- 1Central Analítica—Centro de Apoio Multidisciplinar (CAM), Universidade Federal do Amazonas (UFAM), Manaus, Brazil
- 2Departamento de Química, Universidade Federal do Amazonas (UFAM), Manaus, Brazil
- 3Grupo de Pesquisa em Metabolômica e Espectrometria de Massas, Universidade do Estado do Amazonas (UEA), Manaus, Brazil
Strychnos peckii (Loganiaceae) is an important active ingredient in curare poisons in the Amazon rainforest. Although previous studies have identified this species as a promising source of monoterpene indole alkaloids (MIAs), knowledge about other natural products is still scarce. Thus, to detect and guide the isolation of unprecedented bioactive compounds from the leaves of S. peckii, an untargeted high-performance liquid chromatography coupled with mass spectrometry (HPLC-MS) analysis was performed with the leaf aqueous extract. The HPLC-MS analysis allowed the detection of eleven compounds, including the alkaloids harman-3-carboxylic acid (5) and N,β-glucopyranosyl vincosamide (6), and the flavonoids quercetin 3-O-rhamnopyranoside (9) and kaempferol 3-O-rhamnopyranoside (10), all not previously reported in the Loganiaceae family. These compounds, along with strictosidine (3), 5-carboxystrictosidine (7), and desoxycordifoline (8) were isolated through modern chromatographic techniques and determined by using NMR spectroscopy in combination with MS. Overall, the untargeted HPLC-MS analysis proved to be a simple and effective approach to guide the isolation of substances not yet identified from S. peckii.
1 Introduction
The genus Strychnos, the largest genus of the Loganiaceae family, comprises about 200 species distributed throughout South and Central America, Africa, Asia, Australia, and Polynesia (Ohiri et al., 1983; Adebowale et al., 2014; Guimarães et al., 2015). In Brazil, 68 species (28 endemics) are registered, 50 of which have been described in the North region (Guimarães et al., 2015). Although this genus has gained worldwide notoriety due to its importance in curare preparation (Philippe et al., 2004), several Strychnos species are used in traditional medicine to treat diarrhea, fever, malaria, syphilis, and other diseases (Basílio et al., 2005; Silva et al., 2005; Bonamin et al., 2011). In general, this broad spectrum of biological activities can be attributed to the presence of alkaloids (Quetin-Leclercq et al., 1990; Philippe et al., 2004; Tchinda et al., 2012) and flavonoids (Nicoletti et al., 1984; Brasseur and Angenot, 1986). On the other hand, although many Strychnos species have been investigated from the phytochemical viewpoint, some species, especially those found in the Amazon region like S. peckii, still require complementary studies (Santos et al., 2019).
S. peckii B.L.Rob. is a liana species known worldwide as one of the active ingredients of curare (Philippe et al., 2004). Its distribution occurs in the North, Northeast, and Midwest regions of Brazil (Guimarães et al., 2015), but it is not an endemic species. A previous study based on strictosidine-derived alkaloids-targeted high-performance liquid chromatography coupled with mass spectrometry (HPLC-MS) analysis identified S. peckii as a promising source of monoterpene indole alkaloids (MIAs) (Santos et al., 2019), but no other phytochemical reports on this species are known. Recently, the application of modern analytical techniques, such as HPLC-MS, has been proven essential not only for the dereplication of alkaloids but also for the dereplication of phenolic compounds in plant extracts. (Paz et al., 2019; Silva et al., 2019; Lima et al., 2020). This mass spectrometry-based approach, typically relying on collision-induced dissociation (CID) fragmentation patterns, has demonstrated its effectiveness in identifying complex matrices and guiding the isolation steps of natural products (Paz et al., 2019; Lima et al., 2020). Thus, the aim of the present study was to detect and isolate unprecedented bioactive compounds from the leaf aqueous extract of S. peckii using untargeted HPLC-MS analysis. As a result, eleven compounds were detected, and seven of them were isolated, including the alkaloids harman-3-carboxylic acid (5) and N,β-glucopyranosyl vincosamide (6), as well as the flavonoids quercetin 3-O-rhamnopyranoside (9) and kaempferol 3-O-rhamnopyranoside (10).
