- 1Institute for Molecules and Materials, Radboud University, Nijmegen, Netherlands
- 2Department of Physics, Tokyo Institute of Technology, Tokyo, Japan
- 3Department of Physics, University of York, York, United Kingdom
- 4Institute for Chemical Research, Kyoto University, Kyoto, Japan
- 5Center for Spintronics Research Network (CSRN), Graduate School of Engineering Science, Osaka University, Osaka, Japan
- 6FELIX Laboratory, Radboud University, Nijmegen, Netherlands
All-optical helicity dependent switching (AO-HDS), deterministic control of magnetization by circularly polarized laser pulses, allows to efficiently manipulate spins without the need of a magnetic field. However, AO-HDS in ferromagnetic metals so far requires many laser pulses for fully switching their magnetic states. Using a combination of a short, 90-fs linearly polarized pulse and a subsequent longer, 3-ps circularly polarized pulse, we demonstrate that the number of pulses for full magnetization reversal can be reduced to four pulse pairs in a single stack of Pt/Co/Pt. The obtained results suggest that the dual-pulse approach is a potential route towards realizing efficient AO-HDS in ferromagnetic metals.
Introduction
The explosive growth of big data and artificial intelligence demands faster and more energy-efficient ways to manipulate and store data (Beyond CMOS, 2018; Manipatruni et al., 2018; Dieny et al., 2020). One approach is to use current-induced torque (Brataas et al., 2012; Dieny et al., 2020) to switch magnetization, as in spin-transfer torque magnetic random access memories (Apalkov et al., 2016). Another potential route is to use an ultrashort laser pulse (Kirilyuk et al., 2010; Kimel and Li., 2019). Merging the ultrafast and energy-efficient features of photonics with the nonvolatility of magnets may lead to innovations in information technologies. Even though the leading part of all-optical magnetization switching has been ferrimagnets, represented by GdFeCo (Stanciu et al., 2007; Vahaplar et al., 2009; Ostler et al., 2011; Radu et al., 2011; Khorsand et al., 2012; Mangin et al., 2014), cutting-edge magnetic media consist of ferromagnets (Kryder et al., 2008; Apalkov et al., 2016). This is the reason why all-optical switching in ferromagnets attracts great attention. Ferromagnets, such as Pt/Co/Pt multilayers (Lambert et al., 2014; Hadri et al., 2016a; Hadri et al., 2016b; Medapalli et al., 2017; Parlak et al., 2018; Quessab et al., 2018; Chakravarty et al., 2019; Kichin et al., 2019; Cheng et al., 2020) and granular FePt alloys (Lambert et al., 2014; Takahashi et al., 2016; John et al., 2017), have recently shown all-optical helicity-dependent switching (AO-HDS), magnetization switching depending on the circular polarization of the optical excitation pulses. However, AO-HDS generally requires at least tens and usually hundreds of laser pulses for full switching (Kichin et al., 2019).
AO-HDS is understood to proceed via two stages: helicity-independent nucleation of switched domains and helicity-dependent deterministic domain wall (DW) motion (Hadri et al., 2016a; Medapalli et al., 2017). This two-step mechanism especially motivated us to try a dual-pulse excitation in which each pulse of a pair of pulses is optimized for either the helicity independent demagnetization and nucleation of switched domains or the helicity dependent DW motion. Hence, the goal of our study was to find the optimal combination of pulses that allows switching the magnetization of ferromagnetic materials in a helicity dependent and deterministic way. The studied Pt/Co/Pt trilayer with perpendicular magnetic anisotropy is excited by pairs of laser pulses. The pair consisted of a femtosecond (fs) linearly polarized (π) laser pulse, meant to demagnetize the sample, and a picosecond (ps) circularly polarized (σ) laser pulse coming after a time separation (Δt), meant to steer the magnetization to its final state in a helicity dependent way, but without causing further demagnetization. We found that using only four of such dual pulse pairs are sufficient to deterministically reverse the magnetization, offering great perspectives for AO-HDS of ferromagnetic media.
Materials and Methods
Sample Preparations
We prepared a Ta (4 nm)/Pt (3.0 nm)/Co (0.6 nm)/Pt (3.0 nm)/MgO (2.0 nm)/Ta (1.0 nm) multilayer on a synthetic quartz glass substrate by magnetron sputtering. Pt/Co/Pt systems are relevant for testing the dual-pulse approach because they are typical candidates for spintronic devices (Brataas et al., 2012) as well as for all-optical helicity dependent switching (Lambert et al., 2014; Hadri et al., 2016a; Hadri et al., 2016b; Medapalli et al., 2017; Parlak et al., 2018; Quessab et al., 2018; Chakravarty et al., 2019; Kichin et al., 2019; Cheng et al., 2020). DC and RF sources were used for depositing Ta, Pt, and Co, and MgO, respectively. The MgO/Ta capping layer prevents the magnetic layer from oxidization. The multilayer exhibits a perpendicular easy axis of magnetization.
