- 1Department of Chemical Engineering, Covenant University, Ota, Ogun, Nigeria
- 2Department of Chemical Engineering, Faculty of Engineering and Technology, Parul University, Vadodara, Gujarat, India
- 3Department of Energy Engineering, College of Engineering and Mines, University of North Dakota, Grand Forks, ND, United States
- 4Department of Petroleum and Gas Engineering, University of Port Harcourt, Port Harcourt, Rivers, Nigeria
- 5Parul Institute of Applied Sciences and Research and Development Cell, Parul University, Vadodara, Gujarat, India
- 6Narnarayan Shastri Institute of Technology-Institute of Forensic Sciences and Cyber Security, (NSIT-IFSCS), National Forensic Sciences University, Ahmedabad, Gujarat, India
In many parts of the world, the availability of clean water is almost an impossible task owing to the intrusion of contaminants in flowing or stagnant streams which renders them unsafe for use by man. Water pollution is a serious environmental problem that poses serious dangers to humans, the environment and aquatic life. Also, the recent rise in the spate of emerging contaminants as well as bacteria in waters recovered from process streams, manufacturing and other production activities, have resulted in unforeseen consequences including environmental pollution, health related sicknesses (diarrhoea, dysentery, cholera, skin irritations, lung infection, etc.) as well as loss and extinction of aquatic lives, hence, the need to consider viable methods of treating waste waters so as to render them safe for use by these organisms. This will in turn preserve life below water- SDG 14 as well as promote the use of clean water and a healthy environment- SDG 6. Among the several existing nanotechnologies tailored towards the treatment of wastewater, a couple of inorganic toxic nanomaterials/nanosubstances have been adopted which render such treated waters as potential risks to humans and aquatic lives. However, green nanoparticles are considered potent and viable means of treating these waters, especially when literature has it that some nanomaterials are toxic in nature. Furthermore, the paper also reports on some unpopular but very potent emerging green nanomaterials, alongside recent advances, applications, related challenges and ways to address them. Information on future prospects of green nanoparticles as potential long-term sustainable water purification and wastewater treatment technologies are also included; therefore, this study is focused on addressing issues related to SDGs 3 (ensure healthy lives and promote wellbeing), 6 (clean water and sanitation), 12 (responsible consumption and production) and 14 (life below water).
1 Introduction
In several parts of the world, the availability of water is bedeviled by the degree of wastewater contamination which renders water unsafe for consumption. Human and natural causes both contribute to water pollution (Rosbero and Camacho, 2017). Industries such as the ceramics, super-phosphate, steel, zinc, coal, aluminum, brick, uranium, oil and gas, and zinc usually discharge toxic chemicals in wastewater (Liao et al., 2008; Konishi et al., 2007). However, numerous mechanical and physico-chemical approaches have been adopted as means of overcoming these challenges. Researchers are also exploring other technologies including nanomaterials as possible means of improving low-cost water purification (Jiang et al., 2014; Sanni et al., 2022a). As a result of low cost, high efficiency and reusability, nanotechnology has been proposed as one of the most viable means of purifying water (Mukherjee et al., 2016).
Nano-adsorbents, nano-membranes, nano-metals and photocatalysts are all viable examples of nano-modified materials that hold great prospects as novel alternatives over conventional water treatment technologies with the potential of meeting specific needs of the end-users (Gurushantha et al., 2017; Chowdhury et al., 2016; Karthik et al., 2020; Sanni et al., 2022b).
Recently, nanoscience and nanotechnology have emerged as promising wastewater treatment techniques (O'Carroll et al., 2013). Nanostructured materials have become widely known for use in the removal, conversion and breakdown of dangerous organic (Kalpana and Rajeswari, 2018; Kalpana et al., 2016) and emerging inorganic contaminants due to their unique physicochemical features (Lapworth et al., 2012). These include their high chemical reactivity, thermal stability, strong electron transfer ability, catalytic activity and large specific surface area (Prasad et al., 2014; Kaviya and Prasad, 2015; Nasrollahzadeh et al., 2018a; Nasrollahzadeh et al., 2018b; Yi et al., 2018; Peng et al., 2019; Yi et al., 2019). Biogenic synthetic methods for NPs involve the reduction of metal ions by biological entities to form nanoparticles (Wang, 2000; Lee et al., 2004). For example, Pseudomonas aeruginosa has been used to synthesize silver nanoparticles (AgNPs), with strong antibacterial properties, making them suitable for the disinfection of wastewater (Saxena and Bharagava, 2020; Saud et al., 2024). Similarly, plant extracts, such as those of Azadirachta indica (neem), have been utilized to produce gold (Au) and AgNPs, which exhibit catalytic activities that are useful for degrading organic pollutants in wastewater (Kumar et al., 2019; Li et al., 2024). Biogenic nanomaterials have been applied in the removal of heavy metals (Iwohari et al., 2014), dyes, and organic pollutants from wastewater (Atarod et al., 2015; Ehrampoush et al., 2015; Eskandarloo et al., 2017). For instance, iron oxide nanoparticles synthesized using Magnolia kobus leaf extract have shown high efficiency in removing arsenic from contaminated water (Lunge et al., 2014; Banihashem, et al., 2024). Additionally, biosynthesized zinc oxide nanoparticles (ZnONPs) (Naseem, et al., 2024), Ag-ZnONPs (Patil et al., 2016) and CuNPs have demonstrated the ability to degrade organic dyes under visible light (Mehr et al., 2018).
Photocatalysis is a process where a material, upon absorbing light, generates reactive species capable of breaking down pollutants (Jaafar et al., 2019; Jaafara et al., 2019). Titanium dioxide (TiO₂) is the most extensively studied photocatalyst, but research has expanded to include other materials that can operate under visible light which enhances their performances. TiO₂ is renowned for its photocatalytic activity, especially under UV light. However, its application is limited by the fact that ultraviolet (UV) light constitutes only a small fraction of sunlight (Sah et al., 2024). To overcome this limitation, researchers have explored doping TiO₂ with metals such as Au and Ag or non-metals like nitrogen, to extend its photon characteristics into the visible region, thus enhancing its photocatalytic efficiency for degrading a wide range of organic pollutants in wastewater (Chen et al., 2008). Beyond TiO₂, ZnO, graphitic carbon nitride (g-C₃N₄), and bismuth-based compounds have been investigated. ZnONPs are particularly notable for their high electron mobility, which contributes to the efficient photodegradation of dyes like methylene blue (MB) under both UV and visible light (Nemiwal et al., 2024). Similarly, bismuth oxychloride (BiOCl) has garnered attention due to its unique layered structure, which promotes effective charge separation and enhances its photocatalytic performance when it comes in contact with visible light (Liu and Peng, 2020).
The development of hybrid photocatalysts combining different materials also holds great promise. For instance, composites of TiO₂ and graphene oxide have demonstrated superior photocatalytic properties, which enhances their degradation of persistent organic pollutants such as phenols and pesticides under sunlight (Qasim, et al., 2024). These hybrid systems leverage the strengths of the individual components used to make them to achieve high efficiencies when used in treating wastewater. In the study by Kamali et al. (2019) the sustainability criteria for assessing the applicability of nanotechnology in wastewater treatment applications was presented. Kumar et al. (2023) also carried out a study on the use of bionanoparticles obtained from agricultural waste for waste water treatment.
Reactive green nanostructures are thought to be viable alternatives for water treatment because they have potential features which make them more efficient in converting hazardous/harmful materials into toxic-free substances when used to treat industrial effluents (Gurushantha et al., 2015), thus encouraging responsible consumption and production- SDG 12). Nanomaterials (NMs) and NPs have recently been used in pollution control and environmental monitoring and sensing (Grünberg et al., 2001; Huang et al., 2014a; Huang et al., 2014b). Some nano-catalytic membrane systems and nano-sorbents such as TiO2, Au, Pd, Ag, Cu, Fe3O4, etc. are not cost-effective or environmentally friendly, hence, they are not used on a large scale to treat wastewater.
Over the past few decades, natural resource-based extracellular bio-conjugated metal NPs (Sudhaparimala and Vaishnavi, 2016) which involve the use of capping and reducing agents made from plant-based materials such as proteins, seeds, plants, biomass, starch, fruits (Rupa et al., 2018), leaves (Wang, 2013), and seeds have been studied as potential/sustainable, economically viable, and aesthetically pleasing alternatives relative to chemical production techniques for water treatment applications (Sudhaparimala and Vaishnavi, 2016; Ullah et al., 2017). Accoding to a study, the use of a water-soluble carbohydrate as capping agent gives rise to the green synthesis of metal NPs with impressive superficial characteristics (Markova et al., 2014). Plant extracts containing enzymes, reducing sugars, proteins, alkaloids, polyphenols, amino acids, and flavonoids may be involved in stabilizing metal NPs in the course of reducing metal ions to metal NPs in aqueous solution (Harshiny et al., 2015). Antimicrobial activities against human pathogens and other diseases have been recorded for NPs with plant caps, which have resulted in the emergence of green nanomaterials (NMs) with high activity/efficiency, low impact on the environment, low cost, and easy application (Vijayan et al., 2018). Therefore, green-fabricated NMs can be considered viable options for photo-catalytic reactions in real-world water treatment systems (Njagi et al., 2010), albeit, further in-depth study of their use cannot be undermined (Husein et al., 2019; Gawande and Jenkins-Smith, 2001).
Traditional physico-chemical methods for fabricating nanomaterials have employed flammable and potentially dangerous substances, which have prompted researchers to investigate more sustainable options, such as safer, more cost-effective, bioinspired, biogenic methods for creating efficient and novel nano-scale catalysts and adsorbents that can be used to remove and degrade a wide range of contaminants in water (Gawande and Jenkins-Smith, 2001). Numerous phenolic antioxidants found in plants and other microorganisms serve as reducing and capping agents in the manufacture of NMs in numerous geometrical forms (Ramesh et al., 2018) including rods, flowers, tubes, and wires. Plant extracts contain biomolecules (i.e., polysaccharides, enzymes, vitamins, proteins, amino acids, and organic acids/citrates) that can pair with metal ions to bio-reduce them to their corresponding metal atoms/NPs (Banerjee et al., 2017); this process is chemically complex but benign to the environment.
This critical review examines the state of the art future potential of green-synthesized nano-catalysts and NMs for water and wastewater treatment. Cleaner and more sustainable methods of removing contaminants and metal ions from aqueous solutions like wastewater, groundwater, and drinking water have led to the development of cutting-edge biogenic NMs and novel nano-sorbents, however, a pool of such discourses and their contextual applications are rarely available in literature. In addition, potential applications for wastewater treatment and purification, as well as recent advances, current challenges and future prospects in the use of green-synthesized nano-catalysts and NMs in wastewater treatment are discussed; these clearly situate the insights provided in the contextual framework of this review-paper. For industries that generate chemically-laden waste waters that are hazardous and toxic, the development of new environmentally friendly treatment methods should be seen as a crucial component. In the light of this, this review focuses on synthetically sustainable green NPs obtained from plants, their toxicity assessment techniques and their applications in wastewater treatment. The discourse also centers around recent advances and future considerations for wastewater and water treatment applications of green synthesized NPs alongside the probable challenges associated with their use.
2 Methods for synthesizing inorganic NP and green NPs
2.1 Methods for NP synthesis
The top-down and bottom-up techniques are two ways by which NPs can be synthesized. The top down approach involves the physical break down of large materials into smaller ones, which is accompanied by sequential evaporation-condensation or laser ablation. In contrast, the bottom-up approach, involves growing NPs from assembled atoms into nuclei. The synthesis of NPs via biological and chemical processes is an example of a bottom-up approach. A wide variety of biological, physical, and chemical methods have been used to produce NPs of precisely controlled specifications and forms (Rosbero and Camacho, 2017; Salem and Fouda, 2021).
2.2 Physico-chemical techniques for synthesizing NPs
Several scientists have worked out numerous physical and chemical means of synthesizing NPs that have several applications. Some new methods that have recently emerged to generate NPs with specific geometries include nanoimprint lithography, microcontact printing, photolithography, evaporation-condensation, ion beam lithography, dip pen lithography and electrochemical synthesis. Some mechanical methods that are capable of achieving a few desired geometries have also been discussed (Ahluwalia et al., 2016). In contrast, chemical processes begin by controlling the mass of the source-atoms after reducing the corresponding metal ions to metal atoms (Karlsson et al., 2005; Ahluwalia et al., 2016). Because of their specificity and ability to produce monodisperse NPs, both physical and chemical approaches have often been adopted for the synthesis of other kinds of NPs (Figure 1). A number of techniques have been employed for the production of metal nanoparticles, these include sodium borohydride conversion, gamma-ray irradiation, sol-gel technique, solvothermal synthesis, microwave-assisted synthesis, laser ablation and microemulsion, the use of hydrazine, hydrazine hydrate, and electrochemical reduction. The most popular methods for synthesizing inorganic NPs are associated with a number of drawbacks including energy inefficiencies, high operating costs and toxicity (Soenen et al., 2011; Zoroddu et al., 2014). However, the only meaningful approach to limiting their toxicity is by adopting permissible concentrations that are non-toxic and hazardous but this can only be ascertained by optimizing the process conditions involved in their synthesis. Toxic chemicals, costly machinery, and a number of treatment processes including temperature, pressure, and pH variation are often prevalent in these procedures. Furthermore, other by-products that are harmful to the ecosystem are also produced by these methods. This is because a number of polar and non-polar solvents are part of the chemical toolbox for a wide range of so-called bottom-up NP creation strategies which in turn lead to the synthesis of a wide variety of metallic NPs of various compositions, sizes and shapes (Rosbero and Camacho, 2017). The available physical and chemical methods for NP synthesis are not only expensive, but also generate compounds that are very harmful and hazardous, thus posing severe risks. As a result, there is an immediate advocacy for the adoption of green NPs as viable alternatives (Zhou et al., 2016).
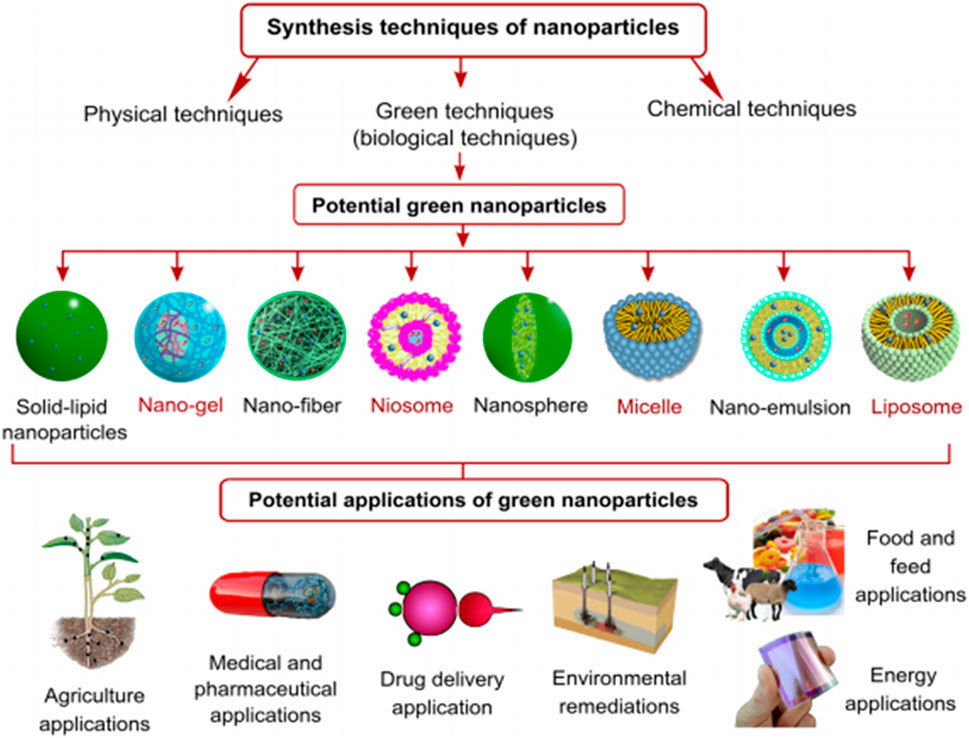
Figure 1. Techniques, forms and synthesis of NPs, biogenic NPs and their applications Osman et al. (2024); open access.
2.3 Methods for synthesizing green NPs
Producing green NPs at moderate pH, temperature, pressure and at a much-reduced cost using plants, bacteria, fungi (Sastry et al., 2003) and other biological materials, helps to circumvent many of the supposed detrimental characteristics associated with those produced by conventional techniques (Harshiny et al., 2015; Korbekandi et al., 2014; Zhou et al., 2016). Actinomycetes, algae, yeast, bacteria, fungus (Bansal et al., 2004), and plant extracts (Iravani, 2011) have been used to synthesize NPs in an environmentally friendly manner (Aswathi et al., 2022). The production of Mg, Ti, Au, Ag, Cu, Zn, and alginate NPs from a variety of bacteria, microbes, and fungus have been studied (Shen et al., 2017). Viruses, bacteria, actinomycetes, and fungi have also proven to be viable sources of bio-synthesized metal NPs such as those of Pt, Au, quantum dots, Te, Ag, Zr, Zn, Se, Ti, magnetite and Cu.
When adopting the bottom-up approach for green NP production, biomolecules including sugars, enzymes, carbs, protein, etc. bring about the release of metallic ions during the oxidation/reduction process, which then leads to the formation of the NPs (Nadaf and Kanase, 2016). Since different types of microbes interact with metal ions in different ways, knowledge-gaps on the mechanisms behind the use of microbes in the synthesis of NPs are yet to be uncovered. The shape, morphology, and size of the synthesized NPs from biomolecules are ultimately impacted by the processing technique, interactions within the microbe, as well as the environmental conditions (temperature and pH) (Arora et al., 2014). Consequently, the following are some of the most important obstacles that could pose delays in the adoption of green synthetic routes for biogenic NP synthesis. Since it is known that the biological activities of green NPs mirror the optimization procedures that are necessary for their green production, a comprehensive chemical analysis of the biological biomass filtrate is also necessary for identifying the function of each component involved in the bio-fabrication of the resulting green NPs. In addition, future research works are to focus on economic considerations for scaling-up green NP application for industrial wastewater treatment. Furthermore, in order to create innovative commercially viable green nanomaterials, multidisciplinary research collaborations across various disciplines must be prioritized as no one discipline has all it takes to pull this through. Figure 2 is an illustration of the synthetic sources and pathways for biogenic NPs.
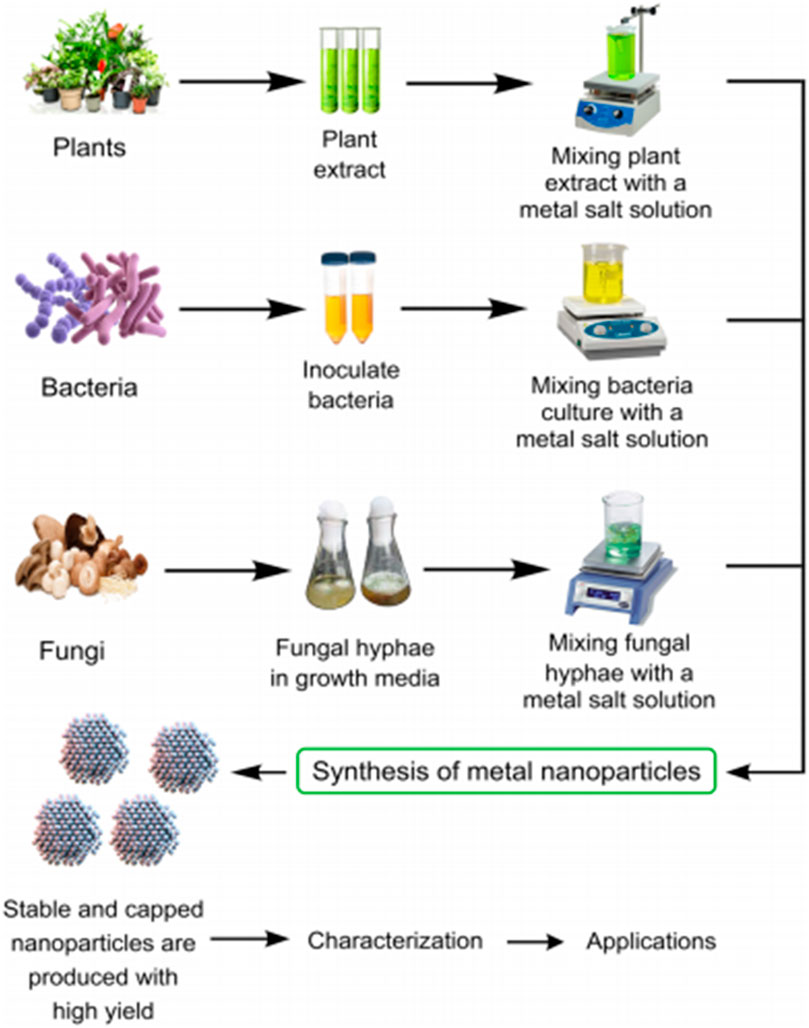
Figure 2. Synthetic sources and pathways of biogenic NPs Osman et al. (2024); open access.
3 Nature/characteristics of green Nps in water/wastewater treatment
Heavy metals, halogenated recalcitrant pollutants, toxic textile dyes, pigments, pharmaceutical/personal care products (PPCP), pesticides (Yadav et al., 2015), refractory organic micro-pollutants and pathogenic microorganisms have been eliminated from contaminated waters using biogenic nanoparticles (BNPs) made from various bacterial species (Bousselmi et al., 2004; De Gusseme et al., 2011; Furgal et al., 2015; Hatamifard et al., 2015; Xiao et al., 2015b; Hatamifard et al., 2016; Xiao et al., 2016; Zhou et al., 2015; Zhou et al., 2016; Martins et al., 2017; Qu et al., 2017; Gautam et al., 2019). De Corte et al. (2012) also found that wastewater and their sediments can be used to generate power and recover useful resources such as metal ions. One promising avenue for recovering resources from polluted water is the capacity of microorganisms to alter the oxidation state of metals (Kashefi and Lovley, 2000; Kim et al., 2007; Kim et al., 2009; Kim et al., 2012).
It is possible to recover used BNPs from wastewater using microbes rather than using a solution of the metal ions (Ahluwalia et al., 2016; Kim et al., 2001). The presence of high specific surface areas, opposing charges, unique sizes, high reactivity, and the availability of bacterial cell matrix make these synthesized BNPs prominent for various applications (e.g., as adsorbents, catalysts, antibacterial agents, fabrication of anti-biofouling membranes, etc. (Sureshkumar et al., 2010; Chokkareddy and Redhi, 2018; Dauthal and Mukhopadhyay, 2016; Dhanker et al., 2023). BNPs have the ability to biodegrade harmful micro-pollutants in water and wastewater when used as adsorbents (Ali et al., 2015; Ali et al., 2019).
3.1 As bio-adsorbents
In some investigations biogenic adsorbents were used as eliminators of contaminants from contaminated waters. This is due to the fact that the rate controlling step in the adsorption process provides clues to the removal mechanism on how this works (Jain et al., 2016a; Jain et al., 2016b). Adsorption isotherms and kinetic models were employed, and the estimated R2 values were used to compare the results. According to Table 1, the majority of the studies confirm that the adsorption kinetics and isotherm data conformed to the pseudo second-order kinetic model as well as the Langmuir isotherm. The results shown in the available publications mostly corroborate the chemisorption hypothesis in relation to the elimination of contaminants/pollutants from the water. According to Jain et al. (2016a), the availability of the functional groups (hydroxyl, carboxyl, amino, etc.) on the BNPs were crucial for the adsorptive removal of contaminants/pollutants via ion exchange and electrostatic interactions. On the other hand, based on some findings, the adsorption of contaminants/pollutants may be caused by surface precipitation (Kim and Baek, 2019), co-metabolism of microbes, oxidation/reduction (Kandasamy, 2017), intraparticle diffusion, chemical monovalent ion exchange, or pseudo first order reaction (Watts et al., 2015a; 2015b). Table 1 supports the fact majority of researches involving adsorption isotherms, assumed that the contaminants or adsorbates were adsorbed on a solid surface characterized by a monolayer, thus giving credence to the Langmuir model.
The adsorptive elimination of toxic heavy metals such as Ni(II), Cr(IV), Mn2+, Co(II), Pb(II), Ag+, Cu(II), Zn2+, and Cd2+ via the use of BNPs are as contained in Table 1. The occurrence of certain functional groups—such as hydroxyl, carboxylate, methyl, and amide I, II, and III, as well as a range of reducing substances produced by bacterial cells, which had charges opposite to the metallic ions are responsible for the removal of the toxic metals. Ion exchange and electrostatic attraction were the main mechanisms by which the metallic ions were removed, with the -OH-groups playing a particularly active role. The surface precipitation that aided the removal of the harmful metal ions was likewise facilitated by carboxylate groups (Watts et al., 2015a). Watts et al. (2015b) noted that the existence of electrostatic contact may cause the H+ ions released by bacterial cells to bind to metallic ions on the surface of an adsorbent. Nevertheless, it was also observed that various BNPs produced by different microbes in salt solutions exhibited varying degrees of adsorption of the metal ions. This variation is attributed to the capabilities of the individual functional groups and the presence of various cell metabolites in the BNPs (Iwohari et al., 2014). In addition, the Langmuir isotherm model was used to determine the maximum daily adsorptive capacity and removal of the heavy metals. Moreover, the metal-ions removal efficiency of the BNPs varied with the metal-types and circumstances surrounding the adsorption process, which may be explained by the presence of the negative charges on the surfaces of the BNPs or the selective adsorption of hazardous metal ions onto BNPs imposed by the smaller ionic radius of the metal and its electronegativity/larger ionization potential. Noting how easily BNPs produce metal hydroxyl species or acetate complexes is another way to track their relative preferences for adsorbates (Tuo et al., 2013).
Factors such as pH, contact time, initial pollutant/contaminant concentration, and adsorbent dosage are known to influence the performance of BNPs (Kumar et al., 2016; Deng et al., 2017). Although, BNPs have the potential to operate throughout a wide pH range, however, their efficacy would differ depending on the specific BNP type and the pollutants in question (Table 1). While utilizing biogenic SeNPs, a maximum of 95% adsorptive removal of Cu2+ ions was obtained at a pH of 5, whereas, at a pH of 12, bio-Pd was found to remove 64% of Cr(VI). According to several studies, the adsorptive removal/performance of BNPs may be affected by changes in pH, which in turn affect the attraction and repulsion between the pollutants and BNP surface-sites (Jain et al., 2016; Watts et al., 2015a; Mystrioti et al., 2016). The effectiveness of BNPs in eliminating certain contaminants may also be affected by factors such as contact time and adsorbent dosage (Fredrickson et al., 2000). More than 96% of the Cr(VI) was eliminated in 24 h, according to the research conducted by Tuo et al. (2013) via biogenic Pd(0) produced by Geobacter sulfur-reducers. The results showed that the biogenic Pd(0) surface and the G. sulfurreducen cells were metabolically active in reducing Cr(VI). Also, adding anthraquinone-2,6-disulfonate (AQDS) to the cells, increased the amount of biogenic Pd(0) and also raised the cell’s dry weight with an improvement in Cr(VI) removal.
3.2 As green catalysts/photocatalysts
According to Table 2, BNPs have been proven to have good catalytic efficiencies when it comes to removing various organic contaminants, including those that are hazardous or difficult to break down. Pesticides, organic/inorganic solvents, medicines, hazardous metals, and other industrial activities are the primary sources of these contaminants. To charge or activate a biogenic catalyst, a reducing agent like formate or hydrogen gas is often used (Durán et al., 2015). On the other hand, there have been instances when bio hydrogen donors were created by using certain strains of microbes or organisms that are grown by fermentation. Furthermore, this choice makes the technology economically viable while also lowering the treatment’s running expenses. Biodegradation or reduction of pollutants mostly includes a combination of biosorption which results from electrostatic interactions, microbial co-metabolism induced by functional groups (Hazarika et al., 2017) and a redox process.
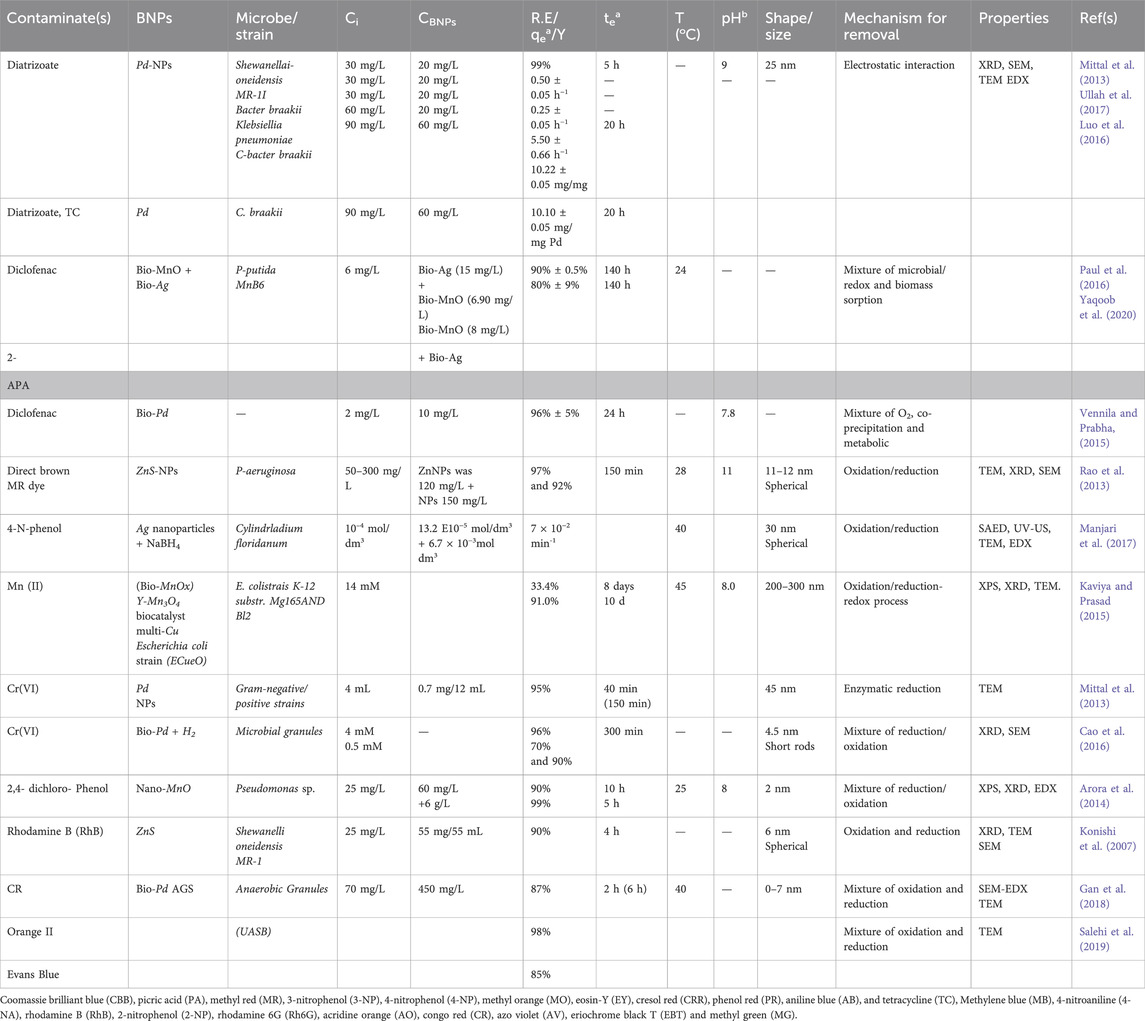
Table 2. Brief summary of the catalytic performances of biogenic nanoparticles (BNPs) in the removal of toxic and emerging pollutants (T&EPs).
Bio-Pd made from wastewater containing bacteria was reported by Deplanche et al. (2014) to completely remove Cr(IV) from wastewater. Using bio-Pd as catalyst and in situ bio hydrogen as an electron donor, Suja et al. (2014) reduced the concentration of Cr(IV) completely within a day. Table 2 shows that BNPs can successfully remove harmful pigments and colours from wastewater. According to these results, the presence of various reducing chemicals produced by bacterial cells helped to biodegrade these harmful colours via co-metabolism of the BNPs and bacterial cells. It should be noted that the use of different bacterial strains and BNPs of different kinds resulted in different biodegradation rates of the same harmful dye. Nevertheless, the total quantity of dye elimination was high. In the study by Xiao et al. (2015a), rhodamine B (RhB), was completely biodegraded after being exposed to UV light for 3 h. Biogenic ZnSNPs were produced when artificial wastewater was treated with the bacterium Shewanella oneidensis MR-1. In addition, it was recounted that photogenerated holes, and not eOH radicals were the primary culprits in the decolourization of the dye. The insufficient power of the photogenerated holes by the biogenic ZnS under UV irradiation alongside the produced OH−/H2O and eOH radicals were seen as the proposed reason for the occurrence. This is because the valence band edge (VB) voltage of the biogenic ZnS was 1.92 V versus that of the semi conductor edge (SCE) voltage, which is lower than the normal voltage potential of 2.7 V for the OH−/·OH. Ahluwalia et al. (2016) attempted to enhance the catalytic performance of Bio-Se by impregnating ZnS with Se through a calcination process. This resulted in the degradation of methyl orange (MO) by the nanophotocatalyst (Se-ZnS). After 160 min of UV irradiation, the dye removal rate was approximately 95%. As the degree of MO degradation rose, its removal fitted the pseudo-first-order kinetic model, and the emission of CO2 grew steadily. Alvaro et al. (2007) observed that photocatalytic performance could be enhanced by photoexcited semiconductor electrons generated from a metal organic framework (MOF), while Xiao et al. (2015a) observed that the extra energy levels of an impregnated Se boosted its photocatalytic performance. By subjecting ZnS to ultraviolet light, electrons were energized and paired with holes in the valence band, which then migrated to the covalent band. Due to their extreme instability, the excited electrons may return to the valence band in a matter of seconds, thus leading to electron-hole pair recombination and the inability of the catalyst to produce the necessary ·OH radicals and superoxide ions for organic pollutant destruction. The results also show that Se may have improved the semiconductor’s electron-hole pair separation by acting as either an electron/hole trap. However, its electrical arrangement would have been upset if it managed to capture the electrons such that the resulting superoxide radical was transported quickly to an oxygen molecule while further degrading the MO. Moreover, the Se can trap the hole and maintain its stable electronic state, thus influencing the adsorption of the resulting ·OH radicals onto the surface of the ZnS. Moreover, the degradation of the organic contaminants was mostly accomplished by the hydroxyl radicals and the superoxide ions. In the study by Yue et al. (2016), biogenic PbS in combination with H2O2 helped to degrade 100% of methylene blue (MB) in only 20 h when the medium bearing the PbS was exposed to UV light. Also, by manipulating the polyethelene glycol (PEG) content in the microbial system, a novel approach was established for the fabrication of high-quality biogenic PbS. The results showed that, rather than the specific surface area of the BNPs, the number of the available crystal planes was the primary factor responsible for the catalytic degradation of the MB. In addition, azo dyes (such as Congo red, orange II, and Evans blue) were degraded with over 80% degradation efficiency in only 4 h of contact time when Bio-Pd was immobilized on an anaerobic granular sludge (Quan et al., 2015). The results demonstrated that glucose, formate, ethanol, acetate, and lactate were the electron and hydrogen donors that triggered the breakdown of the azo dyes. A thorough investigation was carried out by Qu et al. (2017), on the use of biogenic AuNPs in breaking down harmful dyes produced by Trichoderma sp. WL-Go and Aspergillum sp., respectively (Table 2). Other properties of green NPs include, lower toxicity, biocompatibility, small surface area to volume ratios, size tunable features, uniform morphology and high penetration strength through cells, etc.
3.2.1 Advantages/benefits of biophotocatalysts and biogenic nanomaterials for wastewater treatment
The benefits or advantages of green nanomaterials/nanophotocatalysts/nanomaterials used in wastewater treatment include:
- availability of clean water: nanoparticles/biogenic nanoparticles of permissible concentrations are able to render contaminated wastewater free from contaminants (ionic salts, heavy metals, expired drugs, toxic chemicals etc.) (Devi and Ahmaruzzaman, 2016; Lingamdinne et al., 2017) such that the pH of the purified water assumes that (6.8–7.0) which is safe for drinking (Table 3).
- less environmental pollution: bionanocomposite membranes/biosorbents such as multiwalled carbon nanotubes have been used as adsorbents or hybrid-adsorbents to rid off contaminants from wastewater (Lloyd and Macaskie, 2000; Sathyanarayana and Hübner, 2013; Khodadadi et al., 2017a; b; Sanni et al., 2022a; b; Kumar et al., 2023).