2 Materials and methods
2.1 General experimental procedures
1D and 2D NMR experiments were acquired in a Bruker AVANCE III HD NMR spectrometer operating at 11.75 T (1H and 13C at 500 and 125 MHz, respectively). All 1H and 13C NMR chemical shifts (δ) are presented in ppm relative to the tetramethylsilane (TMS) signal at 0.00 ppm as an internal reference, and the coupling constants (J) are given in Hz. HPLC-MS analysis was performed with an Accela HPLC system coupled to a TSQ Quantum Access (QqQ) mass spectrometer. The semi-preparative HPLC analysis was carried out on a Shimadzu UFLC system (LC-6 AD pump; DGU-20A5 degasser; SPD-20AV UV detector; rheodyne injector; CBM-20A communication module). All solvents used for chromatography and MS experiments were HPLC grade purchased from Tedia (Fairfield, OH, United States) and the water was purified by a Milli-Q system.
2.2 Plant material and extraction
Leaves from S. peckii were collected in May 2018 at the Adolpho Ducke Forest Reserve (SisGen registration number, AF4CFA7), Manaus, Amazonas State, Brazil (02° 55′ 56.0″ S, 059° 58′ 16.0″ W). The specimen, which was at the maturity stage, was identified at the Herbarium of the National Institute of Research of Amazonia (INPA), where a voucher (number #282886) was deposited.
S. peckii leaves were immediately dried over ambient temperature (ca. 20°C) for 20 days (Resende et al., 2020), then powdered using an analytical knife micro-mill (80,374, Hamilton Beach, SP, Brazil). An aliquot of the powdered material (20 g) was extracted with distilled water (1,000 mL) by infusion (15 min) as previously described (Santos et al., 2019). The aqueous extract was filtered through Whatman 43 filter paper (Sigma-Aldrich) and then freeze-dried (Alpha 1-2 LDplus, Martin Christ, Germany), yielding the leaf aqueous extract (2.49 g, 12%).
2.3 HPLC-MS analysis
The leaf aqueous extract was diluted to 1 mg/mL in a water-methanol mixture (80:20, v/v) and analyzed (10 μL, injection volume) with an HPLC-MS system equipped with an electrospray ionization (ESI) source. The HPLC was equipped with a Phenomenex Luna C18 (2) column (5 μm, 150 mm × 4.6 mm i.d.) (Torrance, CA, United States). The mobile phase consisted of water (A) and MeOH (B). The gradient elution was as follows: 0–15 min, 20%–80% B; 15–25 min, 80% B at a flow rate of 1.0 mL/min. The ESI source parameters were the following: spray voltage, 4500 V; sheath gas pressure, 35 arbitrary unit (arb); ion sweep gas pressure, 0.0 arb; aux gas pressure, 10 arb; capillary temperature, 230°C; tube lens offset, 109 V; skimmer offset, 0 V; mass range, m/z 100–1,000; collision energy, 35 eV.
2.4 Cleanup procedure and isolation
Before fractionation, an aliquot of the leaf aqueous extract (1 g) was subjected to a cleanup procedure, as previously described (Silva et al., 2019). Briefly, the sample was solubilized in 3 mL of distilled water and passed through a cartridge containing 10 g of C18 phase previously activated with methanol HPLC (100 mL) and conditioned with water (100 mL). The column was washed with water (100 mL) and further eluted with methanol HPLC (100 mL). The methanol phase was dried under a nitrogen gas stream, yielding the methanol fraction (0.63 g).
An aliquot of the methanol fraction (150 mg) was subjected to fractionation by semi-preparative HPLC using water (A) and methanol (B) as mobile phases. The gradient elution was as follows: 0–24 min, 20%–80% B (v:v), at a flow rate of 3.5 mL/min. A C18 column (250 mm × 10 mm, 5 μm) (Phenomenex—Torrance, CA, United States) was employed in the fractionation, monitored by UV channels at 280 and 316 nm. Five injections (30 mg each) were carried out into the column in a water-methanol mixture (80:20, v/v) (100 μL), resulting in thirteen fractions (MF1-MF13). Fractions MF4 (3) (8.4 mg), MF8 (8) (19.9 mg), and MF10 (10) (10.9 mg) were subjected to NMR and MS analysis. The subfractions MF7.1 (5) (2.4 mg) and MF7.4 (7) (3.3 mg) were obtained from MF7 (19.4 mg), and MF9.2 (9) (2.0 mg) from MF9 (9.7 mg), which were individually subjected to fractionation by semi-preparative HPLC using the same mobile phase and C18 column. The gradient elution was as follows: 0–24 min, 30%–80% B (v:v), at a flow rate of 3.5 mL/min. The subfraction MF7.3 (3.2 mg) was further fractionated by semi-preparative HPLC using a PFP column (250 mm × 10 mm, 5 μm) (Phenomenex—Torrance, CA, United States) with a gradient elution as follows: 0–24 min, 30%–80% B (v:v), affording MF7.3.2 (6) (2.0 mg).
