et al., 2016a). A typical brown alga found in the Laminariaceae family, Isenia bicyclis (Kjellman) Setchell is found near the coasts of China, Japan, and Korea. It contains fucoxanthin, using a novel separation technique called centrifugal partition chromatography (CPC). The investigation’s objective was to quantify and prepare isolate fucoxanthin from fresh Eisenia bicyclis. The solvent partition method was used to create the fucoxanthin fraction (Fuco fraction) from the acetone extract of fresh E. bicyclis. Using a two-phase solvent solution of n-hexane, ethyl acetate, ethanol, and water (5:5:7:3, v/v/v/v), fuco fraction was employed for CPC. The mobile phase was flowing at a rate of 2 mL/min while rotating at 1,000 rpm. 410 nm was used to monitor the eluate. HPLC, UV, APCI/MS, and NMR spectra were used to establish the presence and chemical composition of fucoxanthin in the CPC fraction., From 516 mg of fuco fraction containing 4.59% fucoxanthin, a preparative CPC produced 20 mg of fucoxanthin (87% recovery from fuco fraction) in a two-step separation. In the first CPC phase, the purity of the extracted fucoxanthin was approximately 81%, and over 98% (Kim et al., 2011). It has been established that Sargassum horneri contains a significant class of bioactive compounds called phytosterols. This study suggests using counter-current chromatography to separate phytol and two analog sterols from a crude S. horneri extract. The n-hexane-acetonitrile-methanol (5:5:6, v/v) two-phase solvent solution was chosen and optimized. Careful research was done on how rotating speed and flow rate affected the retention of the stationary phase. The ideal conditions led to the baseline separation of phytol and its two analog sterols, fucosterol and saringosterol, yielding 19.8 mg phytol, 23.7 mg fucosterol, and 3.1 mg saringosterol (Xia et al., 2019). For the quick extraction of phytosterols from edible sea algae, a method using microwave-assisted extraction in conjunction with high-speed counter-current chromatography and a UV detector was developed. Orthogonal array design was used to improve the extraction conditions, including the microwave power, liquid/solid ratio, irradiation period, and extraction temperature. Undaria pinnatifida and Sargassum fusiforme’ microwave-assisted extractions were separated and purified using a non-aqueous two-phase solvent solution made up of n-hexane, acetonitrile, and methanol (5:5:3, v/v/v). The isolation took less than 220 min, and 15.0 g of U. pinnatifida yielded 13.0 mg of fucosterol, 1.5 mg of 24-methylene cholesterol, and 10.7 mg of phytol, while 15.0 g of S. fusiforme yielded 4.6 mg of fucosterol, 0.3 mg of 24-methylene cholesterol, and 3.5 mg of phytol. All items had purity levels of over 97% (Xiao et al., 2013).
Natural products are separated using membrane filtration (MF) or gel filtration chromatography (GFC) based on their molecular sizes. The purifying procedure may use membrane filtration or gel filtration chromatography.
The semipermeable membrane in MF lets tiny molecules pass through while retaining larger molecules. Based on the pore size of the membrane used, natural product MF can be classified as microfiltration, ultrafiltration, or nanofiltration. Membrane filtration has proven to be an effective method for impurity concentration, clarity, and removal in the laboratory, as well as in the food and pharmaceutical industries. A commercially accessible, industrial-scale setup was employed. In both filtering setups, a variety of membrane materials, including polyether sulfone, polyacrylonitrile, etc., and mean pore sizes (ranging from 7,000 Da to 0.2 m) (Nurra et al., 2014). Many biomolecules from various sources are separated and purified using ultrafiltration. However, the transmembrane flux, yield, and purity of ultrafiltration differ depending on the biomolecule and process parameters. In the current investigation, R-Phycoerythrin was extracted from the marine macroalgae Gelidium pusillum using ultrafiltration. Standardized parameters were transmembrane pressure (1, 2, and 4 bar), pH (4, 5, 6, and 7), ionic strength (control, 0.5 and 1.0 M), and molecular weight cut-off (30, 50, 100, and 300 kDa). To maximize R-Phycoerythrin purity, ultrafiltration in diafiltration mode and its integration with ammonium sulfate precipitation and aqueous two-phase extraction were also used. The transmembrane (permeate) flux was improved by in-situ ultrasonication (Mittal et al., 2020). Attention has been drawn to membrane separation methods such as diafiltration, ultrafiltration, reverse osmosis, and nanofiltration for the fractionation of marine algae polysaccharides for their potential industrial uses (Xu et al., 2017).
Gel permeation chromatography and size exclusion chromatography are other names for gel filtration chromatography. Small molecules are retained in GFC for a longer period than big compounds. Sephadex is made by cross-linking dextran, and the G-types of Sephadex have been utilized to separate hydrophilic molecules such as peptides, oligosaccharides, and polysaccharides. Sephadex LH20, a hydroxypropylated derivative of Sephadex G25, is hydrophobic as well as hydrophilic. Separation with Sephadex LH-20 also included an adsorption mechanism. Sephadex LH-20 can be used to separate a wide range of natural products in both aqueous and non-aqueous solvent systems. By combining affinity chromatography on pig stomach mucin-Sepharose 6B and gel filtration on Sephadex G-50, a lectin from the green marine algae U. lactuca was recovered. The lectin was divalent cation dependent and stable across a range of pH and temperature (Sampaio A. et al., 1998). Affinity chromatography was used to separate a lectin from the red marine algae Ptilota filicina (PFL) on cross-linked guar gum. PFL was found to be low in basic amino acids but high in acidic and hydroxyl amino acids (Sampaio A. H. et al., 1998).
Ion-exchange chromatography (IEC) separates molecules based on their net surface charge differences. Some natural compounds, such as alkaloids and organic acids with ionizing functional groups, may be separated using IEC. By varying the ionic strength of the mobile phase (e.g., changing pH or salt content), charged molecules could be captured and released by ion-exchange resin. Alkaloids were separated using cation ion-exchange resins, while natural organic acids and phenols were separated using anion ion-exchange resins. R-phycoerythrin was isolated and purified from Gracilaria verrucosa using ion-exchange chromatography and an expanded-bed adsorption column, which successfully addresses the issue of chromatographic column blockage brought on by polysaccharides during the isolation and purification of phycobiliproteins. The expanded-bed column could be best eluted with 0.1 M (NH4)2SO4, and the R-phycoerythrin was purified using an ion-exchange column with desalted 0.1 M (NH4)2SO4 eluate and the yield of purified R-phycoerythrin can reach 0.141 mg/g of the frozen algae (Wang, 2002). Column chromatography techniques like ion-exchange, size-exclusion, and affinity chromatography were predominantly used to purify polysaccharides. The separation of neutral/acidic polysaccharides from negatively charged polysaccharides by gradient salt elution or a change in pH is often a good fit for ion-exchange chromatography. Laminaria japonica aqueous extracts were fractionated using gradient elution with 0.7–0.8 mol/L NaCl on a diethylamino ethyl (DEAE)–A25 anion-exchange column. Polysaccharides from marine algae with various molecular weights or sizes can be separated using size-exclusion chromatography. Three fractions of low molecular weight oligosaccharides were then collected from a Bio-gel P-4 size-exclusion column after enzymatic hydrolysis of a sulfated polysaccharide from green algae that had initially been purified using a Sephacryl S-300 HR size-exclusion column (Xu et al., 2017) Using extraction with 20 mM PBS, precipitation with 70% saturated ammonium sulphate, ion-exchange DEAE-Cellulose chromatography, and RP-HPLC, a lectin from the red marine algae Hypnea musciformis (HML) was isolated. Under certain MALDI-TOF/MS settings, the 9.3 kDa polypeptide exhibits oligomerization tendencies and agglutinates erythrocytes from a variety of sources. N-terminal sequencing preliminary (Nagano et al., 2002).
With its high separation efficiency and rapid separation and analysis, gas chromatography (GC) has the potential to be the ideal preparative technology for the separation of volatile substances. Due to a lack of commercially available equipment, the injection port, column, split device, and trap device of GC equipment must be adapted for preparative separation. Prep-GC. Prep-GC has become a popular approach for separating natural volatile chemicals; nevertheless, a larger sample load and the use of a large-diameter preparative column reduced efficiency. Meanwhile, the disadvantages of Prep-GC, such as the lack of commercial Prep-GC equipment, the large volume of carrier gas consumed, the decomposition of thermolabile compounds at high operation temperatures, the difficulties of fraction collection, and low production, continue to limit its use. Polychlorinated biphenyls (PCBs), organochlorine pesticides (OCPs), polycyclic aromatic hydrocarbons (PAHs), and brominated flame retardants (BFRs) have all been the subject of analysis in marine laboratories since the 1960s. While gas chromatography (GC) has been heavily utilized in the ultimate assessment of these pollutants, column chromatography and liquid chromatography (LC) techniques have been used mostly in the clean-up phase. The usage of packed GC columns, capillary columns, heart-cut multi-dimensional GC, and complete multi-dimensional GC have all undergone developments (de Boer and Law, 2003). The methods for sampling and cleanup, fractionation and enrichment of marine pollutants, capillary gas chromatography (cGC), and high-performance liquid chromatography using both conventional and chiral stationary phases are all covered in the chromatographic separation of marine organic pollutants. For polychlorinated biphenyls (PCBs), the potential of multi-dimensional cGC for the investigation of marine organic trace contaminants is highlighted. An additional perspective on the hazardous potential of these marine organic pollutants comes from the chromatographic separation of coplanar PCBs and the enantiomers of chiral contaminants (Hühnerfuss and Kallenborn, 1992).
The mobile phase in SFC is a supercritical fluid. SFC combines the benefits of both GC and liquid chromatography (LC) since supercritical fluids have the high dissolving capability, high diffusivity, and low viscosity, allowing for rapid and efficient separation. As a result, SFC can utilize a longer column and smaller stationary phase particles than HPLC, resulting in a higher number of theoretical plates and better separation. SFC can be used to separate non-volatile or thermally labile chemicals that GC cannot separate. SFC systems work with a variety of detectors, including those found in LC and GC systems (Taylor, 2010). Because the polarity of the generally utilized mobile phase, S-CO2, in SFC is close to that of hexane, Supercritical fluid chromatography (SFC) is a developing technique for preparative separation because it uses supercritical fluids, which significantly reduces the need for organic solvents and because new hardware has recently become commercially available, making SFC an attractive alternative. Regarding selectivity, adaptability, and sensibility, SFC meets high standards. High-value chemicals from natural sources can be fractionated or purified by SFC (Montañés and Tallon, 2018). Instrumentation for SFC can be obtained commercially or by adapting systems used for either liquid chromatography (LC) or gas chromatography (GC). Most detectors used in GC and HPLC can be used with SFC, but flame ionization detectors (FID) and ultra-violet (UV) are used. Coupled detectors employed are Mass spectrometry (MS) and Fourier-transform infrared spectroscopy (FTIR). SFC is faster than LC because supercritical fluids have low viscosities and less pressure drop across the column. SFC advantages compared to GC include that no derivatization is needed, and it is possible to analyze thermally labile compounds and solutes of higher molecular weight (West and Lesellier, 2011). Open tubular and packed columns are used. Currently, all companies manufacturing SFC-packed columns are offering a similar catalog of products. Due to the finite body of knowledge of the interaction between analytes and SFC systems, it is difficult to select the most appropriate stationary phase a-priori (West and Lesellier, 2006). Due to the high solubility of these analytes in supercritical CO2. SFC now has access to a significantly wider range of polar application locations thanks to innovations in mobile phases. Peptides, phospholipids, polyphenols, naturally occurring antioxidants, trace sugars, and alkaloids are just a few examples. The ability of SFC to analyze more polar substances, like amino acids or carbohydrates, has been demonstrated in several experiments employing mobile phases adjusted with a specific amount of water (Fornari and Stateva, 2015). Polyunsaturated omega-3 fatty acid concentrations, carotenoid-rich extracts, and phospholipid-rich extracts from marine sources. The use of ultra-high pressures for CO2 extraction, the use of propane and dimethyl ether as substitute extraction solvents, and the production of omega-3 concentrates using semi-preparative supercritical chromatography are the main research and development trends observed in the processing of feed streams to produce these high value lipid products (Catchpole et al., 2018).
