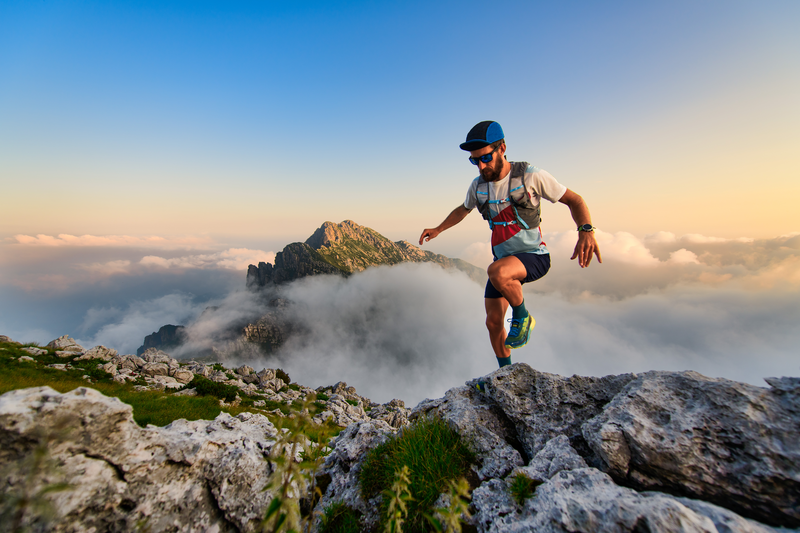
94% of researchers rate our articles as excellent or good
Learn more about the work of our research integrity team to safeguard the quality of each article we publish.
Find out more
MINI REVIEW article
Front. Nanotechnol. , 27 January 2025
Sec. Biomedical Nanotechnology
Volume 6 - 2024 | https://doi.org/10.3389/fnano.2024.1506665
This article is part of the Research Topic The Road toward Nano-Based Diagnostics for Health and Disease View all 7 articles
Various chemical and physical methods have been proposed for the synthesis of nanoparticles (NPs). However, these methods have disadvantages, such as high energy loss and high capital requirements. To overcome these problems, alternative methods for NP synthesis, such as biological or green synthesis, are favoured to overcome these problems. Green synthesis of NPs is environmentally friendly, economical and non-toxic. This review examines the history of green synthesis, focusing on using environmentally friendly methods. The integration of machine learning into NP production and a range of NP applications in healthcare, disease treatment and the environment are also covered.
One of the foundations of the current nanoscience revolution is the semiconductor nanocrystal as a nanomaterial (Kambhampati, 2021). The size of nanoparticles (NPs) is usually smaller than 100 nm (>1 nm) and due to their smaller size, they are chemically stable (Das, 2017; Marslin et al., 2018). The core of nanobiotechnology is the production of NPs and other nanomaterials, which are used in various industries such as electrochemistry, electrical and mechanical engineering, cosmetology, food, pharmaceuticals, medicine and agriculture (Najahi-Missaoui et al., 2020; Khan et al., 2022). In recent years, the U.S. Food and Drug Administration (U.S. FDA) and the European Medicines Agency (EMA) have approved several pharmaceutical companies for the use and development of nanotechnology-based drugs (Halwani, 2022).
Magnetic NPs derived from iron (Fe) are being actively explored for medical diagnosis and therapy, including cancer treatment (Dürr et al., 2013), sorting and manipulation of stem cells (Barrow et al., 2015), drug delivery (Estelrich et al., 2015), genome analysis (Tang et al., 2020) and magnetic resonance imaging (Zhao et al., 2020), and copper (Cu) NPs are used as biosensors and electrochemical sensors (Qing et al., 2019). Many silver (Ag) NPs are commonly used to treat viral and microbial infections (Salleh et al., 2020). Platinum (Pt) NPs have recently been investigated and used for the production of anticancer drugs (Abed et al., 2022).
Nanomaterials are synthesised using two methods, namely, the top-down and the bottom-up approach (Usman et al., 2020). The top-down approach is usually done by physical processes such as vaporisation, lithography, laser ablation, spraying, hydrolysis, photo, irradiation and supersonic. In this method, a larger molecule is broken down into atoms or ions that are much smaller (Gutiérrez-Cruz et al., 2022; Harish et al., 2023). Co-precipitation, photochemistry and reduction are chemical methods that can be used in the bottom-up approach (Wang et al., 2021; Sajid, 2022). The bottom-up method involves using microorganisms plants, algae, bacteria and fungi (Salem and Fouda, 2020; Karunakaran et al., 2023).
This review deals with the green synthesis of nanoparticles through environmentally friendly methods. Different applications of NPs in therapeutics and other fields have also been discussed.
Green synthesis (GS) refers to the biological production of NPs using enzymes from microorganisms or phytochemicals or other sources (plants, algae, fungi, etc.) (Jeevanandam et al., 2022; Sharma et al., 2022). GS is a safe, economical, environmentally friendly and clean method of producing nanomaterials. It uses plants and other biological materials such as bacteria, fungi and algae as substrates (Huston et al., 2021; Dikshit et al., 2021). Many variables can affect the nature and size of NPs, depending on the method and component used for production, including temperature, particle size, pH, extract concentration, reaction time, solvent used and surface charge (Lombardo et al., 2020; Shafey, 2020; Usman et al., 2020). Another important parameter for characterising NPs is the type and intensity of the surface charge, which influences the electrostatic interactions between NPs and their environment (Modena et al., 2019). The final shape and size of NPs are determined by various active molecules and precursors, including a metal salt (Dikshit et al., 2021). Phytochemicals (amides, terpenoids, carboxylic acid, ascorbic acid, etc.) can convert metal salts into metal NPs. These potent phytochemicals present in the extracts are the focus of extensive biodiversity research for green production of NPs (Md Ishak et al., 2019).