- reduced sicknesses and mortality: nanoparticles (encapsulated graphene and reduced graphene oxide nanocomposites) have proved their worth in terms of eliminating and detecting toxic contaminants that are cancerous and capable of causing lung infection which can lead to death or high mortality if not diagnosed early (Sanni et al., 2021; Sanni et al., 2023a Sanni et al., 2023b).
- improved health: several communities where contaminated water is used on daily basis are prone to incessant cases of diarrhoea, cholera, dysentery and other water-related sicknesses which in turn lead to deterioriated health and low productivity since evidence has it that, many rural community dwellers depend on farming for their daily existence.
- preservation of aquatic life and secondary hosts: many contaminants such as microplastics that have high tendencies for bioaccummulation in fish tissues including their stomachs, livers and gills as well as reduce the average life expectancy of the fishes and in turn cause adverse effects in humans when such fishes are consumed by humans, can be trapped by BNPs from such waters.
- improved food production: in biotechnology and agriculture, efforts are in place regarding the use of bionanoparticles as soil supplements (Machado et al., 2013a; Machado et al., 2013b) or composites of membranes used as seed coats for CO2 capture which aid plant photosynthesis as well as boost food production.
- reduced extinction and preservation of the current biodiversity in oceans, rivers, lakes etc.: many aquatic organisms have gone extinct as a result of their ingestion of poisonous contaminants or pollutants in wastewaters; today, a number of marine-habitat species are no longer in existence due to anthropogenic activities that have led to the destruction and elimination of such lives below water. Hence, bionanoparticles/green nanotechnology is an apt technique for securing the current biodiversity.
- Enhancement of equipment service life: The presence of chlorinated salts in fresh/wastewater can lead to the corrosion of submersible pipes lined through such waters. However, the use of nanofluids as corrosion inhibitors has helped to provide protective films that abate corrosion.

Table 3. Biosynthesized metal and metal oxide-based nano-catalysts for the degradation of pollutants in water.
3.2.2 Stability and reusability of green synthesized NPS
Based on literature hitherto, it is clear that green-synthesized NPs hold more promises than their conventionally-prepared counterparts in terms of efficient, safe, nontoxic, clean, and environmental friendliness, and hence their better performance in terms of pollutant removal. The regeneration and reusability of NPs is crucial to the cost-benefit analysis of nano-based water and wastewater treatment technologies and their long-term viability (Grünberg et al., 2001; Ali et al., 2016; Tajbakhsh et al., 2016). Several other studies have shown that NPs have been successfully used as adsorbents with no effluent released/generated (Husein et al., 2019; Prasad et al., 2019; Manjari et al., 2017). The ability of NPs to produce zero-effluent/-industrial wastewater is due, in part, to the presence of organic functional groups on the surface of the adsorbent, which may degrade after a certain amount of time. This phenomenon, however, also reduces the NPs’ reusability (Hou et al., 2018). For economic and commercial reasons, it is crucial that NPs be reusable, recyclable, and somewhat stable. Spherical magnetic NPs synthesized using Lagerstroemia speciosa bark (LB) extract by co-precipitation method were found to be effective in removing Cr(VI) from aqueous solution (Al-Asfar et al., 2018). Both Langmuir isotherm and the pseudo-second-order models gave good descriptions of the kinetics and equilibrium adsorption. Cr(VI) adsorption using magnetic NPs was found to be more than 93.72% after 11 successive adsorption-desorption cycles, and the NPs were easily collected from the aqueous solution by a magnet after the maximum adsorption of Cr(VI); the results are in sync with the observations of Wei et al. (2017) when iron oxide nanoparticles were used to adsorb Cr(VI) from an aqueous system. Iron oxide NPs were synthesized using Eucalyptus globulus plant extract. The results showed that the iron oxide NPs synthesized in the laboratory effectively adsorbed arsenic. However, the As(V) desorption experiments showed that the synthesized iron oxide NPs were readily regenerated in basic solutions of moderate concentrations (Martínez-Cabanas et al., 2016; Farhadi et al., 2017).
However, they asserted alongside Lateef et al., 2016 that more investigations are required to ascertain the effective use of green synthetic nanoparticles in water and wastewater treatment
3.2.3 Limitations of green NPs/biogenic nanomaterials for wastewater treatment
Several agrowastes including those of wheat straw and oat resources are potential sources for the design of sustainable adsorbents for wastewater treatment which do not allow room for any food competition concerns. The development of biowaste materials including cellulose, lignin and hemicellulose into effective, low-cost adsorbents have been discussed (Taleb et al., 2020; Sajjadi et al., 2021). Agriculture prone regions such as North America (Saskatchewan), produce 60% of Canada’s grain crops including oat hulls and wheat straw which are abundant, sustainable and renewable. The benefits of torrefaction as a processing technique include its ability to infuse greater polar characteristics alongside uniform particle size/particle distribution. However, studies have highlighted some of the challenges associated with pelletizing wheat straw residue after undergoing torrefaction (Agu et al., 2021). Thus one strategy that is viable for addressing such challenge is to modify the adhesive and mechanical properties of the microwave mildly torrefied materials at 200–300°C and blending them with high density polyethylene (HDPE) residue, all aimed at achieving a more efficient biocomposite adsorbent for wastewater treatment with better adsorptive properties over those of the pristine oat and wheat waste. Figure 3 is an illustration of the potential limitations in the application of green NPs.
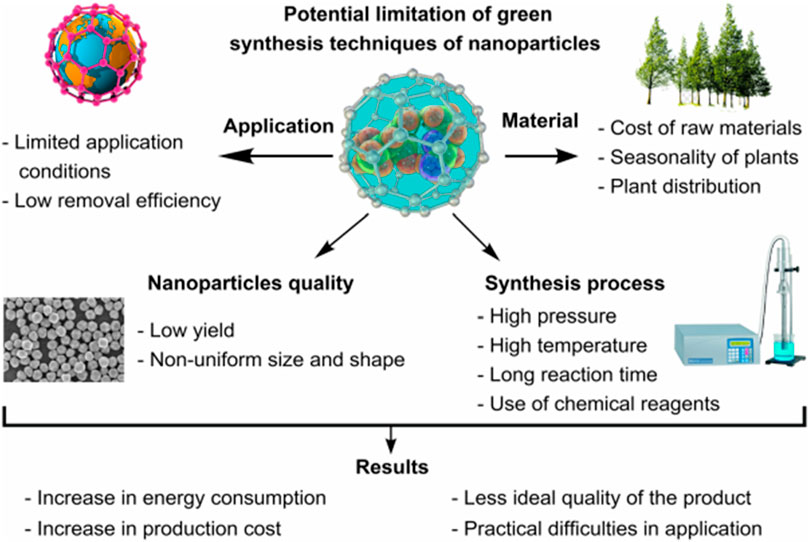
Figure 3. Potential limitations in the industrial application of green NPs (Osman et al., 2024).
Chitosan, a highly abundant biopolymer/biomaterial, which has >50% glucosamine units is a versatile polysaccharide which can be obtained by the deacetylation of chitin, (Ahmed and Ikram, 2017; Mohammadzadeh Pakdel and Peighambardoust, 2018). The degree of deacetylation influences its physicochemical properties, especially those that are related to adsorption (Fierro et al., 2008; Dey and Ghosh, 2020; Tavares et al., 2020). Due to the covalently modified forms of chitosan, its entrenched abundant functional groups can be tailored to form biocomposites of varying structural stability towards the attainment of the controlled adsorption of several friendly/toxic chemical species (Steiger et al., 2021; Parandhaman et al., 2016; Parandhaman et al., 2019). Also, evidence has it that, kaolinite, an inorganic aluminosilicate clay, interacts favourably with chitosan for improved stability owing to the infused synergistic effects offered by its individual components when pelletized or disintegrated into nanoparticles or nanomaterials which afford them higher surface areas to volume ratios for enhanced adsorption. The establishment of chemical interactions (i.e., electrostatic or H-bonding) between kaolinite and the additive components make it an efficient binder for bionanocomposites (Chen et al., 2013). In a study, kaolinite was employed as a proposed filler/binder in ternary pelletized biomaterials (Bezerril et al., 2006) which then informed its merit as a biomaterial with cation exchange capacity and adhesive properties that are needed in chitosan-based bionanocomposites (Unuabonah et al., 2008). The use of physically blended kaolinite, chitosan and agro-waste materials have been exploited as adsorbents for lead-ion removal from drinking water (Mohamed et al., 2022); despite the quantity of Pb removed, evidence has shown that such adsorbents are more effective when they are used as bionanocomposites of the blended components. The authors also proposed an optimum use of 40 wt% agro-waste based on the estimated stability limit of the composite pellets upon immersion in aqueous media. However, when used in their nanoforms, the measure of stability might drop except if compatible supports are used as reinforcement materials for the nanoadsorbents. To address stability limitations in such bionanocomposites, the extended weights and concentrations of the agrowaste may also become feasible owing to the size reduction effect of the nanosized materials/particles relative to the pellet forms; this helps to provide for improved uptake capacity of the effluent dye/contaminant.
In terms of economics, the cost and adsorption properties of adsorbents made from agro-waste sourced from industrial crops were compared with those of spent coffee grounds (SCG) in order to evaluate the valorization potential of agro-waste composites as sustainable adsorbent materials for cationic pollutants (Sabzevari et al., 2018; Kong and Wilson, 2020). In the study by Steiger et al. (2023), it was affirmed that a facile and bottom-up design strategy of biocomposite materials leads to the valorization of agro-waste biomass yields of functional adsorbents with tailored properties (Mohamed et al., 2020). Therefore, their modified pelletized or nanosized forms will provide better adsorptive properties within the circular economy model for agro-waste derived adsorbent materials for wastewater treatment (Nadagouda and Varma, 2008; Omran and Baek, 2022).
3.2.4 Benefits and applications of biophotocatalysts
The Fenton process is one popular approach that uses an acidic medium to induce the reaction between ferrous ion and H2O2 to generate •OH groups for the effective degradation of various recalcitrant natural organic pollutants. The slow rate of generation of ferrous ions in the Fenton process provides for a more effective nanocatalytic degradationan aided by the ions which eventually leads to increased higher sludge production in the treatment step (Ahmadi et al., 2021; Nidheesh, 2015; Guiterrez et al., 2022). This drawback can be partly controlled with the use of heterogeneous catalysts (Karim et al., 2022). Various synthetic heterogeneous nanocatalysts such as FeO NPs (nZVI) (Puiatti et al., 2022; Nadagouda et al., 2010), Fe2O3 (Shahwan et al., 2011; Buarki et al., 2022), mixed iron oxides (Jain et al., 2021), PdNPs (Narasaiah et al., 2017) and bimetallic NPs were biosynthesized using green technology for the degradation of pollutants such as dyes (Sajadi et al., 2018; Zhang et al., 2018; Hassan et al., 2020; Jain et al., 2021), antibiotics (Stan et al., 2017; Ouyang et al., 2019), and nitro-benzene. The photo-Fenton (Puiatti et al., 2022), electro-Fenton, and sono-Fenton (Prakash et al., 2021) operations of different fenton nanocatalysts have been reported alongside their antibacterial activities (Buarki et al., 2022) in several adsorption studies. In addition to the preparation of these nanocatalysts, some synthetic minerals and carbon residue ensue at their production stages which has led to the concentration of efforts in the recent development of Fenton catalyst/Fe3O4 supported on mesoporous carbon or other mineral supports such as bauxite (Al2O3). Also, bentonite-supported on nZVI has also been employed in the chemical degradation of organic pollutants in wastewater (Hassan et al., 2020). Materials such as leaf extract (Ayodhya and Veerabhadram, 2017; Maryami et al., 2016; Maryami et al., 2017; Puiatti et al., 2022), tea extract (Hassan et al., 2020), tea polyphenols (Ouyang et al., 2019), waste-lignin, fruit-peel extract (Jain et al., 2021), flower (Buarki et al., 2022), and plants have been adopted in the green synthesis of Fenton catalysts and it was observed that the green materials acted as reducing agents (Puiatti et al., 2022), stabilizing agents (Puiatti et al., 2022), and capping agents. Franco et al. (2021) examined the catalytic potential of green Fe3O4NPs produced by thermal treatment of Cammelia sinensis (black tea) extract and Fe3+ salt for the removal of azo dye/methyl orange from aqueous solution. Thermal heating aided the removal of tea polyphenols. An illustration of the comprehensive thermal phase transformation of the generated and exposed rare nano β-Fe2O3 phase of the catalysts at 400°C preceded its subsequent conversion to α-Fe2O3 as the temperature increased. Table 4 contains some information on some photocatalysts that have been used for wastewater treatment.
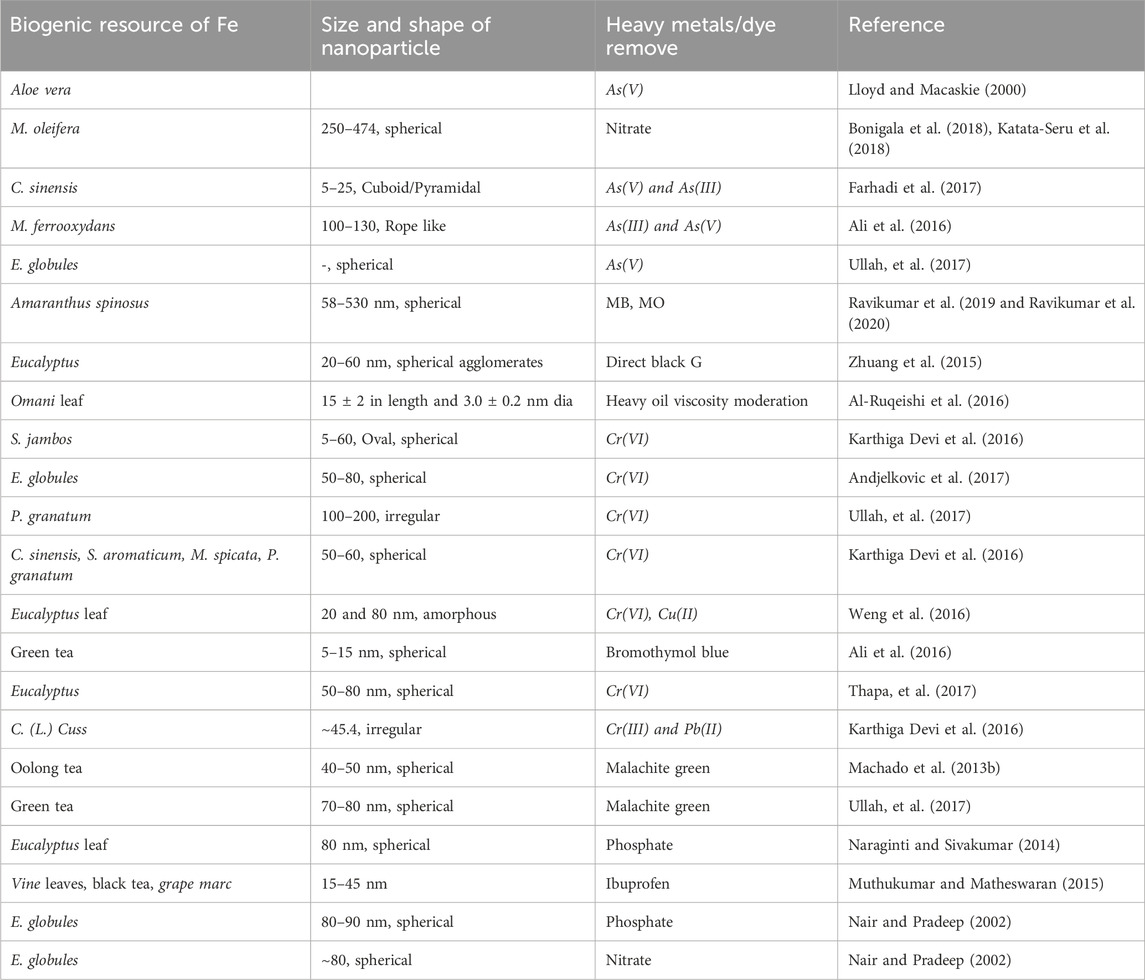
Table 4. Iron based biogenetic nanoparticles resource for the removal of heavy metals and dyes from contaminated waters and other fluids.
4 Potential risks associated with the use of green NPs, their long-term environmental impact and their bioaccumulation potential
Due to rapid industrialization and urbanization activities, a quantum of wastewater containing toxic chemicals and heavy metals is generated consistently, which constitutes high environmental nuisance, thus affecting the average life expectancy/wellbeing of the global populace. The recent advancements in sustainable cost-effective wastewater treatment technologies have attracted more attention from policymakers, to legislators as well as the scientific community. In the study by Kumar et al. (2023), the application of biochar and green NPs obtained from agro-waste were employed in removing refractory pollutants from water and wastewater; the contemporary application, and mechanism of the biochar-supported advanced oxidation process (AOP) incorporated the use of green NPs for the effective removal of organic and inorganic pollutants [noxious chemicals/compounds such as (HMs)] (Bolan et al., 2022a; Bolan et al., 2022b), endocrine disrupting compounds (EDCs) (O’Connor et al., 2022), polyaromatic hydrocarbon (PAHs) (Kumar et al., 2021), personal care products (PCPs), micropollutants (micro-nano-plastics) (Sridharan et al., 2021), pesticides (Sun et al., 2020), etc. from contaminated streams/wastewater. Although, green synthesized NPs/NMs offer significant benefits such as costefectiveness/ease of application (Hano and Abbasi, 2022), they also help avoid the use of harmful chemicals/solvents, which allows for the accurate fabrication of NMs/NPs with consistent shapes and sizes, with minimal or no waste generation (Harish et al., 2023). However, one of their shortcomings include the fact that they are useful within low temperature and pressures (i.e., may lose their potency at high temperatures and pressures) compared to their counterparts which are synthesized via traditional methods, however, these conditions reduce the tendencies for unforseen incidents/events (Dikshit et al., 2021). Green NPs/NMs also facilitate the use of sustainable raw-materials in large-scale production systems (Soltys et al., 2021). Nonetheless, existing literature lacks sufficient information on considerations for human health and the risks associated with in-vivo and ex-vivo nanotechnology deployments. Furthermore, researches on environmental toxicity and bio-accumulation of green NPs/NMs are quite inadequate, however, their existence in very small sizes facilitates their influx into humans, thus causing respiratory disfunction and severe sicknesses (Pietroiusti et al., 2018). Also, the industrial application of green NMs/NPs is relatively limited, as a result of issues related to difficulty in controlling their sizes, shapes and instabilities (Dikshit et al., 2021). In essence, comprehensive toxicological assessments/genetic modifications may necessitate the enhanced synthesis and application efficiency of NMs or NPs. This is because there is a dearth in knowledge/information on universally acceptable plants and seasonal factors that affect the growth of such plants alongside the synthesis of biogenic NPs/NMs, which therefore poses constraints that hinder the widespread application of green NPs/NMs.
5 Bioaccummulation of green NPs and their plausible long term environmental impacts
Due to the nature of the origin of green NPs that are used as reactive adsorbents, they have a low measure of bioaccumulation potential, this is because of their high reactivity or potential to degrade wastewater contaminants or pollutants (Shafey, 2020), hence they may not constitute any nuisance since they end up interacting with contaminants to produce new/value adding products (Oni et al., 2023). This in turn reduces their potential for bioaccummulation except when they are used as catalysts. Catalysts are known to cause reactions to take place without them undergoing any form of consumption all through the entire process; therefore, nanocatalysts that are employed in wastewater treatment have a higher risk of bioaccummulation compared to when they are merely used as adsorbents that have high biodegradation potentials which in turn increases their tendencies for bioaccummulatiin, thus influencing soil fertility, air quality, food production, etc., when they are discarded after use. In another regard, one approach via which this problem can be addressed is by the adoption of nanocatalysts synthesized (whether from chemical constituents or from plants) at optimal/permissible concentrations which are nontoxic and can bring about the conversion of pesticides such as 4-chlorophenol into several multiple useful chemicals to mankind (Sanni et al., 2022a). Also, in another context, the bioaccummulation of bionanocatalysts encapsulated in membrane coated seeds are stable and quite beneficial as potential fertilizers for the stimulation of plant growth. Therefore, for catalysts used as fertilizers, their long term environmental impact may be quite beneficial in terms of their role in boosting food production or the conversion of toxic compounds to value adding products. However, if the nanoparticles do not have high selectivity for the target-toxic compounds in host systems, there may be high risks of bioaccummulation which may in turn result in more complex situations. Even in situations where the NPs can trap the toxic components from such systems, one feasible long term solution to abating issues related to bioaccummulation is the use of nanopartcles of permissible concentrations that are non toxic/within the optimum requirement while ensuring they have a good measure of stability such that they can be recycled for use or have a long reusability span which will help reduce the quantities used per continuous operation during contaminant removal (Verónica et al., 2024); this then implies that the system will be such that it is a continuous and not a batch process, where the nanomaterials and photocatalysts are used and dislodged after the first run for clean ups before being used in another batch of contaminant removal; this will also help to ensure some measure of commercial viability for the green NPs. In addition, since biological wastewater treatment processes rely on biological entities to degrade and remove their inherent contaminants and pollutants, they are prone/vulnerable to high toxicity levels. However, considering the fact that several toxicity measurement methods have been proposed for wastewater treatment processes, most of the known techniques are performed off-line, and are usually not adaptable to on-line monitoring systems, especially in terms of providing early warning signals for potential risks to water treatment operators, systems and the environment (Xiao et al., 2015b). Nonetheless, the past decade has recorded a rapid growth in the research and development of biosensors for the toxicity assessment and effective treatment of contaminated aquatic environments and wastewater. In clear terms, it is needful to begin to consider examining the sensitivity of assays by sensor-based NPs sourced from single organisms that will match one or more toxic contaminants as targets, or better-still, consideration can be given to the development of a matrix of biosensors or a biosensor incorporated with NPs synthesized from multiple organisms, such that they have the potential of eliminating a range of contaminants from wastewater. This can be achieved by testing the micro fuel cell (MFC)-based biosensors with real life-contaminated wastewater and comparing the results with well-established toxicity assays/detectors including those based on oxygen uptake rate (OUR) or CH4-uptake for the detection of biogenic/green NPs (Au, Ag, Pd, etc.), with integrated advanced data acquisition and processing methods for interpreting the on-line toxicity sensor results in real life which reduces the disturbances associated with the fluctuations in the quality and quantity of wastewater. The only challenge here is that biosensors are quite expensive but their long term viability cannot be overemphasized.
6 Challenges associated with scaling up green NP synthesis for industrial application, significant barriers to commercialization and strategies for overcoming them
Over the years, the remarkable advances in the use of green NMs and NPs, have positioned them as top-contenders across various domains/idustries/sectors including agriculture (i.e., boosting soil fertility and crop production), medicine (nanovesicles as drug carriers for efficient drug delivery) (Sengani et al., 2017; Sanni et al., 2022c), environment (abatement of environmental pollution), food (food packaging), sensor technology (smart technologies for the identification of nanotoxic materials), electronics, etc.
Despite the fact that, the fabrication and application of agricultural waste-derived biogenic NPs hold great prospects as a green approach for wastewater treatment, however, prior to scaling up their production and industrial application processes, their toxicological and life-cycle challenges must be taken into account when used in biosensors (Sanni et al., 2023b) or evaluated using correlations that can estimate/measure and detect their toxicity/hazard levels while bearing in mind the process economics, cost-effectiveness and life-cycle assessment of the entire production route of the bio-nanoengineered materials which are often exploited in the generation of green catalysts/adsorbents/materials with enhanced treatment potentials for wastewater treatment (Kumar et al., 2023).
Nanomaterials, such as cellulosic-nanofibrils and nanocrystals have assisted in revolutionizing the era of biobased nanomaterials due to their surface, optical (Lin and Maggard, 2008), crystalline, mechanical, stiffness versatility, light weight, low toxicity, gelation and biodegradability (Kumar et al., 2023). The development of nanomaterials is often faced with challenges ranging from particle agglomeration to limited scalability of the production methods, environmental impacts and human health, hence the need to explore different approaches or options for their broad use on a large scale in the industrial sector.
Other barriers or challenges posed by biogenic NP use in water treatment include, the lack of data on their biotoxicity, scale-up, storage, bioaccummulation/biodistribution, quantity control, loading onto systems, etc. Critical to overcoming the aforementioned challenges bedeviling the industrial production and commercialization of nanomaterials include the availability/low-cost of the raw materials, environmentally friendly manufacturing processes, low production cost, the use of economical means of drying nanoparticle suspensions to recover the particles, avoiding redispersion ambiguities, adherence to international standards of toxicity measurements, adopting rapid/inexpensive characterization techniques, collaboration amongst producers and users as well as the efficient coordination of efforts by the government, industry and academia towards controlling market forces while ensuring an efficient market-pull for nanoproducts. With all of the aforementioned in place, alongside the availability of the needed resources, research and development, cum industrial-scale production of nanomaterials/nanoparticles will guarantee competitive production costs.
Despite issues related to instability of nanocatalysts or biogenic NPs, the stability and reusability of green NPs used in large scale water treatment processes can be ensured by ensuring that biogenic sources to be used for green NP production are sustainable and cultivated on large farm lands which will also culminate in high availability of the capping and stabilizing agents to be optimized during green NP-synthesis since bacteria, plants, algae and fungi are known to possess capping and stabilizing abilities for green NP syntheis. For the NPs used as catalysts, they can be produced on supports which help to increase the service life of each catalyst.
7 Toxicity assessment of nanoparticles/bionanoparticles
Nanoparticles’ sizes range from 1 to 100 nm (Nel et al., 2006). Advancements in technology has led to a drastic increase in their applications (Robertson et al., 2010; Thomas, 2014; Vinay et al., 2017), such as, as additives in paints, foods, ceramics, food packages, paper, drug delivery, cancer therapy and biosensors (Yang et al., 2010), as tumour detectors (Qian et al., 2008), paclitaxel (Gibson et al., 2007) as well as radiotherapy dose enhancers (Hainfeld et al., 2010; McMahon et al., 2011). Their increased demand is due to their small sizes and high surface area-to-volume ratios (Caruthers et al., 2007) which results in high chemical reactivity/reactive oxygen (ROS) production (Choi and Hu, 2008; Zoroddu et al., 2014). In recent times, nanoparticles/nanomaterials have attracted great attention due to their effects in the environment during production/disposal of consumer products containing them (Behra and Krug, 2008). Nanoparticles easily penetrate cell membranes and interfere with intracellular/metabolic activities (Hanley et al., 2009). The identification, detection and measurement of reactive oxygen species (ROS) is one suitable mechanism for determining nanoparticle toxicity (Wang et al., 2014a; Wang et al., 2014b; Elsaesser and Howard, 2012). Nanoparticle interaction with cells induce pro-oxidant effects that lead to the secretion of NADPH-dependent enzymes as well as mitochondrial respiration/ROS generation (Regoli and Giuliani, 2014; Jomova et al., 2012; Chen et al., 2011). Studies have also recorded that nanoparticle internalization/ingestion, leads to phagocytosis-induced production of ROS (Soenen et al., 2011). Some studies on nanoparticle toxicology/toxicity include toxicity assessments of nanoparticles to environmental microorganisms (Hegde et al., 2016), TiO2-NP toxicity (Berghe et al., 2013), AuNP toxicity (Berghe et al., 2013), risk monitoring of inhaled NPs (Bakand and Hayes, 2016), AgNP-induced mitochondrial toxicity (Maurer and Meyer, 2016) and toxicities of single-walled (Jain et al., 2012) and multi-walled carbon nanotubes (Kerfahi et al., 2015). Each article focused on a single nanoparticle’s toxicity on an organ without providing any detailed information on the toxicity assessments of higher organisms/cell lines. Elaborative discussions on the toxicity imposed by nanoparticles on organisms (rat, mouse, pig, guinea pig, human cell lines and humans have been discussed). Figure 4 is an illustration of different biogenic NPs and the experimental models adopted for their toxicity assessment.
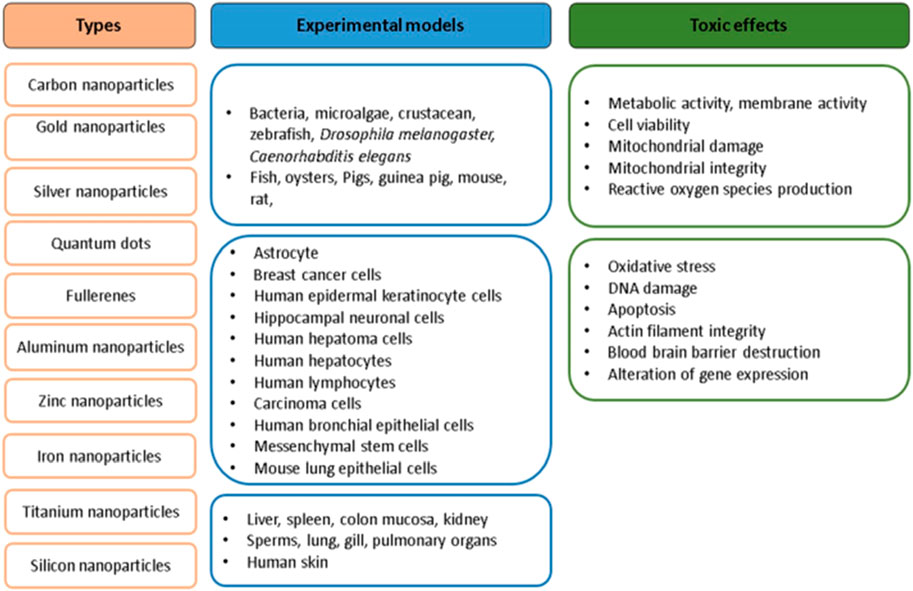
Figure 4. Biogenic NPs and the experimental models adopted for their toxicity assessments. Kumar et al. (2017); open access.
Toxicity assessment of nanoparticles can be classified as in vitro/in vivo assessments (Huang et al., 2004; Huang et al., 2011a; Huang et al., 2011b; Schiavo et al., 2016). Some advantages of the in vitro assessment scheme include, low costs, short time requirement and lesser ethical considerations (Huang et al., 2015). In vitro assessment can be subdivided into proliferation, necrosis, oxidative stress, apoptosis and DNA damage assay (Huang et al., 2017).
7.1 Proliferation assay
This employs 3-(4,5-Dimethylthiazol-2-yl)-2,5-diphenyltetrazolium bromide (MTT) salt for it’s in vitro toxicity assessment of nanoparticles (Sayes et al., 2007) and in turn measures cellular metabolism via the assessment of metabolites/active cells in response to bioNPs (Beveridge and Murray, 1980; Beveridge et al., 1996). The approach accommodates minimal model cell manipulation and gives fast/reproducible results (Marshall et al., 1995). The assay-measurements target tetrazolium salt and may sometimes encounter alterations in the measurements caused by changes in the prepared culture-additives (Molinari et al., 2003), pH (Jabbar et al., 1989), ascorbate (Natarajan et al., 2000) and cholesterol (Abe and Saito, 1999). Since MTT-assay produces formazan, assays such as XTT/WST-1 that can generate soluble synthetic dyes are preferred. Alamar Blue (AB) measures cellular redox potential compared to MTT-assay beause of its simplicity in terms of sample preparation (Punshon et al., 2005). However, literature has it that the success of (AB) may be hindered as a result of the unavailability of the assay’s biochemical stimulation of the interaction between non-porous silicon and (AB) in the absence of these cells (Low et al., 2006). The cologenic assay is another type of assay which makes room for proliferating cell-counts by visual inspection upon exposure to bioNPs (Casey et al., 2007).
7.2 Apoptosis assay
This happens to be one of the main markers that is usually observed in in vitro NP-toxicity assessment. DNA damage, oxidative stress in cell cultures and apostosis induced by BNPs are often caused by excessive free radical generation (Bortner et al., 1995; Collins et al., 1995; Ryter et al., 2007; Li and Osborne, 2008; Kumar et al., 2013a; Kumar and Dhawan, 2013b). In an in vitro study, apoptosis in the embryonic stem cells of a mouse was found to be induced by AgNPs (Ahamed et al., 2008). Apoptosis markers (i.e., caspase-3 and -9) were used to examine the larval tissue treatment of Drosophila melanogaster with AgNPs of 50–100 μg/mL at 24 and 48 h. In a later study, the findings revealed AgNP-interference within the apoptotic pathway of D. melanogaster (Ahamed et al., 2010). Upregulation of p38 protein expression occurred as a result of the timely and increased dosage of AgNPs in D. melanogaster; when this effects extended to the genes and DNA of the organism, they induced cell death/a cascaded apoptosis pathway (Liang and Clarke, 2001; Sherr, 2004). Methods for apoptosis assessment include Annexin-V (Lee et al., 2007), Comet (Jin et al., 2007), TdT-mediated dUTP-biotin nick end labeling (TUNEL) assays (Mo and Lim, 2005) and morphological change inspection (Pan et al., 2007). In addition, the DNA laddering technique aids in the visualization of the products of apoptosis of endonuclease cleavage (Wyllie, 1980; Arends et al., 1990; Suman et al., 2012). Irregular size reduction of cells coupled with DNA-fragmentation can initiate the inducement of apoptosis (Kerr et al., 1972; Abdel-Khalek, 2016). Annexin-V/propidium iodide (PI), an impermeable dye, is a cell death marker employed in toxicity studies. When Annexin-V binds to phosphatidylserine, it causes increased fuorescence which results in plasma membrane extrernalization of the organism, which is induced by the caspase-dependent pathway activation. PI is a dye that stains the nucleus when the cell membrane’s integrity is lost, and hence, is likened to the latter stage of apoptosis (Silva, 2010). In an investigation involving the AgNp treatment of human HepG2 hepatoma cells, changes in the nucleus’morphology with apoptosis induction were observed (Lu et al., 2011) and measured by Annexin V/PI in the AgNP treated HeLa cell lines (Miura and Shinohara, 2009; Baharara et al., 2016).
7.3 Single cell gel electrophoresis (SCGE) assay/comet assay
A tool that detects a test material’s/BNP’s mutagenic potential (Henderson et al., 1998; Kisin et al., 2007), alongside the induced in vitro and in vivo single-/double-stranded DNA breaks in the individual cells (Fairbairn et al., 1995; Singh and Stephens, 1997; Bajpayee et al., 2013; Kumar et al., 2013a). It quantifies DNA–DNA or DNA–protein cross-links, oxidative DNA damage (Lemay and Wood, 1999) as well as basic and alkali-labile sites (Kumar and Dhawan, 2013b; Dusinska and Collins, 2008; Pavanello and Clonfero, 2000). Based on the working principle of the assay, damaged DNA (tail) fragments and undamaged DNA (the head) imposed by BNPs will migrate out of a cell or remain immobile in the nucleus, when an electric current is applied to the cell. The degree of DNA-damage correlates the size/shape of the tail and fragmented DNA (head) in the comet (Malyapa et al., 1998). In this approach, the referred cells are lysed to expel cellular protein while the damaged DNA then migrates from the nucleus via electrophoresis. The samples are stained using DNA-specifc fuorescent dye whose gel is then analyzed for the measure of fuorescence inherent head, tail and tail-length (Singh and Stephens, 1997). The toxicity of ZnONPs of 25 mg Zn/L of D. tertiolecta was assessed using comet assay. The results indicated 55% damage in the cell-nuclei. A similar investigation was conducted with SiO2NPs in D. tertiolecta (125 mg/L) using comet assay and it was observed that after 72 h, the results revealed an increase in genotoxic effects as observed in another investigation by Pavanello and Clonfero (2000), whereas, when TiO2NPs were placed in D. tertiolecta, ,>72% nuclei damage was recorded after 72 h (Handy et al., 2012).
7.4 In-situ TUNEL (IT) assay
A method for detecting, apoptotic cells and DNA damage in in-situ TUNEL staining (Gavrieli et al., 1992). It is a method for staining cells in post-apoptosis programmed cell death/internucleosomal DNA-fragmentation (Loo, 2002). TUNEL assay uses the terminal of the deoxynucleotidyl transferase enzyme incorporated with labeled dUTP which generates free 3′-hydroxyl termini induced by DNA-fragmentation. DNA-destruction, caused by non-apoptotic events (i.e., necrotic cell death caused by the exposure to toxic compounds/biogenic nanomaterials or nanoparticles can be assessed using IT assay (Ansari et al., 1993) which have also been used to stain cells undergoing active DNA repair (Kanoh et al., 1999). TUNEL assay of pancreatic β-cells were used in the estimation of the islet function of the pancrease of Goto Kakizakirats upon treating them with daily insulin-loading of 25 IU/kg SeNPs for one fortnight. In another study, a decline of 17.6%–2.3% in apoptotic cells of similar rat species was observed after subjecting the cells to IT assay (Deng et al., 2017).