Magneto-Optical Imaging
For optical excitation, we used a Ti: sapphire amplified laser system (Solstice Ace, Spectra-Physics) of which the central wavelength and repetition rate were 800 nm and 1 kHz, respectively. We used two compressors of the amplifier system to tune the pulse widths of the two pump laser beams. The laser amplifier was used in external trigger mode. Using voltage pulses from a delay generator (DG645, Stanford Research), we controlled the number of pulses for pumping. The durations of the short and long pump pulses were about 90 fs and 3.0 ps, respectively. The time separation, Δt, was adjusted by a delay line on the pathway of the long pulse. The laser pulses with Gaussian intensity distribution were incident at an angle of 15° from the sample normal. The focused beam sizes (1/e2 radius) of the pump pulses were calculated with the Liu method (Liu, 1982; Khorsand et al., 2012). The laser fluence was calculated using the 1/e2 radius, the repetition rate (1 kHz) and the average power measured with a power meter. The pump intensities were controlled using a combination of a half-wave plate and a Gran-Taylor prism. To obtain a circular polarized beam, we used a quarter-wave plate (AQWP05M-600, Thorlabs Inc.). We used a white light source to visualize the magnetic domains in transmission via the magneto-optical Faraday effect. The white light source, which was linearly polarized by a sheet polarizer, was collimated and incident on the sample surface using a combination of lenses. We collected the transmitted light from the sample by an objective with a magnification of ×20. The polarization axis of an analyzer was nearly orthogonal to the polarization of the incident probe light. Since the magnetized sample rotates the polarization of the incident light, the magnetic domains can be visualized by a charge-coupled device (CCD) camera placed behind the analyzer.
Results
We first measured the switching by only circularly polarized pulses in transmission. Figure 1A shows magneto-optical images taken after the Pt/Co/Pt film was excited with a sequence of 3.0-ps right(σ+)- or left(σ−)-handed circularly polarized light pulses. These images were taken long after the excitation when the magnetization had already reached a stable state. The time interval was more than 2 s. Here, we used ps σ pulses because ps σ pulses are more favorable for the AO-HDS than fs σ pulses (see Medapalli et al., 2017). To fully reverse the magnetization in the area with a diameter of 15-μm, the experiments required 100–150 pulses.
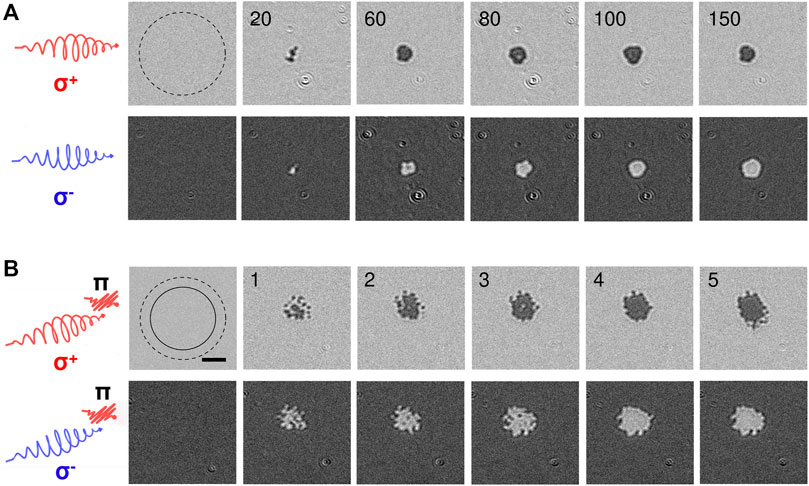
FIGURE 1. Dual-pulse all-optical helicity dependent switching (AO-HDS). (A) AO-HDS by multiple right(σ+) and left (σ−) circularly polarized pulses. Here, the fluence of the 3.0-ps σ pulse was fixed at Fσ = 3.42 mJ/cm2. (B) Dual-pulse AO-HDS for time separation Δt of 5.0 ps. Here, we used the fluence of F π = 2.32 mJ/cm2 for the 90-fs π pulse and Fσ = 1.94 mJ/cm2 for the 3.0-ps σ pulse. The number of pulse (pairs) is indicated on each magneto-optical image. The darker and brighter areas denote up(M↑)- and down(M↓)-magnetized states, respectively. The scale bars correspond to 20 µm. The 1/e2 diameter of the σ (solid line) and π (dash line) pulses were 52.0 ± 0.8 and 68.2 ± 1.8 μm, respectively.