Various microorganisms - from bacteria, fungi and actinomycetes to viruses - have been tested for their suitability for the synthesis of NPs. They are used for biocorrosion, bioremediation, biomineralization, bioleaching and other applications in different industries (Dikshit et al., 2021). Microbial synthesis is favoured due to the numerous advantages it offers. They also have advantages such as scaling up the process and other processes associated with synthesis (bottom-up or top-down approaches) (Dikshit et al., 2021) (Supplementary Table 1A).
In contrast to plants, algae (microalgae and macroalgae) do not require any special additional chemicals (Mukherjee et al., 2021). The production of NPs using algae is referred to as phyco-nanotechnology (Chaudhary et al., 2020). Algae contain a variety of phytochemicals, proteins and pigments that are ideally suited as bioproducts for the production of NPs. Algae have shorter growth times (high growth rate), are easier to process, less expensive, require fewer nutrients and are non-toxic. The production of NPs in the solution containing algal extract and metal solution is indicated by the change in colour. The colour change indicates that the metal ions are in a zero-valent state. In the production of gold (Au) NPs with Ulva intestinalis and Rhizoclonium fontinales, there was a visible colour change from green to purple in the thallus (Chaudhary et al., 2020). Similarly, the colour of the cultured mixture of algal biomass of Clavulina humicola together with Ag nitrate salts changed to yellow, indicating the formation of AgNPs (Chan et al., 2022). Many other algal species such as C. humicola, Padina boergesenii, Gracilaria corticate, Anabaena doliolum and Spirulina were used to produce NPs (Supplementary Table 1B).
Plant viruses are preferred for the GS of NPs to minimise the risk of the virus interacting with human proteins and causing toxic side effects, infections and immune responses (van Kan-Davelaar et al., 2014). Plant viruses are also ideal because they can self-assemble around a nanoparticle in vitro and contain about 10 nm³ of particles (Alemzadeh et al., 2018; Zhang et al., 2018). These properties are mainly used to produce plant-derived viral NPs that are used for targeted cancer treatment (Zhang et al., 2018; Venkataraman and Hefferon, 2021).
Plant viruses can also be used as size-limiting vessels for the production of NPs. Different sizes of co-particles produce virus-like particles with different properties and distinct symmetries. Particles composed of smaller NP cores are smaller than those composed of large cores (Liu et al., 2021). The process for producing plant viral NPs involves infecting the plant leaf with the plant virus. There are several forms of the viral capsid and it can be divided into different subunits such as the inner, middle interface and outer. This enables numerous applications for a single viral NP (Chung et al., 2020; Steinmetz et al., 2020; Nooraei et al., 2021).
Viral NPs have protein monomers and encapsulate negatively charged nucleic acids. This approach has been used to encapsulate complexes of negatively charged polymers and cytotoxic drugs such as doxorubicin so that the viral NPs can be used for targeted drug delivery systems (Krissanaprasit et al., 2021; Li and Champion, 2022). Encapsulation of these synthetic NPs in viral NPs ensures biocompatibility, prevents aggregation and enables bioconjugation of functional ligands, such as targeting molecules, to achieve tissue specificity (Färkkilä et al., 2021; Tufani et al., 2021). Bio-templating is a process that mimics the process of bio-mineralisation, i.e., the formation of minerals through the metabolic activities of microorganisms (Homaeigohar, 2020; Magdum et al., 2024). Protein cages for inorganic nanoparticles: The exterior and interior of the Cowpea chlorotic mottle virus capsule are chemically different environments (Kumari et al., 2021). The inner surface is more positively charged than the outer surface so it can serve as a nucleation site for crystal growth. The inner cavity of the virion restricts mineral growth. Therefore, a spherical nanoparticle with a maximum diameter of about 28 nm is formed (Chakravarty and Rao, 2023).
The simplest green production method for the production of metal and metal oxide NPs is the use of plant extracts (Jeevanandam et al., 2022). Various metabolites or chemical substances can be found in plant extracts. They can act as stabilisers that enable the biodegradation of metal ions to NPs. For example, to produce gold NPs, a metal salt solution such as HAuCl4 and AgNO3 is added to the plant extract, and amino groups (-NH3) help to degrade the metal ions from the salt solution (Bharadwaj et al., 2021). The activation phase, the growth phase and the process completion phase are all involved in the synthesis of NPs with plant extracts. In the activation phase, the reduction of metal ions takes place. As a result, reduced metal atoms are formed, followed by a phase change of the nucleated metal atom to the metal ion. The newly formed metal ion grows and is further reduced in the subsequent growth phase. Plant tissues from Ocimum sanctum, Desmodium trifolium, Cinnamomum zeylanicum, Piper longum, Syzygium cumini and Melia azedarach have been used to reduce Ag ions to particle sizes of 5–40 nm (Gour and Jain, 2019). According to Yadi et al. (2018), extracts from Calotropis procera and Punica granatum are mixed with Cu acetate to produce CuONP. The plant extracts can calcify Cu acetate to CuNPs (Supplementary Table 1B).
The development and synthesis of NPs using in vitro approaches is still in its infancy. Only a few reports have demonstrated the use of in vitro-based synthesis of NPs (Satyavani et al., 2011; Iyer et al., 2016; Jayappa et al., 2020). Satyavani et al. (2011) and Iyer et al. (2016) synthesised 31 and 1 nm AgNPs from stem-derived calli extracts of Citrullus colocynthis (L.) Schrad. (Cucurbitaceae) and calli culture of Vigna radiata (L.). R. Wilczek (Fabaceae) using Murashige and Skoog (MS) medium supplemented with different plant growth regulators. Zinc oxide (ZnO) NPs (5–20 nm) were synthesised by Jayappa et al. (2020) using the leaf callus of Mussaenda frondosa L. cultured on MS medium with NAA 2 mg/L and kinetin (kin) 4 mg/L. Therefore, the in vitro approaches for the synthesis of NPs need to be explored as they could be a potential method for the rapid and efficient generation of NPs.