Both purple perilla leaf (Perilla frutescens var. crispa) and green perilla leaf (Perilla frutescens var. frutescens) are varietal varieties of the herb Perillae Folium (PF), which is widely used as a dietary vegetable and medicinal herb. The components and effectiveness of many PF variations, however, have not yet been thoroughly studied. The difference in chemical contents between green PF and purple PF was investigated in the current work using a nontargeted rapid resolution liquid chromatography coupled with a quadruple-time-of-flight mass spectrometry (RRLC-Q/TOF-MS)-based metabolomics technique. Seven phenolic acids, ten flavonoids, and nine anthocyanins were identified or possibly recognized as differential metabolites out of a total of 71 substances. Additionally, heatmap visualization and quantitative analysis based on ultra-performance liquid chromatography combined with triple-quadrupole tandem mass spectrometry (UPLC-TQ-MS/MS) were revealed. compositions that fall between purple and green PF. Seven phenolic acids, ten flavonoids, and nine anthocyanins were identified or possibly recognized as differential metabolites out of a total of 71 substances. Additionally, a quantitative investigation based on UPLC-TQ-MS/MS-based ultraperformance liquid chromatography and triple-quadrupole tandem mass spectrometry (UPLC-TQ-MS/MS) showed that flavonoids and anthocyanins in particular had higher levels in purple PF. Furthermore, human umbilical vein endothelial cells (HUVECs) and zebrafish were used to test the anti-oxidative properties of two different PFs. Due to the presence of anthocyanins and a higher concentration of flavonoids in the purple PF’s phytochemical profile, the purple PF’s anti-oxidative activities were more prominent than those of the green PF, according to the results. The findings of the current investigation (Zheng Y.-F. et al., 2020). For the extraction and identification of isoflavones in freshwater and saltwater algae as well as cyanobacteria, a new hyphenated approach was created. The procedure includes preparation of the sample with sonication, extraction using supercritical CO2 modified by 3% (v/v) of MeOH/H2O mixture (9:1, v/v) at 35 MPa and 40°C for 60 min, rapid chromatographic analysis using Agilent 1,200 Series Rapid Resolution, and MS/MS determination. With a linear gradient (30% B at 0 min, from 0 min to 3 min up to 50% B, from 3 to 5 min), an Agilent 1,200 Series RRLC was used with a Zorbax SB-CN chromatographic column (100 and 2.1 mm, particle size 3.5 m), 3 L injection volume, and mobile phase made up of 0.2% (v/v) acetic acid in water (solvent A) and acetone (Klejdus et al., 2010). Agilent 1,200 Series RRLC was used with Zorbax SB-CN chromatographic column (100 and 2.1 mm, particle size 3.5 m), 3 L injection volume, mobile phase made up of 0.2% (v/v) acetic acid in water (solvent A) and acetonitrile (solvent B), and linear gradient (30% B at 0 min, 50% B at 3 min, 80% B at 6 min, and 30% B at 10 min) were all used. The column oven’s temperature was 35°C, and the flow rate was 0.4 mL/min. Under the following circumstances, the MS detector Agilent Technologies 6,460 Triple Quadrupole LC/MS with Agilent Jet Stream was employed in a negative ESI mode: 350°C for the gas, 13 L/min for the flow, 50 psi for the nebulizer gas, and sheath gas (Klejdus et al., 2010).
Because of its unique characteristics, such as high selectivity, cheap cost, and ease of preparation, molecular imprinting technology has been an appealing separation approach throughout the previous decade. When the template molecules are removed from the molecular imprinted polymer (MIP), several complementary cavities with the memory of the template molecules’ size, shape, and functional groups are formed. As a result, the template molecule and its analogs will have specific recognition and adsorption for the MIP. MIPs have been frequently utilized to enrich minor components in the separation of natural products or as solid-phase extraction sorbents for sample preparation of herbal items. Through food chains, paralytic shellfish toxins (PSTs) endanger human health. For forecasting the safety of seafood, it is essential to comprehend how PSTs vary in seawater. Because of the extremely low concentrations and matrix interferences, most documented methods for the detection of PSTs in microalgae or shellfish are not relevant in saltwater. Molecularly imprinted solid-phase extraction (MISPE), quadrupole exactive orbitrap, and high-resolution mass spectrometry (HRMS) are considered excellent methods for minimizing matrix interference. Two typical marine toxins found in PSTs are GTXs 2 and 3. In this study, a sensitive technique with a limit of detection (LOD) of 47.4 ng/L in seawater was established using liquid chromatography (LC-HRMS) and MISPE (Zhang et al., 2020).
Multiple columns with stationary phases (bed) are used in simulated moving bed (SMB) chromatography. The bed’s countercurrent movement is imitated by rotary valves that alternately switch the intake (feed and eluent) and outlet (extract and raffinate). The SMB process is a continuous separation method and a potent tool for large-scale natural product separation, with the advantage of decreased solvent consumption over a shorter time. Using a simulated moving bed (SMB), the caulerpin was separated from other phytoconstituents in a feed that was isolated from Caulerpa racemose. The feed underwent pretreatment through ultrasonic separation and drying before separation in the SMB. Welch Ultimate AQ-C18 served as the stationary phase and 76% EtOH served as the eluent in the SMB columns. Caulerpin accumulated at the extract port while the other phytoconstituents accumulated at the raffinate port due to different affinities to the stationary phase and the eluent. Empirical research was done to determine the impact of three switching periods on the effectiveness of the separation and purification process. The average purity of caulerpin was 91.2, 99.0, and 99.3% for switching times of 3.5, 4.0, and 4.5 min, respectively (Kung et al., 2021). An effective method for producing fucose on a large scale from Undaria pinnatifida was created. The created procedure included a hydrolysis, several decolorization and deionization pretreatment steps, and a final stage of purification employing a well-devised simulated moving bed (SMB) procedure. First, it was determined what the ideal acid concentration and reaction duration were for the hydrolysis of marine algae. The hydrolysate produced by this experiment was then utilized to establish the ideal operating parameters for the pretreatment stage, which included electrodialysis, an activated-carbon treatment, and an ion-exchange processing to achieve decolorization and deionization (Hong et al., 2019).
The extract components subjected to separation were complicated, and no pure compound would be isolated in single-column chromatography. The separation effectiveness of multi-dimensional separation based on solid phase extraction and coupling of numerous columns with different stationary phases is considerably improved. Natural product separation is becoming quicker, more efficient, and automated as more commercial multiple-dimensional separation technology enters the market. Typically, the target chemical was enhanced through first-dimensional separation and purified through last-dimensional separation. Multi-dimensional separation can be accomplished with the same or other types of separation equipment (LC or GC).
As a carbon reservoir, dissolved organic matter (DOM) in freshwater and seawater (624 and 750 gT, respectively) is similar to atmospheric CO2. DOM and CO2 are closely related since CO2 is a major byproduct of DOM mineralization, yet DOM is still largely uncharacterized and contains a variety of classes of chemicals in quantities ranging from picomolar to micromolar. Due to considerable co-elution, traditional liquid and gas chromatographic analyses are hindered by the complexity of DOM. Alternate multi-dimensional chromatographic methods to fractionate and isolate single chemicals from this organic pool to get over the analytical difficulties caused by DOM. First, DOM elements were fractionated according to size and polarity using size exclusion chromatography (SEC) and reversed-phase liquid chromatography-tandem mass spectrometry (RP-LC-MS/MS) (Sandron, 2014).
Nanomaterials (NMs) and nanostructures can be characterized using mass spectrometry (MS), which provides trustworthy multi-dimensional data made up of precise mass, isotopic, and molecular structural information. MS has become a potent method for nano-characterization over the past 10 years. The capabilities of MS in numerous aspects of nano-characterization are thoroughly summarized in this paper, considerably enhancing the toolkit for nano research. MS has distinct characteristics for real-time monitoring and tracking reaction byproducts and intermediates in comparison to other characterization approaches (Huang et al., 2021). High-performance liquid chromatography coupled to inductively coupled mass spectrometry (HPLC-ICP-MS) has been developed as a method for identifying arsenic species in marine samples. The separation of a significant number of arsenicals present in the samples was accomplished using cation exchange HPLC with gradient elution utilizing pyridine format as the mobile phase. Overnight mechanical agitation and a 50% (v/v) methanol-water solution were used to extract the various arsenic species. While the cationic arsenocholine ion and tetramethylarsonium ion were unaffected, the effect of the sample matrix on HPLC retention time for arsenobetaine and dimethylarsinoylacetic acid was dramatic. Low detection limits of 0.002–0.005 μg/g dry mass for the various arsenic species were obtained (Sloth et al., 2003).
Marine algae are regarded as a significant source of bioactive substances, including sterols, β-carotenes, sulfated polysaccharides, and polyunsaturated fatty acids. These bioactive ingredients are highly sensitive to heat, light, and oxygen, and they have a poor stability in storage. Furthermore, a lot of the bioactive components found in microalgae undergo physical or chemical changes after ingestion due to exposure to various gastrointestinal tract conditions. These changes result in various metabolic reactions that can change the functional groups of these components, which can change their biological activities and lead to low bioavailability (Bonilla-Ahumada et al., 2018). Thus, in addition to enhancing the delivery and absorption of these substances, it is crucial to investigate other approaches for maintaining the bioactive components of microalgae.
Microencapsulation and nanoencapsulation offer a workable solution to the stability and bioavailability issues associated with microalgae products. Encapsulation is the process of packing tiny core material particles into wall material to create capsules that protect the bioactive components of microalgae and increase their shelf life while also encouraging a controlled release of the encapsulate.
The first kind of encapsulation is called microencapsulation, and it involves packaging minuscule amounts of liquids, solids, and gaseous materials that range in size from submicron to several millimetres. The most used encapsulation method is spray drying (Nazzaro et al., 2012).
The second type of encapsulation is called nanoencapsulation, and it works on the same principles as microencapsulation. The primary distinction is the size of the particles, which can range in diameter from 1 to 1,000 nm. Because of their small size, nanoparticles have a higher surface area and are more soluble, which increases the absorption of the core ingredient (Pinto Reis et al., 2006).
The microencapsulation of fresh algal biomass of Tetraselmis chuii, a rich source of β-carotenes, has demonstrated the great preservation efficiency of the approach. The findings demonstrated that even after 3 months of storage in the dark at 25°C, Tetraselmis chuii had kept 80%–92% of β-carotenes and 46%–81% of phenolic compounds in the microencapsulated specimen. Consequently, microencapsulation may be a more effective method of microalgae product preservation (Bonilla-Ahumada et al., 2018).
Using nanoencapsulation to increase the bioavailability of fucoxanthin from Phaeodactylum tricornutum is another way to increase the bioavailability of microalgae bioactive components. The study created two different kinds of nanoparticles: one type used chitosan, while the other type used casein and alginate. Controlled release of fucoxanthin was seen in both varieties of nanoparticles during enhanced gastrointestinal digestion. Furthermore, after oral administration, the pharmacokinetic behaviour of fucoxanthin demonstrated a 31.8% increase in its absorption in mouse plasma (Koo et al., 2023).
