- 1State Scientific and Production Association “Optics, Optoelectronics and Laser Technology”, Minsk, Belarus
- 2Department of Optics and General Physics, Francisk Skorina Gomel State University, Gomel, Belarus
The DNA molecule is considered as an object of nature-like technologies, with the focus on the special electromagnetic properties of DNA-like helices. This is the difference from the traditional approach to the DNA molecule as the repository of genetic information. DNA-like helices are regarded as artificial micro-resonators, or “meta-atoms,” exhibiting both dielectric and magnetic properties, that are equally pronounced. The article presents methods for creating spatial structures directly from DNA molecules, as well as from DNA-like helices. It is shown that the design of metamaterials and metasurfaces should be carried out considering the special electromagnetic properties of DNA-like helices. This will make it possible to obtain the required properties of metamaterials and metasurfaces and achieve advantages over other types of artificial structures.
Introduction
The DNA molecule is traditionally and reasonably viewed as the repository of genetic information created by nature (Watson and Crick, 1953; Watson et al., 2007). However, there is yet a broader approach to the DNA molecule as an object of nature-like technologies in various fields of science and technology, including electrodynamics. Using nature-like technologies stems from the fact that the natural world inspired many human innovations, as demonstrated in (O’Connell, 2016; Al-Habaibeh, 2022). Electrodynamics does not remain aloof from nature-like technologies. In particular, it is possible to show that DNA-like helices can exhibit special electromagnetic properties that are exotic to those of most molecules. DNA-like helices can be of various sizes, from centimeters to nanometers, but must be scalable to preserve the relative values of the radius and pitch of the helix. When using DNA-like helices in optical and radio engineering devices, it is necessary to employ the method of not only geometric, but also electrodynamic similarity. This means that the wavelength used of the electromagnetic field should be approximately equal to the length of the DNA-like helix turn. Under the electromagnetic wave, equally significant electric dipole moment and magnetic moment can arise in each half of turn of the double helix (Semchenko et al., 2006; Semchenko et al., 2007; Semchenko et al., 2010a; Semchenko et al., 2010b). This equality of the electric dipole moment and the magnetic moment is not typical for the molecules of natural substances; it allows us to consider DNA-like helices as artificial “meta-atoms” that exhibit both dielectric and magnetic properties. Hence, a metamaterial or metasurface based on DNA-like helices will also be special in terms of their dielectric and magnetic properties. Therefore, the design of metamaterials and metasurfaces should be made considering special electromagnetic properties of DNA-like helices. This will make it possible to obtain the required properties of metamaterials and metasurfaces and achieve the advantages of the resulting optical and radio engineering devices, for example, absorbers, polarization converters and phase manipulators of electromagnetic waves.
Structural DNA nanotechnology
DNA has a number of properties, including the ability to self-assemble, which make it ideal for creating two-dimensional and three-dimensional nanoscale artificial structures (Seeman, 2010; Simmons et al., 2016; LuSimon et al., 2023). Therefore, structural DNA nanotechnology has become a promising field in recent years, using DNA as a molecular building block for predictable self-assembly. Structural DNA nanotechnology combines branched DNA junctions with sticky-ended cohesion to create self-assembling macromolecular architectures. One of the key goals of structural DNA nanotechnology is to construct three-dimensional (3D) crystalline lattices. Artificial crystals created in this way are usually considered as an object for solving problems inherent in structural studies. In addition, one of the main factors that prompted the emergence of structural DNA nanotechnology was the ability of DNA as a material that can be used for the precise organization of many other chemical compounds, ranging from small molecules and peptides to nanoparticles, proteins and viruses.
Figure 1 shows a two-dimensional lattice obtained with structural DNA nanotechnology. This is just one example of artificial DNA structures designed and created by Nadrian C. Seeman and researchers belonging to his school of thought.
