- 1Department of Pharmacy, Abdul Wali Khan University, Mardan, Pakistan
- 2Department of Pharmacy, University of Malakand, Chakdara, Pakistan
- 3Department of Pharmaceutics, School of Pharmacy, University College, London, United Kingdom
- 4Department of Pharmaceutical Sciences, College of Pharmacy, Shaqra University, Shaqra, Saudi Arabia
- 5Particle Design and Drug Delivery Laboratory, Faculty of Pharmacy, Gomal University, Dera Ismail Khan, Pakistan
- 6Department of Zoology, University of Malakand, Chakdara, Pakistan
The exposure of human beings to various respiratory diseases has sparked an interest among scientists to propose and promote inhalable nanoparticle-based pharmaceutical products. This new drug delivery system will be an effective and prompt therapy for next-generation respiratory therapeutics. There are many available formulations on account of the treatments for lung diseases, i.e., oral, injectable, aerosol, nebulizer-based, etc. But, due to extended distribution and elimination, repeated intake, and complex systems of application, these treatments create overload on patients along with other expenses of daily life. Amid these treatments, inhalable nanoparticle-based dry powder formulations are considered superior owing to their simplicity, convenience of use, and long-term stability. This review aims to formulate an inhalable nanoparticle dry powder formulation to attain confined distribution of the remedy to the respiratory system. Dry powder formulations based on inhalable nanoparticles provide a novel and cutting-edge method of treating lung conditions. This novel drug delivery system is an improved treatment for lung disorders in terms of its efficacy and reduced dose requirement. Subsequently, the availability of this formulation will be an alternative option for patients to improve treatment compliance and, foremost, therapeutic outcomes in different lung ailments. The salient features of dry formulations that illustrate their uniqueness are deep penetration to lung tissues, enhanced deposition in lung regions, improved solubility, elevated bioavailability, targeted drug delivery, stability at storage, convenient to use, versatile and customizable formulation. This novel inhalable formulation is based on finely dried nanoparticles or freeze-dried particles. This formulation will contain an excessive amount of dried nanoparticles, and if it is combined with a beneficial tool, i.e., a spray, pump, or puffer, it will be a pleasant way to apply drugs directly to the lungs.
1 Introduction
The severe implications of lung diseases, i.e., tuberculosis, cystic fibrosis, persistent blocking diseases of the lungs, i.e., COPD, cryptogenic fibrosing alveolitis, asthma, interstitial diseases of the respiratory system, and lung cancer, have ignited significant attention to develop an intact and efficacious respiratory treatment. A drug administration system that delivers the medicinal agent directly to the pulmonary system is the requirement of the present time. Localized drug delivery to the lungs is proposed over systemic drug administration systems, i.e., oral or intravenous. This type of drug delivery system will show more effects with fewer side effects (Liao et al., 2019).
More approaches in nanotechnology have restored attention to employing nanoparticles (particles < 1,000 nm) as carriers for lung drug delivery (Wan et al., 2020a). Drug particles of nanosize have remarkable advantages in comparison to regular or traditional inhalable drug delivery systems (Figure 1). These advantages can be enumerated in the following forms (Chan et al., 2023):
In the first place, the surfaces of these particles could be adorned with substances, i.e., immunoglobulin, oligopeptides, and others and with specific target cells of various types inside the pulmonary system, e.g., lung carcinoma cells (Mangal et al., 2017).
In the second place, many microparticles that reside in the size limit of 0.5–3 µm are engulfed quickly by scavenger cells that are present in the alveolar region (Costa et al., 2021). But nanoparticles having a size below < 300 nm can rescue themselves from the consumption of macrophages. In that form, drugs are protected from enzymatic decomposition (Kaneko et al., 2018).
Moreover, drugs can be easily incorporated into cells by endocytosis if they are nanosized as compared to the free form of drugs (Thiyagarajan et al., 2021).
Furthermore, the release of a drug can be modified by using its nanosize. Drug release can be converted to sustain or controlled after changing its size to a nanometer (Dandekar et al., 2010).
At last, and particularly, nanoparticles can easily move from air sacs to the blood circulation. In this way, this can be regarded as another technique for distribution into systemic circulation. Nanotechnology-based particles reduce the use of complex forms of drug administration in the body. This characteristic of the drug is especially useful for both lung and body ailments, i.e., spreading lung carcinoma, TB disease, etc., (Farhangi et al., 2019).
Nanoparticles are usually delivered to the lungs by using aerosol as a delivery tool. Other commercially available aerosols are atomizers, pressurized metered-dose inhalers (pMDIs), soft-mist inhalers, and dry powder inhalers (DPIs) (Nemmar et al., 2002).
There is also a disadvantage to using nanoparticles i.e., the elevated superficial energy of these nanoparticles, which promotes their aggregation by Ostwald ripening and recrystallization. Among many inhalers, the DPIs are hence supplementary acceptable regarding the transport of nanograins by aspiration through the mouth because of their considerable material consistency (Porsio et al., 2020). DPIs are easily carried in comparison to nebulizers. These tools also remove the demand for hand-breath parallel use in pMDIs, which are especially used in chronic pulmonary diseases (DAddio and Prud’homme, 2011).
Inhalable nanoparticle-based dry powder formulations can be designed using different methods. Among them, one is to adsorb the drug onto the surface of a carrier powder. It can also be designed by agglomerating the drug into powder or by distributing it equally inside the powder mixture (Hu et al., 2020). The main difference between inhalable nanoparticle-based powders and regular or traditional inhalable powder formulations is the size of the drug particles. In regular aspiration of nano-fleck formulation, the grains or molecules are present in micrometers (Ke et al., 2022). Usually, conventional inhalable formulations have vehicle-admixture particles, big, spongy grains, etc. When conventional forms of inhalable powder formulation are used by patients, the microsized drug particles dissolve in fluid inside the lungs (Khan and Chaudary, 2020). Contrary to this, when nanoparticle-based drug powder is inhaled, it is redistributed into primary nanoparticles inside the fluid of the lung, and this feature makes that type of formulation more unique and attractive (Liang et al., 2020).
The basic requirement of the inhalable-based nanoparticle-based formulation is to get deposits inside the pulmonary system for its efficient effects (Haworth et al., 2019). An optimal particle size is required for inhalable nanoparticle-based formulations. Particles with an aerodynamic diameter (DA) between 1 and 5 µm are known for better deposition in the respiratory system. After deposition, these particles in alveoli are readily redispersed into primary nanoparticles (Honmane et al., 2019; Safety, 2024).
Some precautionary measurements are necessary during the drying the suspension of nanoparticles because stress conditions like shear and thermal stress can cause changes in the integrity, size, and morphology of the nanoparticles. These changes can cause differences in the therapeutic effects of these formulations (Jain et al., 2009; Chan and Chow, 2022).
In a nutshell, dry powder inhalations of nanoparticles have advantages regarding small fleck technology progress and the targeted effect of nanograins in the pulmonary system, particularly as a therapy for TB disease, air sac fibrosis, and lung carcinoma. Nanoparticles are delivered to the pulmonary system by using this technique in order to get more effects, longer retention time inside the lungs, elevated uptake inside cells, a high immunological response, improved pharmacokinetics, a prolonged release profile, better tolerability, and reduced toxicity (Soni et al., 2011).
Some interventions in formulating nanoparticle-based dry powder inhalation can enhance the redispersibility of nanoparticles after pulmonary delivery (Kwok et al., 2011). These approaches will be helpful to target macrophages and are therefore suitable for many ailments of the pulmonary system, i.e., TB disease. It might also be effective to adjust the lymphokine composition discharged from monocytes in a way that helps end cancer growth (Tsapis et al., 2002; Chow et al., 2019).
1.1 Comparative analysis between nanoparticles, microparticles, and other delivery forms
When it comes to treating lung disorders, a comparison of nanoparticles, microparticles, and other drug delivery forms reveals the unique benefits and drawbacks of each approach. Particle size, lung deposition, drug solubility and bioavailability, targeting capabilities, stability, patient compliance, and adaptability are some of the important factors taken into account in this research.
1.1.1 Particle size and lung deposition
Nanoparticles with small size show better absorption into alveoli and other deep lung areas. Their tiny aerodynamic diameter, which enhances deposition and allows for effective delivery to specific lung regions. While microparticles in Comparison to nanoparticles, show better control over release but, they are appropriate for treating upper respiratory tract conditions locally.
Other drug delivery system are good for a variety of lung conditions but are less effective for certain lung areas. They are helpful for administering liquid medications for short-term care and frequently call for greater patient upkeep and coordination.
1.1.2 Drug solubility and bioavailability
Drugs that dissolve poorly are made more soluble by a high surface area-to-volume ratio by converting them to nanoparticles. They have greater absorption and dissolution in lung tissues, leading to increased bioavailability.
While microparticles can include both medications that dissolve in water and those that don't. Their release profiles that can be altered due to encapsulation strategies. But, due to bigger size and slower dissolving rates than nanoparticles, they have decreased bioavailability (Chow et al., 2019).
Other drug delivery systems provide drug availability instantaneously via suspensions and solutions. In general, it is simpler to manufacture and stabilize liquid forms. But, they are restricted in use because improvement of poorly soluble medications is required. Also there is the possibility of quick removal from the lung surface.
1.1.3 Targeting capabilities
In nanoparticles ligands or antibodies can be used to functionalize it so that it is delivered to particular lung cells or tissues on purpose to increased absorption by immune cells like macrophages. But they are more expensive manufacture and intricate design.
Microparticles can be made to release drugs locally in the upper respiratory tract. They are beneficial for conditions involving the trachea and bronchi. In Comparison to nanoparticles, less successful in establishing cellular targeting.
Many different kinds of drug delivery systems may be delivered using nebulizers and MDIs. They are suitable for both emergency and acute care. But their limitations in targeting capacities may result in systemic adverse effects (Weng et al., 2019).
1.1.4 Stability and shelf life
Nanoparticles have improved stability as a powder in a dry state. They show long shelf life if kept and prepared correctly. But, sometimes they are highly susceptible to temperature changes and other environmental factors.
Microparticles because of their bigger and have less surface energy than nanoparticles, they are often more stable. But there is the possibility of settling and gathering in forms of suspension. Other drug delivery systems are easy to administer and store the solutions and suspensions. Nebulizers don’t need as exacting of storage guidelines. But, they suffer from microbiological contamination and deterioration that is common with liquid forms (Tsapis et al., 2002).
1.1.5 Patient compliance and convenience
Nanoparticles are designed to be easily manipulated, either as metered-dose inhalers (MDIs) or dry powder inhalers (DPIs). Compliance is improved by portability and administrative simplicity. Yet, to deliver drugs effectively with DPIs, correct inhaling technique is required.
Contrary to them microparticles are adaptable to DPI formulas and less likely to cause problems with deep lung penetration and are easier to control but, higher dosages could be needed to have therapeutic results.
Additional drug delivery forms like nebulizers are useful for patients—such as little children and elderly patients—who are unable to utilize inhalers. MDIs are useful for providing relief quickly.
But, nebulizers need to be cleaned and maintained on a regular basis and are less portable (Tsapis et al., 2002).
1.1.6 Versatility and customization
Nanoparticles may be designed to meet a range of therapeutic requirements, including as improved solubility, targeted administration, and controlled release. They are adaptable to a variety of medications. But their intricate production procedures make them expensive. Microparticles are adaptable to various medication release patterns and customized therapies. They are appropriate for many different kinds of drugs. But, they are less flexible in focusing on particular lung regions or cells (Chow et al., 2019).
Other drug delivery systems have broad suitability for a variety of medications and illnesses. They are easier to scale up and create. But there are many ways to customize sophisticated delivery capabilities like controlled release and targeting.
Moreover, Regulatory issues, target location, drug type, and intended release profile all influence the precise needs of the drug delivery system, which determine whether delivery form—nanoparticles, microparticles, or another—to use. Although great efficiency and tailored administration are advantages of nanoparticles, stability issues and possible toxicity are drawbacks. Microparticles provide a compromise between controlled release and ease of manufacture, yet their capacity to transport medications inside cells may be restricted. A wide variety of applications with differing levels of complexity, stability, and biocompatibility are available with alternative delivery methods. A thorough evaluation of these variables in light of the treatment objectives determines the best delivery strategy (Chow et al., 2019).
2 Approaches to enhance deep lung deposition
Different procedures have been adopted to deal with the problems of producing nanoparticles in dry powder inhalations to increase lung deposition. These approaches are the following:
2.1 Huge absorptive/spongy type nanoparticle aggregates
Respirable pharmaceutical products are designed to form huge absorptive nanoparticle aggregates. These aggregates are locked up with each other in different ways, i.e., by van der Waals forces or the application of binders, namely, polymers, phospholipids, or sugar. Hollow nanoaggregates are formed by van der Waals forces, and nanocomposites are formed by polymers, phospholipids, or sugar (Kwok et al., 2011).
2.1.1 Formulation of nanoparticle aggregate/lumps
Dull lumps are mixtures of lipid polymer nanoparticles (LPNPs) consisting of hollow nanoparticle lumps that are in merged form. That lump has the capability to NP systems with the easy flowability of huge absorptive microspheres. These nanoaggregates are processed by spray drying of nano-lumps to convert them into dull, dried microparticles. These dried microparticles have an outer cover that consists of nano-flecks or nano-molecules of spongy-like lumps.
Afterward, the spray-drying method dries and vaporizes the NP blend and other fillers. The nanoparticle blend is dried by the application of hot gas. That hot gas causes the drying of wet molecules and converts them to dull, dried molecules (Tsapis et al., 2002).
There are two extremely important points that are indispensable for the drying process to process absorptive particles. At the first point, the time necessary for the suspension of nano-lumps to dry is noted, and at the second point, the time needed for the nano-lumps to separate from all sides and accumulate to the center of the whole nano-molecule mixture is noted. The ratio of both points (the first over the second) describes a Péclet number (Pe) (Chow et al., 2007).