Various constituents of macroalgae or marine algae were successfully isolated, including pigments, polysaccharides, fatty acids, and polyphenolics. In the following subsections, each component shall be discussed and summarized in different tables, Supplementary Tables S1–S4, regarding its types, botanical origin, method of extraction, and yield for comparative purposes between conventional and modern techniques. Prokaryotic and eukaryotic marine algae have been demonstrated in earlier research to be capable of creating a wide range of useful compounds, which can be recovered in biorefineries and used in cleaner industrial processes (Cheng et al., 2022). In addition to being highly nutritious, marine algae are also a rich source of numerous biologically active compounds. As a result, they hold enormous promise for the creation of nutritious meals and medications. Polysaccharides, proteins, terpenes, pigments, sterols, polyphenols, γ-linolenic acid, superoxide dismutase, cyclic polysulfides, and macrolides are among the biologically active components found in marine algae.
One class of potentially useful natural pigments is called carotenoids. They are composed of eight units, each containing five carbons with alternating single and double bonds. The carotenoid chain can form with either an oxygen functional group (astaxanthin) or a cyclic group (β-carotene) depending on the kind of metabolite (Murthy et al., 2005). Among the more than 400 carotenoids, astaxanthin, β-carotene, zeaxanthin, lutein, and so forth have been employed in industrial manufacturing (Berman et al., 2015). Dunaliella salina is a single-celled algae that grows and reproduces quickly, and it has a high rate of light energy conversion. When given the right conditions, this type of algae may produce huge amounts of β-carotene, which is around 500 times more than what carrots do (Boonyaratpalin et al., 2001). A naturally occurring carotenoid, fucoxanthin is extensively found in the cells of diatoms, brown algae, crypto algae, and dinoflagellates. Its allene bond and 5,6-monocyclic oxidation bond define it. Several positive health advantages of fucoxanthin and its derivatives, include impacts on weight loss, anti-inflammatory, anti-cancer, and hypertensive conditions (Xia et al., 2013). When compared to other microorganisms, Haematococcus pluvialis is one of the most plentiful sources of natural astaxanthin. As a result, it's critical to comprehend the entire astaxanthin biorefinery process, from assaying to processing as it moves downstream, for H. pluvialis. Recent advanced biorefinery techniques such as supercritical CO2 extraction, magnetic-assisted extraction, ionic liquids extraction, and supramolecular solvent extraction as well as conventional biorefinery methods such as mechanical disruption, solvent extraction, direct extraction using vegetable oils, and enhanced solvent extraction were used (Khoo et al., 2019). Supplementary Table S1 summarizes the pigments extracted from marine algae using conventional and advanced extraction.
Microalgae PUFA, which is essential for human nutrition and wellbeing, is seen to be a feasible replacement for PUFA obtained from marine life. For long-term production, novel techniques can be applied to produce a sizable amount of polyunsaturated fatty acids from the biomass of microalgae species. A photobioreactor combined with lighting, such as blue or red light bulbs, can also promote the synthesis of eicosapentaenoic acid. Supercritical fluid extraction was found to be the most efficient method for obtaining polyunsaturated fatty acids from the biomass of microalgae species, including docosahexaenoic acid and eicosapentaenoic acid. Treatments based on plant hormones significantly enhance the amount of lipid molecules. For example, in Chlorella sp., the indole acetic acid dramatically increases the release of omega-3 fatty acids. This process allows for increased feedstock and omega fatty acid levels by inducing oxidative stress in Chlorella sp. and stimulating desaturase enzymes (Sivaramakrishnan and Incharoensakdi, 2020).
Given its numerous nutritional benefits, protein is regarded as an essential component of the microalgae biomass biorefinery. The components of the algae cell wall are cellulose, β-glucans, hemicellulose, and polysaccharides. Selecting the right cell disruption approach depends on the microalgae cellular composition. Bead mills and high-pressure homogenizers are examples of mechanical cell damage. Chemical agents, such as NaOH, enzymes, and physicochemical procedures, are examples of non-mechanical cell disturbance methods (Hu et al., 2019). Depending on how quickly they dissolve in water after a disruption, algae proteins are separated from both solid phase and cellular debris before being concentrated. Ammonium sulfate precipitation and compounds that precipitate proteins can both be utilized (Raina et al., 2022). Affinity chromatography, ion exchange, hydrophobic interaction, and molecular separation are needed to purify proteins to maximize their commercial value. Maintaining a solvent system that guarantees the right pH, ionic strength, and salt type is necessary. High protein output was achieved by the researchers using a super-critical CO2 protein extraction approach (Obeid et al., 2018).
Polysaccharides are abundant in marine algae and come from a variety of sources. They are essential for preserving the physiological ecology of marine algae in addition to enhancing the body immune system (Zheng L.-X. et al., 2020). The two main extraction processes used in biorefineries are the traditional alkaline extraction technique and the acid and alkaline extraction process. Bull kelp Durvillaea potatorum was extracted using a two-step selective biorefinery process. The first step was an acidic extraction step that targeted the extraction of fucoidan/laminarin (F/L) fraction and acid-extractable alginate (acid-A1). The second step was an alkaline extraction step that targeted the extraction of alkaline extractable alginate (alkaline-A2). The purpose of the subsequent purification and refining processes is to further purify the final products. The first acidic extraction stage in the biorefinery process is crucial and unique. Three important process parameters—acid concentration (HCl), temperature, and time are optimized for this step (Abraham et al., 2019). Alginates are mostly composed of α-L-guluronic acid (G) and β-D-mannuronic acid (M). They are often taken out of Ecklonia maxima, Laminaria species, M. pyrifera, Ascophyllum nodosum, and Durvillea antarctica for commercial use. Large amounts of alginate are mostly stored in the cell walls of macroalgae. The molecular weight of alginates ranges from 500 to 1,000 kDa. Alginate molecular weight and M/G ratio are crucial in determining its chemical and physical characteristics. Higher viscosity and strong antioxidant activity have been shown in alginates containing more G blocks than M units (Abraham et al., 2019). Supplementary Table S2 listed the different types of polysaccharides extracted from marine algae using conventional and advanced extraction techniques.
Although lipids make up only a minor portion of macroalgae typically 1%–5% of the dry weight their fatty acids are thought to be superior to those found in terrestrial plants (Filote et al., 2021b). Most marine microalgae have fatty acid contents that are more than 10% of their dry weight (Wang, 2002). Numerous marine algae species are abundant in polyunsaturated fatty acids (PUFA), with eicosapentaenoic acid (EPA) and DHA being the two most significant long-chain PUFA in the ω-3 family (Plaza et al., 2010). Certain ω-3 and ω-6 fatty acids are necessary for humans and certain animals, but they may also be linked to specific illnesses (Martins et al., 2013). Algae lipid productivity can be increased by different technical techniques and appropriate environmental conditions. Microalgae lipids can be extracted via solvent extraction, ultrasonic extraction, microwave-assisted extractions, and electroporation. Lipid extraction’s primary drawbacks are that it uses a lot of energy, requires different kinds of solvents depending on the kind of lipids present in the microalgae species, raises operating temperatures, and increases the danger of combustibility. Recently, super-critical extraction techniques have been considered as an alternative to harmful organic solvents. The main advantage of this process is its high selectivity toward triglycerides, which are essential chemicals in the synthesis of biodiesel (Vasistha et al., 2021). Supplementary Table S3 listed the important lipids extracted from marine algae using conventional and advanced extraction techniques.
Concern over algae-derived polyphenols has grown significantly as a result of calls to substitute synthetic and hazardous substances used in the food manufacturing business, such as butylated hydroxytoluene (BHT) and butylated hydroxy anisole (BHA). Additionally, it has been shown that brown macroalgae polyphenolic extracts have bacterial growth inhibitory properties comparable to those of additives like sodium benzoate and sodium nitrite. Removal of polyphenols is required to produce bioethanol and biogas since they hinder the fermentation processes. Formaldehyde is frequently utilized in the alginate industry for the extraction of macroalgae before alginate isolation. Nevertheless, due to its carcinogenic properties, there is a necessity for more eco-friendly alternatives. The extraction of polyphenols from macroalgae is essential within the context of an integrated biorefinery. The separation of these compounds is a critical step in the production of refined extracts and the enhancement of the overall chemical conversion of biomass. Carefully selecting the pre-treatment process parameters is necessary to maximize the yield of polyphenolic compounds from macroalgae, since it can lower the possible consequence. One such method that helps to lower the amount of polyphenolic content and its antioxidant properties is oven-drying. However, when compared to freeze- and microwave-dried biomass, the extraction yield from oven-dried biomass appears to be higher (Filote et al., 2021b). Supplementary Table S4 summarizes the important kinds of polyphenols extracted from marine algae using conventional and advanced extraction techniques.
The three main categories of microalgae are a) species having the ability to produce a single high-value product; b) species having great potential for biorefinery techniques; and c) novel species showing promise for biomass composition and by-products. Microalgae strong areal biomass production contributes to the accumulation of economically significant products that account for more than 50% of their dry weight High-value products, such as astaxanthin from H. pluvialis and β-carotene from D. salina, are effectively commercialized at a significant scale. Additionally, phycocyanin, an extract from cyanobacteria has recently earned a significant amount of market share, and biomass from Spirulina and Chlorella is sold in considerable quantities as nutraceuticals. Novel, promising strains of algae are still being reported, and more strains with industrial potential have been identified for the synthesis of biofuels or high-value compounds like carotenoids and PUFAs (Leu and Boussiba, 2014). However, for both high-value goods and bulk biomass, phototrophic algae product production is thought to be two to five times more costly than alternative approaches. Deciphering the genomes and transcriptomes of several high-value algae species and their metabolic pathways toward carotenoid, lipid, and PUFA biosynthesis has allowed. Using algae reduces the cost, length, and conversion process because lignin separation appears to be a rate-limiting step for other biomasses (Gonzalez-Fernandez et al., 2015). The most elevated level of vitamins and trace elements is discovered within microalgae. Constraints exist for the scientific teams investigating the chemical properties, and assurance of feed quality is imperative. The production of vitamin D3 from Nannochloropsis oceanica could reach 1 μg/g of dried cell mass, with a notable enhancement in vitamin output due to exposure to UVB stressors. the impact of UVB radiation on D. salina and N. oceanica. When exposed to UVB light, most of the cultures (D. salina (145 ± 11 ng/g DM), N. oceanica (285 ± 5 ng/g DM), and N. limnetica (2,700 ± 198 ng/g DM)) were able to synthesize vitamin D3, whereas T. suecica was not able to do so. The study revealed that the wet paste and dry biomass of N. oceanica exposed to UVB had reduced levels of 7-dehydrocholesterol and vitamin D3, measuring 13 ± 0.4 ng/g DM and 2 ± 0.2 ng/g DM, respectively. These findings imply that the growing diluted cultures are producing more 7-dehydrocholesterol during UVB exposure. UVB induced the accumulation of D. salina α-tocopherol and β-carotene, and N. oceanica α-tocopherol (Ljubic et al., 2021). Chlorella sp. exhibited a superior concentration of vitamin B12 compared to both Spirulina and N. gaditana sp., alongside possessing a greater quantity of folate.