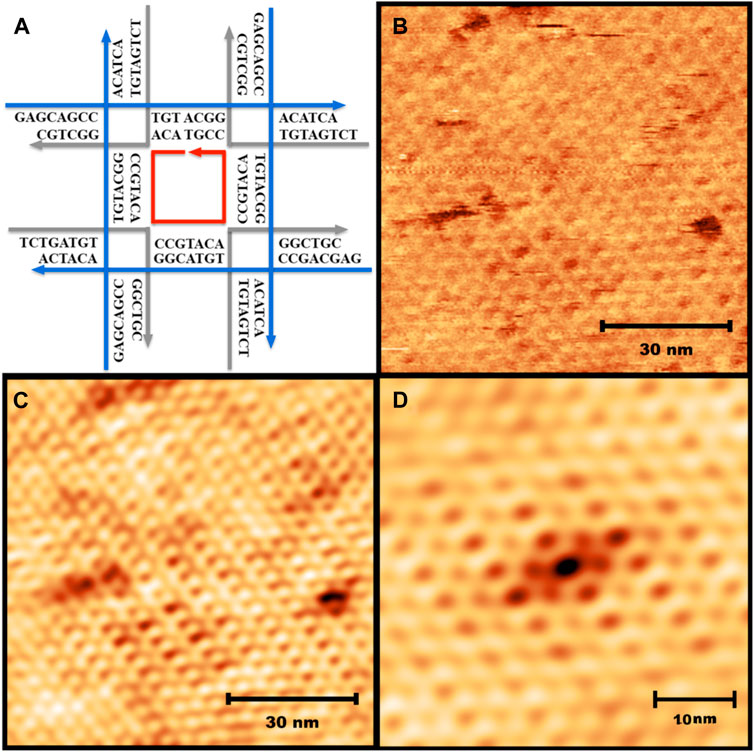
FIGURE 1. The 2D lattice with the 7 nucleotide spacing. (A) A schematic of the design. (B) The raw AFM image of the lattice generated by the system. (C) A Fourier-Flattened Image of (B). (D) The autocorrelation function of (C). Reprinted with permission from Simmons et al.(2016). Copyright 2016 American Chemical Society.
The method proposed by Nadrian C. Seeman had a large following in other countries, including Russia (Yevdokimov and Sytchev, 2008).
Using terminology from another modern and rapidly developing scientific field, namely the field of metamaterials, the artificial structure shown in Figure 1 can be regarded as a metasurface (Asadchy et al., 2016; Asadchy et al., 2018). It is possible to use such metasurfaces to control electromagnetic waves as a result of the effective interaction of waves with an extremely thin layer of micro-resonators. The manipulation of waves involves a wide range of operations, including absorption, anomalous reflection, rotation of the polarization plane and changes in the phase of the waves. For example, it was already noted in (Yevdokimov and Sytchev, 2008) that nanostructures with controlled physicochemical properties included in polymer films (hydrogels) can be used in modern optical and electronic devices (in particular, as optical filters). To manipulate waves, it is possible to use both dielectric and electrically conductive metasurfaces. As for DNA molecules, their electrical conductivity has not yet been fully studied, and currently the understanding of the DNA properties as a non-linear semiconductor prevails, which is confirmed by experimental studies (Wohlgamuth et al., 2013). At the same time, with any type of DNA electrical conductivity, the conductive properties of individual molecules and entire structures can be enhanced, for example, by metallization. Paper (Chen et al., 2018) presents a comprehensive review which summarizes the research activities on DNA metallization that have appeared since the concept was first proposed in 1998. This review is of great interest to promote the further development of DNA metallization by attracting researchers from various communities, including chemistry, biology, physiology, material science, and nanotechnology as well as other disciplines.
Resonant wavelengths possible for DNA helices
The previous section considers technologies that make it possible to create metamaterials and metasurfaces directly from DNA molecules. Such structures should be studied and used in devices to control electromagnetic waves at their characteristic resonant frequencies. Metamaterials and metasurfaces may have different resonant frequencies, where their properties are most pronounced. These resonant frequencies, or frequencies of the eigenmodes of electromagnetic field oscillations, are associated with both the internal molecular structure of DNA and its periodic helical structure. It is well known (Ploeser and Loring, 1949; Voet et al., 1963; Rodger and Roberts, 2013) that the DNA molecule has an absorption band in the ultraviolet range near wavelengths
This characteristic DNA molecular absorption band arises from properties of purine and pyridine bases and is primarily used for DNA quantification analysis. It should also be noted that circular dichroism spectroscopy is often used to analyze the structure of chiral molecules, including DNA (Greenfield, 2006).