Nano-lumps that are processed in the form of dull microparticles by the spray drying method will have a Péclet number greater than 1 (Pe > 1). Three important parameters are necessary to be managed for getting maximum Pe and finding dull absorptive particles. These parameters are: application of temperature, nano-lump spraying efficiency, and supply rate (Chow et al., 2019).
2.2 Nanoparticle based composites (nanocomposites)
Nanocomposites are made by joining NP agglomerations with other excipients to form small specks suitable for distribution to the respiratory system and extensively to alveoli. The principle distinction between nanoparticle composites and nanoaggregates is that nanoparticle composites are not void particles; so far, they hold the needed aerodynamic properties for distribution to the respiratory system due to their spongy surface. Nano-particle composites can be designed by using polymers and active ingredients (Cheow et al., 2011).
2.2.1 Formulation of nanocomposite
Nanoparticle composites are formed by mixing nanoparticle agglomerates with other inactive ingredients. Different studies show the results of passing temperature and the weight of nanoparticles in relation to inactive ingredients in nanoparticle composites. Mostly, it is shown that nanoparticle composites are made up of sugar, i.e., lactose or tremalose, as binder.
An optimal inlet temperature is necessary for different sizes of initial nanoparticles. Big-size particles up to 400 nm have excessive potential to endure high rates of temperature; on the other hand, small nanoparticles up to 200 nm in size that are joined to form nano-particle composites are not dissolvable if the rate of temperature is kept at 80°C and above (Cheow et al., 2011; Yu et al., 2014).
Nanoparticles with a size of 400 nm or nanocomposites are formed effectively with tremalose if the temperature provided is 90°C and the nanoparticle with a non-active ingredient ratio is 45%. By weight (Cheow et al., 2011; Chow et al., 2019).
If 70°C is passed for designing nanoparticle composites with tremalose, then the best results are obtained by getting 200 nm-sized composites, disregarding the weight ratio of nanoparticles and non-active ingredients.
Additional studies showed that if low temperatures are provided, nanoparticle composites of large sizes can be obtained that will have good dissolution properties. A favorable temperature is required both for the size and type of binder that are applied for formulating nanoparticle composites.
The spray drying technique is based on the glass transition temperature of polymers (Tg) if the optimum temperature is used. This method is designed for both small and large nano-particle composites (Cheow et al., 2011).
By the spray-freeze drying method, nanoparticles can be protected from severe physical environments similarly to heating pressure and high tangential stress. These factors affect the polymer matrix and the redispersibility of nanocomposite. The freeze-drying technique formed dehydrated and dried nanoparticles after removing the solvent. These nanoparticles are absorptive, spongy, and spherical in shape and are good for inhalation. In this technique, cold gas is used for both freezing and drying the particles (Yu et al., 2014).
A research study displayed that nanoparticle composites formed using the spray-freeze drying technique have increased dissolution in water as compared to nanoparticle composites that are attained by the spray drying method. Two non-active ingredients, i.e., mannitol and polyvinyl alcohol (PVA), are used in the spray-freeze drying process. These non-active ingredients are used in different concentrations to maintain the nanoparticle composites that are drying by the spray-freeze drying process. A mostly 4:1, 3:2, and 3:7 ratio is used for taking nanoparticles and non-active ingredients in this technique. Higher concentrations of ingredients like mannitol (0%–70%) in the formulation can increase the dissolution rate of the nanoparticle composite from 30% to 85%. The greater dissolution rate of nanoparticle composites is achieved using 60%–70% polyvinyl alcohol (Zhu et al., 2022).
Designed flocculated nanocomposites are formed using different concentrations of active ingredients and flocculating agents like l-leucine solution (1% w/v) and solid sodium chloride crystals. The amount is adjusted to 1:1 for both active ingredients and flocculating agents. The l-leucine is selected as a flocculating agent that is slowly added to the nanoparticle suspension during the homogenization process. Afterward, the flocculated nanoparticle suspension is dried and dehydrated by the freeze drying method (Larhrib et al., 1999).
2.3 Microparticle carrier system
In a pulmonary delivery system, the problem of getting the desired diameter of nanoparticles can be corrected. It is corrected by incorporating the nanoparticles into microparticles. This approach provides optimal dispersibility and deposition properties (Chan and Chow, 2022).
2.3.1 Formulation of microparticles
According to reported research, lactose microparticles are used to load a variety of nanoparticles, like gelatin and poly isobutyl cyanoacrylate. Afterward, the spray-drying technique is used to atomize these nanoparticles. These resultant microparticles containing nanoparticles have a mass median aerodynamic diameter (MMAD) of 3.0 ± 0.2 and a fine particle fraction (FPF) of 40%.
Nanoparticles and microparticle carrier systems are prepared at the same time. A double emulsion without the active ingredient, i.e., IgG, and a polymer solution, i.e., PLGA, is added to the spray drying machine. A bulking material, i.e., lactose, is also added to the machine, and a high temperature is passed. By this technique, a high temperature is passed, i.e., 100°C, without causing any degradation of IgG (Flume et al., 2021).
3 Approach to enhance redispersibility of nanoparticles
The main aim of the drug design technique for nanoparticles is to retain them extensively throughout the pulmonary system. For this purpose, nanoparticles are designed to release readily and redisperse besides changes in size. Both spray-freeze drying and spray drying techniques form different quantities of nanoparticle-based agglomeration. This variable amount causes hindrance in the dissolution process and also causes changes in nanoparticle size (Cheow et al., 2010).
To overcome this problem, non-active ingredients that dissolve readily, like lactose, mannitol, and cyclodextrin, are used. Sometimes, those polymers that are easily solubilized in water are used. Those polymers are polyvinyl alcohol and PEG-6000. Moreover, this issue can be resolved by adding various types of surface active agents, like polysorbate 80, etc. All these remedies work to improve the dissolution of nanoparticles (Jain et al., 2017).
An approach is effectively followed in which an effervescent pair is used in a nanoparticle-based system when it is passed through the spray drying process. After drying technique, the microcarrier system that contains nanoparticles possesses effervescent properties, which cause them to release nanoparticles actively when these particles come into contact with water and humidity, similar to the fluids that are present inside the pulmonary system. (Arpagaus et al., 2018).
In the formulation preparation process, two mixtures are prepared. In mixture (A), the effervescent chemicals, i.e., citric acid and anhydrous sodium carbonate, are mixed with a poly (isobutyl cyanoacrylate) (PIBCA) nanoparticle distribution. For the preparation of blend B, ammonium hydroxide (ammonia) suspension with anhydrous sodium carbonate is mixed to a blend lactose monohydrate that is dried by the spray drying method (Taki et al., 2010).
Then both blends (A) and (B) are added instantly before drying by the spray-drying technique. The action of the ammonia solution is to stop the carbonation effect before going to the drying process. Due to its low boiling point (38°C), it is discarded during drying operations (Arpagaus et al., 2018).
Using the aforementioned formulation process, microcarrier flecks with diameters less than 5 μm, round in shape, and nanoparticles that are dispersed constantly all around the mixture will result. The mean MMAD for carbonated carrier particles will be slightly less than 5.6 µm and 2.17 ± 0.42 µm; the FPF will be about 46.47% ± 15%; and the geometric standard deviation (GSD) will be 2.00 ± 0.06. This is confirmed by scanning electron microscopy (Li et al., 2013).
4 Researches on inhalable nanoparticles-based dry powder formulation
Different strategies had been acquired in previous years in order to develop inhalable nanoparticulate-bbased dry powder formulations (Murday et al., 2009). Those preparation, along with applications, are mentioned in Table 1.
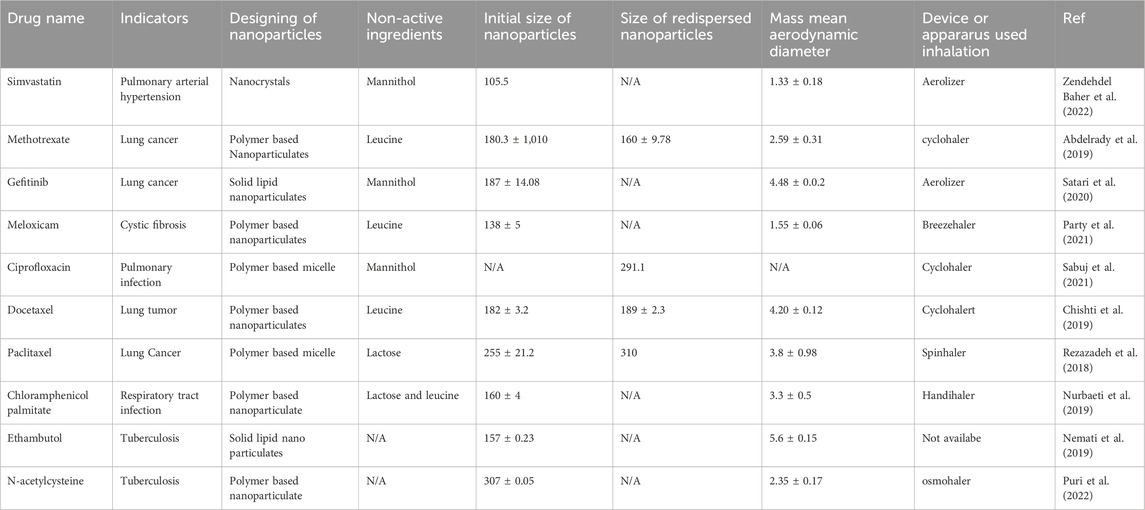
Table 1. Approaches adopted for development of nano particulates based dry powder inhalation formulation.
4.1 Dry powder formulation of simvastatin nanoparticles for treating pulmonary arterial hypertension
It is accepted practically that simvastatin can be applied to treat pulmonary arterial hypertension (PAH). To confirm it, a study was conducted to plan a simvastatin nanoparticle-based dry powder inhalation (DPI) therapy (Zendehdel Baher et al., 2022). Dry lump of simvastatin were designed due to their capability to NP systems with the easy flowability of huge absorptive capacity. Simvastatin nanoaggregates were processed by spray drying of nano-lumps to convert them into dull, dried microparticles. These dried microparticles had an outer cover that consists of nano-flecks or nano-molecules of spongy-like lumps. The main reason for nanoaggregates is to retain them extensively throughout the pulmonary system. Simvastatin nanoaggregates are designed to release readily and redisperse besides changes in size (Kwok et al., 2011).
According to that research study, simvastatin nanoflecks were processed. For this purpose, an emulsification and homogenization-extrusion method were used. Then that formulation was dried by the spray drying technique. The dispersion of simvastatin nanoflecks was dried after adding mannitol. That preparation was converted into a form that could be easily taken by inhalation (Zendehdel Baher et al., 2022).
The prepared drug formulation was analyzed for particle size determination, outer shape, and crystal form. Manufactured nanoparticles that were attained from micro-size particles of the dry form of preparation were further evaluated by dynamic light scattering (DLS), scanning electron microscopy (SEM), and X-ray diffraction pattern (XRPD), respectively (Kononenko et al., 2015). Dry preparation that can be taken by inhalation was analyzed for aerosolization performance by the Next-Generation Impactor (NGI) equipped with an Aerolizer®. Analysis showed that Simvastatin nanoparticulates had a 100 nm size with a small dispersibility, i.e., PDI = 0.105.
The X-ray diffraction results declared that the crystal form of the drug was reduced by the spray drying technique. Microscopic results revealed that accumulated nanoparticulates were in a better range size-wise and had good shape and surface characteristics. Those characteristics were found.
4.2 Utilizing gelatin nanocarriers for pulmonary delivery of methotrexate in lung cancer therapy
Gelatin is used as a nanocarrier to hold anti-cancer drugs. It is used to deliver the drug to the pulmonary system for treating cancer because of its many benefits. Gelatin is an easily decomposed and denatured protein. It retains several functional groups that could be changed. In addition, gelatin has equal hydrophilic and hydrophobic properties, which helps to load anti-cancer drugs easily.
Desired diameter of nanoparticles of drug can be obtained by incorporating the nanoparticles into microparticles. This approach provides optimal dispersibility and deposition properties.
A research study was conducted in which these nanocarriers type microparticles that were used to efficiently deliver methotrexate to the pulmonary system in cases of lung cancer (Flume et al., 2021). According to that study, the solvent discharging method was applied to process gelatin nanoparticulates. Optimized formulations were prepared by Box Behnken’s design of the experiment. Conventional and gelatin-based nanoparticles of anti-cancer drugs were studied on lung adenocarcinoma and macrophages by using flow cytometry (Abdelrady et al., 2019).
Methotrexate analysis was performed on the basis its conjugation with nanoparticles and its encapsulation after loading. A cytotoxicity study was also conducted.
Nano-particulates engraved into microparticles were processed by spraying both nanoparticulates and leucine into the drying technique. Optimization by Box Behnken design showed that nanoparticles were of uniform size and had effective uptake by cancer cells.
Gelatin-based nanoparticles showed a suitable and improved intake by malignant cells. Moreover, methotrexate contained nanoparticulates, which revealed that nanoparticulates had a significant effect on reducing the IC50 up to four times that of the usual drug. Gelatin nanoparticles embedded in microparticles prepared by the spray drying method have good inhalable properties. That quality confirmed lung distribution in the respiratory system disease. In this way, it is proven that nano-in-micro particulates provide a good alternate for lung cancer therapy (Abdelrady et al., 2019).
4.3 Processing and characterization of dry powder preparations containing glucosamine-bounded gefitinib solid lipid nanoparticles for cancer treatment in the lungs
One of the best chemotherapeutic agents used in lung cancer is gefitinib. The drug is an inhibitor of the tyrosine kinase receptor and epidermal growth factor. Conventional therapy with gefitinib causes many toxicities. A recent study was performed to reduce the toxicities caused by gefitinib. Gefitinib was incorporated into the glucosamine dry powder preparation used for inhalation. Gefitinib glucosamine solid lipid nanoparticles (Gef-G-SLNs) were developed in order to locally transfer gefitinib to lung tumors (Taymouri et al., 2020).
