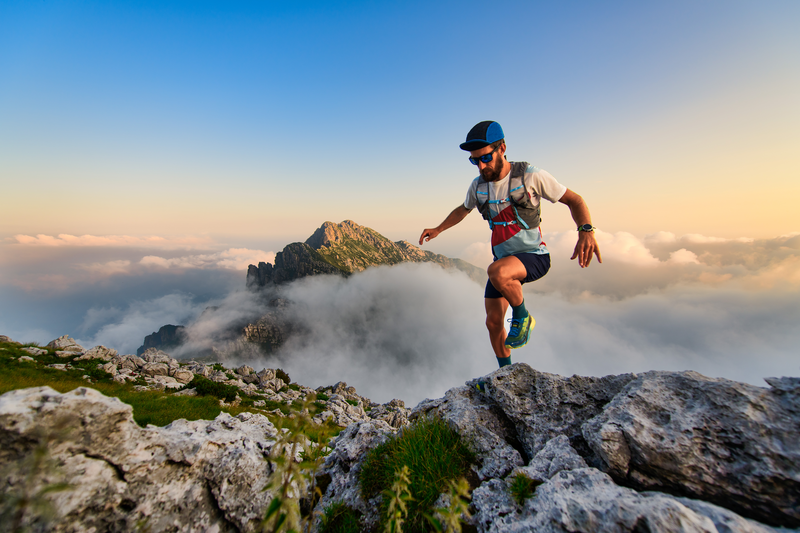
95% of researchers rate our articles as excellent or good
Learn more about the work of our research integrity team to safeguard the quality of each article we publish.
Find out more
ORIGINAL RESEARCH article
Front. Nanotechnol. , 03 February 2022
Sec. Environmental Nanotechnology
Volume 4 - 2022 | https://doi.org/10.3389/fnano.2022.805698
Objective: Water contaminated with heavy metals causes serious environmental issues for people. Mercury and chromium are considered to be extremely toxic for public health. Diversified technologies have been introduced to purify contaminated water; however, only a few become successful in reducing mercury and chromium below the permissible limit of drinking water (0.001 and 0.05 ppm, respectively, WHO/BIS Guidelines). Our main aim was to synthesize suitable nanoscale materials to make water potable by removing mercury and chromium from contaminated water while keeping essential elements as per the WHO/BIS guidelines.
Methods: Copper(II) oxide nanomaterials are synthesized following a wet chemical process as per earlier reported procedures. Nanoparticles with average diameter of 10–20 nm are formed. XRD confirms the formation of orthorhombic CuO nanoparticles.
Results: The nanoparticles are efficient in removing mercury from 200 ppb to less than 50 ppb (75%) and chromium from 200 ppb to less than 16 ppb (92%) over a wide range of pH.
Conclusion: The nanomaterials have great potential in removing toxic heavy metals from contaminated water. The materials can be applied to integrated water purification systems to produce potable water.
Water pollution has become a global problem with the rapid development of human civilization as well as progress in industrialization and technological development (Mahmoud et al., 2021; Gupta et al., 2021). It is believed that water pollution by heavy metals has attracted major concern because of their toxic, non-biodegradable, and persistent nature even at low concentrations (Dubey and Sharma, 2017; Kondabey et al., 2019; Gupta et al., 2016; Holmes et al., 2009; Xia et al., 2019). Inorganic contaminants containing heavy metals and metalloids, e.g., Hg, Pb, As, Cr, and Cd, in various forms have hazardous effects on humans, animals, and the environment (Sumesh et al., 2011; Raul et al., 2014; Zahir et al., 2005). Industrial waste is the principal contributor of water contamination by heavy metals. Among toxic metal ions, chromium causes many health issues due to its high toxicity, mutagenicity, and carcinogenic nature even at low concentrations (Zayed and Terry, 2003; Zahir et al., 2005; Zhitkovich, 2005; Yongsheng et al., 2011; Wolińska et al., 2013).
Chromium enters the ecosystem from various sources, viz. paints, the metal industry, clothing, dye, and leather tanning industries (Gupta et al., 2016; Gopal and Gupta, 2019). Both Cr(III) and Cr(VI) are stable forms, but their toxicity and chemical nature are quite different: Cr(VI) is more toxic than Cr(III) because of its good water solubility, bioavailability, and mobility (Gupta et al., 2016). Liver problems, skin diseases, ulcer, etc., are caused by chromium(VI) (Zahir et al., 2005). The maximum permissible limit of Cr(VI) in drinking water is 0.05 mg/L (regulated by the WHO/USEPA) (Gupta et al., 2016).
