- 1Amity Institute of Nanotechnology, Amity University Uttar Pradesh, Noida, India
- 2Departamento de Mecánica, Facultad de Ingeniería, Universidad Tecnológica Metropolitana, Santiago, Chile
- 3Amity Institute of Click Chemistry Research and Studies, Amity University Uttar Pradesh, Noida, India
Agrochemicals are substances used to promote plant growth and eradicate pests; and are essential to meeting the world food demand both qualitatively and quantitatively. Unfortunately, their extensive usage has resulted in certain significant problems, i.e., soil contamination, bioaccumulation, and the development of pest resistance. Consequently, there is a need for a reduced and more regulated usage of agrochemicals. The development of sustainable nanomaterials is encouraged in agricultural nanotechnology to minimize the usage of conventional fertilizers, herbicides, and pesticides. Metal-organic Framework (MOF)s are porous materials made of functional organic ligands and metal nodes or clusters connected by covalent bonds. Researchers are becoming increasingly interested in using MOF in a variety of sectors. The features of engineered MOFs have the potential to be an optimal strategy for producing impressively unique results in agricultural research. They also have advantages in terms of biocompatibility and biodegradability, which can be leveraged to boost the efficiency of traditional agrochemicals administration. Controlled release of agrochemicals offers an innovative solution to decrease the nutrient uptake rates and contamination of the environment. The application of MOF nanoparticles as nanocarriers to modify the loading and release properties of agrochemicals is particularly highlighted in this review.
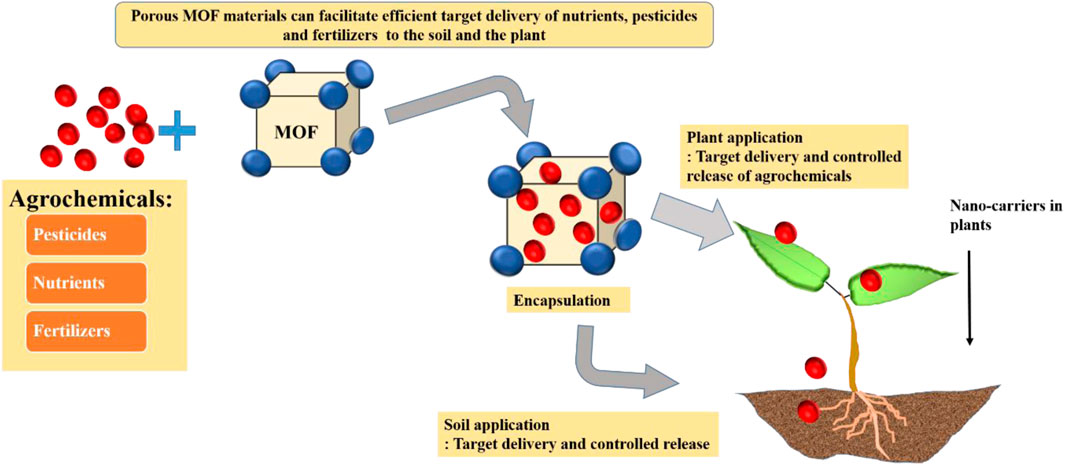
GRAPHICAL ABSTRACT | Probable application of MOFs related to agrochemicals for controlled release to achieve sustainability in agriculture.
1 Introduction
One of the seventeen sustainable development goals set forward by the United Nations (UN) is “Zero hunger,” and achieving this goal will require sustainable agriculture. The global food supply and distribution system is under extreme stress because of growing environmental pollution, population growth, climate change, and elevated water and energy requirements. Annual agricultural production worldwide nowadays utilizes an astounding amount of natural and industrial resources. The yearly requirement of agrochemicals all over the world is approximately 234.2 billion US dollars (USD) in 2019 and this value is expected to rise to more than 300 billion USD by 2025 (Devi et al., 2022). The global food production systems must undergo significant changes in response to this circumstance. Fertilizers and pesticides have become essential components of modern agricultural systems to meet the enormous demand for food. Pesticides can be categorized according to several factors, including toxicity, target, chemical formula and composition, mode of entry or action, and source. However, between 10% and 75% of pesticides fail to reach their intended targets due to improper application techniques, and frequently contaminate both terrestrial and aquatic environments (Rojas Macías et al., 2022). It also promotes insect and disease resistance, reduces soil biodiversity, and increases the bioaccumulation of pesticides in the food. Even with the stringent governmental legislation, chemicals still find their way into the food chain. Concerns related to undeveloped countries are more severe (Rojas Macías et al., 2022). The impacts of conventional agrochemicals can be felt in the soil, where they are released by a variety of exposures. For instance, following application, the loss of fertilisers and pesticides results in significant environmental loss and higher cultivation expenses. This results in the overuse and frequent application of agrochemicals, which over time may contaminate plants, soil, and water. Despite these negative side effects, their use is necessary to increase agricultural output to fulfil the food demand of the growing population. The losses that occur during application can be attributed to several factors such as the weather, the formulations utilised, and the application techniques employed (Pretty and Pervez Bharucha, 2015).
A popular strategy for attaining regional and global sustainability is the circular economy (CE). As a result, certain behaviours and activities including eco-design, reuse, refurbishing, and remanufacturing define CE. To meet the 2030 Agenda for Sustainable Development objectives of combating climate change (SDG11), ending poverty and hunger (SDGs 1 and 2), and maintaining natural resources, food and agriculture play a critical role. This calls for radical adjustments in the direction of sustainability in agriculture as well as more sustainable usage and management of the current agricultural systems to maintain food production and the ecosystems (Nhemachena et al. 2018; Agrawal et al. 2023). Recent studies have demonstrated the promising potential of nanotechnology to boost the efficiency of agricultural inputs, provide solutions for environmental and agricultural issues and enhance food security and productivity (Usman et al., 2020). “Smart” nanocarriers need to exhibit new and distinctive qualities in the delivery of agrochemicals to achieve improved agrochemical efficiency. Recent developments indicate that the extraordinary characteristics of nanoparticles can be used for this purpose (Goenka et al., 2014; Hsu et al., 2023). The term “precision farming,” which aims to produce optimum crops without depleting water and soil resources, uses various carriers to carry and deliver agrochemicals in a more regulated and slow-release profile (Dutta et al., 2022).
After entering the intricate plant-soil system, these modern nanomaterials can change the physiology of plants, which may be easily used to comprehend the impacts. Furthermore, a fundamental understanding of these nanomaterials’ positive or negative interactions with plants is necessary for the regulated delivery of active chemicals. Furthermore, it is thought that the concentration of nanomaterial in the natural environment is far lower than those deemed dangerous (Batley et al., 2013). High-level research is being done to create new delivery systems with nanoscale materials. It has been observed that when used as an agrochemical carrier, nanoparticles exhibit greater permeability, stiffness, thermal stability, and biodegradability than traditional agrochemicals (Nair et al., 2010; Deka et al., 2021). “Smart” nanocarriers provide specific formulation procedures that allow for a regulated release over an extended period of exposure and greatly prevent the early loss of the active components. One benefit of this strategy is that it uses fewer chemicals and requires less manual application (Fincheira et al., 2023). The active ingredients can be evenly distributed across the soil or plant surface due to the enormous surface area of nanoscale particles.
Currently, various nanocarriers for biological applications are being evaluated. They include the “soft” nanoparticles (NPs)- having an organic backbone (Shakiba et al., 2020), “hard” NPs-synthesized from metallic elements (Li et al., 2007; Chandrakala et al., 2022), nanoclays (bin Hussein et al., 2005; Merino et al., 2020), carbon materials (Sarlak et al., 2014; Zare et al., 2021) and so on. However, the most significant issues present in these nanocarriers are long-term instability, initial burst of material entrapped, and bio-toxicity (Petrovic and Barbinta-Patrascu, 2023). These issues need to be addressed soon for sustainability in agriculture.
Metal-organic frameworks (MOFs) are a unique class of porous material that is formed due to the self-assembly of infinite inorganic lattices (metal ions or clusters) and organic ligands via coordination bonds. They comprise of organic linkers, such as carboxylates, nitrogenated, or phosphonates, and inorganic nodes, such as atoms, chains, or clusters (Allendorf et al., 2021). The orientation of the metal and any associated linkers define its final structure. MOFs have been suggested for a wide range of industrial and social applications, including drug delivery (Anstoetz et al., 2016), energy (Srinivasan et al., 2020), adsorption (Ma et al., 2016; Bhattacharyya et al., 2023), separation (Qian et al., 2020), magnetism (Muratović et al., 2020), luminescence (Liu Yang et al., 2019c), conductivity (Su et al., 2020), electrochemical sensing (Zheng et al., 2018; Mohan et al., 2023c), catalysis (Chen et al., 2020a), heavy metal removal (Mohan et al. 2023a) and so many. We are totally dependent on agriculture and food industries for producing and processing food. Food products have been contaminated due to the growing use of hazardous chemicals in agriculture and packaging processes. MOFs in particular demonstrate great potential as agricultural materials because of their intriguing properties such as large specific surface areas and pore volumes, exceptional sorption capacities, large-scale synthesis and stability to carry out their function, preventing associated toxicity in animals/plants due to their accumulation after decomposition (Bazer-Bachi et al., 2014). MOFs usually have a higher porosity level and a more defined and adjustable pore size distribution when compared to other traditional porous materials. Furthermore, a significant deal of flexibility in the synthesis of MOFs with varying pore size, configuration, morphology, and chemical and physical properties is made possible by the many options for metal nodes and organic linkers, as well as the customizable synthesis parameters. These unique qualities have drawn a lot of interest in MOFs and shown a wide range of potential applications. From this perspective, metal-organic frameworks (MOFs) seem to be a more sustainable choice. In addition to bioavailability, the surface deposition of polar molecules can alter the water solubility of MOFs by improving their distribution in soil media. Furthermore, MOF provides stability by avoiding chemical modification of the encapsulated compounds in the empty spaces of the MOF architecture (Nadar and Rathod, 2018).
To increase agrochemicals’ effectiveness and reduce their detrimental effects on the environment, their release must be slow and regulated from the formulation. Several studies on MOF-based carriers have previously shown how they can improve the effectiveness of nitrogen fertilizers. It is also possible to supplement the micronutrient for a specific crop by changing the metal centres of MOFs (Shan et al., 2020a). In this review, we outlined a brief discussion on the synthesis approaches and attributes of a MOF structure. We have also emphasized the application of MOFs and MOF derivatives in agriculture and highlighted different previously published MOF-based materials for their targeted delivery and sustained release of agrochemicals. Finally, we concluded by highlighting the prospects of MOF-based materials in agricultural applications.