Dull lumps of gefitinib mixtures of lipid polymer solid nanoparticles consisting of hollow nanoparticle lumps that were in merged form were designed. That lump had the capability to nanoparticles systems with the easy flowability of huge absorptive microspheres.
These nanoaggregates were processed by spray drying of nano-lumps to convert them into dull, dried microparticles. These dried microparticles had an outer cover that consists of nano-flecks or nano-molecules of spongy-like lumps. Afterward, the spray-drying method converted them into dull, dried molecules. (Kwok et al., 2011).
The emulsion-solvent evaporation and evaporation processes were used for the design of Gef-G-SLNs. Optimization was done through an irregular factorial design. A cancer cell line study was conducted using optimized nanoformulation against A549 cells. Dry powder formulations on the basis of mannitol or lactose were obtained from Gef-G-SLNs after spray drying. Aerosolization characteristics were studied by the Anderson Cascade Impactor. Moreover, analysis in the form of drug loading, entrapment efficiency, zeta potential, particle size, polydispersity index, and release efficiency was conducted. The optimized formulation had a drug loading of 33.29%, an encapsulation efficiency of 97.31 ± 0.23%, a zeta potential of −15.53 ± 0.47 mV, a particle size of 187.23 ± 14.08 nm, a polydispersity index of 0.28 ± 0.02, and a release efficiency of 35.46 ± 2.25%. The Gef-G-SLNs showed improved results in the treatment of cancer in relation to commercial gefitinib (Taymouri et al., 2020).
Flow cytometry and fluorescence microscopy were applied for the drug intake inside the cells. Analysis confirmed elevated drug intake in cancer cells, i.e., A549, by G-SLNs as compared to non-targeted SLNs.
The prepared mannitol containing micro particulates showed appropriate air flow properties with a desired mass median aerodynamic diameter of 4.48 µm and a fine particle fraction of 44.41%. So, it can be decided that the new preparation of the drug showed better results in the treatment of cancer related to the respiratory system (Taymouri et al., 2020).
4.4 Ethambutol-loaded solid lipid nanoparticulates as dry powder inhalation therapy for tuberculosis
Ethambutol hydrochloride (EMB) is used to treat tuberculosis. Many drugs are used to treat tuberculosis in humans. Often, it is used as a protection agent against those drugs that cause unknown resistance inside the body (Nemati et al., 2019).
Ethambutol dull lumps were created by combining solid lipid polymer nanoparticle lumps with hollow nanoparticle lumps in a merged form. That lump was capable of forming nanoparticle systems that could easily flow like massive absorptive microspheres.
The procedure of spray drying the nano-lumps resulted in the production of dull, dried microparticles from these nanoaggregates. The exterior layer of these dried microparticles was composed of tiny, spongy-looking lumps called nanoflecks or nanomolecules. The spray-drying process thereafter turned them into desiccated, drab molecules (Kwok et al., 2011).
Ethambutol is normally used orally in patients. But it causes some side effects and also creates cellular toxicity. Therefore, it was decided to design direct lung administration therapy. Direct administration will be a more attractive and affordable choice among patients. That type of therapy would also decrease side effects and cellular toxicity.
The drug was designed in a drug-form inhalable formulation for direct administration to the lungs. For that purpose, the drug was loaded into solid lipid nanoparticles (SLNs). A dry powder inhaler (DPI) was used to administer the dry powder formulation.
Preparations were processed using two techniques: hot homogenization and ultrasonication. EMB-loaded SLN-based dry inhalable formulations were dried by the spray drying technique along with mannitol. Sometimes, mannitol is not added (Nemati et al., 2019).
Various investigations into formulations were conducted. Among them, flowability of the processed powders, compressibility index, Hausner ratio, and in-vivo settling of the powders were determined. Next-Generation Impactor (NGI) analysis was selected to study the in vitro deposition of powder.
Nanoparticle-based dry powder formulations of ethambutol had a higher encapsulation efficiency of up to 98%, and the particle size of the obtained formulation was sub-100 nm, respectively. Tests for toxicity were conducted for EMB-embedded SLNs by colorimetric assay. That assay exhibited that SLNs of ethambutol were biologically acceptable and non-toxic.
All results confirmed that the prepared powder formulations had good flowability and had suitable aerodynamic properties. It was concluded on the basis of all assessments that EMB-filled SLNs in dried powder form had more capability to treat tuberculosis directly (Nemati et al., 2019).
4.5 Preparation and ex vivo and in silico analysis of “nano-in-micro” dry powder inhalation retaining meloxicam
Drug administration directly to the pulmonary system shows elevated bioavailability, provides a great effective area for assimilation, and has minimal drug destruction. It is required to design and develop nanoparticles of the drug and then formulate it into dry powder form for enhanced therapeutic effect. Meloxicam (MX) is the least soluble in water. Meloxicam was chosen for research work owing to its low water solvability. A new technique was applied to enhance its water solubility. (Party et al., 2021).
Meloxicam (MX) is used to treat cancer in the lungs, mucoviscidosis, and persistent blocking disease of the respiratory system. Meloxicam particles were engineered to form respirable “nano-in-micro” dry powder inhalation preparations, i.e., DPIs. The inhalable meloxicam formulation contained meloxicam and other ingredients, i.e., poly-vinyl-alcohol and leucine.
The process for meloxicam creating nanocomposites involves combining NP agglomerations with other excipients to create tiny particles that may be widely distributed into alveoli and the respiratory system.
The main difference between nanoparticle composites and nanoaggregates is that the former are not empty particles; instead, because of their spongy surface, they currently possess the aerodynamic qualities required for distribution to the respiratory system. Polymers and active substances can be utilized to create nano-particle composites (Larhrib et al., 1999).
Nanoparticles of drug were targeted to the respiratory zone after being converted into microcomposites. The drug in that form reached a higher drug concentration as compared to the conventional form of the active ingredient (Zhu et al., 2022).
Different investigations in the form of particulate size estimation, shape, density, inter-particular connection, crystal form identification, ex vivo distribution, ex vivo absorbent property, ex vivo air flow particle diameter (Andersen cascade impactor), and in silico aerodynamics (stochastic lung model) were conducted.
Meloxicam (MX) nanoparticles in micro-based inhalable dry powder formulations were processed by wet milling and spray-drying. Spherical-shaped particles with sizes 3–4 µm were designed. The nanoparticles of meloxicam had an increased surface area, and its new amorphous form confirmed the improved dispersibility and distribution properties of meloxicam.
All established preparations exhibited suitable aerodynamically acceptable properties. The optimal formulation showed 1.5–2.4 µm MMAD and 72%–76% fine particle fraction (FPF) values. The in silico estimation of the drug confirmed the extensive settling in the respiratory system. MX specimens were found suitable for therapy after many analyses for lung diseases (Party et al., 2021).
4.6 Sustained-release microparticle dry powder aerosol formulation of chloramphenicol palmitate or thiamphenicol for lung delivery
Another study was conducted to prepare respirable, prolonged-release nanoparticles containing chloramphenicol or thiamphenicol. Nano-implanted microparticles of the drug are designed to deliver it to the lungs (Nurbaeti et al., 2019).
It was possible to solve the issue of attaining the required diameter of nanoparticles in a pulmonary delivery system. By combining the nanoparticles with the microparticles, it was fixed. The best dispersibility and deposition qualities was offered by this method.
Lactose microparticles had been found to be effective in loading a range of nanoparticles, including poly isobutyl cyanoacrylate and gelatin. After that, these nanoparticles were atomized using the spray-drying method. The resulting microparticles with nanoparticles had a fine particle fraction (FPF) of 40% and a mass median aerodynamic diameter (MMAD) of 3.0 ± 0.2. Simultaneous preparation of nanoparticles and their carrier systems was carried out (Flume et al., 2021).
Poly (lactic-co-glycolic acid) is a heteropolymer that was used to prepare PLGA-based nanoparticles of two palmitate ester drugs, i.e., chloramphenicol or thiamphenicol.
The emulsion-solvent-evaporation method was used to fill the drug with PLGA and form nanoparticles, or pure drug nanoparticles. Formulation in the form of a suspension of nanoparticles was then dried by spray-drying. Nanoparticle suspensions contained lactose, which is used for bulk, and L-leucine as a diffusing amplifier. The prepared dry microparticle powders were then useful for inhalation. Morphological analysis of microparticles by scanning electron microscopy was done. According to that analysis, existing microparticles were found to be spherical and shrinking, having no crystal-like structures (Figure 2).
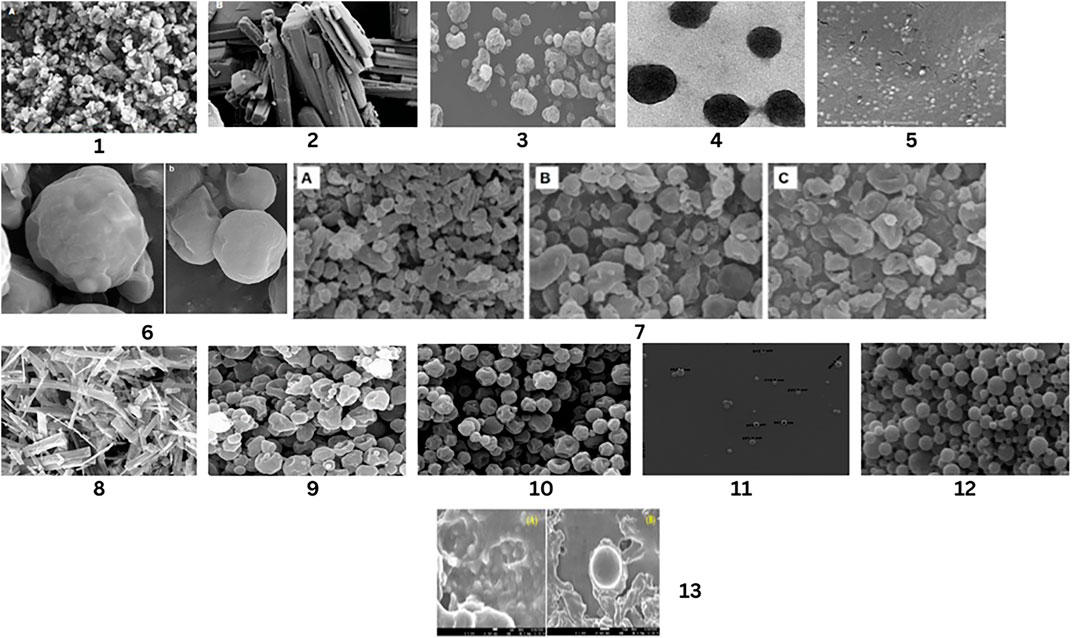
Figure 2. SEM Analysis of Different drug’s Nanoparticles and unprocessed drug. Nanoparticles of simvastatin by Kononenko, V et.al (1), Unprocessed form of simvastatin by Kononenko, V et.al (2),Nano-particles of methotrexate by Abdelrady, et.al. (3), Microparticles of methotrexate after spray drying by Abdelrady, et.al. (4), Optimized gefitinib loaded glucosamine targeted SLN Nazafarin Satari,et.al. (5), Spray-dried EMB-loaded SLN microparticles and Spray-dried EMB-loaded SLN microparticles containing mannitol by Nemati, E et.al. (6), Spray dried meloxicam sample with different concentration of leucine by Party, P et.al. (7), Commercial form of chloramphenicol by Nurbaeti, S.N, et.al (8), Microparticles of chloramphenicol by Nurbaeti, S.N, et.al (9), Nanoaggregate of ciprofloxacin by Sabuj, M.Z.R, et. al. (10), Docetaxel nanoformulation by Chishti, N et.al. (11), Spray-dried PTX-loaded micelles aggregates by Rezazadeh, M., et al. (12), Uncoated NAC-PLGA-MPPS and Coated NAC-PLGA-MPPs conducted by vishal puri, et.al. (13).
The entrapment efficiency of the drug formulation was adequate. It was 14%–34% (m/m). The free-flow properties of particulates were determined with a Next-Generation Impactor that was suitable for the distribution of drugs to the lungs. Formulation had mass median aerodynamic diameters close to 3 μm. The ex vivo release study confirmed that the formulation had prolonged release properties. It was concluded that complete release of formulation in the lungs can occur within 14 days (Nurbaeti et al., 2019).
4.7 Preparation and development of ciprofloxacin-filled polymeric nano-micelles for dry powder inhalation via spray drying
Ciprofloxacin belongs to a class of drugs called quinolone antibiotics. It is a wide-spectrum antibiotic that is commonly used as a therapy for pulmonary inflammation (Sabuj et al., 2021).
Ciprofloxacin was carried in nanocarriers, such as nanomicelles. Those nanocarriers were used to enhance the lodging and retention time of drugs in the lungs. That type of formulation had elevated ciprofloxacin antibacterial effects.
The drug was designed in nanoaggregate form by using nanomicelles. Optimum formulations were prepared that were dried using the spray drying technique. A dry powder nanoaggregate inhalation formulation was prepared to deliver the drug to the pulmonary system. That formulation was converted to dry powder inhalers (DPIs) due to its effective delivery to the lungs (Sabuj et al., 2021).
Optimum formulations were prepared by using a two-level full factorial design, and sixteen formulations were prepared. Two independent variables, i.e., 1. the outcome of carrier kind and anti-sticking agent type; 2. the carrier-to-nano particulate ratio and the anti-sticking agent-to-carrier ratio of microsize particulates; and two independent variables, i.e., formulation, ex vivo pulmonary distribution, and redispersibility, were selected. Ciprofloxacin nano-micelles-based dry formulation was tested for its anti-biotic results against Pseudomonas aeruginosa, Klebsiella pneumoniae, and Streptococcus pneumoniae.