Therefore, the operating wavelength of optical devices based on DNA structures should be close to the indicated wavelength
where L is the full length of the helix segment in the straightened state, n is the integer denoting the ordinal number of the resonance,
For a very long helix that contains a large number of turns, another type of resonance is possible, at which the resonant wavelength is approximately equal to the length of the helix turn
The length of the helix turn can be calculated according to the formula
where r is the radius of the helix turn, h is the helix pitch; the total length of the helix in the straightened state is
As the experimental data show (Watson and Crick, 1953; Watson et al., 2007), a real DNA helix has the following parameters:
It is the strong absorption of electromagnetic waves in the extreme ultraviolet range that leads to the fact that the properties of various objects, including DNA molecules, have not yet been sufficiently studied for these wavelengths. The fundamental theoretical approaches for describing the properties of substances in the extreme ultraviolet range are well known. For example, the classic textbook on theoretical physics (Landau et al., 2013) states that the dielectric permittivity of substances at very high frequencies tends to unity in the limit. Here, we are not talking about the identical equality of the dielectric permittivity of substances to a unit value, namely, the limiting transition to unity. The actual scope of applicability of the limiting formula for
It is also important to note article (Kolpakov et al., 1978), which outlines the fundamentals of the theory and problems of studying the dielectric properties of the medium in the X-ray and gamma ranges. One of the focus points of the article (Kolpakov et al., 1978) is the approximate relation
Visualization of biological materials and cells can be achieved using X-ray scattering and diffraction. Paper (Hémonnot and Köster, 2017) describes the advances in X-ray scattering and diffraction techniques for imaging biological systems at the nanoscale. Different successful approaches, including scanning and full-field techniques, along with prominent examples, are compared. Recent studies that combined several techniques in one experiment in order to collect highly complementary data for a multidimensional sample characterization, are presented. However, article (Hémonnot and Köster, 2017) covers research performed with X-ray energies above 5 keV, which is referred to as hard X-rays. At the same time, radiation with a wavelength
Precise 3D nanostructuring technique based on the rolling-up of strained nanofilms
Metamaterials and metasurfaces can be created not only using the DNA molecules with the help of structural DNA nanotechnology, for example. Also of interest is producing metamaterials and metasurfaces based on DNA-like helices. These helices, considered as meta-atoms, can be scaled considering their geometric and electrodynamic similarity to the natural DNA helix. For such helices, which are larger than the real DNA helix, the resonant wavelengths of the electromagnetic field that satisfy conditions 2) and 3) will also increase. Such an increase of the resonant wavelength of individual DNA-like helices and metamaterials (created on the basis of these helices) would make it possible to create devices to control electromagnetic waves in various frequency ranges, including optical and terahertz ranges.
Metamaterials and metasurfaces with a helical structure for the terahertz frequency range can be produced via precise 3D nanostructuring technique based on the rolling-up of strained nanofilms. This breakthrough method was developed by Russian scientists under the supervision of Academician V. Ya. Prinz and received international commendation as Prinz technology (Prinz et al., 2000; Prinz and Golod, 2006; Naumova et al., 2009). The method makes it possible to control the folding of layered strained films of nanometer thickness. Such folding results in formation of micro-sized helices, which have electrical conductivity due to the presence of a conductive layer, e.g., a layer of gold. The folding of the films is manageable, which allows obtaining micro-helices with a predetermined turn radius and pitch.
Thus, it is possible to design and create micro-helices where the electric dipole moment and the magnetic moment will be equally significant and even equal to each other in magnitude. Such helices can be called balanced (in terms of their electrical and magnetic properties) or optimal. The resulting metamaterial formed by such helical meta-atoms will have both dielectric and magnetic properties (I Semchenko et al., 2014; Semchenko et al., 2017). In other words, such a metamaterial will be exotic compared to natural substances familiar to us, which are either dielectrics or magnets. Using balanced micro-helices as a basis, it is possible to design and create a metamaterial for the terahertz frequency range with equal permittivity and magnetic permeability within the Prinz technology:
Such a metamaterial has a wave impedance approximately equal to the impedance of free space. Therefore, the reflection of terahertz waves for it is very weak, close to zero. At the same time, the metamaterial absorbs terahertz waves due to the presence of a conductive layer in each nanofilm folded into a helix. The combination of weak reflection and strong absorption of waves is a peculiar property of such a metamaterial relative to natural substances, as noted above.