2 Overview of MOF structures
MOFs are renowned for their wide variety of topologies and functionalities. When first considering molecular metal-organic hybrids, it is important to keep in mind that several factors, including design, binding affinity, and chelation of linker, open metal sites on the clusters, ionic strength between metal and organic component' and the symmetry of the moiety, influence the final structural design of the framework (Figure 1). For instance, a well-detailed description of an Ag-MOF framework with all the structural details was described (Figure 2) in a report by (Mohan et al. 2023b). The MOF possessed a monoclinic cc space group structure. Moreover, the unit consisted of a ligand, metal centre Ag (I), and one water molecule lattice. The nitrogen atoms in the pyridine and triazole groups of the organic ligand bond to the metal. Because of this, the core metal in Ag-MOFs has a distorted triangle geometry utilizing one pyridine and two triazole nitrogen from separate ligands.
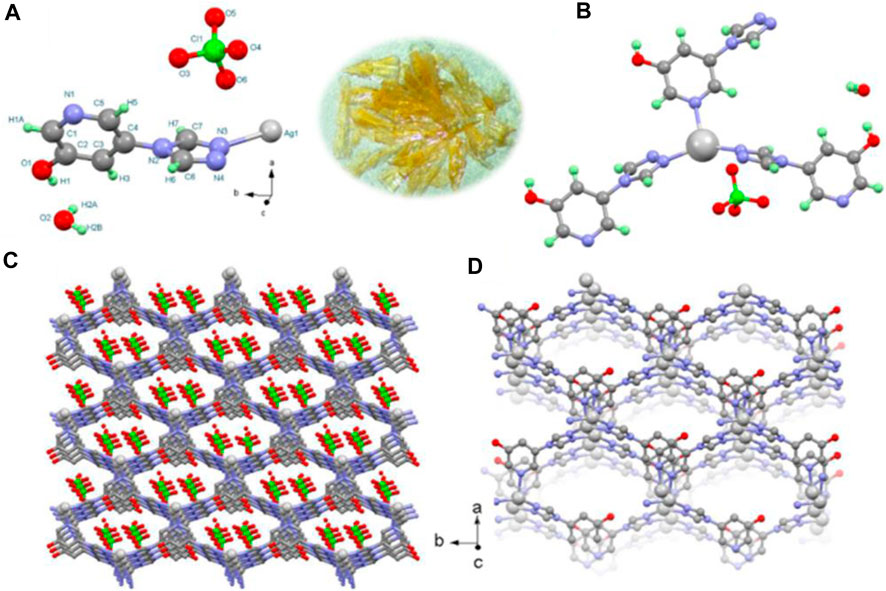
Figure 2. Asymmetric unit, symmetry code X, 2 − Y, 1/2 + Z, and −1/2 + X, 3/2 − Y, 1/2 + Z (A), coordination environment around the metal centre (B), location of anions and lattice water in the pore (C), and formation of a 3D network (D) in Ag-MOF1 (obtained yellow flake crystals photo inserted in the middle of (a) and (b)). “Reprinted with permission from (Mohan et al., 2023b). Copyright (2023) American Chemical Society.”
When taken as a whole, these intricate elements have a big impact on the ultimate structural design, use, and application of a MOF (Kirchon et al., 2018). MOFs are known for having pore sizes that may be adjusted, incredibly large surface areas, and flexible internal surface characteristics. These characteristics can be regulated by changing the combination of inorganic and organic components. There are countless options when the metal ion or cluster and the organic linker are combined to form a MOF. Interesting characteristics for an MOF are provided by the combination of the inorganic and organic components’ physical attributes as well as any potential synergistic interactions between them. Dimensionality is a major factor that may be used to categorize MOFs and determine how intrinsically functional they are. For example, 3D-MOFs typically have substantial pore volumes and are frequently utilized for medicine delivery, gas storage, and water harvesting. Yet, 2D-MOFs can behave as gas separation membranes since they are created by van der Waals interactions. As a result, 2D-MOFs are advantageous in the fields of chemical sensing, supercapacitors, and gas separation. Thus, we can facilitate a new set of applications and properties by designing multidimensional MOFs (Dutta et al. 2021).
The precisely defined and adjustable crystalline structures of MOF provide them with a strong basis for the development of distinct structure–property relationships for making novel functional materials. MOFs are formed by combining metals or metal clusters, also known as secondary building units (SBUs), with organic linkers, primarily ligands that include nitrogen or carboxylic acid. It is possible to customise MOFs to some extent as anticipated by carefully choosing SBUs and linkers since the geometry of SBUs and the dimension and shape of the organic ligands determine the structures of MOFs. Yaghi and Li (1995) demonstrated that by methodically elongating the ligand structure, an isoreticular series of MOF-74 structures (IRMOF-74-n) can be produced. However, different variations of IRMOF can be possible with different porous structures. Theoretically, it is possible to anticipate the MOF structures by considering the metals and organic linker building blocks. MOFs possess pore sizes of microporous to macroporous depending on their organic linker. MOFs with distinct electrical, magnetic, and optical properties can have their metal ions/clusters and organic ligands precisely adjusted to the needs of the application. Furthermore, different functional groups can be placed in the pore space of MOFs to serve a variety of applications. Carboxylates or other organic anions, such as phosphonate, sulfonate, and heterocyclic compounds, are the organic units (linkers/bridging ligands). MOFs with preconfigured structural topologies can be built because of the orientation of the organic linkers. Zinc nitrate [Zn(NO3)2] and terephthalate (bdc) for instance react to form crystalline MOF-5 under solvothermal conditions. On the other hand, slow vapour diffusion of 10 triethylamine/toluene at room temperature into an N,N′-dimethylformamide (DMF)/toluene solution containing a mixture of Zn(NO3)2/H2bdc results in colourless prism-shaped crystals of Zn(bdc)(DMF)(H2O). Moreover, tetranuclear octahedral Zn4O(CO2)6 SBU can be produced hydrothermally in basic zinc acetate. The ditopic carboxylate bdc, which is used in place of the acetate, has a stiff and divergent property that enables the clusters to articulate into a 3D framework with an attractively beautiful cubic shape (Lu et al., 2014). It has been claimed that a key factor in influencing the stability of MOF is the strength of the bond between the metal oxide cluster and the linker. It is discovered that the breakdown of MOF-5 is caused by the dissolution of carboxylic bridges between the benzene rings and Zn4O clusters at 400°C or higher. On the contrary, MOF-177 has low stability as it breaks down in water in 12 h and gradually changes from hexagonal to orthogonal phase and then to monoclinic phase in 5 weeks at room temperature (Kang et al., 2011). The relative stability of the MOFs depends on the metal ions which can be explained by the chemical inertness of the metal ions. Moreover, the bond strength between the metal cluster and the linker material has been used to explain the relative hydrothermal stability of MOFs. For example, CrIII is a well-known inert ion which explains the excellent stability of MIL-100 and MIL-101 in water (Haque et al., 2010; Kang et al., 2011). Another kind of fascinating 3D structure can be obtained by chelation between two bridged Ce3+ centres and eight carboxylate groups (Lu et al., 2014).
A new field of study in MOF is the manipulation of crystal size and form since this allows for the optimization of the physical characteristics for particular uses. Furthermore, thin films or membranes can be fabricated using nanoscale MOF crystals. The literature has documented a variety of techniques for creating MOF thin films or membranes. There are four different ways that growth can occur: direct, layer-by-layer, secondary, and chemical solution deposition (Stock and Biswas, 2012).
However, among different application areas of MOF, agriculture is an evolving one. The intriguing properties of MOFs make them promising materials for agriculture: i) large surface areas and pore volumes leading to exceptional sorption capabilities; ii) Different hybrid compositions; iii) Versatile cavities having specific host-guest interactions; iv) Proposition for large scale synthesis and commercialization v) Adequate stability profile before and after performing their function. These specifically prevent further toxicity in the environment due to accumulation.
3 Synthesis strategies of metal-organic frameworks
The remarkable physicochemical properties of metal-organic frameworks (MOFs) have attracted a lot of interest nowadays. These materials sometimes are called as porous coordination polymers (PCPs), that are built by connecting inorganic nodes with organic ligands. The term MOFs was first defined in the 1990s by Yaghi and Li (Yaghi and Li, 1995). Since then, there has been an accelerated expansion in the field of MOF research in recent decades. By merely adjusting the synthesis settings, the characteristics and structures of MOF can be easily tailored to suit the application that calls for them. These MOFs are two-component systems made up of organic binder and metal or metal clusters, also known as secondary building units (SBUs). During synthesis or afterwards, it is simple to add functional groups into the pores of MOF structures. Because of their typically large porosity and internal surface area, MOF-based materials are used for the majority of applications (Stock and Biswas, 2012). The most remarkable aspects of them are their tunable structures and functionalized pores, which are obtainable by combining metal ions and certain ligands with functional groups. Functionalized linkers with groups like, -NH2, -SH, -OH,-F and -COOH are introduced to create functionalized MOFs (Kumar et al. 2023). Different types of synthesis strategies are illustrated in Figure 3 and discussed below.
Researchers have historically employed two of the most common techniques to synthesise a wide range of MOF-based structures: solvothermal and room temperature synthesis. Solvents with higher boiling points like water, dimethylformamide (DMF), and dimethyl sulfoxide (DMSO) can be used in the solvothermal approach. The heating of the reaction mixture in the closed vessel causes the internal pressure of the container to rise proportionately, changing the structural characteristics of the MOF. The highest yield per batch of synthesis is guaranteed by carefully selecting the reactants, pH, temperature, and reaction duration, and then allowing the container to cool gradually. For instance, fumaric acid and ZrO Cl2·8 H2O were dissolved in a solvent mixture of DMF and of formic acid under constant stirring. The resulting solution was then heated at 130 °C for 10 h. The white product MOF-801 was filtrated, washed and dried overnight at ambient temperature (Aghajani Hashjin et al. 2023).
On the other hand, room temperature synthesis is accomplished in open flasks with air pressure (Tranchemontagne et al., 2008; McKinstry et al., 2016). Defect cracks can occur on a MOF’s surface as a result of solvent evaporation by heat treatment in the open atmosphere. These cracks are crucial for the introduction of sizable meso and macropores without the drawbacks of microporosity. Avoiding the process of direct heat evaporation of a non-volatile solvent trapped in a MOF’s micropores is common since it tends to marginally decrease the porosity overall. Yang and team (2014) described a template-free method for synthesizing porous hierarchical structures MOF-1 (IRMOF-1). This method features a template-free method for introducing large-sized meso- and macropores with a uniform distribution inside the MOF structure (Yang et al., 2014).