The optimal formulation was obtained by using a two-factorial interchanging framework. The appropriate nanocomposite formulations were processed by adding mannitol and L-phenylalanine with a D0.5 of 1.7 µm and 60% fine particles. Ciprofloxacin nano-micelles dry powder inhalation preparation were processed by spray drying technique (Sabuj et al., 2021). Analysis of formulations showed that the whole operation had no ineffective results on the consistency of the drug. Also, there was no impact on the drug release profile of the nano-micelles. Using nano-micelles-based nano-particle-based dry formulations enhanced the anti-microbial effects of ciprofloxacin against micro-organisms. That dry powder inhalation formulation was tested and confirmed the increased usefulness of ciprofloxacin in treating pulmonary infections. (Sabuj et al., 2021).
4.8 Composition, development, and in vivo assessment of a nanoparticulate-based docetaxel formulation for lung cancer treatment
Cancer is a death-causing disease. Among many forms of cancer, lung cancer has a high mortality rate. It is more prevalent in many countries. This disease can be managed by the long-term use of chemotherapeutic drugs. One of the new approaches is to encapsulate the drug in nanoparticles and form a dry, inhalable formulation (Chishti et al., 2019).
According to that, the main target of the researchers is to manage lung cancer. Research was performed to characterize the docetaxel inhalable nanoparticulates for effective use in massive lung cancer. This form of treatment was found effective for the long-term delivery of drugs.
Therapeutic medications can be delivered specifically to polymeric nanoparticles because of their facile morphological modification. Biocompatible and biodegradable are polymeric nanoparticles. These particles have been modified to increase their effectiveness for targeted therapy while minimizing their harmful consequences. It is discovered that the dispersion and deposition in the lungs are adequate.(Kwok et al., 2011; Chow et al., 2019).
Docetaxel nanoparticles were prepared by poloxamer (PLX-188) and poly (lactic-co-glycolic acid) (PLGA). The particles were coated with PLGA. Preparation was continued by an easy method, i.e., the oil in water (o/w) single emulsification-solvent vaporization process.
The formulation was centrifuged after preparation to get pellets of nanoparticles of docetaxel. Those pellets were then spread in an osmitrol solution and freeze-dried to get the dry form of nanoparticles of docetaxel (DTX-NPs) (Chishti et al., 2019).
The optimized formulation of docetaxel nanoparticulates showed flat and round particles structure-wise. Particles had a size of around 200 nm, a zeta potential of around −36 mV, and a drug-enclosing capability of around 60%. DTX-NPs were analyzed for in vitro cytotoxic effects. A nanoparticle-based formulation of d9(ocetaxel revealed increased cytotoxicity up to 48 h in contrast to the free form of the drug.
Optimum formulations were studied for ex vivo drug analysis. Results showed that free drug release was slow but continuous up to 96 h. The Higuchi release kinetics model was applied for ex vivo drug release. A stability test was also conducted for DTX-NPs, which confirmed that DTX-NPs had maintained their desired characteristics in the accelerated stability condition (Chishti et al., 2019).
The in vivo analysis report exhibited that the optimized preparation of docetaxel nanoparticles based on dry powder inhalation formulation was well kept inside the lungs for a longer duration of time. At the end, it was decided that a new approach for direct delivery of the drug to the pulmonary system could open the way for other drugs to be used by the pulmonary route. Docetaxel nanoparticles were found to be least invasive to normal cells if they were used as targeted area delivery treatments in cases of massively spreading lung cancer, i.e., NSCLC (Chishti et al., 2019).
4.9 Production and assessment of spray-dried inhalable paclitaxel-loaded polymeric micelles for lung cancer treatment
Direct transfer of anti-cancer drugs to the lungs is a local-type drug delivery system. This local drug administration has numerous benefits for patients. Local treatment for lung cancer is more effective than conventional systemic treatment (Rezazadeh et al., 2018).
A research study was conducted to form blended polymeric micelles that depend on tocopheryl succinate-polyethylene glycol 1,000 and 5,000 Da (TPGS1K and TPGS5K) and are filled with paclitaxel (PTX).
For drug delivery, polymeric micelles offer a number of benefits, such as enhanced solubility, regulated release, and elevated bioavailability. They can shield highly concentrated medications from the harsh environment of the digestive system, improving absorption. Moreover, polymeric micelles are easily sterilizable and stable. They may also be adapted to certain targeting scenarios and have a large medication loading capacity (Larhrib et al., 1999).
After preparation, the designed aggregates were added in scattered form to create a new system of drug distribution. Lactose is added as a carrier material in the drying process, i.e., the spray drying procedure. The micelles of various molar ratios (10:0, 7:3, 5:5, 3:7, 0:10) were designed using TPGS5K and TPGS1K. All prepared formulations were tested for physicochemical properties, i.e., particle size, zeta potential, critical micelle concentration (CMC), drug loading drug release rate, and ex vivo cytotoxicity.
Paclitaxel-prepared nanoparticles were sprayed with lactose carriers and then dried. That technique produced the spherical-shaped particles of the inhalable formulation (Rezazadeh et al., 2018).
Analysis of nanoparticles that were present in different formulations confirmed the particle sizes and zeta potential values. The particle size was in the range of 102–196 nm, and the zeta potential was found to be −9.4 to −13.8 mV, particularly.
Another test concluded that critical micelle concentrations were confirmed up to 5:5 and 7:3 in combinations (16.33 and 17.89 µM, particularly). It was also established that the delivery assessment of various formulations was very slow, up to 30%, but it was released after 72 h.
Paclitaxel-loaded mixed micelles formulation was found to be more toxic to cancer cells after conducting cytotoxicity assays as compared to the free drug. The ex vivo distribution study showed that spray drying of PTX-loaded micelles with lactose formed suitable inhalable powders that had a high fine particle fraction (60%) (Rezazadeh et al., 2018). All tests had described that novel paclitaxel-filled loosely bounded aggregates were dried to be taken easily by inhalation. That form could be a good alternative treatment for lung cancer (Rezazadeh et al., 2018).
4.10 Inhalation capability of an N-acetylcysteine-blended PLGA nanoparticulate dry powder formulation for tuberculosis management
Mycobacterium tuberculosis (TB) is also a fatal ailment that causes deaths among people. It is generated by the bacillus M. tuberculosis (MTB). MTB spreads this infection by forming soft tissue granuloma inside the lungs (Puri et al., 2022).
Many research studies have expressed that sticky fluid in the respiratory system is a serious obstacle to the transfer of drugs to the mucus membrane. In order to overcome that issue, polymeric nanoparticles of the drug were designed to control the release and transfer of the drug to the inside cells of the lungs. Drugs encapsulated in polymeric nanoparticles can be delivered at high concentrations inside the macrophages.
Polymeric nanoparticles are targeted delivery of therapeutic drugs as they are easy amendable their morphology can be easily altered. Polymeric nanoparticles are biodegradable and biocompatible. Some modification makes these particles more effective for targeted therapy with least toxic effects. For lungs there deposition and distribution is found satisfactory (Kwok et al., 2011; Chow et al., 2019).
N-acetylcysteine (NAC) is an antioxidant that stimulates the glutathione level, acts as a free radical, a decaying agent, has mucokinetics, is anti-inflammatory, and is an is an anti-mycobacterial agent. It works by increasing interleukins and interferon-gamma (INF-γ) creation.
Research was conducted to produce respirable N-acetylcysteine (NAC) designed with PLGA that can penetrate fluid in the lungs for maximum outcomes.
Formulations were prepared using the double-emulsion method (w/o/w). After that, an equal volume of 2% v/v of Pluronic F127 was mixed into the preparation. Then the resultant formulation was stirred for 4 h in order to entirely vaporize the carbon-based solvent from the distributed phase under decreased pressure.
Finally, the emulsion was lyophilized at −30°C for 24 h before being freeze-dried at 40°C for 48 h (Puri et al., 2022).
That type of formulation had targeted drug delivery properties to alveolar macrophages. Also, nanoparticle-based formulations had many benefits, i.e., causing minimal dose-related side effects. That formulation had incorporated hydrophilic drugs properly and had a sustained release rate. So, it was confirmed that the new approach of N-acetylcysteine had prolonged retention time in treating tuberculosis.
The optimal formulation was selected among many formulations. That optimized formulation contained a drug/polymer ratio of 1:10 with a 0.50% PVA concentration and a sonication time of 2 min (Puri et al., 2022).
Analysis of the preparation revealed the mean particle size of nanoparticles was 307.50 ± 9.54 nm, the polydispersity index (PDI) was 0.136 ± 0.02, and the zeta potential was about −11.3 ± 0.4 mV. Also, it had enclosing efficiency (55.46% ± 2.40%) and drug filling capability (9.05% ± 0.22%), along with high flow properties.
Other analyses in the form of FTIR revealed that N-acetylcysteine and PLGA were appropriate for each other. Scanning electron micrographs established that the nanoparticulates of the drug were round in shape with a little bit of an uneven surface. Transmission electron micrograph assessment confirmed nanoparticulate size in nanometers and the covering of lipid over the nanoparticulate surface (Chaudhary et al., 2022) (Figure 3).
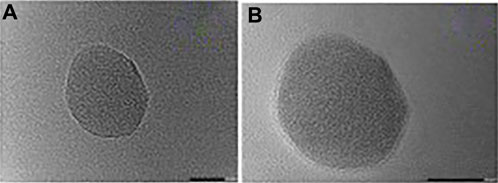
Figure 3. Transmission electron microphotograph (TEM) of (A) Uncoated NAC-PLGA-MPPS and (B) Coated NAC-PLGA-MPPs conducted by vishal puri, et.al.
The PXRD spectrum disclosed the conversion of the drug from crystal form to amorphous form in preparation. Ex vivo liberation model results were found to be biphasic. At the first stage, it started exploding liberation (64.67% ± 1.53% within 12 h), followed by continuous release for 48 h. That showed that NAC nanoparticles had prolonged releasing properties.
Ex vivo pulmonary distribution investigation exhibited that surface-covered N-acetylcysteine-loaded PLGA mucus penetrating polymer nanoparticles (NAC-PLGA-MPPs) had beneficial outcomes in the form of emitted dose (86.67 ± 2.52%), with MMAD value (2.57 ± 0.12 μm), GSD value (1.55 ± 0.11 μm), and FPF value of 62.67 ± 2.08% for the distribution and focusing the lungs.
In a nutshell, ex vivo useful results revealed that NAC-PLGA-MPPs showed improved anti-microbial action averse to MTB H37Rv type in contrast to conventional N-acetylcysteine. Therefore, PLGA-bounded particulates could be a good option to transfer N-acetylcysteine for treating pulmonary diseases (Puri et al., 2022).
5 Characterization of inhalable nanoparticles
Dry powder inhalation formulations are characterized and evaluated using different techniques. The characterization of DPIs can determine their therapeutic potential (Malamataris et al., 2020).
Following are some techniques by which different formulations of dry powders can be characterized effectively.
5.1 Determination of particle size
The size of the nanoparticulates was determined by photon correlation spectroscopy by Zetasizer. The mean particle size and polydispersity index can be determined by Zetasizer (Zhong, 2018). The Zetasizer test has several advantages and disadvantages. It is given in Table 2. It is frequently used to determine particle size and zeta potential.
5.2 Calculation of the mass median aerodynamic diameter of nanoparticles
The mass median aerodynamic diameter (MMAD) of the fine grain was calculated using a Mark II Anderson Cascade. This test is done to learn about particle deposition in the airways (Faria-Urbina et al., 2021) Based on the aerodynamic behavior and mass distribution of the particles, the MMAD represents the median diameter of the particles. The MMAD test has the following advantages and disadvantages that are given in Table 3.
5.3 Particle morphology by scanning electron microscopy
Different shapes of nanoparticles are studied by Scanning electron microscopy. Scanning electron microscopy (SEM) is a powerful tool used in materials science, biology, and nanotechnology for the examination of particle morphology. The following are the main advantages and difficulties of particle morphology analysis using SEM (Table 4): Gradon and Sosnowski (2014):
5.4 Particle physico-chemical characterization
A crystal phase transition study is conducted using differential scanning calorimetry (DSC) and X-ray diffraction (XRD) (Figures 4, 5). The compatibility study of drug particles with other excipients is done by Fourier transform infrared spectroscopy (Figure 6). (Gradon and Sosnowski, 2014). Advantages and disadvantages of DSC, XRD and FTIR are given in Tables 5, 6.
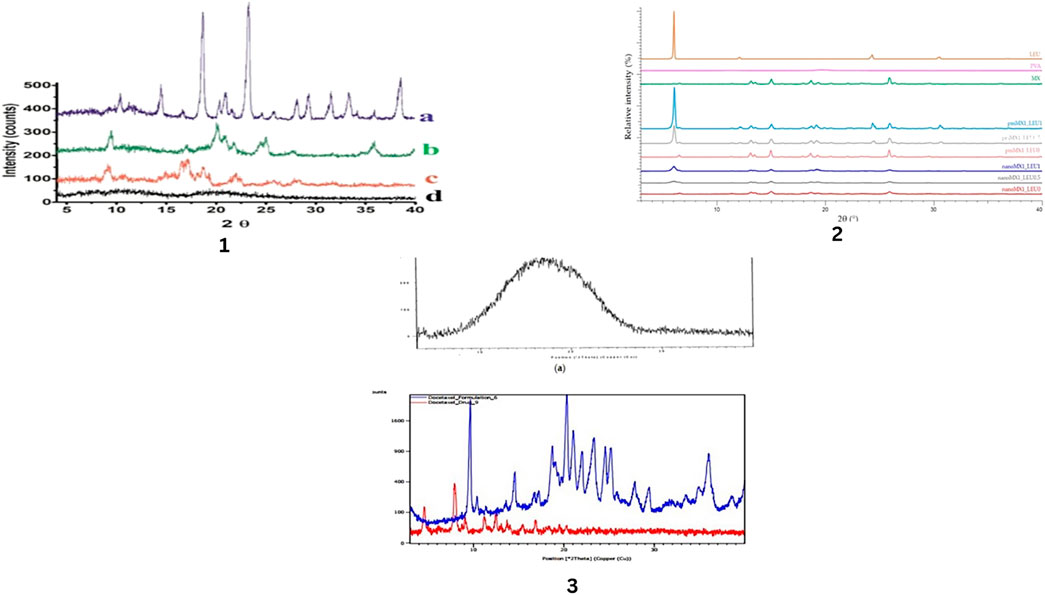
Figure 4. X-ray Diffraction pattern of different chemicals and drugs. Mannitol (a), the spray dried optimized nano simvastatin (formulation 13, (b), raw simvastatin (c), and lecithin (d) by Kononenko, V et.al (1), The raw materials (L-leucine (LEU), poly-vinyl-alcohol (PVA), and meloxicam (MX)), the physical mixtures (pmMX1_LEU0, pmMX1_LEU0.5, and pmMX1_LEU1), and the spray-dried es (nanoMX1_LEU0, nanoMX1_LEU0.5, and nanoMX1_LEU1) by Party, P et.al. (2), (a) PLGA, (b) docetaxel and DTX-NPs by Chishti, N et.al. (3).
