- Laboratory of Pharmacology and Toxicology of Natural Products, Institute of Biological Science, Federal University of Pará, Belém, Brazil
The essential oil of rosemary (Rosmarinus officinalis) (EORO) is widely used in folk medicine and has proven therapeutic effects. Our research evaluated high doses of rosemary essential oil in 54 Wistar rats between 180 and 200 g. The study consisted of three experiments: 1) behavioral monitoring of the animals after administration of 500 mg/kg i.p.; 2) electrocorticographic records after drug administration; 3) anticonvulsant drug reaction, where phenytoin, phenobarbital, and diazepam 10 mg/kg i.p were applied. The results showed that the application of EORO presented two phases. Phase 1 was characterized by the appearance of myorelaxation and a reduction in the power of the electrocorticogram in low-frequency cerebral oscillations. Phase 2 was characterized by increased excitability, with the appearance of convulsions and the increased power of electrocorticographic recordings in cerebral oscillations up to 40 Hz. In this phase, three tracing patterns were observed. Beta oscillations were the most prevalent and were better controlled by diazepam, which demonstrates that the excitatory activity of EORO is related to the reduction of GABAergic activity.
1 Introduction
Essential oils and their constituents have been presented as possible modulators of the central nervous system (CNS) (Figuêredo et al., 2019), and the presence of components such as terpenes in their constitutions is known to have anxiolytic, antidepressant, analgesic, and anticonvulsant activities, and to provoke excitability of the central nervous system (de Sousa et al., 2015; Chen et al., 2020). Rosmarinus officinalis (rosemary), belonging to the Lamiaceae botanical family, is an aromatic plant cultivated in different regions worldwide, with the Mediterranean as its center of origin (Murata et al., 2013; Oliveira et al., 2019). Rosemary is used to accelerate digestion, clear nasal passages, stimulate hair growth, and relieve rheumatic pain, as well as myalgias, neuralgias, and physical and mental fatigue.
Essential oil of R. officinalis (EORO) is also used as a memory and cognition stimulator, have antidiabetic, anti-inflammatory, and hepatoprotective properties, relieve dyslipidemia, and protect against glial cell tumors (Rašković et al., 2014; Rodrigues et al., 2020; Olah et al., 2017; Ozdemir and Goktuk, 2018; Borges et al., 2019; Allegra et al., 2020; Bao et al., 2020; Chen et al., 2020; Zappalà et al., 2021). According to Bellumori et al. (2021), Ahmed and Babakir-Mina (2020) and Paixão and de Carvalho (2021), rosemary has immunomodulatory and antimicrobial activity against bacteria (Staphylococcus epidermidis, S. aureus, Bacillus subtilis, Proteus vulgaris, Pseudomonas aeruginosa, and Escherichia coli) and fungi (Candida albicans and Aspergillus niger). Several studies have demonstrated the interactive relationship of EORO with the CNS, having activity in the cholinergic and dopaminergic pathways (Park et al., 2010; Sasaki et al., 2012; Borrás-Linares et al., 2014; Kayashima et al., 2020). Studies of stimulant effects are related to the components 1,8-cineole (oxide) and α-pinene (monoterpene) which, through sympathetic activity, stimulate the autonomic nervous system and increase blood pressure and respiratory rate measurements (Howes and Houghton, 2003; Sasaki et al., 2012; Kayashima et al., 2020). In line with these studies, Schriever et al., 2017 and DeGuzman et al., 2020 recorded by electroencephalography a significant decrease in the power of alpha waves in the bilateral middle frontal regions; they associated this result with increased alertness they clinically observed.
It is known that despite advances in the understanding of epilepsy, the mechanisms responsible for the epileptic phenomenon and its cellular bases are still not fully understood, although existing studies indicate that EORO interacts with the CNS. The behavioral and electrocorticographic characterization during these interactions caused by EORO have never been described and can help us understand the pathophysiological mechanisms that underlie epilepsies.
2 Materials and methods
2.1 Animals
For this study, 54 heterogeneous male Wistar rats, aged 8–10 weeks and weighing 180–200 g were used. They originated from the Central Animal Facility of the Federal University of Pará ICB (UFPA) and accommodated in the vivarium of its Laboratory of Pharmacology and Toxicology of Natural Products (LFTPN/UFPA). The animals were acclimatized to laboratory conditions 5 days before the experimental manipulation in boxes measuring 50 cm × 60 cm × 20 cm (height × width × depth) with wood shavings, at a temperature adjusted to 25–28 °C, 12-h light/dark cycle, receiving rodent food and filtered water during the tests. The experimental procedures followed the guidelines of the Ethics Committee in Research with Experimental Animals of the Federal University of Pará – (CEPAE–UFPA) under CUS approval number 6301260821.
2.2 Acquisition and composition of essential oil
Essential oil of R. officinalis (EORO) was purchased from Harmonie Aromatherapy (Florianópolis, SC, Brazil, CNPJ: 11.938.821/0001-90). It was extracted by steam distillation and analyzed by high-performance gas chromatography on an AGILENT 7820A Gas Chromatograph under the following conditions. Column: RXi-5MS 30 m × 0.25 mm × 0.25 μm (Restek). Temp.: column: 50 °C (0 min), 3° C/min at 200 °C; injector: 200 °C split: 1/50; FID detector: 220 °C. Vol. injection: 1 µL (1% in ethyl acetate) (Figure 1). The phytoconstituents that make up the oil are eucalyptol (47.5%), camphor (19.3%), α-pinene (12.2%), β-pinene (7.8%), and β-caryophyllene (4.6%) (Table 1).
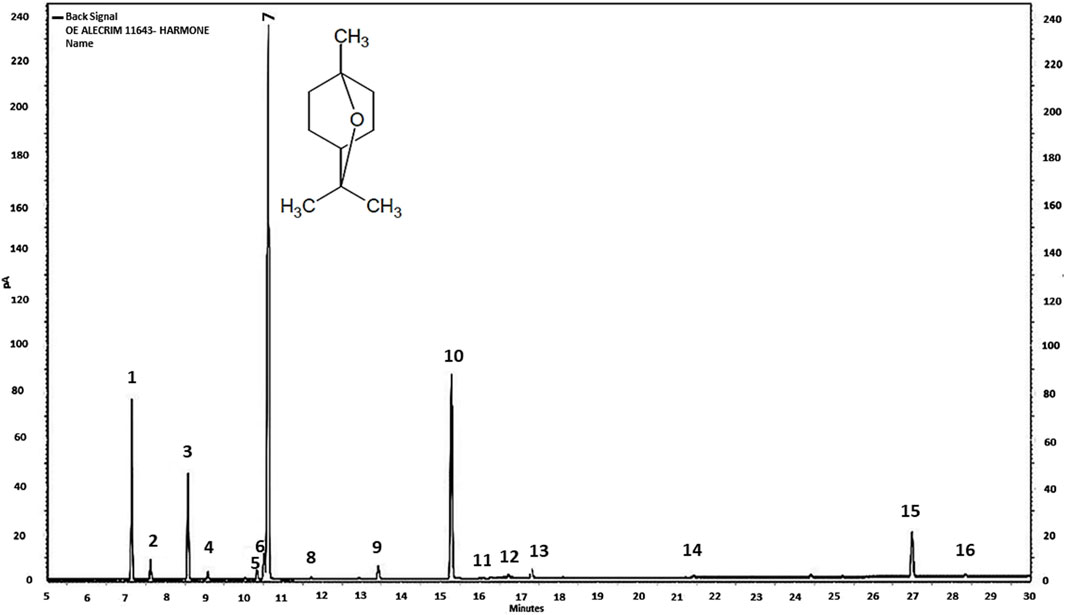
Figure 1. Chromatogram of R. officinalis essential oil sample Chromatography Laboratory, Department of Chemistry—Federal University of Minas Gerais, Belo Horizonte, 19 August 2019. 1 (alpha-pinene), 2 (camphene), 3 (beta-pinene), 4 (myrcene), 5 (para-cymene), 6 (limonene), 7 (eucalyptol), 8 (gamma), 9 (linalool), 10 (camphor), 11 (borneol), 12 (terpinen-4-ol), 13 (alpha terpineol), 14 (bornyl acetate), 15 (beta-caryophyllene), and 16 (humolene).