Mercury is another neurotoxic element that is extensively used in spite of its high toxicity (Holmes et al., 2009). Once released into the environment, it can combine with cysteine in human proteins and produce CH3Hg through sulfate methylation (Holmes et al., 2009; Xia et al., 2019). This causes high bioaccumulation of mercury in the food chain. Consumption of Hg(II) causes damage in the brain, heart, liver, kidney, and nervous system, and it even leads to cancer (Xia et al., 2019). The permissible limit of mercury is set at 0.001 mg/L by the World Health Organization (WHO), and mercury is found to have highest toxicity among metals and metalloids (Holmes et al., 2009).
Simple synthetic procedure and cost-effectiveness make adsorption methods the most attractive and feasible to remove heavy metals from water (Blue et al., 2008; Gupta et al., 2016). Owing to their high surface area (Subramaniam et al., 2019) (surface/volume ratio), nanomaterials can adsorb heavy metals effectively from contaminated water. Metal oxide nanoparticles are found to be good candidates for the adsorption of heavy metals (Salam et al., 2011; Sheet et al., 2014; Carolin et al., 2017; Duncan et al., 2018).
Among the metal oxide nanoparticles, CuO nanoparticles exhibit efficient antibacterial activity. Therefore, it is anticipated that CuO will be able to remove inorganic and biological contaminants from wastewater. Based on this, in the present work, we used previously prepared CuO nanoparticles (Raul et al., 2014) to remove Cr(VI) and Hg(II) from spiked water at room temperature. Various parameters, viz., initial concentration, adsorption isotherm, adsorbent dosage, pH, and competing ions, are studied here. Nano-CuO was used to remove the contaminants from water for its probable practical application.
During the total course of the study, double distilled water was used as per requirement. CuCl2 (Merck), HCl (SRL), NaOH (SRL), capping agent (SRL, Mumbai), potassium dichromate (Merck), mercuric chloride (Merck), acetone (Merck), and ethanol (BC Pvt. Ltd., Kolkata) were brought as AR (analytical grade) reagent and used as such.
Cupric oxide nanomaterials were synthesized according to our reported procedure (Raul et al., 2021). Cupric chloride (CuCl2), capping solvent, and NaOH were taken in 2:1:1 ratio in a round bottom flask with 250 ml ethanol. The mixture was heated for 16 h and allowed to cool at room temperature. The obtained black precipitate was separated by centrifugation and washed with alcohol and hot water repeatedly. Then, the product was allowed to dry at room temperature and utilized for adsorption experiments. Alcohol and hot water were used to wash out excess amounts of capping agent (polyethylene glycol) and alkali used during the synthesis of nanoparticles, respectively. It is suggested to wash repeatedly to confirm complete removal of unwanted substances and to use alcohol before hot water in the washing process to wash out excess alcohol. The CuO material was characterized by TEM and found to have particle size within 20 nm (Figure 1).
Stock solutions of standard chromium(VI) and mercury(II) of 1,000 mgL−1 were prepared separately in volumetric flasks (1 L) with double distilled water. Of each working standard of chromium(VI) and mercury(II), 100 ml was taken separately in 250 ml conical flasks, a known amount of adsorbent was added, and the mixture was studied for adsorption. The flasks were stirred for 3 h using a mechanical shaker (model: IKA 400 ic, make: IKA) for optimization. The solution was allowed to settle for a few hours, and the supernatant was analyzed for the presence of contaminants using an Atomic Absorption Spectrophotometer (AAS) (model: AA7000, make: LabIndia) with flame and hydride generation systems to measure chromium and mercury concentrations, respectively. For each case, 10 ml of aliquots was taken. All adsorption experiments were performed at room temperature (25°C ± 0.2°C). Various parameters, viz., adsorbent and adsorbate dosage, initial concentration, competing ions, and pH, were studied through batch adsorption.