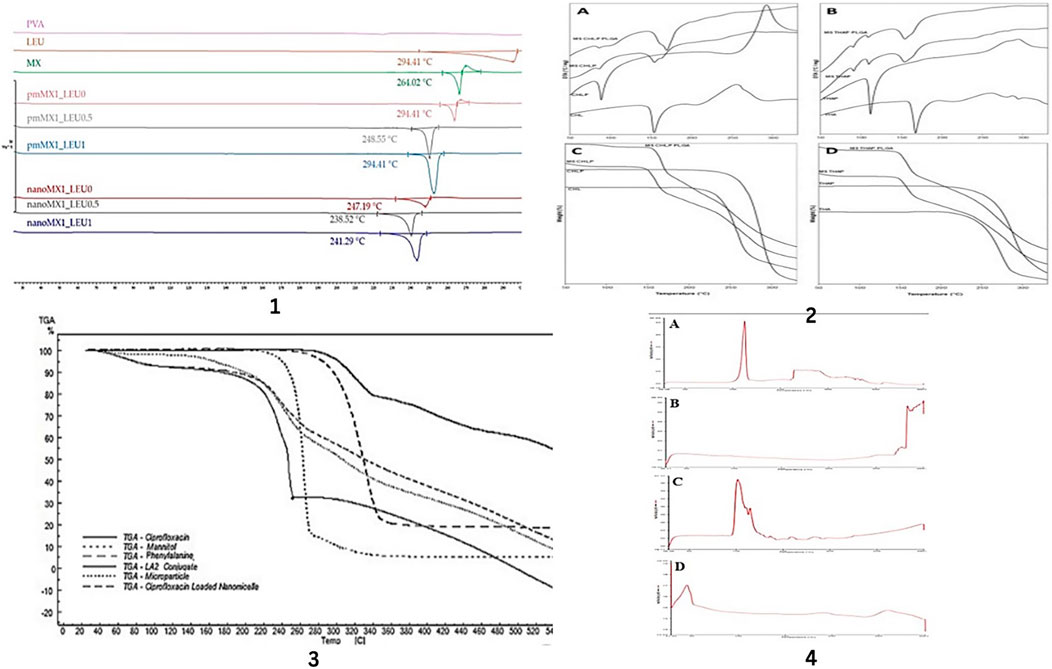
Figure 5. DSC and TGA analysis of drugs and excipients used in formulations. DSC results of the raw materials, (PVA, LEU, and MX), the physical mixtures (pmMX1_LEU0, pmMX1_LEU0.5 and pmMX1_LEU1), and the spray-dried samples (nanoMX1_LEU0, nanoMX1_LEU0.5, and nanoMX1_LEU1) by Party, P et.al. (1), DTA (A,B) and TGA (C,D) thermograms of raw materials (CHL, CHLP, THA and THAP) and of dry microparticle powders made with CHLP or THAP nanoparticles (MS CHLP or MS THAP) or with CHLP- or THAP-loaded PLGA nanoparticles (MS PLGA CHLP or MS PLGA THAP). Total weight loss (%) is indicated on TGA curves by Nurbaeti, S.N, et.al. (2), TGA thermograms of ciprofloxacin, LA2 conjugate, ciprofloxacin loaded nanomicelles, mannitol, phenylalanine and optimum nanoaggregates by Sabuj, M.Z.R, et. al. (3), DSC of (A) NAC (B) PLGA (C) NAC-PLGA-F127 and (D) NAC-PLGA-MPPs conducted by vishal puri, et.al. (4).
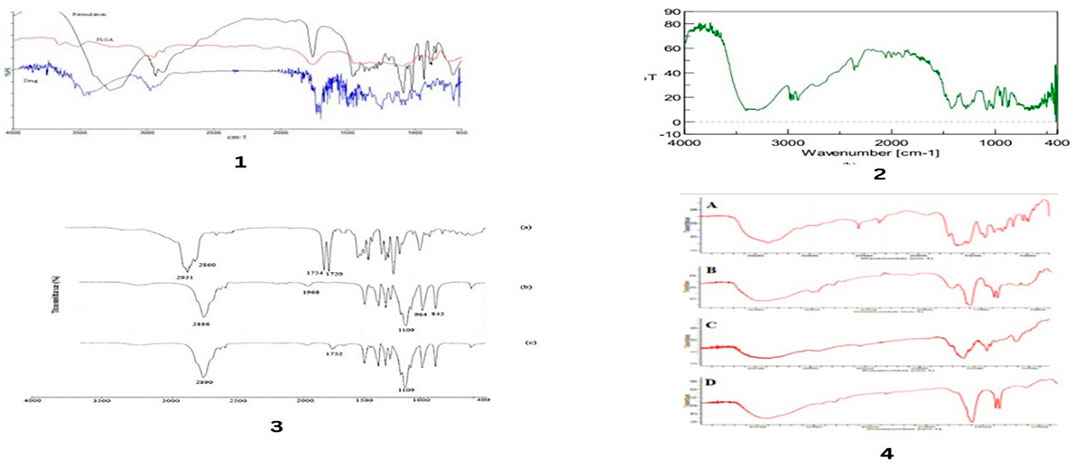
Figure 6. FTIR spectra of drug and different excipients used in formulations. FTIR spectra for, polymer and docetaxel drug by Chishti, N et.al (1), FTIR spectra for mannitol by Chishti, N et.al. (2), FT-IR spectra of (a) α-TS, (b) MePEG, and (c) TPGS5K by Rezazadeh, M., et al. (3), Fourier transform infrared (FTIR) spectrum of (A) pure NAC (B) PLGA (C) physical mixture of NAC-PLGA-F127 and (D) NAC-PLGA-MPPs conducted by vishal puri, et.al. (4).
5.5 Particle tap density and flowability
Tapped density of powder formulation can be performed by using a volumetric and graduated cylinder. By this method, the Carr index and angle of repose can be determined (D’Addio et al., 2013).
The density of a powder after it has been compacted—typically by tapping or shaking the container holding it—is referred to as tap density. This characteristic affects material handling, processing, and product quality, making it essential in a variety of sectors, such as pharmaceuticals, metallurgy, and ceramics.
Advantages and disadvantages of tapped density and flowability are given in Table 7.
5.6 Electrostatic charge of nanoparticles
An electronometer is used to study the electric charge on nanoparticles. That charge should be the minimum in order to make a better correlation between drug particles and airflow rate (Abdelaziz et al., 2022).
An extremely sensitive tool for determining electrical potential differences or electric charge is an electrometer. Applications in physics and engineering frequently employ it, especially in areas like radiation detection, semiconductor testing, and electromagnetic. The following are some advantages and difficulties of using an electrometer test. These are given in Table 8.
5.7 Drug loading analysis
HPLC, LCMS, and MS are usually used to study the loading efficiency of the drug by nanoparticle. This study is important to design flexible doses in order to reduce dose size and dose frequency (El Baihary et al., 2019). Highly effective analytical methods for determining loading efficiency are mass spectrometry (MS), liquid chromatography-mass spectrometry (LC-MS), and high-performance liquid chromatography (HPLC). These methods are widely applied in a variety of fields, most notably materials science and medicines. The following lists the advantages and difficulties of each approach: (El Baihary et al., 2019). These are given Tables 9, 10.
5.8 Thermal gravimetric analysis (TGA)
This analysis is applied to evaluate the residual solvent content for product quality and safety (Nozohouri et al., 2019).
It is a potent analytical method for determining how a sample’s mass varies as it is heated, cooled, or kept at a constant temperature in a controlled environment is thermal gravimetric analysis (TGA). This method has several advantages but also has some drawbacks. These are given in Table 11.
5.9 Dynamic vapor sorption (DVS)
Another test to study the hygroscopicity of the formulation is done by dynamic vapor sorption. Hygroscopicity should be minimized for the desired formulation design (Yiu et al., 2021).
The quantity of vapor (often water vapor) that a material absorbs or desorbs as a function of relative humidity (RH) at a fixed temperature is measured using the analytical method known as dynamic vapor sorption (DVS). Among other things, this method is useful for comprehending the characteristics of materials’ interactions with moisture. These are some advantages and difficulties related to DVS. (Yiu et al., 2021). These are given in Table 12.
5.10 Stability analysis
A stability chamber is used to study the stability of formulations. This study is done according to ICH and local regulatory guidelines (Craparo et al., 2022).
5.11 Re-dissolution and stability of redispersed primary nanoparticulates
The redispersibility index (RdI) is applied as an uncomplicated measurement to assess powder redispersibility in water or liquid. This is performed by a sonicator, hand-shaking, etc. But, for study, real re-distribution of powder in the human body as nanoflecks come into contact with pleural fluid in the absence of mechanical disturbance (Radivojev et al., 2021). It is suggested to acquire an instant aqueous re-dissolution process, i.e., mix the powder with the mixing liquid and allow the suspension to settle down until no distinct particulates are noticed before doing the process sizing. Also, there is a remarkable difference in mixing liquids that are applied for the formation of solutions or suspensions. At the same time, mixing and constitution in pure water are almost uncomplicated to evaluate redispersibility and have been acquired by various researchers (Stocke et al., 2015; Weng et al., 2020b).
5.12 Ex vivo nanoparticle drug release study
Ex vivo nanoparticulate-based drug discharge comprises uninterrupted flow, dialysis membrane flow study, and sample and separate technique. Among these techniques, uninterrupted flow and the dialysis membrane process are almost always applied in research on respirable nanoparticulate grounded fine grains (Figure 7). (Price et al., 2017).
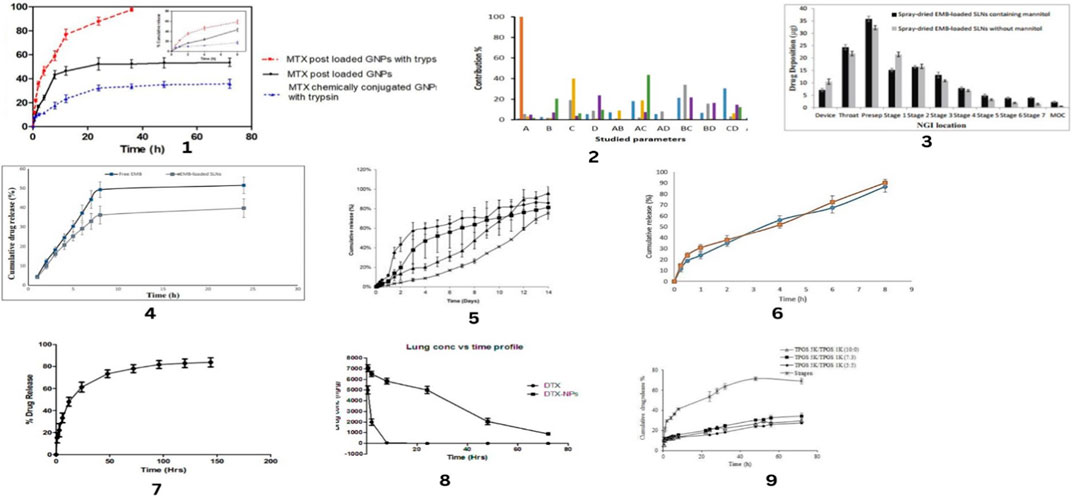
Figure 7. In-vitro release profiles of drug along with Contribution percent of different studied parameters and Drug deposition pattern study. In-vitro release profiles for MTX post loaded NPs and MTX chemically conjugated NPs in absence and presence of trypsin in phosphate buffer pH 7.4 by Abdelrady, et.al (1), Contribution percent of different studied parameters and their interactions on physicochemical characteristics of gefitinib loaded glucosamine targeted SLNs by Nazafarin Satari, et.al. (2),Drug deposition pattern study of spray-dried EMB-loaded SLNs with and without mannitol in the Next Generation Impactor by Nemati, E et.al. (3), Drug release profile of EMB from EMB-loaded SLNs and free EMB in 24 h by Nemati, E et.al. (4), In vitro prodrug release profiles in HBSS medium, 37°C, for dry microparticle powders made with CHLP nanoparticles (×), with THAP nanoparticles (), with CHLP-loaded PLGA nanoparticles () or with THAP-loaded PLGA nanoparticles by Nurbaeti, S.N, et.al. (5), Release profiles of ciprofloxacin from optimum microparticles and freshly prepared nanomicelles in PBS medium at 37℃ (n = 3, mean ± SD) by Sabuj, M.Z.R, et. al. (6), In vitro drug release test profile of docetaxel from DTX-NPs by Chishti, N et.al.(7), In vivo lung retention study performed in rats for free drug and DTX-NPs by Chishti, N et.al. (8), In vitro drug-release profiles from Stragen® and the mixed micelles with different molar ratios of the structural composition. Data represent the mean ± standard deviation (n = 3) by Rezazadeh, M., et al. (9).