2.3 Drugs used
The drugs were acquired as follows: ketamine hydrochloride from König (Santana de Parnaíba, SP, Brazil); xylazine hydrochloride from Vallée (Montes Claros, MG, Brazil); phenobarbital anticonvulsant compounds from Aventis-Pharma (Ribeirão Preto, SP, Brazil), and phenytoin and diazepam from União Química (Embu-Guaçu, SP, Brazil).
2.4 Experimental design
2.4.1 Experiment I: Behavioral characterization
The convulsant doses used were 300 mg/kg and 600 mg/kg i.p; through linear regression, considering the behavior of clonic seizure with partial loss of posture reflex, the indicated dose was 500 mg/kg. The drug was previously diluted in reconstituted peanut oil at 10% (100 mg/mL). The control group just with the injection of peanut oil in a volume equivalent did not present any behavioral alterations. The animals were submitted to behavioral analysis to evaluate the latency period of the appearance of behaviors after the administration of 500 mg/kg i.p. EORO forf 60 min (n = 9). Preliminary tests made it possible to determine the effective dose (ED50) at 500 mg/kg to trigger seizures with loss of postural reflex.
2.4.2 Experiment II: Electrocorticographic characterization
Five days after electrode implantation, electrocorticograms (ECoGs) were performed for 50 min after the injection of EORO 500 mg/kg i.p. This experiment was designed as follows. The control group received i.p. of 0.9% saline solution in equivalent volume, then ECoG recording was performed (n = 9). The animals in the EORO group received an EORO injection of 500 mg/kg i.p., then ECoG recording was performed (n = 9).
2.4.3 Experiment III: Action of anticonvulsants
EORO-induced seizures were attenuated with three different anticonvulsants: phenytoin (PHT) 10 mg/kg i.p., phenobarbital (PBT) 10 mg/kg i.p., and diazepam (DZP) 10 mg/kg i.p. The groups were organized as follows. a) EORO (500 mg/kg i.p.) 10 min before the application of phenytoin at a dose of 10 mg/kg i.p. followed by the ECoG recording for 30 min. b) EORO (500 mg/kg i.p.) 10 min before application of phenobarbital at a dose of 10 mg/kg i.p. followed by the ECoG recording for 30 min. c) EORO (500 mg/kg i.p.) 10 min before the application of diazepam at a dose of 10 mg/kg i.p. followed by the ECoG recording for 30 min. To evaluate the seizure control by anticonvulsants, the animals were submitted to the same electrocorticographic recording protocol as step two and received intraperitoneal EORO and 10 min later received phenytoin, phenobarbital, and diazepam, followed by ECoG recording for 30 min.
2.5 Surgery for electrode implantation
The animals were anesthetized by intraperitoneal injection of an association of ketamine hydrochloride at a dose of 100 mg/kg and xylazine hydrochloride at a dose of 10 mg/kg. The degree of anesthetic depth was evaluated. After anesthesia, the animals were placed in a stereotaxic apparatus. Stainless steel electrodes, with an exposed tip 1.0 mm in diameter, were placed on the dura above the frontal cortex at the bregma coordinates −0.96 mm and ±1.0 mm laterally (Hamoy et al., 2018) in the motor cortex region. A screw was fixed in the skull, and the electrodes were fixed with dental acrylic cement (self-curing acrylic).
2.6 Electrocorticography record
The recordings were obtained through a differential amplifier with high impedance AC input (Grass Technologies, Model P511) adjusted with 0.3 Hz and 0.3 KHz filtering, monitored with an oscilloscope (Protek, Model 6510), and continuously digitized at a 1 KHz rate by a computer equipped with a data acquisition card (National Instruments, Austin, TX). The analyses were performed using a tool built using the Python programming language (version 5.0). The “Numpy” and “Scipy” libraries were used for mathematical processing, and the “matplolib” library was used to obtain graphs and plots. The results were submitted to descriptive statistics as mean and standard deviation. One-way analysis of variance (ANOVA) was used, followed by the Tukey test. A significance index of *p < 0.05, **p < 0.01, and ***p < 0.001 was adopted. Analyses were performed at a frequency of up to 40 Hz, and divided into bands according to Jalilifar et al. (2018) in beta (1–4 Hz), theta (4–8 Hz), alpha (8–12), beta (12–28), and gamma (28–40 Hz) for the interpretation of dynamics during the development of crises.
3 Results
3.1 Behavioral characterization
The behavioral observations obtained after the administration of essential oil of R. officinalis (EORO) at a dose of 500 mg/kg i.p, were characterized by two phases: CNS depression (phase 1) followed by CNS excitability (phase 2) (Table 2). In phase 1, it is possible to perceive two distinct stages: immobility and myorelaxation. Immobility starts after approximately 5 min, with a latency of 301.2 ± 42.78 s. Myorelaxation, in turn, starts at approximately 8 min, with a latency of 458.9 ± 62.20 s (Table 2). Phase 2 begins approximately 15 min after the application of EORO, characterized by excitability and the appearance of generalized clonic convulsion. The convulsion observed in animals demonstrated four characteristics: head and neck spasms, clonic convulsion of the thoracic limbs, generalized clonic convulsion with transient loss of postural reflex, and generalized clonic convulsion with loss of postural reflex (Table 2).
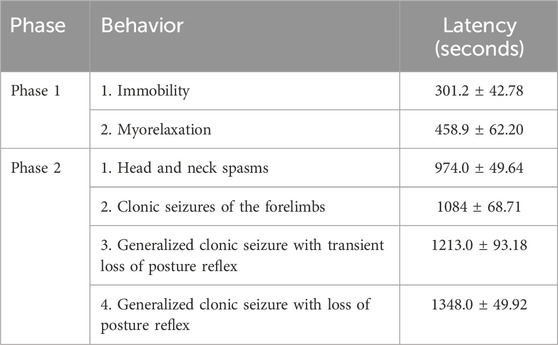
Table 2. Characterization of the phases and latency of the occurrence of behaviors (60-min observation) (n = 9).