Common techniques used to characterise NPs are surface-enhanced Raman spectroscopy (SERS), X-ray diffraction (XRD), X-ray photoelectron spectroscopy (XPS), energy dispersive spectroscopy (EDS) and ultraviolet (UV)-visible spectroscopy, Fourier transform infrared spectroscopy (FTIR), atomic force microscopy (AFM), atomic absorption spectroscopy (AAS), dynamic light scattering (DLS), scanning and transmission electron microscopy (SEM and TEM) and high angle dark field (HAADF) (Ingham, 2015; Ealias and Saravanakumar, 2017; Sharma et al., 2022; Vijayaram et al., 2024).
The GS of NPs depends on various parameters, such as the optimisation of the chemical processes involved in the synthesis, the required precision and their production. These steps are usually very expensive, labour-intensive, dependent and time-consuming. The synthesis processes also require control of reagent concentrations, temperature, mixing conditions and the reactor (Tao et al., 2021; Nathanael et al., 2023). Varying the type, amount and concentration of stabilising agents could lead to a change in the size of the NPs to be synthesised (Tao et al., 2021; Nathanael et al., 2023). Due to the progress and need for data collection and processing, artificial intelligence and machine learning have been integrated with nanotechnology in the synthesis and prediction of characteristic features of NPs (Shafaei and Khayati, 2020; Mekki-Berrada et al., 2021; Nathanael et al., 2023).
Various factors influence or determine the synthesis of NPs, including features, characterisation, application, types and concentrations of stabilisers, ionic stabilisers (citrate) and pH (Patra and Baek, 2015; Nathanael et al., 2023). Some of the other important parameters are the concentrations of the extracts and raw materials used, the pH of the solution, the temperature, the size of the raw materials and the process of synthesising the NPs (Patra and Baek, 2015).
Process of synthesis: There are different types of NP synthesis, namely, physical (arc discharge, mechanical grinding, electron beam lithography, etc.), chemical (chemical reduction of metals, electrolysis, microemulsion, etc.) and biological (with plant extracts, algae, enzymes and biomolecules, etc.). However, biological approaches to synthesise NPs are non-toxic and use environmentally friendly materials and are therefore environmentally friendly, efficient and more acceptable than conventional methods (Patra and Baek, 2014).
pH: Maintaining pH is an essential factor as it may be involved in the synthesis of NPs. The pH plays an important role in living organisms as it influences the basic processes and therefore requires optimal conditions for better functioning (Patra and Baek, 2014).
Temperature and pressure: It is also an important factor in determining NP synthesis. It can be observed that physical methods require about >350°C, while chemical methods require about 350°C or less. However, biological methods require lower ambient temperatures (<100°C). The temperature of the medium is crucial as it helps to determine the nature of the NPs (Patra and Baek, 2014).
Time: Time is one of the most important factors in the synthesis of NPs, as it controls the formation of NPs (quality and type), which depends on the time allowed for the reaction and incubation time. The time allowed for storage can influence the shelf life of the NPs and the aggregation of the NPs.
Biomolecules and functional groups: The functional groups (thiols, amines, sulphides, phosphines, carboxylic acids) have a major influence on the nature of NPs to be synthesised and can change the surface coating of the NPs. Therefore, the immobilisation of the groups will lead to the synthesis of the desired NPs (Shreyash et al., 2021).
Other factors: Plants and microorganisms produce secondary metabolites that serve as reducing and stabilising agents for the synthesis of NPs. Particle size, pore size and shape play an important role in determining the properties of NPs. It has been found that the melting point of NPs decreases with increasing particle size in the nanometre range (Akbari et al., 2011). Similarly, the light conditions (quality, exposure time and intensity) can alter the synthesis of NPs.
There are various applications of NPs in different fields and sectors such as agriculture, environment, targeted drug delivery, cancer treatment, food and beverage industry and paints, etc. Some of these applications are described below.
Cancer treatment: Cancer is one of the biggest global challenges. Due to their anti-proliferative and cell death-inducing properties, NPs (AgNPs, AuNPs, etc.) are very actively used in the treatment of cancer. As they are small particles, they are easily circulated, bioavailable, soluble, and non-toxic and are distributed and transported by capillaries (Dikshit et al., 2021; Shreyash et al., 2021; Ying et al., 2022).
Targeted drug delivery: The use of nanoparticles as effective drug delivery systems is of great importance due to their size, bioavailability, continuous drug release, significant drug delivery capacity, prolonged circulation time, effective intracellular penetration and ability to protect the active ingredient from physiological barriers. The efficacy of cancer treatment can be improved by dynamically focusing drugs at preferred sites in vivo (Khatik, 2022). Biomaterials (growth factors with living cells) or bioinks based (quantum dots, polymeric micelles, gelatin methacrylate, etc.) on NPs have potential applications in tissue engineering, regeneration and treatment through artificial techniques such as human organs (Salahshour et al., 2024). Similarly, nanocomposites (microbial- and plant-based gums) can also be used for different therapeutic purposes (cancer, water treatment, etc.) (Dhanda et al., 2025).
Anti-microbial properties: GS-NPs are effective against a range of diseases caused by pathogens such as fungi, viruses and bacteria. They can reduce the likelihood of antibiotic resistance and prevent infectious diseases. This creates new opportunities for the improvement of environmentally friendly nanobased antimicrobials (Vanlalveni et al., 2021). AgNPs have been reported to be excellent against multidrug-resistant strains of Pseudomonas aeruginosa, Bacillus subtilis, Staphylococcus aureus and Escherichia coli (Roy et al., 2019).