5.13 Ex-vivo atomizer action
The ex vivo action of an atomizer composed of nanoparticulate-contained dry fines is crucial for anticipating their lung distribution capability after inhalation. Basic guidelines are necessary to be investigated (Ungaro et al., 2012). These guidelines are, i.e., the fine particle fraction (FPF), which is usually described as a fraction of particles with dA ≤ 5 μm, the mass median aerodynamic diameter (MMAD), and the geometric standard deviation (GSD) (Talegaonkar et al., 2012). Many types of pharmacopoeial equipment are present for this objective. These pieces of equipment are, i.e., multi-stage liquid impingers (MSLI), Andersen cascade impactors (ACI), Next-Generation Impactors (NGI), etc., (Dal Negro, 2015; Haynes et al., 2016; Clark et al., 2020; Faria-Urbina et al., 2021). These are some advantages and disadvantages of atomizer. These are given in Table 13.
6 Challenges in nanoparticle-based dry powder inhalation formulation
There are some challenges due to which nanoparticle-based dry powder inhalation formulations are not gaining popularity like other pharmaceutical products. (Sung et al., 2009; Foulkes et al., 2020). These obstacles are following;
1. Lack of adaptation at the clinical level
2. Not manufacturing on a large scale
3. Deficiency in designing optimized formulations
4. Causing toxicity in the pulmonary system
5. Limited clinical trials
6.1 Lack of adaptation at the clinical level
One of the challenges in the development of nanoparticles is the adaptation of that formulation at the clinical level. Inspiring nanoparticulate-based dry nanofleck formulation has a main problem, which is its path of application, i.e., oral inhalation.
The main issue with its adaptation is the knowledge gap and research direction on this site. If that issue is addressed, then regulatory guidance can suggest applying this formulation at the commercial level (Feng et al., 2019).
6.2 Not manufacturing at a large level
Another challenge for the common use of nanomedicines is their production on a large scale. Manufacturing on a large scale is very complex, mostly for inhalable nanoparticle-based dry powder formulation. The hardest step in accomplishment is the fabrication of nano-suspension and then drying. Variation in particle sizes can arise in these two steps while processing batches of nanoparticle-based inhalation formulations (Tomoda et al., 2008; McNeil, 2009).
6.3 Deficiency in designing optimized formulations
Furthermore, nanoparticles-based dry powder inhalation formulations require optimum properties regarding size of particles, external charge and shape of flecks. These properties are important in terms of redispersibility and in vitro aerosols performance. Moreover, these physiochemical properties of nanoparticle-based dry powder formulations are important for enhanced therapeutic effects. Therefore, designing an optimized formulation, it is required to indicate an association between nanoparticulates physical and chemical properties i.e., individual and associative outcomes with the future repercussion on patients. For that purpose, an optimum nanoparticle size and surface charge, along with shapes, are necessary to consider for appropriate designing of formulation (Ensign et al., 2012; Valsalakumari et al., 2021; Weng et al., 2022).
6.4 Causing toxicity in the pulmonary system
Additional difficulty in designing the nanoparticle-based dry powder inhalation formulation is dealing with the toxicity that can occur if very fine inorganic particulates, i.e., titanium dioxide and gold nanoparticles, are used in the formulation.
Ultrafine inorganic nanoparticles have variable physicochemical properties that can create toxicity if inhaled (Ferreira et al., 2013).
6.5 Limited clinical trials
Research regarding the in vivo study of nanoparticle-based dry powder inhalation formulations has been based on predictions due to restricted clinical tests that have been performed up to date.
The big hurdle is the dearth of ex vivo and clinical facts and figures that can establish a powerful ex vivo-in vivo correlation between nanoparticle-based dry powder inhalation formulations. Although some current data is available that can make a better relationship between ex vivo falling drug testing impactor and clinical pulmonary distribution data, due to the unavailability of developed preclinical models that can precisely display the future of nano particulate-based dried flecks after oral inspiration, i.e., distribution of dried flecks inside the pulmonary system, redistribution of nano particulates in pulmonary fluid, and subsequent assimilation and removal of nano particulates from the respiratory system. (Ahmad et al., 2009; Lim et al., 2021; Tai et al., 2021; Zhu et al., 2021).
7 Exploration of marketed products
Investigating the market for dry powder formulations based on inhalable nanoparticles entails looking at commercial goods, patents, and clinical studies. Below is a summary of every category:
7.1 Marketed products
1. PulmoSphere™ Technology:
• Developed by Novartis.
• Used in products like TOBI® Podhaler® (tobramycin inhalation powder) for cystic fibrosis.
• Involves liposomal encapsulation and spray-drying to create porous particles.
2. Arikayce® (amikacin liposome inhalation suspension):
• Approved for the treatment of Mycobacterium avium complex (MAC) lung disease.
• Uses liposomal delivery to target the lungs.
3. Dry Powder Inhalers (DPIs):
• Products like Advair Diskus® and Spiriva® use dry powder formulations, though not necessarily nanoparticles, they are relevant in the context of pulmonary drug delivery (Kumbhar et al., 2022).
• Utilize carrier particles to aid in the dispersion of the drug.
7.2 Patents
1. WO2020031767A1 - Dry Powder Inhalation Compositions:
• Covers formulations of nanoparticles for pulmonary delivery.
• Focus on enhancing stability and dispersion of nanoparticles.
2. US20180028735A1 - Nanoparticles for Pulmonary Delivery:
• Describes methods for preparing nanoparticles for inhalation.
• Emphasizes on particle size control and surface properties to enhance delivery efficiency.
3. EP2718224B1 - Inhalable Nanoparticles for Treating Pulmonary Disorders:
• Involves the use of biodegradable polymers to create nanoparticles for the treatment of lung diseases.
• Discusses methods to improve bioavailability and reduce systemic side effects (Kumbhar et al., 2022).
7.3 Clinical trails
1. NCT03252807 - A Study of Inhaled Dry Powder of Lopinavir/Ritonavir for the Treatment of COVID-19:
• Investigates the use of dry powder formulations of antiviral drugs.
• Explores the efficacy and safety of nanoparticle-based inhalable drugs in treating respiratory infections.
2. NCT04589931 - Inhaled Dry Powder Amikacin for Non-Cystic Fibrosis Bronchiectasis:
• Focuses on the use of inhalable amikacin dry powder.
• Evaluates the pharmacokinetics and therapeutic outcomes in patients with chronic lung conditions.
3. NCT04193878 - Study of Inhaled Ciprofloxacin Dry Powder for Chronic Lung Infections:
• Examines the use of ciprofloxacin in nanoparticle dry powder form.
• Aims to enhance the drug delivery to the lungs and improve patient outcomes (Kumbhar et al., 2022).
Research on inhalable nanoparticle-based dry powder formulations is constantly progressing, with the goal of enhancing drug delivery effectiveness, patient adherence, and therapy results for a range of pulmonary ailments.
8 Future prospects of dry powder nanoparticles
With the goal of improving medication delivery, effectiveness, and patient outcomes, cutting-edge technology and novel methodologies are driving tremendous progress in the field of inhalable dry powder nano-formulations. This is a thorough assessment of the opportunities, possible developments, new technology, and expected difficulties for the future:
8.1 Potential advancement
8.1.1 Improved drug delivery system
Targeted delivery refers to the creation of nanoparticles that can precisely target sick lung tissues or cells in order to maximize therapeutic benefits and reduce adverse effects.
8.1.2 Controlled release
Preparations that provide a medication’s release over time, extending its therapeutic benefits and lowering the frequency of doses.
8.2 Personalized medicine
8.2.1 Customized formulation
Developing inhalable formulations according to the unique characteristics of each patient, such as genetic, phenotypic, and illness-specific variables.
8.2.2 Smart inhalers
Combining digital health technology with sensors to track inhaler usage and improve dosage schedules (Kumbhar et al., 2022).
8.3 Biocompatible and biodegradable materials
8.3.1 Advanced polymers
Increasing safety and lowering possible toxicity by using biocompatible and biodegradable polymers in the production of nanoparticles.
8.3.2 Natural biomaterials
Investigating the use of natural substances such as alginate and chitosan to produce safe and efficient inhalable nanoparticles.
8.4 Emerging technologies
8.4.1 Advanced manufacturing and nanotechnology
Nano-engineering: The precise manipulation of nanoparticles to regulate their dimensions, form, surface characteristics, and ability to load drugs.
3D Printing: Complex and personalized dry powder inhalers and formulations may be made by the use of 3D printing technology.
8.4.2 Lipid-based and liposomal formulations
Lipid nanoparticles: Solid lipid nanoparticles and liposomes are being developed for the effective encapsulation and distribution of both hydrophilic and hydrophobic medications.
Advanced Lipid Carriers: Advances in lipid carriers help increase the bioavailability and stability of medications breathed in. (Kumbhar et al., 2022).
8.4.3 Microfluidics and nano fluidics
Accurate Formulation: Nano fluidic and microfluidic technology for scalable nanoparticle manufacturing and accurate formulation.
On-Demand Production: Possibility of producing inhalable formulations on demand that are customized to meet specific requirements.
8.5 Anticipated challenges
8.5.1 Regulatory obstacles
Robust Approval Procedures: Handling the intricate regulatory environment for inhalable medications based on nanoparticles, which necessitates comprehensive safety and effectiveness information.
Standardization: Testing procedures and evaluation standards for inhalable nanoparticle compositions must be standardized.
8.5.2 Production and expandability
Production Consistency: Guaranteeing a constant level of performance and quality in the large-scale production of nanoparticles.
Cost-Effectiveness: To keep treatments inexpensive, cutting-edge technology are balanced with economical production techniques.
8.5.3 Patient instruction and compliance
Assuring patients can appropriately utilize sophisticated inhalers to ensure optimal medicine delivery is part of the inhaler technique (Kumbhar et al., 2022). Raising patient and healthcare professional awareness of the advantages and appropriate usage of novel inhalable formulations.
8.5.4 Sturdiness and storage
Shelf Life: Creating formulations that are stable and have an appropriate shelf life for a range of storage environments.
Environmental Factors: Making certain that formulations are stable and functional in various environmental settings. (Kumbhar et al., 2022).
8.6 Future outlook
8.6.1 Combining digital health with integration
Smart Monitoring: Personalized feedback and real-time monitoring are provided by combining inhalable compositions with digital health instruments.
Data-Driven Insights: Using information from smart inhalers to learn more about patient compliance and the effectiveness of medication.
8.6.2 Research and innovation collaboration
Interdisciplinary Collaboration: The joint efforts of technology developers, research institutes, and pharmaceutical corporations to foster innovation.
Collaborations between the public and private sectors: It is to promote the discovery, creation, and marketing of innovative inhaled nano-formulations (Kumbhar et al., 2022).
8.6.3 Expanded therapeutic applications
Beyond Respiratory Diseases: Exploration of inhalable nanoparticles for systemic delivery of drugs to treat conditions like diabetes, cancer, and infectious diseases.
Vaccines and Biologics: Development of inhalable vaccines and biologics, leveraging the mucosal immune response for enhanced protection.
With major breakthroughs and developments in the works, the future of inhalable dry powder nano-formulations seems bright. Although there are obstacles to be addressed, the sector will probably advance thanks to the incorporation of cutting-edge technology, customized medical strategies, and multidisciplinary cooperation, providing patients all over the world with new and enhanced treatment alternatives (Khan and Schneider, 2014; Shafique et al., 2017; Rehman et al., 2018; Kumbhar et al., 2022).
9 Conclusion
The development of nanoparticle-based dry powder formulations has taken a new position in the field of operational research. Research on inhalable nanoparticles in the solid form as powders for targeted pulmonary administration is new and offers special benefits. There are restrictions, though. For the creation of safe inhalable dry powder inhalation formulations, the toxicity of nanoparticles (i.e., nanotoxicity), polymers, and other excipients is essential. Recently, research on nasal administration of inhalable nanoparticulate powders has increased.
By using this pathway, many drugs can be protected from enzymatic degradation in the liver after oral administration. This route of administration has many therapeutic benefits. Present-day research has opened the way to successfully develop inhalable nanoparticle-based dry powders for drugs that can maintain their integrity and activity in different ailments.
In many cases, the nano-in microparticle technique is usually applied for maximum activity of inhalable nanoparticle based dry drug formulation. Another approach for getting high response of drug is to designed nano-agglomeration. Nano-agglomerates are designed from microparticles for improved effect of the particular drug.
The reason behind new approaches is the emergence different respiratory diseases, i.e., cystic fibrosis, lung cancer, tuberculosis, chronic respiratory conditions, and viral infections like COVID, etc. These problems has stimulated significant attention among researchers to develop new formulations i.e., nanoparticles, nano-aggregates, nano-in microparticles, microparticles and many others.
The design of different form of nano-particles are indispensable for successful delivery of drug to lung diseases. These approaches have important role in promoting new drug delivery system that is based on nanotechnology.
With significant advancements and discoveries underway, inhaled dry powder nano-formulations appear to have a promising future. The industry will likely progress despite the challenges it faces as a result of integrating state-of-the-art technology, personalized treatment plans, and interdisciplinary collaboration, offering patients worldwide access to improved and novel treatment options.
The purpose of this review is to outline the development of a dry powder formulation incorporating inspirable nanoparticulates. Furthermore, to highlight its achievements at the clinical level, if researchers show enough interest in this novel drug delivery system, it will be developed and processed at an advanced level. A great deal of understanding and worry about the relationship between inspired nanoparticulates’ physicochemical properties, ex vivo findings, and clinical outcomes might lead to an increase in their industrial manufacture.
Author contributions
FN: Investigation, Writing–original draft. YS: Supervision, Writing–review and editing. MR: Writing–review and editing. PC: Funding acquisition, Writing–review and editing. AN: Funding acquisition, Writing–review and editing. JK: Investigation, Writing–review and editing. Abdullah: Conceptualization, Writing–review and editing. MS: Funding acquisition, Investigation, Writing–review and editing. KS: Investigation, Writing–review and editing. BA: Investigation, Writing–review and editing.