3.2 ECoG according to phases of depression and cerebral excitability with different tracing patterns caused by EORO
The animals in the control group walked normally, showed ECoG characteristics with low tracing amplitude (Figure 2A, left), and revealed a frequency spectrogram with energy intensity concentrated at frequencies below 10 Hz (Figure 2A, right). During phase 1, which occurred shortly after the application of EORO, muscle relaxation was observed in behavioral analysis. Furthermore, the ECoG recorded a decrease in the amplitude of the trace (Figure 2B, left), with a slight reduction of the energy level in the frequency oscillations up to 40 HZ (Figure 2B, right) compared to the control spectrogram (Figure 2A, right). During phase 2, much larger amplitude traces can be noticed (Figure 2C, left) with signal intensification according to the frequency spectrogram achieved (Figure 2C, right), contrasting with the patterns observed in control and during phase 1. The behavioral responses were in line with the two distinct phases observed in the ECoG analysis (Figure 3). In phase 2, three tracing patterns were observed: pattern A characterized by a change in the tracing at the beginning of excitability with an amplitude below 0.5 mV; pattern B characterized by a polypoint wave that repeats with an amplitude of 2 mV but at a lower frequency than pattern A; Pattern C that presents a high-frequency polypoint with a burst firing pattern of potentials with an amplitude of 4 mV (Figures 3A,B).
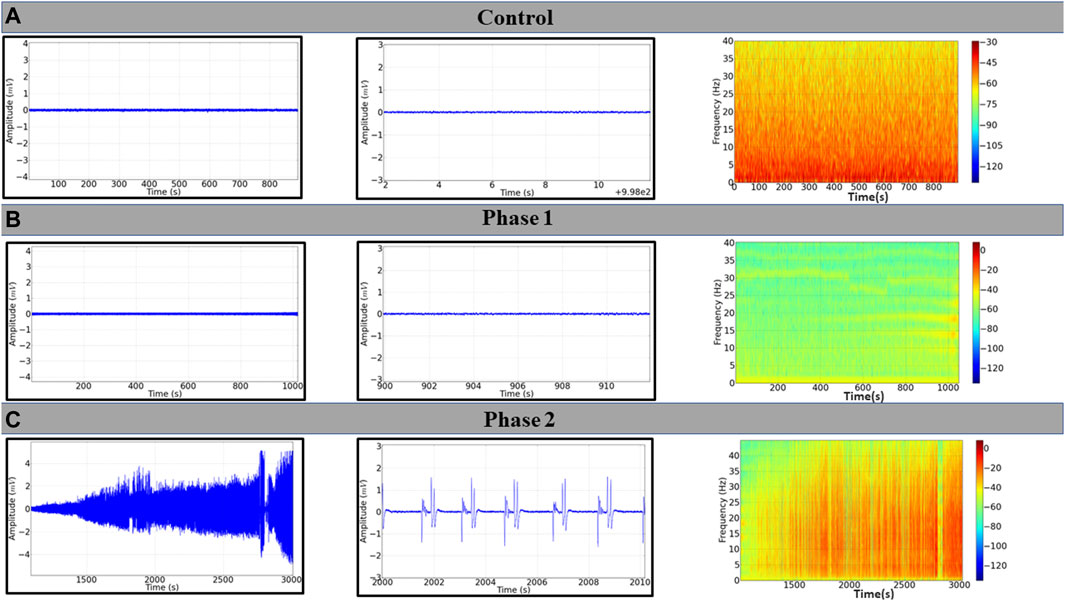
Figure 2. Electrocorticographic (ECoG) tracings of Wistar rat after application of 500 mg/kg i.p. of EORO. (A) ECoG traces of the control group; (B) ECoG registration during Phase 1; (C) seizure pattern in Phase 2. Corresponding records are shown on the central panels (10 s); frequency spectrograms are shown on the right.
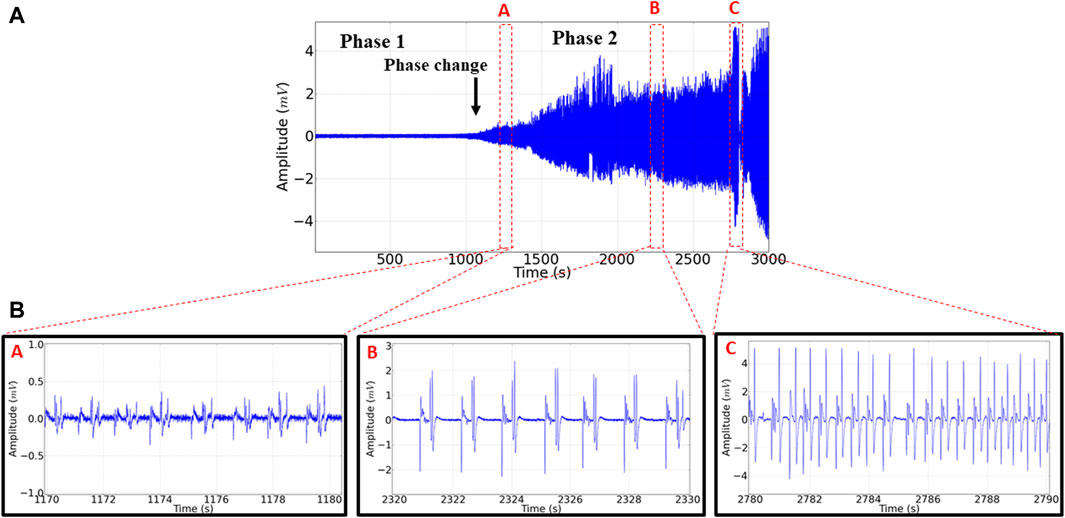
Figure 3. (A) Electrocorticographic recording (ECoG) obtained after application of EORO at 500 mg/kg i.p. showing phases 1 and 2. The black arrow indicates the beginning of phase 2. Three ECOG tracing patterns were identified in the second phase, shown as red dots: pattern (A) (1,170–1,180 s), pattern (B) (2,320–2,330 s), and pattern (C) (2,780–2,790 s). All with 10 s recording in phase 2.