Agriculture and environment: In agriculture, the use of nano-fertilisers has recently increased and attracted great interest (Dikshit et al., 2021). However, biofertilisers (TiO2, SiO2 including others) are preferred over fertilisers and the former can also be applied to plants using nanocarriers (Kumar et al., 2021; Zafar et al., 2024), taking advantage of the benefits of nanotechnology. The NPs are also used in bioremediation (metallic NPs), water purification (or wastewater treatment) (MgO, TiO2 and ZnO), soil purification and detection of heavy metal NPs (arsenic, chromium, mercury) (Dikshit et al., 2021).
Food preservation, packaging, supplements and value addition: NPs (Ag-Cu, Au, Pt) are used in the food industry for the production, storage, packaging and transport of products. They increase shelf life, reduce the risk of microbial growth (nanocomposite of starch and sorbic acid), increase the absorption of vitamins, and vitamin-containing sprays with nanodroplets (Fe, Zn) as nano-nutrients and develop nanoparticles for smart packaging to trap oxygen and moisture absorbers (Dikshit et al., 2021).
Renewable energy: Renewable energy is another area where green synthesised NPs are very promising (Samuel et al., 2022). Nanoparticles are used to improve the efficiency and performance of solar cells, photocatalysts and energy storage devices. For example, green synthesised ZnO nanoparticles produced from papaya leaf extracts have been employed to improve the ability of solar cells to store light, resulting in higher energy conversion efficiency (Rathnasamy et al., 2017; Ali et al., 2023).
The common problem encountered in the NPs synthesis is the ability to control the size, regularity and shape of the particles (Shreyash et al., 2021).
Consistency: The use of plant extracts or plant calli can influence NP synthesis. The growth conditions such as the pH value and temperature of the plants used in the GS also affect the consistency of the synthesis of the nanoparticles. For example, the absorption peaks of plant extracts and calli in Ag nitrate solution for the AgNP production differ significantly, resulting in different properties (Ying et al., 2022).
Synthesis pathway: The basic technique involves the use of solutions of biological components (microbes, plants or their extracts) mixed with metal salts such that metals get reduced to produce NPs. As a result, the GS technique is in most cases very arbitrary and without any control over product quality (Pal et al., 2022).
Size and shape: During the manufacturing process, there is limited control over the shape and dimensions of the desired end product. The final dimensions of the NPs produced also depend on the starting solutions and stabilising agents used (Baig et al., 2021; Huston et al., 2021; Pal et al., 2022).
Commercial production: Green NPs are produced according to need and application, so the desired size also varies according to use thus limiting the scope for commercial purposes (Gupta et al., 2023).
Reaction time (RT): The RT varies depending on the biochemicals and biosynthesis pathways used. It can be shorter or longer, which affects the properties of the end product (Alharbi et al., 2022; Ying et al., 2022).
Stability: The stability of the NPs produced by GS is impaired. The biological materials may also contain impurities in the form of free radicals that affect their stability (Gupta et al., 2023). There is a need for standardisation of GS processes as batch-to-batch variations are observed in GS (Rogers and Jensen, 2019).
Green synthesis enables efficient use of resources and cost efficiency. The nanoparticles produced by GS are highly biocompatible and can therefore be used safely as nanomedicines. Despite its importance, GS technology is subject to limitations in terms of scalability, reproducibility and standardisation. In conclusion, green synthesis is an important technique for the environment-friendly production of nanoparticles and represents a promising direction for sustainable nanotechnology.
AP: Conceptualization, Data curation, Resources, Writing–original draft, Writing–review and editing. AR: Data curation, Resources, Writing–original draft, Writing–review and editing. SG: Resources, Supervision, Validation, Writing–review and editing. SS: Conceptualization, Formal Analysis, Funding acquisition, Supervision, Validation, Visualization, Writing–review and editing.
The author(s) declare that no financial support was received for the research, authorship, and/or publication of this article.
The authors declare that the research was conducted in the absence of any commercial or financial relationships that could be construed as a potential conflict of interest.
The author(s) declare that no Generative AI was used in the creation of this manuscript.
All claims expressed in this article are solely those of the authors and do not necessarily represent those of their affiliated organizations, or those of the publisher, the editors and the reviewers. Any product that may be evaluated in this article, or claim that may be made by its manufacturer, is not guaranteed or endorsed by the publisher.
The Supplementary Material for this article can be found online at: https://www.frontiersin.org/articles/10.3389/fnano.2024.1506665/full#supplementary-material
Abed, A., Derakhshan, M., Karimi, M., Shirazinia, M., Mahjoubin-Tehran, M., Homayonfal, M., et al. (2022). Platinum nanoparticles in biomedicine: preparation, anti-cancer activity, and drug delivery vehicles. Front. Pharmacol. 13, 797804. doi:10.3389/fphar.2022.797804
Akbari, B., Tavandashti, M. P., and Zandrahimi, M. (2011). Particle size characterization of nanoparticles–a practical approach. Iran. J. Mat. Sci. Eng. 8 (2), 48–56.