At the industry level, two distinct electrochemical approaches, namely, anodic deposition and cathodic deposition are commonly used for MOF synthesis. Electrochemical synthesis of MOF has opened a versatile route for environment-friendly materials (Khazalpour et al., 2015). Mueller and the team were the first to report MOF synthesis via this technique (Mueller et al., 2006). The quantity of the product obtained in this process is one of the biggest advantages. The reaction time is also subsequently reduced in this method, where an electrode is immersed in an appropriate electrolyte and organic ligand solution. This allows the metal ions from the electrode to dissolve into the solution. There are two ways to perform MOF electrochemical synthesis: batch mode and continuous flow operation (Khazalpour et al., 2015). Being able to run continuous, high-frequency production of MOF is a distinct advantage of electrochemical synthesis as compared to conventional batch reactions (Mueller et al., 2006). Many of the risks associated with large-scale batch reactions, such as mass transfer, mixing, and heat runaway, are lessened in such continuous flow processes. Van Assche et al. (2016) published an important study on the electrosynthesis of HKUST-1 (Hong Kong University of Science and Technology -1) by anodic dissolution. A three-electrode electrochemical setup was used to synthesize HKUST-1. The working, counter, and reference electrodes in this configuration were Ag/AgCl, Pt foil, and Cu-coated silicon wafers, respectively. The electrodes were submerged in a solution of a solvent mixture of EtOH: H2O and BTC (Benzotrichloride). The four stages of this method are initial nucleation, island development, intergrowth, and MOF crystal detachment (Varsha and Nageswaran 2020).
Sonochemical synthesis offers homogeneous nucleation and reduced crystallization time compared to oven heating. Acoustic cavitation, another name for sonochemical synthesis, is the process of forming a bubble, growing it, and then watching it burst and disintegrate in the liquid. As the bubble collapses it generates extremely high temperatures and pressures locally. This is achieved via the application of high-frequency ultrasound. Son and the team have reported the use of this technique to synthesize high-quality MOF-5 crystals with 8 min of sonication (Son et al., 2008). Lower pH values resulted in the formation of monodispersed particles, however yield of this process is lower than 5% which was a major drawback (Son et al., 2008).
Microwave synthesis of MOF is another popular method because of its shorter reaction time. Electrical and magnetic components of electromagnetic radiation travel simultaneously and are perpendicular to each other. Electromagnetic waves, or microwaves, have energies ranging from 300 to approximately 300,000 MHz. The wave’s energy instantly heats the solvent, causing rapid crystallization, the production of nanoscale particles, and the elimination of impurities all at once. While the quality of the crystals produced by the microwave-aided approach is comparable to that of classic solvothermal methods, the procedure is significantly faster (Son et al., 2008). On the other hand, for industrial operations, this microwave synthesis method seems more appealing due to its shorter reaction times. Couzon et al. (2022) reported a novel synthesis method of MOF–textile composites UiO-66-NH2 by a simple microwave-assisted method. Excellent fiber-like samples have been produced that are further employed for nuclear and radiological protection tests. Because the crystals nucleate over the majority of the solution as a result of the localized solvent heating, this method is very effective in creating particles with tiny dimensions (Couzon et al., 2022).
A solvent-free method of synthesis or mechanical synthesis of MOF has attracted much attention in recent times. The procedure of grinding the solid reactants is quicker, more convenient, and uses little or no solvent. Grinding is one of the mechanochemical techniques used to create coordination polymers with open porosity. For example, to synthesize [Cu(INA)2] [INA = Isonicotinic acid] porous frameworks, Pichon and the team used a ball mill to crush isonicotinic acid and copper acetate for a short period. The successful formation of the product was signified by a change in the colour of the sample from green to blue (Pichon et al., 2006).
Another potent method for creating sophisticated materials with complex shapes, hierarchical organization, and controlled size, shape, and polymorphism in aquatic settings at ambient temperatures is biomimetic mineralization. The potential of biomimetic mineralization techniques has significantly increased with the growing comprehension of biomineralization principles (Xu et al., 2007). This process uses biomolecules like proteins, lipids, and polysaccharides to help form biominerals. The resulting biominerals frequently have complex morphological features and superior material qualities that far outweigh those of their synthetic counterparts. Consequently, the development of specialized organic-inorganic hybrid materials has recently required the application of biomimetic techniques meant to imitate the processes involved in biomineralization (Liang et al., 2017). Typical processes that produce metal-organic frameworks (MOFs) often result in poor conductivity and poor water stability, which may hinder their use in electrochemical sensing applications. Using a biomimetic approach, one-dimensional Ce-MOF nanowires with ultrafine structures have been produced. The produced mixed-valence Ce-MOF nanowires were found to have strong conductivity and good water stability, with a typical size of about 50 nm (Ma et al., 2021).
Despite advancements in MOF synthesis techniques, there is still a strong need for more rapid and dependable methods to achieve controlled synthesis of the targeted molecules. Microfluidic synthesis is one of these methods; it allows the synthesis of monodisperse nanomaterials with specific characteristics because of its small liquid volumes, quick mass transfer, and great mixing efficiency. The ability to precisely modify the physico-chemical properties of nanomaterials through structural deviations achievable by adjusting the flow reagents in microchannels is a remarkable aspect of the microfluidic synthesis approach. Microfluidics has also been used to realize the synthesis of various MOFs through droplet or flow-focusing regimes. The synthesis of MOF-enzyme composites was also accomplished using a continuous-flow microfluidic synthesis system. It was shown that the induction of defects (mesopores) in a MOF structure was caused by a change in the MOFs reactants in the microchannels. Koryakina et al. (2023) presented a continuous flow regime microfluidic production of HKUST-1 microcrystals with interesting structural properties. To accomplish the necessary reagent mixing, the reagent flows via the microchannels in a microfluidic chip (MFC) have been optimized through numerical simulation. Moreover, additions (metallic salts) and changes in reaction temperature provide control over the shape of HKUST-1 (Koryakina et al., 2023).
There are some alternative synthesis methods also available for synthesizing MOFs. For instance, in the diffusion method of synthesis, chemical solutions are layered on top of one another, divided by a solvent layer, or dispersed gradually through physical barriers. Gels are utilized occasionally as diffusion and crystallization media. Following the progressive diffusion of the precipitate solvent into the distinct layer, crystals develop at the interface between the layers. For example, Chen and team developed three-dimensional Zn(II) complex Zn(INA)2(H2O)4 (INA = isonicotinate) by a unique ammonia vapour diffusion method (Chen et al., 2016). Along with this, the Table 1 summarizes the advantages and disadvantages of different MOF synthesis methods.
The goal of MOF synthesis was initially to create novel chemicals and structures with intriguing properties, but the discipline has been expanding since then. The domains of mechano-, sono-, and electrochemical synthesis as well as microwave-assisted synthesis are emerging extensively. They have proven to be useful for a few chemicals, frequently with milder reaction conditions, producing materials with various particle sizes and characteristics. This may have implications for upscaling synthesis processes for different types of MOFs. These alternate methods have, however, mostly been used to synthesize popular MOF compounds, thus its reproducibility is still questionable. Therefore, systematic studies under well-defined conditions need to be performed before opting for a method for synthesizing a particular MOF.
4 Application of MOF in agrochemical delivery
MOFs, more specifically, nanoscale MOFs - have emerged as promising carriers for drug delivery and sustained release in recent years because of their superior encapsulating capabilities (He et al., 2021). In agriculture also MOFs are being used for the slow release of agrochemicals, and a few examples are discussed here.
4.1 Application of MOFs for sustained release of fertilizers
Anstoetz and team (2017) have reported on the use of oxalate-phosphate-amine metal-organic frameworks (OPA-MOFs) as growth regulators and nutrients to boost grain yield in wheat plants. The result of their study suggested that the synthetic MOF could serve as a productive source of N fertilizer (Anstoetz et al., 2017). Abdelhameed and the team conducted a study that demonstrated the potential of Fe-MOF with ethylenediamine tetra-acetic acid (EDTA) as a source of iron nutrition in Phaseolus vulgaris. The study found that compared to other iron resources, there was a 9.6% improvement in plant weight, protein, enzyme content, and chlorophyll II content (Abdelhameed et al., 2019). The results of the study indicated that the mechanism of Fe-MOF-EDTA-based growth promotion is the result of an increase in the chlorophyll content that ultimately resulted in better photosynthesis and later in more absorption of the nutrients by plants to grow.
A new MOF was synthesized using ferric chloride (FeCl3∙6H2O), phosphoric acid (H3PO4), oxalic acid (H2C2O4∙2H2O), urea (CO(NH2)2), and de-ionized water (H2O) under mild hydrothermal conditions (Wu et al., 2019). After adding in the field, the chemical structures of MOF changed, and oxalate-consuming bacteria in the soil caused its degradation. This ultimately resulted in the release of N, P, and K from the elemental components (Wu et al., 2019). A later study reported a Fe-MOF on a pilot scale under hydrothermal reaction conditions, and its degradation behavior and nutrient release were analyzed in field conditions (Wu et al., 2022). The N, P, and Fe content in their MOF was around 6.03, 14.48, and 14.69%, respectively. When applied in rice fields it was found that the MOF has supported the plant growth and can be used as an innovative fertilizer with sustained release. This large-scale synthesized Fe-MOFs displayed faster nutrient release when added in soil, which may be due to the consumption of oxalate by native oxalotrophic bacteria (Figure 4) (Wu et al., 2022). In an earlier study, Fe based MOFs were synthesized by a solvent-free mechanochemical method and used as slow release fertilizer (Du et al. 2021). The MOF were affirmed as oxalate phosphate amine frameworks (OPA-MOF), and its elemental composition was as- N (4.91%), P (15.71%), and Fe (18.60%). The potential application of these MOFs in agriculture as a novel slow-release fertilizer was indicated by the slow release rate of N, P, and K in soil (Du et al., 2021).