3.3 Analysis of brain oscillations during the phases and tracing patterns observed in phase 2
The linear power between control and phase 1 were similar (p = 0.999). However, the control group was smaller than the other groups in phase 2. Phase 2 of the registration was similar to pattern B (=0.9551). Linear power was increased according to patterns A, B, and C (Figure 4A). For linear power in delta oscillations, the control group was similar to phase 1 (p = 0.9701) and pattern A group (p = 0.9785). The phase 1 group was similar to pattern A (p = 0.6673). Phase 2 was larger than pattern A and smaller than patterns B and C (Figure 4B). For theta oscillations, the animals in the control group were similar to phase 1 (p = 0.9988) and pattern A group (p = 0.5503). The phase 1 group and firing pattern A were similar (p = 0.2806). The power of the recordings in phase 2 was greater than the control, phase 1, and standard A groups. The groups of patterns B and C presented the highest powers recorded in theta oscillations (Figure 4C). For alpha oscillations, the control group was similar to phase 1 (p = 0.999), and it was similar to standard group A (p = 0.5765). Phase 1 was similar to pattern A (0.5068), as was phase 2 (p = 0.3406). Patterns A, B, and C showed an increase in alpha power according to the evolution of the recording (Figure 4D). For beta oscillations, recordings from animals that received EORO showed greater beta wave power in phase 2 than the control and phase 1 groups. Phase 2 was similar to standard group A (p = 0.0867). The control group showed oscillations in the beta band similar to the group in phase 1 (p = 0.999). Standard groups B and C showed greater beta potency (Figure 4E). For gamma oscillations, the phase 2 group showed greater power in relation to the control and phase 1 groups. The control group and phase 1 were similar (p = 0.999). Phase 2 was similar to standard group A, (p = 0.0632). Groups B and C presented the highest average power in gamma oscillations (Figure 4F).
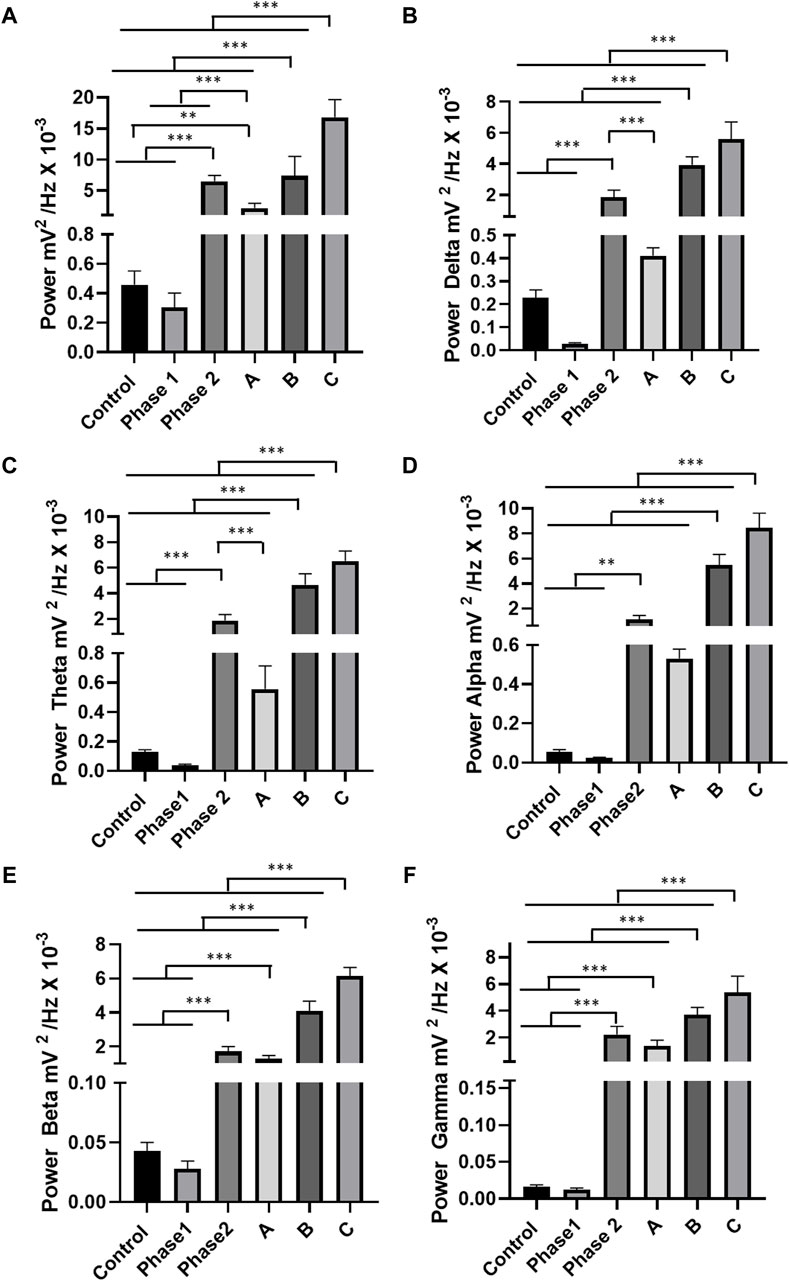
Figure 4. Quantitative linear frequency distribution of brain waves: For oscillations of 0–40 Hz in phases 1 and 2 and patterns A, B, and C of the tracings (A); delta oscillations (1–4 Hz) (B); theta oscillations (4–8 Hz) (C); alpha oscillations (8–13 Hz) (D); beta oscillations (13–28 Hz) (E); gamma oscillations (28–40 Hz) (F). The test used was one-way ANOVA. Data expressed as mean ± SD (n = 9 animals per group; *p < 0.05, **p < 0.01, and ***p < 0.001).
3.4 Evaluation of anticonvulsant drugs
To assess the control of the seizures observed in phase 2, anticonvulsants were applied after EORO administration: phenytoin (10 mg/kg i.p.), phenobarbital (10 mg/kg i.p.), and diazepam (10 mg/kg i.p.). The recording patterns obtained with the use of anticonvulsants are shown in Figure 5 A, B, and C. Oscillations in the beta band showed an increase in phase 2 characterized by seizures; thus, the action of anticonvulsant drugs has been tested for oscillations in beta (12–28 Hz). For the control group, the fluctuations in beta were lower than the other groups, except for the group treated with diazepam (p = 0.7758). Registration in phase 2 was higher than the other groups. The group treated with phenytoin was larger than the groups treated with phenobarbital and diazepam. The diazepam-treated group was similar to the phenobarbital-treated group (p = 0.0879) (Figure 5D).