Figure 2 shows a schematic of an individual helix and a SEM image of the described metamaterial which was formed by paired single-turn right- and left-handed helices. For each individual helix, Formula 2 can be applied at n = 1, i.e., the half-wave resonance condition is satisfied. Since the length of each helix in the straightened state is 77 μm, the operating frequency of the metamaterial is close to 2 THz. This metamaterial uses paired right- and left-handed helices, which, strictly, are not DNA-like. However, this method has no fundamental restrictions on the creation of paired helices with the same winding direction, similar to the DNA helix in the geometric and electrodynamic sense. Resonant wavelengths defined by Formulae 2, 3 will be possible for such helices as well as for metamaterials and metasurfaces which are based on these helices.
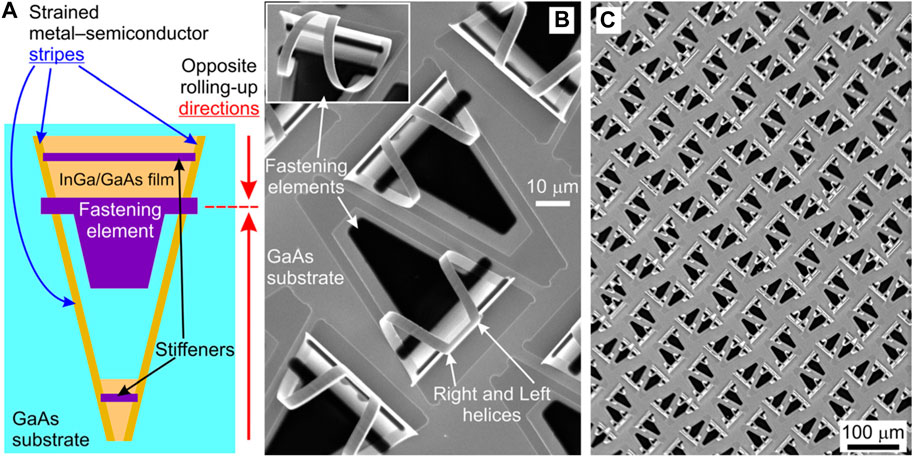
FIGURE 2. Metamaterial based on microsized paired right- and left-handed helices. (A) Schematic of the initial plane element made of strained multilayer nanofilm. Dark-yellow V-like strips with length of 77 µm and width of 3 µm are prepared from the In0.2Ga0.8As/GaAs/Ti/Au (16/40/4/40 nm) film. The orange crosspieces between the strips are made of the semiconductor InGaAs/GaAs film. The purple fastening element of negative photoresist keeps the helices on the substrate after the etching out of the underlying sacrificial layer. (B) and (C) SEM images of an array of right-handed and left-handed single-coil metal-semiconductor helices in top and oblique (inset) views. Reproduced from Semchenko et al. (2017), with permission from AIP Publishing.
Discussion
This article aims to draw attention to the DNA molecule not in the classic sense, not only as a repository of genetic information. In this research, an attempt is made to bring to the fore and to the discussion the electromagnetic properties of the DNA helix. DNA-like helices, scaled for their geometric and electrodynamic similarity to the real DNA molecule, can be used in a variety of devices to control electromagnetic fields. First of all, these are polarizers, absorbers, phase manipulators, as well as chiral near-field sources (Gansel et al., 2009; Slobozhanyuk et al., 2013; Schäferling et al., 2014; Ji et al., 2016; Kaschke and Wegener, 2016). Although the classic DNA-like helix is cylindrical and contains two strands, its promising applications have inspired researchers to develop new geometries. These include tapered double helix (Gansel et al., 2012; Zhao et al., 2012), N-intertwined helices, together with triple and quadruple helices, for which N = 3 and N = 4 (Yang et al., 2011; Esposito et al., 2014; Esposito et al., 2015; Kaschke and Wegener, 2015), as well as connected left and right helices following each other (Kaschke et al., 2015). To produce such helices and metamaterials on their basis, the most modern technologies are used, including structural DNA nanotechnology (Yevdokimov and Sytchev, 2008; Seeman, 2010; Simmons et al., 2016; LuSimon et al., 2023), precise 3D nanostructuring technique based on the rolling-up of strained nanofilms (Prinz et al., 2000; Prinz and Golod, 2006; Naumova et al., 2009; I Semchenko et al., 2014; Semchenko et al., 2017), direct laser writing (DLW) (Gansel et al., 2009; Gansel et al., 2012; Kaschke et al., 2015; Kaschke and Wegener, 2015), focused-ion-beam induced deposition (FIBID) (Esposito et al., 2014; Esposito et al., 2015), glancing-angle deposition (GLAD) (Singh et al., 2013).