The amount of contaminant adsorbed by the adsorbent was deduced by
Here, Qe is the equilibrium adsorption capacity (mgg−1) of the adsorbent; C0 and Ce are the initial and equilibrium concentrations of contaminants (Hg or Cr) (mgL−1), respectively; and V and W are the amount of the solution and weight (g) of the adsorbent, respectively (Viswanathan et al., 2009). Studies on the removal of metals vs pH variation were performed by adjusting the solution pH over a broad range (using 0.1 N HCl or 0.1 N NaOH). Copper oxide nanoparticle is found to be stable over the pH range of 5–10.5, and hence adsorption studies were performed in both acidic and basic conditions. Experiments on the competing ion effect on adsorption were carried out with various ions, viz., sodium, magnesium, hydroxide, and iodide. Reusability studies for both contaminants were performed for real field application.
Langmuir and Freundlich adsorption isotherms were used to describe the adsorption behavior of the adsorbate and adsorbent, assuming no interaction had taken place among the adsorbed molecules. Langmuir isotherm (Langmuir, 1918), which is valid for monolayer adsorption on the surface of an adsorbent, is given by
where qe is the equilibrium quantity adsorbed (mg/g), qm is the maximum capacity of monomer adsorption (mg/g), Ce is the equilibrium concentration (mg/L), and b is the adsorption equilibrium constant (L/mg) related to the energy of adsorption. The essential characteristics of the Langmuir isotherm can be determined in terms of a dimensionless equilibrium parameter or the Langmuir isotherm constant parameter (RL) which can be related to b by the following equation:
where Co (mg/L) is the initial Cr concentration.
The Freundlich isotherm is employed to model multilayer adsorption based on sorption onto a heterogeneous surface and is given by H. Z. Freundlich (Freundlich, 1906) as follows:
where (mg1−1/n L1/n g−1) is the Freundlich constant indicative of the relative adsorption capacity of the CuO nanoparticle adsorbent and n is the empirical parameter representing the adsorption intensity of the CuO nanoparticles.
Removal of Hg(II) and Cr(VI) is influenced by the amount of adsorbent (CuO-nano) used in the adsorption study. Dose effects were studied using various amounts (0.1–2 g L−1) of CuO nanoparticles (Figure 2). The removal of metals is increasingly proportional to sorbent dosage because of the availability of more active sites on the adsorbent (Viswanathan et al., 2009). A study was conducted to find the optimum dosage for both the metals: 1.0 g L−1 of adsorbent was found to be the optimum dosage from this study. When the concentration of the adsorbent was increased by 2-fold (say 2 gm), the removal efficiency increased only by a few percent. Hence, the optimum dosage was taken to be 1 gmL−1. Compared to other metal oxides, copper oxide nanoparticles have been proven as an effective adsorbent toward the removal of mercury and chromium from water (Xiaobing et al., 1999; Joshi and Shrivastava, 2011; Sumesh et al., 2011).
To estimate the optimum time required for complete adsorption of contaminants, studies were performed with varying times. It was found that quick adsorption happened within 1 h, and then the process slowed down followed by attaining of equilibrium within 180 min. No further adsorption occurred (less than 3%), even when mechanically shaken for up to 6 h, for both Hg and Cr (Figure 3A), which clearly indicates that complete adsorption took place within 3 h. Hence, further adsorption studies were performed by fixing the time as 3 h. We also studied the rate of adsorption with time and found that maximum adsorption occurred after 10 min of shaking (Figure 3B). After that, the adsorption rate gradually decreased until 60 min and then tended to be stable. It may be predicted that the adsorbent surface gets more adsorbate molecules and becomes saturated with time, thus the decreased rate of adsorption as time increases.
The effect of initial concentrations of the adsorbate (Hg and Cr) was studied with an adsorbent dose of 1 g L−1 and shaking time of 3 h. The outcomes are depicted in Figure 4A for the adsorption of both mercury and chromium. At the initial stage, the adsorption rate is fast, but with an increase in metal concentration, it slows down. As the concentration of contaminants (Hg and Cr) increases, active adsorption sites are occupied more and, subsequently, the adsorption process slows down (Joshi and Shrivastava, 2011). We used high concentrations of chromium and mercury to assess the efficiency of nanoparticles. We also performed experiments with 10, 20, and 30 ppb of mercury as the initial concentration to affirm whether the adsorbent is able to reduce the concentration to the permissible limit of drinking water (Hg, 1 ppb, BIS/WHO). It was found that CuO is able to reduce Hg to 1 ppb from an initial concentration of 20 ppb (Figure 4B).
FIGURE 4. (A) Removal of adsorbate vs its initial concentration. (B) Removal of adsorbate vs low concentration of mercury.