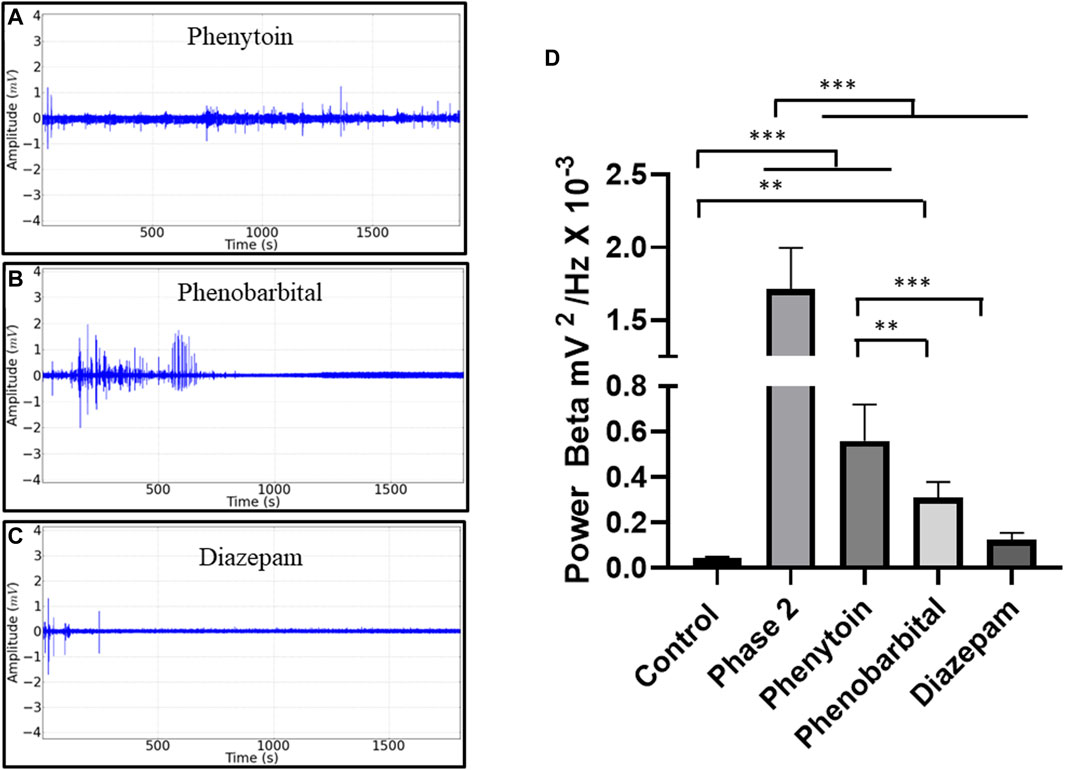
Figure 5. Electrocorticographic recordings (ECoG) obtained in phase 2 in beta brain oscillations after anticonvulsant application. Demonstration of the recording pattern found after application of phenytoin 10 mg/kg i.p. (A), phenobarbital at 10 mg/kg ip (B), diazepam at 10 mg/kg ip (C), and mean potency values showing the seizure activity in Phase 2 (D) ANOVA and Tukey’s test (n = 9). The p values between the mean amplitudes are represented by asterisks (*p < 0.05; **p < 0.01; ***p < 0.001)
4 Discussion
Many substances contained in essential oils have anticonvulsant effects and may benefit people with epilepsy. Compounds such as carvone, citral, eugenol, or linalool are present as promising agents with antiepileptic activity. However, some essential oils are proconvulsant or are even present anticonvulsant and proconvulsant compounds in the same essential oil (Filho et al., 2006; Lopes et al., 2008; Quintans-Júnior et al., 2008; Sousa et al., 2008; Subhan et al., 2008; Bahr et al., 2019; de Oliveira et al., 2020; Mathew et al., 2021; de Oliveira et al., 2022; de Araújo et al., 2023). The behavior of the animals after the application of EORO initially showed depression of the central nervous system with the presence of intense myorelaxation that characterized the first phase of the behavior. However, a phase of excitability was revealed with the appearance of convulsive crises in the second phase, which demonstrated to the same components of the essential oil a decrease of excitability in the initial period and then an increase of excitability. A similar behavior was observed in the ethanolic extract of Nerium oleander, but with different components—in this case oleandrin, which corresponds to a digitaloid (de Melo et al., 2020). Figuêredo et al. (2019) studied the effects of 1,8-cineol (eucalyptol 50 mg/kg)—the key phytochemical component of EORO—in the CNS of mice through the analysis of a behavioral model, finding that the latency of death was significantly prolonged in the groups which were submitted to convulsion induced by PTZ, which is a power stimulant of CNS due to its inhibitory capacity of the receptor GABAA in the control group. This corroborates our phase 1 results that EORO seems to act as a depressor of CNS. A study on the essential oil of Ocimum basilicum also suggested the hypnotic and anticonvulsant activities of this oil in the presence of terpene like the 1,8—cineole and linalool (Ismail, 2006). Camphor, the second biggest key phytochemical component of EORO, was studied by Ferreira et al. (2020) through electrocardiographic analysis where moderate neuronal hyperexcitability, fast evolution to tonic-clonic convulsion, and alterations in the electrocardiographic registers presented characters of epileptiform activity with an increase in the total power of the wave, revealing an increase in the delta and theta waves. This corroborated our phase 2 results that the EORO was a CNS exciter, and therefore a proconvulsant. In the phytochemical analysis of the oil used in the study, the compound linalool was identified. This is an acyclic monoterpene that is well-known for its potential in aromatherapy and cosmetics. It is a compound that has anxiolytic potential in animal models capable of potentiating the function of GABA in the GABA A receptor (Milano et al., 2017) and inhibiting the excitatory action of glutamate receptors (Elisabetsky et al., 1999; Kessler et al., 2012, Ohkuma et al., 2002). Linalool derivatives and metabolites including linalool oxide, linalyl acetate, eight-oxolinalyl acetate, 8-carboxylinalyl acetate, and 8-oxolinalool also increase GABAergic activity and may have anticonvulsant effects (Linck et al., 2009; Vatanparast et al., 2017; Bahr et al., 2019) (Table 1). Hydroxylation at C8 of linalyl acetate led to reduced GABAergic responses (Granger et al., 2005; Milanos et al., 2017). The possible oxidation of linalool by P450 system oxidases may have contributed to the formation of different oxygenated byproducts that express distinct affinities and properties regarding the activation of GABA receptors (Boachon et al., 2015). The hydrophobicity of linalool favors greater allosteric interaction with GABA, since these receptors are embedded in the hydrophobic lipid bilayer of neurons (Khom et al., 2007). The oxidation of these compounds results in reduced modulation of the GABAA receptor (Khom et al., 2007). The linalool metabolism can thus directly contribute to the onset of seizures, reducing its effects on GABA, since other substances such as camphor and eucalyptol are found in the essential oil. These are known to promote epileptic events in humans, possibly due to their significant presence (Teis and Koren, 1995; Zibrowski et al., 1998; Pearce, 2008; Culić et al., 2009; Ferreira et al., 2020). This context justifies the two antagonistic phases dependent on the time of contact with the EORO, which can be observed in the electrocorticogram. During phase 2, there are characteristic peaks of asynchronous brain activity (Figure 2) with low amplitude patterns and high frequency, low frequency and high amplitude with polypoint wave, and high amplitude and high frequency with similar morphographic elements between shots with an increase in recorded power, demonstrating the intensity of the convulsive condition (Figures 3A,B). The evaluation of brain band oscillations during phase 1 showed a decrease in delta oscillations (1–4 Hz) in relation to the control group. For phase 2, all oscillations up to 40 Hz showed an increase in potency, but beta oscillations showed greater amplitude during seizures. According to Jalilifar et al. (2018) and Hamoy et al. (2018), who studied different pro-convulsant substances, there is an increased preponderance of beta oscillations in the electrocorticogram . All anticonvulsants tested reduced beta oscillations during seizures, although phenobarbital and diazepam performed better, demonstrating the inhibition of the GABAergic pathway by EORO. Research has already described the ability of camphor to induce seizures (Dubovsky, 1995; Pearce, 2008; Burkhard et al., 1999; Ferreira et al., 2020). EORO has components capable of initially depressing and subsequently causing excitability in the central nervous system with characteristic recording patterns that are repeated during the ECoG tracing. The excitability phase is related to the decrease in GABA activity, which can be reversed more effectively with the use of diazepam. EORO has antagonistic effects that can be observed depending on the time of contact with the body.