3 Results and discussions
The HPLC-MS total ion chromatogram (TIC) of the leaf aqueous extract (Figure 1) showed the presence of at least 7 major compounds (3, 5, 6, 7, 8, 9, and 10) and 4 minor compounds (1, 2, 4, and 11). Thus, all these compounds were subjected to HPLC-MS/MS analysis. The MS/MS spectra of the major compounds 3 (m/z 531), 7 (m/z 575), and 8 (m/z 571) displayed fragmentation patterns (Table 1) that are in agreement with the MIAs strictosidine (3), 5-carboxystrictosidine (7), and desoxycordifoline (8) (Figure 2), all previously reported from S. peckii (Santos et al., 2019). Also, the MS/MS spectra of the compounds 1 (m/z 547), 2 (m/z 529), 4 (m/z 517), and 11 (m/z 499) are in accordance with the MIAs 10-hydroxy strictosidine (1), 3,4-dehydro-strictosidine (2), strictosidinic acid (4), and strictosidine lactam (11) (Santos et al., 2019).
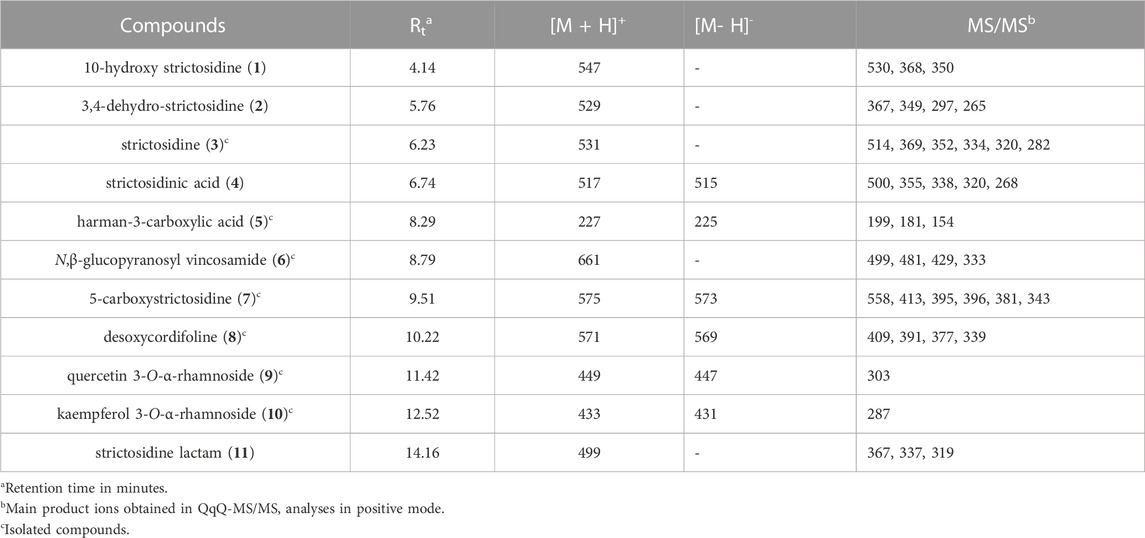
TABLE 1. Alkaloids and flavonoids reported in S. peckii with their precursor ions and major fragments.
Moreover, the MS/MS spectrum of the compound 6 (m/z 661) showed a high spectral similarity with strictosidine lactam (11) (−162 Da, m/z 661 → m/z 499; −18 Da, m/z 499 → m/z 481; −70 Da, m/z 499 → m/z 429), suggesting that these compounds differ by an additional sugar unit. Since the initial sugar loss did not affected the other competitive losses of 18 and 70 Da, also observed to 11, its glycosylation position was supposed to be at the tetrahydroharman moiety. To the best of our knowledge, there are no structures previously described in the Loganiaceae family with these characteristics. On the other hand, the MS/MS spectrum of the compound 5 (m/z 227) showed subsequent losses of CO (−28 Da, m/z 227 → m/z 199) and H2O (−18 Da, m/z 199 → m/z 181), also incompatible with any compound previously reported from the Loganiaceae family. Moreover, the MS/MS spectra of the compound 9 (m/z 449) and 10 (m/z 433) showed losses of 146 Da (m/z 449 → m/z 303; m/z 433 → m/z 287), suggesting the presence of rhamnoside derivatives in the sample (March et al., 2004), also not previously reported in Loganiaceae family. Thus, these compounds along with other majority compounds were isolated for further structural confirmation by 1D and 2D NMR analysis.