Algae are quickly expanding photosynthesizing organisms that can produce useful biomass from roughly 10% of sunshine, or about 77 g/biomass/m2/day, or about 280 tons/ha/year (Masoud et al., 2021). Because most microalgae have a high lipid content (50%–80% of biomass), they are good candidates for biodiesel synthesis. The 58,700 L/hac microalgae oil that microalgae can produce can be transformed into 121,104 L/hac biodiesels (Khan et al., 2018). One of the most promising sustainable biofuels is biogas, given the growing awareness of issues like greenhouse gas emissions and the depletion of fossil fuels. The use of microalgae as a biodiesel source is growing in popularity. Because there is a limited supply of surplus fertilizer in the world, recycling fertilizer nutrients is essential to maintaining large-scale biodiesel production. The lipid fraction separation did not considerably reduce the energy yield (which amounted to 9% of algae dry weight). Hydrolytic enzymes were able to access algae biopolymers more easily through sonication, partially offsetting the energy lost during lipid extraction (Ayala-Parra et al., 2017). To produce bioethanol, starch-enriched microalgae biomass has been studied. Different pre-treatment methods have been used to release simple sugars to increase the yield of bioethanol. Some of the most seen algae in freshwater and saltwater environments are Chlamydomonas sp., Chlorella sp., Dunaliella sp., Gracilariaceae sp., Nannochloropsis sp., Oscillatoria sp., Scenedesmus sp., and Spirulina sp (Deviram et al., 2020). There are many benefits to using algae as a substrate to produce biofuels, such as a quicker harvest period, lower farming expenses, reduced CO2 emissions, a higher process efficiency, a faster rate of algae feedstock growth, and significantly less water usage than traditional plant biomasses. Four processes are involved in the breakdown of microalgae biomass into bioethanol: pretreatment, saccharification, fermentation, and downstream processing. Because it doesn't contain lignin and doesn't need to be separated from other lignocellulosic materials like rice and wheat straw, algae biomass is a desirable raw material (Masoud et al., 2021). Through cell lysis, the glycogen from algae biomass can be recovered using an acidic, enzymatic, or solvent extraction technique. To convert the polymer complex into monomer form, which may then be subjected to fermentation to make bioethanol, pre-treatment of the extracted carbohydrates is necessary. A few carbohydrate-enriched microalgae, such as Chlamydomonas reinhardtii and C. vulgaris are thought to have potential for biofuel (bioethanol) production. More suitable for the production of butanol are microalgae biomass, such as Clostridium acetobutylicum, Saccharina sp., C. saccharobutylicum, Chlorella sorokiniana, C. tetanomorphum, Nannochloropsis sp., C. aurantibutyricum, Arthrospira platensis, and U. lactuca (Eloka-Eboka et al., 2021). A few challenges exist in the large-scale production and commercialization of such clean biofuels, notwithstanding the remarkable contribution of bioethanol generated from microalgae to the development of renewable fuels (Khan et al., 2018).
Bioplastics are degradable materials with applications comparable to plastics, yet plastics are not biodegradable and toxic to humans, animals, or the environment. Because of their enormous biomass, marine algae is a more efficient source of bioplastics than plants, animals, or other sources—research on this topic is ongoing. It is stronger, less brittle, and more microwave-resistant (Thiruchelvi et al., 2021). Algae are self-feeding entities that exist in either a single-celled or multi-celled form. Bioplastics are derived from microalgae, such as the challenging-to-collect remnants of spirulina. Macroalgae, for instance, marine algae, exhibit more potential than the aforementioned sources due to their abundant biomass, cost-effectiveness, suitability for cultivation in natural environments, capacity to thrive in various conditions for growth, and ability for year-round harvesting. Despite not yet being utilized within the plastic industry. They generate cost-effective, superior-quality bioplastics that are free from harmful substances and possess similar chemical resistance and tensile strength (Thiruchelvi et al., 2021). Marine algae polysaccharide content is what allows them to produce bioplastics. Among the marine algae polysaccharides are alginate, carrageenan, agar, and Floridian starch. Marine algae is collected methodically, dried fast, and then baked to preserve its freshness and quality. The dissolved polysaccharide combination is first filtered to remove any remaining smaller particles, then centrifuged to remove any dense, larger cellulose particles. Lastly, the solution is concentrated (Stevens, 2002). It was possible to fabricate bioplastic film by employing Halimeda opuntia. It was possible to successfully create a thin bioplastic film with improved mechanical and physical properties by adjusting the ratio of polyvinyl alcohol (PVA) to marine algae biomass (El-Sheekh et al., 2024). Cordium fragile, and Macrocystis integrifolia-derived bioplastics are stronger, more resilient to microwave radiation, and less brittle. The marine algae goes through a lot of processes (Thiruchelvi et al., 2021).
Spirulina has long been utilized as food and feed. Because they are abundant in proteins, vitamins, minerals, antioxidants, carotenoids, and other nutrients, they can be used to create a variety of products with additional value. Long ago, microalgae may have been a source of superfood for humans (Chandra et al., 2019). For many years, researchers have looked at food waste and microalgae as possible sources of molecules that may be used in a variety of industries, including packaging, textiles, and pharmaceuticals. Despite several study attempts to extract rich components (such as tocopherols, tocotrienols, and phenolic compounds) from these sources, they continue to be disregarded as two important sources of bioactive chemicals and pigments, primarily because of ineffective extraction methods (Martins et al., 2023). The unique natural bioactive biomolecules as vitamins (thiamine, riboflavin, α-tocopherol), proteins (C-phycocyanin, A-phycocyanin), pigments (b-carotene, chlorophyll, etc.), and fatty acids (palmitic acid, linoleic acid, oleic acid, stearic acid, and c-linolenic acid, etc.) source to replace the chemical-based compounds have recently sparked increased interest in microalgae and cyanobacteria (Cuellar-Bermudez et al., 2017). Since each component must be removed from the algae biomass to be used, a key prerequisite for algae or cyanobacterial bioactive chemicals with practical applications is the efficient use of the extraction process (Chandra et al., 2019).
Numerous chemicals derived from algae, including peptides, carotenoids, steroids, fatty acids, polysaccharides, and halogenated compounds, possess a wide range of biological characteristics, including antibacterial and anticancer activities (Rani et al., 2021). These bioactive compounds attach to a variety of locations and, by activating cellular pathways, reduce cell proliferation or induce death (Martins et al., 2023). Since the fatty acids found in oily microalgae extracts contain antimicrobial, antiviral, antibacterial, and antifungal qualities, microalgae have also demonstrated good medicinal capabilities. These features have led to their widespread use as components in formulations for sun protection, skincare, and hair care. It demonstrated how to extract total lipids and bio-oil from algae in an efficient and environmentally friendly way. With an EtOH/CPME (8:2) solution, the highest total algae lipid production (39.4% on a dry basis) was achieved at 80°C and 60 min of reaction time. This extraction condition helps obtain oil that is rich in SFA and can be used to produce biofuel. Using an EtOH/CPME, 6:4, at the same temperature and reaction period, an oily extract rich in bio-compounds that support human health, such as docosahexaenoic acid, DHA (C22: 6, ω-3) and eicosapentaenoic acid, EPA (C20: 5, ω-3) was produced. When using SC-CO2, the oil extraction yield is 30.0%, however, under these extraction circumstances it is 32.8%. Compared to traditional extraction technologies, this approach has several benefits, including higher total lipid yield, more selectivity, and maintained thermo-labile chemicals. It is a different approach to producing algae biofuel sustainably and creating valuable byproducts (Santoro et al., 2019).
Marine algae are recognized to support a circular bioeconomy in several industries, such as bioenergy, food, feed, fertilizer, building, and cosmetics. Making better use of marine algae resources is extremely significant because of their benefits of strong adaptability and high yield, which are also considered to be the most important sources of many biologically active chemicals. One of the primary components of Marine algae products (MAPs), polysaccharides for instance, exhibits tremendous biological activity. MAPs have the potential to be used in a variety of medicinal and functional food items. The current study of MAPs does, however, still have certain flaws. Although cutting-edge extraction methods like microwave-, ultrasound-, and enzyme-assisted extraction methods demonstrated relatively high extraction efficiencies and additionally benefit from ease of use, low costs, and environmental friendliness, their use is currently restricted to laboratory research for MAPs. Aside from the benefits already highlighted, the possibility of scaling up these procedures adds interest from an industrial standpoint. However, the effectiveness of extraction varies depending on the species, target phytochemicals, and operating conditions. Moreover, chromatography have been emergeed as the predominant technique employed for the purification of natural substances from complex mixtures. Future work in marine algae, particularly, with regard to the new goals of UN and circular blue bioeconomy are promising for vast kinds of products, including biofuels and bioactive metabolites at industrial scale.
AZ: Writing–original draft, Resources. HM: Investigation, Writing–original draft. EA: Writing–original draft, Supervision. MI: Formal Analysis, Writing–original draft. UA: Supervision, Writing–review and editing.
The author(s) declare that no financial support was received for the research, authorship, and/or publication of this article.
We thank Deraya University, Minia University, and Tanta University for supporting this work.
The authors declare that the research was conducted in the absence of any commercial or financial relationships that could be construed as a potential conflict of interest.
The author(s) declared that they were an editorial board member of Frontiers, at the time of submission. This had no impact on the peer review process and the final decision.
All claims expressed in this article are solely those of the authors and do not necessarily represent those of their affiliated organizations, or those of the publisher, the editors and the reviewers. Any product that may be evaluated in this article, or claim that may be made by its manufacturer, is not guaranteed or endorsed by the publisher.
The Supplementary Material for this article can be found online at: https://www.frontiersin.org/articles/10.3389/fntpr.2024.1425242/full#supplementary-material
Abdelmohsen, U. R., Sayed, A. M., and Elmaidomy, A. H. (2022). Natural products’ extraction and isolation-between conventional and modern techniques. Front. Nat. Prod. 1. doi:10.3389/fntpr.2022.873808
Abdel-Rahman, I. a.M., Attia, E. Z., Aly, O. M., Saber, H., Rushdi, M. I., and Abdelmohsen, U. R. (2022a). Metabolite profiling of green algae Halimeda opuntia to target hepatitis C virus-796 polymerase inhibitors assisted by molecular docking. S. Afr. J. Bot. 151, 538–543. doi:10.1016/j.sajb.2022.10.038
Abdel-Rahman, I. a.M., Raslan, A., Dawy, A., Wail, A., Ahmed, F., Hany, K., et al. (2022b). Cymbopogon schoenanthus (L.) Spreng: a comprehensive review on phytochemical and pharmacological biodiversity. Trends Phytochem. Res. 6, 259–281. doi:10.30495/tpr.2022.1966529.1276
Aboubakr, E., Rushdi, M. I., and Babekir, E. Y. (2024). Improving Captopril cardioprotective activities against Doxorubicin induced cardiotoxicity by co-administration with allicin. SVU Int. J. Med. Sci. 7, 49–61. doi:10.21608/svuijm.2023.248450.1735
Abraham, R. E., Su, P., Puri, M., Raston, C. L., and Zhang, W. (2019). Optimisation of biorefinery production of alginate, fucoidan and laminarin from brown seaweed Durvillaea potatorum. Algal Res. 38, 101389. doi:10.1016/j.algal.2018.101389
Abreu, M. H., Varela, D. A., Henríquez, L., Villarroel, A., Yarish, C., Sousa-Pinto, I., et al. (2009). Traditional vs. Integrated multi-trophic aquaculture of Gracilaria chilensis, C. J. Bird, J. McLachlan and E. C. Oliveira: productivity and physiological performance. Aquaculture 293, 211–220. doi:10.1016/j.aquaculture.2009.03.043
Alvarado-Morales, M., Gunnarsson, I. B., Fotidis, I. A., Vasilakou, E., Lyberatos, G., and Angelidaki, I. (2015). Laminaria digitata as a potential carbon source for succinic acid and bioenergy production in a biorefinery perspective. Algal Res. 9, 126–132. doi:10.1016/j.algal.2015.03.008
Álvarez-Viñas, M., Flórez-Fernández, N., Torres, M. D., and Domínguez, H. (2019). Successful approaches for a red seaweed biorefinery. Mar. Drugs 17, 620. doi:10.3390/md17110620
Aravind, S., Kumar, P. S., Kumar, N. S., and Siddarth, N. (2020). Conversion of green algal biomass into bioenergy by pyrolysis. A review. Environ. Chem. Lett. 18, 829–849. doi:10.1007/s10311-020-00990-2
Attia, E. Z., Abdel-Rahman, I. a.M., Aly, O. M., Saber, H., Rushdi, M. I., and Abdelmohsen, U. R. (2024). In vitro cytotoxic potential of ethanol extract of dictyopteris acrostichoides against human cancer cells. Rev. Bras. Farmacogn. 34, 212–216. doi:10.1007/s43450-023-00474-8
Attia, E. Z., Youssef, N. H., Saber, H., Rushdi, M. I., Abdel-Rahman, I. a.M., Darwish, A. G., et al. (2022). Halimeda opuntia and Padina pavonica extracts improve growth and metabolic activities in maize under soil-saline conditions. Appl. Phycol. 34, 3189–3203. doi:10.1007/s10811-022-02844-6
Ayala-Parra, P., Liu, Y., Field, J. A., and Sierra-Alvarez, R. (2017). Nutrient recovery and biogas generation from the anaerobic digestion of waste biomass from algal biofuel production. Renew. Energy 108, 410–416. doi:10.1016/j.renene.2017.02.085
Baghel, R. S. (2023). Developments in seaweed biorefinery research: a comprehensive review. Chem. Eng. J. 454, 140177. doi:10.1016/j.cej.2022.140177
Bare, W. R., Jones, N. B., and Middlebrooks, E. J. (1975). Algae removal using dissolved air flotation. J. Water Pollut. Control Fed. 47, 153–169.