Data availability statement
The original contributions presented in the study are included in the article/supplementary materials; further inquiries can be directed to the corresponding authors.
Ethics statement
The animal study was approved by Ethics Committee in Research with Experimental Animals of the Federal University of Pará—CEPAE—UFPA under CUS approval number 6301260821. The study was conducted in accordance with the local legislation and institutional requirements.
Author contributions
DB: Methodology, writing–original draft, writing–review and editing. YR: Methodology, writing–original draft, writing–review and editing. MO: Methodology, writing–original draft. LV: Methodology, writing–original draft. PP: writing–original draft, writing–review and editing. RG: Methodology, writing–original draft. LS: Methodology, writing–original draf. LL: Methodology, writing–original draf. MF: Methodology, writing–original draf. YS: Methodology, writing–original draf. RC: writing–original draft, writing–review and editing. RV: Methodology, writing–original draft. GB: Methodology, writing–original draft. MH: Methodology, writing–original draft, writing–review and editing, Data curation, Supervision, Conceptualization, Funding acquisition, Resources, Visualization, Software, Formal analysis, Project administration, Validation, Investigation.
Funding
The author(s) declare that financial support was received for the research, authorship, and/or publication of this article. This research was funded by Fundação Amazônia de Amparo a Estudos e Pesquisas do Estado do Pará (FAPESPA) and The APC was funded by Pró-Reitoria de Pesquisa e PósGraduação—PROPESP/UFPA.
Acknowledgments
This study was financed by grants to encourage undergraduate and graduate research from the Federal University of Pará.
Conflict of interest
The authors declare that the research was conducted in the absence of any commercial or financial relationships that could be construed as a potential conflict of interest.
Publisher’s note
All claims expressed in this article are solely those of the authors and do not necessarily represent those of their affiliated organizations, or those of the publisher, the editors, and the reviewers. Any product that may be evaluated in this article, or claim that may be made by its manufacturer, is not guaranteed or endorsed by the publisher.
References
Ahmed, H. M., and Babakir-Mina, M. (2020). Investigation of rosemary herbal extracts (<i>Rosmarinus officinalis</i>) and their potential effects on immunity. Phytother. Res. 34 (8), 1829–1837. doi:10.1002/ptr.6648
Allegra, A., Tonacci, A., Pioggia, G., Musolino, C., and Gangemi, S. (2020). Anticancer activity of Rosmarinus officinalis L.: mechanisms of action and therapeutic potentials. Nutrients 12 (6), 1739. doi:10.3390/nu12061739
Bahr, T. A., Rodriguez, D., Beaumont, C., and Allred, K. (2019). The effects of various essential oils on epilepsy and acute seizure: a systematic review. Evid. Based Complement. Altern. Med. 2019, 1–14. doi:10.1155/2019/6216745
Bao, T. Q., Li, Y., Qu, C., Zheng, Z. G., Yang, H., and Li, P. (2020). Antidiabetic effects and mechanisms of rosemary (Rosmarinus officinalis L.) and its phenolic components. Am. J. Chin. Med. 48 (06), 1353–1368. doi:10.1142/S0192415X20500664
Bellumori, M., Innocenti, M., Congiu, F., Cencetti, G., Raio, A., Menicucci, F., et al. (2021). Within-plant variation in Rosmarinus officinalis L. Terpenes and phenols and their antimicrobial activity against the rosemary phytopathogens Alternaria alternata and Pseudomonas viridiflava. Molecules 26 (11), 3425. doi:10.3390/molecules26113425
Boachon, B., Junker, R. R., Miesch, L., Bassard, J. E., Höfer, R., Caillieaudeaux, R., et al. (2015). CYP76C1 (Cytochrome P450)-mediated linalool metabolism and the formation of volatile and soluble linalool oxides in Arabidopsis flowers: a strategy for defense against floral antagonists. Plant Cell. 27 (10), 2972–2990. doi:10.1105/tpc.15.00399
Borges, R. S., Ortiz, B. L. S., Pereira, A. C. M., Keita, H., and Carvalho, J. C. T. (2019). Rosmarinus officinalis essential oil: a review of its phytochemistry, anti-inflammatory activity, and mechanisms of action involved. J. Ethnopharmacol. 229, 29–45. doi:10.1016/j.jep.2018.09.038
Borrás-Linares, I., Stojanović, Z., Quirantes-Piné, R., Arráez-Román, D., Švarc-Gajić, J., Fernández-Gutiérrez, A., et al. (2014). Rosmarinus officinalis leaves as a natural source of bioactive compounds. Int. J. Mol. Sci. 15 (11), 20585–20606. doi:10.3390/ijms151120585
Burkhard, P. R., Burkhardt, K., Haenggeli, C. A., and Landis, T. (1999). Plant-induced seizures: reappearance of an old problem. J. Neurol. 246, 667–670. doi:10.1007/s004150050429
Chen, X. L., Luo, Q. Y., Hu, W. Y., Chen, J. J., and Zhang, R. P. (2020). Abietane diterpenoids with antioxidative damage activity from Rosmarinus officinalis. J. Agric. Food Chem. 68 (20), 5631–5640. doi:10.1021/acs.jafc.0c01347
Ćulić, M., Keković, G., Grbić, G., Martać, L., Soković, M., Podgorac, J., et al. (2009). Wavelet and fractal analysis of rat brain activity in seizures evoked by camphor essential oil and 1, 8-cineole. Gen. Physiol. Biophys. 28, 33–40.