7.5 Necrosis assay
This assay assessment method is rapid, reliable, reproducible and inexpensive. It measures necrosis as a nanoparticle-screening criterion by examining the cell membrane integrity (i.e., the uptake of neutral red (Monteiro-Riviere et al., 2005) and trypan blue dyes (Bitensky, 1963) by BNPs which is a function of the cell’s viability. Neutral red also known as 2-amino-3 methyl-7-dimethylaminophenazoniumchloride, is a weak cationic dye with a deep red colour at sightly acidic pH. It can diffuse into the plasma membrane, concentrate in the lysosomes and bind to the anionic sites within the lysosome-matrix via hydrophobic electrostatic bonds (Nemes et al., 1979; Wang et al., 2013). However, the fragility of the lysosome may ensue from alterations induced by xenobiotics and nanoparticles in the cell surface (Lüllamann-Rauch et al., 1979; Borenfreund and Puerner, 2017) which may result in low uptake/binding by neutral red, thus making it possible to differentiate dead and viable cells (Borenfreund and Shopsis, 1985). According to an investigation, the endosome–lysosome stability dropped upon exposing the lysosome to 30% AgNPS (Miranda et al., 2017). In situations involving the trypan blue dye exclusion test, the dye enters into dead cells before being removed from the living cells (Strober, 2001). In an investigation involving the evaluation of the stability of a cell membrane, trypan blue dye exclusion assay was adopted, which was later improvised with different ZnNP sizes (i.e., 12, 61, 123, 184, 369 and 737 μM). The findings revealed that ZnNP concentrations ≥369 μM exhibited considerable cytotoxicity (Kononenko et al., 2017).
7.6 Oxidative stress assay
Nanoparticle exposure to cells can result in the production of reactive oxygen (ROS) and nitrogen species (RNS) (Magder, 2006). ROS/RNS detection entails reacting 2,2,6,6-tetramethylpiperidine (TEMP) with stable oxygen radical whose detection is aided by the X-band electron paramagnetic resonance (EPR) (Zang et al., 1995), however, the application of this method is limited due to high costs. Therefore, the emergence of an alternative/cost-effective approach that makes use of fluorescent probe was proposed (Gomes et al., 2005). Moreover, literature has it that some limitations of the fluorescent probe technique include inefficiency, caused by the high reactivity potential of the fluorescent probe molecules with a plethora of reactive species which may generate results that may be somewhat misleading (Halliwell and Whiteman, 2004). The aforementioned problem can be abated using a non-fluorescent probe [2′,7′-dichlorofuorescein diacetate (DCFDA)], which can react with HO, RO, ROO·and H2O2 molecules/radicals in the presence of cellular peroxidase (Wagner et al., 2007). In another study, lipid peroxidation C11-BIODIPY and Thiobarbituric acid (TBA) assays for malondialdehyde were combined for the assessment of oxidative stress (Fantel, 1996). Combining several assays during toxicity assessment of BNPs stimulates convenience, some of which include the Amplex Red assay, 5,5′-dithiobis-(2-nitrobenzoic acid) (DTNB) and the Nitro blue tetrazolium assays for the measurement of lipid hydro peroxide, antioxidant depletion (Kora and Rastogi, 2018) and superoxide dismutase (SOD) respectively (Hussain et al., 2006).
7.7 In vivo toxicity assessment methods for NPs
In vivo toxicity assessment procedures (biodistribution, haematology, serum chemistry, hispathology and clearance) are usually conducted using animals (mices and rats) (Kim and Baek, 2019).
7.7.1 Biodistribution
In biodistribution studies, the localization route of BNPs in the tissues/organs of dead rats/mices are examined via radiolabels (Kim et al., 2001).
7.7.2 Clearance technique
The clearance technique entails continuous monitoring of NP-excretion and metabolism by the organisms at different times of exposure (Li et al., 2001).
7.7.3 Serum chemistry
For the serum chemistry in vivo toxicity assessment, chemistry and cell type of the dead rat/mice were examined upon their exposure to BNPs (Baker et al., 2008). Histopathology of the cell/tissue/organ of the rat/mice is examined so as to ascertain the level of toxicity infused by the NPs (Lei et al., 2008).
7.7.4 Hispathology
The histopathology of exposed tissues (lung, eyes, brain (Delcroix et al. (2009), heart, kidneys, spleen and liver) of mice have been reported by Zhu et al. (2008). Some advanced toxicity assessments include the use of micro-electrochemistry/microfuidics (Ewing et al., 1983).
7.7.5 Cell viability and lethality
Cell viability and lethal tendency are two basic parameters used to examine BNP-toxicity. Of the available NPs, CNTs (single or multi-walled) are often adopted for viability and lethal assessment of cells as a result of their unique properties which in turn influence their wide patronage in the chemical, industrial and biomedical sectors (Guo et al., 2012; Sathyanayarana and Hübner, 2013; Madani et al., 2013). Some studies have documented the anti-microbial properties of CNTs in bacteria in which they observed that the CNTs infused mechanical damage to the sampled cells (Amarnath et al., 2012; Krause, 2014; Pasquini et al., 2012). Functionalized CNTs have also been found to affect soil-bacteria diversity (Kerfahi et al., 2015).
The toxicity assessment of the effect of SW-CNT in a micro crustacean (Daphnia magna), a fish (Oryzias latipes) and two freshwater microalgae, (R. subcapitata and Chlorella vulgaris) revealed hindered growth in C. vulgaris and R. subcapitata having SW-CNT concentrations of 30.96 and 29.99 m/L, respectively (Sohn et al., 2015).
FeONPs toxicity in human macrophages, murine macrophage (Hainfeld et al., 2010), hepatocellular carcinoma, and human/rat mesenchymal stem cells have been studied. The NPs had toxic effects on the murine macrophage cells bearing 25–200 μgNPs/mL concentration within 2 h of exposure with an attendant decrease in the cell’s viability (Naqvi et al., 2009).
Cell viability reduction was also documented for murine macrophage cells exposed to 7-day treatment using 0.1 mg/mL FeONPs (Jeng and Swanson, 2006), while in another investigation, rat mesenchymal stem cells treated with 0.1 mg/mL FeONPs over a period of 2 days revealed a reduction in the stem cell’s viability (Delcroix et al., 2009). SiO2NPs-toxicity to human keratinocytes at concentrations ranging from 30 to 300 µg/mL were evaluated using human keratinocytes (CHK), the results also suggested a reduction in the viability of the cell (Park et al., 2010).
Toxicity effects of SW-CNTs on the cell lines of human HEL 293, HEK, A549, human macrophage and human epithelial-like Hela cell lines have been investigated (Yehia et al., 2007; Fiorito et al., 2006; Davoren et al., 2007). The toxicity of CNTs in CNT treated lung fibroblast has also been evaluated. A549 cells exposed to 250–500 μg/mL SWCNTs over a 72 h period, resulted in oxidative activity/membrane damage induced by inflammatory response (Choi et al., 2009) and in vitro suppression of inflammatory mediators (IL-6, IL-8 and MCP-1) (Herzog et al., 2009). The toxicity effect of MWCNTS on human epidermal keratinocytes have also been investigated (Monteiro-Riviere et al., 2005), where they observed that the toxicity induced by the MWCNTs was mediated by pro-inflammatory symptoms facilitated by the transcription nuclear factor (NF-κB and ROS) (Ye et al., 2009). In vitro toxicological effects (oxidative stress, DNA damage and apoptosis) of MW-CNTs in the mammalian cell lines alongside VE-cadherin distribution and actin filament integrity in the human aortic endothelial cells have been documented (Cveticanin et al., 2009; Patlolla et al., 2010; Ravichandran et al., 2013; Reddy et al., 2010; Walker et al., 2009). AuNPs in contact with MRC-5 human lung fibroblasts were found to have induced autophagy and oxidative stress in the referred fibroblasts (Li et al., 2010). The cytotoxicity of Au nanorods and quantum dots/semiconductor NPs on animal cells was assessed based on cellular motility using the electrical cell-substrate impedance analysis. The results were validated using the dark field microscopy and fluorescence techniques (Tarantola et al., 2008). The toxicity of AgNPs coated with starch were observed on human lung fibroblast and (IMR-90) human glioblastoma (U251) cells. The findings revealed dose-dependent decrease in adenosine triphosphate (ATP) content and deoxyribonucleic acid (DNA) destruction which were induced by the deposition of AgNPs that altered the DNA of the cells followed by cell cycle arrest in the G2/M-phase (Asharani et al., 2008).
Cytotoxic examination of AgNPs on the fibroblast NIH3T3 cells revealed the inducement of mitochondria-dependent apoptosis with JNK activation and ROS (Hsin et al., 2008). AgNP toxicity effects on human hematoma cell line HepG2 was conducted using micro-nucleus test, DNA micro-array analysis and viability assay (Kawata et al., 2009). AgNPs in HeLa cells led to the upregulation of ho-1, mt-2A and oxidative stress genes (Miura and Shinohara, 2009). AgNP-treatment of E. coli revealed that the risk gene’s replication fidelity was compromised (Yang et al., 2009). CdTe quantum dots exposure on live cells over a long period of time revealed intracellular concentration of Cd2+ in human breast cancer cells (MCF-7), cysteamine-capped cadmium selenide-zinc sulphide (CdSe-ZnS) NPs, as well as N-acetyl cysteine and mercaptopropionic acid conjugated to cysteamine coupled with lysosomal destruction and ROS production (Lüllmann-Rauch et al., 1979; Cho et al., 2007). Surface coats of carboxylic acid on quantum dots in contact with human epidermal keratinocytes (HEKs) revealed the release of IL-1β, IL-6 and IL-8. The surface coating was confirmed to be the primary determinant of the immuno- and cyto-toxicity in the HEKs (Ryman-Rasmussen et al., 2007). CdTe, CdTe/CdS/ZnS core–shell–shell quantum dots and CdTe/CdS core shells were stabilized with thiols on the cell lines (HEK293T and K562) and the results showed that the quantum dots were severely toxic (Su et al., 2009). A neurotoxicity study on the effect of CdSe quantum dots using hippocampal neuronal culture model was carried out with the intent of observing the cytoplasmic-calcium and voltage-gated sodium channel-levels; the results revealed an increase in the cytoplasmic-calcium/voltage-gated sodium channel as well as the death of neurons (Tang et al., 2008).
CdSe quantum dot exposure to enterocyte-like Caco-2 cells as model intestine epithelium were investigated. Based on the results, acid treated polyethylene glycol (PEG)-coated quantum dots were seen to accelerate the cells’ toxicity (Wang et al., 2008). In vitro-in vivo toxicity assessment of CdTeNPs on human hepatoma HepG2 cells were conducted using AlNPs (Zhang et al., 2007). 1–10 μM AlNPs in contact with human brain microvascular endothelial cells (HBMVECs), showed that there was a decrease in cell viability and mitochondrial function with an increase in oxidative stress (OS) (Chen et al., 2008).
Mammalian cells treated with 10–400 μg/mL AlNPs were examined in relation to cell viability as a way of determining their toxic effects on the cells (Radziun et al., 2011). The cell’s viability was determined in relation to human bone marrow derived mesenchymal stem cell (HMSC) interaction with 25–40 μg/mL AlNP, which showed a reduction in the cell’s viability (Alshatwi et al., 2012). Increased concentrations of 500–2000 mg AlNPs/kg rat blood cells for 72 h revealed that the AlNP-toxicity increased with an increase in the NP-concentration (Balasubramanyam et al., 2009; Radziun et al., 2011). Mammalian cell lines were treated with 0–5,000 μg/mL AlNPs which were found to be responsible for the destruction in the cell’s DNA after 2 h (Kim et al., 2009). In addition to the aforementioned toxicity measurement methods, are other viable approaches that include those of well established standards for NP-testing by the Kupffer cell isolation protocol.
8 Global regulatory policies and established frameworks for the use of nanomaterials and nanotechnology-based products
Safety assessment being an integral part of product development also serves as a prerequisite for their release for use by mankind within systems and the environment. Hence, it is imperative to consider these elements early enough within the value chain. Nanotechnology entails product innovation which brings about enhanced material properties, reduction in material consumption, waste alleviation as well as emission reduction in the environment. Sharing research facilities and the results from nano-science/technology-based research, strengthens the science-base for the regulation and use of nanomaterials/nanotechnology-based products (Devasahayam, 2017; 2019; Hodge et al., 2009). Although, major inventions of nanotecchnolgy for food packaging, medicine, agriculture, water-treatment etc., are deemed huge successes in terms of their abilities to meet modern society needs, however, their associated adverse impacts are somewhat ignored, thus resulting in consequential threats to health (Jiang, 2019), the environment and society at large.
Some information on the Global Coalition for Regulatory Science Research (GCRSR) members [which include Argentina- National Administration of Drugs, Food and Medical Devices (ANMAT); Australia- Food Standards Australia Newzealand (FSANZ); Brazil—National Health Surveillance Agency (ANVISA); Canada- Canadian Food Inspection Agency (CFIA); China- National Institute of Food and Drug Control (NIFDC); European Union- European Commission, Joint Research Center (JRC) and European Food Safety Authority (EFSA); Japan- Food Safety Commission of Japan (FSCJ), Ministry of Health, Labour and Welfare (MHLW) and National Institute of Health Sciences (NIHS); Korea- Ministry of Food and Drug Safety (MFDS); Singapore- Singapore Food Agency (SFA) and United States- US Food and Drug Administration (FDA)] (US Food and Drug Administration, 2020a; US Food and Drug Administration, 2020b) have provided an overview on the regulatory landscape/framework and future challenges associated with he use of green nanotechnology.
EU’s nanosafety concept entails safe design and creation of NMs for future use (Lima Da Cuha, 2019). This implies that products of nanomaterials/nanotechnology ought to be safe for use throughout their entire life-cycle, from production through to waste, recycling and reuse (Krageloh, et al., 2018). The safe design concept was established for industrial innovations and formulated for nanomaterials within the confines of the EU flagship project NANoREG (Gottardo et al., 2017). The approach drives innovation using support mechanisms such as digitalized innovation hubs alongside open innovation test-beds. With research being core, the European Commission in 2017, via the establishment of digital innovation hubs, aims at becoming a one-stop-shop for companies/SMEs start-up/mid-cap companies with a market value in the range of 2–10 billion USD to invest in nanotechnology (i.e., testing instruments, receiving financial advice, adoption of market intelligence and networking opportunities) (European Commission, 2019). The established Open innovation test-bed of the EU provides access to physical facilities/services required for the development/testing and up-scaling of advanced NMs within industries (Lima Da Cuha, 2019). In addition, an independent agency funded by the European Union—the European Chemicals Agency (ECHA), addresses issues related to the safety assessment of chemicals such as manufactured NMs (European Chemicals Agency, 2019; European Chemicals Agency, 2020), under the European Chemical legislation REACH (registration, evaluation, authorisation and restriction of chemicals) EC 1907/2006 (European Union, 2009). The registration of chemicals/nanoproducts is based on information provided by companies, and the evaluation of the referred chemicals by the EU Member States (European Union, 2019). REACH is a part of EU legislation that addresses NMs/BNPs, as these products fall under pieces of legislation pacts on occupational safety, food packaging, feeds, biocides, cosmetics, water and health. In 2018, REACH was amended to include nano-specific information and modern provisions on chemical safety assessment and downstream user obligations (European Chemicals Agency, 2019). Till date, there are about 37 substance registration dossier information on nano-products with no transition phase for the implementation of the new requirements, nonetheless, fully harmonized/standard testing measures may still be lacking in terms of their availability. The ECHA hosts the European Union Observatory for NMs which provides the objectives and desired information on innovation as well as the safety aspects of NMs in the EU market (Sumrein, 2019; European Chemicals Agency, 2020). EFSA-assesses the risks associated with food and feed safety (EFSA, 2016; EFSA, 2020a; b), nutrition/health claims, animal health and welfare, biological hazards, contaminants, feed/food additives as well as plant protection/plant health even though some are exploited for use in the synthesis of bionanomaterials/bionanophotocatalysts/biogenic nanoparticles. The EFSA also provides scientific advice to the European policymakers and supports the regulation/implementation of nanomaterial application in relation to human, animal and plant-health. In addition, it adopts an environmental risk assessment measure to explore the possible impact of nanotechnology on the food chain, which in turn affects the biodiversity of plant/animal habitats. To redress issues related to the exploitation of nanotechnologies/NMs, the EFSA’ scientific network for risk assessment on nanotechnology application in food was established (EFSA, 2020b) which facilitates information exchange between EU Member States while prioritizing risk assessment activities. With its special team/group on NMs use in food and feed, there are published guidelines on the risk assessment in relation to the application of nanoscience/nanotechnologies within the food/feed chain. Regulatory aspects of nanotechnology in relation to agriculture, feed and the food sectors within the EU and non-EU countries were reviewed by Amenta et al. (2015). The European Medicines Agency (EMA)- defines nanomedicine as a field that bothers on medicinal products, purposely designed for clinical applications with an integration of at least one component at the nano-scale, that possesses specific definite proprieties/characteristics targeted at providing clinical advantages and other benefits ranging from dosage to drug targeting and reduced toxicity (Perez de la Ossa and Bremer-Hoffmann, 2019). Furthermore, the EU Commission’s Green Deal (European Commission, 2019) which drives the new industrial strategy for Europe, is a road-map designed for a climate-neutral/zero-pollution, circular, sustainable and an all inclusive economy (European Commission, 2020a).
Others include those of the US-FDA which define regulatory science as one that devolves new tools/standards and procedures for assessing safety, quality, efficacy and performance of all FDA-regulated products (US Food and Drug Administration, 2020a; US Food and Drug Administration, 2020b; US National Institute for Standards and Technology, 2020), including NMs and green NPs (Goering, 2019). The FDA-regulation on nanotechnology is to ensure that product developers/users accept and promise that risk and uncertainty abatement accompany all emerging forms of nanotechnology. There is a resolve not to introduce new/specific regulations for NMs only, while being hopeful that the existing framework is somewhat sufficient to regulate NMs/products sourced from them. Thus, horizon scanning/internal reviews of nanotech submissions are key components of the FDA approach.
The FDA provides core nanotechnology facilities with capacity for lab-testing which enables the administration of test methods/standards relating to safety assessment of NMs or newly developed biomaterials. The FDA CORES (Collaborative Opportunities for Research Excellence in Science) is a programme that fosters collaborative and interdisciplinary research on product characterization/safety assessment and the evaluation of scientific data and gap analyses for regulatory applications involving nanotechnologies in non-collaborative and collaborative researches. The outcomes of the programme provides for the preparation of documents that guide or support the industrial exploitation of nanotechnology (US Food and Drug Administration, 2020b) with intent on the development and recognition of apt frameworks, all aimed at establishing standard protocols within the nanotech industry/sector. Therefore, with this intent, the FDA in collaboration with other US government departments/agencies via the National Nanotechnology Initiative (NNI) seeks to dialogue with industry at the early product development phase of any nano-driven technology.
NNI comprises of 20 US departments and agencies, under the National Science and Technology Council and the White House Office of Science and Technology Policy Department. Its focus is on six core areas: NM measurement infrastructure, environment, human exposure assessment, human health, risk assessment and management, informatics and modelling. The policy document of the aforementioned department contains guiding information on workers’ safety and organized webinars related to the characterization and quantification of NMs (Friedersdorf, 2019). The National Nanotechnology Coordination Office (NNCO) alongside the EU commission, facilitate a science led initiative, which is open to all researchers globally, via the EU-US Nanotechnology Communities of Research (CoRs). This brought about the collaborative project of the EU Nanomedicine Characterization Laboratory (EU-NCL) – comprising of multiple European key professional laboratories and the US National Cancer Institute of Nanotechnology characterization laboratory (NCI-NCL), which in turn has accelerated the development of innovative therapeutic and diagnostic nanotechnology-based products for patients’ benefit across the globe (Borgos, 2019).
The US-FDA also holds bilateral agreements with organizations from Canada, India and other nations across the globe, on the characterization of NMs, amongst others via active participation in technical sessions hosted by the international standards organization. Despite the considerable progress made so far, the number of approved nanotechnology-based products is relatively low. In spite of the much scientific reporting on the findings related to nanotechnology-based products’ application in cancer research, there is need to intensify efforts in bringing such products to the market. Evidence has it that the FDA consistently receives new submissions of NMs containing products with many still in their clinical trial phases while some others are already approved for future drugs/medical devices (Tyner, 2019).
8.1 Landscapes/agencies with established frameworks and policies for NP-use in wastewater treatment
8.1.1 Canada
A number of Canadian departments/agencies including Health Canada, Agri-Food Canada, Environment and Climate Change Canada, as well as the Agriculture and Canadian Food Inspection Agency, have developed and established frameworks/protocols for the safe use of nanotechnology, their associated risk mitigation plans using an inventory NM-based product, and their assessment of biological effects bearing in mind a good understanding of how NMs/products containing them are consumed in relation to the products’ life-cycle and exposure. Reports have it that the Chemical Management Plan (CMP) of the Canadian Government has doled out regulations on new potentially harmful/existing NMs. Also, The Canadian nanomaterial regulatory protocol follows the mandate of the Organization for Economic Co-operation and Development (OECD) Council on safety testing and assessment of manufactured NMs (OECD, 2020a; OECD, 2020b) under the Canadian Environmental Act. In addition, the: Canadian Food and Drugs Act, Consumer Product Safety and Hazardous Products Act, Fertilizers Act, Pest Control Products Act, Feeds Act, as well as the Health of Animals Act also entail considerations for NMs (Health Canada, 2020). There are a couple of similarities regarding the US and Canadian concepts/classification of NMs, hence the need for the close regulatory council-tie between both countries dubbed, the US-Canada Regulatory Cooperation Council. The council has developed an approach that prioritizes actions on the conduct of a harmonized NM-risk assessment in variance with what obtains with respect to products beyond the nanoscale. Nanomedicine-evaluation, which is aimed at targeting drug delivery/gene therapy and diagnostics, rests within the purview of the Canadian National Centre of Excellence. Canada, a major contributor to the development of nanomedics for chemo and gene therapy, has revealed that non-viral NP-systems are helpful in the delivery of genetic information which facilitate the drug manufacturing process at lower costs. In lieu of the aforementioned, a couple of regulatory challenges still subsist, especially in cases where these drugs are designed for single or small groups of patients (Cullis, 2019).
8.1.2 Asia
The stipulations of regulatory science in relation to health in Japan is the responsibility of the: Japanese Ministry of Health, Labour and Welfare (MHLW), Agency for Medical Research and Development (AMED), National Institute of Health Sciences (NIHS) and the Pharmaceutical and Medical Devices Agency (PMDA). The PMDA, houses a centre that was established in 2018, which promotes innovative methods/advanced therapies integrated with nanotechnologies which assists regulators in keeping pace with novel developments. Research findings on cutting-edge pharmaceutical nanoproducts are regulated by the Promotion of Healthcare Industries and Advancement of Healthcare Technologies Act. In the year 2019, the Indian government released some guidelines for the evaluation of nanopharmaceuticals with the scientific rationale for developing new/existing drugs, profiled in vitro and in vivo methods, safety, drug efficacy, toxicity profile, drug dosage/frequency of administration, patient-recovery, cost implications and other benefits (Dinda, 2019). The National University of Singapore, supports drug developers and regulatory bodies in conducting clinical and laboratory tests towards identifying qualitative attributes of nano-medicines/nanovesicles/drugs drawn from clinical trial data which in turn provide information on which in vitro and in vivo ptotocols will provide relevant biomarkers for optimal clinical performance. However, some underlying challenges such as the accurate prediction and elimination of long-circulating liposomes, increased the rate of complex in vitro models which do not improve clinical performance predictions, and low data availability on the characterization of nano-carriers (Wacker, 2019).
8.1.3 Chile
Similar to a couple of existing citizen-science-project protocols in the EU, including the European Plastics Pirate Project (European Commission, 2020b; European Commission, 2020c), many institutions located on Chile main and Eastern Islands are involved in the‘National Sampling of Small Plastic Debris” in response to a dire need to establish a baseline for nano-based products such as micro/nanoplastics in the marine environment at a fish farming site in southern Chile.
The International Pharmaceutical Regulators Programme (IPRP), organized by the International Council for Harmonisation of Technical Requirements of Pharmaceuticals for Human use (ICH) identified/addressed issues of shared interest that bother on pharmaceutical regulation ranging from nanomedicine to NM use in drugs, borderline/combination products alongside procedures for their development and performance-evaluation (International Pharmaceutical Regulators Programme, 2020). The membership/organizers of the IPRP include government/agency representatives from America, Oceania, Asia, and Europe; its objectives include non-confidential information sharing/regulation-harmonization, international regulators’ training collaborations and community-outreaches to innovators/other stakeholders in nanomedicine (Johnston, 2019). It is pertinent to note that what needs to be reported on NMs by regulators include the necessary metrics based on particle size/number/weight, which depends on the applicable regulation in relation to the specific sector’s jurisdiction. For instance, particle size distribution/particle spread is a major requirement for identifying NMs/their products based on the EU-REACH legislation, whereas, the weight fractions/concentrations of nanoscale particles determine whether reporting a material with USEPA falls under the US Toxic Substances Control Act (US Environmental Protection Agency, 2017). However, both metrics may be required for the documentation of a nanomaterial within the European Commission Cosmetics Product Notification Portal (CPNP).
9 Proposed policy directions
Considering the fact that there are a few existing frameworks without a globally unified policy on the use of NMs in relation to human subjects and water treatment. The following directives are herein proposed for enforcement:
- ethical approval need be sought prior to conducting studies involving green NP use on human subjects.
- there must be a statement to ascertain the level of toxicity or risks associated with every biogenic NM/green nanopartcicle (McNamara and Tofail, 2017; Mech et al., 2020a; Mech et al., 2020b). In lieu of the fact that, most of the toxicity studies reported in literature, did not reveal the sources of the NPs, most of the referred NPs were synthesized from chemical precursors and not from plant or organic sources, hence, studies are still ongoing in a bid to ascertain the toxicity/non-toxixity of biogenic nanomaterials/green/bio-sourced nanoparticles (Lynch, 2019).
- bionanoparticles are perceived to be somewhat biodegradable (Iram et al., 2010), hence exhibit a low level of bioaccummulation potential. Although studies are yet to be conducted to ascertain this fact, efforts can be directed to examine the biodegradability of biosourced NPs/BMPs which will give a clue as to whether they constitute any form of environmental nuisance when discarded or may bioaccummulate in human cells and cause adverse effects when used as treatment aids (Patra et al., 2018).
- every NP-synthetic methodology must comply with global best safety standards/protocols, methodology and proceure.
- Zero-hazard/risk and pollution tolerance: every synthesized biogenic nanoparticle should not be hazardous or pose any health risk but must turn out being a non-contaminant/non-pollutant in soils or environmental waters when they come in contact with these systems (Jeliazkova et al., 2015).
- The government of every nation/management of research institutions must set out guidelines and legal frameworks (Nelson, 2019) that will address the challenges associated with classifying nanoparticles, as well as ensure the safe production and consumption of biogenic nanoparticles/nano, materials (Jantunen et al., 2017). These systems will impose fines, bans, litigation, seizure of production facilities and closure of firms, organization of professional training and jail-sentence for any lack of compliance.
10 Sustainability criteria for Apt implementation of biogenic nanotechnology for wastewater treatment
Considering the variability of the treatment outcomes of nanoparticles and the need for sustainable treatment approaches, Kamali et al. (2019) proposed a Fuzzy-Delphi approach for selecting the best wastewater treatment technique amongst a list of criteria; the criteria can be adopted for selecting the best biogenic nanomaterials/nanoparticles/bionanocatalsyst for the treatment of any wastewater sample. Considering the work of Kamali et al. (2019) with a few modifications by the authors of the current study, the criteria for selecting apt/sustainable techniques for treating wastewater can be ranked in the following order of importance: Health and safety risks > Treatment efficiency > Possibility of combining techniques > Ease of implementation > Material/green NP stability > Solid waste generation tendency > Potential for chemical substance release > Potential for CO2 emissions > Water reuse potential > Potential to recover by-products > Initial investments > Equipment maintenance costs > Operating cost > Odour impact > Noise Impact > Visual impact > public acceptance.
11 Industrial application of Bio-NMs: case studies on large scale industrial use of green NPs for wastewater treatment
11.1 Fe3O4 NPs
Fe3O4 NPs are being used in various industries including wastewater purification/treatment. They offer advantages ranging from tiny sizes for high molecular absorption to large surface areas for heavy metal-elimination from water (Guiterrez et al., 2022). The small sizes of NPs enable their effective removal of metals from water effluents of industries (mining and manufacturing industries). Reports have shown that coating Fe3O4NP with chitosan, derived from chitin, enhances the NPs removal of organic pollutants from water.
11.2 TiO2NPs
TiO2NPs have been used extensively as photocatalysts for environmental remediation (Chong et al., 2010), antimicrobial and energy generation applications. They can degrade and eliminate organic pollutants and bacteria found in air/water by the aid of ultraviolet (UV) light (Rathore et al., 2023).
11.3 Co/Co3O4NPs
Co/Co3O4NPs have been proven to exhibit antimicrobial properties against staphillococcus found in water. They have been used in the medical, food, and water industries. Youseffu et al. (2021) investigated the catalytic and peroxidase-semblance of Co3O4NPs in the industrial processing of water involving hydrogenation, reduction and oxidation reactions.
11.4 ZnONPs
ZnONPs being essential for both plants and humans have found applications in the cosmetic, electronic, food, medicinal, and process industries. It was discovered that ZnONPs were able to rid off contaminants from wastewater that may likely impede wheat growth (Haidri et al., 2023). Furthermore, ZnONPs have been adopted as antibacterial agents to fight against infectious disease-carrying vectors in water.
11.5 AgNPs
AgNPs are highly active against bacteria by preventing DNA duplication/cell division or multiplication of harmful microbes (staphillocccoccus aureus- ATCC25923, gram negative bacteria (Ecoli—25,922) in wastewater (Dhanker, et al., 2023). AgNPs have high catalytic activity due to their large surface area and unique electronic properties (Das et al., 2013). They can act as catalysts for the reduction of harmful pollutants and based on their high surface area to volume ratio, they have been used in the removal of harmful viruses and bacteria during water purification. They have also been incorporated in filters and added to industrial water tanks to prevent bacterial growth.
11.6 Nanaochitosan
Chitosan NPs possess physico-chemical characteristics and specially differentiated structures for the effective catalytic reduction of organic pollutants found in industrial wastewater (Balik and Serdar, 2016; Karthiga Devi et al., 2016; Nakum and Bharracharya, 2022). Other applications of biogenic NPs are as illustrated in Figure 5.
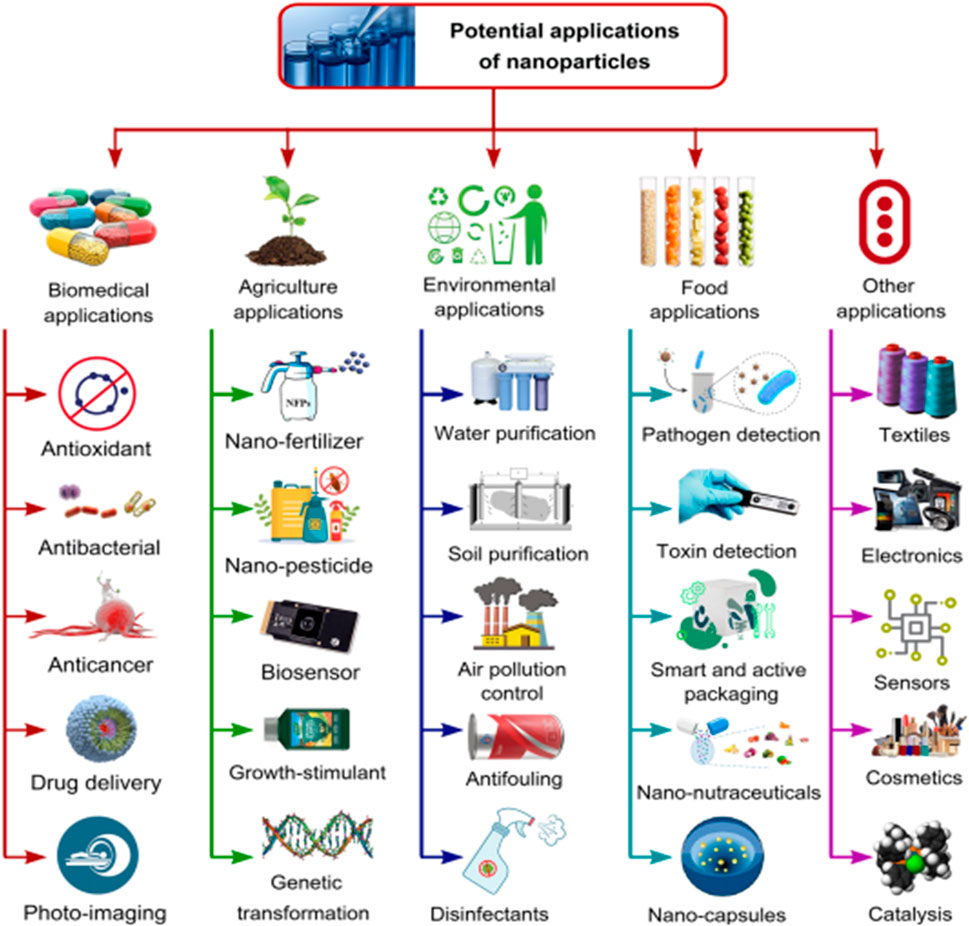
Figure 5. Applications of green nanomaterials in various industries and sectors. Osman et al. (2024); open access.
12 Issues related with economics and commercialization of green NPs/NMs for large scale water treatment applications
12.1 Initial investments
Initial investments, such as cost of land, civil works, equipment, etc. Are important in deciding the fate of the industrial relevance of a technology/application, especially as it relates to the treatment of wastewater (Ren et al., 2017; Molinos-Senante et al., 2010; Molinos-Senante et al., 2012; Kalbar et al., 2012; Jozsef and Blaga, 2014). There are relatively few studies on the economic feasibility/viability of various nano-treatment technologies. Buyukkamaci and Koken (2010) treated several types of pulp and paper mill effluents and conducted a detailed analysis on the required initial investment costs. They asserted that, in terms of economic/technical feasibility, the best method for treating medium strength effluents is the up-flow anaerobic sludge blanket (UASB) technique followed by an aeration basin, while for the high strength effluents they proposed the combination of a UASB method with an aeration basin/in connection to a conventional activated sludge process. With respect to large scale nano-based wastewater treatment technologies, there is the need for more research works on ascertaining the required initial investment costs and this is somewhat lacking in the existing body of literature for nano-based water treatment plants. Therefore, technical specifications may become helpful to determine the amount of initial investment required, where the kinetics of the treatment process can be adopted for design considerations and size of reactor needed alongside the desired nanomaterials (Sathian et al., 2013). Looking at it from a technical point of view, the assumed capital costs/total direct plant cost, such as land, buildings, equipment and the associated indirect costs (i.e., cost of supervision), of the nano-enabled treatment plants can be compared to those of advanced oxidation/Fenton treatment techniques, in order to make rough estimates of the investments required. The comparative investment costs of several chemical oxidation effluent treatment plants from olive oil mills and fine-chemical manufacturing plants was conducted by Cañizares et al. (2009) and they observed that the nature of the effluents had a remarkable influence on the investment cost required, whereas, the associated capital cost of treating the effluent via electrochemical oxidation was worth €15 × 103/m2 in comparison to Fenton oxidation; based on their findings, the capital cost can be taken to mean the rough estimate of treating such effluent using emerging biomaterials (ENMs). However, if enhanced efficiency of the ENM-based treatment is desired, the reactor can be re-sized and made smaller which will also impose a reduction in the capital cost (Adeleye et al., 2016).