Benedetti, G., Zabini, F., Tagliavento, L., Meneguzzo, F., Calderone, V., and Testai, L. (2023). An overview of the health benefits, extraction methods and improving the properties of pomegranate. Antioxidants 12, 1351. doi:10.3390/antiox12071351
Berman, J., Zorrilla-López, U., Farré, G., Zhu, C., Sandmann, G., Twyman, R. M., et al. (2015). Nutritionally important carotenoids as consumer products. Phytochem. Rev. 14, 727–743. doi:10.1007/s11101-014-9373-1
Bitou, N., Ninomiya, M., Tsujita, T., and Okuda, H. (1999). Screening of lipase inhibitors from marine algae. Lipids 34, 441–445. doi:10.1007/s11745-999-0383-7
Biz, A. P., Cardozo-Filho, L., and Zanoelo, E. F. (2019). Drying dynamics of microalgae (Chlorella pyrenoidosa) dispersion droplets. Chem. Eng. Process. Process Intensif. 138, 41–48. doi:10.1016/j.cep.2019.03.007
Bonilla-Ahumada, F. D. J., Khandual, S., and Lugo-Cervantes, E. D. C. (2018). Microencapsulation of algal biomass (Tetraselmis chuii) by spray-drying using different encapsulation materials for better preservation of beta-carotene and antioxidant compounds. Algal Res. 36, 229–238. doi:10.1016/j.algal.2018.10.006
Boonyaratpalin, M., Thongrod, S., Supamattaya, K., Britton, G., and Schlipalius, L. E. (2001). Effects of β-carotene source,Dunaliella salina, and astaxanthin on pigmentation, growth, survival and health ofPenaeus monodon: coloration ofPenaeus monodon. Dunaliella salina, astaxanthin pigmentation, growth, Surviv. health Penaeus monodon 32, 182–190. doi:10.1046/j.1355-557x.2001.00039.x
Brink, J., and Marx, S. (2013). Harvesting of Hartbeespoort Dam micro-algal biomass through sand filtration and solar drying. Fuel 106, 67–71. doi:10.1016/j.fuel.2012.10.034
Carreira-Casais, A., Otero, P., Garcia-Perez, P., Garcia-Oliveira, P., Pereira, A. G., Carpena, M., et al. (2021). Benefits and drawbacks of ultrasound-assisted extraction for the recovery of bioactive compounds from marine algae. Int. J. Env. Res. Pub He 18, 9153. doi:10.3390/ijerph18179153
Castejón, N., Thorarinsdottir, K. A., Einarsdóttir, R., Kristbergsson, K., and Marteinsdóttir, G. (2021). Exploring the potential of Icelandic seaweeds extracts produced by aqueous pulsed electric fields-assisted extraction for cosmetic applications. Mar. Drugs 19, 662. doi:10.3390/md19120662
Catchpole, O., Moreno, T., Montañes, F., and Tallon, S. (2018). Perspectives on processing of high value lipids using supercritical fluids. J. Supercrit. Fluids 134, 260–268. doi:10.1016/j.supflu.2017.12.001
Catchpole, O., Ryan, J., Zhu, Y., Fenton, K., Grey, J., Vyssotski, M., et al. (2010). Extraction of lipids from fermentation biomass using near-critical dimethylether. J. Supercrit. Fluids 53, 34–41. doi:10.1016/j.supflu.2010.02.014
Chandra, R., Iqbal, H. M. N., Vishal, G., Lee, H.-S., and Nagra, S. (2019). Algal biorefinery: a sustainable approach to valorize algal-based biomass towards multiple product recovery. Bioresour. Technol. 278, 346–359. doi:10.1016/j.biortech.2019.01.104
Chandrasekaran, S., Ramanathan, S., and Basak, T. (2013). Microwave food processing-A review. Food Res. Int. 52, 243–261. doi:10.1016/j.foodres.2013.02.033
Chatsungnoen, T., and Chisti, Y. (2019). “Flocculation and electroflocculation for algal biomass recovery,” in Biofuels from algae (Elsevier), 257–286. doi:10.1016/B978-0-444-64192-2.00011-1
Chemat, F., Rombaut, N., Sicaire, A.-G., Meullemiestre, A., Fabiano-Tixier, A.-S., and Abert-Vian, M. (2017). Ultrasound assisted extraction of food and natural products. Mechanisms, techniques, combinations, protocols and applications. A review. Ultrason. Sonochem. 34, 540–560. doi:10.1016/j.ultsonch.2016.06.035
Chen, C.-L., Chang, J.-S., and Lee, D.-J. (2015). Dewatering and drying methods for microalgae. Dry. Technol. 33, 443–454. doi:10.1080/07373937.2014.997881
Chen, C.-Y., Yeh, K.-L., Aisyah, R., Lee, D.-J., and Chang, J.-S. (2011). Cultivation, photobioreactor design and harvesting of microalgae for biodiesel production: a critical review. Bioresour. Technol. 102, 71–81. doi:10.1016/j.biortech.2010.06.159
Cheng, P., Li, Y., Wang, C., Guo, J., Zhou, C., Zhang, R., et al. (2022). Integrated marine microalgae biorefineries for improved bioactive compounds: a review. Sci. Total Environ. 817, 152895. doi:10.1016/j.scitotenv.2021.152895
Cuellar-Bermudez, S. P., Aleman-Nava, G. S., Chandra, R., Garcia-Perez, J. S., Contreras-Angulo, J. R., Markou, G., et al. (2017). Nutrients utilization and contaminants removal. A review of two approaches of algae and cyanobacteria in wastewater. Algal Res. 24, 438–449. doi:10.1016/j.algal.2016.08.018
De Boer, J., and Law, R. J. (2003). Developments in the use of chromatographic techniques in marine laboratories for the determination of halogenated contaminants and polycyclic aromatic hydrocarbons. J. Chromatogr. A 1000, 223–251. doi:10.1016/S0021-9673(03)00309-1
De Farias Neves, F., Demarco, M., and Tribuzi, G. (2019). “Drying and quality of microalgal powders for human alimentation,” in Microalgae-from physiology to application (London, United Kingdom: IntechOpen). doi:10.5772/intechopen.89324
Delrue, F., Setier, P.-A., Sahut, C., Cournac, L., Roubaud, A., Peltier, G., et al. (2012). An economic, sustainability, and energetic model of biodiesel production from microalgae. Bioresour. Technol. 111, 191–200. doi:10.1016/j.biortech.2012.02.020
Desmorieux, H., and Hernandez, F. (2004). “Biochemical and physical criteria of Spirulina after different drying processes,” in Proceedings of the 14th international drying symposium (IDS), 900–907.
De Souza, L. M., Sassaki, G. L., Romanos, M. T. V., and Barreto-Bergter, E. (2012). Structural characterization and anti-HSV-1 and HSV-2 activity of glycolipids from the marine algae Osmundaria obtusiloba isolated from southeastern Brazilian coast. Mar. Drugs 10, 918–931. doi:10.3390/md10040918
Deviram, G., Mathimani, T., Anto, S., Ahamed, T. S., Ananth, D. A., and Pugazhendhi, A. (2020). Applications of microalgal and cyanobacterial biomass on a way to safe, cleaner and a sustainable environment. J. Clean. Prod. 253, 119770. doi:10.1016/j.jclepro.2019.119770
Dixon, C., and Wilken, L. R. (2018). Green microalgae biomolecule separations and recovery. Bioresour. bioprocess. 5, 14–24. doi:10.1186/s40643-018-0199-3
Eloka-Eboka, A. C., Maroa, S., and Behera, S. (2021). “16 - algal biofuels—technologies, scope, opportunities, challenges, and applications,” in Sustainable biofuels. Editor R. C. Ray (Academic Press), 449–470. doi:10.1016/B978-0-12-820297-5.00016-5
El-Shabasy, R. M., F. Eissa, T., Emam, Y., Zayed, A., Fayek, N., and Farag, M. A. (2024). Valorization potential of Egyptian mango kernel waste product as analyzed via GC/MS metabolites profiling from different cultivars and geographical origins. Sci. Rep. 14, 2886. doi:10.1038/s41598-024-53379-4
El-Shamy, S., and Farag, M. A. (2021). Novel trends in extraction and optimization methods of bioactives recovery from pomegranate fruit biowastes: valorization purposes for industrial applications. Food Chem. 365, 130465. doi:10.1016/j.foodchem.2021.130465
El-Sheekh, M. M., Alwaleed, E. A., Ibrahim, A., and Saber, H. (2024). Preparation and characterization of bioplastic film from the green seaweed Halimeda opuntia. Int. J. Biol. Macromol. 259, 129307. doi:10.1016/j.ijbiomac.2024.129307
Fasaei, F., Bitter, J., Slegers, P., and Van Boxtel, A. (2018). Techno-economic evaluation of microalgae harvesting and dewatering systems. Algal Res. 31, 347–362. doi:10.1016/j.algal.2017.11.038
Fawzy, M. A., Gomaa, M., Hifney, A. F., and Abdel-Gawad, K. M. (2017). Optimization of alginate alkaline extraction technology from Sargassum latifolium and its potential antioxidant and emulsifying properties. Carbohydr. Polym. 157, 1903–1912. doi:10.1016/j.carbpol.2016.11.077
Filote, C., Santos, S. C. R., Popa, V. I., Botelho, C. M. S., and Volf, I. (2021a). Biorefinery of marine macroalgae into high-tech bioproducts: a review. Environ. Chem. Lett. 19, 969–1000. doi:10.1007/s10311-020-01124-4
Filote, C., Santos, S. C. R., Popa, V. I., Botelho, C. M. S., and Volf, I. (2021b). Biorefinery of marine macroalgae into high-tech bioproducts: a review. Environ. Chem. Lett. 19, 969–1000. doi:10.1007/s10311-020-01124-4
Firouzi, J., Gohari, A. R., Rustaiyan, A., Larijani, K., and Saeidnia, S. (2013). Composition of the essential oil of Nizamuddinia zanardinii, a brown alga collected from Oman Gulf. J. Essent. Oil-Bear. Plants 16, 689–692. doi:10.1080/0972060X.2013.862072
Flodin, C., and Whitfield, F. B. (2000). Brominated anisoles and cresols in the red alga Polysiphoniasphaerocarpa. Phytochemistry 53, 77–80. doi:10.1016/S0031-9422(99)00429-X
Fornari, T., and Stateva, R. P. (2015). High pressure fluid technology for green food processing. Springer. doi:10.1007/978-3-319-10611-3
Fouda, W. M., Youssef, N. H., Dawy, A., and Rushdi, M. I. (2024). Natural product diversity of buckthorns (rhamnus cathartica L. And rhamnus disperma boiss). ODR 11, 1–12. doi:10.21608/ODR.2024.286154.1040
Fudholi, A., Sopian, K., Othman, M. Y., and Ruslan, M. H. (2014). Energy and exergy analyses of solar drying system of red seaweed. Energy Build. 68, 121–129. doi:10.1016/j.enbuild.2013.07.072
Garcia-Vaquero, M., Rajauria, G., and Tiwari, B. (2020). “Chapter 7 - conventional extraction techniques: solvent extraction,” in Sustainable seaweed technologies. Editors M. D. Torres, S. Kraan, and H. Dominguez (Elsevier), 171–189. doi:10.1016/B978-0-12-817943-7.00006-8
Gomez, L., Tiwari, B., and Garcia-Vaquero, M. (2020). “Chapter 9 - emerging extraction techniques: microwave-assisted extraction,” in Sustainable seaweed technologies. Editors M. D. Torres, S. Kraan, and H. Dominguez (Elsevier), 207–224. doi:10.1016/B978-0-12-817943-7.00008-1
Gonzalez-Fernandez, C., Sialve, B., and Molinuevo-Salces, B. (2015). Anaerobic digestion of microalgal biomass: challenges, opportunities and research needs. Bioresour. Technol. 198, 896–906. doi:10.1016/j.biortech.2015.09.095
Gressler, V., Colepicolo, P., and Pinto, E. (2009). Useful strategies for algal volatile analysis. Curr. Anal. Chem. 5, 271–292. doi:10.2174/157341109788680255
Guiry, M., and Guiry, G. (2024). AlgaeBase. World-wide electronic publication. National University of Ireland. Available at: https://www.algaebase.org/.