de Araújo, D. B., do Amaral, A. L. G., da Fonseca, S. M., de Souza, K. R., da Paz, A. P. S., de Mello, V. J., et al. (2023). Lippia origanoides essential oil possesses anticonvulsant effect in pentylenetetrazol-induced seizures in rats: a behavioral, electroencephalographic, and electromyographic study. Front. Pharmacol. 14, 1289336. doi:10.3389/fphar.2023.1289336
DeGuzman, P., Jain, A., Tabert, M. H., and Parra, L. C. (2020). Olfaction modulates inter-subject correlation of neural responses. Front. Neurosci. 14, 702. doi:10.3389/fnins.2020.00702
de Melo, B. S., de Morais, B. P., Sá, V. S. D. S. F., Lourinho, F. D., Toda, I. P. S. P., do Nascimento, J. L. M., et al. (2020). Behavioural, electrocorticographic, and electromyographic alterations induced by Nerium oleander ethanolic extract: anticonvulsant therapeutics assessment. Neurotoxicology 78, 21–28. doi:10.1016/j.neuro.2020.02.001
de Oliveira, F. R., da Silva, N. M., Hamoy, M., Crespo-López, M. E., Ferreira, I. M., da Silva, E. O., et al. (2022). The GABAergic system and endocannabinoids in epilepsy and seizures: what can we expect from plant oils? Molecules 27 (11), 3595. doi:10.3390/molecules27113595
de Oliveira, F. R., Rodrigues, K. E., Hamoy, M., Sarquis, Í. R., Hamoy, A. O., Lopez, M. E. C., et al. (2020). Fatty acid amides synthesized from andiroba oil (carapa guianensis aublet.) exhibit anticonvulsant action with modulation on GABA-A receptor in mice: a putative therapeutic option. Pharm. (Basel) 13 (3), 43. doi:10.3390/ph13030043
de Oliveira, J. R., Camargo, S. E. A., and de Oliveira, L. D. (2019). Rosmarinus officinalis L.(rosemary) as therapeutic and prophylactic agent. J. Biomed. Sci. 26 (1), 5–22. doi:10.1186/s12929-019-0499-8
de Sousa, D. P., Soares Hocayen, P. D. A., Andrade, L. N., and Andreatini, R. (2015). A systematic review of the anxiolytic-like effects of essential oils in animal models. Molecules 20 (10), 18620–18660. doi:10.3390/molecules201018620
Dubovsky, S. L. (1995). “Electroconvulsive therapy,” in Comprehensive textbook of psychiatry. Editors H. I. Kaplan, and B. J. Sadock (Baltimore: Williams & Wilkins), 2129.
Elisabetsky, E., Brum, L. S., and Souza, D. O. (1999). Anticonvulsant properties of linalool in glutamate-related seizure models. Phytomedicine 6 (2), 107–113. doi:10.1016/S0944-7113(99)80044-0
Ferreira, L. O., de Souza, R. D., Silva, F. D. S., Costa, F. F. M., Farias, R. A. F., Hamoy, A. O., et al. (2020). Electrocorticographic patterns dominated by low-frequency waves in camphor-induced seizures. Sci. Rep. 10 (1), 18222. doi:10.1038/s41598-020-75309-w
Figuêredo, F. R. S. D. N., Monteiro, A. B., Menezes, I. R. A., Sales, V. S., Nascimento, E. P., Rodrigues, C. K. S., et al. (2019). Effects of the Hyptis martiusii Benth. leaf essential oil and 1,8-cineole (eucalyptol) on the central nervous system of mice. Food Chem. Toxicol. 133, 110802. doi:10.1016/j.fct.2019.110802
Filho, J. M. B., Medeiros, K. C. P., Diniz, M. D. F. F., Batista, L. M., Athayde-Filho, P. F., Silva, M. S., et al. (2006). Natural products inhibitors of the enzyme acetylcholinesterase. Rev. Bras. Farmacogn. 16, 258–285. doi:10.1590/S0102-695X2006000200021
Granger, R. E., Campbell, E. L., and Johnston, G. A. (2005). (+)- and (−)-borneol: efficacious positive modulators of GABA action at human recombinant α1β2γ2L GABAA receptors. Biochem. Pharmacol. 69 (7), 1101–1111. doi:10.1016/j.bcp.2005.01.002
Hamoy, M., Batista, L. D. S., de Mello, V. J., Gomes-Leal, W., Farias, R. A. F., Batista, P. D. S., et al. (2018). Cunaniol-elicited seizures: behavior characterization and electroencephalographic analyses. Toxicol. Appl. Pharmacol. 360, 193–200. doi:10.1016/j.taap.2018.10.008
Howes, M. J. R., and Houghton, P. J. (2003). Plants used in Chinese and Indian traditional medicine for improvement of memory and cognitive function. Pharmacol. Biochem. Behav. 75 (3), 513–527. doi:10.1016/s0091-3057(03)00128-x
Ismail, M. (2006). Central properties and chemical composition of Ocimum basilicum essential oil. Pharm. Biol. 44, 619–626. doi:10.1080/13880200600897544
Jalilifar, M., Yadollahpour, A., Moazedi, A. A., and Ghotbeddin, Z. (2018). Quantitative analysis of the antiepileptogenic effects of low frequency stimulation applied prior or after kindling stimulation in rats. Front. Physiol. 9, 711. doi:10.3389/fphys.2018.00711
Kayashima, T., Nagao, K., Umino, M., Kaikiri, H., Shibata, S., and Matsubara, K. (2020). Anti-stress effects of rosemary (Rosmarinus officinalis L.) leaf extract on intestinal goblet cells and immobility of forced-swimming test in BALB/c mice. Biosci. Biotechnol. Biochem. 84 (11), 2385–2389. doi:10.1080/09168451.2020.1800445
Kessler, A., Villmann, C., Sahin-Nadeem, H., Pischetsrieder, M., and Buettner, A. (2012). GABAA receptor modulation by the volatile fractions of Sideritis species used as ‘Greek’or ‘Turkish’mountain tea. Flavour Fragr. J. 27 (4), 297–303. doi:10.1002/ffj.3099
Khom, S., Baburi, E. U., Timin, E., Hohaus, A., Trauner, G., Kopp, B., et al. (2007). Valerenic acid potentiates and inhibits GABA(A) receptors: molecular mechanism and subunit specificity. Neuropharmacology 53, 178–187. doi:10.1016/j.neuropharm.2007.04.018
Linck, V. D. M., da Silva, A. L., Figueiró, M., Piato, A. L., Herrmann, A. P., Birck, F. D., et al. (2009). Inhaled linalool-induced sedation in mice. Phytomedicine 16 (4), 303–307. doi:10.1016/j.phymed.2008.08.001
Lopes, E. M. C., Carreira, R. C., Agripino, D. G., Torres, L. M. B., Cordeiro, I., Bolzani, V. D. S., et al. (2008). Screening for antifungal, DNA-damaging and anticholinesterasic activities of Brazilian plants from the atlantic rainforest: ilha do cardoso state park. Rev. Bras. Farmacogn. 18, 655–660. doi:10.1590/S0102-695X2008000500002
Mathew, T., John, S. K., Kamath, V., Kumar, R. S., Jadav, R., Swamy, S., et al. (2021). Essential oil related seizures (EORS): a multi-center prospective study on essential oils and seizures in adults. Epilepsy Res. 173, 106626. doi:10.1016/j.eplepsyres.2021.106626
Milanos, S., Elsharif, S. A., Janzen, D., Buettener, A., and Villmann, C. (2017). Metabolic products of linalool and modulation of GABAA receptors. Front. Chem. 5, 46. doi:10.3389/fchem.2017.00046
Murata, K., Noguchi, K., Kondo, M., Onishi, M., Watanabe, N., Okamura, K., et al. (2013). Promotion of hair growth by Rosmarinus officinalis leaf extract. Phytother. Res. 27 (2), 212–217. doi:10.1002/ptr.4712
Olah, N. K., Benedec, D., Socaci, S., Toma, C. C., Filip, L., Morgovan, C., et al. (2017). Terpenic profile of different Rosmarinus officinalis extracts. Pak J. Pharm. Sci. 30 (4), 1439–1443.