Alemzadeh, E., Dehshahri, A., Izadpanah, K., and Ahmadi, F. (2018). Plant virus nanoparticles: novel and robust nanocarriers for drug delivery and imaging. Colloids Surf. B Biointerfaces 167, 20–27. doi:10.1016/j.colsurfb.2018.03.026
Alharbi, N. S., Alsubhi, N. S., and Felimban, A. I. (2022). Green synthesis of silver nanoparticles using medicinal plants: characterization and application. J. Radiat. Res. Appl. Sci. 15 (3), 109–124. doi:10.1016/j.jrras.2022.06.012
Ali, J., Bibi, S., Jatoi, W. B., Tuzen, M., Jakhrani, M. A., Feng, X., et al. (2023). Green synthesized zinc oxide nanostructures and their applications in dye-sensitized solar cells and photocatalysis: a review. Mater. Today Commun. 36, 106840. doi:10.1016/j.mtcomm.2023.106840
Baig, N., Kammakakam, I., and Falath, W. (2021). Nanomaterials: a review of synthesis methods, properties, recent progress, and challenges. Mater. Adv. 2 (6), 1821–1871. doi:10.1039/d0ma00807a
Barrow, M., Taylor, A., Murray, P., Rosseinsky, M. J., and Adams, D. J. (2015). Design considerations for the synthesis of polymer coated iron oxide nanoparticles for stem cell labelling and tracking using MRI. Chem. Soc. Rev. 44 (19), 6733–6748. doi:10.1039/c5cs00331h
Bharadwaj, K. K., Rabha, B., Pati, S., Sarkar, T., Choudhury, B. K., Barman, A., et al. (2021). Green synthesis of gold nanoparticles using plant extracts as beneficial prospect for cancer theranostics. Molecules 26 (21), 6389. doi:10.3390/molecules26216389
Chakravarty, A., and Rao, A. L. N. (2023). Modulation of capsid dynamics in bromoviruses by the host and heterologous viral replicase. J. Virol. 97 (3), e0128422. doi:10.1128/jvi.01284-22
Chan, S. S., Low, S. S., Chew, K. W., Ling, T. C., Rinklebe, J., Juan, J. C., et al. (2022). Prospects and environmental sustainability of phyconanotechnology: a review on algae-mediated metal nanoparticles synthesis and mechanism. Environ. Res. 212, 113140. doi:10.1016/j.envres.2022.113140
Chaudhary, R., Nawaz, K., Khan, A. K., Hano, C., Abbasi, B. H., and Anjum, S. (2020). An overview of the algae-mediated biosynthesis of nanoparticles and their biomedical applications. Biomolecules 10 (11), 1498. doi:10.3390/biom10111498
Chung, Y. H., Cai, H., and Steinmetz, N. F. (2020). Viral nanoparticles for drug delivery, imaging, immunotherapy, and theranostic applications. Adv. Drug Deliv. Rev. 156, 214–235. doi:10.1016/j.addr.2020.06.024
Das, S. K. (2017). Nanoparticles advanced characterization techniques: a view point. J. Atoms Mol. 7 (4), 1091–1098.
Dhanda, G., Manjhani, A., Miglani, N., Ranjan, S., Prakash, A., Verma, A., et al. (2025). “Antimicrobial gum: a bio-based nanocomposite,” in Antimicrobial materials and coatings. Woodhead publishing in materials. Editors A. K. Singh, M. Dhayal, and C. H. Hussain (Elsevier), 4–24. doi:10.1016/B978-0-323-95460-0.00001-0
Dikshit, P. K., Kumar, J., Das, A. K., Sadhu, S., Sharma, S., Singh, S., et al. (2021). Green synthesis of metallic nanoparticles: applications and limitations. Catalysts 11 (8), 902. doi:10.3390/catal11080902
Dürr, S., Janko, C., Lyer, S., Tripal, P., Schwarz, M., Zaloga, J., et al. (2013). Magnetic nanoparticles for cancer therapy. Nanotechnol. Rev. 2 (4), 395–409. doi:10.1515/ntrev-2013-0011
Ealias, A. M., and Saravanakumar, M. P. (2017). “A review on the classification, characterisation, synthesis of nanoparticles and their application,” in IOP conference series: materials science and engineering (IOP Publishing), 263 (3), 032019. doi:10.1088/1757-899X/263/3/032019
Estelrich, J., Escribano, E., Queralt, J., and Busquets, M. A. (2015). Iron oxide nanoparticles for magnetically-guided and magnetically-responsive drug delivery. Int. J. Mol. Sci. 16 (4), 8070–8101. doi:10.3390/ijms16048070
Färkkilä, S. M., Kiers, E. T., Jaaniso, R., Mäeorg, U., Leblanc, R. M., Treseder, K. K., et al. (2021). Fluorescent nanoparticles as tools in ecology and physiology. Biol. Rev. 96 (5), 2392–2424. doi:10.1111/brv.12758
Gour, A., and Jain, N. K. (2019). Advances in green synthesis of nanoparticles. Art. cells Nanomed Biotechnol. 47 (1), 844–851. doi:10.1080/21691401.2019.1577878
Gupta, D., Boora, A., Thakur, A., and Gupta, T. K. (2023). Green and sustainable synthesis of nanomaterials: recent advancements and limitations. Environ. Res. 231, 116316. doi:10.1016/j.envres.2023.116316
Gutiérrez-Cruz, A., Ruiz-Hernández, A. R., Vega-Clemente, J. F., Luna-Gazcón, D. G., and Campos-Delgado, J. (2022). A review of top-down and bottom-up synthesis methods for the production of graphene, graphene oxide and reduced graphene oxide. J. Mater. Sci. 57 (31), 14543–14578. doi:10.1007/s10853-022-07514-z
Halwani, A. A. (2022). Development of pharmaceutical nanomedicines: from the bench to the market. Pharmaceutics 14 (1), 106. doi:10.3390/pharmaceutics14010106