This article represents a mini-review and, accordingly, has limited length and a small number of figures allowed, which can provide a fuller understanding of the structures created on the DNA basis and the technologies involved. Therefore, we can only mention some additional interesting directions, for example, DNA origami. Survey paper (Dey et al., 2021) describes the DNA origami technology methods, including origami design, synthesis, functionalization, and characterization. It also highlights the applications of origami structures in nanotechnology, nanophotonics and nanoelectronics, computer calculations, molecular machines, drug delivery, and biophysics. At the same time, various problems such as size restrictions, stability issues and the production scale were identified and discussed in order to arrive at workable solutions. Next comes an overview of the next-generation DNA origami methods that will allow in vivo synthesis and large-scale production.
Another interesting area is the synthesis of metal clusters using DNA as a template. Survey paper (Ijiro and Mitomo, 2017) discusses and compares various techniques for producing DNA-templated metal nanoarchitecture. The techniques include DNA-mediated metal nanoparticle formation, DNA-templated conductive nanowire production via metal depositions, sequence-selective metal deposition onto DNA to elaborate nanowire production and DNA brushes as templates to be used on solid substrates. Article (Molotsky et al., 2010), in particular, observes chiroptical properties in small silver nanoparticles of <2 nm grown on a double-stranded DNA template. Paper (Petty et al., 2004) provides major conclusions stating that DNA acts as a template for the time-dependent and size-specific formation of nanoclusters because the ions of silver have a strong interaction with the DNA strands. Article (Yang et al., 2020) discusses the DNA-templated silver nanoclusters in terms of synthesis and fluorescence properties, and summarizes their biological applications for fluorescence sensing and imaging.
The resulting metamaterials are designed for various frequency ranges of the electromagnetic field, including microwave, terahertz, infrared and visible. Considering the list of all these methods and the wide spectral range of application of metamaterials, it can be concluded that DNA-like helices play a significant role in nature-like technologies.
Author contributions
IS prepared the material by sections: introduction, structural DNA nanotechnology, resonant wavelengths possible for DNA helices, discussion. SK prepared the material by sections: precise 3D nanostructuring technique based on the rolling-up of strained nanofilms, discussion.
Acknowledgments
The article was partially completed within the framework of task 3.04.2 of the State Research Program “Convergence-2025” and projects F23KI-027 and F22KITG-021 of the Belarusian Republican Foundation for Basic Research.
Conflict of interest
The authors declare that the research was conducted in the absence of any commercial or financial relationships that could be construed as a potential conflict of interest.
Publisher’s note
All claims expressed in this article are solely those of the authors and do not necessarily represent those of their affiliated organizations, or those of the publisher, the editors and the reviewers. Any product that may be evaluated in this article, or claim that may be made by its manufacturer, is not guaranteed or endorsed by the publisher.
References
Al-Habaibeh, A. (2022). Five human technologies inspired by nature from velcro to racing cars. Conversat. https://theconversation.com/five-human-technologies-inspired-by-nature-from-velcro-to-racing-cars-195593.30 December 2022.