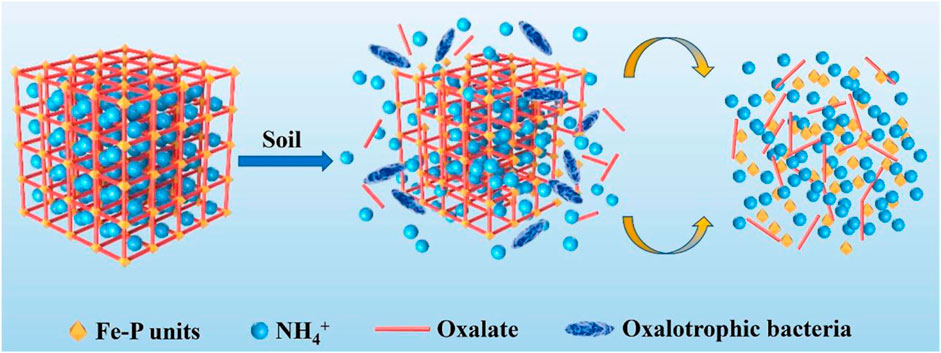
Figure 4. Schematic diagram indicating the MOF degradation in soil. “Reprinted with permission from (Wu et al. 2022) Copyright (2022) American Chemical Society.”
Liu and team documented a multipurpose porous carbon by employing β-cyclodextrin MOF (β-CD MOF) as a potassium source and precursor (Liu et al., 2019a). Comprehensive characterization results indicated that the produced carbon material (β-CD MOF-NPC) has abundant potassium element content, large surface areas, and a microporous structure. The results of the batch adsorption experiments showed that β-CD MOF-NPC could effectively remove four amide herbicides from water by adsorptive means, possibly by hydrogen bonding, electrostatic contact, or π–π interaction (Figure 5). Furthermore, by simultaneously adsorbing herbicides and releasing potassium ions in the pot trials, the β-CD MOF-NPC could efficiently support the growth of rice seedlings cultivated in wastewater contaminated with herbicides. The potassium released from MOF increased the chlorophyll content of rice leaves. So the results indicated that β-CD MOF-NPC combined the functions of fertilizer and adsorbent, making it a potential substance for cost-effective and sustainable agriculture (Liu et al., 2019a).
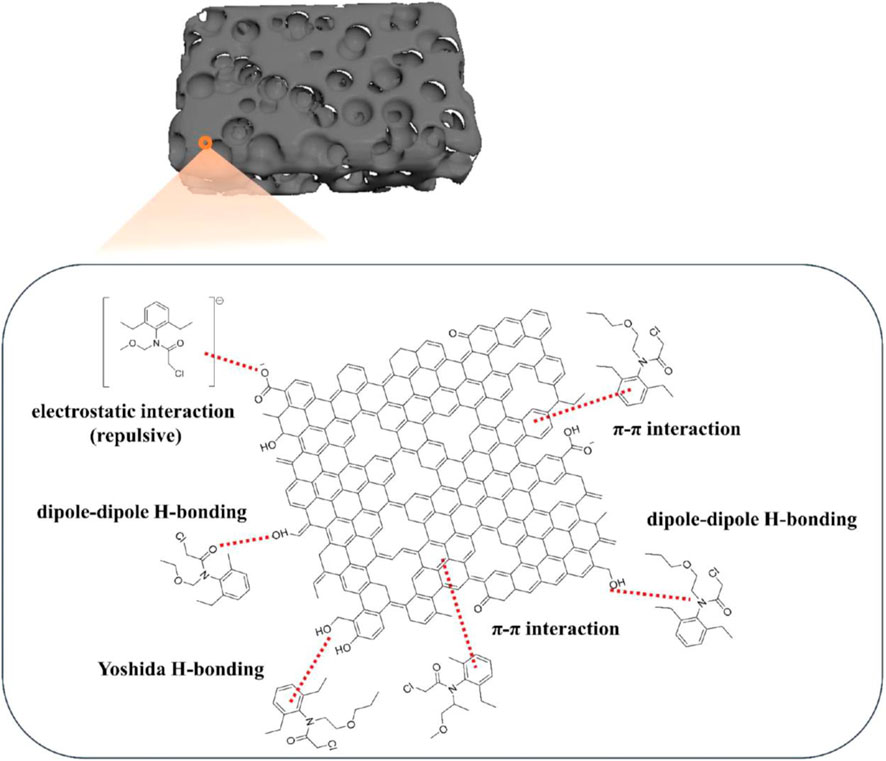
Figure 5. Possible interactions between β-cyclodextrin, MOF-NPC, and four amide herbicides. “Reprinted with permission from (Liu et al. 2019a). Copyright (2019) American Chemical Society.”
In another study, an easy technique of ion cross-linking was used to create a novel MOF(Fe)@NaAlg aerogels composite, which was then characterized using different techniques (Wu et al., 2020). The ammonium (NH4+)-loaded MOF(Fe)@NaAlg aerogels were ready as the slow-release fertilizer (SRF). MOF(Fe)@NaAlg (2:10) has an adsorption capacity of 29.4 mg/g and a swelling of 73 g/g, respectively. They looked at the swelling behaviors of MOF(Fe)@NaAlg(2:10) in various saline solutions. The produced formulation’s slow-release property was validated by the release assessments, indicating that MOF(Fe) plays an effective function in it. Additionally, the produced fertilizer compound showed outstanding soil water-retention capabilities; therefore have a wide range of possible uses in the agricultural sector (Wu et al., 2020). These earlier studies point to MOF’s possible use as fertilizers carrier in agriculture to achieve sustainability.
An innovative method was utilized to synthesize urea-templated Fe- OPA-MOF(I) / (II). The components P, N, and Fe found in plant nutrients were all present in both compounds (C8Fe8N16O52P8). These elements are arranged in corner-shared FeO6 and PO4 units, which link to oxalate units in both a and c directions to form the framework. Although the N and P levels of both compounds are appropriate for their use as fertilizers, compound I) exhibits the superior oxalate solubility needed to start the bacterial mineralization of the structural oxalate, which leads to structural collapse and release the plant nutrients in the soil. Compound II) has an excessively high solubility in oxalate, which may be due to a stronger surface connectivity of bigger macroscopic pores (Anstoetz et al. 2016). In addition to offering a route for more effective nutrient supply control than conventional fertilizers, the use of such MOFs in agriculture may assist address decreased soil fertility caused by acidification. Some more studies are listed in Table 2.
4.2 Pesticides
To protect the crops, yield, and quality, the application of pesticides is an indispensable part of modern-day agriculture. Their applications have assured the increasing the food demand of worlds’ growing population to some extent. However, the contamination of pesticides in food and environment is a serious concern. Earlier studies have indicated that the application of nanopesticides has many advantages like more scattering of the active ingredient, more bioavailability, longer durability, effective control, and sustainability (Balah and Pudake, 2019; Ray et al., 2023). Some of the studies using the MOF for sustainable release of pesticides are discussed below.
The exceptional chemical and thermal stability, non-toxicity, and mass production of nanoscale UiO-66 made it a promising candidate for use as a pesticide carrier (Meng et al., 2020). The nanoscale UiO-66 (LC@UiO-66) was used in this study to encapsulate lambda-cyhalothrin (LC) molecules, which had excellent sustained-release properties and an extremely high loading (87.71%). There had never been a loading of the active ingredient of this high for LC@UiO-66. Using nitrogen adsorption-desorption isotherms, thermogravimetric analysis measurements (TGA), Fourier transform infrared (FT-IR) spectroscopy, transmission electron microscopy (TEM), and X-ray diffraction (XRD), LC@UiO-66 nanoparticles were uniquely identified. The aggregate particle size of LC@UiO-66 nanoparticles was around 270 nm, and they exhibited a cubic form. LC@UiO-66 demonstrated a longer-lasting insecticidal impact in comparison to free LC and commercially available microcapsules, as demonstrated by the bioassay against Musca domestica (hygiene pests). In comparison to free LC and commercially available microcapsules, the bioassay demonstrated that LC@UiO-66 had a long-term insecticidal effect. Furthermore, the insecticidal activity of LC@UiO-66 was shown to be superior to that of commercially available microcapsules. Moreover, LC@UiO-66 effectively inhibited the activity of Aphis craccivora Koch, a pest of many crops. The aforementioned findings all showed that LC@UiO-66 has outstanding insecticidal properties in both agricultural and public hygiene (Meng et al., 2020).
In another study, the smart core-shell carrier was designed for the stimuli-based release of pesticides. It includes MIL-101(Fe)@silica for site-specific delivery of chlorantraniliprole (CAP) and also as a source of plant nutrition (Gao et al., 2021). The encapsulated CAP was 16.5 times more stable under the sunlight than the conventional formulation. The premature release of active ingredient was prevented by silica shell, and the release from MIL-101(Fe) core was triggered by basic environment. During the laboratory assay with Plutella xylostella larvae the LC50 values for the MOF based nanoformulation was found to be 0.389 mg/L. In green house experiment they found that CAP@MIL-101(Fe)@silica formulation was significantly more effective in achieving mortality rate and was safe for crops. The treatment enhanced the crop biomass by 18.6%–19.6% and 4.8%–17.7% in terms of fresh and dry weight, respectively. This growth promotion was attributed to the simultaneous action of MIL-101(Fe)@silica in site-specific pesticide delivery and acting as a fertilizer for crop (Gao et al., 2021).
In another study, zinc-based zeolitic imidazolate frameworks (ZIF-8) with encapsulated ortho-disulfides have been produced (Mejías et al., 2021). After 2-hydroxypropyl-β-cyclodextrin HP-β-CD surface functionalization, an in-situ approach was used to achieve encapsulation, which in turn improved the water solubility by more than eight times. The effectiveness of the encapsulation process and the sample’s crystallinity were confirmed further. Studies on the main weeds that infect rice, maize, and potato filed have shown that inhibition produces better results than the commercial herbicide- Logran. This discovery, in conjunction with a brief synthesis time of 2 hours at 25°C, represents a novel class of herbicides derived from natural products that have immediate agricultural applications (Mejías et al., 2021).
A degradable and intelligent MOF-based nanopesticide with MIL-101(FeIII) that is gated using FeIII-tannic acid (TA) networks was developed. In this formulation, the coordinatively unsaturated FeIII sites on the surfaces of the MIL-101(FeIII) nanocarriers naturally orient the capping of the FeIII-TA network gatekeepers. In this study, the release from the nanocarrier was stimuli based; and was tested for seven stimuli including the pH, H2O2, glutathione, phosphates, ethylene diamine tetraacetate (EDTA), and sunlight. The release of pesticide was studied in field conditions and was nanocarrier was degradable. The reported nanocarrier improved the wettability of aqueous formulation due to the naturally occurring polyphenol in model crops. It increased the pesticide adhesion and retention on foliage and was found to be effective against Rhizoctonia solani (rice sheath blight) and Fusarium graminearum (wheat head blight) by stimuli release of tebuconazole (Dong et al., 2021).