The effect of pH on Hg(II) and Cr(VI) adsorption by nanoparticles was studied (Figure 5). Adsorption of Hg(II) is highly influenced by pH, and optimum removal is achieved at pH 7.27.
The effect of pH was studied by varying pH from 3 to 11 without changing other parameters (metal dose of 1 g L−1, 3 h, and RT). The removal efficiency of CuO nanoparticles is better at basic pH than at an acidic one for both the contaminants. Removal of Hg(II) was observed to be >75% at alkaline conditions (pH 9.03), whereas <55% was found at acidic conditions (pH 3.97). At very high pH (10.89), mercury is precipitated in the hydroxide form, which is why it is found to have 87.5% adsorption efficiency for 200 ppb mercury. The variation in adsorption can be cleared from the ZPC of the adsorbent. Zero point charge (ZPC) of nano-CuO is determined by plotting the solution’s initial pH vs final pH (Figure 6). CuO nanoparticles are found to be neutral at pH 7.27, which is the zero point charge. When the adsorbate/adsorbent system (mercury/copper oxide nanoparticle) was maintained in the range of 7.27 < pH < 9.03, the adsorbent became negatively charged, and thus suitable to adsorb positively charged contaminants (Hg). But in acidic medium (below pH 7), the adsorbent surface becomes positively charged, thereby repelling positive contaminants such as Hg. Thus, the mechanism of removal of mercury by nano-CuO follows physical instead of chemical techniques.
The same conclusions can be drawn for chromium removal.
Mercury and chromium ion adsorption may be interfered by the presence of various common cations/anions present in contaminated drinking water, viz., OH−, Mg2+, Na+, Cl−, and I−. We used different concentrations (0.001, 0.01, 0.1, and 0.1 mol/L) of various cations and anions in mercury/chromium solution. Adsorption was performed taking [Hg2+]initial and [Cr6+]initial as 200 and 200 ppb, respectively, in the presence of different concentrations of diverse ions (Treybal, 1981). The findings are given in Figure 7 without altering other parameter, e.g., shaking frequency of 175 rpm, 25°C, and contact time of 3 h. It was found that removal of mercury/chromium is inversely proportional to the concentration of metals. This may be due to the competitive adsorption of metals on the surface of the adsorbent, which indirectly depends on the concentration, size, and charge of the ions (Erdem et al., 2004). Hydroxide ion has the most prominent effect as a competitor followed by magnesium. In alkaline conditions, CuO nanoparticles are bonded more with hydroxide ions compared to Hg2+/Cr6+. Being almost the same size as both chromium and mercury, magnesium also competes during adsorption. Thus, it may be inferred that the nanoparticles are efficient in removing both mercury and chromium ions from drinking water over a broad pH range even in the presence of common interfering cations/anions.
It has been found that an increase in temperature has a positive impact on the adsorption of mercury and chromium by CuO nanoparticles. It indicates the endothermic nature of adsorption. Additionally, the thermodynamics of adsorption depending on temperature was studied at three different temperatures, viz., 298, 308, and 318 K. Parameters such as Gibb’s free energy (ΔG0), enthalpy (ΔH0), and entropy (ΔS0) were calculated using the following equations (Namasivayam and Kavitha, 2002).
For unit adsorbent mass, Eq. 2 became
Here, qe indicates the amount of metals adsorbed by the adsorbent (µg/g), Ce is the concentration in equilibrium (µg/L), and m and T indicate adsorbent mass (g/L) and temperature (K), respectively. qe/Ce reveals the affinity of adsorption. Gibb’s free energy (ΔG0) is determined with the help of enthalpy (ΔH0) (deduced from graph of log (qe/Ce) vs 1/T) and entropy (ΔS0). The thermodynamic parameters were determined [Table 1] using the concentration of [Hg2+]initial = 200 ppb (µg/L). The endothermic nature of adsorption is confirmed from the positive ΔH0 value, whereas the negative ΔG0 values reveal that the metal adsorptions are spontaneous in nature. The low ΔS0 value clearly indicates that no remarkable change in entropy happened in the process. But the positive value of entropy clearly supports the increased randomness. The ΔH0 (69.68 KJmol−1) falls in the range of 80–200 kJmol−1 [heat of chemisorption], which reveals the physicochemical nature of the adsorption process, instead of either physical or chemical sorption.