For the operating costs, nano-based treatments include costs of purchase/synthesis of ENMs, utilities (i.e., electricity for photocatalytic treatments) and labour. Other costs such as NM-cost have been found to be highly dependent on the source and properties/characteristics including purity (wt%), particle size and surface functionalization of the formed NPs. Reports have it that TiO2NPs used in wastewater treatment plants are priced within the range of 0.03- $1.21/g, with the corresponding treatment costs ranging from 0.50-$1.00/g pollutant (Adeleye et al., 2016). The low cost ($0.05–0.10/g) purchase of nano-zero-valent iron (nZVI) NPs is premised on the improvement in the technology involved in its production process. Whereas, in terms of synthesis, micro/bulk zero-valent iron is considerably cheaper (i.e., $0.001/g pollutant) (Hoag et al., 2009; Crane and Scott, 2012). Magnetite NPs used in a continuous flow process have been estimated to be worth € 0.0035/g by Simeonidis et al. (2015) and in lieu of their large scale production costs, finding cheaper feed stocks for the development and synthesis of green and cost effective ENMs at reasonable costs has become a matter of urgency. Also, the possibility of the recovery, recirculation and reuse of NMs via ceramic membranes can potentially reduce the total cost of producing them (Gehrke et al., 2015; Zinatloo-Ajabshir et al., 2018). Pires et al. (2015) compared the cost of different NPs (copper oxide catalysts) on different supports (γ-Al2O3, pillared clay/TiO2). CuO supported on γ-Al2O3 was found to be the most cost effective material with an estimated treatment cost of 0.07 $ at 0.175/g of metal for 80% pollutant-removal. Therefore, comparative treatment costs with different NMs is mandatory in order to decide on the optimized cost for the purpose of commercialization. According to Pirkarami et al. (2014), the large scale treatment of 1 m3 of dye solution using UV/Ni–TiO2 of NM, required an energy consumption of 4 kWh/m3 estimated at 0.042 USD/kWh with a total estimate of 0.168 USD/m3. However, in landscapes where the cost of electricity is high, the use of UV light active NMs (N-TiO2) or solar energy is preferred (Yoshida et al., 2014). While labour costs in relation to NM use in water treatment plants are scarcely available in literature, Long et al. (2018) observed that, for the treatment of wastewater effluents from industrial parks in Taihu and Haihe water basins (China), the associated operating costs of the treatment plants can be ranked in the following order electricity > labor > used chemicals. Li et al. (2017b) also observed that these costs also vary regionally.
12.2 Maintenance costs
Emerging or green NMs for industrial wastewater treatment may impose significant reduction in the plant’s maintenance costs. For instance, when membranes are employed in wastewater treatment plants, membrane-fouling by inorganic suspended solids, dissolved organics/biofilms all add to the overall maintenance costs. However, the single use/incorporation of NMs in the structures of the membranes is one sure way of mitigating against such risks. Lifen et al. (2012) incorporated TiO2 in polyvinyl alcohol (PVA) for the treatment of industrial wastewater and found that the composite membrane had improved hydrophilicity/anti-fouling property; they also asserted that low solid waste generation has the potential to reduce maintenance costs that may be incurred from sludge thickening and dewatering systems/facilities. Since green NPs/ENMs used in the treatment of industrial effluents are still far from being evaluated in terms of their negative consequences under real-life scenarios, suggestions are in view to encourage studies in this direction so as to have a holistic/more realistic assessment of the associated maintenance costs of the systems involving their use.
12.3 Environmental considerations
12.3.1 Solid wastes generation
Several treatment technologies (primary, secondary, and tertiary) for industrial effluents have been investigated in terms of their treatment efficiencies and capacity to cut down the quantity of final wastes generated. Activated sludge treatment from industrial effluents generates a large amount of waste (Kamali and Khodaparast, 2015) and generally produces activated sludge which increases disposal costs (Balik and Serdar, 2016). Thus, it is preferable to adsorb pollutants/heavy metals using NMs where the recovery of the adsobates (heavy metals) and adsorbents (NMs) may prompt the reusability of the latter without the generation of any waste.
CNTs and nZVI were used by Vilardi et al. (2018a) to recover hexavalent Cr, Se and Co from aqueous solutions and observed that the NMs or Fenton-like process may yield products such as H2O and CO2 with no waste generated; this is in sync with the observations from the photocatalytic degradation of industrial effluents by Yu et al. (2005), Sudha & Sivakumar (2015) and (Kumar et al., 2015). The disposal of NMs is another means of solid waste generation, hence, the need to begin to consider the use of biogenic NPs/NMs with biodegradation potentials/complementary potentials to soils when discarded.
The release of chemical substances/by-products into the treated industrial effluents is another issue that needs attention during NM use in the treatment of industrial effluents. Recently, chemical methods based on the production of hydroxyl radicals which help in eliminating recalcitrant organic pollutants are being discussed. Fenton oxidation which takes advantage of Fe2+/Fe3+ generation alongside H2O2, combined with Green NMs have been widely adopted for wastewater treatments due to their short reaction time (Kuang et al., 2013; Koba and Biro, 2015), amid the generation of secondary pollutants caused by the release of Fe2+/Fe3 in the treated effluent (Sze et al., 2005). To resolve this problem, additional treatment techniques may be employed (Kuan et al., 2015), such as the use of other inorganic NMs such as TiO2 and copper-based NMs, which can induce ferric ion dissolution during the process (Adeleye et al., 2014); other techniques include pH, crystallinity, temperature and particle size moderation/control (Schmidt and Vogelsberger, 2009). Others include CO2 emission (Chiemchaisri et al., 2007) and water reuse potential of the treated industrial/chemically complex effluents, such as those from metal-works (Jagadevan et al., 2012) including highly toxic compounds with good potential for by-products recovery.
12.4 Social considerations
These include determining the odour impact of industrial wastewater by detecting the smell of malodorous compounds [i.e., volatile fatty acids (VFA)] with adverse olfactory effects (Ronteltap et al., 2011). Some successful applications of green NM photocatalysts for the elimination of odorous compounds include the works of Bordbar, (2017); Bordbar and Mortazavimanesh, (2017b); Bordbar et al., 2018, Li et al. (2018), Muthukumar et al., 2017; and Azzouz et al. (2018).
12.5 Noise and visual impacts
Studies have shown that the use of NPs in wastewater treatment plants cited near a crowded neighbourhood may impose social and environmental disadvantages (Zock et al., 2018). The toxic constituents that may ensue from the interactions may result in the release of volatile undesirable products that induce noise nuisances generated from wastewater treatment process units/plants which may affect nearby residents, however, some decibel-coded models can help detect safe and unsafe noise levels within such vicinity (De Heyder et al., 2001).
12.6 Social acceptability
Another criteria that will significantly influence the commercialization of green NM technology for the sustainable treatment of industrial wastewater is the inherent social benefits, besides its technical, environmental/economic viability (Ren et al., 2016). Gupta et al. (2012) received the opinions of respondents in North Western Europe in relation to the factors that may influence the societal acceptability of various NMs. Their conclusion implied the need for any adopted NM technology to be beneficial, useful, and able to address societal needs (clean water availability and abundance).
13 Sustainable approaches and future research directions on the use of green NPs for water purification
Investigations on the use of green-synthesized and biogenic NPs for wastewater remediation in membrane bioreactors, sewage systems, treatment plants, and other water treatment systems help to provide information on dangerous contaminants/pollutants in water resources (Zhang et al., 2019; Philipse and Maas, 2002). Since commercial application of biogenic NPs in water treatment have continued to face difficulties, which are imposed by sedimentation, stability, aggregation, and size control phenomena, such issues can be tackled using nano-films/nanocatalysts, and nano-adsorbents because they are effective in the removal and degradation of pharmaceutical pollutants (Kim et al., 2007; Rizzo et al., 2009) heavy metals, inorganic, organic, radioactive, nitro compounds (e.g., nitroarenes) (Gnanaprakasam and Selvaraju, 2014), nitrophenols (Khoshnamvand et al., 2019), nitrates (Tyagi et al., 2018), phosphates (Xu et al., 2020), and hazardous dyes like rhodamine B (RhB), Congo red (CR) (Vidya et al., 2017), methyl orange (MO) (Robati et al., 2016) and Eosin Y (EY).
The behaviour of NPs in the environment and living cells depend critically on their sizes, shapes, monodispersity, surface charges, plasmonic responses, medical diagnostics, biofunctionality, and catalytic activities as well as the controlled synthesis of the designed green products by safer processes, while maintaining NP efficacy and efficiency, is one of the most difficult and persistent problems to be solved in the development and deployment of novel green NP-synthetic protocols (Lai et al., 2016). Since the organisms used in NP synthesis can range from simple prokaryotic bacterial cells to complex eukaryotic organisms, developing green, sustainable synthetic routes for the production of metal NPs still requires extensive research and innovative solutions. However these approaches need be refined and optimized for improved efficiencies as occasion demands. It is the diversity of these NP-systems that poses the greatest barrier to their widespread and routine application in sustainable green wastewater treatment operations. Since green extracts vary in terms of type, quality, concentration, alongside reagent ratios, reaction conditions (i.e., time, temperature, and pH), yield, and product characterization which are lacking or inadequate, concerted efforts ought to be made in relation to making accurate comparisons of NP-performances in these systems. The regulation of crystal growth, size and morphology, dispersity, and nanoparticle stability are advantageous to NPs since they induce property modifications for improved wastewater treatments (Dauthal and Mukhopadhyay, 2013). In lieu of the fact that most published studies on green NP synthesis from microbial/plant extracts have provided dependable proofs on the latter’s toxicity, only a small number have reported on their comparison with conventionally produced NPs (Naraginti and Sivakumar, 2014; Husein, et al., 2019; Qu et al., 2017). So, it has become pertinent to acquaint with existing techniques and develop new ones for ascertaining the toxicities of green NPs while also exploring ways of mass-producing them safely to meet industrial demands. To this end, researchers need to focus their energy on developing precise and dependable synthetic protocols for detecting, controlling and abating the toxic effects of biogenic NPs (Vellaichamy and Periakaruppan, 2016; Dauthal and Mukhopadhyay, 2015; Renuka, et al., 2016). The properties of green NPs sourced from microorganisms/plant extracts vary greatly, hence, a proper selection of apt reducing agents is vital at the production phase of BNPs (Vennila and Prabha, 2015; Moulton et al., 2010; Bano et al., 2018; Al-Ruqeishi et al., 2016). In order to maintain high reproducibility and thus guarantee good performance of NPs, a large scale synthesis from plant species of the same type, quality and composition must be adopted in each synthetic cycle. Some studies have revealed that different tea extracts of unique compositions of caffeine/polyphenols, acted as both reducing and capping agents during green NP synthesis from the extacts, thus resulting in NPs of controlled shapes and sizes (Al-Ruqeishi et al., 2016; Bonigala et al., 2018; Reddy et al., 2018; De Corte et al., 2012). In addition, the enforcement of standard protocols and guidelines for the characterization and qualitative/quantitative quantification of plant extract-compositions used in NP-synthesis for wastewater treatment will help overcome some of the associated barriers posed by their potential toxins. It is also crucial to take into account the optimization of the influential parameters during green-NP synthesis for qualitative synthesis of NPs (Kaliraj et al., 2019; Peng et al., 2019). High yield and production rates are crucial factors in the successful industrial application of microorganisms or plants for the synthesis of metal NPs. Therefore, it is important to carefully regulate and optimize the extract-to-salt concentration/ratio, as well as the production time, pH, temperature, buffer concentration, and stirring velocity. Nanoparticle size, shape selectivity, and concentration are all controllable by adjusting the amount of green extracts precursors (Shen et al., 2017; Zare et al., 2019). The estimation of the expected NP-features may be difficult/complex when the green solution composition is high. Most of the time, it is either impossible to completely isolate the components of the green extracts from the extracting solvents, thus leading to contamination of the biogenic NPs; however, this can be abated by employing combined techniques with synergistic or follow-up complementary separability/affinity of the solvent used without jeopardizing the quality of the green NPs. As the stirring time increases, the average particle size also grows an increase in temperature decreases the mean particle size and number of NPs (Ijaz et al., 2017; Bordbar, 2017; Bordbar and Mortazavimanesh, 2017b; Bordbar et al., 2017; Bordbar et al., 2018). pH affects the binding of metal ions to biomolecules of green materials, which in turn leads to the formation of particles with a wide variety of shapes at different pH levels. In most cases, lower sized biogenic NPs can be synthesized at lower temperatures and higher pHs, since high temperatures may be undesirable. Nonetheless, since extracting and purifying the synthesized NPs from intra-cellular and extracellular living/non-living biological sources including bacteria (Zhang et al., 1996), fungi, and yeasts is herein a major concern, the development of topnotch intra- and extracellular approaches coupled with less-energy-intensive physical and/or chemical extraction techniques on preference in relation to heating, freezing, thawing, sonication, osmotic shock, as well as multiple centrifugation and washing of the NPs need be established (Andjelkovic et al., 2017; Lloyd and Macaskie, 1996; Karthika et al., 2017) because these processes involve multiple steps that require a great deal of energy and solvent, which may give rise to excessive wastes. As a result, the proposed technique will limit the level of refinement imposed by these wastes on the inherent/superficial characteristics of the NPs (Salehi et al., 2019; Martínez-Cabanas et al., 2016). NPs may form aggregates, sediment, and precipitate under certain conditions, thus resulting in undesirable and uncontrollable properties and behaviours. Enzymatic lysis is one method used in extracting NPs; however, particles formed in vitro bacterial cells are typically less stable compared to those formed in vivo, and thus, severe aggregation may accompany the extraction step. Additionally, this purification method has not been up-scaled for industrial production because of the anticipated costs. In terms of both production efficiency and ease of purification by filteration/centrifugation, the extracellular method of NP production is preferrable hence, strategies for up-scaling this technique with low cost implications must be deployed to address this concern (Ali et al., 2016; Sinha and Ahmaruzzaman, 2015; Mittal et al., 2013; Karthika et al., 2017). For instance, Pseudomonas was used in the extracellular synthesis of AgNPs which were precipitated by centrifuging the resulting nano-suspension for 10 min at 12,000 rpm and 25°C (Pradhan et al., 2001; Varadavenkatesan et al., 2019). In this type of synthesis, particle aggregation is quite common, but it should be noted that applying these purification steps can also alter the stability of the NPs. The possession of NP-capping and stability characteristics by plants prevents the aggregation of green NPs (Naraginti and Sivakumar, 2014). When thinking about how to scale-up the process of creating green NPs, it is important to consider how the purification process impacts and alters the NPs’ properties. In addition, the toxicity and life cycle assessments of green NPs need be ascertained via quantitative analyses of the cost implications and consequences of adopting these synthetic-protocols (Sreeju et al., 2017; Ali et al., 2016; Wu et al., 2019). The main limitation of conducting these analyses is the dearth in the specificity of such information. Detailed information on green NP synthetic pathways, toxicity, sources, mechanism, characterization, industrial application, bioaccummulation, environmental and health risks are lacking, and neither is there any experimental data covering all stages of a particular biogenic NPs’ life cycle. Since the reduction mechanism of metal ions to NPs has not yet been elucidated, considerations for the reducing agent as a defining factor during NP-synthesis is often excluded from most life cycle analyses-calculations, thus resulting in constrained modeling and, ultimately, an incomplete assessment of such processes (Naraginti and Sivakumar, 2014). Also, the lack of uniform regulations at the national or international levels that would help curb such issues in terms of describing the quality/origin of the materials, restricting the conditions for NP production, standardizing and controlling the quality of the NPs formed, as well as determining their use and long-term effects, further muddles the assessment of the green methods that engender NP-synthesis. Thus, it is a challenging task to design, estimate, and compare the behaviour of NPs based on the different properties exhibited by various green entities. Given these obstacles, more in-depth study is required to establish uniform, safe, and cost-effective green synthetic methods, as well as determine the effects and potential toxicity/innocuousness of the designed and obtained BNPs. In recent times, hybrid NMs have become trendy in wastewater treatment applications, which in turn suggests the need to explore the possibility of adopting the synergistic properties of hybrid nanobiogenics for the production of hybrid nanoparticles which have great prospects in water purification as this will help to curb issues related to the abundance of some species relative to others, thus ensuring longevity and sustainable application of synthetic NMs for water treatment purposes.
Despite the several efforts channeled towards harmonizing the guidelines/analytical approaches for quantifying NMs alongside their risk-assessments, the unprecedented increase in medical nano-based products and their number of agri-/food sector-applications/issues and their associated risks need be given attention by regulatory authorities/sponsors of research. From a technical, regulatory and policy perspective, biogenic NP application is not yet in the market, owing to the fact that researches conducted thus far, are either in their early stages or are technologically oriented, but lack considerations for potential risks and safety/hazards. As the development and application of biogenic nanomaterials/NPs advances, their associated risks and benefits ought to be accounted for. A holistic way of addressing concerns related to the risks and potential benefits associated with the use of complex nanomedicine is necessary if the regulatory/scientific community and research funder must work in synergy, to ensure testing the nanomaterials/nanoproducts rigorously while also ensuring that the methods used in producing the products are reproducible and compare favourably with standard methods and risk assessment plans. Furthermore, specific analytical/complementary equipment need be adopted for the assessment of specific complex products in relation to their toxicities and potency; for instance, every pharmaceutical company needing such products, must ensure that all regulatory authorities are involved at the early stages of the development of the products and not at their final synthetic stages. The need for public-private partnership cannot be overemphasized, especially with respect to the new nanomedical solutions for patients. Currently, the available legal frameworks begin considerations on the specificity of new nanomaterials and their impacts. However, safe design is an appropriate mechanism or check for such materials, especially with respect to beginning early in the innovation process while balancing safety with functionality of the nanomaterial/nanoproduct. As a way of facilitating the implementation of safe design as a strategy, effective dialogues with stakeholders and regulators must ensue regarding all anticipated innovations in a trusted environment in order to ensure that there is apt regulatory preparedness and governance in relation to the possibility of having cheaper, fast, effective and safe products in the market. With the recent widespread increase in the use of nanomaterials, issues related to definitions, reuse, containment, toxicity and bioavailability are of major concern. However, literature has it that since green NMs and NPs are from biosources, they are generally deemed to have low toxicities or hazard potentials; nonetheless, their actual effects have to be quantified so as to have a clear perspective of their toxicities and hazard potentials. Despite the concerted efforts by researchers to address issues related to terminologies, definitions, characterization, sampling and assessment of their toxicity, hazard potential and impact, the associated regulatory challenges include lack of a globally accepted standardized nomenclature, assessment routines and characterization, which have resulted in a global divergence in regulatory approaches on these fronts. Therefore, apt alignment/harmonization of regulatory and legislative stipulations must be complied with by all stakeholders including scientists, standard organizations, regulators, patient representatives, industries and consumers in creating a globally relevant/harmonized regulatory system/framework for NM/NP production and use. In addition, the GCRSR needs to continue to hold discussions on such crucial matters in their future meetings/summits. Since the JRC is the European Commission’s scientific in-house service platform, a partner member of the European and International Research Project (EIRP), as well as a member of the GCRSR, its open/free access nanobiotechnology laboratory infrastructure to academia and small medium enterprises (SMEs) makes room for the ease of investigations related to NM-characterization (European Commission and Joint Research Centre, 2020a; European CommissionJoint Research Centre, 2020b).
In order to maximize these efforts (i.e., synergies related to standardization), the establishment of an international working group–placed ideally under the GCRSR to monitor the development of guidelines and global standards that identify priority areas and gaps that need urgent attention must come to bear. The GCRSR will facilitate communications among Green NM/NP-standardization bodies while providing oversight coordination of laboratory-related activities/inter-laboratory validation. Also, it is necessary to mention at this point that nanoscience is still at its developmental phase, hence, scientifically proven and well-established methods/standards of assessment, production and use are still scarce to-date. This then suggests the need for increased research efforts in this area so as to proffer solutions to the myriad of challenges surrounding the commercialization and large scale implementation of biogenic NM/NPs and nanophotocatalysts.
14 Conclusion
In the near future, it is anticipated that the use and commercialization of green/biogenic NPs/NMs/photocatalysts for water treatment will somewhat have a more significant impact on water treatment plants/industries. However, it would be more cost-effective to focus on enhancing the efficiencies of existing treatment methods by maximizing their reusability, stability and non-toxicity. While current wastewater treatment technologies involving chemically synthesized NPs are able to degrade organic and inorganic pollutants in water and wastewater, they neither completely purify them nor render the treated streams fit for reuse, thus making the process more energy intensive and economically unfeasible. However, on the other hand, the imposed capping and stabilizing abilities by green NP-precursors, makes it easier to recover green NPs for reuse compared to their inorganic counterparts that are capped with capping agents of inorganic chemicals with the perceived capabilities. Based on the available information in literature, global standards/protocols for synthesizing biogenic NPs alongside their toxicity assessments need be established in relation to specific NPs as this will provide proper guidance and information on the pros and cons of using a particular protocol or standard; in addition, the aforementioned should be accompanied by penalties for noncompliance. Hybridizing different plants with complementary properties for toxicity control of the constituents of NMs/NPs is also a viable technique that is envisaged to abate the ills associated with the availability of toxins in biogenic NPs. Moreover, large scale industrial application of NPs in treating wastewater can be brought to bear by carrying out apt comparative cost assessments of the initial investments, design costs, operating cost, energy, etc., required for the industrial use of their inorganic counterparts which are produced by conventional means. Biogenic NPs have lower levels of toxicity compared to their inorganic counterparts, which is the reason for their recent consideration for application in biomedicine as this will reduce the levels of complications that may ensue when they are used. Furthermore, using green synthesized NPs for wastewater treatment is not only a green option, but also a promising technology for fulfilling the zero effluent/waste/toxin discharge obligation via low cost and energy adoption. Therefore, it is envisaged that green NPs will play a more dominant/crucial role in water and sewage purification systems. In addition, more studies on the commercial viability of green NPs for water purification and wastewater treatment is also necessary. Nonetheless, it is pertinent to develop and grow several microbial cultures of prospective microbial precursors which will help ensure a massive production of the desired NPs for sustainable water treatment applications. Therefore, in order to ensure that nanomaterial/particle precursors for wastewater/water treatment applications are highly sustainable, their methods of production, the stabilization process and conditions of synthesis for these systems need be optimized.
Author contributions
SS: Conceptualization, Investigation, Supervision, Writing–original draft, Writing–review and editing. BO: Investigation, Writing–original draft. EO: Investigation, Validation, Writing–original draft. SP: Data curation, Validation, Writing–original draft.
Funding
The author(s) declare that no financial support was received for the research, authorship, and/or publication of this article.
Acknowledgments
The authors herein acknowledge Covenant University for allowing access to her archived resources during this investigation.
Conflict of interest
The authors declare that the research was conducted in the absence of any commercial or financial relationships that could be construed as a potential conflict of interest.
Publisher’s note
All claims expressed in this article are solely those of the authors and do not necessarily represent those of their affiliated organizations, or those of the publisher, the editors and the reviewers. Any product that may be evaluated in this article, or claim that may be made by its manufacturer, is not guaranteed or endorsed by the publisher.
References
Abdel-Khalek, A. A. (2016). Comparative evaluation of genotoxic effects induced by CuO bulk and nano-particles in nile Tilapia, Oreochromis niloticus. Water Air Soil Pollut. 227 (1), 35. doi:10.1007/s11270-015-2737-3
Abe, K., and Saito, H. (1999). Cholesterol does not affect the toxicity of amyloid β fragment but mimics its effect on MTT formazan exocytosis in cultured rat hippocampal neurons. Neurosci. Res. 35 (3), 165–174. doi:10.1016/s0168-0102(99)00048-6
Adeleye, A. S., Conway, J. R., Garner, K., Huang, Y., Su, Y., and Keller, A. A. (2016). Engineered nanomaterials for water treatment and remediation: costs, benefits, and applicability. Chem. Eng. J. 286, 640–662. doi:10.1016/j.cej.2015.10.105
Adeleye, A. S., Conway, J. R., Perez, T., Rutten, P., and Keller, A. A. (2014). Influence of extracellular polymeric substances on the long-term fate, dissolution, and speciation of copper-based nanoparticles. Environ. Sci. Technol. 48, 12561–12568. doi:10.1021/es5033426
Agu, O. S., Tabil, L. G., Mupondwa, E., and Emadi, B. (2021). Torrefaction and pelleting of wheat and barley straw for biofuel and energy applications. Front. Energy Res. 9. doi:10.3389/fenrg.2021.699657
Ahamed, M., Karns, M., Goodson, M., Rowe, J., Hussain, S. M., Schlager, J. J., et al. (2008). DNA damage response to different surface chemistry of silver nanoparticles in mammalian cells. Toxicol. Appl. Pharmacol. 233 (3), 404–410. doi:10.1016/j.taap.2008.09.015
Ahamed, M., Posgai, R., Gorey, T. J., Nielsen, M., Hussain, S. M., and Rowe, J. J. (2010). Silver nanoparticles induced heat shock protein 70, oxidative stress and apoptosis in Drosophila melanogaster. Toxicol. Appl. Pharmacol. 242 (3), 263–269. doi:10.1016/j.taap.2009.10.016
Ahluwalia, S., Prakash, N. T., Prakash, R., and Pal, B. (2016). Improved degradation of methyl orange dye using bio-co-catalyst Se nanoparticles impregnated ZnS photocatalyst under UV irradiation. Chem. Eng. J. 306, 1041–1048. doi:10.1016/j.cej.2016.08.028
Ahmadi, A., Zarei, M., Hassani, A., Ebratkhahan, M., and Olad, A. (2021). Facile synthesis of iron (II) doped carbonaceous aerogel as a three-dimensional cathode and its excellent performance in electro-Fenton degradation of ceftazidime from water solution. Sep. Purif. Technol. 278, 119559. doi:10.1016/j.seppur.2021.119559
Ahmed, S., and Ikram, S. (2017). in Chitosan. Editors S. Ahmed,, and S. Ikram (Hoboken, NJ, USA: John Wiley and Sons Inc). doi:10.1002/9781119364849
Al-Asfar, A., Zaheer, Z., and Aazam, E. S. (2018). Eco-friendly green synthesis of Ag@ Fe bimetallic nanoparticles: antioxidant, antimicrobial and photocatalytic degradation of bromothymol blue. J. Photochem. Photobiol. B, Biol. 185, 143–152. doi:10.1016/j.jphotobiol.2018.05.028
Ali, I., AL-Othman, Z. A., and Alwarthan, A. (2016). Green synthesis of functionalized iron nano particles and molecular liquid phase adsorption of ametryn from water. J. Mol. Liq. 221, 1168–1174. doi:10.1016/j.molliq.2016.06.089
Ali, I., AL-Othman, Z. A., and Sanagi, M. M. (2015). Green synthesis of iron nano-impregnated adsorbent for fast removal of fluoride from water. J. Mol. Liq. 211, 457–465. doi:10.1016/j.molliq.2015.07.034
Ali, I., Peng, C., Khan, Z. M., Naz, I., Sultan, M., Ali, M., et al. (2019). Overview of microbes based fabricated biogenic nanoparticles for water and wastewater treatment. J. Environ. Manag. 230, 128–150. doi:10.1016/j.jenvman.2018.09.073
Al-Ruqeishi, M. S., Mohiuddin, T., and Al-Saadi, L. K. (2016). Green synthesis of iron oxide nanorods from deciduous Omani mango tree leaves for heavy oil viscosity treatment. Arab. J. Chem. 12, 4084–4090. doi:10.1016/j.arabjc.2016.04.003
Alshatwi, A. A., Vaiyapuri Subbarayan, P., Ramesh, E., Al-Hazzani, A. A., Alsaif, M. A., and Alwarthan, A. A. (2012). Al2O3 nanoparticles induce mitochondriamediated cell death and upregulate the expression of signaling genes in human mesenchymal stem cells. J. Biochem. Mol. Toxicol. 26 (11), 469–476. doi:10.1002/jbt.21448
Alvaro, M., Carbonell, E., Ferrer, B., Llabrés i Xamena, F. X., and Garcia, H. (2007). Semiconductor behavior of a metal-organic framework (MOF). Chem. Eur. J. 13, 5106–5112. doi:10.1002/chem.200601003
Amarnath, S., Hussain, M. A., Nanjundiah, V., and Sood, A. K. (2012). β-Galactosidase leakage from Escherichia coli points to mechanical damageas likely cause of carbon nanotube toxicity. Soft Nanosci. Let. 2 (3), 41–45. doi:10.4236/snl.2012.23008
Amenta, V., Aschberger, K., Arena, M., Bouwmeester, H., Botelho Moniz, F., Brandhoff, P., et al. (2015). Regulatory aspects of nanotechnology in the agri/feed/food sector in EU and non-EU countries. Regul. Toxicol. Pharmacol. 73, 463–476. doi:10.1016/j.yrtph.2015.06.016
Andjelkovic, I., Azari, S., Erkelens, M., Forward, P., Lambert, M. F., and Losic, D. (2017). Bacterial iron-oxide nanowires from biofilm waste as a new adsorbent for the removal of arsenic from water. RSC Adv. 7, 3941–3948. doi:10.1039/c6ra26379h
Ansari, B., Coates, P. J., Greenstein, B. D., and Hall, P. A. (1993). In situ end-labelling detects DNA strand breaks in apoptosis and other physiological and pathological states. J. Pathol. 170 (1), 1–8. doi:10.1002/path.1711700102
Arends, M., Morris, R., and Wyllie, A. (1990). Apoptosis. The role of the endonuclease. Am. J. Pathol. 136 (3), 593–608.
Arora, P. K., Srivastava, A., and Singh, V. P. (2014). Bacterial degradation of nitrophenols and their derivatives. J. Hazard. Mater. 266, 42–59. doi:10.1016/j.jhazmat.2013.12.011
Arunachalam, R., Dhanasingh, S., Kalimuthu, B., Uthirappan, M., Rose, C., and Mandal, A. B. (2012). Phytosynthesis of silver nanoparticles using Coccinia grandis leaf extract and its application in the photocatalytic degradation. Colloids Surf. B Biointerfaces 94, 226–230. doi:10.1016/j.colsurfb.2012.01.040
AshaRani, P., Low Kah Mun, G., Hande, M. P., and Valiyaveettil, S. (2008). Cytotoxicity and genotoxicity of silver nanoparticles in human cells. ACS Nano 3 (2), 279–290. doi:10.1021/nn800596w
Aswathi, V. P., Meera, S., Ann Maria, C. G., and Nidhin, M. (2022). Green synthesis of nanoparticles from biodegradable waste extracts and their applications: a critical review. Nanotechnol. Environ. Eng. 8, 377–397. doi:10.1007/s41204-022-00276-8
Atarod, M., Nasrollahzadeh, M., and Sajadi, S. M. (2015). Green synthesis of a Cu/reduced graphene oxide/Fe3O4 nanocomposite using Euphorbia wallichii leaf extract and its application as a recyclable and heterogeneous catalyst for the reduction of 4-nitrophenol and rhodamine B. RSC Adv. 5, 91532–91543. doi:10.1039/c5ra17269a
Ayodhya, D., and Veerabhadram, G. (2017). Preparation, characterization, photocatalytic, sensing and antimicrobial studies of Calotropis gigantea leaf extract capped CuS NPs by a green approach. J. Inorg. Organomet. Polym. Mater. 27, 215–230. doi:10.1007/s10904-017-0672-z
Azzouz, I., Habba, Y. G., Capochichi-Gnambodoe, M., Marty, F., Vial, J., Leprince-Wang, Y., et al. (2018). Zinc oxide nano-enabled microfluidic reactor for water purification and its applicability to volatile organic compounds. Microsyst. Nanoeng. 4, 17093. doi:10.1038/micronano.2017.93
Baharara, J., Ramezani, T., Divsalar, A., Mousavi, M., and Seyedarabi, A. (2016). Induction of apoptosis by green synthesized gold nanoparticles through activation of caspase-3 and 9 in human cervical cancer cells. Biotechnol. 8 (2), 75–83.
Bajpayee, M., Kumar, A., and Dhawan, A. (2013). “The comet assay: assessment of in vitro and in vivo DNA damage,” in Genotoxicity assessment. Methods in molecular biology (methods and protocols). Editors A. Dhawan,, and M. Bajpayee (Totowa, NJ: Humana Press), 1044, 325–345. doi:10.1007/978-1-62703-529-3_17
Bakand, S., and Hayes, A. (2016). Toxicological considerations, toxicity assessment, and risk management of inhaled nanoparticles. Int. J. Mol. Sci. 17 (6), 929. doi:10.3390/ijms17060929
Baker, G. L., Gupta, A., Clark, M. L., Valenzuela, B. R., Staska, L. M., Harbo, S. J., et al. (2008). Inhalation toxicity and lung toxicokinetics of C60 fullerene nanoparticles and microparticles. Toxicol. Sci. 101 (1), 122–131. doi:10.1093/toxsci/kfm243
Balasubramanyam, A., Sailaja, N., Mahboob, M., Rahman, M. F., Hussain, S. M., and Grover, P. (2009). In vivo genotoxicity assessment of aluminium oxide nanomaterials in rat peripheral blood cells using the comet assay and micronucleus test. Mutagenesis 24 (3), 245–251. doi:10.1093/mutage/gep003
Balik, Ö. Y., and Serdar, A. (2016). Coagulation/flocculation optimization and sludge production for pre-treatment of paint industry wastewater. Desalin. Water Treat. 57, 12692–12699. doi:10.1080/19443994.2015.1051125
Banerjee, A., Halder, U., and Bandopadhyay, R. (2017). Preparations and applications of polysaccharide based green synthesized metal nanoparticles: a state-of-the-art. J. Clust. Sci. 28, 1803–1813. doi:10.1007/s10876-017-1219-8
Banihashem, S. M., Moradi, A., Evazzadeh, B., Namvar, F., and Fang, Z. N. (2024). Biogenically synthesized nanoparticles in wastewater treatment; a greener approach: a review. Clean Technol. Environ. Policy 26, 1731–1754. doi:10.1007/s10098-023-02720-y
Bano, D., Kumar, V., Singha, V. K., and Hasan, S. H. (2018). Green synthesis of fluorescent carbon quantum dots for the detection of mercury(II) and glutathione. New J. Chem. 42, 5814–5821. doi:10.1039/c8nj00432c
Bansal, V., Rautaray, D., Ahmad, A., and Sastry, M. (2004). Biosynthesis of zirconia nanoparticles using the fungus Fusarium oxysporum. J. Mater. Chem. 14, 3303–3305. doi:10.1039/b407904c
Behra, R., and Krug, H. (2008). Nanoecotoxicology: nanoparticles at large. Nat. Nanotechnol. 3 (5), 253–254. doi:10.1038/nnano.2008.113
Berghe, T. V., Grootjans, S., Goossens, V., Dondelinger, Y., Krysko, D. V., Takahashi, N., et al. (2013). Determination of apoptotic and necrotic cell death in vitro and in vivo. Methods 61 (2), 117–129. doi:10.1016/j.ymeth.2013.02.011
Beveridge, T., Hughes, M., Lee, H., Leung, K., Poole, R., Savvaidis, I., et al. (1996). “Metal-microbe interactions: contemporary approaches,” in Advances in microbial physiology (Elsevier), 177–243.
Beveridge, T., and Murray, R. (1980). Sites of metal deposition in the cell wall of Bacillus subtilis. J. Bacteriol. 141, 876–887. doi:10.1128/jb.141.2.876-887.1980
Bezerril, L. M., de Vasconcelos, C. L., Dantas, T. N. C., Pereira, M. R., and Fonseca, J. L. C. (2006). Rheology of chitosan-kaolin dispersions. Colloids Surf. A Physicochem. Eng. Asp. 287 (1–3), 24–28. doi:10.1016/j.colsurfa.2006.03.017
Bitensky, L. (1963). “The reversible activation of lysosomes in normal cells and the efects of pathological conditions,” in Ciba foundation symposium—lysosomes (Wiley Online Library), 362–383.
Bolan, N., Hoang, S. A., Beiyuan, J., Gupta, S., Hou, D., Karakoti, A., et al. (2022a). Multifunctional applications of biochar beyond carbon storage. Int. Mater. Rev. 67, 150–200. doi:10.1080/09506608.2021.1922047
Bolan, N., Kumar, M., Singh, E., Kumar, A., Singh, L., Kumar, S., et al. (2022b). Antimony contamination and its risk management in complex environmental settings: a review. Environ. Int. 158, 106908. doi:10.1016/j.envint.2021.106908
Bonigala, B., Kasukurthi, B., Konduri, V. V., Mangamuri, U. K., Gorrepati, R., and Poda, S. (2018). Green synthesis of silver and gold nanoparticles using Stemona tuberosa Lour and screening for their catalytic activity in the degradation of toxic chemicals. Environ. Sci. Pollut. Res. Int. 25, 32540–32548. doi:10.1007/s11356-018-3105-9
Bordbar, M. (2017). Biosynthesis of Ag/almond shell nanocomposite as a cost-effective and efficient catalyst for degradation of 4-nitrophenol and organic dyes. RSC Adv. 7, 180–189. doi:10.1039/c6ra24977a
Bordbar, M., and Mortazavimanesh, N. (2017b). Green synthesis of Pd/walnut shell nanocomposite using Equisetum arvense L. Leaf extract and its application for the reduction of 4-nitrophenol and organic dyes in a very short time. Environ. Sci. Pollut. Res. Int. 24, 4093–4104. doi:10.1007/s11356-016-8183-y
Bordbar, M., Negahdar, N., and Nasrollahzadeh, M. (2018). Melissa Officinalis L. Leaf extract assisted green synthesis of CuO/ZnO nanocomposite for the reduction of 4-nitrophenol and Rhodamine B. Sep. Purif. Technol. 191, 295–300. doi:10.1016/j.seppur.2017.09.044
Bordbar, M., Sharifi-Zarchi, Z., and Khodadadi, B. (2017). Green synthesis of copper oxide nanoparticles/clinoptilolite using Rheum palmatum L. Root extract: high catalytic activity for reduction of 4-nitro phenol, rhodamine B, and methylene blue. J. Solgel Sci. Technol. 81, 724–733. doi:10.1007/s10971-016-4239-1
Borenfreund, E., and Puerner, J. A. (2017). Toxicity determined in vitro by morphological alterations and neutral red absorption. Toxicol. Lett. 24 (2–3), 119–124. doi:10.1016/0378-4274(85)90046-3
Borenfreund, E., and Shopsis, C. (1985). Toxicity monitored with a correlated set of cell-culture assays. Xenobiotica 15 (8–9), 705–711. doi:10.3109/00498258509047431
Borgos, S. E. (2019). “Joining transatlantic forces via the EU-US communities of research and the EU-NCL,” in Private communication at global Summit on regulatory science 2019 on nanotechnology and nanoplastics. EUR 30195 EN.