Guldhe, A., Singh, B., Rawat, I., Ramluckan, K., and Bux, F. (2014). Efficacy of drying and cell disruption techniques on lipid recovery from microalgae for biodiesel production. Fuel 128, 46–52. doi:10.1016/j.fuel.2014.02.059
He, Z., Zhang, A., Ding, L., Lei, X., Sun, J., and Zhang, L. (2010). Chemical composition of the green alga Codium divaricatum Holmes. Fitoterapia 81, 1125–1128. doi:10.1016/j.fitote.2010.07.011
Heimann, T. (2019). Bioeconomy and SDGs: does the bioeconomy support the achievement of the SDGs? Earth's Future 7, 43–57. doi:10.1029/2018EF001014
Herrero, M., and Ibáñez, E. (2015). Green processes and sustainability: an overview on the extraction of high added-value products from seaweeds and microalgae. J. Supercrit. Fluids 96, 211–216. doi:10.1016/j.supflu.2014.09.006
Herrero, M., Sánchez-Camargo, A. D. P., Cifuentes, A., and Ibáñez, E. (2015). Plants, seaweeds, microalgae and food by-products as natural sources of functional ingredients obtained using pressurized liquid extraction and supercritical fluid extraction. Trac. Trends Anal. Chem. 71, 26–38. doi:10.1016/j.trac.2015.01.018
Hong, S.-B., Choi, J.-H., Chang, Y. K., and Mun, S. (2019). Production of high-purity fucose from the seaweed of Undaria pinnatifida through acid-hydrolysis and simulated-moving bed purification. Sep. Purif. Technol. 213, 133–141. doi:10.1016/j.seppur.2018.12.020
Hu, Y., Gong, M., Feng, S., Xu, C., and Bassi, A. (2019). A review of recent developments of pre-treatment technologies and hydrothermal liquefaction of microalgae for bio-crude oil production. Renew. Sustain. Energy Rev. 101, 476–492. doi:10.1016/j.rser.2018.11.037
Huang, X., Liu, H., Lu, D., Lin, Y., Liu, J., Liu, Q., et al. (2021). Mass spectrometry for multi-dimensional characterization of natural and synthetic materials at the nanoscale. Chem. Soc. Rev. 50, 5243–5280. doi:10.1039/D0CS00714E
Hühnerfuss, H., and Kallenborn, R. (1992). Chromatographic separation of marine organic pollutants. J. Chromatogr. B Biomed. Appl. 580, 191–214. doi:10.1016/0378-4347(92)80535-X
Jeffrey, S. W. (1968). Quantitative thin-layer chromatography of chlorophylls and carotenoids from marine algae. Biochim. Biophys. Acta - Biomembr. Ezoic. 162, 271–285. doi:10.1016/0005-2728(68)90109-6
Jimenez-Lopez, C., Pereira, A. G., Lourenço-Lopes, C., Garcia-Oliveira, P., Cassani, L., Fraga-Corral, M., et al. (2021). Main bioactive phenolic compounds in marine algae and their mechanisms of action supporting potential health benefits. Food Chem. 341, 128262. doi:10.1016/j.foodchem.2020.128262
Kardung, M., Cingiz, K., Costenoble, O., Delahaye, R., Heijman, W., Lovrić, M., et al. (2021). Development of the circular bioeconomy: drivers and indicators. Sustainability 13, 413. doi:10.3390/su13010413
Kempkes, M. A. (2016). Pulsed electric fields for algal extraction and predator control. Handb. electroporation, 1–16. doi:10.1007/978-3-319-26779-1_215-1
Khan, M. I., Shin, J. H., and Kim, J. D. (2018). The promising future of microalgae: current status, challenges, and optimization of a sustainable and renewable industry for biofuels, feed, and other products. Microb. Cell. Fact. 17, 36. doi:10.1186/s12934-018-0879-x
Khoo, K. S., Lee, S. Y., Ooi, C. W., Fu, X., Miao, X., Ling, T. C., et al. (2019). Recent advances in biorefinery of astaxanthin from Haematococcus pluvialis. Bioresour. Technol. 288, 121606. doi:10.1016/j.biortech.2019.121606
Kim, H.-H., Kim, H.-S., Ko, J.-Y., Kim, C.-Y., Lee, J.-H., and Jeon, Y.-J. (2016a). A single-step isolation of useful antioxidant compounds from Ishige okamurae by using centrifugal partition chromatography. Fish. Aquat. Sci. 19, 22. doi:10.1186/s41240-016-0023-y
Kim, J., Um, M., Yang, H., Kim, I., Lee, C., Kim, Y., et al. (2016b). Method development and validation for dieckol in the standardization of phlorotannin preparations. Fish. Aquat. Sci. 19, 3. doi:10.1186/s41240-016-0003-2
Kim, S. M., Shang, Y. F., and Um, B.-H. (2011). A preparative method for isolation of fucoxanthin from Eisenia bicyclis by centrifugal partition chromatography. Phytochem. Anal. 22, 322–329. doi:10.1002/pca.1283
Klejdus, B., Lojková, L., Plaza, M., Šnóblová, M., and Štěrbová, D. (2010). Hyphenated technique for the extraction and determination of isoflavones in algae: ultrasound-assisted supercritical fluid extraction followed by fast chromatography with tandem mass spectrometry. J. Chromatogr. A 1217, 7956–7965. doi:10.1016/j.chroma.2010.07.020
Konan, N. G. F. D. S., Li, M., Shi, S., Liu, X., Tang, Y., Kojo, A., et al. (2022). Simple column chromatography separation procedure for polycyclic aromatic hydrocarbons: controlling factor(s). Arab. J. Geosci. 15, 1350. doi:10.1007/s12517-022-10625-1
Kondeti, R. R., Mulpuri, K. S., and Meruga, B. (2014). Advancements in column chromatography: a review. World J. Pharm. Res., 1375–1383.
Koo, S. Y., Hwang, K. T., Hwang, S., Choi, K. Y., Park, Y. J., Choi, J.-H., et al. (2023). Nanoencapsulation enhances the bioavailability of fucoxanthin in microalga Phaeodactylum tricornutum extract. Food Chem. 403, 134348. doi:10.1016/j.foodchem.2022.134348
Ktari, L., Mdallel, C., Aoun, B., Chebil Ajjabi, L., and Sadok, S. (2021). Fucoxanthin and phenolic contents of six Dictyotales from the Tunisian Coasts with an emphasis for a green extraction using a supercritical CO2 method. Front. Mar. Sci. 8, 647159. doi:10.3389/fmars.2021.647159
Kumar, S., Gupta, R., Kumar, G., Sahoo, D., and Kuhad, R. C. (2013). Bioethanol production from Gracilaria verrucosa, a red alga, in a biorefinery approach. Bioresour. Technol. 135, 150–156. doi:10.1016/j.biortech.2012.10.120
Kung, H.-C., Liang, K.-Y., Mutuku, J. K., Huang, B.-W., and Chang-Chien, G.-P. (2021). Separation and purification of caulerpin from algal Caulerpa racemosa by simulated moving bed chromatography. Food Bioprod. process. 130, 14–22. doi:10.1016/j.fbp.2021.09.002
Kuzhiumparambil, U., Labeeuw, L., Commault, A., Vu, H. P., Nguyen, L. N., Ralph, P. J., et al. (2022). Effects of harvesting on morphological and biochemical characteristics of microalgal biomass harvested by polyacrylamide addition, pH-induced flocculation, and centrifugation. Bioresour. Technol. 359, 127433. doi:10.1016/j.biortech.2022.127433
Lafarga, T. (2020). Cultured microalgae and compounds derived thereof for food applications: strain selection and cultivation, drying, and processing strategies. Food Rev. Int. 36, 559–583. doi:10.1080/87559129.2019.1655572
Lahiri, A., Daniel, S., Kanthapazham, R., Vanaraj, R., Thambidurai, A., and Peter, L. S. (2023). A critical review on food waste management for the production of materials and biofuel. J. Hazard. Mater. Adv. 10, 100266. doi:10.1016/j.hazadv.2023.100266
Larrosa, A., Comitre, A., Vaz, L., and Pinto, L. (2017). Influence of air temperature on physical characteristics and bioactive compounds in vacuum drying of Arthrospira spirulina. J. Food Process Eng. 40, e12359. doi:10.1111/jfpe.12359
Lee, D.-J., Chen, G.-Y., Chang, Y.-R., Mujumdar, A. S., and Chang, J.-S. (2013). Cyclic filtration-cleaning of Chlorella vulgaris using surface-modified hydrophilic polytetrafluoroethylene membrane with polyaluminum chloride as coagulant. Dry. Technol. 31, 207–212. doi:10.1080/07373937.2012.700358
Leu, S., and Boussiba, S. (2014). Advances in the production of high-value products by microalgae. Ind. Biotechnol. 10, 169–183. doi:10.1089/ind.2013.0039
Leyton, A., Vergara-Salinas, J. R., Pérez-Correa, J. R., and Lienqueo, M. E. (2017). Purification of phlorotannins from Macrocystis pyrifera using macroporous resins. Food Chem. 237, 312–319. doi:10.1016/j.foodchem.2017.05.114
Liu, N., Li, X., Zhao, P., Zhang, X., Qiao, O., Huang, L., et al. (2021). A review of chemical constituents and health-promoting effects of citrus peels. Food Chem. 365, 130585. doi:10.1016/j.foodchem.2021.130585
Liu, X., Clarens, A. F., and Colosi, L. M. (2012). Algae biodiesel has potential despite inconclusive results to date. Bioresour. Technol. 104, 803–806. doi:10.1016/j.biortech.2011.10.077
Ljubic, A., Thulesen, E. T., Jacobsen, C., and Jakobsen, J. (2021). UVB exposure stimulates production of vitamin D3 in selected microalgae. Algal Res. 59, 102472. doi:10.1016/j.algal.2021.102472
López, A., Rico, M., Rivero, A., and Suárez De Tangil, M. (2011). The effects of solvents on the phenolic contents and antioxidant activity of Stypocaulon scoparium algae extracts. Food Chem. 125, 1104–1109. doi:10.1016/j.foodchem.2010.09.101