The compound 6 was isolated as an amorphous solid. As previously discussed, the mass spectra pointed this compound as a strictosidine lactam derivative containing a glucose unity in the tetrahydroharman moiety. Therefore, its structural confirmation was based mainly in key correlations for characteristic signals at this moiety. Several diagnostic signals for tetrahydroharman moiety were observed in the 1H NMR spectrum (Table 2), among them, aromatic protons at δH 7.52 (1H, d, J = 8.3 Hz, H-12), 7.47 (1H, d, J = 7.8 Hz, H-9), 7.10 (m, H-11), and 7.05 (m, H-10), confirming the absence of replacement in ring A. According to the HMBC spectrum, H-11 showed correlation with carbons C-13 (δC 135.0) and C-9 (δC 117.8). Also, the anomeric hydrogen H-1´´ [δH 5.06 (1H, d, J = 9.3 Hz)] showed correlations with C-13 (δC 135.0), which confirmed the N-substitution. The anomeric configuration was attributed as β through the characteristic chemical shift and large coupling constant (Gurst, 1991; Henriques et al., 2004). Thus, through the comparison of NMR data (Table 1) with the literature (Zhang et al., 2001; Henriques et al., 2004), compound 6 was identified as N,β-glucopyranosyl vincosamide. To the best of our knowledge this compound is being reported in Loganiaceae family for the first time. Regarding the biological activities of N-β-glucopyranosyl vincosamide, only one study describing its antioxidant potential has been previously reported (Matsuura and Fett-Neto, 2013). In this study, N-β-glucopyranosyl vincosamide demonstrated significant hydrogen peroxide quenching activity in situ. Additionally, in vitro antioxidant tests have also shown efficient quenching activity for this alkaloid. (Matsuura and Fett-Neto, 2013).
The other compounds were identified by analysis NMR and MS data and comparison with literature as strictosidine (3) (Patthy-Lukáts et al., 1997), harman-3-carboxylic acid (5) (Pereira et al., 2006), 5-carboxystrictosidine (7) (Rakumitsu et al., 2019), desoxycordifoline (8) (Brandt et al., 1999), quercetin 3-O-α-rhamnopyranoside (9) (Yuk et al., 2018), and kaempferol 3-O-rhamnopyranoside (10) (Yuk et al., 2018). To the best of our knowledge, the harman-3-carboxylic acid (5), quercetin 3-O-rhamnopyranoside (9), and kaempferol 3-O-rhamnopyranoside (10) are also reported in Loganiaceae family for the first time.
Similarly to N-β-glucopyranosyl vincosamide (6), the MIAs 3,4-dehydro-strictosidine (2), strictosidine (3), strictosidinic acid (4), harman-3-carboxylic acid (5), 5-carboxystrictosidine (7), and desoxycordifoline (8) have also been previously reported to exhibit significant antioxidant potential (Cardoso et al., 2004). In the same study, desoxycordifoline (8), reported here as the major compound of the leaf aqueous extract, exhibited significant acetylcholinesterase activity. On the other hand, the flavonoids quercetin 3-O-rhamnopyranoside (9) and kaempferol 3-O-rhamnopyranoside (10) have also demonstrated efficient antioxidant potential (Babujanarthanam et al., 2011; Akter et al., 2022). Moreover, kaempferol 3-O-rhamnopyranoside (10), the second major compound in the leaf aqueous extract, has been identified as a potential therapeutic agent in preventing oxidative stress-induced damage in melanocytes and other biomolecules. It has also been suggested for therapeutic use in chemotherapy and the treatment of vitiligo (Jung et al., 2017; Akter et al., 2022). Additionally, kaempferol 3-O-rhamnopyranoside (10) has shown potential for preventing PM-induced inflammatory skin diseases (Kim et al., 2019).