Bortner, C. D., Oldenburg, N. B., and Cidlowski, J. A. (1995). The role of DNA fragmentation in apoptosis. Trends Cell Biol. 5 (1), 21–26. doi:10.1016/s0962-8924(00)88932-1
Bousselmi, L., Geissen, S.-U., and Schroeder, H. (2004). Textile wastewater treatment and reuse by solar catalysis: results from a pilot plant in Tunisia. Water Sci. Technol. 49, 331–337. doi:10.2166/wst.2004.0298
Bremner, D. H., Molina, R., Martínez, F., Melero, J. A., and Segura, Y. (2009). Degradation of phenolic aqueous solutions by high frequency sono-Fenton systems (US–Fe2O3/SBA- 15–H2O2). Appl. Catal. B 90, 380–388. doi:10.1016/j.apcatb.2009.03.028
Buarki, F., AbuHassan, H., Al Hannan, F., and Henari, F. Z. (2022). Green synthesis of iron oxide nanoparticles using Hibiscus rosa sinensis flowers and their antibacterial activity. J. Nanotechnol. 2022, 1–6. doi:10.1155/2022/5474645
Buyukkamaci, N., and Koken, E. (2010). Economic evaluation of alternative wastewater treatment plant options for pulp and paper industry. Sci. Total Environ. 408, 6070–6078. doi:10.1016/j.scitotenv.2010.08.045
Cañizares, P., Paz, R., Sáez, C., and Rodrigo, M. A. (2009). Costs of the electrochemical oxidation of wastewaters: a comparison with ozonation and Fenton oxidation processes. J. Environ. Manag. 90, 410–420. doi:10.1016/j.jenvman.2007.10.010
Cao, D., Jin, X., Gan, L., Wang, T., and Chen, Z. (2016). Removal of phosphate using iron oxide nanoparticles synthesized by eucalyptus leaf extract in the presence of CTAB surfactant. Chemosphere 159, 23–31. doi:10.1016/j.chemosphere.2016.05.080
Caruthers, S. D., Wickline, S. A., and Lanza, G. M. (2007). Nanotechnological applications in medicine. Curr. Opin. Biotechnol. 18 (1), 26–30. doi:10.1016/j.copbio.2007.01.006
Casey, A., Herzog, E., Davoren, M., Lyng, F., Byrne, H., and Chambers, G. (2007). Spectroscopic analysis confirms the interactions between single walled carbon nanotubes and various dyes commonly used to assess cytotoxicity. Carbon 45 (7), 1425–1432. doi:10.1016/j.carbon.2007.03.033
Chen, H., Yoshioka, H., Kim, G. S., Jung, J. E., Okami, N., Sakata, H., et al. (2011). Oxidative stress in ischemic brain damage: mechanisms of cell death and potential molecular targets for neuroprotection. Antioxid. Redox. Signal. 14 (8), 1505–1517. doi:10.1089/ars.2010.3576
Chen, L., Yokel, R. A., Hennig, B., and Toborek, M. (2008). Manufactured aluminum oxide nanoparticles decrease expression of tight junction proteins in brain vasculature. J. Neuroimmune Pharmacol. 3 (4), 286–295. doi:10.1007/s11481-008-9131-5
Chen, X., Lu, W., Xu, T., Li, N., Qin, D., Zhu, Z., et al. (2017). A bio-inspired strategy to enhance the photocatalytic performance of g-C3N4 under solar irradiation by axial coordination with hemin. Appl. Catal. B 201, 518–526. doi:10.1016/j.apcatb.2016.08.020
Chen, Z., Wang, T., Jin, X., Chen, Z., Megharaj, M., and Naidu, R. (2013). Multifunctional kaolinite supported nanoscale zero-valent iron used for the adsorption and degradation of crystal violet in aqueous solution. J. Colloid Interface Sci. 398, 59–66. doi:10.1016/j.jcis.2013.02.020
Chiemchaisri, C., Jaitrong, L., Honda, R., Fukushi, K., and Yamamoto, K. (2007). Photosynthetic bacteria pond system with infra-red transmitting filter for the treatment and recovery of organic carbon from industrial wastewater. Water Sci. Technol. 56, 109–116. doi:10.2166/wst.2007.686
Cho, S. J., Maysinger, D., Jain, M., Röder, B., Hackbarth, S., and Winnik, F. M. (2007). Long-term exposure to CdTe quantum dots causes functional impairments in live cells. Langmuir 23 (4), 1974–1980. doi:10.1021/la060093j
Choi, O., and Hu, Z. (2008). Size dependent and reactive oxygen species related nanosilver toxicity to nitrifying bacteria. Environ. Sci. Technol. 42 (12), 4583–4588. doi:10.1021/es703238h
Choi, S.-J., Oh, J.-M., and Choy, J.-H. (2009). Toxicological effects of inorganic nanoparticles on human lung cancer A549 cells. J. Inorg. Biochem. 103 (3), 463–471. doi:10.1016/j.jinorgbio.2008.12.017
Chokkareddy, R., and Redhi, G. G. (2018). “Green synthesis of metal nanoparticles and its reaction mechanisms,” in Green metal nanoparticles, 113–139.
Chong, M. N., Jin, B., Chow, C. W. K., and Saint, C. (2010). Recent developments in photocatalytic water treatment technology: a review. Water Res. 44, 2997–3027. doi:10.1016/j.watres.2010.02.039
Chowdhury, I. H., Ghosh, S., and Naskar, M. K. (2016). Aqueous-based synthesis of mesoporous TiO2 and Ag–TiO2 nanopowders for efficient photodegradation of methylene blue. Ceram. Int. 42, 2488–2496. doi:10.1016/j.ceramint.2015.10.049
Coker, V. S., Bennett, J. A., Telling, N. D., Henkel, T., Charnock, J. M., van der Laan, G., et al. (2010). Microbial engineering of nanoheterostructures: biological synthesis of a magnetically recoverable palladium nanocatalyst. ACS Nano 4, 2577–2584. doi:10.1021/nn9017944
Collins, A. R., Ai-Guo, M., and Duthie, S. J. (1995). The kinetics of repair of oxidative DNA damage (strand breaks and oxidised pyrimidines) in human cells. Mutat. Res. DNA Repair 336 (1), 69–77. doi:10.1016/0921-8777(94)00043-6
Crane, R. A., and Scott, T. B. (2012). Nanoscale zero-valent iron: future prospects for an emerging water treatment technology. J. Hazard. Mater. 211–212, 112–125. doi:10.1016/j.jhazmat.2011.11.073
Cullis, P. (2019). “Nanomedicines innovation network (NMIN): Canada’s national centre of excellence in nanomedicines,” in Private communication at global Summit on regulatory science 2019 on nanotechnology and nanoplastics. EUR 30195 EN.
Cveticanin, J., Joksic, G., Leskovac, A., Petrovic, S., Sobot, A. V., and Neskovic, O. (2009). Using carbon nanotubes to induce micronuclei and double strand breaks of the DNA in human cells. Nanotechnology 21 (1), 015102. doi:10.1088/0957-4484/21/1/015102
Das, M. C., Xu, H., Wang, Z., Srinivas, G., Zhou, W., Yue, Y.-F., et al. (2011). A Zn4O-containing doubly interpenetrated porous metal–organic framework for photocatalytic decomposition of methyl orange. Chem. Commun. 47, 11715–11717. doi:10.1039/c1cc12802g
Das, R. K., Borthakur, B. B., and Bora, U. (2010b). Green synthesis of gold nanoparticles using ethanolic leaf extract of Centella asiatica. Mater Lett. 64, 1445–1447. doi:10.1016/j.matlet.2010.03.051
Das, S. K., Das, A. R., and Guha, A. K. (2010a). Microbial synthesis of multishaped gold nanostructures. Small 6, 1012–1021. doi:10.1002/smll.200902011
Das, S. K., Khan, M. M. R., Guha, A. K., and Naskar, N. (2013). Bio-inspired fabrication of silver nanoparticles on nanostructured silica: characterization and application as a highly efficient hydrogenation catalyst. Green Chem. 15, 2548–2557. doi:10.1039/c3gc40310f
Das, S. K., Liang, J., Schmidt, M., Laffir, F., and Marsili, E. (2012). Biomineralization mechanism of gold by zygomycete fungi Rhizopous oryzae. ACS Nano 6, 6165–6173. doi:10.1021/nn301502s
Dauthal, P., and Mukhopadhyay, M. (2013). Biosynthesis of palladium nanoparticles using Delonix regia leaf extract and its catalytic activity for nitro-aromatics hydrogenation. Ind. Eng. Chem. Res. 52, 18131–18139. doi:10.1021/ie403410z
Dauthal, P., and Mukhopadhyay, M. (2015). Agro-industrial waste-mediated synthesis and characterization of gold and silver nanoparticles and their catalytic activity for 4- nitroaniline hydrogenation. Korean J. Chem. Eng. 32, 837–844. doi:10.1007/s11814-014-0277-y
Dauthal, P., and Mukhopadhyay, M. (2016). AuPd bimetallic nanoparticles: single step biofabrication, structural characterization and catalytic activity. J. Ind. Eng. Chem. 35, 45–53. doi:10.1016/j.jiec.2015.12.005
Davoren, M., Herzog, E., Casey, A., Cottineau, B., Chambers, G., Byrne, H. J., et al. (2007). In vitro toxicity evaluation of single walled carbon nanotubes on human A549 lung cells. Toxicol. Vitro 21 (3), 438–448. doi:10.1016/j.tiv.2006.10.007
De Corte, S., Sabbe, T., Hennebel, T., Vanhaecke, L., De Gusseme, B., Verstraete, W., et al. (2012). Doping of biogenic Pd catalysts with Au enables dechlorination of diclofenac at environmental conditions. Water Res. 46, 2718–2726. doi:10.1016/j.watres.2012.02.036
De Gusseme, B., Hennebel, T., Vanhaecke, L., Soetaert, M., Desloover, J., Wille, K., et al. (2011). Biogenic palladium enhances diatrizoate removal from hospital wastewater in a microbial electrolysis cell. Environ. Sci. Technol. 45, 5737–5745. doi:10.1021/es200702m
De Heyder, B., Ockier, P., Jansen, R., and Huiberts, R. (2001). Predicting the sound power and impact of a wastewater treatment plant. Water Sci. Technol. 44, 235–242. doi:10.2166/wst.2001.0775
Delcroix, G.J.-R., Jacquart, M., Lemaire, L., Sindji, L., Franconi, F., Le Jeune, J. J., et al. (2009). Mesenchymal and neural stem cells labeled with HEDP-coated SPIO nanoparticles: in vitro characterization and migration potential in rat brain. Brain Res. 1255, 18–31. doi:10.1016/j.brainres.2008.12.013
Deng, W., Xie, Q., Wang, H., Ma, Z., Wu, B., and Zhang, X. (2017). Selenium nanoparticles as versatile carriers for oral delivery of insulin: insight into the synergic antidiabetic effect and mechanism. Nanomed. Nanotechnol. Biol. Med. 13 (6), 1965–1974. doi:10.1016/j.nano.2017.05.002
Deplanche, K., Bennett, J. A., Mikheenko, I. P., Omajali, J., Wells, A. S., Meadows, R. E., et al. (2014). Catalytic activity of biomass-supported Pd nanoparticles: influence of the biological component in catalytic efficacy and potential application in ‘green’ synthesis of fine chemicals and pharmaceuticals. Appl. Catal. B Environ. 147, 651–665. doi:10.1016/j.apcatb.2013.09.045
Devasahayam, S. (2017). Overview of an internationally integrated nanotechnology governance. Int. J. Meterol Qual. Eng. 8, 8. doi:10.1051/ijmqe/2017002
Devasahayam, S. (2019). “Nanotechnology and nanomedicine in market: a global perspective on regulatory issues,” in Characterization and biology of nanomaterials for drug delivery; micro and nano technologies series: nanoscience and nanotechnology in drug delivery. Editors S. S. Mohapatra, S. Ranjan, N. Dasgupta, K. M. Raghvendra, and T. Sabu (Elsevier), 488–522.
Devi, T. B., and Ahmaruzzaman, M. (2016). Bio-inspired sustainable and green synthesis of plasmonic Ag/AgCl nanoparticles for enhanced degradation of organic compound from aqueous phase. Environ. Sci. Pollut. Res. Int. 23, 17702–17714. doi:10.1007/s11356-016-6945-1
Devi, T. B., and Ahmaruzzaman, M. (2017). Bio-inspired facile and green fabrication of Au@ Ag@ AgCl core–double shells nanoparticles and their potential applications for elimination of toxic emerging pollutants: a green and efficient approach for wastewater treatment. Chem. Eng. J. 317, 726–741. doi:10.1016/j.cej.2017.02.082
Devi, T. B., Begum, S., and Ahmaruzzaman, M. (2016). Photo-catalytic activity of Plasmonic Ag@ AgCl nanoparticles (synthesized via a green route) for the effective degradation of Victoria blue B from aqueous phase. J. Photochem. Photobiol. B, Biol. 160, 260–270. doi:10.1016/j.jphotobiol.2016.03.033
Dey, K. K., and Ghosh, M. (2020). Understanding the effect of deacetylation on chitin by measuring chemical shift anisotropy tensor and spin lattice relaxation time. Chem. Phys. Lett. 738, 136782. doi:10.1016/j.cplett.2019.136782
Dhanker, R., Kumar, R., Kamble, S., Kamakshi, S., and Goyal, S. (2023). Biosynthesis and characterization of bionanoparticles generated from peels of solanum tuberosum (potato) and their antibacterial and wastewater treatment potential. Front. Nanotechnol. 5, 1213160. doi:10.3389/fnano.2023.1213160
Dikshit, P. K., Kumar, J., Das, A. K., Sadhu, S., Sharma, S., Singh, S., et al. (2021). Green synthesis of metallic nanoparticles: applications and limitations. Catalysts 11 (8), 902. doi:10.3390/catal11080902
Dinda, A. (2019). “Challenges in the development of an Indian guideline for nanopharmaceuticals,” in Private communication at global Summit on regulatory science 2019 on nanotechnology and nanoplastics. EUR 30195 EN.
Dinda, G., Halder, D., Mitra, A., Pal, N., Vázquez-Vázquez, C., and López-Quintela, M. A. (2017). Study of the antibacterial and catalytic activity of silver colloids synthesized using the fruit of Sapindus mukorossi. New J. Chem. 41, 10703–10711. doi:10.1039/c7nj00704c
Durán, M., Silveira, C. P., and Durán, N. (2015). Catalytic role of traditional enzymes for biosynthesis of biogenic metallic nanoparticles: a mini-review. IET Nanobiotechnol 9, 314–323. doi:10.1049/iet-nbt.2014.0054
Dusinska, M., and Collins, A. R. (2008). The comet assay in human biomonitoring: gene–environment interactions. Mutagenesis 23 (3), 191–205. doi:10.1093/mutage/gen007
Ehrampoush, M. H., Miria, M., Salmani, M. H., and Mahvi, A. H. (2015). Cadmium removal from aqueous solution by green synthesis iron oxide nanoparticles with tangerine peel extract. J. Environ. Health Sci. Eng. 13, 84. doi:10.1186/s40201-015-0237-4
Elsaesser, A., and Howard, C. V. (2012). Toxicology of nanoparticles. Adv. Drug Deliv. Rev. 64 (2), 129–137. doi:10.1016/j.addr.2011.09.001
Eskandarloo, H., Kierulf, A., and Abbaspourrad, A. (2017). Nano-and micromotors for cleaning polluted waters: focused review on pollutant removal mechanisms. Nanoscale 9, 13850–13863. doi:10.1039/c7nr05494g
European Chemicals Agency (2020). ECHA. Available at: https://echa.europa.eu/regulations/nanomaterials.
European Chemicals Agency (ECHA) (2019). Microplastics. Available at: https://www.echa.europa.eu/hot-topics/microplastics.
European Commission (2019). The European green deal – communication from the commission to the European parliament, the European council, the council, the European economic and social committee and the committee of the regions. 640 final.
European Commission (2020a). NanoREGII: development and implementation of grouping and safe-by-design approaches within regulatory frameworks. Available at: https://cordis.europa.eu.project/id/646221.
European Commission (2020b). “A new industrial strategy for Europe – communication from the commission to the European parliament,” in The European council, the council, the European economic and social committee and the committee of the regions. 102 final.
European Commission (2020c). SCCS – opinions 2016-2021. Available at: https://ec.europa.eu/health/scientific_committees/consumer_safety/opinions_en#fragment2.
European CommissionJoint Research Centre (JRC) (2020b). Certified reference materials catalogue. Available at: https://crm.jrc.ec.europa.eu.
European CommissionJoint Research Centre (JRC) (2020a). JRC nanomaterials repository. Available at: https://ec.europa.eu/jrc/en/scientific-tool/jrc-nanomaterials-repository.
European Food Safety Authority (EFSA) (2016). Presence of microplastics and nanoplastics in food, with particular focus on seafood. EFSA J. 14, 4501. doi:10.2903/j.efsa.2016.4501
European Food Safety Authority (EFSA) (2020a). EFSA Scientific network for risk assessment of the use of nanotechnologies in food and feed. Available at: https://www.efsa.europa.eu/sites/default/files/Nanonetwork.pdf.
European Food Safety Authority (EFSA) (2020b). European food safety authority (EFSA). Available at: https://www.efsa.europa.eu/en/topics/topic/nanotechnology.
European Union (2009). Regulation (EC) No 1223/2009 of the European parliament and of the Council of 30 november 2009 on cosmetic products. Europe: Official Journal of the European Union. L342/59. Available at: http://data.europa.eu/eli/reg/2009/1223/oj.
European Union (2019). Directive (EU) 2019/904 of the European Parliament and of the Council on the reduction of the impact of certain plastic products on the environment. Europe: Official Journal of the European Union. L155/1. Available at: http://data.europa.eu/eli/dir/2019/904/oj.
Ewing, A. G., Bigelow, J. C., and Wightman, R. M. (1983). Direct in vivo monitoring of dopamine released from two striatal compartments in the rat. Science 221 (4606), 169–171. doi:10.1126/science.6857277
Fairbairn, D. W., Olive, P. L., and O’Neill, K. L. (1995). The comet assay: a comprehensive review. Mutat. Res. Rev. Genet. Toxicol. 339 (1), 37–59. doi:10.1016/0165-1110(94)00013-3
Fantel, A. G. (1996). Reactive oxygen species in developmental toxicity: review and hypothesis. Teratology 53 (3), 196–217. doi:10.1002/(sici)1096-9926(199603)53:3<196::aid-tera7>3.0.co;2-2
Farhadi, S., Ajerloo, B., and Mohammadi, A. (2017). Green biosynthesis of spherical silver nanoparticles by using date palm (phoenix dactylifera) fruit extract and study of their antibacterial and catalytic activities. Acta Chim. Slov. 64, 129–143. doi:10.17344/acsi.2016.2956
Fierro, S., Sa´nchez-Saavedra, M. P., and Copalcu´a, C. (2008). Nitrate and phosphate removal by chitosan immobilized Scenedesmus. Bioresour. Technol. 99, 1274–1279. doi:10.1016/j.biortech.2007.02.043
Fiorito, S., Serafino, A., Andreola, F., and Bernier, P. (2006). Effects of fullerenes and single-wall carbon nanotubes on murine and human macrophages. Carbon 44 (6), 1100–1105. doi:10.1016/j.carbon.2005.11.009
Franco, R. T., Silva, A. L., Licea, Y. E., Serna, J. D. P., Alzamora, M., Sánchez, D. R., et al. (2021). Green synthesis of iron oxides and phosphates via thermal treatment of iron polyphenols synthesized by a Camellia sinensis extract. Inorg. Chem. 60, 5734–5746. doi:10.1021/acs.inorgchem.0c03794
Fredrickson, J. K., Kostandarithes, H. M., Li, S., Plymale, A. E., and Daly, M. (2000). Reduction of Fe (III), Cr (VI), U (VI), and tc (VII) by Deinococcus radiodurans R1. Appl. Environ. Microbiol. 66, 2006–2011. doi:10.1128/aem.66.5.2006-2011.2000
Friedersdorf, L. (2019). “Leveraging advances in nanotechnology and international collaboration to address emerging contaminants,” in Private communication at global summit on regulatory science 2019 on nanotechnology and nanoplastics. EUR 30195 EN.
Furgal, K. M., Meyer, R. L., and Bester, K. (2015). Removing selected steroid hormones, biocides and pharmaceuticals from water by means of biogenic manganese oxide nanoparticles in situ at ppb levels. Chemosphere 136, 321–326. doi:10.1016/j.chemosphere.2014.11.059
Gan, L., Lu, Z., Cao, D., and Chen, Z. (2018). Effects of cetyltrimethylammonium bromide on the morphology of green synthesized Fe3O4 nanoparticles used to remove phosphate. Mater. Sci. Eng. C 82, 41–45. doi:10.1016/j.msec.2017.08.073
Ganesh, M., Lee, S. G., Jayaprakash, J., Mohankumar, M., and Jang, H. T. (2019). Hydnocarpus alpina Wt extract mediated green synthesis of ZnO nanoparticle and screening of its anti-microbial, free radical scavenging, and photocatalytic activity. Biocatal. Agric. Biotechnol. 19, 101129. doi:10.1016/j.bcab.2019.101129
Garai, C., Hasan, S. N., Barai, A. C., Ghorai, S., Panja, S. K., and Bag, B. G. (2018). Green synthesis of Terminalia arjuna-conjugated palladium nanoparticles (TA-PdNPs) and its catalytic applications. J. Nanostructure Chem. 8, 465–472. doi:10.1007/s40097-018-0288-z
Garole, V., Choudhary, B., Tetgure, S., Garole, D., and Borse, A. (2019). Palladium nanocatalyst: green synthesis, characterization, and catalytic application. Int. J. Environ. Sci. Technol. 16, 7885–7892. doi:10.1007/s13762-018-2173-1
Gautam, P. K., Singh, A., Misra, K., Sahoo, A. K., and Samanta, S. K. (2019). Synthesis and applications of biogenic nanomaterials in drinking and wastewater treatment. J. Environ. Manage. 231, 734–748. doi:10.1016/j.jenvman.2018.10.104
Gavrieli, Y., Sherman, Y., and Ben-Sasson, S. A. (1992). Identification of programmed cell death in situ via specific labeling of nuclear DNA fragmentation. J. Cell Biol. 119, 493–501. doi:10.1083/jcb.119.3.493
Gawande, K., and Jenkins-Smith, H. (2001). Nuclear waste transport and residential property values: estimating the effects of perceived risks. J. Environ. Econ. Manage. 42, 207–233. doi:10.1006/jeem.2000.1155
Gehrke, I., Geiser, A., and Somborn-Schulz, A. (2015). Innovations in nanotechnology for water treatment. Nanotechnol. Sci. Appl. 8, 1–17. doi:10.2147/NSA.S43773
Gibson, J. D., Khanal, B. P., and Zubarev, E. R. (2007). Paclitaxel-functionalized gold nanoparticles. J. Am. Chem. Soc. 129 (37), 11653–11661. doi:10.1021/ja075181k
Gnanaprakasam, P., and Selvaraju, T. (2014). Green synthesis of self-assembled silver nanowire decorated reduced graphene oxide for efficient nitroarene reduction. RSC Adv. 4, 24518–24525. doi:10.1039/c4ra01798f
Goering, P. (2019). “Nanotechnology and medical devices: a regulatory science approach involving research, standards, and risk assessment,” in Private communication at global summit on regulatory science 2019 on nanotechnology and nanoplastics. EUR 30195 EN.
Gomes, A., Fernandes, E., and Lima, J. L. (2005). Fluorescence probes used for detection of reactive oxygen species. J. Biochem. Biophys. Methods 65 (2), 45–80. doi:10.1016/j.jbbm.2005.10.003
Gopalakrishnan, R., Loganathan, B., and Raghu, K. (2015). Green synthesis of Au–Ag bimetallic nanocomposites using Silybum marianum seed extract and their application as a catalyst. RSC Adv. 5, 31691–31699. doi:10.1039/c5ra03571f
Gottardo, S., Mech, A., Quiros Pesudo, L., Rasmussen, K., Rauscher, H., Riego Sintes, J., et al. (2017). NANoREG framework for the safety assessment of nanomaterials. Editors H. Crutzen, P. Jantunen, and S. Gottardo (Luxembourg: Publications Office of the European Union). 28550 EN. EUR.
Grünberg, K., Wawer, C., Tebo, B. M., and Schüler, D. (2001). A large gene cluster encoding several magnetosome proteins is conserved in different species of magnetotactic bacteria. Appl. Environ. Microbiol. 67, 4573–4582. doi:10.1128/aem.67.10.4573-4582.2001
Guiterrez, G. A., Dziubla, T. D., and Hilt, J. Z. (2022). Recent advances on iron oxide magnetic nanoparticles as sorbents for organic pollutants in water and wastewater treatment. Rev. Environ. Health 32 (`1-2), 111–117. doi:10.1513/reveh-2015-0083
Guo, N. L., Wan, Y. W., Denvir, J., Porter, D. W., Pacurari, M., Wolfarth, M. G., et al. (2012). Multiwalled carbon nanotube-induced gene signatures in the mouse lung: potential predictive value for human lung cancer risk and prognosis. J. Toxicol. Environ. Health Part A 75 (18), 1129–1153. doi:10.1080/15287394.2012.699852
Gupta, N., Fischer, A. R. H., Van Der Lans, I. A., and Frewer, L. J. (2012). Factors influencing societal response of nanotechnology: an expert stakeholder analysis. J. Nanopart. Res. 14, 857. doi:10.1007/s11051-012-0857-x
Gurushantha, K., Anantharaju, K., Nagabhushana, H., Sharma, S., Vidya, Y., Shivakumara, C., et al. (2015). Facile green fabrication of iron-doped cubic ZrO2 nanoparticles by Phyllanthus acidus: structural, photocatalytic and photoluminescent properties. J. Mol. Catal. A Chem. 397, 36–47. doi:10.1016/j.molcata.2014.10.025
Gurushantha, K., Anantharaju, K., Renuka, L., Sharma, S., Nagaswarupa, H., Prashantha, S., et al. (2017). New green synthesized reduced graphene oxide–ZrO2 composite as high performance photocatalyst under sunlight. RSC Adv. 7, 12690–12703. doi:10.1039/c6ra25823a
Haidri, I., Shahid, M., Hussain, S., Shahzad, T., Mahmood, F., Umair, M., et al. (2023). Efficacy of biogenic zinc oxide nanoparticles in treating wastewater for sustainable wheat cultivation. Plants 12, 3058. doi:10.3390/plants12173058
Hainfeld, J. F., Dilmanian, F. A., Zhong, Z., Slatkin, D. N., Kalef-Ezra, J. A., and Smilowitz, H. M. (2010). Gold nanoparticles enhance the radiation therapy of a murine squamous cell carcinoma. Phys. Med. Biol. 55 (11), 3045–3059. doi:10.1088/0031-9155/55/11/004
Halliwell, B., and Whiteman, M. (2004). Measuring reactive species and oxidative damage in vivo and in cell culture: how should you do it and what do the results mean? Br. J. Pharmacol. 142 (2), 231–255. doi:10.1038/sj.bjp.0705776
Handy, R. D., van den Brink, N., Chappell, M., Mühling, M., Behra, R., Dušinská, M., et al. (2012). Practical considerations for conducting ecotoxicity test methods with manufactured nanomaterials: what have we learnt so far? Ecotoxicology 21 (4), 933–972. doi:10.1007/s10646-012-0862-y
Hanley, C., Thurber, A., Hanna, C., Punnoose, A., Zhang, J., and Wingett, D. G. (2009). The influences of cell type and ZnO nanoparticle size on immune cell cytotoxicity and cytokine induction. Nanoscale Res. Lett. 4 (12), 1409. doi:10.1007/s11671-009-9413-8
Hano, C., and Abbasi, B. H. (2022). Plant-based green synthesis of nanoparticles: production, characterization and applications. Charact. Appl. Biomol. 12 (1), 31. doi:10.3390/biom12010031
Harish, V., Ansari, M. M., Tewari, D., Yadav, A. B., Sharma, N., Bawarig, S., et al. (2023). Cutting-edge advances in tailoring size, shape, and functionality of nanoparticles and nanostructures: a review. J. Taiwan Inst. Chem. Eng. 149, 105010. doi:10.1016/j.jtice.2023.105010
Harshiny, M., Iswarya, C. N., and Matheswaran, M. (2015). Biogenic synthesis of iron nanoparticles using Amaranthus dubius leaf extract as a reducing agent. Powder Technol. 286, 744–749. doi:10.1016/j.powtec.2015.09.021
Hassan, A. K., Al-Kindi, G. Y., and Ghanim, D. (2020). Green synthesis of bentonite-supported iron nanoparticles as a heterogeneous Fenton-like catalyst: kinetics of decolorization of reactive blue 238 dye. Water Sci. Eng. 13, 286–298. doi:10.1016/j.wse.2020.12.001
Hatamifard, A., Nasrollahzadeh, M., and Lipkowski, J. (2015). Green synthesis of a natrolite zeolite/palladium nanocomposite and its application as a reusable catalyst for the reduction of organic dyes in a very short time. RSC Adv. 5, 91372–91381. doi:10.1039/c5ra18476b
Hatamifard, A., Nasrollahzadeh, M., and Sajadi, S. M. (2016). Biosynthesis, characterization and catalytic activity of an Ag/zeolite nanocomposite for base-and ligand-free oxidative hydroxylation of phenylboronic acid and reduction of a variety of dyes at room temperature. New J. Chem. 40, 2501–2513. doi:10.1039/c5nj02909k
Hazarika, M., Borah, D., Bora, P., Silva, A. R., and Das, P. (2017). Biogenic synthesis of palladium nanoparticles and their applications as catalyst and antimicrobial agent. PLoS One 12, e0184936. doi:10.1371/journal.pone.0184936
Health Canada (2020). Policy statement on health Canada’s working definition for nanomaterial. Available at: https://www.canada.ca/en/health-canada/services/science-research/reports-publications/nanomaterial/policy-statement-health-canada-working-definition.html.
Hegde, K., Brar, S. K., Verma, M., and Surampalli, R. Y. (2016). Current understandings of toxicity, risks and regulations of engineered nanoparticles with respect to environmental microorganisms. Nanotechnol. Environ. Eng. 1 (1), 5. doi:10.1007/s41204-016-0005-4
Henderson, L., Wolfreys, A., Fedyk, J., Bourner, C., and Windebank, S. (1998). The ability of the Comet assay to discriminate between genotoxins and cytotoxins. Mutagenesis 13 (1), 89–94. doi:10.1093/mutage/13.1.89
Herzog, E., Byrne, H. J., Casey, A., Davoren, M., Lenz, A. G., Maier, K. L., et al. (2009). SWCNT suppress inflammatory mediator responses in human lung epithelium in vitro. Toxicol. Appl. Pharmacol. 234 (3), 378–390. doi:10.1016/j.taap.2008.10.015
Hoag, G. E., Collins, J. B., Holcomb, J. L., Hoag, J. R., Nadagouda, M. N., and Varma, R. S. (2009). Degradation of bromothymol blue by ‘greener’nano-scale zero-valent iron synthesized using tea polyphenols. J. Mater. Chem. 19, 8671–8677. doi:10.1039/b909148c
Hodge, G., Bowman, D., and Ludlow, K. (2009). “New global frontiers in regulation. The age of nanotechnology,” in Monash studies in global movements series (United Kingdom: Edward Elgar Publishing).
Hou, S., Wu, Y.-n., Feng, L., Chen, W., Wang, Y., Morlay, C., et al. (2018). Green synthesis and evaluation of an iron-based metal–organic framework MIL-88B for efficient decontamination of arsenate from water. Dalton Trans. 47, 2222–2231. doi:10.1039/c7dt03775a
Hsin, Y.-H., Chen, C. F., Huang, S., Shih, T. S., Lai, P. S., and Chueh, P. J. (2008). The apoptotic effect of nanosilver is mediated by a ROS- and JNK-dependent mechanism involving the mitochondrial pathway in NIH3T3 cells. Toxicol. Lett. 179 (3), 130–139. doi:10.1016/j.toxlet.2008.04.015
Huang, D., Hu, C., Zeng, G., Cheng, M., Xu, P., Gong, X., et al. (2017). Combination of Fenton processes and biotreatment for wastewater treatment and soil remediation. Sci. Total Environ. 574, 1599–1610. doi:10.1016/j.scitotenv.2016.08.199
Huang, J., Zhan, G., Zheng, B., Sun, D., Lu, F., Lin, Y., et al. (2011a). Biogenic silver nanoparticles by Cacumen platycladi extract: synthesis, formation mechanism, and antibacterial activity. Ind. Eng. Chem. Res. 50, 9095–9106. doi:10.1021/ie200858y
Huang, L., Luo, F., Chen, Z., Megharaj, M., and Naidu, R. (2015). Green synthesized conditions impacting on the reactivity of Fe NPs for the degradation of malachite green. Spectrochim. Acta A 137, 154–159. doi:10.1016/j.saa.2014.08.116
Huang, L., Weng, X., Chen, Z., Megharaj, M., and Naidu, R. (2014a). Green synthesis of iron nanoparticles by various tea extracts: comparative study of the reactivity. Spectrochim. Acta A. Mol. Biomol. Spectrosc. 130, 295–301. doi:10.1016/j.saa.2014.04.037
Huang, L., Weng, X., Chen, Z., Megharaj, M., and Naidu, R. (2014b). Synthesis of iron-based nanoparticles using oolong tea extract for the degradation of malachite green. Spectrochim. Acta A. Mol. Biomol. Spectrosc. 117, 801–804. doi:10.1016/j.saa.2013.09.054
Huang, M., Khor, E., and Lim, L.-Y. (2004). Uptake and cytotoxicity of chitosan molecules and nanoparticles: effects of molecular weight and degree of deacetylation. Pharm. Res. 21 (2), 344–353. doi:10.1023/b:pham.0000016249.52831.a5
Huang, X., Liao, X., and Shi, B. (2011b). Synthesis of highly active and reusable supported gold nanoparticles and their catalytic applications to 4-nitrophenol reduction. Green Chem. 13, 2801–2805. doi:10.1039/c1gc15873b
Huo, C., Khoshnamvand, M., Liu, P., Yuan, C.-G., and Cao, W. (2018). Eco-friendly approach for biosynthesis of silver nanoparticles using Citrus maxima peel extract and their characterization, catalytic, antioxidant and antimicrobial characteristics. Mater. Res. Express 6, 015010. doi:10.1088/2053-1591/aae34c
Husein, D. Z., Hassanien, R., and Al-Hakkani, M. F. (2019). Green-synthesized copper nanoadsorbent for the removal of pharmaceutical pollutants from real wastewater samples. Heliyon 5, e02339. doi:10.1016/j.heliyon.2019.e02339
Hussain, S. M., Javorina, A. K., Schrand, A. M., Duhart, H. M., Ali, S. F., and Schlager, J. J. (2006). The interaction of manganese nanoparticles with PC-12 cells induces dopamine depletion. Toxicol. Sci. 92 (2), 456–463. doi:10.1093/toxsci/kfl020
Ijaz, F., Shahid, S., Khan, S. A., Ahmad, W., and Zaman, S. (2017). Green synthesis of copper oxide nanoparticles using Abutilon indicum leaf extract: antimicrobial, antioxidant and photocatalytic dye degradation activitie. Trop. J. Pharm. Res. 16, 743–753. doi:10.4314/tjpr.v16i4.2
International Pharmaceutical Regulators Programme (2020). International pharmaceutical regulators programme. Available at: http://www.iprp.global/home.InternationalStandardsOrganization(ISO),2015.ISO/TS80004-1:2015(en)Nanotechnologies–Vocabulary–Part1:CoreTerms.