In another study, Chen and team synthesized aluminum-based metal-organic framework material (NH2-Al-MIL-101) with a “cauliflower-like” structure and a high surface area of 2,359.0 m2/g by using Al3+ as the metal node and 2-amino terephthalic acid as the organic chain (Chen et al., 2021). The fungicides diniconazole (Dini) and azoxystrobin (AZOX) were concurrently encapsulated into NH2-Al-MIL-101, with loading contents of 29.72% and 6.71%, respectively. The AZOX@Dini@NH2-Al-MIL-101 dual fungicide delivery system showed pH-responsive and prolonged release patterns. Dini and AZOX had respective release times of 46 and 136 h when their maximum cumulative release rates reached almost 90%. Moreover, AZOX@Dini@ NH2-Al-MIL-101 demonstrated improved germicidal efficiency against rice sheath blast (Chen et al., 2021).
Tang and team reported the construction of a porphyrin metal-organic framework (MOF) nanocomposite by introducing 5,10,15,20-tetrakis(1-methyl-4-pyridinio) porphyrin tetra(p-toluenesulfonate) (TMPyP) as a photosensitizer (PS) within the cage of MOF (HKUST-1) to effectively produce singlet oxygen under light irradiation (Tang et al., 2021). As a consequence of its good and broad-spectrum photodynamic antimicrobial activity against two pathogenic bacteria and three plant pathogenic fungi, the produced PS@MOF was shown to have a loading rate of roughly 12% (w/w). In the pot experiment, PS@MOF additionally showed exceptional control efficiency against Sclerotinia sclerotiorum on cucumber. A safety test on Chinese cabbage and cucumber along with Allium cepa chromosome aberration assay showed that PS@MOF was safe for plants and did not cause any genotoxicity. Porphyrin MOF thus showed significant promise as a new and effective alternative pesticide for sustainably managing plant diseases (Tang et al., 2021).
Yang and team developed two Ca2+ ions and l-lactate [CH3CH(OH)COO−] enriched porous, chiral metal-organic frameworks (MOFs): MOF-1201 and MOF-1203 (Yang et al., 2017). The carboxylate and hydroxyl groups of lactate and the carboxylate group of acetate bridge Ca2+ ions to produce a three-dimensional arrangement of Ca(-COO, -OH) polyhedra that support one-dimensional pores with apertures and internal diameters of 7.8 and 9.6 Å (MOF-1201) and 4.6 and 5.6 Å (MOF-1203), respectively. These MOFs provide lactate and Ca2+−based porous structures. They used it to encapsulate an important fumigant cis-1,3-dichloropropene in agriculture. The hydrolysis of MOF-1201 released the fumigant and indicated the development of biodegradable porous solid carrier for agrochemicals (Yang et al., 2017).
In a recent study, an intelligent stimuli-triggered release formulation of pyraclostrobin (PYR) was developed using the binding of pectin with FeMOF nanoparticles (Liang et al., 2022). The loading of PYR in FeMOF-pectin nanoparticles was achieved up to 20.6%, and release from the MOF was achieved with the action of dual stimuli of redox and pectinase. The MOF and pectin protected PYR from photodegradation and improved the spreading on the rice leaves. This resulted in achieving better efficiency in controlling Magnaporthe oryzae as compared to the control. The formulation also promoted the growth of rice without any phytotoxicity effects (Liang et al., 2022). In a similar study, the Fe-based MOF was used as a multifunctional carrier of Azoxystrobin (Shan et al., 2020a). A simple octahedral Fe-MOF achieved the loading of 16.2% fungicide active ingredient. Along with this, it also acted as a source of Fe nutrients and promoted wheat growth. The initial release from this Fe-MOFs was pH-responsive and then the fungicide was sustained released. It effectively controlled the two pathogens - wheat head scab (F. graminearum) and tomato late blight (Phytophthora infestans) (Shan et al., 2020a).
To explore the mechanism of plant growth promotion due to the MOF-based fungicide carrier, a study was done by Zhao and team (2022). In their study, the physiological, biochemical, and metabolic levels were estimated in wheat, and it was found that many metabolites were upregulated by the application of Fe-MOF. In the comparative study, the team also found that the negative effects of fungicides were reduced in wheat seedlings when treated with tebuconazole-loaded Fe-MOF. The result of this study indicated that MOF regulates the metabolic pathways, and results in mitigation of the negative effects from pesticides (Zhao et al., 2022). These results and other studies (Table 3) on MOF-based nanopesticides have indicated their potential in the field of modern agriculture for achieving high quality and yield.
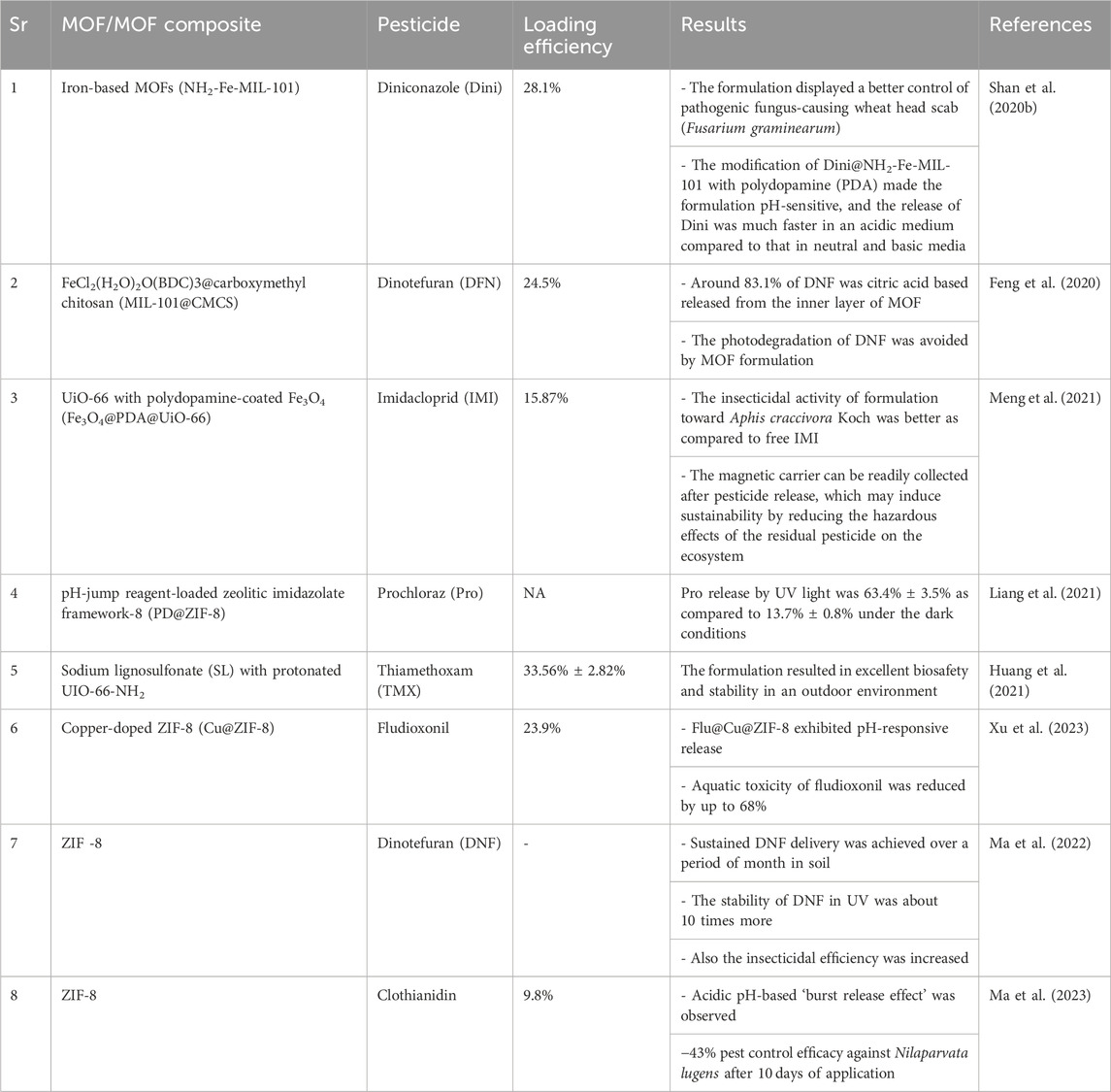
Table 3. List of MOF-based materials associated with the slow release of pesticides for sustainability in agriculture.
4.3 MOF-based nanoplatforms for delivery of plant hormones
Plant growth hormones are naturally occurring or synthetic organic compounds that can regulate plant growth and development. They mainly include auxins, cytokinins, abscisic acid, salicylic acid, nitric oxide, gibberellins (GAs), jasmonates, ethylene, and brassinosteroids. When applied judiciously these chemicals have the potential to enhance crop resistance and increase crop production and quality. So, it is needed to have a delivery method for the slow release and protection of plant hormones for sustainability. For overcoming the defects of traditional drug delivery methods MOF can be the alternative. For GA a smart supramolecular delivery system was prepared with CLT6@PCN-Q and found to have a loading capacity of 25.96%. The delivery system possesses multiple stimuli release based on pH, temperature, and competitive agent spermine (SPM). In plant growth studies, it was found that formulation was efficient in achieving stable GA bioactivity, promotion of wheat seeds germination, and stem growth of Chinese cabbage (Yang et al., 2021).
5 Conclusion and prospects
In conventional farming, overuse of agrochemicals, such as pesticides and fertilizers, is a dire problem since it can contaminate water and worsen the condition of the soil. In these circumstances, MOFs have emerged as a perfect candidate for the controlled release of agrochemicals and the liquid-phase adsorption of several dangerous compounds comprising sulfur and nitrogen. This review emphasizes the application of MOF as a novel carrier for agrochemical delivery and paves a novel path for the modern agriculture economy. They can be used for stimuli-based release, and the trigger can be achieved due to the changes happening in the crop microenvironment because of their interaction with pests. Along with these advantages of MOF for sustainability in crop production, they can also function as the source of some macro and micro-nutrients. Although this integrated strategy has many advantages, the transport and distribution in plants need further investigation on toxicology and safety. To effectively use agrochemicals, future research must concentrate on enhancing the loading capacity of MOF materials. MOF nanocarriers for agrochemicals provide enormous potential for lowering chemical needs and advancing sustainability.
Author contributions
SB: Writing–review and editing, Writing–original draft. PB: Writing–review and editing, Writing–original draft. RP: Writing–review and editing, Writing–original draft, Supervision, Conceptualization. PL: Writing–review and editing, Writing–original draft. UR: Writing–review and editing, Writing–original draft. SC: Writing–review and editing, Writing–original draft, Supervision.