4 Conclusion
In conclusion, this study demonstrates that untargeted HPLC-MS analysis was a promising approach for identifying unprecedented bioactive compounds in S. peckii. The analysis revealed the presence of eleven compounds, including harman-3-carboxylic acid (5), N,β-glucopyranosyl vincosamide (6), quercetin 3-O-rhamnopyranoside (9), and kaempferol 3-O-rhamnopyranoside (10), which have not been reported in the Loganiaceae family. Along with other three MIAs, these compounds were successfully isolated using modern chromatographic techniques and identified through NMR spectroscopy in combination with MS. Overall, our study highlights the potential of untargeted HPLC-MS analysis as a simple and effective tool for guiding the isolation of unprecedented bioactive compounds from S. peckii.
Data availability statement
The original contributions presented in the study are included in the article/Supplementary Material, further inquiries can be directed to the corresponding author.
Author contributions
Conceived and designed the experiments: CS, MP, and FS. Performed the experiments: CS, KN, FS-F, and BL; analyzed the data: CS, EC, AS, HK, MP, and FS. writing—original draft: CS, HK, and FS. All authors contributed to the article and approved the submitted version.
Funding
We would like to thank Conselho Nacional de Desenvolvimento Científico e Tecnológico Tecnolgico (CNPq), Fundacao de Amparo à Pesquisa do Estado do Amazonas (FAPEAM), and Financiadora de Estudos e Projetos (FINEP) for financial support. This study was financed in part by the Coordenacao de Aperfeiçoamento de Pessoal de Nível Superior—Brasil (CAPES)—Finance Code 001.
Acknowledgments
The authors are grateful to Central Analítica (UFAM) for analysis.
Conflict of interest
The authors HK, FS, and EC declared that they were an editorial board member of Frontiers, at the time of submission. This had no impact on the peer review process and the final decision.
The authors declare that the research was conducted in the absence of any commercial or financial relationships that could be construed as a potential conflict of interest.
Publisher’s note
All claims expressed in this article are solely those of the authors and do not necessarily represent those of their affiliated organizations, or those of the publisher, the editors and the reviewers. Any product that may be evaluated in this article, or claim that may be made by its manufacturer, is not guaranteed or endorsed by the publisher.
Supplementary material
The Supplementary Material for this article can be found online at: https://www.frontiersin.org/articles/10.3389/fntpr.2023.1189619/full#supplementary-material
References
Adebowale, A., Naidoo, Y., Lamb, J., and Nicholas, A. (2014). Comparative foliar epidermal micromorphology of southern african Strychnos L. (Loganiaceae): taxonomic, ecological and cytological considerations. Plant Syst. Evol. 300, 127–138. doi:10.1007/s00606-013-0865-z
Akter, M., Parvin, M. S., Hasan, M. M., Rahman, M. A. A., and Islam, M. E. (2022). Anti-tumor and antioxidant activity of kaempferol-3-O-alpha-L-rhamnoside (Afzelin) isolated from Pithecellobium dulce leaves. BMC Complementary Med. Ther. 22 (1), 169. doi:10.1186/s12906-022-03633-x
Babujanarthanam, R., Kavitha, P., Mahadeva Rao, U. S., and Pandian, M. R. (2011). Quercitrin a bioflavonoid improves the antioxidant status in streptozotocin: induced diabetic rat tissues. Mol. Cell. Biochem. 358, 121–129. doi:10.1007/s11010-011-0927-x
Basílio, I. J. L. D., Nurit, K., and Agra, M. F. (2005). Estudo farmacobotânico das folhas de três espécies do gênero Strychnos L. (Loganiaceae) do Nordeste do Brasil. Acta Farm. Bonaer. 24, 356–365.
Bonamin, F., Moraes, T. M., Kushima, H., Silva, M. A., Rozza, A. L., Pellizzon, C. H., et al. (2011). Can a Strychnos species be used as antiulcer agent? Ulcer healing action from alkaloid fraction of Strychnos pseudoquina st. Hil. (Loganiaceae). J. Ethnopharmacol. 138, 47–52. doi:10.1016/j.jep.2011.08.020
Brandt, V., Tits, M., Geerlings, A., Frédérich, M., Penelle, J., Delaude, C., et al. (1999). β-Carboline glucoalkaloids from Strychnos mellodora. Phytochemistry 51, 1171–1176. doi:10.1016/s0031-9422(99)00129-6
Brasseur, T., and Angenot, L. (1986). Flavonol glycosides from leaves of Strychnos variabilis. Phytochemistry 25, 563–564. doi:10.1016/s0031-9422(00)85534-x
Cardoso, C. L., Castro-Gamboa, I., Silva, D. H. S., Furlan, M., Epifanio, R. A., Pinto, A. C., et al. (2004). Indole glucoalkaloids from Chimarrhis turbinata and their evaluation as antioxidant agents and acetylcholinesterase inhibitors. J. Nat. Prod. 67 (11), 1882–1885. doi:10.1021/np049863m
Guimarães, E. F., Manoel, E. A., Siqueira, C. E., and Zappi, D. (2015). Loganiaceae in Lista de Espécies da Flora do Brasil. Jardim Botânico do Rio de Janeiro: Biotaxa. Available at: http://floradobrasil.jbrj.gov.br/jabot/floradobrasil/FB8663.