Iram, M., Guo, C., Guan, Y. P., Ishfaq, A., and Liu, H. Z. (2010). Adsorption and magnetic removal of neutral red dye from aqueous solution using Fe3O4 hollow nanospheres. J. Hazard Mater 181, 1039–1050. doi:10.1016/j.jhazmat.2010.05.119
Iravani, S. (2011). Green synthesis of metal nanoparticles using plants. Green Chem. 13, 2638–2650. doi:10.1039/c1gc15386b
Iwohari, K., Watanabe, J.-I., Tani, Y., Seyama, H., and Miyatan, N. (2014). Removal of heavy metal cations by biogenic magnetite nanoparticles produced in Fe(III)- reducing microbial enrichment cultures. J. Biosci. Bioeng. 117 (3), 333–335. doi:10.1016/j.jbiosc.2013.08.013
Jaafar, A., El Ayouchia, H. B., Lakbaibi, Z., Boussaoud, A., Jodeh, S., Azzaoui, K., et al. (2019). Degradation of pollutant dye in aqueous solution using Fenton reaction: a DFT study. GP Glob. Res. J. Chem. 2 (1), 53–61.
Jaafara, A., Driouichb, A., Lakbaibib, Z., El Ayouchiac, H. B., Azzaouid, K., Boussaouda, A., et al. (2019). Central composite design for the optimization of Basic Red V degradation in aqueous solution using Fenton reaction. Desalin. Water Treat. 158, 364–371. doi:10.5004/dwt.2019.24261
Jabbar, S., Twentyman, P., and Watson, J. (1989). The MTT assay underestimates the growth inhibitory effects of interferons. Br. J. Cancer 60 (4), 523–528. doi:10.1038/bjc.1989.306
Jadoun, S., Arif, R., Jangid, N. K., and Meena, R. K. (2020). Green synthesis of nanoparticles using plant extracts: a review. Environ. Chem. Lett. 19, 355–374. doi:10.1007/s10311-020-01074-x
Jafarirad, S., Rasoulpour, I., Divband, B., Hammami Torghabe, I., and Kosari-Nasab, M. (2018). Innovative biocapped CuO nano-photocatalysts: a rapid and green method for photocatalytic degradation of 4-nitrophenol. Mater. Res. Innov. 22, 1–7. doi:10.1080/14328917.2017.1336838
Jagadevan, S., Jayamurthy, M., Dobson, P., and Thompson, I. P. (2012). A novel hybrid nano zerovalent iron initiated oxidation - biological degradation approach for remediation of recalcitrant waste metalworking fluids. Water Res. 46, 2395–2404. doi:10.1016/j.watres.2012.02.006
Jain, R., Dominic, D., Jordan, N., Rene, E. R., Weiss, S., van Hullebusch, E. D., et al. (2016a). Preferential adsorption of Cu in a multi-metal mixture onto biogenic elemental selenium nanoparticles. Chemical Engineering Journal 284, 917–925. doi:10.1016/j.cej.2015.08.144
Jain, R., Matassa, S., Singh, S., van Hullebusch, E. D., Esposito, G., and Lens, P. N. L. (2016b). Reduction of selenite to elemental selenium nanoparticles by activated sludge. Environmental Science and Pollution Research 23 (2), 1193–1202. doi:10.1007/s11356-015-5138-7
Jain, R., Jordan, N., Schild, D., Van Hullebusch, E. D., Weiss, S., Franzen, C., et al. (2015). Adsorption of zinc by biogenic elemental selenium nanoparticles. Chem. Eng. J. 260, 855–863. doi:10.1016/j.cej.2014.09.057
Jain, R., Mendiratta, S., Kumar, L., and Srivastava, A. (2021). Green synthesis of iron nanoparticles using Artocarpus heterophyllus peel extract and their application as a heterogeneous Fenton-like catalyst for the degradation of fuchsin basic dye. Curr. Opin. Green Sustain. Chem. 4, 100086. doi:10.1016/j.crgsc.2021.100086
Jain, S., Singh, S. R., and Pillai, S. (2012). Toxicity issues related to biomedical applications of carbon nanotubes. J. Nanomed. Nanotechnol. 3 (5), 1–15. doi:10.4172/2157-7439.1000140
Jantunen, P., Gottardo, S., and Crutzen, H. (2017). NANoREG Toolbox for the safety assessment of nanomaterials. European Commission, Joint Research Centre (JRC). EUR 28676 EN. Available at: http://data.europa.eu/89h/jrc-nano-ehs-ring-nanoreg-tb.
Jeliazkova, N., Chomenidis, C., Doganis, P., Fadeel, B., Grafström, R., Hardy, B., et al. (2015). The eNanoMapper database for nanomaterial safety information. Beilstein J. Nanotechnol. 6, 1609–1634. doi:10.3762/bjnano.6.165
Jeng, H. A., and Swanson, J. (2006). Toxicity of metal oxide nanoparticles in mammalian cells. J. Environ. Sci. Health Part A 41 (12), 2699–2711. doi:10.1080/10934520600966177
Jiang, W. (2019). “Regulating generic nanotechnology drug products: guidances and standards,” in Private communication at global Summit on regulatory science 2019 on nanotechnology and nanoplastics. EUR 30195 EN.
Jiang, Z., Liu, D., Jiang, D., Wei, W., Qian, K., Chen, M., et al. (2014). Bamboo leaf assisted formation of carbon/nitrogen co-doped anatase TiO 2 modified with silver and graphitic carbon nitride: novel and green synthesis and cooperative photocatalytic activity. J. Chem. Soc. Dalton Trans. 43, 13792–13802. doi:10.1039/c4dt02101k
Jin, Y., Kannan, S., Wu, M., and Zhao, J. X. (2007). Toxicity of luminescent silica nanoparticles to living cells. Chem. Res. Toxicol. 20 (8), 1126–1133. doi:10.1021/tx7001959
Jomova, K., Baros, S., and Valko, M. (2012). Redox active metal-induced oxidative stress in biological systems. Met. Chem. 37 (2), 127–134. doi:10.1007/s11243-012-9583-6
Jozsef, B., and Blaga, P. (2014). Production cost optimization in industrial wastewater treatment. Procedia Econ. Financ. 15, 1463–1469. doi:10.1016/S2212-5671(14)00612-1
Kalbar, P. P., Karmakar, S., and Asolekar, S. R. (2012). Selection of an appropriate wastewater treatment technology: a scenario-based multiple-attribute decision-making approach. J. Environ. Manag. 113, 158–169. doi:10.1016/j.jenvman.2012.08.025
Kaliraj, L., Ahn, J. C., Rupa, E. J., Abid, S., Lu, J., and Yang, D. C. (2019). Synthesis of panos extract mediated ZnO nano-flowers as photocatalyst for industrial dye degradation by UV illumination. J. Photochem. Photobiol. B, Biol. 199, 111588. doi:10.1016/j.jphotobiol.2019.111588
Kalpana, V., Chakraborthy, P., Palanichamy, V., and Rajeswari, V. D. (2016). Synthesis and characterization of copper nanoparticles using Tridax procumbens and its application in degradation of bismarck brown. Analysis 10, 17.
Kalpana, V., and Rajeswari, V. D. (2018). Synthesis of palladium nanoparticles via a green route using Lagenaria siceraria: assessment of their innate antidandruff, insecticidal and degradation activities. Mater. Res. Express 5, 115406. doi:10.1088/2053-1591/aaddef
Kamali, M., and Khodaparast, Z. (2015). Review on recent developments on pulp and paper mill wastewater treatment. Ecotoxicol. Environ. Saf. 114, 326–342. doi:10.1016/j.ecoenv.2014.05.005
Kamali, M., Persson, K. M., Costa, M. E., and Capela, I. (2019). Sustainability criteria for assessing nanotechnology applicability in industrial wastewater treatment: current status and future outlook. Environ. Int. 125, 261–276. doi:10.1016/j.envint.2019.01.055
Kandasamy, R. (2017). A novel single step synthesis and surface functionalization of iron oxide magnetic nanoparticles and thereof for the copper removal from pigment industry effluent. Sep. Purif. Technol. 188, 458–467. doi:10.1016/j.seppur.2017.07.059
Kanoh, M., Takemura, G., Misao, J., Hayakawa, Y., Aoyama, T., Nishigaki, K., et al. (1999). Significance of myocytes with positive DNA in situ nick end-labeling (TUNEL) in hearts with dilated cardiomyopathy: not apoptosis but DNA repair. Circulation 99 (21), 2757–2764. doi:10.1161/01.cir.99.21.2757
Karim, A. V., Hassani, A., Eghbali, P., and Nidheesh, P. V. (2022). Nanostructured modified layered double hydroxides (LDHs)-based catalysts: a review on synthesis, characterization, and applications in water remediation by advanced oxidation processes. Curr. Opin. Solid State Mater. Sci. 26, 100965. doi:10.1016/j.cossms.2021.100965
Karlsson, M. N. A., Deppert, K., Wacaser, B. A., Karlsson, L. S., and Malm, J. O. (2005). Size-controlled nanoparticles by thermal cracking of iron pentacarbonyl. Appl. Phys. A Mater Sci. Process 80, 1579–1583. doi:10.1007/s00339-004-2987-1
Karthiga Devi, G., Senthil Kumar, P., and Sathish Kumar, K. (2016). Green synthesis of novel silver nanocomposite hydrogel based on sodium alginate as an efficient bio-sorbent for the dye wastewater treatment: prediction of isotherm and kinetic parameters. Desalin. Water Treat. 57, 27686–27699. doi:10.1080/19443994.2016.1178178
Karthik, C., Swathi, N., Pandi Prabha, S., and Caroline, D. G. (2020). Green synthesized rGOAgNP hybrid nanocomposite – an effective antibacterial adsorbent for photocatalytic removal of DB-14 dye from aqueous solution. J. Environ. Chem. Eng. 8, 103577. doi:10.1016/j.jece.2019.103577
Karthika, V., Arumugam, A., Gopinath, K., Kaleeswarran, P., Govindarajan, M., Alharbi, N. S., et al. (2017). Guazuma ulmifolia barksynthesized Ag, Au and Ag/Au alloy nanoparticles: photocatalytic potential, DNA/protein interactions, anticancer activity and toxicity against 14 species of microbial pathogens. J. Photochem. Photobiol. B, Biol. 167, 189–199. doi:10.1016/j.jphotobiol.2017.01.008
Kashefi, K., and Lovley, D. R. (2000). Reduction of Fe (III), Mn (IV), and toxic metals at 100 C by Pyrobaculum islandicum. Appl. Environ. Microbiol. 66, 1050–1056. doi:10.1128/aem.66.3.1050-1056.2000
Katata-Seru, L., Moremedi, T., Aremu, O. S., and Bahadur, I. (2018). Green synthesis of iron nanoparticles using Moringa oleifera extracts and their applications: removal of nitrate from water and antibacterial activity against Escherichia coli. J. Mol. Liq. 256, 296–304. doi:10.1016/j.molliq.2017.11.093
Kathiravan, V. (2018). Green synthesis of silver nanoparticles using different volumes of Trichodesma indicum leaf extract and their antibacterial and photocatalytic activities. Res. Chem. Intermed. 44, 4999–5012. doi:10.1007/s11164-018-3405-1
Kaviya, S., and Prasad, E. (2015). Biogenic synthesis of ZnO–Ag nano custard apples for efficient photocatalytic degradation of methylene blue by sunlight irradiation. RSC Adv. 5, 17179–17185. doi:10.1039/c4ra15293j
Kawata, K., Osawa, M., and Okabe, S. (2009). In vitro toxicity of silver nanoparticles at noncytotoxic doses to HepG2 human hepatoma cells. Environ. Sci. Technol. 43 (15), 6046–6051. doi:10.1021/es900754q
Kerfahi, D., Tripathi, B. M., Singh, D., Kim, H., Lee, S., Lee, J., et al. (2015). Effects of functionalized and raw multi-walled carbon nanotubes on soil bacterial community composition. PLoS One 10 (3), e0123042. doi:10.1371/journal.pone.0123042
Kerr, J. F., Wyllie, A. H., and Currie, A. R. (1972). Apoptosis: a basic biological phenomenon with wide-ranging implications in tissue kinetics. Br. J. Cancer 26 (4), 239–257. doi:10.1038/bjc.1972.33
Khan, S. A., Noreen, F., Kanwal, S., Iqbal, A., and Hussain, G. (2018). Green synthesis of ZnO and Cu-doped ZnO nanoparticles from leaf extracts of Abutilon indicum, Clerodendrum infortunatum, Clerodendrum inerme and investigation of their biological and photocatalytic activities. Mater. Sci. Eng. C 82, 46–59. doi:10.1016/j.msec.2017.08.071
Khan, Z. U. H., Khan, A., Shah, A., Wan, P., Chen, Y., Khan, G. M., et al. (2016). Enhanced photocatalytic and electrocatalytic applications of green synthesized silver nanoparticles. J. Mol. Liq. 220, 248–257. doi:10.1016/j.molliq.2016.04.082
Khan, Z. U. H., Sadiq, H. M., Shah, N. S., Khan, A. U., Muhammad, N., Hassan, S. U., et al. (2019). Greener synthesis of zinc oxide nanoparticles using Trianthema portulacastrum extract and evaluation of its photocatalytic and biological applications. J. Photochem. Photobiol. B, Biol. 192, 147–157. doi:10.1016/j.jphotobiol.2019.01.013
Khodadadi, B., Bordbar, M., and Nasrollahzadeh, M. (2017a). Achillea millefolium L. Extract mediated green synthesis of waste peach kernel shell supported silver nanoparticles: application of the nanoparticles for catalytic reduction of a variety of dyes in water. J. Colloid Interface Sci. 493, 85–93. doi:10.1016/j.jcis.2017.01.012
Khodadadi, B., Bordbar, M., Yeganeh-Faal, A., and Nasrollahzadeh, M. (2017b). Green synthesis of Ag nanoparticles/clinoptilolite using Vaccinium macrocarpon fruit extract and its excellent catalytic activity for reduction of organic dyes. J. Alloys. Compd. 719, 82–88. doi:10.1016/j.jallcom.2017.05.135
Khoshnamvand, M., Huo, C., and Liu, J. (2019). Silver nanoparticles synthesized using Allium ampeloprasum L. Leaf extract: characterization and performance in catalytic reduction of 4-nitrophenol and antioxidant activity. J. Mol. Struct. 1175, 90–96. doi:10.1016/j.molstruc.2018.07.089
Kim, D.-H., Kanaly, R. A., and Hur, H. G. (2012). Biological accumulation of tellurium nanorod structures via reduction of tellurite by Shewanella oneidensis MR-1. Bioresour. Technol. 125, 127–131. doi:10.1016/j.biortech.2012.08.129
Kim, E. J., and Baek, K. (2019). Selective recovery of ferrous oxalate and removal of arsenic and other metals from soil-washing wastewater using a reduction reaction. J. Clean. Prod. 221, 635–643. doi:10.1016/j.jclepro.2019.03.014
Kim, S. C., Kim, D. W., Shim, Y. H., Bang, J. S., Oh, H. S., Kim, S. W., et al. (2001). In vivo evaluation of polymeric micellar paclitaxel formulation: toxicity and efficacy. J. Control. Release 72 (1), 191–202. doi:10.1016/s0168-3659(01)00275-9
Kim, S. D., Cho, J., Kim, I. S., Vanderford, B. J., and Snyder, S. A. (2007). Occurrence and removal of pharmaceuticals and endocrine disruptors in South Korean surface, drinking, and waste waters. Water Res. 41, 1013–1021. doi:10.1016/j.watres.2006.06.034
Kim, Y.-J., Choi, H. S., Song, M., Youk, D., Kim, J., and Ryu, J. (2009). Genotoxicity of aluminum oxide (Al2O3) nanoparticle in mammalian cell lines. Mol. Cell. Toxicol. 5 (2), 172–178.
Kisin, E. R., Murray, A. R., Keane, M. J., Shi, X. C., Schwegler-Berry, D., Gorelik, O., et al. (2007). Single-walled carbon nanotubes: geno- and cytotoxic effects in lung fibroblast V79 cells. J. Toxicol. Environ. Health Part A 70 (24), 2071–2079. doi:10.1080/15287390701601251
Koba, O., and Biro, L. (2015). Fenton-like reaction: a possible way to efficiently remove illicit drugs and pharmaceuticals from wastewater. Environ. Toxicol. Pharmacol. 9, 483–488. doi:10.1016/j.etap.2014.12.016
Kong, D., and Wilson, L. D. (2020). Uptake of methylene blue from aqueous solution by pectin–chitosan binary composites. J. Compos. Sci. 4 (3), 95. doi:10.3390/jcs4030095
Konishi, Y., Tsukiyama, T., Tachimi, T., Saitoh, N., Nomura, T., and Nagamine, S. (2007). Microbial deposition of gold nanoparticles by the metal-reducing bacterium Shewanella algae. Electrochim. Acta 53, 186–192. doi:10.1016/j.electacta.2007.02.073
Kononenko, V., Repar, N., Marušič, N., Drašler, B., Romih, T., Hočevar, S., et al. (2017). Comparative in vitro genotoxicity study of ZnO nanoparticles, ZnO macroparticles and ZnCl2 to MDCK kidney cells: size matters. Toxicol. Vitro 40, 256–263. doi:10.1016/j.tiv.2017.01.015
Kora, A. J., and Rastogi, L. (2018). Green synthesis of palladium nanoparticles using gum ghatti (Anogeissus latifolia) and its application as an antioxidant and catalyst. Arab. J. Chem. 11, 1097–1106. doi:10.1016/j.arabjc.2015.06.024
Korbekandi, H., Chitsazi, M. R., Asghari, G., Najafi, R. B., Badii, A., and Iravani, S. (2014). Green biosynthesis of silver nanoparticles using Azolla pinnata whole plant hydroalcoholic extract. Green process. Synth. 3, 365–373. doi:10.1515/gps-2014-0042
Krageloh, A., Suarez-Merino, B., Sluijters, T., and Micheletti, C. (2018). Implementation of safe-by-design for nanomaterial development and safe innovation: why we need a comprehensive approach. Nanomaterials 8, 239. doi:10.3390/nano8040239
Krause, C. J. (2014). Evaluating the toxicity of nitrogen-functionalized carbon nanotubes MS (Master of Science) thesis. United States: University of Iowa. Available at: http://ir.uiowa.edu/etd/4667 (Accessed August 15, 2014).
Kuan, C., Chang, S., and Schroeder, S. L. M. (2015). Fenton-like oxidation of 4 - chlorophenol: homogeneous or heterogeneous? Ind. Eng. Chem. Res. 54, 8122–8129. doi:10.1021/acs.iecr.5b02378
Kuang, Y., Wang, Q., Chen, Z., Megharaj, M., and Naidu, R. (2013). Heterogeneous Fenton-like oxidation of monochlorobenzene using green synthesis of iron nanoparticles. J. Colloid Interface Sci. 410, 67–73. doi:10.1016/j.jcis.2013.08.020
Kumar, A., and Dhawan, A. (2013b). Genotoxic and carcinogenic potential of engineered nanoparticles: an update. Arch. Toxicol. 87 (11), 1883–1900. doi:10.1007/s00204-013-1128-z
Kumar, A., Sharma, V., and Dhawan, A. (2013a). “Methods for detection of oxidative stress and genotoxicity of engineered nanoparticles,” in Oxidative stress and nanotechnology. Methods in molecular biology (methods and protocols). Editors D. Armstrong,, and D. Bharali (Totowa, NJ: Humana Press), 1028, 231–246. doi:10.1007/978-1-62703-475-3_15
Kumar, V., Sharma, N., and Maitra, S. S. (2017). In vitro and in vivo toxicity assessment of nanoparticles. International Nano Letters 7, 243–256. doi:10.1007/s40089-017-0221-3
Kumar, B., Smita, K., Angulo, Y., and Cumbal, L. (2016). Valorization of rambutan peel for the synthesis of silver-doped titanium dioxide (Ag/TiO2) nanoparticles. Green process. Synth. 5, 371–377. doi:10.1515/gps-2016-0003
Kumar, B., Smita, K., Cumbal, L., and Debut, A. (2015). Ultrasound agitated phyto-fabrication of palladium nanoparticles using Andean blackberry leaf and its photocatalytic activity. J. Saudi Chem. Soc. 19, 574–580. doi:10.1016/j.jscs.2015.05.008
Kumar, B., Vizuete, K. S., Sharma, V., Debut, A., and Cumbal, L. (2019). Ecofriendly synthesis of monodispersed silver nanoparticles using Andean Mortiño berry as reductant and its photocatalytic activity. Vacuum 160, 272–278. doi:10.1016/j.vacuum.2018.11.027
Kumar, J. A., Krithiga, T., Manigandan, S., Sathish, S., Renita, A. A., Prakash, P., et al. (2021). A focus to green synthesis of metal/metal based oxide nanoparticles: various mechanisms and applications towards ecological approach. J. Clean. Prod. 324, 129198. doi:10.1016/j.jclepro.2021.129198
Kumar, K. M., Mandal, B. K., Kumar, K. S., Reddy, P. S., and Sreedhar, B. (2013). Biobased green method to synthesise palladium and iron nanoparticles using Terminalia chebula aqueous extract. Spectrochim. Acta Part A 102, 128–133. doi:10.1016/j.saa.2012.10.015
Kumar, M., Ambika, S., Hassani, A., and Nidheesh, P. V. (2023). Waste to catalyst: role of agricultural waste in water and wastewater treatment. Sci. Total Environ. 858, 159762. doi:10.1016/j.scitotenv.2022.159762
Lai, C., Wang, M.-M., Zeng, G.-M., Liu, Y.-G., Huang, D.-L., Zhang, C., et al. (2016). Synthesis of surface molecular imprinted TiO2/graphene photocatalyst and its highly efficient photocatalytic degradation of target pollutant under visible light irradiation. Appl. Surf. Sci. 390, 368–376. doi:10.1016/j.apsusc.2016.08.119
Lapworth, D., Baran, N., Stuart, M., and Ward, R. (2012). Emerging organic contaminants in groundwater: a review of sources, fate and occurrence. Environ. Pollut. 163, 287–303. doi:10.1016/j.envpol.2011.12.034
Lateef, A., Akande, M. A., Ojo, S. A., Folarin, B. I., Gueguim-Kana, E. B., and Beukes, L. S. (2016). Paper wasp nest-mediated biosynthesis of silver nanoparticles for antimicrobial, catalytic, anticoagulant, and thrombolytic applications. 3 Biotech. 6, 140. doi:10.1007/s13205-016-0459-x
Lee, H., Purdon, A. M., Chu, V., and Westervelt, R. M. (2004). Controlled assembly of magnetic nanoparticles from magnetotactic bacteria using microelectromagnets arrays. Nano Lett. 4, 995–998. doi:10.1021/nl049562x
Lee, J. Y., Tang, C. Y., and Huo, F. (2014). Fabrication of porous matrix membrane (PMM) using metal-organic framework as green template for water treatment. Sci. Rep. 4, 3740. doi:10.1038/srep03740
Lee, K. J., Nallathamby, P. D., Browning, L. M., Osgood, C. J., and Xu, X. H. N. (2007). In vivo imaging of transport and biocompatibility of single silver nanoparticles in early development of zebrafish embryos. ACS Nano 1 (2), 133–143. doi:10.1021/nn700048y
Lei, R., Wu, C., Yang, B., Ma, H., Shi, C., Wang, Q., et al. (2008). Integrated metabolomic analysis of the nano-sized copper particle-induced hepatotoxicity and nephrotoxicity in rats: a rapid invivo screening method for nanotoxicity. Toxicol. Appl. Pharmacol. 232 (2), 292–301. doi:10.1016/j.taap.2008.06.026
Lemay, M., and Wood, K. A. (1999). Detection of DNA damage and identifcation of UV-induced photoproducts using the CometAssay [TM] Kit. Biotechniques 27, 846–848. doi:10.2144/99274pf01
Li, G.-Y., and Osborne, N. N. (2008). Oxidative-induced apoptosis to an immortalized ganglion cell line is caspase independent but involves the activation of poly (ADP-ribose) polymerase and apoptosis-inducing factor. Brain Res. 1188, 35–43. doi:10.1016/j.brainres.2007.10.073
Li, J. J., Hartono, D., Ong, C. N., Bay, B. H., and Yung, L. Y. L. (2010). Autophagy and oxidative stress associated with gold nanoparticles. Biomaterials 31 (23), 5996–6003. doi:10.1016/j.biomaterials.2010.04.014
Li, W., Li, L., and Qiu, G. (2017b). Energy consumption and economic cost of typical wastewater treatment systems in Shenzhen, China. J. Clean. Prod. 163, S374–S378. doi:10.1016/j.jclepro.2015.12.109
Li, X., Yang, L., Zhou, J., Dai, B., Gan, D., Yang, Y., et al. (2024). Biogenic palladium nanoparticles for wastewater treatment: formation, applications, limitations, and future directions. J. Water Process Eng. 64, 105641. doi:10.1016/j.jwpe.2024.105641
Li, Y. H., Cheng, S. W., Yuan, C. S., Lai, T. F., and Hung, C. H. (2018). Removing volatile organic compounds in cooking fume by nano-sized TiO2 photocatalytic reaction combined with ozone oxidation technique. Chemosphere 208, 808–817. doi:10.1016/j.chemosphere.2018.06.035
Li, Y.-P., Pei, Y. Y., Zhang, X. Y., Gu, Z. H., Zhou, Z. H., Yuan, W. F., et al. (2001). PEGylated PLGA nanoparticles as protein carriers: synthesis, preparation and biodistribution in rats. J. Control. Release 71 (2), 203–211. doi:10.1016/s0168-3659(01)00218-8
Liang, S. H., and Clarke, M. F. (2001). Regulation of p53 localization. FEBS J. 268 (10), 2779–2783. doi:10.1046/j.1432-1327.2001.02227.x
Liao, Z.-L., Li, G.-D., Bi, M.-H., and Chen, J.-S. (2008). Preparation, structures, and photocatalytic properties of three new uranyl− organic assembly compounds. Inorg. Chem. 47, 4844–4853. doi:10.1021/ic800109y
Lifen, L., Chuanqi, Z., and Fenglin, Y. (2012). TiO2 and polyvinyl alcohol (PVA) coated polyester filter in bioreactor for wastewater treatment. Water Res. 46, 1969–1978. doi:10.1016/j.watres.2012.01.017
Lima Da Cuha, C. E. (2019). “Safe by design: creating nanomaterials of tomorrow,” in Private communication at global Summit on regulatory science 2019 on nanotechnology and nanoplastics. EUR 30195 EN.
Lin, H., and Maggard, P. A. (2008). Synthesis and structures of a new series of silver-vanadate hybrid solids and their optical and photocatalytic properties. Inorg. Chem. 47, 8044–8052. doi:10.1021/ic8004129
Lingamdinne, L. P., Chang, Y.-Y., Yang, J.-K., Singh, J., Choi, E.-H., Shiratani, M., et al. (2017). Biogenic reductive preparation of magnetic inverse spinel iron oxide nanoparticles for the adsorption removal of heavy metals. Chem. Eng. J. 307, 74–84. doi:10.1016/j.cej.2016.08.067
Liu, W.-W., and Peng, R.-F. (2020). Recent advances of bismuth oxychloride photocatalytic material: property, preparation and performance enhancement. J. Electron. Sci. Technol. 18 (2), 100020. doi:10.1016/j.jnlest.2020.100020
Lloyd, J., and Macaskie, L. (1996). A novel PhosphorImager-Based technique for monitoring the microbial reduction of technetium. Appl. Environ. Microbiol. 62, 578–582. doi:10.1128/aem.62.2.578-582.1996
Lloyd, J. R., and Macaskie, L. E. (2000). “Bioremediation of radionuclide-containing wastewaters,” in Environmental microbe-metal interactions. Abstr. Gen. Meet. Am. Soc. Microbiol, 277–327.
Long, S., Zhao, L., Shi, T., Li, J., Yang, J., Liu, H., et al. (2018). Pollution control and cost analysis of wastewater treatment at industrial parks in Taihu and Haihe water basins, China. J. Clean. Prod. 172, 2435–2442. doi:10.1016/j.jclepro.2017.11.167
Loo, D. T. , and (2002). “An overview of techniques,” in detection of DNA damage: methods and protocols. Editors Didenko,, and V. Vladimir (New York, United States: Humana Press), 21–30.
Lopez-Miranda, J. L., González, M. V., Mares-Briones, F., Cervantes-Chávez, J., Esparza, R., Rosas, G., et al. (2018). Catalytic and antibacterial evaluation of silver nanoparticles synthesized by a green approach. Res. Chem. Intermed. 44, 7479–7490. doi:10.1007/s11164-018-3568-9
Low, S. P., Williams, K., Canham, L., and Voelcker, N. (2006). Evaluation of mammalian cell adhesion on surface-modified porous silicon. Biomaterials 27 (26), 4538–4546. doi:10.1016/j.biomaterials.2006.04.015
Lu, X., Liu, C., Lu, J., Tang, W., Gan, Q., Zhou, H., et al. (2011). In vitro cytotoxicity and induction of apoptosis by silica nanoparticles in human HepG2 hepatoma cells. Int. J. Nanomed. 6, 1889. doi:10.2147/ijn.s24005
Lunge, S., Singh, S., and Sinha, A. (2014). Magnetic iron oxide (Fe3O4) nanoparticles from tea waste for arsenic removal. J. Magn. Magn. Mater. 356, 21–31. doi:10.1016/j.jmmm.2013.12.008
Luo, F., Yang, D., Chen, Z., Megharaj, M., and Naidu, R. (2016). One-step green synthesis of bimetallic Fe/Pd nanoparticles used to degrade Orange II. J. Hazard. Mater. 303, 145–153. doi:10.1016/j.jhazmat.2015.10.034
Lynch, I. (2019). “Harmonising detection, quantification and impact assessment of micro/nanoplastics in freshwater environmental hotspots,” in Private communication at global summit on regulatory science 2019 on nanotechnology and nanoplastics. EUR 30195 EN.
Machado, S., Pinto, S. L., Grosso, J. P., Nouws, H. P. A., Albergaria, J. T., and Delerue-Matos, C. (2013b). Green production of zero-valent iron nanoparticles using tree leaf extracts. Sci. Total Environ. 445–446, 1–8. doi:10.1016/j.scitotenv.2012.12.033
Machado, S., Stawiński, W., Slonina, P., Pinto, A., Grosso, J., Nouws, H., et al. (2013a). Application of green zero-valent iron nanoparticles to the remediation of soils contaminated with ibuprofen. Sci. Total Environ. 461, 323–329. doi:10.1016/j.scitotenv.2013.05.016
Madani, S. Y., Mandel, A., and Seifalian, A. M. (2013). A concise review of carbon nanotube’s toxicology. Nano Rev. Exp. 4, 21521–21534. doi:10.3402/nano.v4i0.21521
Madhavi, V., Prasad, T., Reddy, A. V. B., Reddy, B. R., and Madhavi, G. (2013). Application of phytogenic zerovalent iron nanoparticles in the adsorption of hexavalent chromium. Spectrochim. Acta A. Mol. Biomol. Spectrosc. 116, 17–25. doi:10.1016/j.saa.2013.06.045
Magder, S. (2006). Reactive oxygen species: toxic molecules or spark of life? Crit. Care 10 (1), 208. doi:10.1186/cc3992
Malyapa, R. S., Bi, C., Ahern, E. W., and Roti, J. L. R. (1998). Detection of DNA damage by the alkaline comet assay after exposure to low-dose gamma radiation. Radiat. Res. 149 (4), 396–400. doi:10.2307/3579703
Manjari, G., Saran, S., Arun, T., Rao, A. V. B., and Devipriya, S. P. (2017). Catalytic and recyclability properties of phytogenic copper oxide nanoparticles derived from Aglaia elaeagnoidea flower extract. J. Saudi Chem. Soc. 21, 610–618. doi:10.1016/j.jscs.2017.02.004
Markova, Z., Novak, P., Kaslik, J., Plachtova, P., Brazdova, M., Jancula, D., et al. (2014). Iron(II,III)–Polyphenol complex nanoparticles derived from green tea with remarkable ecotoxicological impact. ACS Sustain. Chem. Eng. 2, 1674–1680. doi:10.1021/sc5001435
Marshall, N. J., Goodwin, C. J., and Holt, S. J. (1995). A critical assessment of the use of microculture tetrazolium assays to measure cell growth and function. Growth Regul. 5 (2), 69–84.
Martínez-Cabanas, M., López-García, M., Barriada, J. L., Herrero, R., and Sastre de Vicente, M. E. (2016). Green synthesis of iron oxide nanoparticles. Development of magnetic hybrid materials for efficient As(V) removal. Chem. Eng. J. 301, 83–91. doi:10.1016/j.cej.2016.04.149
Martins, M., Mourato, C., Sanches, S., Noronha, J. P., Crespo, M. B., and Pereira, I. A. (2017). Biogenic platinum and palladium nanoparticles as new catalysts for the removal of pharmaceutical compounds. Water Res. 108, 160–168. doi:10.1016/j.watres.2016.10.071
Maruthai, J., Muthukumarasamy, A., and Baskaran, B. (2018). Optical, biological and catalytic properties of ZnO/MgO nanocomposites derived via Musa paradisiaca bract extract. Ceram. Int. 44, 13152–13160. doi:10.1016/j.ceramint.2018.04.138
Maryami, M., Nasrollahzadeh, M., Mehdipour, E., and Sajadi, S. M. (2016). Preparation of the Ag/RGO nanocomposite by use of Abutilon hirtum leaf extract: a recoverable catalyst for the reduction of organic dyes in aqueous medium at room temperature. Int. J. Hydrogen Energy 41, 21236–21245. doi:10.1016/j.ijhydene.2016.09.130
Maryami, M., Nasrollahzadeh, M., and Sajadi, S. M. (2017). Green synthesis of the Pd/perlite nanocomposite using Euphorbia neriifolia L. Leaf extract and evaluation of its catalytic activity. Sep. Purif. Technol. 184, 298–307. doi:10.1016/j.seppur.2017.05.003
Maurer, L., and Meyer, J. (2016). A systematic review of evidence for silver nanoparticle-induced mitochondrial toxicity. Environ. Sci. Nano 3 (2), 311–322. doi:10.1039/c5en00187k
McMahon, S. J., Hyland, W. B., Muir, M. F., Coulter, J. A., Jain, S., Butterworth, K. T., et al. (2011). Biological consequences of nanoscale energy deposition near irradiated heavy atom nanoparticles. Sci. Rep. 1 (18), 18. doi:10.1038/srep00018
McNamara, K., and Tofail, S. A. M. (2017). Nanoparticles in biomedical applications. Advances in Physics: X 2 (1), 54–88. doi:10.1080/23746149.2016.1254570
Mech, A., et al. (2020b). The NanoDefine methods manual. Part 1: the NanoDefiner framework and tools; Part 2: evaluation of methods; Part 3: standard operating procedures. Luxembourg: Publications Office of the European Union. 29876. EUR.