Funding
The author(s) declare that no financial support was received for the research, authorship, and/or publication of this article.
Conflict of interest
The authors declare that the research was conducted in the absence of any commercial or financial relationships that could be construed as a potential conflict of interest.
Publisher’s note
All claims expressed in this article are solely those of the authors and do not necessarily represent those of their affiliated organizations, or those of the publisher, the editors and the reviewers. Any product that may be evaluated in this article, or claim that may be made by its manufacturer, is not guaranteed or endorsed by the publisher.
References
Abdelhameed, R. M., Abdelhameed, R. E., and Kamel, H. A. (2019). Iron-based metal-organic-frameworks as fertilizers for hydroponically grown Phaseolus vulgaris. Mater. Lett. 237, 72–79. doi:10.1016/j.matlet.2018.11.072
Aghajani Hashjin, M., Zarshad, S., Motejadded Emrooz, H. B., and Sadeghzadeh, S. (2023). Enhanced atmospheric water harvesting efficiency through green-synthesized MOF-801: a comparative study with solvothermal synthesis. Sci. Rep. 13, 16983. doi:10.1038/s41598-023-44367-1
Agrawal, R., Bhagia, S., Satlewal, A., and Ragauskas, A. J. (2023). Urban mining from biomass, brine, sewage sludge, phosphogypsum and e-waste for reducing the environmental pollution: current status of availability, potential, and technologies with a focus on LCA and TEA. Environ. Res. 224, 115523. doi:10.1016/j.envres.2023.115523
Allendorf, M. D., Stavila, V., Witman, M., Brozek, C. K., and Hendon, C. H. (2021). What lies beneath a metal–organic framework crystal structure? New design principles from unexpected behaviors. J. Am. Chem. Soc. 143, 6705–6723. doi:10.1021/jacs.0c10777
Anstoetz, M., Clark, M. W., and Yee, L. H. (2017). Response surface optimisation of an oxalate–phosphate–amine metal–organic framework (OPA-MOF) of iron and urea. J. Inorg. Organomet. Polym. Mater. 27, 996–1013. doi:10.1007/s10904-017-0547-3
Anstoetz, M., Sharma, N., Clark, M., and Yee, L. H. (2016). Characterization of an oxalate-phosphate-amine metal–organic framework (OPA-MOF) exhibiting properties suited for innovative applications in agriculture. J. Mater. Sci. 51, 9239–9252. doi:10.1007/s10853-016-0171-6
Bailey, T., Pinto, M., Hondow, N., and Wu, K.-J. (2021). Continuous microfluidic synthesis of zirconium-based UiO-67 using a coiled flow invertor reactor. MethodsX 8, 101246. doi:10.1016/j.mex.2021.101246
Balah, M. A., and Pudake, R. N. (2019). Use nanotools for weed control and exploration of weed plants in nanotechnology. Nanosci. Sustain. Agric., 207–231. doi:10.1007/978-3-319-97852-9_10
Batley, G. E., Kirby, J. K., and McLaughlin, M. J. (2013). Fate and risks of nanomaterials in aquatic and terrestrial environments. Accounts Chem. Res. 46, 854–862. doi:10.1021/ar2003368
Bazer-Bachi, D., Assié, L., Lecocq, V., Harbuzaru, B., and Falk, V. (2014). Towards industrial use of metal-organic framework: impact of shaping on the MOF properties. Powder Technol. 255, 52–59. doi:10.1016/j.powtec.2013.09.013
Bhattacharyya, P., Parmar, P. R., Basak, S., Dubey, K. K., Sutradhar, S., Bandyopadhyay, D., et al. (2023). Metal organic framework–derived recyclable magnetic coral Co@ Co3O4/C for adsorptive removal of antibiotics from wastewater. Environ. Sci. Pollut. Res. 30, 50520–50536. doi:10.1007/s11356-023-25846-4
bin Hussein, M. Z., Yahaya, A. H., Zainal, Z., and Kian, L. H. (2005). Nanocomposite-based controlled release formulation of an herbicide, 2, 4-dichlorophenoxyacetate incapsulated in zinc–aluminium-layered double hydroxide. Sci. Technol. Adv. Mater. 6, 956–962. doi:10.1016/j.stam.2005.09.004
Chandrakala, V., Aruna, V., and Angajala, G. (2022). Review on metal nanoparticles as nanocarriers: current challenges and perspectives in drug delivery systems. Emergent Mater. 5, 1593–1615. doi:10.1007/s42247-021-00335-x
Chen, D.-D., Yi, X.-H., Zhao, C., Fu, H., Wang, P., and Wang, C.-C. (2020a). Polyaniline modified MIL-100 (Fe) for enhanced photocatalytic Cr (VI) reduction and tetracycline degradation under white light. Chemosphere 245, 125659. doi:10.1016/j.chemosphere.2019.125659
Chen, H., Shan, Y., Cao, L., Zhao, P., Cao, C., Li, F., et al. (2021). Enhanced fungicidal efficacy by Co-delivery of azoxystrobin and diniconazole with cauliflower-like metal–organic frameworks NH2-Al-MIL-101. Int. J. Mol. Sci. 22, 10412. doi:10.3390/ijms221910412
Chen, W., Du, L., and Wu, C. (2020b). “Hydrothermal synthesis of MOFs,” in Metal-organic frameworks for biomedical applications (Amsterdam, Netherlands: Elsevier), 141–157.
Chen, Y., Yang, C., Wang, X., Yang, J., Ouyang, K., and Li, J. (2016). Kinetically controlled ammonia vapor diffusion synthesis of a Zn (ii) MOF and its H 2 O/NH 3 adsorption properties. J. Mater. Chem. A 4, 10345–10351. doi:10.1039/c6ta03314h
Couzon, N., Ferreira, M., Duval, S., El-Achari, A., Campagne, C., Loiseau, T., et al. (2022). Microwave-assisted synthesis of porous composites MOF–textile for the protection against chemical and nuclear hazards. ACS Appl. Mater. Interfaces 14, 21497–21508. doi:10.1021/acsami.2c03247
Deka, B., Babu, A., Baruah, C., and Barthakur, M. (2021). Nanopesticides: a systematic review of their prospects with special reference to tea pest management. Front. Nutr. 8, 686131. doi:10.3389/fnut.2021.686131
Devi, P. I., Manjula, M., and Bhavani, R. (2022). Agrochemicals, environment, and human health. Annu. Rev. Environ. Resour. 47, 399–421. doi:10.1146/annurev-environ-120920-111015
Dong, J., Chen, W., Feng, J., Liu, X., Xu, Y., Wang, C., et al. (2021). Facile, smart, and degradable metal–organic framework nanopesticides gated with FeIII-tannic acid networks in response to seven biological and environmental stimuli. ACS Appl. Mater. Interfaces 13, 19507–19520. doi:10.1021/acsami.1c04118
Du, Y., Xu, X., Ma, F., and Du, C. (2021). Solvent-free synthesis of iron-based metal-organic frameworks (MOFs) as slow-release fertilizers. Polymers 13, 561. doi:10.3390/polym13040561
Dubadi, R., Huang, S. D., and Jaroniec, M. (2023). Mechanochemical synthesis of nanoparticles for potential antimicrobial applications. Materials 16, 1460. doi:10.3390/ma16041460
Dutta, A., Pan, Y., Liu, J.-Q., and Kumar, A. (2021). Multicomponent isoreticular metal-organic frameworks: principles, current status and challenges. Coord. Chem. Rev. 445, 214074. doi:10.1016/j.ccr.2021.214074
Dutta, S., Pal, S., Panwar, P., Sharma, R. K., and Bhutia, P. L. (2022). Biopolymeric nanocarriers for nutrient delivery and crop biofortification. ACS omega 7, 25909–25920. doi:10.1021/acsomega.2c02494
Feng, P., Chen, J., Fan, C., Huang, G., Yu, Y., Wu, J., et al. (2020). An eco-friendly MIL-101@ CMCS double-coated dinotefuran for long-acting active release and sustainable pest control. J. Clean. Prod. 265, 121851. doi:10.1016/j.jclepro.2020.121851
Fincheira, P., Hoffmann, N., Tortella, G., Ruiz, A., Cornejo, P., Diez, M. C., et al. (2023). Eco-efficient systems based on nanocarriers for the controlled release of fertilizers and pesticides: toward smart agriculture. Nanomaterials 13, 1978. doi:10.3390/nano13131978
Gao, Q., Bai, Q., Zheng, C., Sun, N., Liu, J., Chen, W., et al. (2022). Application of metal–organic framework in diagnosis and treatment of diabetes. Biomolecules 12, 1240. doi:10.3390/biom12091240
Gao, Y., Liang, Y., Zhou, Z., Yang, J., Tian, Y., Niu, J., et al. (2021). Metal-organic framework nanohybrid carrier for precise pesticide delivery and pest management. Chem. Eng. J. 422, 130143. doi:10.1016/j.cej.2021.130143
Goenka, S., Sant, V., and Sant, S. (2014). Graphene-based nanomaterials for drug delivery and tissue engineering. J. Control. Release 173, 75–88. doi:10.1016/j.jconrel.2013.10.017
Haque, E., Jeong, J. H., and Jhung, S. H. (2010). Synthesis of isostructural porous metal-benzenedicarboxylates: effect of metal ions on the kinetics of synthesis. CrystEngComm 12, 2749–2754. doi:10.1039/b927113a
He, S., Wu, L., Li, X., Sun, H., Xiong, T., Liu, J., et al. (2021). Metal-organic frameworks for advanced drug delivery. Acta Pharm. Sin. B 11, 2362–2395. doi:10.1016/j.apsb.2021.03.019
Hsu, C.-Y., Rheima, A. M., Kadhim, M. M., Ahmed, N. N., Mohammed, S. H., Abbas, F. H., et al. (2023). An overview of nanoparticles in drug delivery: properties and applications. South Afr. J. Chem. Eng. 46, 233–270. doi:10.1016/j.sajce.2023.08.009
Huang, G., Deng, Y., Zhang, Y., Feng, P., Xu, C., Fu, L., et al. (2021). Study on long-term pest control and stability of double-layer pesticide carrier in indoor and outdoor environment. Chem. Eng. J. 403, 126342. doi:10.1016/j.cej.2020.126342
Inamuddin, A., and Mohammad, A. (2018). Applications of nanocomposite materials in drug delivery. Amsterdam, Netherlands: Elsevier.