Asadchy, V. S., Albooyeh, M., Tcvetkova, S. N., D´ıaz-Rubio, A., Ra’di, Y., and Tretyakov, S. A. (2016). Perfect control of reflection and refraction using spatially dispersive metasurfaces. Phys. Rev. B 94, 075142. doi:10.1103/physrevb.94.075142
Asadchy, V. S., Díaz-Rubio, A., and Tretyakov, S. A. (2018). Bianisotropic metasurfaces: Physics and applications. Nanophotonics 7 (6), 1069–1094. doi:10.1515/nanoph-2017-0132
Lu, B., Simon, V., Ohayon, Y. P., Woloszyn, K., Markus, T., and Mao, C., Highly symmetric, self-assembling 3D DNA crystals with cubic and trigonal lattices. Small. 19, 3, 2023, 2205830, doi:10.1002/smll.202205830
Chen, Z., Liu, C., Cao, F., Ren, J., and Qu, X. (2018). DNA metallization: Principles, methods, structures, and applications. Chem. Soc. Rev. 47 (11), 4017–4072. doi:10.1039/c8cs00011e
Dey, S., Fan, C., Gothelf, K. V., Li, J., Lin, C., Liu, L., et al. (2021). DNA origami. Nat. Rev. Methods Prim. 1 (1), 13. doi:10.1038/s43586-020-00009-8
Esposito, M., Tasco, V., Todisco, F., Benedetti, A., Sanvitto, D., and Passaseo, A. (2014). Three dimensional chiral metamaterial nanospirals in the visible range by vertically compensated focused ion beam induced-deposition. Adv. Opt. Mater 2, 154–161. doi:10.1002/adom.201300323
Esposito, M., Tasco, V., Todisco, F., Cuscunà, M., Benedetti, A., Sanvitto, D., et al. (2015). Triple-helical nanowires by tomographic rotatory growth for chiral photonics. Nat. Commun. 6, 6484. doi:10.1038/ncomms7484
Gansel, J. K., Latzel, M., Frölich, A., Kaschke, J., Thiel, M., and Wegener, M. (2012). Tapered gold-helix metamaterials as improved circular polarizers. Appl. Phys. Lett. 100, 101109. doi:10.1063/1.3693181
Gansel, J. K., Thiel, M., Rill, M. S., Decker, M., Bade, K., Saile, V., et al. (2009). Gold helix photonic metamaterial as broadband circular polarizer. Science 325, 1513–1515. doi:10.1126/science.1177031
Greenfield, N. (2006). Using circular dichroism spectra to estimate protein secondary structure. Nat. Protoc. 1, 2876–2890. doi:10.1038/nprot.2006.202
Hémonnot, Clément Y. J., and Köster, Sarah (2017). Imaging of biological materials and cells by X-ray scattering and diffraction. ACS Nano 11, 8542–8559. doi:10.1021/acsnano.7b03447
I Semchenko, I. V., Khakhomov, S. A., Asadchy, V. S., Naumova, E. V., Prinz, V. Y., Golod, S. V., et al. (2014). Investigation of the properties of weakly reflective metamaterials with compensated chirality. Crystallogr. Rep. 59 (4), 480–485. doi:10.1134/S1063774514040178
Ijiro, K., and Mitomo, H. (2017). Metal nanoarchitecture fabrication using DNA as a biotemplate. Polym. J. 49 (12), 815–824. doi:10.1038/pj.2017.63
Ji, Ruonan, Wang, Shao-Wei, Liu, Xingxing, Guo, Huijie, and Lu, Wei (2016). Hybrid helix metamaterials for giant and ultra-wide circular dichroism. ACS Photonics. 3 (12), 2368–2374. doi:10.1021/acsphotonics.6b00575
Kaschke, J., Blume, L., Wu, L., Thiel, M., Bade, K., Yang, Z., et al. (2015). A helical metamaterial for broadband circular polarization conversion. Adv. Opt. Mater 3, 1411–1417. doi:10.1002/adom.201500194
Kaschke, J., and Wegener, M. (2015). Gold triple-helix mid-infrared metamaterial by STED-inspired laser lithography. Opt. Lett. 40, 3986–3989. doi:10.1364/ol.40.003986
Kaschke, Johannes, and Wegener, Martin (2016). Optical and infrared helical metamaterials. Nanophotonics 5, 510–523. doi:10.1515/nanoph-2016-0005
Kolpakov, A. V., Bushuev, V. A., and Kuz'min, R. N. (1978). Dielectric permittivity in the x-ray region. Sov. Phys. Usp. 21, 959–977. doi:10.1070/pu1978v021n11abeh005717
Landau, L., Bell, J., Kearsley, M., Pitaevskii, L., Lifshitz, E., and Sykes, J. (2013). Electrodynamics of continuous media. Pergamon Press, Oxford, UK.