Luque De Castro, M. D., and Priego-Capote, F. (2010). Soxhlet extraction: past and present panacea. J. Chromatogr. A 1217, 2383–2389. doi:10.1016/j.chroma.2009.11.027
Martins, D. A., Custódio, L., Barreira, L., Pereira, H., Ben-Hamadou, R., Varela, J., et al. (2013). Alternative sources of n-3 long-chain polyunsaturated fatty acids in marine microalgae. Microalgae 11, 2259–2281. doi:10.3390/md11072259
Martins, R., Sales, H., Pontes, R., Nunes, J., and Gouveia, I. (2023). Food wastes and microalgae as sources of bioactive compounds and pigments in a modern biorefinery: a review. A Rev. 12, 328. doi:10.3390/antiox12020328
Masoud, A. A., El-Horiny, M. M., Khairy, H. M., and El-Sheekh, M. M. (2021). Phytoplankton dynamics and renewable energy potential induced by the environmental conditions of Lake Burullus, Egypt. Environ. Sci. Pollut. Res. 28, 66043–66071. doi:10.1007/s11356-021-15625-4
Mata, T. M., Martins, A. A., and Caetano, N. S. (2010). Microalgae for biodiesel production and other applications: a review. Renew. Sustain. Energy Rev. 14, 217–232. doi:10.1016/j.rser.2009.07.020
Mee-Ngern, B., Lee, S. J., Choachamnan, J., and Boonsupthip, W. (2014). Penetration of juice into rice through vacuum drying. LWT - Food Sci. Technol. 57, 640–647. doi:10.1016/j.lwt.2014.02.001
Michalak, I., and Chojnacka, K. (2014). Algal extracts: technology and advances. Eng. Life Sci. 14, 581–591. doi:10.1002/elsc.201400139
Mittal, R., Lamdande, A. G., Sharma, R., and Raghavarao, K. (2020). Membrane processing for purification of R-Phycoerythrin from marine macro-alga, Gelidium pusillum and process integration. Sep. Purif. Technol. 252, 117470. doi:10.1016/j.seppur.2020.117470
Montañés, F., and Tallon, S. (2018). Supercritical fluid chromatography as a technique to fractionate high-valued compounds from lipids. Separations 5, 38. doi:10.3390/separations5030038
Murthy, K. N. C., Vanitha, A., Rajesha, J., Swamy, M. M., Sowmya, P. R., and Ravishankar, G. A. (2005). In vivo antioxidant activity of carotenoids from Dunaliella salina — a green microalga. Life Sci. 76, 1381–1390. doi:10.1016/j.lfs.2004.10.015
Nagano, C., Moreno, F., Bloch Jr, C., Prates, M., Calvete, J., Saker-Sampaio, S., et al. (2002). Purification and characterization of a new lectin from the red marine alga Hypnea musciformis. Protein Pept. Lett. 9, 159–165. doi:10.2174/0929866023408931
Nazzaro, F., Orlando, P., Fratianni, F., and Coppola, R. (2012). Microencapsulation in food science and biotechnology. Curr. Opin. Biotechnol. 23, 182–186. doi:10.1016/j.copbio.2011.10.001
Nurra, C., Clavero, E., Salvadó, J., and Torras, C. (2014). Vibrating membrane filtration as improved technology for microalgae dewatering. Bioresour. Technol. 157, 247–253. doi:10.1016/j.biortech.2014.01.115
Obeid, S., Beaufils, N., Camy, S., Takache, H., Ismail, A., and Pontalier, P.-Y. (2018). Supercritical carbon dioxide extraction and fractionation of lipids from freeze-dried microalgae Nannochloropsis oculata and Chlorella vulgaris. Algal Res. 34, 49–56. doi:10.1016/j.algal.2018.07.003
Onofrejová, L., Vašíčková, J., Klejdus, B., Stratil, P., Mišurcová, L., Kráčmar, S., et al. (2010). Bioactive phenols in algae: the application of pressurized-liquid and solid-phase extraction techniques. J. Pharm. Biomed. Anal. 51, 464–470. doi:10.1016/j.jpba.2009.03.027
Orejuela-Escobar, L. M., Landázuri, A. C., and Goodell, B. (2021). Second generation biorefining in Ecuador: circular bioeconomy, zero waste technology, environment and sustainable development: the nexus. J. Bioresour. Bioprod. 6, 83–107. doi:10.1016/j.jobab.2021.01.004
Otero, P., Quintana, S. E., Reglero, G., Fornari, T., and García-Risco, M. R. (2018). Pressurized Liquid Extraction (PLE) as an innovative green technology for the effective enrichment of Galician algae extracts with high quality fatty acids and antimicrobial and antioxidant properties. Mar. Drugs 16, 156. doi:10.3390/md16050156
Pauli, G. A. (2010) The blue economy: 10 years, 100 innovations, 100 million jobs. Paradigm publications.
Perez-Vazquez, A., Carpena, M., Barciela, P., Cassani, L., Simal-Gandara, J., and Prieto, M. A. (2023). Pressurized liquid extraction for the recovery of bioactive compounds from seaweeds for food industry application: a review. Antioxidants 12, 612. doi:10.3390/antiox12030612
Pinto, E., Catalani, L. H., Lopes, N. P., Di Mascio, P., and Colepicolo, P. (2000). Peridinin as the major biological carotenoid quencher of singlet oxygen in marine algae Gonyaulax polyedra. Biochem. Biophys. Res. Commun. 268, 496–500. doi:10.1006/bbrc.2000.2142
Pinto Reis, C., Neufeld, R. J., Ribeiro, A. J., and Veiga, F. (2006). Nanoencapsulation I. Methods for preparation of drug-loaded polymeric nanoparticles. Nanomedicine Nanotechnol. Biol. Med. 2, 8–21. doi:10.1016/j.nano.2005.12.003
Plaza, M., Santoyo, S., Jaime, L., Reina, G. G. -B, Herrero, M., Señoráns, F. J., et al. (2010). Screening for bioactive compounds from algae. Journal of pharmaceutical and biomedical analysis 51, 450–455. doi:10.1016/j.jpba.2009.03.016
Raina, D., Kumar, V., and Saran, S. (2022). A critical review on exploitation of agro-industrial biomass as substrates for the therapeutic microbial enzymes production and implemented protein purification techniques. Chemosphere 294, 133712. doi:10.1016/j.chemosphere.2022.133712
Rani, A., Saini, K. C., Bast, F., Varjani, S., Mehariya, S., Bhatia, S. K., et al. (2021). A review on microbial products and their perspective application as antimicrobial agents. Biomolecules 11, 1860. doi:10.3390/biom11121860
Rodriguez-Jasso, R. M., Mussatto, S. I., Pastrana, L., Aguilar, C. N., and Teixeira, J. A. (2011). Microwave-assisted extraction of sulfated polysaccharides (fucoidan) from brown seaweed. Carbohydr. Polym. 86, 1137–1144. doi:10.1016/j.carbpol.2011.06.006
Roge, A., Firke, S., Kawade, R., Sarje, S., and Vadvalkar, S. (2011). Brief review on: flash chromatography. Int. J. Pharm. Sci. Rev. Res. 2, 1930–1937.
Rushdi, M. I., Abdel-Rahman, I. A., Saber, H., Attia, E. Z., and Abdelmohsen, U. R. (2022a). The natural products and pharmacological biodiversity of brown algae from the genus Dictyopteris. J. Mex. Chem. Soc. 66, 154–172. doi:10.29356/jmcs.v66i1.1639
Rushdi, M. I., Abdel-Rahman, I. a.M., Attia, E. Z., Abdelraheem, W. M., Saber, H., Madkour, H. A., et al. (2020a). A review on the diversity, chemical and pharmacological potential of the green algae genus Caulerpa. S. Afr. J. Bot. 132, 226–241. doi:10.1016/j.sajb.2020.04.031
Rushdi, M. I., Abdel-Rahman, I. a.M., Attia, E. Z., Saber, H., Saber, A. A., Bringmann, G., et al. (2022b). The biodiversity of the genus Dictyota: phytochemical and pharmacological natural products prospectives. Molecules 27, 672. doi:10.3390/molecules27030672
Rushdi, M. I., Abdel-Rahman, I. a.M., Saber, H., Attia, E. Z., Abdelraheem, W. M., Madkour, H. A., et al. (2020b). Pharmacological and natural products diversity of the brown algae genus Sargassum. RSC Adv. 10, 24951–24972. doi:10.1039/D0RA03576A
Rushdi, M. I., Abdel-Rahman, I. a.M., Saber, H., Attia, E. Z., Madkour, H. A., and Abdelmohsen, U. R. (2021). A review on the pharmacological potential of the genus Padina. S. Afr. J. Bot. 141, 37–48. doi:10.1016/j.sajb.2021.04.018
Rushdi, M. I., Saber, H., Abdel-Rahman, I. a.M., Shihata, E., Mahmoud, W., Madkour, H., et al. (2020c). The genus Turbinaria: chemical and pharmacological diversity. Nat. Prod. Res. 35, 4560–4578. doi:10.1080/14786419.2020.1731741
Salem, M. A., Mansour, H. E. A., Mosalam, E. M., El-Shiekh, R. A., Ezzat, S. M., and Zayed, A. (2023). Valorization of by-products derived from onions and potato: extraction optimization, metabolic profile, outstanding bioactivities, and industrial applications. Waste Biomass Valori 14, 1823–1858. doi:10.1007/s12649-022-02027-x
Sampaio, A., Rogers, D., and Barwell, C. (1998a). Isolation and characterization of the lectin from the green marine alga Ulva lactuca L. Bot. Mar. 41. doi:10.1515/botm.1998.41.1-6.427
Sampaio, A. H., Rogers, D. J., and Barwell, C. J. (1998b). A galactose-specific lectin from the red marine alga Ptilota filicina. Phytochemistry 48, 765–769. doi:10.1016/S0031-9422(97)00966-7
Sandron, S. (2014). Multi-dimensional and multi-modal separation of dissolved organic matter. Dublin, Ireland: Dublin City University.