Kang, I. J., Khan, N. A., Haque, E., and Jhung, S. H. (2011). Chemical and thermal stability of isotypic metal–organic frameworks: effect of metal ions. Chemistry–A Eur. J. 17, 6437–6442. doi:10.1002/chem.201100316
Khazalpour, S., Safarifard, V., Morsali, A., and Nematollahi, D. (2015). Electrochemical synthesis of pillared layer mixed ligand metal–organic framework: DMOF-1–Zn. RSC Adv. 5, 36547–36551. doi:10.1039/c5ra04446d
Kirchon, A., Feng, L., Drake, H. F., Joseph, E. A., and Zhou, H.-C. (2018). From fundamentals to applications: a toolbox for robust and multifunctional MOF materials. Chem. Soc. Rev. 47, 8611–8638. doi:10.1039/c8cs00688a
Koryakina, I. G., Bachinin, S. V., Gerasimova, E. N., Timofeeva, M. V., Shipilovskikh, S. A., Bukatin, A. S., et al. (2023). Microfluidic synthesis of metal-organic framework crystals with surface defects for enhanced molecular loading. Chem. Eng. J. 452, 139450. doi:10.1016/j.cej.2022.139450
Kumar, S., Mohan, B., Fu, C., Gupta, V., and Ren, P. (2023). Decoration and utilization of a special class of metal–organic frameworks containing the fluorine moiety. Coord. Chem. Rev. 476, 214876. doi:10.1016/j.ccr.2022.214876
Li, Z. Z., Chen, J. F., Liu, F., Liu, A. Q., Wang, Q., Sun, H. Y., et al. (2007). Study of UV-shielding properties of novel porous hollow silica nanoparticle carriers for avermectin. Pest Manag. Sci. Former. Pesticide Sci. 63, 241–246. doi:10.1002/ps.1301
Liang, K., Wang, R., Boutter, M., Doherty, C. M., Mulet, X., and Richardson, J. J. (2017). Biomimetic mineralization of metal–organic frameworks around polysaccharides. Chem. Commun. 53, 1249–1252. doi:10.1039/c6cc09680h
Liang, W., Xie, Z., Cheng, J., Xiao, D., Xiong, Q., Wang, Q., et al. (2021). A light-triggered pH-responsive metal–organic framework for smart delivery of fungicide to control Sclerotinia diseases of oilseed rape. ACS Nano 15, 6987–6997. doi:10.1021/acsnano.0c10877
Liang, Y., Wang, S., Jia, H., Yao, Y., Song, J., Dong, H., et al. (2022). Pectin functionalized metal-organic frameworks as dual-stimuli-responsive carriers to improve the pesticide targeting and reduce environmental risks. Colloids Surfaces B Biointerfaces 219, 112796. doi:10.1016/j.colsurfb.2022.112796
Liu, C., Wang, P., Liu, X., Yi, X., Zhou, Z., and Liu, D. (2019a). Multifunctional β-cyclodextrin MOF-derived porous carbon as efficient herbicides adsorbent and potassium fertilizer. ACS Sustain. Chem. Eng. 7, 14479–14489. doi:10.1021/acssuschemeng.9b01911
Liu, Y., Liu, M., Yin, D., Zhu, D., and Swihart, M. T. (2019b). A general and rapid room-temperature synthesis approach for metal sulphide nanocrystals with tunable properties. Nanoscale 11, 136–144. doi:10.1039/c8nr07483f
Liu, Y., Xie, X.-Y., Cheng, C., Shao, Z.-S., and Wang, H.-S. (2019c). Strategies to fabricate metal–organic framework (MOF)-based luminescent sensing platforms. J. Mater. Chem. C 7, 10743–10763. doi:10.1039/c9tc03208h
Lu, W., Wei, Z., Gu, Z.-Y., Liu, T.-F., Park, J., Park, J., et al. (2014). Tuning the structure and function of metal–organic frameworks via linker design. Chem. Soc. Rev. 43, 5561–5593. doi:10.1039/c4cs00003j
Ma, S., Wang, Y., Yang, X., Ni, B., and Lu, S. (2022). MOF hybrid for long-term pest management and micronutrient supply triggered with protease. ACS Appl. Mater. Interfaces 14, 17783–17793. doi:10.1021/acsami.2c01187
Ma, X., Li, L., Wang, S., Lu, M., Li, H., Ma, W., et al. (2016). Ammonia-treated porous carbon derived from ZIF-8 for enhanced CO 2 adsorption. Appl. Surf. Sci. 369, 390–397. doi:10.1016/j.apsusc.2016.01.274
Ma, X., Pang, C., Li, S., Li, J., Wang, M., Xiong, Y., et al. (2021). Biomimetic synthesis of ultrafine mixed-valence metal–organic framework nanowires and their application in electrochemiluminescence sensing. ACS Appl. Mater. Interfaces 13, 41987–41996. doi:10.1021/acsami.1c10074
Ma, Y., Zhao, K., Ding, Y., Wu, S., Liao, X., Yin, X., et al. (2023). A facile one-pot route to fabricate clothianidin-loaded ZIF-8 nanoparticles with biocompatibility and long-term efficacy. Pest Manag. Sci. 79, 2603–2610. doi:10.1002/ps.7440
McKinstry, C., Cathcart, R. J., Cussen, E. J., Fletcher, A. J., Patwardhan, S. V., and Sefcik, J. (2016). Scalable continuous solvothermal synthesis of metal organic framework (MOF-5) crystals. Chem. Eng. J. 285, 718–725. doi:10.1016/j.cej.2015.10.023
Mejías, F. J., Trasobares, S., Varela, R. M., Molinillo, J. M., Calvino, J. J., and Macías, F. A. (2021). One-step encapsulation of ortho-disulfides in functionalized zinc MOF. Enabling metal–organic frameworks in agriculture. ACS Appl. Mater. Interfaces 13, 7997–8005. doi:10.1021/acsami.0c21488
Meng, W., Gao, Y., Tian, Z., Xu, W., Cheng, J., Li, S., et al. (2021). Fe3O4 magnetic cores coated with metal–organic framework shells as collectable composite nanoparticle vehicles for sustained release of the pesticide imidacloprid. ACS Appl. Nano Mater. 4, 5864–5870. doi:10.1021/acsanm.1c00800
Meng, W., Tian, Z., Yao, P., Fang, X., Wu, T., Cheng, J., et al. (2020). Preparation of a novel sustained-release system for pyrethroids by using metal-organic frameworks (MOFs) nanoparticle. Colloids Surfaces A Physicochem. Eng. Aspects 604, 125266. doi:10.1016/j.colsurfa.2020.125266
Merino, D., Tomadoni, B., Salcedo, M. F., Mansilla, A. Y., Casalongué, C. A., and Alvarez, V. A. (2020). Nanoclay as carriers of bioactive molecules applied to agriculture. Handb. Nanomater. nanocomposites energy Environ. Appl., 1–22. doi:10.1007/978-3-030-11155-7_62-1
Mirzaie, A., Musabeygi, T., and Afzalinia, A. (2017). Sonochemical synthesis of magnetic responsive Fe3O4@ TMU-17-NH2 composite as sorbent for highly efficient ultrasonic-assisted denitrogenation of fossil fuel. Ultrason. Sonochemistry 38, 664–671. doi:10.1016/j.ultsonch.2016.08.013
Mohan, B., Kamboj, A., Singh, K., Singh, G., Pombeiro, A. J., Ren, P., et al. (2023a). Metal-organic frameworks (MOFs) materials for pesticides, heavy metals, and drugs removal: environmental safety. Sep. Purif. Technol. 310, 123175. doi:10.1016/j.seppur.2023.123175
Mohan, B., Ma, S., Kumar, S., Yang, Y., and Ren, P. (2023b). Tactile sensors: hydroxyl decorated silver metal–organic frameworks for detecting Cr2O72–, MnO4–, humic acid, and Fe3+ ions. ACS Appl. Mater. Interfaces 15, 17317–17323. doi:10.1021/acsami.2c22871
Mohan, B., Singh, G., Chauhan, A., Pombeiro, A. J., and Ren, P. (2023c). Metal-organic frameworks (MOFs) based luminescent and electrochemical sensors for food contaminant detection. J. Hazard. Mater. 453, 131324. doi:10.1016/j.jhazmat.2023.131324
Mueller, U., Schubert, M., Teich, F., Puetter, H., Schierle-Arndt, K., and Pastre, J. (2006). Metal–organic frameworks—prospective industrial applications. J. Mater. Chem. 16, 626–636. doi:10.1002/chin.200623294
Muratović, S., Karadeniz, B., Stolar, T., Lukin, S., Halasz, I., Herak, M., et al. (2020). Impact of dehydration and mechanical amorphization on the magnetic properties of Ni (ii)-MOF-74. J. Mater. Chem. C 8, 7132–7142. doi:10.1039/d0tc00844c
Nadar, S. S., and Rathod, V. K. (2018). Encapsulation of lipase within metal-organic framework (MOF) with enhanced activity intensified under ultrasound. Enzyme Microb. Technol. 108, 11–20. doi:10.1016/j.enzmictec.2017.08.008
Nair, R., Varghese, S. H., Nair, B. G., Maekawa, T., Yoshida, Y., and Kumar, D. S. (2010). Nanoparticulate material delivery to plants. Plant Sci. 179, 154–163. doi:10.1016/j.plantsci.2010.04.012
Nhemachena, C., Matchaya, G., Nhemachena, C. R., Karuaihe, S., Muchara, B., and Nhlengethwa, S. (2018). Measuring baseline agriculture-related sustainable development goals index for Southern Africa. Sustainability 10, 849. doi:10.3390/su10030849
Nie, Z., Zhang, Y., Tang, R., and Wang, X. (2022). Biomimetic mineralization: an emerging organism engineering strategy for biomedical applications. J. Inorg. Biochem. 232, 111815. doi:10.1016/j.jinorgbio.2022.111815
Pandey, K., Omar, R. A., Verma, N., and Gupta, G. (2024). Fe-carbon nanofiber-modified Mo-MOF for the controlled release and translocation of micronutrients in plants. Environ. Sci. Nano. doi:10.1039/d3en00833a
Petrovic, S. M., and Barbinta-Patrascu, M.-E. (2023). Organic and biogenic nanocarriers as bio-friendly systems for bioactive compounds’ delivery: state-of-the art and challenges. Materials 16, 7550. doi:10.3390/ma16247550
Pichon, A., Lazuen-Garay, A., and James, S. L. (2006). Solvent-free synthesis of a microporous metal–organic framework. CrystEngComm 8, 211–214. doi:10.1039/b513750k
Pretty, J., and Pervez Bharucha, Z. (2015). Integrated pest management for sustainable intensification of agriculture in Asia and Africa. Insects 6, 152–182. doi:10.3390/insects6010152