Gurst, J. E. (1991). NMR and the structure of D-glucose. J. Chem. Educ. 68, 1003–1004. doi:10.1021/ed068p1003
Henriques, A. T., Lopes, S. O., Paranhos, J. T., Gregianini, T. S., Fett-Neto, A. G., Schripsema, J., et al. (2004). N,β-D-Glucopyranosyl vincosamide, a light regulated indole alkaloid from the shoots of Psychotria leiocarpa. Phytochemistry 65, 449–454. doi:10.1016/j.phytochem.2003.10.027
Jung, E., Kim, J. H., Kim, M. O., Lee, S. Y., and Lee, J. (2017). Melanocyte-protective effect of afzelin is mediated by the Nrf2-ARE signalling pathway via GSK-3β inactivation. Exp. Dermatol. 26 (9), 764–770. doi:10.1111/exd.13277
Kim, J. H., Kim, M., Kim, J. M., Lee, M. K., Seo, S. J., and Park, K. Y. (2019). Afzelin suppresses proinflammatory responses in particulate matter-exposed human keratinocytes. Int. J. Mol. Med. 43 (6), 2516–2522. doi:10.3892/ijmm.2019.4162
Lima, B. R., Silva, F. M. A., Soares, E. R., Almeida, R. A., Silva-Filho, F. A., Barison, A., et al. (2020). Integrative approach based on leaf spray mass spectrometry, HPLC-DAD-MS/MS, and NMR for comprehensive characterization of isoquinoline-derived alkaloids in leaves of Onychopetalum amazonicum RE Fr. J. Braz. Chem. Soc. 31, 79–89. doi:10.21577/0103-5053.20190125
March, R. E., Miao, X. S., and Metcalfe, C. D. (2004). A fragmentation study of a flavone triglycoside, kaempferol-3-O-robinoside-7-O-rhamnoside. Rapid Commun. mass Spectrom. 18, 931–934. doi:10.1002/rcm.1428
Matsuura, H. N., and Fett-Neto, A. G. (2013). The major indole alkaloid N,β-D-glucopyranosyl vincosamide from leaves of Psychotria leiocarpa Cham. & Schltdl. is not an antifeedant but shows broad antioxidant activity. Nat. Prod. Res. 27 (4-5), 402–411. doi:10.1080/14786419.2012.715293
Nicoletti, M., Goulart, M. O. F., Lima, R. A. D., Goulart, A. E., Monache, F. D., and Bettolo, G. B. M. (1984). Flavonoids and alkaloids from Strychnos pseudoquina. J. Nat. Prod. 47, 953–957. doi:10.1021/np50036a007
Ohiri, F. C., Verpoorte, R., and Baerheim, A. S. (1983). The african Strychnos species and their alkaloids: a review. J. Ethnopharmacol. 9, 167–223. doi:10.1016/0378-8741(83)90032-6
Patthy-Lukáts, Á., Károlyházy, L., Szabó, L. F., and Podányi, B. (1997). First direct and detailed stereochemical analysis of strictosidine. J. Nat. Prod. 60, 69–75. doi:10.1021/np960324u
Paz, W. H. P., Oliveira, R. N., Heerdt, G., Angolini, C. F. F., Medeiros, L. S., Silva, V. R., et al. (2019). Structure-based molecular networking for the target discovery of oxahomoaporphine and 8-oxohomoaporphine alkaloids from Duguetia surinamensis. J. Nat. Prod. 82, 2220–2228. doi:10.1021/acs.jnatprod.9b00287
Pereira, M. M., Souza Júnior, S. N., Alcântara, A. F. C., Piló-Veloso, D., Alves, R. B., Machado, P. O., et al. (2006). Constituintes químicos e estudo biológico de Aspidosperma nitidum (Apocynaceae). Rev. Bras. Plantas Med. 8, 1–8.