For chromium, the same observations were recorded with negative ΔG0 values, which clearly indicates favorable adsorption [Table 2].
The study on adsorption isotherm was performed using Langmuir and Freundlich models. Basic equations with an explanation of energy terms are given in the Experimental section. Values obtained from the Langmuir adsorption model and the Freundlich model for the different parameters are presented in Table 3. The maximum adsorption capacity, qm, in the case of chromium was found to be 1.43 mg, and in the case of mercury, it was 1.03 mg adsorbed per gram of CuO nanoparticles. If the value of the Langmuir isotherm constant (RL) is in the order 0 < RL < 1, then the Langmuir isotherm is favorable; if RL = 0, it is irreversible; if RL = 1, it is linear; and if RL > 1, the isotherm is unfavorable. The calculated value of RL was found to be between 0.6649 and 0.0764, indicating a favorable adsorption for all initial concentrations of chromium and mercury.
A computed value of the adsorption intensity (n) of the CuO nanoparticles between 1 and 10 shows a favorable condition for Freundlich adsorption isotherms. The value was found to be 1.89 for chromium adsorption and 1.72 for mercury adsorption, indicating favorable conditions for Freundlich adsorption isotherms. From the nonlinear chi square (χ2) test, it was observed that the data fitted better to the Langmuir model than the Freundlich model in both the removal of chromium and mercury by CuO nanoparticles (Figure 8), indicating a monolayer than a heterolayer adsorption behavior.
FIGURE 8. Adsorption isotherms for the removal of (A) chromium and (B) mercury by CuO nanoparticles.
The mercury/chromium adsorbed nano-copper oxides were desorbed with eluents, viz., dilute hydrochloric acid and dilute sodium hydroxide (Viswanathan et al., 2009). The adsorbed materials were filtered on filter paper, and 100 ml of eluent was passed slowly through the filtered and spent adsorbent. The solution collected after treatment with acid/alkali was analyzed for the concentration of metal ions adsorbed.
The desorption efficiency was determined by comparing the initial and posttreatment concentrations of the solution. All experiments were performed at RT. Both hydrochloric acid and sodium hydroxide at concentrations of 0.2(M) and 0.5(M), respectively, were used as eluents. The desorption efficiency for Hg and Cr was found to be 82 and 85%, respectively, with HCl [Table 4]. Sodium hydroxide is a weaker eluent than hydrochloric acid. But NaOH provides a maximum desorption efficiency of 70%.
o CuO nanoparticles were prepared using the thermal reflux method and work as a good adsorbent for Hg(II) and Cr(VI) removal from water.
o The ΔG0 and ΔH0 values reflect the spontaneous and physicochemical nature of the adsorption process, respectively.
o CuO is effective in detoxifying water over a wide pH range even with interfering ions. The effectiveness of CuO at low pH shows that the adsorbent may be used to successfully clean up industrial toxicants.
o It was found that chromium and mercury undergo monolayer sorption on the surface of the nano-CuO. The maximum adsorption capacity was found to be 1.43 mg and 1.03 mg in the case of chromium and mercury, respectively, adsorbed per gram of nano-CuO.
o The desorption study confirms that spent adsorbents can be regenerated and used more than 3 times after acid/alkali treatment.
The original contributions presented in the study are included in the article/Supplementary Material; further inquiries can be directed to the corresponding author.
PR designed and studied the experiments. PR carried out the adsorption experiments. BD designed and performed the thermodynamic study. IU performed the isotherm studies. GT analyzed the data. RD guided and reviewed the manuscript. DK supervised the whole study. All authors read and approved the final manuscript.
The authors declare that the research was conducted in the absence of any commercial or financial relationships that could be construed as a potential conflict of interest.
All claims expressed in this article are solely those of the authors and do not necessarily represent those of their affiliated organizations, or those of the publisher, the editors, and the reviewers. Any product that may be evaluated in this article, or claim that may be made by its manufacturer, is not guaranteed or endorsed by the publisher.
The authors acknowledge DRL Tezpur for providing financial assistance and research facilities to carry out this research. The authors acknowledge Mr. Ananta Saikia, Scientist “E,” for his review and suggestion to improve the manuscript.