Qian, Q., Asinger, P. A., Lee, M. J., Han, G., Mizrahi Rodriguez, K., Lin, S., et al. (2020). MOF-based membranes for gas separations. Chem. Rev. 120, 8161–8266. doi:10.1021/acs.chemrev.0c00119
Rautenberg, M., Gernhard, M., Radnik, J., Witt, J., Roth, C., and Emmerling, F. (2022). Mechanochemical synthesis of fluorine-containing co-doped zeolitic imidazolate frameworks for producing electrocatalysts. Front. Chem. 10, 840758. doi:10.3389/fchem.2022.840758
Ray, M. K., Mishra, A. K., Mohanta, Y. K., Mahanta, S., Chakrabartty, I., Kungwani, N. A., et al. (2023). Nanotechnology as a promising tool against phytopathogens: a futuristic approach to agriculture. Agriculture 13, 1856. doi:10.3390/agriculture13091856
Rojas Macías, S., Rodríguez Diéguez, A., and Horcajada, P. (2022). Metal− organic frameworks in agriculture. ACS Appl. Mater. Interfaces 14, 16983–17007. doi:10.1021/acsami.2c00615
Sarlak, N., Taherifar, A., and Salehi, F. (2014). Synthesis of nanopesticides by encapsulating pesticide nanoparticles using functionalized carbon nanotubes and application of new nanocomposite for plant disease treatment. J. Agric. food Chem. 62, 4833–4838. doi:10.1021/jf404720d
Shakiba, S., Astete, C. E., Paudel, S., Sabliov, C. M., Rodrigues, D. F., and Louie, S. M. (2020). Emerging investigator series: polymeric nanocarriers for agricultural applications: synthesis, characterization, and environmental and biological interactions. Environ. Sci. Nano 7, 37–67. doi:10.1039/c9en01127g
Shan, Y., Cao, L., Muhammad, B., Xu, B., Zhao, P., Cao, C., et al. (2020a). Iron-based porous metal–organic frameworks with crop nutritional function as carriers for controlled fungicide release. J. colloid interface Sci. 566, 383–393. doi:10.1016/j.jcis.2020.01.112
Shan, Y., Xu, C., Zhang, H., Chen, H., Bilal, M., Niu, S., et al. (2020b). Polydopamine-modified metal–organic frameworks, NH2-Fe-MIL-101, as pH-sensitive nanocarriers for controlled pesticide release. Nanomaterials 10, 2000. doi:10.3390/nano10102000
Singh, R., White, J. F., de Vries, M., Beddome, G., Dai, M., Bean, A. G., et al. (2022). Biomimetic metal-organic frameworks as protective scaffolds for live-virus encapsulation and vaccine stabilization. Acta Biomater. 142, 320–331. doi:10.1016/j.actbio.2022.02.002
Son, W.-J., Kim, J., Kim, J., and Ahn, W.-S. (2008). Sonochemical synthesis of MOF-5. Chem. Commun., 6336–6338. doi:10.1039/b814740j
Srinivasan, R., Elaiyappillai, E., Nixon, E. J., Lydia, I. S., and Johnson, P. M. (2020). Enhanced electrochemical behaviour of Co-MOF/PANI composite electrode for supercapacitors. Inorganica Chim. acta 502, 119393. doi:10.1016/j.ica.2019.119393
Stock, N., and Biswas, S. (2012). Synthesis of metal-organic frameworks (MOFs): routes to various MOF topologies, morphologies, and composites. Chem. Rev. 112, 933–969. doi:10.1021/cr200304e
Su, J., He, W., Li, X.-M., Sun, L., Wang, H.-Y., Lan, Y.-Q., et al. (2020). High electrical conductivity in a 2D MOF with intrinsic superprotonic conduction and interfacial pseudo-capacitance. Matter 2, 711–722. doi:10.1016/j.matt.2019.12.018
Tang, J., Tang, G., Niu, J., Yang, J., Zhou, Z., Gao, Y., et al. (2021). Preparation of a porphyrin metal–organic framework with desirable photodynamic antimicrobial activity for sustainable plant disease management. J. Agric. food Chem. 69, 2382–2391. doi:10.1021/acs.jafc.0c06487
Tranchemontagne, D. J., Hunt, J. R., and Yaghi, O. M. (2008). Room temperature synthesis of metal-organic frameworks: MOF-5, MOF-74, MOF-177, MOF-199, and IRMOF-0. Tetrahedron 64, 8553–8557. doi:10.1016/j.tet.2008.06.036
Usman, M., Farooq, M., Wakeel, A., Nawaz, A., Cheema, S. A., ur Rehman, H., et al. (2020). Nanotechnology in agriculture: current status, challenges and future opportunities. Sci. Total Environ. 721, 137778. doi:10.1016/j.scitotenv.2020.137778
Van Assche, T. R., Campagnol, N., Muselle, T., Terryn, H., Fransaer, J., and Denayer, J. F. (2016). On controlling the anodic electrochemical film deposition of HKUST-1 metal–organic frameworks. Microporous Mesoporous Mater. 224, 302–310. doi:10.1016/j.micromeso.2015.11.060
Varsha, M., and Nageswaran, G. (2020). Review—direct electrochemical synthesis of metal organic frameworks. J. Electrochem. Soc. 167, 155527. doi:10.1149/1945-7111/abc6c6
Wang, Z., Miao, R., He, L., Guan, Q., and Shi, Y. (2022). Green synthesis of MIL-100 (Fe) derivatives and revealing their structure-activity relationship for 2, 4-dichlorophenol photodegradation. Chemosphere 291, 132950. doi:10.1016/j.chemosphere.2021.132950
Wu, C., Dan, Y., Tian, D., Zheng, Y., Wei, S., and Xiang, D. (2020). Facile fabrication of MOF (Fe)@ alginate aerogel and its application for a high-performance slow-release N-fertilizer. Int. J. Biol. Macromol. 145, 1073–1079. doi:10.1016/j.ijbiomac.2019.09.200
Wu, K., Du, C., Ma, F., Shen, Y., Liang, D., and Zhou, J. (2019). Degradation of metal-organic framework materials as controlled-release fertilizers in crop fields. Polymers 11, 947. doi:10.3390/polym11060947
Wu, K., Xu, X., Ma, F., and Du, C. (2022). Fe-based metal–organic frameworks for the controlled release of fertilizer nutrients. ACS omega 7, 35970–35980. doi:10.1021/acsomega.2c05093
Xie, X., Wang, Y., Siu, S.-Y., Chan, C.-W., Zhu, Y., Zhang, X., et al. (2022). Microfluidic synthesis as a new route to produce novel functional materials. Biomicrofluidics 16, 041301. doi:10.1063/5.0100206
Xu, A.-W., Ma, Y., and Cölfen, H. (2007). Biomimetic mineralization. J. Mater. Chem. 17, 415–449. doi:10.1039/b611918m
Xu, C., Cao, L., Liu, T., Chen, H., and Li, Y. (2023). pH-responsive copper-doped ZIF-8 MOF nanoparticles for enhancing the delivery and translocation of pesticides in wheat plants. Environ. Sci. Nano 10, 2578–2590. doi:10.1039/d3en00300k
Yaghi, O., and Li, H. (1995). Hydrothermal synthesis of a metal-organic framework containing large rectangular channels. J. Am. Chem. Soc. 117, 10401–10402. doi:10.1021/ja00146a033
Yang, J., Dai, D., Cai, Z., Liu, Y.-Q., Qin, J.-C., Wang, Y., et al. (2021). MOF-based multi-stimuli-responsive supramolecular nanoplatform equipped with macrocycle nanovalves for plant growth regulation. Acta Biomater. 134, 664–673. doi:10.1016/j.actbio.2021.07.050
Yang, J., Trickett, C. A., Alahmadi, S. B., Alshammari, A. S., and Yaghi, O. M. (2017). Calcium L-lactate frameworks as naturally degradable carriers for pesticides. J. Am. Chem. Soc. 139, 8118–8121. doi:10.1021/jacs.7b04542
Yang, S. J., Kim, T., Lee, K., Kim, Y. S., Yoon, J., and Park, C. R. (2014). Solvent evaporation mediated preparation of hierarchically porous metal organic framework-derived carbon with controllable and accessible large-scale porosity. Carbon 71, 294–302. doi:10.1016/j.carbon.2014.01.056
Zare, H., Ahmadi, S., Ghasemi, A., Ghanbari, M., Rabiee, N., Bagherzadeh, M., et al. (2021). Carbon nanotubes: smart drug/gene delivery carriers. Int. J. nanomedicine 16, 1681–1706. doi:10.2147/ijn.s299448
Zeng, R., He, T., Lu, L., Li, K., Luo, Z., and Cai, K. (2021). Ultra-thin metal–organic framework nanosheets for chemo-photodynamic synergistic therapy. J. Mater. Chem. B 9, 4143–4153. doi:10.1039/d1tb00528f
Zhao, P., Cao, L., Wang, C., Zheng, L., Li, Y., Cao, C., et al. (2022). Metabolic pathways reveal the effect of fungicide loaded metal-organic frameworks on the growth of wheat seedlings. Chemosphere 307, 135702. doi:10.1016/j.chemosphere.2022.135702
Keywords: sustainable agriculture, MOF, nano-carriers, insecticide, control release, slow release, agrochemicals
Citation: Basak S, Bhattacharyya P, Pudake RN, Lokhande PE, Rednam U and Chakrabarti S (2024) Metal-organic framework as nanocarriers for agricultural applications: a review. Front. Nanotechnol. 6:1385981. doi: 10.3389/fnano.2024.1385981
Received: 14 February 2024; Accepted: 08 April 2024;
Published: 26 April 2024.
Edited by:
Ruchi Agrawal, TERI Gram, IndiaReviewed by:
Badreddine Channab, University of Hassan II Casablanca, MoroccoBrij (Briz) Mohan, University of Lisbon, Portugal
Copyright © 2024 Basak, Bhattacharyya, Pudake, Lokhande, Rednam and Chakrabarti. This is an open-access article distributed under the terms of the Creative Commons Attribution License (CC BY). The use, distribution or reproduction in other forums is permitted, provided the original author(s) and the copyright owner(s) are credited and that the original publication in this journal is cited, in accordance with accepted academic practice. No use, distribution or reproduction is permitted which does not comply with these terms.
*Correspondence: Ramesh Namdeo Pudake, cm5wdWRha2VAYW1pdHkuZWR1; Sandip Chakrabarti, c2NoYWtyYWJhcnRpQGFtaXR5LmVkdQ==