Harish, V., Ansari, M. M., Tewari, D., Yadav, A. B., Sharma, N., Bawarig, S., et al. (2023). Cutting-edge advances in tailoring size, shape, and functionality of nanoparticles and nanostructures: a review. JTaiwan Inst. Chem. Eng. 149, 105010. doi:10.1016/j.jtice.2023.105010
Homaeigohar, S. (2020). The nanosized dye adsorbents for water treatment. Nanomaterials 10, 295. doi:10.3390/nano10020295
Huston, M., DeBella, M., DiBella, M., and Gupta, A. (2021). Green synthesis of nanomaterials. Nanomaterials 11 (8), 2130. doi:10.3390/nano11082130
Ingham, B. (2015). X-ray scattering characterisation of nanoparticles. Crystallogr. Rev. 21 (4), 229–303. doi:10.1080/0889311x.2015.1024114
Iyer, R. I., Selvaraju, C., and Santhiya, S. T. (2016). Biosynthesis of silver nanoparticles by callus cultures of Vigna radiata. Ind. J. Sci. Technol. 9 (9), 1–5. doi:10.17485/ijst/2016/v9i9/87987
Jayappa, M. D., Ramaiah, C. K., Kumar, M. A. P., Suresh, D., Prabhu, A., Devasya, R. P., et al. (2020). Green synthesis of zinc oxide nanoparticles from the leaf, stem and in vitro grown callus of Mussaenda frondosa L.: characterization and their applications. Appl. Nanosci. 10, 3057–3074. doi:10.1007/s13204-020-01382-2
Jeevanandam, J., Kiew, S. F., Boakye-Ansah, S., Lau, S. Y., Barhoum, A., Danquah, M. K., et al. (2022). Green approaches for the synthesis of metal and metal oxide nanoparticles using microbial and plant extracts. Nanoscale 14 (7), 2534–2571. doi:10.1039/d1nr08144f
Kambhampati, P. (2021). Nanoparticles, nanocrystals, and quantum dots: what are the implications of size in colloidal nanoscale materials? J. Phys. Chem. Lett. 12 (20), 4769–4779. doi:10.1021/acs.jpclett.1c00754
Karunakaran, G., Sudha, K. G., Ali, S., and Cho, E. B. (2023). Biosynthesis of nanoparticles from various biological sources and its biomedical applications. Molecules 28 (11), 4527. doi:10.3390/molecules28114527
Khan, Y., Sadia, H., Ali Shah, S. Z., Khan, M. N., Shah, A. A., and Ullah, N. A. (2022). Classification, synthetic, and characterization approaches to nanoparticles, and their applications in various fields of nanotechnology: A review. Catalysts 12 (1386), 1–27. doi:10.3390/catal12111386
Khatik, N. (2022). Green synthesis of nanomaterials and their utilization as potential vehicles for targeted cancer drug delivery. Adv. Pharmacol. Pharm. 10 (2), 114–121. doi:10.13189/app.2022.100205
Krissanaprasit, A., Key, C. M., Pontula, S., and LaBean, T. H. (2021). Self-assembling nucleic acid nanostructures functionalized with aptamers. Chem. Rev. 121 (22), 13797–13868. doi:10.1021/acs.chemrev.0c01332
Kumari, V., Sharma, M. M., Mishra, R., and Singh, A. (2021). “Biocompatible viruses for nanoparticle and targeted drug delivery,” in Emerging technologies; Micro To Nano, Proceedings of ETMN-2021, Jaipur, India, October 8–9, Editor A. S. Shahbaz, S. Kulwant, L. Ahmed, L. G. Ford, and N. S. Niti (New York, United States: AIP Publishing) 2752, 020003. doi:10.1063/5.0136116
Kumar J, J., Ramlal, A., Mallick, D., and Mishra, V. (2021). An overview of some biopesticides and their importance in plant protection for commercial acceptance. Plants 10 (6), 1185. doi:10.3390/plants10061185
Li, Y., and Champion, J. A. (2022). Self-assembling nanocarriers from engineered proteins: design, functionalization, and application for drug delivery. Adv. Drug Deliv. Rev. 189, 114462. doi:10.1016/j.addr.2022.114462
Liu, Q., Shaukat, A., Kyllönen, D., and Kostiainen, M. A. (2021). Polyelectrolyte encapsulation and confinement within protein cage-inspired Nanocompartments. Pharmaceutics 13 (10), 1551. doi:10.3390/pharmaceutics13101551
Lombardo, D., Calandra, P., Pasqua, L., and Magazù, S. (2020). Self-assembly of organic nanomaterials and biomaterials: the bottom-up approach for functional nanostructures formation and advanced applications. Materials 13 (5), 1048. doi:10.3390/ma13051048
Magdum, A. B., Waghmode, R. S., Shinde, K. V., Mane, M. P., Kamble, M. V., Kamble, R. S., et al. (2024). Biogenic synthesis of silver nanoparticles from leaves extract of Decaschistia trilobata an endemic shrub and its application as antioxidant, antibacterial, anti-inflammatory and dye reduction. Catal. Commun. 187, 106865. doi:10.1016/j.catcom.2024.106865
Marslin, G., Siram, K., Maqbool, Q., Selvakesavan, R. K., Kruszka, D., Kachlicki, P., et al. (2018). Secondary metabolites in the green synthesis of metallic nanoparticles. Materials 11 (6), 940. doi:10.3390/ma11060940
Md Ishak, N. A. I., Kamarudin, S. K., and Timmiati, S. N. (2019). Green synthesis of metal and metal oxide nanoparticles via plant extracts: an overview. Mater. Res. Express 6 (11), 112004. doi:10.1088/2053-1591/ab4458
Mekki-Berrada, F., Ren, Z., Huang, T., Wong, W. K., Zheng, F., Xie, J., et al. (2021). Two-step machine learning enables optimized nanoparticle synthesis. npj Comput. Mater 7 (1), 55. doi:10.1038/s41524-021-00520-w