Molotsky, T., Tamarin, T., Moshe, A. B., Markovich, G., and Kotlyar, A. B. (2010). Synthesis of chiral silver clusters on a DNA template. J. Phys. Chem. C 114 (38), 15951–15954. doi:10.1021/jp911968x
Naumova, E. V., Prinz, V. Ya., Golod, S. V., Seleznev, V. A., Soots, R. A., and Kubarev, V. V. (2009). Manufacturing chiral electromagnetic metamaterials by directional 10.1364/oe.19.004255 rolling of strained heterofilms. J. Opt. A Pure Appl. Opt. 11, 074010. doi:10.1088/1464-4258/11/7/074010
O’Connell, Cathal (2016). 10 technologies inspired by nature. Cosmos, Technology. Adelaide, Australia, https://cosmosmagazine.com/technology/10-technologies-inspired-by-nature/April 12, 2016).
Petty, J. T., Zheng, J., Hud, N. V., and Dickson, R. M. (2004). DNA-templated Ag nanocluster formation. J. Am. Chem. Soc. 126 (16), 5207–5212. doi:10.1021/ja031931o
Ploeser, J. McT., and Loring, H. S. (1949). The ultraviolet absorption spectra of the pyrimidine ribonucleosides and ribonucleotides. J. Biol. Chem. 178, 431–437. doi:10.1016/s0021-9258(18)56973-8
Prinz, V. Ya., and Golod, S. V. (2006). Elastic silicon-film-based nanoshells: Formation, properties, and applications. J. Appl. Mech. Tech. Phys. 47, 867–878. doi:10.1007/s10808-006-0126-9
Prinz, V. Ya., Seleznev, V. A., Gutakovsky, A. K., Chehovskiy, A. V., Preobrazenskii, V. V., Putyato, M. A., et al. (2000). Free-standing and overgrown InGaAs/GaAs nanotubes, nanohelices and their arrays. Phys. E 6, 828–831. doi:10.1016/S1386-9477(99)00249-0
Ramzan, Ullah, and Zheng, Yuxiang (2016). A review on why are spectroscopic techniques different below and above 200 nm. Opt. Instrum. 38 (6), 523–528.
Rodger, A. (2013). “UV absorbance spectroscopy of biological macromolecules,” in Encyclopedia of biophysics. Editor G. C. K. Roberts (Berlin, Germany: Springer), 2714–2718. doi:10.1007/978-3-642-16712-6_780
Schäferling, M., Yin, X., Engheta, N., and Giessen, H. (2014). Helical plasmonic nanostructures as prototypical chiral near-field sources. ACS Photonics 1, 530–537. doi:10.1021/ph5000743
Seeman, N. C. (2010). Nanomaterials based on DNA. Annu. Rev. Biochem. 79, 65–87. doi:10.1146/annurev-biochem-060308-102244
Semchenko, I. V., Balmakov, A. P., Khakhomov, S. A., et al. (2006). Polarization selectivity of electromagnetic radiation of DNA. Bianisotropics, 45–46.