Funding
The author(s) declare financial support was received for the research, authorship, and/or publication of this article. The authors are thankful to Higher Education Commission of Pakistan for sponsoring NRPU Projet No.14913.
Acknowledgments
The author would like to thank the Deanship of Scientific Research at Shaqra University for supporting this work.
Conflict of interest
The authors declare that the research was conducted in the absence of any commercial or financial relationships that could be construed as a potential conflict of interest.
Publisher’s note
All claims expressed in this article are solely those of the authors and do not necessarily represent those of their affiliated organizations, or those of the publisher, the editors and the reviewers. Any product that may be evaluated in this article, or claim that may be made by its manufacturer, is not guaranteed or endorsed by the publisher.
References
Abdelaziz, M. M., Hefnawy, A., Anter, A., Abdellatif, M. M., Khalil, M. A., and Khalil, I. A. (2022). Respirable spray dried vancomycin coated magnetic nanoparticles for localized lung delivery. Int. J. Pharm. 611, 121318. doi:10.1016/j.ijpharm.2021.121318
Abdelrady, H., Hathout, R. M., Osman, R., Saleem, I., and Mortada, N. D. (2019). Exploiting gelatin nanocarriers in the pulmonary delivery of methotrexate for lung cancer therapy. Eur. J. Pharm. Sci. 133, 115–126. doi:10.1016/j.ejps.2019.03.016
Ahmad, A. J., Mittal, G., Jain, G. K., Malhotra, G., Khar, R. K., and Bhatnagar, A. (2009). Nano-salbutamol dry powder inhalation: a new approach for treating broncho-constrictive conditions. Eur. J. Pharm. Biopharm. 71 (2), 282–291. doi:10.1016/j.ejpb.2008.09.018
Arpagaus, C., Collenberg, A., Rütti, D., Assadpour, E., and Jafari, S. M. (2018). Nano spray drying for encapsulation of pharmaceuticals. Int. J. Pharm. 546 (1-2), 194–214. doi:10.1016/j.ijpharm.2018.05.037
Chan, H., and Chow, S. (2022). “Development of redispersible remdesivir nanoagglomerate for inhaled therapy: Box-Behnken design, optimization and in vitro aerosol performance,” in CRS 2022 annual meeting.
Chan, H. W., Chow, S., Zhang, X., Zhao, Y., Tong, H. H. Y., and Chow, S. F. (2023). Inhalable nanoparticle-based dry powder formulations for respiratory diseases: challenges and strategies for translational research. AAPS PharmSciTech 24 (4), 98. doi:10.1208/s12249-023-02559-y
Cheow, W. S., Li, S., and Hadinoto, K. (2010). Spray drying formulation of hollow spherical aggregates of silica nanoparticles by experimental design. Chem. Eng. Res. Des. 88 (5-6), 673–685. doi:10.1016/j.cherd.2009.11.012
Cheow, W. S., Ng, M. L. L., Kho, K., and Hadinoto, K. (2011). Spray-freeze-drying production of thermally sensitive polymeric nanoparticle aggregates for inhaled drug delivery: effect of freeze-drying adjuvants. Int. J. Pharm. 404 (1-2), 289–300. doi:10.1016/j.ijpharm.2010.11.021
Chow, A. H., Tong, H. H. Y., Chattopadhyay, P., and Shekunov, B. Y. (2007). Particle engineering for pulmonary drug delivery. Pharm. Res. 24, 411–437. doi:10.1007/s11095-006-9174-3
Chow, S. F., Weng, J., Xuan, B., and Wan, K. Y. (2019). How can the challenges faced by nanoparticle-based pulmonary drug formulations be overcome. Future Sci. 10, 87–89. doi:10.4155/tde-2019-0001
Clark, A. R., Weers, J. G., and Dhand, R. (2020). The confusing world of dry powder inhalers: it is all about inspiratory pressures, not inspiratory flow rates. J. aerosol Med. Pulm. drug Deliv. 33 (1), 1–11. doi:10.1089/jamp.2019.1556
Chishti, N., Jagwani, S., Dhamecha, D., Jalalpure, S., and Dehghan, M. H. (2019). Preparation, optimization, and in vivo evaluation of nanoparticle-based formulation for pulmonary delivery of anticancer drug. Medicina 55 (6), 294. doi:10.3390/medicina55060294
Costa, C., Nobre, B., Matos, A. S., Silva, A. S., Casimiro, T., Corvo, M. L., et al. (2021). Inhalable hydrophilic molecule-loaded liposomal dry powder formulations using supercritical CO2–assisted spray-drying. J. CO2 Util. 53, 101709. doi:10.1016/j.jcou.2021.101709
Craparo, E. F., Drago, S. E., Quaglia, F., Ungaro, F., and Cavallaro, G. (2022). Development of a novel rapamycin loaded nano-into micro-formulation for treatment of lung inflammation. Drug Deliv. Transl. Res. 12 (8), 1859–1872. doi:10.1007/s13346-021-01102-5
DAddio, S. M., and Prud'homme, R. K. (2011). Controlling drug nanoparticle formation by rapid precipitation. Adv. drug Deliv. Rev. 63 (6), 417–426. doi:10.1016/j.addr.2011.04.005
D’Addio, S. M., Chan, J. G. Y., Kwok, P. C. L., Benson, B. R., Prud’homme, R. K., and Chan, H. K. (2013). Aerosol delivery of nanoparticles in uniform mannitol carriers formulated by ultrasonic spray freeze drying. Pharm. Res. 30, 2891–2901. doi:10.1007/s11095-013-1120-6
Dal Negro, R. W. (2015). Dry powder inhalers and the right things to remember: a concept review. Multidiscip. Respir. Med. 10, 13–14. doi:10.1186/s40248-015-0012-5
Dandekar, P., Venkataraman, C., and Mehra, A. (2010). Pulmonary targeting of nanoparticle drug matrices. J. aerosol Med. Pulm. drug Deliv. 23 (6), 343–353. doi:10.1089/jamp.2009.0784
El Baihary, D., Osman, R., Abdel-Bar, H. M., and Sammour, O. A. (2019). Pharmacokinetic/pulmokinetic analysis of optimized lung targeted spray dried ketotifen-dextran core shell nanocomplexes–in-microparticles. Int. J. Biol. Macromol. 139, 678–687. doi:10.1016/j.ijbiomac.2019.08.011
Ensign, L. M., Schneider, C., Suk, J. S., Cone, R., and Hanes, J. (2012). Mucus penetrating nanoparticles: biophysical tool and method of drug and gene delivery. Adv. Mater. 24 (28), 3887–3894. doi:10.1002/adma.201201800
Farhangi, M., Mahboubi, A., Kobarfard, F., Vatanara, A., and Mortazavi, S. A. (2019). Optimization of a dry powder inhaler of ciprofloxacin-loaded polymeric nanomicelles by spray drying process. Pharm. Dev. Technol. 24 (5), 584–592. doi:10.1080/10837450.2018.1545237
Faria-Urbina, K. T., Ung, K. T., Lawler, L., Zisman, L. S., and Waxman, A. B. (2021). Inspiratory flow patterns with dry powder inhalers of low and medium flow resistance in patients with pulmonary arterial hypertension. Pulm. Circ. 11 (2), 20458940211012591–10. doi:10.1177/20458940211012591
Feng, J., Markwalter, C. E., Tian, C., Armstrong, M., and Prud’homme, R. K. (2019). Translational formulation of nanoparticle therapeutics from laboratory discovery to clinical scale. J. Transl. Med. 17 (1), 200–209. doi:10.1186/s12967-019-1945-9
Ferreira, A., Cemlyn-Jones, J., and Cordeiro, C. R. (2013). Nanoparticles, nanotechnology and pulmonary nanotoxicology. Rev. Port. Pneumol. English Ed. 19 (1), 28–37. doi:10.1016/j.rppnen.2013.01.004
Flume, P. A., Amelina, E., Daines, C. L., Charlton, B., Leadbetter, J., Guasconi, A., et al. (2021). Efficacy and safety of inhaled dry-powder mannitol in adults with cystic fibrosis: an international, randomized controlled study. J. Cyst. Fibros. 20 (6), 1003–1009. doi:10.1016/j.jcf.2021.02.011
Foulkes, R., Man, E., Thind, J., Yeung, S., Joy, A., and Hoskins, C. (2020). The regulation of nanomaterials and nanomedicines for clinical application: current and future perspectives. Biomaterials Sci. 8 (17), 4653–4664. doi:10.1039/d0bm00558d
Gradon, L., and Sosnowski, T. R. (2014). Formation of particles for dry powder inhalers. Adv. Powder Technol. 25 (1), 43–55. doi:10.1016/j.apt.2013.09.012
Honmane, S., Hajare, A., More, H., Osmani, R. A. M., and Salunkhe, S. (2019). Lung delivery of nanoliposomal salbutamol sulfate dry powder inhalation for facilitated asthma therapy. J. liposome Res. 29 (4), 332–342. doi:10.1080/08982104.2018.1531022
Haynes, A., Geller, D., Weers, J., Ament, B., Pavkov, R., Malcolmson, R., et al. (2016). Inhalation of tobramycin using simulated cystic fibrosis patient profiles. Pediatr. Pulmonol. 51 (11), 1159–1167. doi:10.1002/ppul.23451
Haworth, C. S., Bilton, D., Chalmers, J. D., Davis, A. M., Froehlich, J., Gonda, I., et al. (2019). Inhaled liposomal ciprofloxacin in patients with non-cystic fibrosis bronchiectasis and chronic lung infection with Pseudomonas aeruginosa (ORBIT-3 and ORBIT-4): two phase 3, randomised controlled trials. lancet Respir. Med. 7 (3), 213–226. doi:10.1016/s2213-2600(18)30427-2
Hu, X., Yang, F., Liao, Y., Li, L., Zhao, G., and Zhang, L. (2020). Docetaxel-loaded cholesterol-PEG co-modified poly (n-butyl) cyanoacrylate nanoparticles for antitumor drug pulmonary delivery: preparation, characterization, and in vivo evaluation. Int. J. Nanomedicine Vol. 15, 5361–5376. doi:10.2147/ijn.s249511
Huang, F., Zhu, Q., Zhou, X., Gou, D., Yu, J., Li, R., et al. (2021). Role of CFD based in silico modelling in establishing an in vitro-in vivo correlation of aerosol deposition in the respiratory tract. Adv. Drug Deliv. Rev. 170, 369–385. doi:10.1016/j.addr.2020.09.007
Jain, G. K., Iqbal, Z., Talegaonkar, S., Pandit, P., Sule, S., et al. (2009). Development and clinical trial of nano-atropine sulfate dry powder inhaler as a novel organophosphorous poisoning antidote. Nanomedicine Nanotechnol. Biol. Med. 5 (1), 55–63. doi:10.1016/j.nano.2008.07.001
Jain, P., Pawar, R., Pandey, R., Madan, J., Pawar, S., Lakshmi, P., et al. (2017). In-vitro in-vivo correlation (IVIVC) in nanomedicine: is protein corona the missing link? Biotechnol. Adv. 35 (7), 889–904. doi:10.1016/j.biotechadv.2017.08.003
Ke, W.-R., Chang, R. Y. K., and Chan, H.-K. (2022). Engineering the right formulation for enhanced drug delivery. Adv. drug Deliv. Rev. 191, 114561. doi:10.1016/j.addr.2022.114561
Khan, O., and Chaudary, N. (2020). The use of amikacin liposome inhalation suspension (Arikayce) in the treatment of refractory nontuberculous mycobacterial lung disease in adults. Drug Des. Dev. Ther. Vol. 14, 2287–2294. doi:10.2147/dddt.s146111
Khan, S. A., and Schneider, M. (2014). Stabilization of gelatin nanoparticles without crosslinking. Macromol. Biosci. 14 (11), 1627–1638. doi:10.1002/mabi.201400214
Kononenko, V., Narat, M., and Drobne, D. (2015). Nanoparticle interaction with the immune system/Interakcije nanodelcev z imunskim sistemom. Archives Industrial Hyg. Toxicol. 66 (2), 97–108. doi:10.1515/aiht-2015-66-2582
Kumbhar, P., Manjappa, A., Shah, R., Jha, N. K., Singh, S. K., Dua, K., et al. (2022). Inhalation delivery of repurposed drugs for lung cancer: approaches, benefits and challenges. J. Control. Release 341, 1–15. doi:10.1016/j.jconrel.2021.11.015
Kwok, P. C. L., Tunsirikongkon, A., Glover, W., and Chan, H. K. (2011). Formation of protein nano-matrix particles with controlled surface architecture for respiratory drug delivery. Pharm. Res. 28, 788–796. doi:10.1007/s11095-010-0332-2
Larhrib, H., Zeng, X. M., Martin, G. P., Marriott, C., and Pritchard, J. (1999). The use of different grades of lactose as a carrier for aerosolised salbutamol sulphate. Int. J. Pharm. 191 (1), 1–14. doi:10.1016/s0378-5173(99)00164-7
Li, Y., Wan, J., Yang, M., Zhu, W. F., Wang, C. H., et al. (2013). Study on formability of solid nanosuspensions during nanodispersion and solidification: I. Novel role of stabilizer/drug property. Int. J. Pharm. 454 (1), 269–277. doi:10.1016/j.ijpharm.2013.06.050
Liang, W., Pan, H. W., Vllasaliu, D., Lam, J. K. W., et al. (2020). Pulmonary delivery of biological drugs. Pharmaceutics 12 (11), 1025. doi:10.3390/pharmaceutics12111025
Liao, Q, Yip, L., Chow, M. Y., Chow, S. F., Chan, H. K., Kwok, P. C., et al. (2019). Porous and highly dispersible voriconazole dry powders produced by spray freeze drying for pulmonary delivery with efficient lung deposition. Int. J. Pharm. 560, 144–154. doi:10.1016/j.ijpharm.2019.01.057