Blue, L. Y., Van Aelstyn, M. A., Matlock, M., and Atwood, D. A. (2008). Low-level Mercury Removal from Groundwater Using a Synthetic Chelating Ligand. Water Res. 42, 2025–2028. doi:10.1016/j.watres.2007.12.010
Carolin, C. F., Kumar, P. S., Saravanan, A., Joshiba, G. J., and Naushad, M. (2017). Efficient Techniques for the Removal of Toxic Heavy Metals from Aquatic Environment: A Review. J. Environ. Chem. Eng. 5, 2782–2799. doi:10.1016/j.jece.2017.05.029
Dubey, S., and Sharma, Y. C. (2017). Calotropis Procera Mediated One Pot green Synthesis of Cupric Oxide Nanoparticles (CuO-NPs) for Adsorptive Removal of Cr(VI) from Aqueous Solutions. Appl. Organometal Chem. 31, e3849. doi:10.1002/aoc.3849
Duncan, A. E., de Vries, N., and Nyarko, K. B. (2018). Assessment of Heavy Metal Pollution in the Sediments of the River Pra and its Tributaries. Water Air Soil Pollut. 229, 272–282. doi:10.1007/s11270-018-3899-6
Erdem, E., Karapinar, N., and Donat, R. (2004). The Removal of Heavy Metal Cations by Natural Zeolites. J. Colloid Interf. Sci. 280, 309–314. doi:10.1016/j.jcis.2004.08.028
Gopal, B., and Gupta, A. (2019). Integrated Approach for Hazardous Cr(VI) Removal: Reduction, Extraction, and Conversion into a Photoactive Composite, CuO/CuCr2O4. ACS Omega 4, 20443–20449. doi:10.1021/acsomega.9b01452
Gupta, M. K., Tandon, P. K., Afroz, M., and Agrahari, S. (2021). Combined Application of MMT K10 Supported Copper Oxide Nanoparticles for Complete Removal of Cr(VI) from Aqueous Solution and Their Antibacterial Potential. ACSi 68, 617–628. doi:10.17344/acsi.2020.6547
Gupta, V. K., Chandra, R., Tyagi, I., and Verma, M. (2016). Removal of Hexavalent Chromium Ions Using CuO Nanoparticles for Water Purification Applications. J. Colloid Interf. Sci. 478, 54–62. doi:10.1016/j.jcis.2016.05.064
Holmes, P., James, K. A. F., and Levy, L. S. (2009). Is Low-Level Environmental Mercury Exposure of Concern to Human Health? Sci. Total Environ. 408, 171–182. doi:10.1016/j.scitotenv.2009.09.043
Joshi, K. M., and Shrivastava, V. S. (2011). Photocatalytic Degradation of Chromium (VI) from Wastewater Using Nanomaterials like TiO2, ZnO, and CdS. Appl. Nanosci 1, 147–155. doi:10.1007/s13204-011-0023-2
Kondabey, J., Ghorbani, M. H., Aghaie, H., and Fazaeli, R. (2019). Study of the Adsorption and Photocatalytic Properties of Copper Oxide with Different Morphologies in Removal of Cr(III) Ion from Aqueous media. Water Sci. Tech. 80 (5), 827–835. doi:10.2166/wst.2019.313
Langmuir, I. (1918). The Adsorption of Gases on Plane Surfaces of Glass, Mica and Platinum. J. Am. Chem. Soc. 40, 1361–1403. doi:10.1021/ja02242a004
Mahmoud, A. E. D., Al-Qahtani, K. M., Alfaij, S. O., Al-Qahtani, S. F., and Alsamhan, F. A. (2021). Green Copper Oxide Nanoparticles for lead, Nickel, and Cadmium Removal from Contaminated Water. Scientific Rep. 11, 12547–12559. doi:10.1038/s41598-021-91093-7
Namasivayam, C., and Kavitha, D. (2002). Removal of congo Red from Water by Adsorption onto Activated Carbon Prepared from Coir Pith, an Agricultural Solid Waste. Dyes Pigm. 54, 47–58. doi:10.1016/s0143-7208(02)00025-6
Raul, P. K., Das, B., Devi, R. R., and Dwivedi, S. K. (2021). Nanoscale Copper(II) Oxide: A efficient and reusable adsorbent to remove nickel(II) from contaminated water. Defence Life Sci. J. 6 (2), 146–154. doi:10.14429/dlsj.6.16289
Salam, O. A., Reiad, N. A., and Eishafei, M. M. (2011). A study of the removal characteristics of heavy metals from wastewater by low-cost adsorbents. J. Adv. Res. 2, 297–303. doi:10.1016/j.jare.2011.01.008