Semchenko, I. V., Khakhomov, S. A., Asadchy, V. S., Golod, S. V., Naumova, E. V., Prinz, V. Y., et al. (2017). Investigation of electromagnetic properties of a high absorptive, weakly reflective metamaterial-substrate system with compensated chirality. J. Appl. Phys. 121, 015108-1–015108-8. doi:10.1063/1.4973679
Semchenko, I. V., Khakhomov, S. A., and Balmakov, A. P. (2010). Polarization selectivity of artificial anisotropic structures based on DNA-like helices. Crystallogr. Rep. 55 (6), 921–926. doi:10.1134/S1063774510060040
Semchenko, I. V., Khakhomov, S. A., and Balmakov, A. P. (2007). Polarization selectivity of electromagnetic radiation of deoxyribonucleic acid. J. Commun. Technol. Electron. 52 (9), 996–1001. doi:10.1134/S1064226907090070
Semchenko, I. V., Khakhomov, S. A., and Balmakov, A. P. (2010). Polarization selectivity of interaction of DNA molecules with X-ray radiation. Biophysics 55 (2), 194–198. doi:10.1134/S0006350910020053
Simmons, Chad R., Zhang, Fei, Birktoft, Jens J., Qi, Xiaodong, Han, Dongran, Liu, Yan, et al. (2016). Construction and structure determination of a three-dimensional DNA crystal. J. Am. Chem. Soc. 138. doi:10.1021/jacs.6b06508
Singh, J. H., Nair, G., Ghosh, A., and Ghosh, A. (2013). Wafer scale fabrication of porous three-dimensional plasmonic metamaterials for the visible region: Chiral and beyond. Nanoscale 5, 7224–7228. doi:10.1039/c3nr02666c
Slobozhanyuk, Alexey P., Lapine, Mikhail, Powell, David A., Shadrivov, Ilya V., Kivshar, Yuri S., McPhedran, Ross C, et al. (2013). Flexible helices for nonlinear metamaterials. Adv. Mat. 25, 3409–3412. doi:10.1002/adma.201300840
Voet, D., Gratzer, W. B., Cox, R. A., and Doty, P. (1963). Absorption spectra of nucleotides, polynucleotides, and nucleic acids in the far ultraviolet. Biopolymers 1, 193–208. doi:10.1002/bip.360010302
Watson, J. D., and Crick, F. H. C. (1953). Molecular structure of nucleic acids: A structure for deoxyribose nucleic acid. Nature 171, 737–738. doi:10.1038/171737a0
Watson, J. D., Baker, T. A., Bell, S. P., Gann, A. F. A., Levine, M., Losick, R. M., et al. (2007). Molecular biology of the gene. London, UK: Pearson. Available at: https://www.amazon.co.uk/Molecular-Biology-Gene-James-Watson/dp/0321507819.
Wohlgamuth, C. H., McWilliams, M. A., and Slinker, J. D. (2013). DNA as a molecular wire: Distance and sequence dependence. Anal. Chem. 85 (18), 8634–8640. doi:10.1021/ac401229q
Yang, M., Chen, X., Su, Y., Liu, H., Zhang, H., Li, X., et al. (2020). The fluorescent palette of DNA-templated silver nanoclusters for biological applications. Front. Chem. 8, 601621. doi:10.3389/fchem.2020.601621
Yang, Z., Zhao, M., and Lu, P. X. (2011). Improving the signal-to-noise ratio for circular polarizers consisting of helical metamaterials. Opt. Express 19, 4255–4260. doi:10.1364/oe.19.004255
Yevdokimov, Yu.M., and Sytchev, V. V. (2008). Principles of the design of nanostructures with nucleic acid molecules as building blocks. Russ. Chem. Rev. 77 (2), 193–204. doi:10.1070/RC2008v077n02ABEH003734
Keywords: DNA molecule, DNA-like helices, metasurface, electrodynamic similarity method, helical structure, nature-like technologies
Citation: Semchenko IV and Khakhomov SA (2023) Application of DNA molecules in nature- inspired technologies: a mini review. Front. Nanotechnol. 5:1185429. doi: 10.3389/fnano.2023.1185429
Received: 13 March 2023; Accepted: 17 April 2023;
Published: 28 April 2023.
Edited by:
Cristina Satriano, University of Catania, ItalyReviewed by:
Ivan Shishkin, ITMO University, RussiaCopyright © 2023 Semchenko and Khakhomov. This is an open-access article distributed under the terms of the Creative Commons Attribution License (CC BY). The use, distribution or reproduction in other forums is permitted, provided the original author(s) and the copyright owner(s) are credited and that the original publication in this journal is cited, in accordance with accepted academic practice. No use, distribution or reproduction is permitted which does not comply with these terms.
*Correspondence: Igor V. Semchenko, igor.semchenko@internet.ru