Sanjeewa, K. K. A., Herath, K. H. I. N. M., Kim, Y.-S., Jeon, Y.-J., and Kim, S.-K. (2023). Enzyme-assisted extraction of bioactive compounds from seaweeds and microalgae. Trac. Trends Anal. Chem. 167, 117266. doi:10.1016/j.trac.2023.117266
Santoro, I., Nardi, M., Benincasa, C., Costanzo, P., Giordano, G., Procopio, A., et al. (2019). Sustainable and selective extraction of lipids and bioactive compounds from microalgae. Molecules 24, 4347. doi:10.3390/molecules24234347
Schlotterbeck, J., Kolb, A., and Lämmerhofer, M. (2018). Free fatty acid profiling in marine algae extract by LC-MS/MS and isolation as well as quantification of the ω-3 fatty acid hexadeca-4,7,10,13-tetraenoic acid. J. Sep. Sci. 41, 4286–4295. doi:10.1002/jssc.201800780
Schmidt, L. M., Andersen, L. F., Dieckmann, C., Lamp, A., and Kaltschmitt, M. (2019). “The biorefinery approach,” in Energy from organic materials (biomass): a volume in the encyclopedia of sustainability science and technology. Editor M. Kaltschmitt Second Edition (New York, NY: Springer New York), 1383–1412. doi:10.1007/978-1-4939-7813-7_1050
Shibata, S., Ishihara, C., and Matsumoto, K. (2004). Improved separation method for highly purified lutein from chlorella powder using jet mill and flash column chromatography on silica gel. J. Agric. Food Chem. 52, 6283–6286. doi:10.1021/jf0495901
Show, K.-Y., Lee, D.-J., and Chang, J.-S. (2013). Algal biomass dehydration. Bioresour. Technol. 135, 720–729. doi:10.1016/j.biortech.2012.08.021
Show, K.-Y., Yan, Y.-G., and Lee, D.-J. (2019). “Algal biomass harvesting and drying,” in Biofuels from algae (Elsevier), 135–166. doi:10.1016/B978-0-444-64192-2.00007-X
Simon, D., and Helliwell, S. (1998). Extraction and quantification of chlorophyll a from freshwater green algae. Water Res. 32, 2220–2223. doi:10.1016/S0043-1354(97)00452-1
Singh, D. P., Khattar, J. S., Rajput, A., Chaudhary, R., and Singh, R. (2019). High production of carotenoids by the green microalga Asterarcys quadricellulare PUMCC 5.1. 1 under optimized culture conditions. PloS one 14, e0221930. doi:10.1371/journal.pone.0221930
Sivaramakrishnan, R., and Incharoensakdi, A. (2020). Plant hormone induced enrichment of Chlorella sp. omega-3 fatty acids. Biotechnol. Biofuels. 13, 7. doi:10.1186/s13068-019-1647-9
Sloth, J. J., Larsen, E. H., and Julshamn, K. (2003). Determination of organoarsenic species in marine samples using gradient elution cation exchange HPLC-ICP-MS. Anal. A. T. Spectrom. 18, 452–459. doi:10.1039/B300508A
Sosa-Hernández, J. E., Escobedo-Avellaneda, Z., Iqbal, H. M., and Welti-Chanes, J. (2018). State-of-the-art extraction methodologies for bioactive compounds from algal biome to meet bio-economy challenges and opportunities. Molecules 23, 2953. doi:10.3390/molecules23112953
Stevens, E. S. (2002). Green plastics: an introduction to the new science of biodegradable plastics. Princeton University Press.
Surve, N. S., Thomas, A. B., Bhole, R. P., and Patil, C. Y. (2023). Flash chromatography and semi-preparative HPLC: review on the applications and recent advancements over the last decade. Eurasian J. Chem. 109, 20–30. doi:10.31489/2959-0663/1-23-9
Taylor, L. T. (2010). Supercritical fluid chromatography. Anal. Chem. 82, 4925–4935. doi:10.1021/ac101194x
Thiruchelvi, R., Das, A., and Sikdar, E. (2021). Bioplastics as better alternative to petro plastic. Mater. Today Proc. 37, 1634–1639. doi:10.1016/j.matpr.2020.07.176
Tiwari, B. K. (2015). Ultrasound: a clean, green extraction technology. Trac. Trends Anal. Chem. 71, 100–109. doi:10.1016/j.trac.2015.04.013
Van Dyne, D. L., Blase, M. G., and Clements, L. D. (1999). A strategy for returning agriculture and rural America to long-term full employment using biomass refineries. Alexandria, VA: ASHS Press, 114–123.
Vasistha, S., Khanra, A., Clifford, M., and Rai, M. P. (2021). Current advances in microalgae harvesting and lipid extraction processes for improved biodiesel production: a review. Renew. Sustain. Energy Rev. 137, 110498. doi:10.1016/j.rser.2020.110498
Vázquez-Rodríguez, B., Gutiérrez-Uribe, J. A., Antunes-Ricardo, M., Santos-Zea, L., and Cruz-Suárez, L. E. (2020). Ultrasound-assisted extraction of phlorotannins and polysaccharides from Silvetia compressa (Phaeophyceae). J. Appl. Phycol. 32, 1441–1453. doi:10.1007/s10811-019-02013-2
Villagracia, A. R. C., Mayol, A. P., Ubando, A. T., Biona, J. B. M. M., Arboleda, N. B., David, M. Y., et al. (2016). Microwave drying characteristics of microalgae (Chlorella vulgaris) for biofuel production. Clean. Technol. Environ. Policy. 18, 2441–2451. doi:10.1007/s10098-016-1169-0
Wan, C., Zhao, X.-Q., Guo, S.-L., Alam, M. A., and Bai, F.-W. (2013). Bioflocculant production from Solibacillus silvestris W01 and its application in cost-effective harvest of marine microalga Nannochloropsis oceanica by flocculation. Bioresour. Technol. 135, 207–212. doi:10.1016/j.biortech.2012.10.004
Wang, G. (2002). Isolation and purification of phycoerythrin from red algaGracilaria verrucosa by expanded-bed-adsorption and ion-exchange chromatography. Chromatographia 56, 509–513. doi:10.1007/BF02492017
West, C., and Lesellier, E. (2006). Characterisation of stationary phases in subcritical fluid chromatography with the solvation parameter model IV: aromatic stationary phases. J. Chromatogr. A 1115, 233–245. doi:10.1016/j.chroma.2006.02.050
West, C., and Lesellier, E. (2011). “Comments on the paper “Characterization of stationary phases by a linear solvation energy relationship utilizing supercritical fluid chromatography” by C. R. Mitchell, N. J. Benz, S. Zhang,” Editors C. R. Mitchell, N. J. Benz, and S. Zhang 34, 1917–1924. doi:10.1002/jssc.201100278J. Sep. Sci.
Xia, M., Liu, C., Gao, L., and Lu, Y. (2019). One-step preparative separation of phytosterols from edible Brown seaweed Sargassum horneri by high-speed countercurrent chromatography. Mar. Drugs 17, 691. doi:10.3390/md17120691
Xia, S., Wang, K., Wan, L., Li, A., Hu, Q., and Zhang, C. (2013). Production, characterization, and antioxidant activity of fucoxanthin from the marine diatom odontella aurita. Mar. Drugs 11, 2667–2681. doi:10.3390/md11072667
Xiao, X.-H., Yuan, Z.-Q., and Li, G.-K. (2013). Preparation of phytosterols and phytol from edible marine algae by microwave-assisted extraction and high-speed counter-current chromatography. Sep. Purif. Technol. 104, 284–289. doi:10.1016/j.seppur.2012.11.032
Xu, L., Wang, F., Li, H. Z., Hu, Z. M., Guo, C., and Liu, C. Z. (2010). Development of an efficient electroflocculation technology integrated with dispersed air flotation for harvesting microalgae. J. Chem. Technol. Biot. 85, 1504–1507. doi:10.1002/jctb.2457
Xu, S.-Y., Huang, X., and Cheong, K.-L. (2017). Recent advances in marine algae polysaccharides: isolation, structure, and activities. Mar. Drugs 15, 388. doi:10.3390/md15120388
Yücetepe, A., Saroğlu, Ö., Daşkaya Dikmen, C., Bildik, F., and Özçelik, B. (2018). Optimisation of ultrasound-assisted extraction of protein from Spirulina platensis using RSM. Czech J. Food Sci. 36, 98–108. doi:10.17221/64/2017-CJFS
Zayed, A., Badawy, M. T., and Farag, M. A. (2021). Valorization and extraction optimization of Citrus seeds for food and functional food applications. Food Chem. 355, 129609. doi:10.1016/j.foodchem.2021.129609
Zayed, A., and Farag, M. A. (2020). Valorization, extraction optimization and technology advancements of artichoke biowastes: food and non-food applications. LWT 132, 109883. doi:10.1016/j.lwt.2020.109883
Zhang, Q.-W., Lin, L.-G., and Ye, W.-C. (2018). Techniques for extraction and isolation of natural products: a comprehensive review. Chin. Med. 13, 20. doi:10.1186/s13020-018-0177-x
Zhang, Y., Qu, J., Du, W., Wu, M., and Liu, L. (2020). Molecularly imprinted polymer solid phase extraction coupled with liquid chromatography-high resolution mass spectrometry for the detection of gonyautoxins 2and3 in seawater. Mar. Pollut. Bull. 157, 111333. doi:10.1016/j.marpolbul.2020.111333
Zhang, Z., and Su, Z. (2000). Recovery of taxol from the extract of Taxus cuspidate callus cultures with Al2O3 chromatography. J. Liq. Chromatogr. Relat. Technol. 23, 2683–2693. doi:10.1081/JLC-100101826
Zhao, G., Chen, X., Wang, L., Zhou, S., Feng, H., Chen, W. N., et al. (2013). Ultrasound assisted extraction of carbohydrates from microalgae as feedstock for yeast fermentation. Bioresour. Technol. 128, 337–344. doi:10.1016/j.biortech.2012.10.038
Zheng, H., Zhao, Y., and Guo, L. (2022). A bioactive substance derived from Brown seaweeds: phlorotannins. Mar. Drugs 20, 742. doi:10.3390/md20120742
Zheng, L.-X., Chen, X.-Q., and Cheong, K.-L. (2020a). Current trends in marine algae polysaccharides: the digestive tract, microbial catabolism, and prebiotic potential. Int. J. Biol. Macromol. 151, 344–354. doi:10.1016/j.ijbiomac.2020.02.168
Zheng, Y.-F., Li, D.-Y., Sun, J., Cheng, J.-M., Chai, C., Zhang, L., et al. (2020b). Comprehensive comparison of two color varieties of Perillae folium using rapid resolution liquid chromatography coupled with quadruple-time-of-flight mass spectrometry (RRLC-Q/TOF-MS)-based metabolic profile and in vivo/in vitro anti-oxidative activity. J. Agric. Food Chem. 68, 14684–14697. doi:10.1021/acs.jafc.0c05407
Keywords: natural products, marine algae, macroalgae, extraction techniques, separation techniques
Citation: Zayed A, M. El-Seadawy H, Attia EZ, I. Rushdi M and Abdelmohsen UR (2024) Adopting biorefinery and a circular bioeconomy for extracting and isolating natural products from marine algae. Front. Nat. Produc. 3:1425242. doi: 10.3389/fntpr.2024.1425242
Received: 29 April 2024; Accepted: 04 June 2024;
Published: 20 September 2024.
Edited by:
Leonel Pereira, University of Coimbra, PortugalReviewed by:
Félix López Figueroa, University of Malaga, SpainCopyright © 2024 Zayed, M. El-Seadawy, Attia, I. Rushdi and Abdelmohsen. This is an open-access article distributed under the terms of the Creative Commons Attribution License (CC BY). The use, distribution or reproduction in other forums is permitted, provided the original author(s) and the copyright owner(s) are credited and that the original publication in this journal is cited, in accordance with accepted academic practice. No use, distribution or reproduction is permitted which does not comply with these terms.
*Correspondence: Usama Ramadan Abdelmohsen, dXNhbWEucmFtYWRhbkBtdS5lZHUuZWc=
Disclaimer: All claims expressed in this article are solely those of the authors and do not necessarily represent those of their affiliated organizations, or those of the publisher, the editors and the reviewers. Any product that may be evaluated in this article or claim that may be made by its manufacturer is not guaranteed or endorsed by the publisher.
Research integrity at Frontiers
Learn more about the work of our research integrity team to safeguard the quality of each article we publish.