Lim, S. H., Park, S., Lee, C. C., Ho, P. C. L., Kwok, P. C. L., and Kang, L. (2021). A 3D printed human upper respiratory tract model for particulate deposition profiling. Int. J. Pharm. 597, 120307. doi:10.1016/j.ijpharm.2021.120307
Malamataris, M., Charisi, A., Malamataris, S., Kachrimanis, K., and Nikolakakis, I. (2020). Spray drying for the preparation of nanoparticle-based drug formulations as dry powders for inhalation. Processes 8 (7), 788. doi:10.3390/pr8070788
Mangal, S., Gao, W., Li, T., and Zhou, Q. (2017). Pulmonary delivery of nanoparticle chemotherapy for the treatment of lung cancers: challenges and opportunities. Acta Pharmacol. sin. 38 (6), 782–797. doi:10.1038/aps.2017.34
McNeil, S. E. (2009). Nanoparticle therapeutics: a personal perspective. Wiley Interdiscip. Rev. Nanomedicine Nanobiotechnology 1 (3), 264–271. doi:10.1002/wnan.6
Murday, J. S., Siegel, R. W., Stein, J., and Wright, J. F. (2009). Translational nanomedicine: status assessment and opportunities. Nanomedicine Nanotechnol. Biol. Med. 5 (3), 251–273. doi:10.1016/j.nano.2009.06.001
Nemati, E., Mokhtarzadeh, A., Panahi-Azar, V., Mohammadi, A., Hamishehkar, H., and Mesgari-Abbasi, M. (2019). Ethambutol-loaded solid lipid nanoparticles as dry powder inhalable formulation for tuberculosis therapy. AAPS PharmSciTech 20, 120–129. doi:10.1208/s12249-019-1334-y
Nemmar, A., Hoet, P., Vanquickenborne, B., Dinsdale, D., Thomeer, M., Hoylaerts, M., et al. (2002). Passage of inhaled particles into the blood circulation in humans. Circulation 105 (4), 411–414. doi:10.1161/hc0402.104118
Nurbaeti, S. N., Brillault, J., Tewes, F., and Olivier, J. C. (2019). Sustained-release microparticle dry powders of chloramphenicol palmitate or thiamphenicol palmitate prodrugs for lung delivery as aerosols. Eur. J. Pharm. Sci. 138, 105028. doi:10.1016/j.ejps.2019.105028
Nozohouri, S., Salehi, R., Ghanbarzadeh, S., Adibkia, K., and Hamishehkar, H. (2019). A multilayer hollow nanocarrier for pulmonary co-drug delivery of methotrexate and doxorubicin in the form of dry powder inhalation formulation. Mater. Sci. Eng. C 99, 752–761. doi:10.1016/j.msec.2019.02.009
Osman, N., Kaneko, K., Carini, V., Saleem, I., et al. (2018). Carriers for the targeted delivery of aerosolized macromolecules for pulmonary pathologies. Expert Opin. Drug Deliv. 15 (8), 821–834. doi:10.1080/17425247.2018.1502267
Party, P., Bartos, C., Farkas, Á., Szabó-Révész, P., Ambrus, R., et al. (2021). Formulation and in vitro and in silico characterization of “nano-in-micro” dry powder inhalers containing meloxicam. Pharmaceutics 13 (2), 211. doi:10.3390/pharmaceutics13020211
Porsio, B., Lentini, L., Ungaro, F., Di Leonardo, A., Quaglia, F., Giammona, G., et al. (2020). Inhalable nano into micro dry powders for ivacaftor delivery: the role of mannitol and cysteamine as mucus-active agents. Int. J. Pharm. 582, 119304. doi:10.1016/j.ijpharm.2020.119304
Price, D. N., Stromberg, L. R., Kunda, N. K., and Muttil, P. (2017). In vivo pulmonary delivery and magnetic-targeting of dry powder nano-in-microparticles. Mol. Pharm. 14 (12), 4741–4750. doi:10.1021/acs.molpharmaceut.7b00532
Puri, V., Chaudhary, K. R., Singh, A., and Singh, C. (2022). Inhalation potential of N-Acetylcysteine loaded PLGA nanoparticles for the management of tuberculosis: in vitro lung deposition and efficacy studies. Curr. Res. Pharmacol. Drug Discov. 3, 100084. doi:10.1016/j.crphar.2022.100084
Radivojev, S., Luschin-Ebengreuth, G., Pinto, J. T., Laggner, P., Cavecchi, A., Cesari, N., et al. (2021). Impact of simulated lung fluid components on the solubility of inhaled drugs and predicted in vivo performance. Int. J. Pharm. 606, 120893. doi:10.1016/j.ijpharm.2021.120893
Rehman, M. U., Khan, M. A., Khan, W. S., Shafique, M., and Khan, M. (2018). Fabrication of Niclosamide loaded solid lipid nanoparticles: in vitro characterization and comparative in vivo evaluation. Artif. cells, nanomedicine, Biotechnol. 46 (8), 1926–1934. doi:10.1080/21691401.2017.1396996
Rezazadeh, M., Davatsaz, Z., Emami, J., Hasanzadeh, F., and Jahanian-Najafabadi, A. (2018). Preparation and characterization of spray-dried inhalable powders containing polymeric micelles for pulmonary delivery of paclitaxel in lung cancer. J. Pharm. Pharm. Sci. 21 (1s), 200s–214s. doi:10.18433/jpps30048
Safety, T. (2024). Pharmacokinetics of inhaled nanoparticle formulation of remdesivir (GS-5734) and NA-831.
Sabuj, M. Z. R., Dargaville, T. R., Nissen, L., and Islam, N. (2021). Inhaled ciprofloxacin-loaded poly (2-ethyl-2-oxazoline) nanoparticles from dry powder inhaler formulation for the potential treatment of lower respiratory tract infections. PLoS One 16 (12), e0261720. doi:10.1371/journal.pone.0261720
Shafique, M., Khan, M. A., Khan, W. S., Ahmad, W., and Khan, S. (2017). Fabrication, characterization, and in vivo evaluation of famotidine loaded solid lipid nanoparticles for boosting oral bioavailability. J. Nanomater. 2017 (1), 1–10. doi:10.1155/2017/7357150
Satari, N., Taymouri, S., Varshosaz, J., Rostami, M., and Mirian, M. (2020). Preparation and evaluation of inhalable dry powder containing glucosamine-conjugated gefitinib SLNs for lung cancer therapy. Drug Dev. Industrial Pharm. 46 (8), 1265–1277. doi:10.1080/03639045.2020.1788063
Soni, S., Jaimini, A., Ahmad, F. J., Bhatnagar, A., Mittal, G., et al. (2011). Edetate calcium disodium nanoparticle dry powder inhalation: a novel approach against heavy metal decorporation. Int. J. Pharm. 416 (1), 376–383. doi:10.1016/j.ijpharm.2011.06.038
Stocke, N. A., Meenach, S. A., Arnold, S. M., Mansour, H. M., and Hilt, J. Z. (2015). Formulation and characterization of inhalable magnetic nanocomposite microparticles (MnMs) for targeted pulmonary delivery via spray drying. Int. J. Pharm. 479 (2), 320–328. doi:10.1016/j.ijpharm.2014.12.050
Sung, J. C., Padilla, D. J., Garcia-Contreras, L., VerBerkmoes, J. L., Durbin, D., Peloquin, C. A., et al. (2009). Formulation and pharmacokinetics of self-assembled rifampicin nanoparticle systems for pulmonary delivery. Pharm. Res. 26, 1847–1855. doi:10.1007/s11095-009-9894-2
Tai, W., Chang, R. Y. K., Chan, H. K., Kwok, P. C. L., et al. (2021). In vitro-in vivo correlation of cascade impactor data for orally inhaled pharmaceutical aerosols. Adv. Drug Deliv. Rev. 177, 113952. doi:10.1016/j.addr.2021.113952
Talegaonkar, S., Ali, R., Mittal, G., Ahmad, F. J., Bhatnagar, A., et al. (2012). Inhalation of alendronate nanoparticles as dry powder inhaler for the treatment of osteoporosis. J. Microencapsul. 29 (5), 445–454. doi:10.3109/02652048.2012.655428
Taki, M., Marriott, C., Zeng, X. M., and Martin, G. P. (2010). Aerodynamic deposition of combination dry powder inhaler formulations in vitro: a comparison of three impactors. Int. J. Pharm. 388 (1-2), 40–51. doi:10.1016/j.ijpharm.2009.12.031
Thiyagarajan, D., Huck, B., Nothdurft, B., Koch, M., Rudolph, D., Rutschmann, M., et al. (2021). Spray-dried lactose-leucine microparticles for pulmonary delivery of antimycobacterial nanopharmaceuticals. Drug Deliv. Transl. Res. 11 (4), 1766–1778. doi:10.1007/s13346-021-01011-7
Tomoda, K., Ohkoshi, T., Kawai, Y., Nishiwaki, M., Nakajima, T., and Makino, K. (2008). Preparation and properties of inhalable nanocomposite particles: effects of the temperature at a spray-dryer inlet upon the properties of particles. Colloids Surfaces B Biointerfaces 61 (2), 138–144. doi:10.1016/j.colsurfb.2007.07.016
Tsapis, N., Bennett, D., Jackson, B., Weitz, D. A., Edwards, D. A., et al. (2002). Trojan particles: large porous carriers of nanoparticles for drug delivery. Proc. Natl. Acad. Sci. 99 (19), 12001–12005. doi:10.1073/pnas.182233999
Ungaro, F., dAngelo, I., Coletta, C., d'Emmanuele di Villa Bianca, R., Sorrentino, R., Perfetto, B., et al. (2012). Dry powders based on PLGA nanoparticles for pulmonary delivery of antibiotics: modulation of encapsulation efficiency, release rate and lung deposition pattern by hydrophilic polymers. J. Control. release 157 (1), 149–159. doi:10.1016/j.jconrel.2011.08.010
Valsalakumari, R., Yadava, S. K., Szwed, M., Pandya, A. D., Mælandsmo, G. M., Torgersen, M. L., et al. (2021). Mechanism of cellular uptake and cytotoxicity of paclitaxel loaded lipid nanocapsules in breast cancer cells. Int. J. Pharm. 597, 120217. doi:10.1016/j.ijpharm.2021.120217
Wan, K. Y., Weng, J., Wong, S. N., Kwok, P. C. L., Chow, S. F., Chow, A. H. L., et al. (2020a). Converting nanosuspension into inhalable and redispersible nanoparticles by combined in-situ thermal gelation and spray drying. Eur. J. Pharm. Biopharm. 149, 238–247. doi:10.1016/j.ejpb.2020.02.010
Weng, J., Tong, H. H., and Chow, S. F. (2020b). In vitro release study of the polymeric drug nanoparticles: development and validation of a novel method. Pharmaceutics 12 (8), 732. doi:10.3390/pharmaceutics12080732
Weng, J., Shao, Z., Chan, H. W., Li, S. P. Y., Lam, J. K. W., Tsang, C. K., et al. (2022). Mediating bio-fate of polymeric cholecalciferol nanoparticles through rational size control. Biomater. Adv. 140, 213074. doi:10.1016/j.bioadv.2022.213074
Yiu, H. H. P., McLean, P., Brown, W., Boyles, M., et al. (2021). Simulated biological fluids–a systematic review of their biological relevance and use in relation to inhalation toxicology of particles and fibres. Crit. Rev. Toxicol. 51 (3), 217–248. doi:10.1080/10408444.2021.1903386
Yu, L. X., Amidon, G., Khan, M. A., Hoag, S. W., Polli, J., Raju, G. K., et al. (2014). Understanding pharmaceutical quality by design. AAPS J. 16, 771–783. doi:10.1208/s12248-014-9598-3
Zendehdel Baher, S., Yaqoubi, S., Asare-Addo, K., Hamishehkar, H., and Nokhodchi, A. (2022). Dry powder formulation of simvastatin nanoparticles for potential application in pulmonary arterial hypertension. Pharmaceutics 14 (5), 895. doi:10.3390/pharmaceutics14050895
Zhong, Q. (2018). Co-spray dried mannitol/poly (amidoamine)-doxorubicin dry-powder inhaler formulations for lung adenocarcinoma: morphology, in vitro evaluation, and aerodynamic performance. AAPS PharmSciTech 19, 531–540. doi:10.1208/s12249-017-0859-1
Keywords: inhalation, dry-powder nano formulation, lung diseases, nano medicine, bioavailability
Citation: Naureen F, Shah Y, Rehman Mu, Chaubey P, Nair AK, Khan J, Abdullah , Shafique M, Shah KU and Ahmad B (2024) Inhalable dry powder nano-formulations: advancing lung disease therapy-a review. Front. Nanotechnol. 6:1403313. doi: 10.3389/fnano.2024.1403313
Received: 19 March 2024; Accepted: 10 June 2024;
Published: 01 August 2024.
Edited by:
Omid Bavi, Shiraz University of Technology, IranReviewed by:
Islam A. Khalil, Misr University for Science and Technology, EgyptMohaddeseh Vafaiee, Amirkabir University of Technology, Iran
Copyright © 2024 Naureen, Shah, Rehman, Chaubey, Nair, Khan, Abdullah, Shafique, Shah and Ahmad. This is an open-access article distributed under the terms of the Creative Commons Attribution License (CC BY). The use, distribution or reproduction in other forums is permitted, provided the original author(s) and the copyright owner(s) are credited and that the original publication in this journal is cited, in accordance with accepted academic practice. No use, distribution or reproduction is permitted which does not comply with these terms.
*Correspondence: Maqsood ur Rehman, bXJlaG1hbkB1b20uZWR1LnBr; Muhammad Shafique, bXNoYWZpcXVlQHN1LmVkdS5zYQ==