Mech, A., Wohlleben, W., Ghanem, A., Hodoroaba, V. D., Weigel, S., Babick, F., et al. (2020a). Nano or not nano? A structured approach for identifying nanomaterials according to the European Commission’s definition. Small 16, 2002228. doi:10.1002/smll.202002228
Mehr, E. S., Sorbiun, M., Ramazani, A., and Fardood, S. T. (2018). Plant-mediated synthesis of zinc oxide and copper oxide nanoparticles by using ferulago angulata (schlecht) boiss extract and comparison of their photocatalytic degradation of Rhodamine B (RhB) under visible light irradiation. J. Mater. Sci. Mater. Electron. 29, 1333–1340. doi:10.1007/s10854-017-8039-3
Miranda, R. R., Bezerra Jr, A. G., Oliveira Ribeiro, C. A., Randi, M. A. F., Voigt, C. L., Skytte, L., et al. (2017). Toxicological interactions of silver nanoparticles and non-essential metals in human hepatocarcinoma cell line. Toxicol. Vitro 40, 134–143. doi:10.1016/j.tiv.2017.01.003
Miri, A., Mousavi, S. R., Sarani, M., and Mahmoodi, Z. (2018a). Using biebersteinia multifida aqueous extract, the photocatalytic activity of synthesized silver nanoparticles. Orient. J. Chem. 34, 1513–1517. doi:10.13005/ojc/340342
Miri, A., Vahed, H. O. S., and Sarani, M. (2018b). Biosynthesis of silver nanoparticles and their role in photocatalytic degradation of methylene blue dye. Res. Chem. Intermed. 44, 6907–6915. doi:10.1007/s11164-018-3529-3
Mishra, A., Kumari, M., Pandey, S., Chaudhry, V., Gupta, K., and Nautiyal, C. (2014). Biocatalytic and antimicrobial activities of gold nanoparticles synthesized by Trichoderma sp. Bioresour. Technol. 166, 235–242. doi:10.1016/j.biortech.2014.04.085
Mittal, A. K., Chisti, Y., and Banerjee, U. C. (2013). Synthesis of metallic nanoparticles using plant extracts. Biotechnol. Adv. 31, 346–356. doi:10.1016/j.biotechadv.2013.01.003
Miura, N., and Shinohara, Y. (2009). Cytotoxic effect and apoptosis induction by silver nanoparticles in HeLa cells. Biochem. Biophys. Res. Commun. 390 (3), 733–737. doi:10.1016/j.bbrc.2009.10.039
Mo, Y., and Lim, L.-Y. (2005). Paclitaxel-loaded PLGA nanoparticles: potentiation of anticancer activity by surface conjugation with wheat germ agglutinin. J. Control. Release 108 (2–3), 244–262. doi:10.1016/j.jconrel.2005.08.013
Mohamed, H. H., Alomair, N. A., Akhtar, S., and Youssef, T. E. (2019). Eco-friendly synthesized α-Fe2O3/TiO2 heterojunction with enhanced visible light photocatalytic activity. J. Photochem. Photobiol. A Chem. 382, 111951. doi:10.1016/j.jphotochem.2019.111951
Mohamed, M. H., Udoetok, I. A., Solgi, M., Steiger, B. G. K., Zhou, Z., and Wilson, L. D. (2022). Design of sustainable biomaterial composite adsorbents for point-of-use removal of lead ions from water. Front. Water 4. doi:10.3389/frwa.2022.739492
Mohamed, M. H., Udoetok, I. A., and Wilson, L. D. (2020). Animal biopolymer-plant biomass composites: synergism and improved sorption efficiency. J. Compos. Sci. 4 (1), 15. doi:10.3390/jcs4010015
Mohammadzadeh Pakdel, P., and Peighambardoust, S. J. (2018). Review on recent progress in chitosan-based hydrogels for wastewater treatment application. Carbohydr. Polym. 201, 264–279. doi:10.1016/j.carbpol.2018.08.070
Molinari, B. L., Tasat, D. R., Palmieri, M. A., O'Connor, S. E., and Cabrini, R. L. (2003). Cell-based quantitative evaluation of the MTT assay. Anal. Quant. Cytol. Histol. 25 (5), 254–262.
Molinos-Senante, M., Garrido-Baserba, M., Reif, R., Hernández-Sancho, F., and Poch, M. (2012). Assessment of wastewater treatment plant design for small communities: environmental and economic aspects. Sci. Total Environ. 427–428, 11–18. doi:10.1016/j.scitotenv.2012.04.023
Molinos-Senante, M., Hernández-Sancho, F., and Sala-Garrido, R. (2010). Economic feasibility study for wastewater treatment: a cost-benefit analysis. Sci. Total Environ. 408, 4396–4402. doi:10.1016/j.scitotenv.2010.07.014
Momeni, S. S., Nasrollahzadeh, M., and Rustaiyan, A. (2016). Green synthesis of the Cu/ZnO nanoparticles mediated by Euphorbia prolifera leaf extract and investigation of their catalytic activity. J. Colloid Interface Sci. 472, 173–179. doi:10.1016/j.jcis.2016.03.042
Monteiro-Riviere, N. A., Nemanich, R. J., Inman, A. O., Wang, Y. Y., and Riviere, J. E. (2005). Multi-walled carbon nanotube interactions with human epidermal keratinocytes. Toxicol. Lett. 155 (3), 377–384. doi:10.1016/j.toxlet.2004.11.004
Moulton, M. C., Braydich-Stolle, L. K., Nadagouda, M. N., Kunzelman, S., Hussain, S. M., and Varma, R. S. (2010). Synthesis, characterization and biocompatibility of “green” synthesized silver nanoparticles using tea polyphenols. Nanoscale 2, 763–770. doi:10.1039/c0nr00046a
Mukherjee, D., Ghosh, S., Majumdar, S., and Annapurna, K. (2016). Green synthesis of α- Fe2O3 nanoparticles for arsenic (V) remediation with a novel aspect for sludge management. J. Environ. Chem. Eng. 4, 639–650. doi:10.1016/j.jece.2015.12.010
Mukherjee, P., Ahmad, A., Mandal, D., Senapati, S., Sainkar, S. R., Khan, M. I., et al. (2001). Fungus-mediated synthesis of silver nanoparticles and their immobilization in the mycelial matrix: a novel biological approach to nanoparticle synthesis. Nano Lett. 1, 515–519. doi:10.1021/nl0155274
Muthukumar, H., Gire, A., Kumari, M., and Manickam, M. (2017). Biogenic synthesis of nanobiomaterial for toxic naphthalene photocatalytic degradation optimization and kinetics studies. Int. Biodeterior. Biodegrad. 119, 587–594. doi:10.1016/j.ibiod.2016.10.036
Muthukumar, H., and Matheswaran, M. (2015). Amaranthus spinosus leaf extract mediated FeO nanoparticles: physicochemical traits, photocatalytic and antioxidant activity. ACS Sustain. Chem. Eng. 3, 3149–3156. doi:10.1021/acssuschemeng.5b00722
Mystrioti, C., Xanthopoulou, T., Tsakiridis, P., Papassiopi, N., and Xenidis, A. (2016). Comparative evaluation of five plant extracts and juices for nanoiron synthesis and application for hexavalent chromium reduction. Sci. Total Environ. 539, 105–113. doi:10.1016/j.scitotenv.2015.08.091
Nadaf, N. Y., and Kanase, S. S. (2016). Biosynthesis of gold nanoparticles by Bacillus marisflavi and its potential in catalytic dye degradation. Arab. J. Chem. 12 (8), 4806–4814. doi:10.1016/j.arab.jc.2016.09.020
Nadagouda, M. N., Castle, A. B., Murdock, R. C., Hussain, S. M., and Varma, R. S. (2010). In vitro biocompatibility of nanoscale zerovalent iron particles (NZVI) synthesized using tea polyphenols. Green Chem. 12, 114–122. doi:10.1039/b921203p
Nadagouda, M. N., and Varma, R. S. (2008). Green synthesis of silver and palladium nanoparticles at room temperature using coffee and tea extract. Green Chem. 10, 859–862. doi:10.1039/b804703k
Nair, B., and Pradeep, T. (2002). Coalescence of nanoclusters and formation of submicron crystallites assisted by Lactobacillus strains. Cryst. Growth Des. 2, 293–298. doi:10.1021/cg0255164
Nakum, J., and Bharracharya, D. (2022). Various green nanomaterials used for wastewater and soil treatment: a mini-review. Front. Environ. Sci. 9, 724814. doi:10.3389/fenvs.2021.724814
Naqvi, S., Naqvi, , Samim, M., Abdin M.Z, , Ahmad F.J, , prashant C.K, , et al. (2009). Concentration-dependent toxicity of iron oxide nanoparticles mediated by increased oxidative stress. Int. J. Nanomed. 5, 983–989. doi:10.2147/ijn.s13244
Naraginti, S., and Sivakumar, A. (2014). Eco-friendly synthesis of silver and gold nanoparticles with enhanced bactericidal activity and study of silver catalyzed reduction of 4-nitrophenol. Spectrochim. Acta A. Mol. Biomol. Spectrosc. 128, 357–362. doi:10.1016/j.saa.2014.02.083
Narasaiah, P., Mandal, B. K., and Sarada, N. (2017). Green synthesis of PdNPs from Pimpinella tirupatiensis plant extract and their application in photocatalytic activity dye degradation. Mater. Sci. Eng. Conf. Ser., 022013. doi:10.1088/1757-899X/263/2/022013
Naseem, K., Aziz, A., Tahir, M. H., Ameen, A., Ahmad, A., Ahmad, K., et al. (2024). Biogenic synthesized nanocatalysts and their potential for the treatment of toxic pollutants: environmental remediation, a review. Int. J. Environ. Sci. Technol. 21 (2), 2163–2194. doi:10.1007/s13762-023-05166-3
Nasrollahzadeh, M., Atarod, M., and Sajadi, S. M. (2016). Green synthesis of the Cu/Fe3O4 nanoparticles using Morinda morindoides leaf aqueous extract: a highly efficient magnetically separable catalyst for the reduction of organic dyes in aqueous medium at room temperature. Appl. Surf. Sci. 364, 636–644. doi:10.1016/j.apsusc.2015.12.209
Nasrollahzadeh, M., Issaabadi, Z., and Sajadi, S. M. (2018b). Green synthesis of Pd/Fe3O4 nanocomposite using Hibiscus tiliaceus L. Extract and its application for reductive catalysis of Cr (VI) and nitro compounds. Sep. Purif. Technol. 197, 253–260. doi:10.1016/j.seppur.2018.01.010
Nasrollahzadeh, M., Sajjadi, M., and Sajadi, S. M. (2018a). Biosynthesis of copper nanoparticles supported on manganese dioxide nanoparticles using Centella asiatica L. Leaf extract for the efficient catalytic reduction of organic dyes and nitroarenes. Chin. J. Catal. 39, 109–117. doi:10.1016/s1872-2067(17)62915-2
Natarajan, M., Mohan, S., Martinez, B. R., Meltz, M. L., and Herman, T. S. (2000). Antioxidant compounds interfere with the 3. Prev. 24 (5), 405–414.
Nel, A., Xia, T., and Mädler, L. (2006). Toxic potential of materials at the nanolevel. Science 311 (5761), 622–627. doi:10.1126/science.1114397
Nelson, B. (2019). “Challenges in standardising in vitro assays for the evaluation of nanoenabled drug products,” in Private communication at global summit on regulatory science 2019 on nanotechnology and nanoplastics. EUR 30195 EN.
Nemes, Z., Dietz, R., Lüth, J. B., Gomba, S., Hackenthal, E., and Gross, F. (1979). The pharmacological relevance of vital staining with neutral red. Experientia 35 (11), 1475–1476. doi:10.1007/bf01962793
Nemiwal, M., Zhang, T. C., and Kumar, D. (2024). Recent progress in g-C3N4, TiO2 and ZnO based photocatalysts for dye degradation: strategies to improve photocatalytic activity. Sci. Total Environ. 767, 144896. doi:10.1016/j.scitotenv.2020.144896
Nidheesh, P. V. (2015). Heterogeneous Fenton catalysts for the abatement of organic pollutants from aqueous solution: a review. RSC Adv. 5, 40552–40577. doi:10.1039/C5RA02023A
Njagi, E. C., Huang, H., Stafford, L., Genuino, H., Galindo, H. M., Collins, J. B., et al. (2010). Biosynthesis of iron and silver nanoparticles at room temperature using aqueous sorghum bran extracts. Langmuir 27, 264–271. doi:10.1021/la103190n
O'Carroll, D., Sleep, B., Krol, M., Boparai, H., and Kocur, C. (2013). Nanoscale zero valent iron and bimetallic particles for contaminated site remediation. Adv. Water Resour. 51, 104–122. doi:10.1016/j.advwatres.2012.02.005
O’Connor, J., Bolan, N. S., Kumar, M., Nitai, A. S., Ahmed, M. B., Bolan, S. S., et al. (2022). Distribution, transformation and remediation of poly- and per-fluoroalkyl substances (PFAS) in wastewater sources. Process Saf. Environ. Prot. 164, 91–108. doi:10.1016/j.psep.2022.06.002
OECD (2020a). Organisation for economic Co-operation and development. Available at: https://www.oecd.org/sti/emerging-tech/.
OECD (2020b). Organisation for economic Co-operation and development. Available at: www.oecd.org/chemicalsafety/nanosafety/publications-series-safety-manufactured-nanomaterials.htm.
Ojo, S. A., Lateef, A., Azeez, M. A., Oladejo, S. M., Akinwale, A. S., Asafa, T. B., et al. (2016). Biomedical and catalytic applications of gold and silver-gold alloy nanoparticles biosynthesized using cell-free extract of Bacillus SafensisLAU 13: antifungal, dye degradation, anti-coagulant and thrombolytic activities. IEEE Trans. Nanobioscience 15, 433–442. doi:10.1109/tnb.2016.2559161
Omran, B. A., and Baek, K. (2022). Valorization of agro-industrial biowaste to green nanomaterials for wastewater treatment: approaching green chemistry and circular economy principles. J. Environ. Manag. 311, 114806. doi:10.1016/j.jenvman.2022.114806
Oni, B. A., Sanni, S. E., Agu, K. C., and Tomomewo, O. S. (2023). Green synthesis of Ag nanoparticles from Argemone mexicana L. leaf extract coated with MOF-5 for the removal of metronidazole antibiotics from aqueous solution. J. Environ. Manage 342, 118161. doi:10.1016/j.jenvman.2023.118161
Osman, A. I., Zhang, Y., Farghali, M., Rashwan, A. K., Eltaweil, A. S., Abd El-Monaem, E. M., et al. (2024). Synthesis of green nanoparticles for energy, biomedical, environmental, agricultural, and food applications: a review. Environ. Chem. Lett. 22, 841–887. doi:10.1007/s10311-023-01682-3
Ouyang, Q., Kou, F., Tsang, P. E., Lian, J., Xian, J., Fang, J., et al. (2019). Green synthesis of Fe-based material using tea polyphenols and its application as a heterogeneous Fenton-like catalyst for the degradation of lincomycin. J. Clean. Prod. 232, 1492–1498. doi:10.1016/j.jclepro.2019.06.043
Pan, Y., Neuss, S., Leifert, A., Fischler, M., Wen, F., Simon, U., et al. (2007). Size-dependent cytotoxicity of gold nanoparticles. Small 3 (11), 1941–1949. doi:10.1002/smll.200700378
Parandhaman, T., Dey, M. D., and Das, S. K. (2019). Biofabrication of supported metal nanoparticles: exploring the bioinspiration strategy to mitigate the environmental challenges. Green Chem. 21, 5469–5500. doi:10.1039/c9gc02291k
Parandhaman, T., Pentela, N., Ramalingam, B., Samanta, D., and Das, S. K. (2016). Metal nanoparticle loaded magnetic-chitosan microsphere: water dispersible and easily separable hybrid metal nano-biomaterial for catalytic applications. ACS Sustain. Chem. Eng. 5, 489–501. doi:10.1021/acssuschemeng.6b01862
Park, Y.-H., Kim, J. N., Jeong, S. H., Choi, J. E., Lee, S. H., Choi, B. H., et al. (2010). Assessment of dermal toxicity of nanosilica using cultured keratinocytes, a human skin equivalent model and an invivo model. Toxicology 267 (1), 178–181. doi:10.1016/j.tox.2009.10.011
Pasquini, L. M., Hashmi, S. M., Sommer, T. J., Elimelech, M., and Zimmerman, J. B. (2012). Impact of surface functionalization on bacterial cytotoxicity of single-walled carbon nanotubes. Environ. Sci. Technol. 46 (11), 6297–6305. doi:10.1021/es300514s
Patil, S. S., Mali, M. G., Tamboli, M. S., Patil, D. R., Kulkarni, M. V., Yoon, H., et al. (2016). Green approach for hierarchical nanostructured Ag-ZnO and their photocatalytic performance under sunlight. Catal. Today 260, 126–134. doi:10.1016/j.cattod.2015.06.004
Patlolla, A., Patlolla, B., and Tchounwou, P. (2010). Evaluation of cell viability, DNA damage, and cell death in normal human dermal fibroblast cells induced by functionalized multiwalled carbon nanotube. Mol. Cell. Biochem. 338 (1–2), 225–232. doi:10.1007/s11010-009-0356-2
Patra, J. K., Das, G., Fraceto, L. F., Campos, E. V. R., Rodriguez-Torres, M. d. P., Acosta-Torres, L. S., et al. (2018). Nano based drug delivery systems: recent developments and future prospects. J. Nanobiotechnol. 16, 71. doi:10.1186/s12951-018-0392-8
Paul, B., Bhuyan, B., Purkayastha, D. D., and Dhar, S. S. (2016). Photocatalytic and antibacterial activities of gold and silver nanoparticles synthesized using biomass of Parkia roxburghii leaf. J. Photochem. Photobiol. B, Biol. 154, 1–7. doi:10.1016/j.jphotobiol.2015.11.004
Paul, B., Vadivel, S., Dhar, S. S., Debbarma, S., and Kumaravel, M. (2017). One-pot green synthesis of zinc oxide nano rice and its application as sonocatalyst for degradation of organic dye and synthesis of 2-benzimidazole derivatives. J. Phys. Chem. Solids 104, 152–159. doi:10.1016/j.jpcs.2017.01.007
Pavanello, S., and Clonfero, E. (2000). Biological indicators of genotoxic risk and metabolic polymorphisms. Mutat. Res. Rev. Mutat. Res. 463 (3), 285–308. doi:10.1016/s1383-5742(00)00051-x
Peng, X., Bai, X., Cui, Z., and Liu, X. (2019). Green synthesis of Pd truncated octahedrons using of firmiana simplex leaf extract and their catalytic study for electro-oxidation of methanol and reduction of p-nitrophenol. Appl. Organomet. Chem. e5045. doi:10.1002/aoc.5045
Perez de la Ossa, D. H., and Bremer-Hoffmann, S. (2019). “Nanomedicines: EU regulatory experience, challenges and regulatory research work,” in Private communication at global Summit on regulatory science 2019 on nanotechnology and nanoplastics. EUR 30195 EN.
Philipse, A. P., and Maas, D. (2002). Magnetic colloids from magnetotactic bacteria: chain formation and colloidal stability. Langmuir 18, 9977–9984. doi:10.1021/la0205811
Pietroiusti, A., Stockmann-Juvala, H., Lucaroni, F., and Savolainen, K. (2018). Nanomaterial exposure, toxicity, and impact on human health. Wires Nanomed Nanobiotechnol 10 (5), e1513. doi:10.1002/wnan.1513
Pires, C. A., Santos, A. C. C. D., and Jordão, E. (2015). Oxidation of phenol in aqueous solution with copper oxide catalysts supported on γ-Al2O3, pillared clay and TiO2: comparison of the performance and costs associated with each catalyst. Braz. J. Chem. Eng. 32, 837–848. doi:10.1590/0104-6632.20150324s00002232
Pirkarami, A., Olya, M. E., and Raeis Farshid, S. (2014). UV/Ni-TiO2 nanocatalyst for electrochemical removal of dyes considering operating costs. Water Resour. Ind. 5, 9–20. doi:10.1016/j.wri.2014.02.001
Plachtová, P., Medříková, Z., Zbořil, R., Tuček, J., Varma, R. S., and Maršálek, B. (2018). Iron and iron oxide nanoparticles synthesized with green tea extract: differences in ecotoxicological profile and ability to degrade malachite green. ACS Sustain. Chem. Eng. 6, 8679–8687. doi:10.1021/acssuschemeng.8b00986
Poguberović, S. S., Krčmar, D. M., Maletić, S. P., Kónya, Z., Pilipović, D. D. T., Kerkez, D. V., et al. (2016). Removal of as (III) and Cr (VI) from aqueous solutions using “green” zero-valent iron nanoparticles produced by oak, mulberry and cherry leaf extracts. Ecol. Eng. 90, 42–49. doi:10.1016/j.ecoleng.2016.01.083
Pradhan, N., Pal, A., and Pal, T. (2001). Catalytic reduction of aromatic nitro compounds by coinage metal nanoparticles. Langmuir 17, 1800–1802. doi:10.1021/la000862d
Prakash, L. V., Gopinath, A., Gandhimathi, R., Velmathi, S., Ramesh, S. T., and Nidheesh, P. V. (2021). Ultrasound aided heterogeneous Fenton degradation of acid blue 15 over green synthesized magnetite nanoparticles. Sep. Purif. Technol. 266, 118230. doi:10.1016/j.seppur.2020.118230
Prasad, A. R., Garvasis, J., Oruvil, S. K., and Joseph, A. (2019). Bio-inspired green synthesis of zinc oxide nanoparticles using Abelmoschus esculentus mucilage and selective degradation of cationic dye pollutants. J. Phys. Chem. Solids 127, 265–274. doi:10.1016/j.jpcs.2019.01.003
Prasad, K. S., Gandhi, P., and Selvaraj, K. (2014). Synthesis of green nano iron particles (GnIP) and their application in adsorptive removal of as (III) and as (V) from aqueous solution. Appl. Surf. Sci. 317, 1052–1059. doi:10.1016/j.apsusc.2014.09.042
Puiatti, G. A., de Carvalho, J. P., de Matos, A. T., and Lopes, R. P. (2022). Green synthesis of Fe0 nanoparticles using Eucalyptus grandis leaf extract: characterization and application for dye degradation by a (Photo)Fenton-like process. J. Environ. Manag. 311, 114828. doi:10.1016/j.jenvman.2022.114828
Punshon, G., Vara, D., Sales, K., Kidane, A., Salacinski, H., and Seifalian, A. (2005). Interactions between endothelial cells and a poly(carbonate-silsesquioxane-bridge-urea)urethane. Biomaterials 26 (32), 6271–6279. doi:10.1016/j.biomaterials.2005.03.034
Qasim, M., Arif, M. I., Naseer, A., Ali, L., Aslam, R., Abbasi, S. A., et al. (2024). Biogenic nanoparticles at the forefront: transforming industrial wastewater treatment with TiO2 and graphene. Sch. J. Agric. Vet. Sci. 5, 56–76. doi:10.36347/sjavs.2024.v11i05.002
Qian, X., Peng, X. H., Ansari, D. O., Yin-Goen, Q., Chen, G. Z., Shin, D. M., et al. (2008). In vivo tumor targeting and spectroscopic detection with surface-enhanced Raman nanoparticle tags. Nat. Biotechnol. 26 (1), 83–90. doi:10.1038/nbt1377
Qu, Y., Pei, X., Shen, W., Zhang, X., Wang, J., Zhang, Z., et al. (2017). Biosynthesis of gold nanoparticles by Aspergillum sp. WL-Au for degradation of aromatic pollutants. Phys. E Low. Syst. Nanostruct. 88, 133–141. doi:10.1016/j.physe.2017.01.010
Quan, Z., Zhang, X., and Xu, H. (2015). In-situ formation and immobilization of biogenic nanopalladium into anaerobic granular sludge enhances azo dyes degradation. Water Res. 78, 74–83. doi:10.1016/j.watres.2015.03.024
Radziun, E., Dudkiewicz Wilczyńska, J., Książek, I., Nowak, K., Anuszewska, E., Kunicki, A., et al. (2011). Assessment of the cytotoxicity of aluminium oxide nanoparticles on selected mammalian cells. Toxicol. Vitro 25 (8), 1694–1700. doi:10.1016/j.tiv.2011.07.010
Raj, R., Dalei, K., Chakraborty, J., and Das, S. (2016). Extracellular polymeric substances of a marine bacterium mediated synthesis of CdS nanoparticles for removal of cadmium from aqueous solution. J. Colloid Interface Sci. 462, 166–175. doi:10.1016/j.jcis.2015.10.004
Rajendaran, K., Muthuramalingam, R., and Ayyadurai, S. (2019). Green synthesis of Ag-Mo/CuO nanoparticles using Azadirachta indica leaf extracts to study its solar photocatalytic and antimicrobial activities. Mater. Sci. Semicond. process. 91, 230–238. doi:10.1016/j.mssp.2018.11.021
Rambabu, K., Bharath, G., Banat, F., and Show, P. L. (2021). Green synthesis of zinc oxide nanoparticles using Phoenix dactylifera waste as bioreductant for effective dye degradation and antibacterial performance in wastewater treatment. J. Hazard Mater. 402, 123560–123572. doi:10.1016/j.jhazmat.2020.123560
Ramesh, A., Devi, D. R., Battu, G., and Basavaiah, K. (2018). A Facile plant mediated synthesis of silver nanoparticles using an aqueous leaf extract of Ficus hispida Linn. F. For catalytic, antioxidant and antibacterial applications. South Afr. J. Chem. Eng. 26, 25–34. doi:10.1016/j.sajce.2018.07.001
Ranjith, R., Renganathan, V., Chen, S.-M., Selvan, N. S., and Rajam, P. S. (2019). Green synthesis of reduced graphene oxide supported TiO2/Co3O4 nanocomposite for photocatalytic degradation of methylene blue and crystal violet. Ceram. Int. 45, 12926–12933. doi:10.1016/j.ceramint.2019.03.219
Rao, A., Bankar, A., Kumar, A. R., Gosavi, S., and Zinjarde, S. (2013). Removal of hexavalent chromium ions by Yarrowia lipolytica cells modified with phyto-inspired Fe0/Fe3O4 nanoparticles. J. Contam. Hydrol. 146, 63–73. doi:10.1016/j.jconhyd.2012.12.008
Rathore, C., Yadav, V. K., Gacem, A., AbdelRahim, S. K., Verma, R. K., Chundawat, R. J., et al. (2023). Microbial synthesis of titanium dioxide nanoparticles and their importance in wastewater treatment and antimicrobial activities: a review. Front. Microbiol. 14, 1270245. doi:10.3389/fmicb.2023.1270245
Ravichandran, P., Periyakaruppan, A., Sadanandan, B., Ramesh, V., Hall, J. C., Jejelowo, O., et al. (2013). Induction of apoptosis in rat lung epithelial cells by multiwalled carbon nanotubes. J. Biochem. Mol. Toxicol. 27 (5), 286. doi:10.1002/jbt.21479
Ravikumar, K. V. G., Kubendiran, H., Ramesh, K., Rani, S., Mandal, T. K., Pulimi, M., et al. (2020). Batch and column study on tetracycline removal using green synthesized NiFe nanoparticles immobilized alginate beads. Environ. Technol. Innov. 17, 100520. doi:10.1016/j.eti.2019.100520
Ravikumar, K. V. G., Sudakaran, S. V., Ravichandran, K., Pulimi, M., Natarajan, C., and Mukherjee, A. (2019). Green synthesis of NiFe nano particles using Punica granatum peel extract for tetracycline removal. J. Clean. Prod. 210, 767–776. doi:10.1016/j.jclepro.2018.11.108
Reddy, A. R. N., Reddy, Y. N., Krishna, D. R., and Himabindu, V. (2010). Multi wall carbon nanotubes induce oxidative stress and cytotoxicity in human embryonic kidney (HEK293) cells. Toxicology 272 (1), 11–16. doi:10.1016/j.tox.2010.03.017
Reddy, J., Mata, R., Raja, K., Khub, V., and Rani, S. (2018). Green synthesized silver nanoparticles: catalytic dye degradation, in vitro anticancer activity and in vivo toxicity in rats. Mater. Sci. Eng. C 91, 372–381. doi:10.1016/j.msec.2018.05.048
Regoli, F., and Giuliani, M. E. (2014). Oxidative pathways of chemical toxicity and oxidative stress biomarkers in marine organisms. Mar. Environ. Res. 93, 106–117. doi:10.1016/j.marenvres.2013.07.006
Ren, J., Liang, H., and Chan, F. T. S. (2017). Urban sewage sludge, sustainability, and transition for Eco-City: multi-criteria sustainability assessment of technologies based on bestworst method. Technol. Forecast. Soc. Chang. 116, 29–39. doi:10.1016/j.techfore.2016.10.070
Ren, J., Xu, D., Cao, H., Wei, S., Dong, L., and Goodsite, M. E. (2016). Sustainability decision support framework for industrial system prioritization. Process Syst. Eng. 62, 108–130. doi:10.1002/aic.15039
Renuka, L., Anantharaju, K., Sharma, S., Nagaswarupa, H., Prashantha, S., Nagabhushana, H., et al. (2016). Hollow microspheres Mg-doped ZrO2 nanoparticles: green assisted synthesis and applications in photocatalysis and photoluminescence. J. Alloys. Compd. 672, 609–622. doi:10.1016/j.jallcom.2016.02.124
Rizzo, L., Meric, S., Guida, M., Kassinos, D., and Belgiorno, V. (2009). Heterogenous photocatalytic degradation kinetics and detoxification of an urban wastewater treatment plant effluent contaminated with pharmaceuticals. Water Res. 43, 4070–4078. doi:10.1016/j.watres.2009.06.046
Robati, D., Mirza, B., Rajabi, M., Moradi, O., Tyagi, I., Agarwal, S., et al. (2016). Removal of hazardous dyes-BR 12 and methyl orange using graphene oxide as an adsorbent from aqueous phase. Chem. Eng. J. 284, 687–697. doi:10.1016/j.cej.2015.08.131
Robertson, T. A., Sanchez, W. Y., and Roberts, M. S. (2010). Are commercially available nanoparticles safe when applied to the skin? J. Biomed. Nanotechnol. 6 (5), 452–468. doi:10.1166/jbn.2010.1145
Rong, K., Wang, J., Zhang, Z., and Zhang, J. (2020). Green synthesis of iron nanoparticles using Korla fragrant pear peel extracts for the removal of aqueous Cr(VI). Ecological Engineering 149, 105793–111268. doi:10.1016/j.ecoleng.105793
Ronteltap, A., Fischer, A. R. H., and Tobi, H. (2011). Societal response to nanotechnology: converging technologies-converging societal response research? J. Nanopart. Res. 13, 4399–4410. doi:10.1007/s11051-011-0473-1
Rosbero, T. M. S., and Camacho, D. H. (2017). Green preparation and characterization of tentacle-like silver/copper nanoparticles for catalytic degradation of toxic chlorpyrifos in water. J. Environ. Chem. Eng. 5, 2524–2532. doi:10.1016/j.jece.2017.05.009
Roy, N., Mondal, S., Laskar, R. A., Basu, S., Mandal, D., and Begum, N. A. (2010). Biogenic synthesis of Au and Ag nanoparticles by Indian propolis and its constituents. Colloids Surf. B 76, 317–325. doi:10.1016/j.colsurfb.2009.11.011
Rupa, E. J., Anandapadmanaban, G., Mathiyalagan, R., and Yang, D. C. (2018). Synthesis of zinc oxide nanoparticles from immature fruits of Rubus coreanus and its catalytic activity for degradation of industrial dye. Optik 172, 1179–1186. doi:10.1016/j.ijleo.2018.07.115
Ryman-Rasmussen, J. P., Riviere, J. E., and Monteiro-Riviere, N. A. (2007). Surface coatings determine cytotoxicity and irritation potential of quantum dot nanoparticles in epidermal keratinocytes. J. Investig. Dermatol. 127 (1), 143–153. doi:10.1038/sj.jid.5700508
Ryter, S. W., Kim, H. P., Hoetzel, A., Park, J. W., Nakahira, K., Wang, X., et al. (2007). Mechanisms of cell death in oxidative stress. Antioxid. Redox Signal. 9 (1), 49–89. doi:10.1089/ars.2007.9.49
Saad, M., Tahir, H., and Ali, D. (2017). Green synthesis of Ag-Cr-AC nanocomposites by Azadirachta indica and its application for the simultaneous removal of binary mixture of dyes by ultrasonicated assisted adsorption process using Response Surface Methodology. Ultrason. Sonochem. 38, 197–213. doi:10.1016/j.ultsonch.2017.03.022
Sabzevari, M., Cree, D. E., and Wilson, L. D. (2018). Graphene oxide–chitosan composite material for treatment of a model dye effluent. ACS Omega 3 (10), 13045–13054. doi:10.1021/acsomega.8b01871
Sah, P. M., Gite, S. G., Sonawane, R., and Raut, R. W. (2024). “Biogenic nanomaterials as a catalyst for photocatalytic dye degradation,” in Biogenic nanomaterials for environmental sustainability: principles, practices, and opportunities (Cham: Springer International Publishing).
Sajadi, S. M., Kolo, K., Hamad, S. M., Mahmud, S. A., Barzinjy, A. A., and Hussein, S. M. (2018). Green synthesis of the Ag/bentonite nanocomposite using Euphorbia larica extract: a reusable catalyst for efficient reduction of nitro compounds and organic dyes. ChemistrySelect 3, 12274–12280. doi:10.1002/slct.201802707
Sajjadi, M., Ahmadpoor, F., Nasrollahzadeh, M., and Ghafuri, H. (2021). Lignin-derived (nano) materials for environmental pollution remediation: current challenges and future perspectives. Int. J. Biol. Macromol. 178, 394–423. doi:10.1016/j.ijbiomac.2021.02.165
Salehi, H., Yousefi, M., Hekmati, M., and Balali, E. (2019). Application of palladium nanoparticle-decorated Artemisia abrotanum extract-modified graphene oxide for highly active catalytic reduction of methylene blue, methyl orange and rhodamine B. Appl. Organomet. Chem. 33, e5123. doi:10.1002/aoc.5123
Salem, S. S., and Fouda, A. (2021). Green synthesis of metallic nanoparticles and their prospective biotechnological applications: an overview. Biol. trace Elem. Res. 199 (1), 344–370. doi:10.1007/s12011-020-02138-3
Sanni, E. S., Mshelia, H., Okoro, E. E., Alaba, P. A., Oni, B. A., and Ayoola, A. (2022b). Estimation of critical fluxes, thermal stabilities and failure criteria of cellulose-based membranes and modelling of salt diffusivity during pervaporative desalination. Nanotechnol. Environ. Eng. 7, 857–881. doi:10.1007/s41204-022-00221-9
Sanni, S. E., Agboola, O., Sadiku, R., and Emetere, M. E. (2021). “Nature of graphene, its chemical structure, synthesis, properties and applications,” in Handbook of graphenes: chemistry, physics and biology. Editor T. Stauber (Wiley-Scrivener) 2.
Sanni, S. E., Favour, I., and Adeyanju, A. (2022c). “Nanovesicles for the delivery of siRNA,” in Applications of nanovesicular drug delivery. Editors A. K. Nayak, M. S. Hasnain, T. M. Aminabhavi, and V. P. Torchilin (Elsevier) 24, 457–466. doi:10.1016/B978-0-323-91865-7.00010-9
Sanni, S. E., Olowoyeye, P., Okoro, E. E., Oni, B. A., Idowu, T. I., and Adegbite, O. (2022a). Heterogeneous catalytic conversion of 4-chlorophenol via atomic hydrogen substitution induced by size-controlled polydisperse nanocobalt. Chem. Eng. Sci. 247, 117018. doi:10.1016/j.ces.2021.117018
Sanni, S. E., Sadiku, E. R., and Okoro, E. E. (2023a). “Graphene and graphene oxide-based nanocomposites for theranostic applications,” in Inorganic nanosystems: theranostic nanosystems. Editors M. S. Hasnain, A. K. Naya, and T. M. Aminabhavi Ch. 4, 2, 103–135. doi:10.1016/B978-0-323-85784-0.00011-X
Sanni, S. E., Sadiku, E. R., Okoro, E. E., Oni, B. A., and Ndubuisi, A. O. (2023b). “Biocatalytic sensors: potentials, maxims and mechanisms for optimal performance,” in Biomaterials-based sensors. Editors P. Kumar, S. K. Dash, S. Ray, and S. Parween (Singapore: Springer). doi:10.1007/978-981-19-8501-0_6
Sastry, M., Ahmad, A., Khan, M. I., and Kumar, R. (2003). Biosynthesis of metal nanoparticles using fungi and actinomycete. Curr. Sci. 85, 162–170.
Sathian, S., Radha, G., Shanmugapriya, V., Rajasimman, M., and Karthikeyan, C. (2013). Optimization and kinetic studies on treatment of textile dye wastewater using Pleurotus floridanus. Appl. Water Sci. 3, 41–48. doi:10.1007/s13201-012-0055-0
Sathyanarayana, S., and Hübner, C. (2013). “Thermoplastic nanocomposites with carbon nanotubes,” in Structural nanocomposites. Engineering materials. Editor J. Njuguna (Berlin: Springer), 19–60.