Sheet, I., Kabbani, A., and Holail, H. (2014). Removal of Heavy Metals Using Nanostructured Graphite Oxide, Silica Nanoparticles and Silica/Graphite Oxide Composite. Energ. Proced. 50, 130–138. doi:10.1016/j.egypro.2014.06.016
Subramaniam, M. N., Goh, P. S., Lau, W. J., and Ismail, A. F. (2019). The Roles of Nanomaterials in Conventional and Emerging Technologies for Heavy Metal Removal: A State-Of-The-Art Review. Nanomaterials 9 (4), 625–630. doi:10.3390/nano9040625
Sumesh, E., Bootharaju, M. S., Anshup, A. T., and Pradeep, T. (2011). A Practical Silver Nanoparticle-Based Adsorbent for the Removal of Hg2+ from Water. J. Hazard. Mater. 189, 450–457. doi:10.1016/j.jhazmat.2011.02.061
Viswanathan, N., Sundaram, C. S., and Meenakshi, S. (2009). Removal of Fluoride from Aqueous Solution Using Protonated Chitosan Beads. J. Hazard. Mater. 161, 423–430. doi:10.1016/j.jhazmat.2008.03.115
Wolińska, A., Stępniewska, Z., and Włosek, R. (2013). The Influence of Old Leather Tannery District on Chromium Contamination of Soils, Water and Plants. Nat. Sci. 5 (2A), 253–258. doi:10.4236/ns.2013.52a037
Xia, M., Chen, Z., Li, Y., Li, C., Ahmad, N. M., Cheema, W. A., et al. (2019). Removal of Hg(II) in Aqueous Solutions through Physical and Chemical Adsorption Principles. RSC Adv. 9, 20941–20953. doi:10.1039/c9ra01924c
Xiaobing, C., Feng, X., Liu, J. J., Fryxell, G., and Gong, M. (1999). Mercury Separation and Immobilization Using Self-Assembled Monolayers on Mesoporous Supports (SAMMS). Sep. Sci. Techn. 34 (6-7), 1121–1132.
Yongsheng, W., Qihui, L., and Qian, T. (2011). Effect of Pb on Growth, Accumulation and Quality Component of tea Plant. Proced. Eng. 18, 214–219. doi:10.1016/j.proeng.2011.11.034
Zahir, F., Rizwi, S. J., Haq, S. K., and Khan, R. H. (2005). Low Dose Mercury Toxicity and Human Health. Environ. Toxicol. Pharmacol. 20, 351–360. doi:10.1016/j.etap.2005.03.007
Zayed, A. M., and Terry, N. (2003). Chromium in the Environment: Factors Affecting Biological Remediation. Plant Soil 249 (1), 139–156. doi:10.1023/a:1022504826342
Keywords: copper oxide nanoparticle, chromium(VI), mercury(II), adsorption, water
Citation: Raul PK, Das B, Umlong IM, Devi RR, Tiwari G and Kamboj DV (2022) Toward a Feasible Solution for Removing Toxic Mercury and Chromium From Water Using Copper Oxide Nanoparticles. Front. Nanotechnol. 4:805698. doi: 10.3389/fnano.2022.805698
Received: 30 October 2021; Accepted: 06 January 2022;
Published: 03 February 2022.
Edited by:
José Leandro da Silva Duarte, Federal University of Alagoas, BrazilReviewed by:
Leonardo Oliveira, Federal University of Alagoas, BrazilCopyright © 2022 Raul, Das, Umlong, Devi, Tiwari and Kamboj. This is an open-access article distributed under the terms of the Creative Commons Attribution License (CC BY). The use, distribution or reproduction in other forums is permitted, provided the original author(s) and the copyright owner(s) are credited and that the original publication in this journal is cited, in accordance with accepted academic practice. No use, distribution or reproduction is permitted which does not comply with these terms.
*Correspondence: Prasanta K. Raul, cHJhc2FudGEuZHJkb0BnbWFpbC5jb20=
Disclaimer: All claims expressed in this article are solely those of the authors and do not necessarily represent those of their affiliated organizations, or those of the publisher, the editors and the reviewers. Any product that may be evaluated in this article or claim that may be made by its manufacturer is not guaranteed or endorsed by the publisher.
Research integrity at Frontiers
Learn more about the work of our research integrity team to safeguard the quality of each article we publish.