Modena, M. M., Rühle, B., Burg, T. P., and Wuttke, S. (2019). Nanoparticle characterization: what to measure? Adv. Mater. 31 (32), 1901556. doi:10.1002/adma.201901556
Mukherjee, A., Sarkar, D., and Sasmal, S. (2021). A review of green synthesis of metal nanoparticles using algae. Front. Microbiol. 12, 693899. doi:10.3389/fmicb.2021.693899
Najahi-Missaoui, W., Arnold, R. D., and Cummings, B. S. (2020). Safe nanoparticles: are we there yet? Int. J. Mol. Sci. 22 (1), 385–406. doi:10.3390/ijms22010385
Nathanael, K., Cheng, S., Kovalchuk, N. M., Arcucci, R., and Simmons, M. J. (2023). Optimization of microfluidic synthesis of silver nanoparticles: a generic approach using machine learning. Chem. Engin Res. Des. 193, 65–74. doi:10.1016/j.cherd.2023.03.007
Nooraei, S., Bahrulolum, H., Hoseini, Z. S., Katalani, C., Hajizade, A., Easton, A. J., et al. (2021). Virus-like particles: preparation, immunogenicity and their roles as nanovaccines and drug nanocarriers. J. Nanobiotechnol 19, 59–27. doi:10.1186/s12951-021-00806-7
Pal, K., Chakroborty, S., and Nath, N. (2022). Limitations of nanomaterials insights in green chemistry sustainable route: review on novel applications. Green Process Syn. 11 (1), 951–964. doi:10.1515/gps-2022-0081
Patra, J. K., and Baek, K. H. (2014). Green nanobiotechnology: factors affecting synthesis and characterization techniques. J. Nanomater. 2014 (1), 417305. doi:10.1155/2014/417305
Patra, J. K., and Baek, K. H. (2015). Green nanobiotechnology: factors affecting synthesis and characterization techniques. J. Nanomater. 2014, 219. doi:10.1155/2014/417305
Qing, Z., Bai, A., Xing, S., Zou, Z., He, X., Wang, K., et al. (2019). Progress in biosensor based on DNA-templated copper nanoparticles. Biosens. Bioelectron. 137, 96–109. doi:10.1016/j.bios.2019.05.014
Rathnasamy, R., Thangasamy, P., Thangamuthu, R., Sampath, S., and Alagan, V. (2017). Green synthesis of ZnO nanoparticles using Carica papaya leaf extracts for photocatalytic and photovoltaic applications. J. Mater Sci. Mater Electron 28, 10374–10381. doi:10.1007/s10854-017-6807-8
Rogers, L., and Jensen, K. F. (2019). Continuous manufacturing–the green chemistry promise? Green Chem. 21 (13), 3481–3498. doi:10.1039/c9gc00773c
Roy, A., Bulut, O., Some, S., Mandal, A. K., and Yilmaz, M. D. (2019). Green synthesis of silver nanoparticles: biomolecule-nanoparticle organizations targeting antimicrobial activity. RSC Adv. 9 (5), 2673–2702. doi:10.1039/c8ra08982e
Sajid, M. (2022). Nanomaterials: types, properties, recent advances, and toxicity concerns. Curr. Opin. Environ. Sci. Health 25, 100319. doi:10.1016/j.coesh.2021.100319
Salahshour, P., Abdolmaleki, S., Monemizadeh, S., Gholizadeh, S., and Khaksar, S. (2024). Nanobiomaterials/bioinks based scaffolds in 3d bioprinting for tissue engineering and artificial human organs. Adv. Biol. Earth Sci. 9, 97–104. doi:10.62476/abes9s97
Salem, S. S., and Fouda, A. (2020). Green synthesis of metallic nanoparticles and their prospective biotechnological applications: an overview. Biol. Trace Elem. Res. 199, 344–370. doi:10.1007/s12011-020-02138-3
Salleh, A., Naomi, R., Utami, N. D., Mohammad, A. W., Mahmoudi, E., Mustafa, N., et al. (2020). The potential of silver nanoparticles for antiviral and antibacterial applications: a mechanism of action. Nanomaterials 10 (8), 1566. doi:10.3390/nano10081566
Samuel, M. S., Ravikumar, M., John J, A., Selvarajan, E., Patel, H., Chander, P. S., et al. (2022). A review on green synthesis of nanoparticles and their diverse biomedical and environmental applications. Catalysts 12 (5), 459. doi:10.3390/catal12050459
Satyavani, K., Gurudeeban, S., Ramanathan, T., and Balasubramanian, T. (2011). Biomedical potential of silver nanoparticles synthesized from calli cells of Citrullus colocynthis (L.) Schrad. J. Nanobiotechnol 9, 43. doi:10.1186/1477-3155-9-43
Shafaei, A., and Khayati, G. R. (2020). A predictive model on size of silver nanoparticles prepared by green synthesis method using hybrid artificial neural network-particle swarm optimization algorithm. Measurement 151, 107199. doi:10.1016/j.measurement.2019.107199
Shafey, A. M.El. (2020). Green synthesis of metal and metal oxide nanoparticles from plant leaf extracts and their applications: a review. Green Process. Synthesis 9 (1), 304–339. doi:10.1515/gps-2020-0031
Sharma, N. K., Vishwakarma, J., Rai, S., Alomar, T. S., AlMasoud, N., and Bhattarai, A. (2022). Green route synthesis and characterization techniques of silver nanoparticles and their biological adeptness. ACS Omega 7 (31), 27004–27020. doi:10.1021/acsomega.2c01400
Shreyash, N., Bajpai, S., Khan, M. A., Vijay, Y., Tiwary, S. K., and Sonker, M. (2021). Green synthesis of nanoparticles and their biomedical applications: a review. ACS Appl. Nano Mater. 4 (11), 11428–11457. doi:10.1021/acsanm.1c02946