Saud, A., Gupta, S., Allal, A., Preud’Homme, H., Shomar, B., and Zaidi, S. J. (2024). Progress in the sustainable development of biobased (nano) materials for application in water treatment technologies. ACS omega 9 (27), 29088–29113. doi:10.1021/acsomega.3c08883
Saxena, G., and Bharagava, R. N. (2020). “Bioremediation of industrial waste for environmental safety (volume i: industrial waste and its management),” in Green synthesis of nanoparticles and their applications in water and wastewater treatment, 349–379. doi:10.1007/978-981-13-1891-7_16
Sayes, C. M., Reed, K. L., and Warheit, D. B. (2007). Assessing toxicity of fine and nanoparticles: comparing in vitro measurements to in vivo pulmonary toxicity profiles. Toxicol. Sci. 97 (1), 163–180. doi:10.1093/toxsci/kfm018
Schiavo, S., Oliviero, M., Miglietta, M., Rametta, G., and Manzo, S. (2016). Genotoxic and cytotoxic efects of ZnO nanoparticles for Dunaliella tertiolecta and comparison with SiO2 and TiO2 efects at population growth inhibition levels. Sci. Total Environ. 550, 619–627. doi:10.1016/j.scitotenv.2016.01.135
Schmidt, J., and Vogelsberger, W. (2009). Aqueous long-term solubility of titania nanoparticles and titanium(IV) hydrolysis in a sodium chloride system studied by adsorptive stripping voltammetry. J. Solut. Chem. 38, 1267–1282. doi:10.1007/s10953-009-9445-9
Sebastian, A., Nangia, A., and Prasad, M. N. V. (2018). A green synthetic route to phenolics fabricated magnetite nanoparticles from coconut husk extract: implications to treat metal contaminated water and heavy metal stress in Oryza sativa L. J. Clean. Prod. 174, 355–366. doi:10.1016/j.jclepro.2017.10.343
Sengani, M., Grumezescu, A. M., and Rajeswari, V. D. (2017). Recent trends and methodologies in gold nanoparticle synthesis–A prospective review on drug delivery aspect. OpenNano 2, 37–46. doi:10.1016/j.onano.2017.07.001
Shafey, A. M. E. (2020). Green synthesis of metal and metal oxide nanoparticles from plant leaf extracts and their applications: a review. Green process. Synth. 9, 304–339. doi:10.1515/gps-2020-0031
Shahwan, T., Sirriah, S. A., Nairat, M., Boyacı, E., Eroglu, A. E., Scottc, T. B., et al. (2011). Green synthesis of iron nanoparticles and their application as a Fenton-like catalyst for the degradation of aqueous cationic and anionic dyes. Chem. Eng. J. 172, 258–266. doi:10.1016/j.cej.2011.05.103
Sharmila, G., Thirumarimurugan, M., and Sivakumar, V. M. (2016). Optical, catalytic and antibacterial properties of phytofabricated CuO nanoparticles using Tecoma castanifolia leaf extract. Opt. - Int. J. Light Elect. Opt. 127, 7822–7828. doi:10.1016/j.ijleo.2016.05.142
Shen, W., Qu, Y., Pei, X., Li, S., You, S., Wang, J., et al. (2017). Catalytic reduction of 4-nitrophenol using gold nanoparticles biosynthesized by cell-free extracts of Aspergillus sp. WL-Au. J. Hazard. mater. 321, 299–306. doi:10.1016/j.jhazmat.2016.07.051
Sherin, L., Sohail, A., Amjad, U. e.S., Mustafa, M., Jabeen, R., and Ul-Hamid, A. (2020). Facile green synthesis of silver nanoparticles using Terminalia bellerica kernel extract for catalytic reduction of anthropogenic water pollutants. Colloid. Interface Sci. Commun. 37, 100276–100287. doi:10.1016/j.colcom.2020.100276
Sherr, C. J. (2004). Principles of tumor suppression. Cell 116 (2), 235–246. doi:10.1016/s0092-8674(03)01075-4
Shivaji, K., Monica, E. S., Devadoss, A., Kirubakaran, D. D., Dhas, C. R., Jain, S. M., et al. (2020). Synthesizing Green photocatalyst using plant leaf extract for Water pollutant treatment. Environ. Chem. a Sustain. World, 25–46. doi:10.1007/978-3-030-15608-4_2
Shukla, A. K. (2015). Electron magnetic resonance (EMR) technique and nanoparticle characterization,” in Nanoparticle’s promises and risks-characterization, manipulation and potential hazards to humanity and the environment. Editors M. Lungu, A. Neculae, M. Bunoiu, and C. Biris (Switzerland: Springer), 235–244.
Shukla, A. K., and Iravani, S. (2017). Metallic nanoparticles: green synthesis and spectroscopic characterization. Environ. Chem. Lett. 15, 223–231. doi:10.1007/s10311-017-0618-2
Silva, M. T. (2010). Secondary necrosis: the natural outcome of the complete apoptotic program. FEBS Lett. 584 (22), 4491–4499. doi:10.1016/j.febslet.2010.10.046
Simeonidis, K., Kaprara, E., Samaras, T., Angelakeris, M., Pliatsikas, N., Vourlias, G., et al. (2015). Optimizing magnetic nanoparticles for drinking water technology: the case of Cr(VI). Sci. Total Environ. 535, 61–68. doi:10.1016/j.scitotenv.2015.04.033
Singh, K., Kukkar, D., Singh, R., Kukkar, P., and Kim, K. H. (2018). Exceptionally stable green-synthesized gold nanoparticles for highly sensitive and selective colorimetric detection of trace metal ions and volatile aromatic compounds. J. Ind. Eng. Chem. 68, 33–41. doi:10.1016/j.jiec.2018.07.026
Singh, N., and Stephens, R. (1997). Microgel electrophoresis: sensitivity, mechanisms, and DNA electrostretching. Mutat. Res. DNA Repair 383 (2), 167–175. doi:10.1016/s0921-8777(96)00056-0
Sinha, A. K., Basu, M., Sarkar, S., Pradhan, M., and Pal, T. (2013). Synthesis of gold nanochains via photoactivation technique and their catalytic applications. J. Colloid Interface Sci. 398, 13–21. doi:10.1016/j.jcis.2013.01.061
Sinha, T., and Ahmaruzzaman, M. (2015). Biogenic synthesis of Cu nanoparticles and its degradationbehavior for methyl red. Mater. Lett. 159, 168–171. doi:10.1016/j.matlet.2015.06.099
Sinha, T., Ahmaruzzaman, M., Adhikari, P. P., and Bora, R. (2017). Green and environmentally sustainable fabrication of Ag-SnO2 nanocomposite and its multifunctional efficacy as photocatalyst and antibacterial and antioxidant agent. ACS Sustain. Chem. Eng. 5, 4645–4655. doi:10.1021/acssuschemeng.6b03114
Soenen, S. J., Rivera-Gil, P., Montenegro, J. M., Parak, W. J., De Smedt, S. C., and Braeckmans, K. (2011). Cellular toxicity of inorganic nanoparticles: common aspects and guidelines for improved nanotoxicity evaluation. Nano Today 6 (5), 446–465. doi:10.1016/j.nantod.2011.08.001
Sohn, E. K., Chung, Y. S., Johari, S. A., Kim, T. G., Kim, J. K., Lee, J. H., et al. (2015). Acute toxicity comparison of single-walled carbon nanotubes in various freshwater organisms. Biomed. Res. Int. 2015, 1–7. doi:10.1155/2015/323090
Sohrabnezhad, S., and Seifi, A. (2016). The green synthesis of Ag/ZnO in montmorillonite with enhanced photocatalytic activity. Appl. Surf. Sci. 386, 33–40. doi:10.1016/j.apsusc.2016.05.102
Solano, R. A., Herrera, A. P., Maestre, D., and Cremades, A. (2019). Fe-TiO2 nanoparticles synthesized by green chemistry for potential application in waste water photocatalytic treatment. J. Nanotechnol. 2019, 1–11. doi:10.1155/2019/4571848
Soltys, L., Olkhovyy, O., Tatarchuk, T., and Naushad, M. n. (2021). Green synthesis of metal and metal oxide nanoparticles: principles of green chemistry and raw materials. Magnetochemistry 7 (11), 145. doi:10.3390/magnetochemistry7110145
Sreeju, N., Rufus, A., and Philip, D. (2017). Studies on catalytic degradation of organic pollutants and anti-bacterial property using biosynthesized CuO nanostructures. J. Mol. Liq. 242, 690–700. doi:10.1016/j.molliq.2017.07.077
Sridharan, S., Kumar, M., Bolan, N. S., Singh, L., Kumar, S., Kumar, R., et al. (2021). Are microplastics destabilizing the global network of terrestrial and aquatic ecosystem services? Environ. Res. 198, 111243. doi:10.1016/j.envres.2021.111243
Srivastava, N., and Mukhopadhyay, M. (2014). Biosynthesis of SnO2 nanoparticles using bacterium Erwinia herbicola and their photocatalytic activity for degradation of dyes. Ind. Eng. Chem. Res. 53, 13971–13979. doi:10.1021/ie5020052
Srivastava, V., Sharma, Y., and Sillanpää, M. (2015). Green synthesis of magnesium oxide nanoflower and its application for the removal of divalent metallic species from synthetic wastewater. Ceram. Int. 41, 6702–6709. doi:10.1016/j.ceramint.2015.01.112
Stan, M., Lung, I., Soran, M.-L., Leostean, C., Popa, A., Stefan, M., et al. (2017). Removal of antibiotics from aqueous solutions by green synthesized magnetite nanoparticles with selected agro-waste extracts. Saf. Environ. Prot. 107, 357–372. doi:10.1016/j.psep.2017.03.003
Steiger, B. G. K., Udoetok, I. A., Faye, O., and Wilson, L. D. (2021). Counterion effects in metal hybrid biopolymer materials for sulfate adsorption: an experimental and computational study. acsapm.1c00706 ACS Appl. Polym. Mater. 3, 4595–4606. doi:10.1021/acsapm.1c00706
Steiger, B. G. K., Zhou, Z., Anisimov, Y. A., Evitts, R. W., and Wilson, L. D. (2023). Valorization of agro-waste biomass as composite adsorbents for sustainable wastewater treatment. Industrial Crops and Prod. 191, 115913. doi:10.1016/j.indcrop.2022.115913
Strober, W. (2001). “Trypan blue exclusion test of cell viability,” in Current protocols in immunology (John Wiley and Sons. Unites States), A3. B.1–A3. B.3.
Su, Y., He, Y., Lu, H., Sai, L., Li, Q., Li, W., et al. (2009). The cytotoxicity of cadmium based, aqueous phasesynthesized, quantum dots and its modulation by surface coating. Biomaterials 30 (1), 19–25. doi:10.1016/j.biomaterials.2008.09.029
Suárez-Cerda, J., Alonso-Nuñez, G., Espinoza-Gómez, H., and Flores-López, L. Z. (2015). Synthesis, kinetics and photocatalytic study of “ultra-small” Ag-NPs obtained by a green chemistry method using an extract of Rosa ‘andeli’double delight petals. J. Colloid Interface Sci. 458, 169–177. doi:10.1016/j.jcis.2015.07.049
Sudha, D., and Sivakumar, P. (2015). Review on the photocatalytic activity of various composite catalysts. Chem. Eng. Process. Process Intensif. 97, 112–133. doi:10.1016/j.cep.2015.08.006
Sudhaparimala, S., and Vaishnavi, M. (2016). Biological synthesis of nano composite SnO2- ZnO–screening for efficient photocatalytic degradation and antimicrobial activity. Mater. Today Proc. 3, 2373–2380. doi:10.1016/j.matpr.2016.04.150
Suja, E., Nanacharaiah, Y. V., and Venugopalan, V. P. (2014). Biogenic nanopalladium Production by self-immobilized Granular biomass: application for contaminant remediation. Water Res. 65, 395–401. doi:10.1016/j.watres.2014.08.005
Suman, S., Pandey, A., and Chandna, S. (2012). An improved non-enzymatic “DNA ladder assay” for more sensitive and early detection of apoptosis. Cytotechnology 64 (1), 9–14. doi:10.1007/s10616-011-9395-0
Sumrein, A. (2019). “Safety assessment of nanomaterials under REACH,” in Private communication at global Summit on regulatory science 2019 on nanotechnology and nanoplastics. EUR 30195 EN.
Sun, Y., Kumar, M., Wang, L., Gupta, J., Tsang, D. C. W., et al. (2020). “Biotechnology for soil decontamination: opportunity, challenges, and prospects for pesticide biodegradation,” in Bio-based materials and biotechnologies for ecoefficient construction. Editor F. Pacheco-Torgal (Sawston, Cambridge: Woodhead Publishing), 261–283.
Sureshkumar, M., Siswanto, D. Y., and Lee, C.-K. (2010). Magnetic antimicrobial nanocomposite based on bacterial cellulose and silver nanoparticles. J. Mater. Chem. 20, 6948–6955. doi:10.1039/c0jm00565g
Sweeney, R. Y., Mao, C., Gao, X., Burt, J. L., Belcher, A. M., Georgiou, G., et al. (2004). Bacterial biosynthesis of cadmium sulfide nanocrystals. Chem. Biol. 11, 1553–1559. doi:10.1016/j.chembiol.2004.08.022
Sze, O., Sum, N., Feng, J., Hu, X., and Lock, P. (2005). Photo-assisted Fenton mineralization of an azo-dye acid black 1 using a modified laponite clay-based Fe nanocomposite as a heterogeneous catalyst. Top. Catal. 33, 233–242. doi:10.1007/s11244-005-2532-2
Tajbakhsh, M., Alinezhad, H., Nasrollahzadeh, M., and Kamali, T. A. (2016). Green synthesis of the Ag/HZSM-5 nanocomposite by using Euphorbia heterophylla leaf extract: a recoverable catalyst for reduction of organic dyes. J. Alloys. Compd. 685, 258–265. doi:10.1016/j.jallcom.2016.05.278
Taleb, F., Ammar, M., Mosbah, M. B., Salem, R. B., and Moussaoui, Y. (2020). Chemical modification of lignin derived from spent coffee grounds for methylene blue adsorption. Sci. Rep. 10 (1), 11048. doi:10.1038/s41598-020-68047-6
Tamuly, C., Hazarika, M., Bordoloi, M., Bhattacharyya, P. K., and Kar, R. (2014). Biosynthesis of Ag nanoparticles using pedicellamide and its photocatalytic activity: an ecofriendly approach. Spectrochim. Acta A. Mol. Biomol. Spectrosc. 132, 687–691. doi:10.1016/j.saa.2014.05.024
Tang, M., Xing, T., Zeng, J., Wang, H., Li, C., Yin, S., et al. (2008). Unmodified CdSe quantum dots induce elevation of cytoplasmic calcium levels and impairment of functional properties of sodium channels in rat primary cultured hippocampal neurons. Environ. Health Perspect. 116 (7), 915–922. doi:10.1289/ehp.11225
Tarantola, M., Schneider, D., Sunnick, E., Adam, H., Pierrat, S., Rosman, C., et al. (2008). Cytotoxicity of metal and semiconductor nanoparticles indicated by cellular micromotility. ACS Nano 3 (1), 213–222. doi:10.1021/nn800721j
Tavares, L., Esparza Flores, E. E., Rodrigues, R. C., Hertz, P. F., and Nore˜na, C. P. Z. (2020). Effect of deacetylation degree of chitosan on rheological properties and physical chemical characteristics of genipin-crosslinked chitosan beads. Food Hydrocoll. 106, 105876. doi:10.1016/j.foodhyd.2020.105876
Thapa, R., Bhagat, C., Shrestha, P., Awal, S., and Dudhagara, P. (2017). Enzyme-mediated formulation of stable elliptical silver nanoparticles tested against clinical pathogens and MDR bacteria and development of antimicrobial surgical thread. Ann. Clin. Microbiol. Antimicrob. 16, 39. doi:10.1186/s12941-017-0216-y
Thomas, T. A. (2014). “Nanotechnology in consumer products,” in Nanotoxicology: progress toward nanomedicine (Boca Raton: CRC Press), 97–112.
Toyao, T., Saito, M., Horiuchi, Y., Mochizuki, K., Iwata, M., Higashimura, H., et al. (2013). Efficient hydrogen production and photocatalytic reduction of nitrobenzene over a visible-light-responsive metal–organic framework photocatalyst. Catal. Sci. Technol. 3, 2092–2097. doi:10.1039/c3cy00211j
Tu, J., Yang, Z., and Hu, C. (2015). Efficient catalytic aerobic oxidation of chlorinated phenols with mixed-valent manganese oxide nanoparticles. J. Chem. Technol. Biotechnol. 90, 80–86. doi:10.1002/jctb.4289
Tuo, Y., Liu, G., Zhou, J., Wang, A., Wang, J., Jin, R., et al. (2013). Microbial formation of palladium nanoparticles by Geobacter sulfurreducens for chromate reduction. Bioresour. Technol. 133, 606–611. doi:10.1016/j.biortech.2013.02.016
Turunc, E., Binzet, R., Gumus, I., Binzet, G., and Arslan, H. (2017). Green synthesis of silver and palladium nanoparticles using Lithodora hispidula (Sm.) Griseb.(Boraginaceae) and application to the electrocatalytic reduction of hydrogen peroxide. Mater. Chem. Phys. 202, 310–319. doi:10.1016/j.matchemphys.2017.09.032
Tyagi, S., Rawtani, D., Khatri, N., and Tharmavaram, M. (2018). Strategies for nitrate removal from aqueous environment using nanotechnology: a review. J. Water Process. Eng. 21, 84–95. doi:10.1016/j.jwpe.2017.12.005
Tyner, K. (2019). “Regulatory research supporting the development of drug products containing nanomaterials: a US-FDA perspective,” in Private communication at global summit on regulatory science 2019 on nanotechnology and nanoplastics. EUR 30195 EN.
Ullah, H., Wilfred, C. D., and Shaharun, M. S. (2017). Synthesis of silver nanoparticles using ionic-liquid-based microwave-assisted extraction from polygonum minus and photodegradation of methylene blue. J. Chin. Chem. Soc. 64, 1164–1171. doi:10.1002/jccs.201700144
Unuabonah, E. I., Adebowale, K. O., Olu-Owolabi, B. I., Yang, L. Z., and Kong, L. X. (2008). Adsorption of Pb (II) and Cd (II) from aqueous solutions onto sodium tetraboratemodified kaolinite clay: equilibrium and thermodynamic studies. Hydrometallurgy 93 (1–2), 1–9. doi:10.1016/j.hydromet.2008.02.009
US Environmental Protection Agency (US EPA) (2017). Chemical substances when manufactured or processed as nanoscale materials; TSCA reporting and recordkeeping requirements. Fed. Regist. 82 (8), 3641.
US Food and Drug Administration (FDA) (2020b). Europe Office. Available at: https://www.fda.gov/about-fda/office-global-operations/europe-office.
US Food and Drug Administration (FDA) (2020a). Nanotechnology guidance documents. Available at: https://www.fda.gov/science-research/nanotechnology-programs-fda/nanotechnology-guidance-documents.
US National Institute for Standards and Technology (NIST) (2020). Standard reference materials. Available at: https://www.nist.gov/srm.
Vanaamudan, A., Soni, H., and Sudhakar, P. P. (2016). Palm shell extract capped silver nanoparticles-as efficient catalysts for degradation of dyes and as SERS substrates. J. Mol. Liq. 215, 787–794. doi:10.1016/j.molliq.2016.01.027
Varadavenkatesan, T., Lyubchik, E., Pai, S., Pugazhendhi, A., Vinayagam, R., and Selvaraj, R. (2019). Photocatalytic degradation of Rhodamine B by zinc oxide nanoparticles synthesized using the leaf extract of Cyanometra ramiflora. J. Photochem. Photobiol. B, Biol. 199, 111621. doi:10.1016/j.jphotobiol.2019.111621
Vellaichamy, B., and Periakaruppan, P. (2016). Afacile, one-pot and eco-friendly synthesis of gold/silver nanobimetallics smartened rGO for enhanced catalytic reduction of hexavalent chromium. RSC Adv. 6, 57380–57388. doi:10.1039/c6ra10544k
Vennila, M., and Prabha, N. (2015). Plant mediated green synthesis of silver nano particles from the plant extract of Morinda tinctoria and its application in effluent water treatment. Int. J. Chemtech Res. 7, 2993–2999.
Verónica, R., Lago, A., Silva, B., Barros, Ó., Neves, I. C., and Tavares, T. (2024). Immobilization of biogenic metal nanoparticles on sustainable materials–green approach applied to wastewater treatment: a systematic review. Environ. Sci. Nano 11, 36–60. doi:10.1039/D3EN00623A
Vidya, C., Manjunatha, C., Chandraprabha, M., Rajshekar, M., and Mal, A. R. (2017). Hazard free green synthesis of ZnO nano-photo-catalyst using Artocarpus heterophyllus leaf extract for the degradation of Congo red dye in water treatment applications. J. Environ. Chem. Eng. 5, 3172–3180. doi:10.1016/j.jece.2017.05.058
Vijayan, R., Joseph, S., and Mathew, B. (2018). Eco-friendly synthesis of silver and gold nanoparticles with enhanced antimicrobial, antioxidant, and catalytic activities. IET Nanobiotechnol 12, 850–856. doi:10.1049/iet-nbt.2017.0311
Vilardi, G., Mpouras, T., Dermatas, D., Verdone, N., Polydera, A., and Di Palma, L. (2018a). Nanomaterials application for heavy metals recovery from polluted water: the combination of nano zero-valent iron and carbon nanotubes. Competitive adsorption nonlinear modeling. Chemosphere 201, 716–729. doi:10.1016/j.chemosphere.2018.03.032
Vinay, K., Neha, S., and Maitra, S. S. (2017). In vitro and in vivo toxicity assessment of nanoparticles. Int. Nano Lett. 7, 243–256. doi:10.1007/s40089-017-0221-3
Wacker, M. (2019). “Translational nanomedicine how to predict clinical performance?,” in Private communication at global Summit on regulatory science 2019 on nanotechnology and nanoplastics. EUR 30195 EN.
Wagner, A. J., Bleckmann, C. A., Murdock, R. C., Schrand, A. M., Schlager, J. J., and Hussain, S. M. (2007). Cellular interaction of different forms of aluminum nanoparticles in rat alveolar macrophages. J. Phys. Chem. B 111 (25), 7353–7359. doi:10.1021/jp068938n
Walker, V. G., Li, Z., Hulderman, T., Schwegler-Berry, D., Kashon, M. L., and Simeonova, P. P. (2009). Potential in vitro effects of carbon nanotubes on human aortic endothelial cells. Toxicol. Appl. Pharmacol. 236 (3), 319–328. doi:10.1016/j.taap.2009.02.018
Wang, F., Yu, L., Monopoli, M. P., Sandin, P., Mahon, E., Salvati, A., et al. (2013). The biomolecular corona is retained during nanoparticle uptake and protects the cells from the damage induced by cationic nanoparticles until degraded in the lysosomes. Nanomed. Nanotechnol. Biol. Med. 9 (8), 1159–1168. doi:10.1016/j.nano.2013.04.010
Wang, L., Nagesha, D. K., Selvarasah, S., Dokmeci, M. R., and Carrier, R. L. (2008). Toxicity of CdSe nanoparticles in Caco-2 cell cultures. J. Nanobiotechnol. 6 (1), 11. doi:10.1186/1477-3155-6-11
Wang, R., Wang, S., Tai, Y., Tao, R., Dai, Y., Guo, J., et al. (2017). Biogenic manganese oxides generated by green algae Desmodesmus sp. WR1 to improve bisphenol A removal. J. Hazard. Mater. 339, 310–319. doi:10.1016/j.jhazmat.2017.06.026
Wang, T., Jin, X., Chen, Z., Megharaj, M., and Naidu, R. (2014a). Green synthesis of Fe nanoparticles using eucalyptus leaf extracts for treatment of eutrophic wastewater. Sci. Total Environ. 466, 210–213. doi:10.1016/j.scitotenv.2013.07.022
Wang, T., Lin, J., Chen, Z., Megharaj, M., and Naidu, R. (2014b). Green synthesized iron nanoparticles by green tea and eucalyptus leaves extracts used for removal of nitrate in aqueous solution. J. Clean. Prod. 83, 413–419. doi:10.1016/j.jclepro.2014.07.006
Wang, X., Zhang, D., Qian, H., Liang, Y., Pan, X., and Gadd, G. M. (2018). Interactions between biogenic selenium nanoparticles and goethite colloids and consequence for remediation of elemental mercury contaminated groundwater. Sci. Total Environ. 613, 672–678. doi:10.1016/j.scitotenv.2017.09.113
Wang, Y.-T. (2000). “Microbial reduction of chromate,” in Environmental microbe-metal interactions. Abstr. Gen. Meet. Am. Soc. Microbiol., 225–235.
Wang, Z. (2013). Iron complex nanoparticles synthesized by eucalyptus leaves. ACS Sustain. Chem. Eng. 1, 1551–1554. doi:10.1021/sc400174a
Wang, Z., Xu, C., Li, X., and Liu, Z. (2015). In situ green synthesis of Ag nanoparticles on tea polyphenols-modified graphene and their catalytic reduction activity of 4-nitrophenol. Colloids and Surfaces A: Physicochemical and Engineering Aspects. doi:10.1016/j.colsurfa.2015.09.015
Watts, M. P., Coker, V. S., Parry, S. A., Pattrick, R. A. D., Thomas, R. A. P., Kalin, R., et al. (2015a). Biogenic nano-magnetite and nano-zero valent iron treatment of alkaline Cr(VI) leachate and chromite ore processing residue. Appl. Giochemistry 54, 27–42. doi:10.1016/j.apgeochem.2014.12.001
Watts, M. P., Coker, V. S., Parry, S. A., Pattrick, , Thomas, R. A. P., Kalin, R., et al. (2015b). Effective treatment of alkaline Cr(VI) contaminated leachate using a novel Pd-bionanocatalyst: impact of electron donor and aqueous geochemistry. Appl. Catal. B Environ. 170-171, 162–172. doi:10.1016/j.apcatb.2015.01.017
Wei, Y., Fang, Z., heng, L., and Tsang, E. P. (2017). Biosynthesized iron nanoparticles in aqueous extracts of Eichhornia crassipes and its mechanism in the hexavalent chromium removal. Appl. Surf. Sci. 399, 322–329. doi:10.1016/j.apsusc.2016.12.090
Weng, X., Huang, L., Chen, Z., Megharaj, M., and Naidu, R. (2013). Synthesis of iron-based nanoparticles by green tea extract and their degradation of malachite. Ind. Crops Prod. 51, 342–347. doi:10.1016/j.indcrop.2013.09.024
Weng, X., Jin, X., Lin, J., Naidu, R., and Chen, Z. (2016). Removal of mixed contaminants Cr (VI) and Cu (II) by green synthesized iron-based nanoparticles. Ecol. Eng. 97, 32–39. doi:10.1016/j.ecoleng.2016.08.003
Wu, Z., Su, X., Lin, Z., Owens, G., and Chen, Z. (2019). Mechanism of As(V) removal by greensynthesized iron nanoparticles. J. Hazard. Mater. 379, 120811. doi:10.1016/j.jhazmat.2019.120811
Wyllie, A. H. (1980). Glucocorticoid-induced thymocyte apoptosis is associated with endogenous endonuclease activation. Nature 284 (5756), 555–556. doi:10.1038/284555a0
Xiao, X., Ma, X.-B., Yuan, H., Liu, P.-C., Lei, Y.-B., Xu, H., et al. (2015a). Photocatalytic properties of zinc sulfide nanoceystals fabricated bymetal-reducing bacterium Shewanella oneidensis MR-1. J. Harzadous Mater. 288, 134–139. doi:10.1016/j.jhazmat.2015.02.009
Xiao, Y., De Araujo, C., Chau Sze, C., and Stuckey, D. C. (2015b). Toxicity measurement in biological wastewater treatment processes: a review. J. Hazard. Mater. 286, 15–29. doi:10.1016/j.jhazmat.2014.12.033
Xiao, Z., Yuan, M., Yang, B., Liu, Z., Huang, J., and Sun, D. (2016). Plant-mediated synthesis of highly active iron nanoparticles for Cr (VI) removal: investigation of the leading biomolecules. Chemosphere 150, 357–364. doi:10.1016/j.chemosphere.2016.02.056
Xu, Q., Li, W., Ma, L., Cao, D., Owens, G., and Chen, Z. (2020). Simultaneous removal of ammonia and phosphate using green synthesized iron oxide nanoparticles dispersed onto zeolite. Sci. Total Environ. 703, 135002. doi:10.1016/j.scitotenv.2019.135002
Yadav, I. C., Devi, N. L., Syed, J. H., Cheng, Z., Li, J., Zhang, G., et al. (2015). Current status of persistent organic pesticides residues in air, water, and soil, and their possible effect on neighboring countries: a comprehensive review of India. Sci. Total Environ. 511, 123–137. doi:10.1016/j.scitotenv.2014.12.041
Yang, W., Shen, C., Ji, Q., An, H., Wang, J., Liu, Q., et al. (2009). Food storage material silver nanoparticles interfere with DNA replication fidelity and bind with DNA. Nanotechnology 20 (8), 085102. doi:10.1088/0957-4484/20/8/085102
Yang, X., Liu, J., He, H., Zhou, L., Gong, C., Wang, X., et al. (2010). SiO2 nanoparticles induce cytotoxicity and protein expression alteration in HaCaT cells. Part. Fibre Toxicol. 7 (1), 1. doi:10.1186/1743-8977-7-1
Yaqoob, A. A., Parveen, T., Umar, K., and Mohamad Ibrahim, M. N. (2020). Role of nanomaterials in the treatment of wastewater: a review. Water 12, 495. doi:10.3390/w12020495
Ye, S.-F., Wu, Y. H., Hou, Z. Q., and Zhang, Q. Q. (2009). ROS and NF-κB are involved in upregulation of IL-8 in A549 cells exposed to multi-walled carbon nanotubes. Biochem. Biophys. Res. Commun. 379 (2), 643–648. doi:10.1016/j.bbrc.2008.12.137
Yehia, H. N., Draper, R. K., Mikoryak, C., Walker, E. K., Bajaj, P., Musselman, I. H., et al. (2007). Single-walled carbon nanotube interactions with HeLa cells. J. Nanobiotechnol. 5 (1), 8. doi:10.1186/1477-3155-5-8
Yi, H., Jiang, M., Huang, D., Zeng, G., Lai, C., Qin, L., et al. (2018). Advanced photocatalytic Fenton-like process over biomimetic hemin-Bi2WO6 with enhanced pH. J. Taiwan Inst. Chem. Eng. 93, 184–192. doi:10.1016/j.jtice.2018.06.037
Yi, H., Yan, M., Huang, D., Zeng, G., Lai, C., Li, M., et al. (2019). Synergistic effect of artificial enzyme and 2D nano-structured Bi2WO6 for ecofriendly and efficient biomimetic photocatalysis. Appl. Catal. B 250, 52–62. doi:10.1016/j.apcatb.2019.03.008
Yoshida, T., Niimi, S., Yamamoto, M., Nomoto, T., and Yagi, S. (2014). Effective nitrogen doping into TiO2(N-TiO2) for visible light response photocatalysis. J. Colloid Interface Sci. 447, 278–281. doi:10.1016/j.jcis.2014.12.097
Youseffu, S. R., Alshamsi, H. A., Amiri, O., and Salavati-Niasari, M. (2021). Synthesis, characterization and application of Co/Co3O4 nanocomposites as an effective photocatalyst for the discolorization of organic dye contaminants in wastewater and antibacterial properties. J. Mol. Liq. 337, 116405. doi:10.1016/j.molliq.2021.116405
Yu, Z. T., Liao, Z. L., Jiang, Y. S., Li, G. H., and Chen, J. S. (2005). Water-insoluble Ag–U–Organic assemblies with photocatalytic activity. Chem. Eur. J. 11, 2642–2650. doi:10.1002/chem.200401189
Yue, L., Wang, J., Zhang, Y., Qi, S., and Xin, B. (2016). Controllable biosynthesis of high-purity lead-sulfide (PbS) nanocrystals by regulating the concentration of polyethylene glycol in microbial system. Bioprocess Biosyst. Eng. 39, 1839–1846. doi:10.1007/s00449-016-1658-x
Zang, L. Y., van Kuijk, F. J., Misra, B. R., and Misra, H. P. (1995). The specificity and product of quenching singlet oxygen by 2,2,6,6-tetramethylpiperidine. Biochem. Mol. Biol. Int. 37 (2), 283–293. PMID: 8673011.
Zare, M., Namratha, K., Thakur, M., and Byrappa, K. (2019). Biocompatibility assessment and photocatalytic activity of bio-hydrothermal synthesis of ZnO nanoparticles by Thymus vulgaris leaf extract. Mater. Res. Bull. 109, 49–59. doi:10.1016/j.materresbull.2018.09.025
Zhang, C., Liu, S., Logan, J., Mazumder, R., and Phelps, T. J. (1996). Enhancement of Fe (III), Co (III), and Cr (VI) reduction at elevated temperatures and by a thermophilic bacterium. Appl. Biochem. Biotechnol. 57, 923–932. doi:10.1007/bf02941773
Zhang, P., Hou, D., O’Connor, D., Li, X., Pehkonen, S., Varma, R. S., et al. (2018). Green and size-specific synthesis of stable Fe–Cu oxides as earth-abundant adsorbents for malachite green removal. ACS Sustain. Chem. Eng. 6, 9229–9236. doi:10.1021/acssuschemeng.8b01547
Zhang, W., Zhang, D., and Liang, Y. (2019). Nanotechnology in remediation of water contaminated by poly- and perfluoroalkyl substances: a review. Environ. Pollut. 247, 266–276. doi:10.1016/j.envpol.2019.01.045
Zhang, Y., Chen, W., Zhang, J., Liu, J., Chen, G., and Pope, C. (2007). In vitro and in vivo toxicity of CdTe nanoparticles. J. Nanosci. Nanotechnol. 7 (2), 497–503. doi:10.1166/jnn.2007.125
Zheng, Y., Wang, Z., Peng, F., and Fu, L. (2017). Biosynthesis of silver nanoparticles by Plectranthus amboinicus leaf extract and their catalytic activity towards methylene blue degradation. Rev. Mex. Ing. Quím. 16, 41–45. doi:10.24275/rmiq/bio700
Zhou, D., Kim, D.-G., and Ko, S.-O. (2015). Heavy metal adsorption with biogenic manganese oxides generated by Pseudomonas putida strain MnB1. J. Ind. Eng. Chem. 24, 132–139. doi:10.1016/j.jiec.2014.09.020
Zhou, H., Pan, H., Xu, J., Xu, W., and Liu, L. (2016). Acclimation of a marine microbial consortium for efficient Mn (II) oxidation and manganese containing particle production. J. Hazard Mater. 304, 434–440. doi:10.1016/j.jhazmat.2015.11.019
Zhu, M.-T., Feng, W. Y., Wang, B., Wang, T. C., Gu, Y. Q., Wang, M., et al. (2008). Comparative study of pulmonary responses to nano-and submicron-sized ferric oxide in rats. Toxicology 247 (2), 102–111. doi:10.1016/j.tox.2008.02.011
Zhuang, Z., Huang, L., Wang, F., and Chen, Z. (2015). Effects of cyclodextrin on the morphology and reactivity of iron-based nanoparticles using Eucalyptus leaf extract. Ind. Crops Prod. 69, 308–313. doi:10.1016/j.indcrop.2015.02.027
Zinatloo-Ajabshir, S., Salehi, Z., and Salavati-Niasari, M. (2018). Green synthesis and characterization of Dy2Ce2O7 ceramic nanostructures with good photocatalytic properties under visible light for removal of organic dyes in water. J. Clean. Prod. 192, 678–687. doi:10.1016/j.jclepro.2018.05.042
Zock, J.-P., Verheij, R., Helbich, M., Volker, B., Spreeuwenberg, P., Strak, M., et al. (2018). The impact of social capital, land use, air pollution and noise on individual morbidity in Dutch neighbourhoods. Environ. Int. 121, 453–460. doi:10.1016/J.ENVINT.2018.09.008
Keywords: contaminants, green nanomaterials, wastewater, wastewater treatment, water pollution
Citation: Sanni SE, Oni BA, Okoro EE and Pandya S (2024) Recent advances in the use of biogenic nanomaterials and photocatalysts for wastewater treatment: challenges and future prospects. Front. Nanotechnol. 6:1469309. doi: 10.3389/fnano.2024.1469309
Received: 23 July 2024; Accepted: 09 September 2024;
Published: 10 October 2024.
Edited by:
Farshid Ghanbari, Abadan University of Medical Sciences, IranReviewed by:
Mahsa Moradi, Polytechnic University of Madrid, SpainAydin Hassani, Near East University, Cyprus
Copyright © 2024 Sanni, Oni, Okoro and Pandya. This is an open-access article distributed under the terms of the Creative Commons Attribution License (CC BY). The use, distribution or reproduction in other forums is permitted, provided the original author(s) and the copyright owner(s) are credited and that the original publication in this journal is cited, in accordance with accepted academic practice. No use, distribution or reproduction is permitted which does not comply with these terms.
*Correspondence: Samuel Eshorame Sanni, YWRleHozMDAwQHlhaG9vLmNvbQ==, c2FtdWVsLnNhbm5pQGNvdmVuYW50dW5pdmVyc2l0eS5lZHUubmc=; Babalola Aisosa Oni, b25pLmJhYmFsb2xhQHVuZC5lZHU=