Philippe, G., Angenot, L., Tits, M., and Frédérich, M. (2004). About the toxicity of some Strychnos species and their alkaloids. Toxicon 44, 405–416. doi:10.1016/j.toxicon.2004.05.006
Quetin-Leclercq, J., Angenot, L., and Bisset, N. G. (1990). South American Strychnos species. Ethnobotany (except curare) and alkaloid screening. J. Ethnopharmacol. 28, 1–52. doi:10.1016/0378-8741(90)90064-z
Rakumitsu, K., Sakamoto, J., and Ishikawa, H. (2019). Total syntheses of (-)-secologanin, (–)-5-carboxystrictosidine, and (–)-rubenine. Chem. A Eur. J. 25, 8996–9000. doi:10.1002/chem.201902073
Resende, J. V. M., de Sá, N. M., de Oliveira, M. T. L., Lopes, R. C., Garrett, R., and Borges, R. M. (2020). Chemical profiling of herbarium samples of solanum (Solanaceae) using mass spectrometry. Phytochem. Lett. 36, 99–105. doi:10.1016/j.phytol.2020.01.021
Santos, C. L. G., Angolini, C. F. F., Neves, K. O. G., Costa, E. V., Souza, A. D. L., Pinheiro, M. L. B., et al. (2019). Molecular networking-based dereplication of strictosidine-derived monoterpene indole alkaloids from the curare ingredient Strychnos peckii. Rapid Commun. Mass Spectrom. 34, e8683. doi:10.1002/rcm.8683
Silva, F. M. A., Hanna, A. C. S., Souza, A. A., Silva-Filho, F. A., Canhoto, O. M. F., Magalhães, A., et al. (2019). Integrative analysis based on HPLC-DAD-MS/MS and NMR of Bertholletia excelsa bark biomass residues: determination of ellagic acid derivatives. J. Braz. Chem. Soc. 30, 830–836. doi:10.21577/0103-5053.20180215
Silva, M. A., Souza-Brito, A. R. M., Hiruma-Lima, C. A., Santos, L. C., Sannomiya, M., and Vilegas, W. (2005). Strychnos L. da América do Sul e Central. Rev. Bras. Farmacogn. 15, 256–267. doi:10.1590/s0102-695x2005000300017
Tchinda, A. T., Tamze, V., Ngono, A. R., Ayimele, G. A., Cao, M., Angenot, L., et al. (2012). Alkaloids from the stem bark of Strychnos icaja. Phytochem. Lett. 5, 108–113. doi:10.1016/j.phytol.2011.11.004
Yuk, H. J., Lee, Y. L., Ryu, H. W., Kim, S. H., and Kim, D. S. (2018). Effects of Toona sinensis leaf extract and its chemical constituents on xanthine oxidase activity and serum uric acid levels in potassium oxonate-induced hyperuricemic rats. Molecules 23, 3254. doi:10.3390/molecules23123254
Keywords: amazon rainforest, dereplication, flavonoid glycosides, monoterpene indole alkaloid, loganiaceae
Citation: Santos CLG, Neves KOG, Silva-Filho FA, Lima BR, Costa EV, Souza ADLd, Koolen HHF, Pinheiro MLB and Silva FMAd (2023) LC-MS guided isolation of N,β-glucopyranosyl vincosamide and other compounds from the curare ingredient Strychnos peckii. Front. Nat. Produc. 2:1189619. doi: 10.3389/fntpr.2023.1189619
Received: 19 March 2023; Accepted: 27 July 2023;
Published: 10 August 2023.
Edited by:
Shihua Wu, Zhejiang University, ChinaReviewed by:
Abdelnaser Abdelghany Elzaawely, Tanta University, EgyptAhmed M. Sayed, Nahda University, Egypt
Copyright © 2023 Santos, Neves, Silva-Filho, Lima, Costa, Souza, Koolen, Pinheiro and Silva. This is an open-access article distributed under the terms of the Creative Commons Attribution License (CC BY). The use, distribution or reproduction in other forums is permitted, provided the original author(s) and the copyright owner(s) are credited and that the original publication in this journal is cited, in accordance with accepted academic practice. No use, distribution or reproduction is permitted which does not comply with these terms.
*Correspondence: Felipe M. A. da Silva, ZmVsaXBlbWFzQHVmYW0uZWR1LmJy