Ohkuma, M., Kawai, F., and Miyachi, E. (2002). Direct suppression by odorants of ionotropic glutamate receptors in newt retinal neurons. J Neural Transm (Vienna). 109 (11), 1365–71. doi:10.1007/s00702-002-0741-4
Ozdemir, M. D., and Gokturk, D. (2018). The effect of Rosmarinus officinalis and chemotherapeutic etoposide on glioblastoma (U87 MG) cell culture. Turk Neurosurg. 28, 853–857. doi:10.5137/1019-5149.JTN.20401-17.3
Paixão, V. L. B., and de Carvalho, J. F. (2021). Essential oil therapy in rheumatic diseases: a systematic review. Complement. Ther. Clin. Pract. 43, 101391. doi:10.1016/j.ctcp.2021.101391
Park, S. E., Kim, S., Sapkota, K., and Kim, S. J. (2010). Neuroprotective effect of Rosmarinus officinalis extract on human dopaminergic cell line, SH-SY5Y. Cell. Mol. Neurobiol. 30 (5), 759–767. doi:10.1007/s10571-010-9502-3
Pearce, J. M. S. (2008). Leopold Auenbrugger: camphor-induced epilepsy - remedy for manic psychosis. Eur. Neurol. 59 (1-2), 105–107. doi:10.1159/000109581
Quintans Júnior, L. J., Almeida, J. R. G. S., Lima, J. T., Nunes, X. P., Siqueira, J. S., Oliveira, L. E. G., et al. (2008). Plants with anticonvulsant properties - a review. Rev. Bras. Farmacogn. 18, 798–819. doi:10.1590/S0102-695X2008000500026
Rašković, A., Milanović, I., Pavlović, N., Ćebović, T., Vukmirović, S., and Mikov, M. (2014). Antioxidant activity of rosemary (Rosmarinus officinalis L.) essential oil and its hepatoprotective potential. BMC Complement. Altern. Med. 14, 225. doi:10.1186/1472-6882-14-225
Rodrigues, A. P. S., Souza, B. S. F., Barros, A. S. A., Carvalho, H. O., Duarte, J. L., Boettger, M. L. E., et al. (2020). The effects of Rosmarinus officinalis L. essential oil and its nanoemulsion on dyslipidemic Wistar rats. J. Appl. Biomed. 18 (4), 126–135. doi:10.32725/jab.2020.016
Sasaki, K., Omri, A. E., Kondo, S., Han, J., and Isoda, H. (2012). Rosmarinus officinalis polyphenols produce anti-depressant like effect through monoaminergic and cholinergic functions modulation. Behav. Brain Res. 238, 86–94. doi:10.1016/j.bbr.2012.10.010
Schriever, V. A., Han, P., Weise, S., Hösel, F., Pellegrino, R., and Hummel, T. (2017). Time frequency analysis of olfactory induced EEG-power change. PLoS One 12 (10), e0185596. doi:10.1371/journal.pone.0185596
Sousa, F. C. F., Melo, C. T. V., Citó, M. C. O., Félix, F. H. C., Vasconcelos, S. M. M., Fonteles, M. M. F., et al. (2008). Plantas medicinais e seus constituintes bioativos: uma revisão da bioatividade e potenciais benefícios nos distúrbios da ansiedade em modelos animais. Rev. Bras. Farmacogn. 18, 642–654. doi:10.1590/S0102-695X2008000400023
Subhan, N., Alam, M. A., Ahmed, F., Shahid, I. J., Nahar, L., and Sarker, S. D. (2008). Bioactivity of Excoecaria agallocha. Rev. Bras. Farmacogn. 18, 521–526. doi:10.1590/S0102-695X2008000400004
Theis, J. G., and Koren, G. (1995). Camphorated oil: still endangering the lives of Canadian children. CMAJ 152 (11), 1821–1824.
Vatanparast, J., Bazleh, S., and Janahmadi, M. (2017). The effects of linalool on the excitability of central neurons of snail Caucasotachea atrolabiata. Comp. Biochem. Physiol. C Toxicol. Pharmacol. 192, 33–39. doi:10.1016/j.cbpc.2016.12.004
Zappalà, A., Vicario, N., Calabrese, G., Turnaturi, R., Pasquinucci, L., Montenegro, L., et al. (2021). Neuroprotective effects of Rosmarinus officinalis L. extract in oxygen glucose deprivation (OGD)-injured human neural-like cells. Nat. Prod. Res. 35 (4), 669–675. doi:10.1080/14786419.2019.1587428
Keywords: ethnopharmacology, electrocorticographic record, behavioral 7. characterization, rosemary essential oil, Rosmarinus officinalis
Citation: Bastos de Araújo D, Raiol de Almeida YN, Otake Hamoy MK, Vasconcelos de Souza L, Pacheco Hartcopff PF, Gonçalves dos Santos R, Santos da Silva LL, Lima da Rocha L, Farias dos Santos M, da Silva Deiga Y, da Cunha Ferreira R, Vieira de Souza R, Barbosa GB and Hamoy M (2024) Rosmarinus officinalis essential oil triggers depression followed by CNS excitability in Wistar rats. Front. Nat. Produc. 3:1394657. doi: 10.3389/fntpr.2024.1394657
Received: 01 March 2024; Accepted: 16 May 2024;
Published: 12 August 2024.
Edited by:
Ping-Jyun Sung, National Museum of Marine Biology and Aquarium, TaiwanReviewed by:
Ankanahalli N. Nanjaraj Urs, Washington University in St. Louis, United StatesAtallah F. Ahmed, King Saud University, Saudi Arabia
Copyright © 2024 Bastos de Araújo, Raiol de Almeida, Otake Hamoy, Vasconcelos de Souza, Pacheco Hartcopff, Gonçalves dos Santos, Santos da Silva, Lima da Rocha, Farias dos Santos, da Silva Deiga, da Cunha Ferreira, Vieira de Souza, Barbosa and Hamoy. This is an open-access article distributed under the terms of the Creative Commons Attribution License (CC BY). The use, distribution or reproduction in other forums is permitted, provided the original author(s) and the copyright owner(s) are credited and that the original publication in this journal is cited, in accordance with accepted academic practice. No use, distribution or reproduction is permitted which does not comply with these terms.
*Correspondence: Daniella Bastos de Araújo, ZGFuaXZpdmFmQGdtYWlsLmNvbQ==; Moisés Hamoy, aGFtb3l1ZnBhQGdtYWlsLmNvbQ==