Steinmetz, N. F., Lim, S., and Sainsbury, F. (2020). Protein cages and virus-like particles: from fundamental insight to biomimetic therapeutics. Biomater. Sci. 8 (10), 2771–2777. doi:10.1039/d0bm00159g
Tang, C., He, Z., Liu, H., Xu, Y., Huang, H., Yang, G., et al. (2020). Application of magnetic nanoparticles in nucleic acid detection. J. Nanobiotechnol 18, 62–19. doi:10.1186/s12951-020-00613-6
Tao, H., Wu, T., Aldeghi, M., Wu, T. C., Aspuru-Guzik, A., and Kumacheva, E. (2021). Nanoparticle synthesis assisted by machine learning. Nat. Rev. Mater. 6 (8), 701–716. doi:10.1038/s41578-021-00337-5
Tufani, A., Qureshi, A., and Niazi, J. H. (2021). Iron oxide nanoparticles based magnetic luminescent quantum dots (MQDs) synthesis and biomedical/biological applications: a review. Mater Sci. Eng. C 118, 111545. doi:10.1016/j.msec.2020.111545
Usman, K. A. S., Maina, J. W., Seyedin, S., Conato, M. T., Payawan Jr, L. M., Dumée, L. F., et al. (2020). Downsizing metal–organic frameworks by bottom-up and top-down methods. NPG Asia Mater. 12 (1), 58. doi:10.1038/s41427-020-00240-5
van Kan-Davelaar, H. E., Van Hest, J. C. M., Cornelissen, J. J. L. M., and Koay, M. S. T. (2014). Using viruses as nanomedicines. Brit J. Pharmacol. 171 (17), 4001–4009. doi:10.1111/bph.12662
Vanlalveni, C., Lallianrawna, S., Biswas, A., Selvaraj, M., Changmai, B., and Rokhum, S. L. (2021). Green synthesis of silver nanoparticles using plant extracts and their antimicrobial activities: a review of recent literature. RSC Adv. 11 (5), 2804–2837. doi:10.1039/d0ra09941d
Venkataraman, S., and Hefferon, K. (2021). Application of plant viruses in biotechnology, medicine, and human health. Viruses 13 (9), 1697. doi:10.3390/v13091697
Vijayaram, S., Razafindralambo, H., Sun, Y. Z., Vasantharaj, S., Ghafarifarsani, H., Hoseinifar, S. H., et al. (2024). Applications of green synthesized metal nanoparticles—a review. Biol. Trace Elem. Res. 202 (1), 360–386. doi:10.1007/s12011-023-03645-9
Wang, Y., Hu, F. L., Mi, Y., Yan, C., and Zhao, S. (2021). Single-metal-atom catalysts: an emerging platform for electrocatalytic oxygen reduction. Chem. Eng. J. 406, 127135. doi:10.1016/j.cej.2020.127135
Yadi, M., Mostafavi, E., Saleh, B., Davaran, S., Aliyeva, I., Khalilov, R., et al. (2018). Current developments in green synthesis of metallic nanoparticles using plant extracts: a review. Art. Cells Nanomed Biotechnol. 46 (Suppl. 3), 336–343. doi:10.1080/21691401.2018.1492931
Ying, S., Guan, Z., Ofoegbu, P. C., Clubb, P., Rico, C., He, F., et al. (2022). Green synthesis of nanoparticles: current developments and limitations. Environ. Technol. Innov. 26, 102336. doi:10.1016/j.eti.2022.102336
Zafar, S., Bilal, M., Ali, M. F., Mahmood, A., Kijsomporn, J., Wong, L. S., et al. (2024). Nano-biofertilizer an eco-friendly and sustainable approach for the improvement of crops under abiotic stresses. Environ. Sustainab. Indic. 100470. doi:10.1016/j.indic.2024.100470
Zhang, Y., Dong, Y., Zhou, J., Li, X., and Wang, F. (2018). Application of plant viruses as a biotemplate for nanomaterial fabrication. Molecules 23 (9), 2311. doi:10.3390/molecules23092311
Keywords: green synthesis, nanomaterial, machine learning, sustainable, nanotechnology, healthcare
Citation: Parab AR, Ramlal A, Gopinath SCB and Subramaniam S (2025) Forging the future of nanotechnology: embracing greener practices for a resilient today and a sustainable tomorrow. Front. Nanotechnol. 6:1506665. doi: 10.3389/fnano.2024.1506665
Received: 05 October 2024; Accepted: 30 December 2024;
Published: 27 January 2025.
Edited by:
Abhishek Singh, Yerevan State University, ArmeniaReviewed by:
Ajaya Bhattarai, Tribhuvan University, NepalCopyright © 2025 Parab, Ramlal, Gopinath and Subramaniam. This is an open-access article distributed under the terms of the Creative Commons Attribution License (CC BY). The use, distribution or reproduction in other forums is permitted, provided the original author(s) and the copyright owner(s) are credited and that the original publication in this journal is cited, in accordance with accepted academic practice. No use, distribution or reproduction is permitted which does not comply with these terms.
*Correspondence: Sreeramanan Subramaniam, c3JlZXJhbWFuYW5AZ21haWwuY29t
†ORCID: Ankita Rajendra Parab, orcid.org/0000-0001-6155-4814; Ayyagari Ramlal, orcid.org/0000-0002-1093-9877; Subash C. B. Gopinath, orcid.org/0000-0002-8347-4687; Sreeramanan Subramaniam, orcid.org/0000-0003-4392-285X
Disclaimer: All claims expressed in this article are solely those of the authors and do not necessarily represent those of their affiliated organizations, or those of the publisher, the editors and the reviewers. Any product that may be evaluated in this article or claim that may be made by its manufacturer is not guaranteed or endorsed by the publisher.
Research integrity at Frontiers
Learn more about the work of our research integrity team to safeguard the quality of each article we publish.