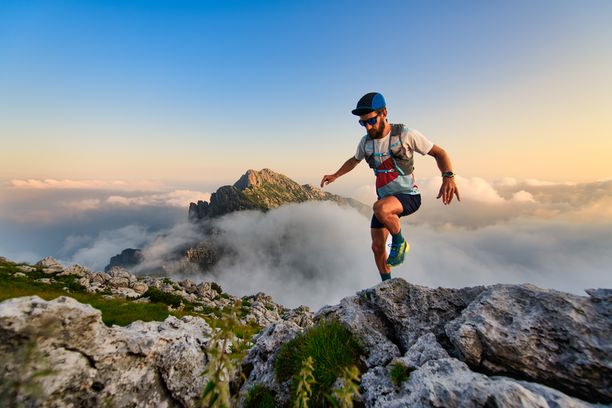
94% of researchers rate our articles as excellent or good
Learn more about the work of our research integrity team to safeguard the quality of each article we publish.
Find out more
REVIEW article
Front. Nanotechnol.
Sec. Nanomaterials
Volume 7 - 2025 | doi: 10.3389/fnano.2025.1549713
This article is part of the Research TopicMyconanotechnology for SustainabilityView all articles
The final, formatted version of the article will be published soon.
Select one of your emails
You have multiple emails registered with Frontiers:
Notify me on publication
Please enter your email address:
If you already have an account, please login
You don't have a Frontiers account ? You can register here
Multimetallic nanoparticles (MMNPs) produced by fungus-mediated synthesis have attracted a lot of interest as an environmentally friendly and sustainable nanotechnology method.Fungi are effective bio-factories that create complex nanoparticles with special qualities by using their metabolic and enzymatic capabilities. When compared to their monometallic counterparts, MMNPs-which are composed of combinations of two or more metals-offer synergistic benefits such increased catalytic activity, higher stability, and superior biocompatibility. In addition to highlighting the structural diversity of MMNPs, such as coreshell, alloy, and Janus configurations, this review investigates the mechanisms underpinning fungal-mediated synthesis, including enzymatic reduction and stabilisation pathways.Additionally covered are characterisation methods for examining functionality, morphology, and composition. The potential applications of MMNPs synthesized by fungi in biomedicine, environmental remediation, biosensing, and catalysis are highlighted in the article. It also outlines future approaches in strain optimisation, genetic engineering, and integration with hybrid systems, while addressing issues like reproducibility, scalability, and control over particle composition. This green synthesis method, which makes use of the natural benefits of fungus and multimetallic systems, responds to the increasing need for sustainable nanomaterials and opens the door to novel uses in both the scientific and industrial fields.
Keywords: Biological nanoparticle synthesis, Catalysis and biomedicine, Eco-friendly nanotechnology, Fungal-mediated synthesis, Green nanomaterials, Multimetallic nanoparticles
Received: 21 Dec 2024; Accepted: 24 Mar 2025.
Copyright: © 2025 Sidhu, Agrawal, Verma, Kaushal and Sharma. This is an open-access article distributed under the terms of the Creative Commons Attribution License (CC BY). The use, distribution or reproduction in other forums is permitted, provided the original author(s) or licensor are credited and that the original publication in this journal is cited, in accordance with accepted academic practice. No use, distribution or reproduction is permitted which does not comply with these terms.
* Correspondence: Madhvi Sharma, Department of Entomology, International Crops Research Institute for the Semi-Arid Tropics (ICRISAT), Hyderabad, 502324, Andhra Pradesh, India
Disclaimer: All claims expressed in this article are solely those of the authors and do not necessarily represent those of their affiliated organizations, or those of the publisher, the editors and the reviewers. Any product that may be evaluated in this article or claim that may be made by its manufacturer is not guaranteed or endorsed by the publisher.
Supplementary Material
Research integrity at Frontiers
Learn more about the work of our research integrity team to safeguard the quality of each article we publish.