We next performed similar experiments with pairs of pulses. Figure 1B shows the results, in which the same Pt/Co/Pt stack was excited with one 90-fs π and one 3.0-ps σ pulses, separated by Δt = 5.0 ps. By varying the delay between the long and short pulse, we experimentally found that the optimum delay was around 5 ps. Figure 1B shows that four pairs of these dual pulses can completely switch the magnetizations of the same 15-μm area in a deterministic way. The switched area can be switched back by switching the helicity of the longer pulse. We again note that in the case of excitation without π pulses, i.e., with σ pulses only, a similar result would require more than 100 excitation events (Figure 1A). The total laser fluence for full magnetization switching with the dual-pulse method, 4 × (Fπ + Fσ) ∼ 17 mJ/cm2, is much smaller than 100 × Fσ ∼ 342 mJ/cm2 used for the multi single-pulse approach. The dual-pulse approach not only minimizes the number of pulses but also dramatically reduces the net laser fluence required for full magnetization reversal. A pulse pair makes many reversed domains in an overlapping area of the two pulses (Figure 1B), in contrast to that, Gaussian σ pulses make a single reversed domain in the spot center (Figure 1A). This distinctive feature suggests that the switching process of the dual-pulse AO-HDS is different from that of the multi-pulse AO-HDS.
The pulse duration is critical for the multi-pulse AO-HDS. We checked that the pulse width of the long pulse must be pico-second wide. Figure 2A show the snapshots when using a σ pulse with a duration τσ of 0.5, 1.0, 2.0, and 3.0 ps for Δt = 5.0 ps. Full magnetization reversal by four pulse pairs is possible only for τσ = 3.0 ps. To evaluate the net magnetization <M>, we determined the average intensity of a 15-μm area and normalized it to the intensity due to uniform magnetization switching. Then, we estimated the switching efficiency defined as [<M> (M↓, σ−) - <M> (M↑, σ+)]/2, where the superscript ↑ (↓) denote that the initial magnetization was saturated upward (downward). Figure 2B shows the switching efficiency as a function of the number of pulse pairs for τσ = 0.5, 1.0, 2.0, and 3.0 ps. The switching efficiency increases with increasing τσ, which indicates that a longer σ pulse is more suitable also for the dual-pulse AO-HDS.
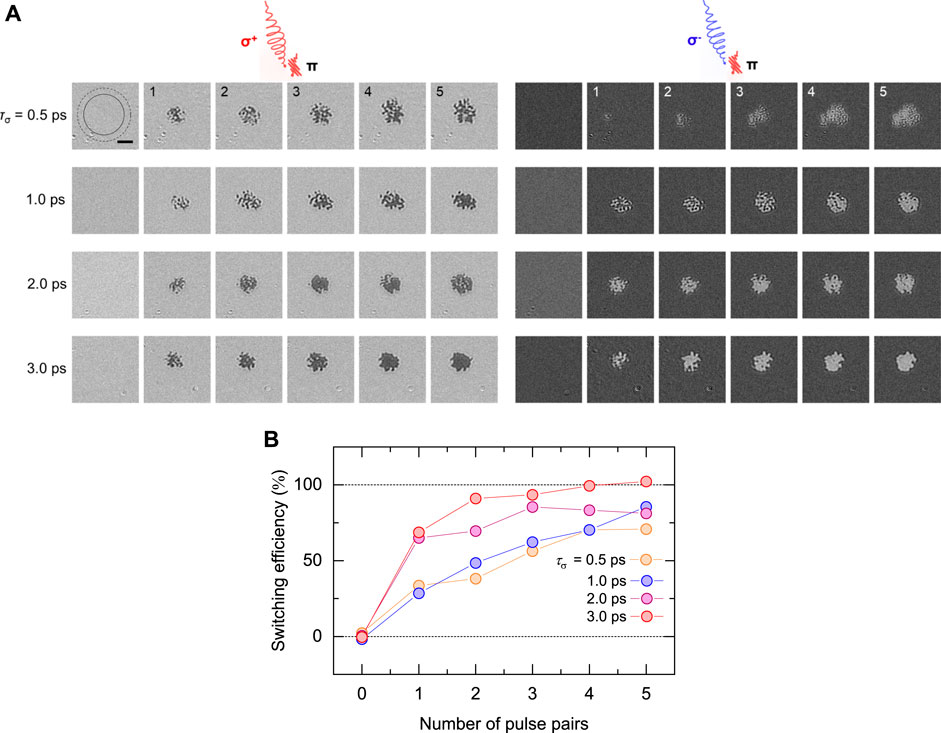
FIGURE 2. The pulse width τσ dependence of the dual-pulse AO-HDS. (A) Snapshots before and after illuminating the uniformly magnetized sample with 1-5 pairs of pulses at Δt of 5.0 ps are displayed for τσ = 0.5, 1.0, 2.0, and 3.0 ps. Here, we used fluences Fπ = 2.29 mJ/cm2 for the π pulse. The fluences of the σ pulses were Fσ = 1.91, 1.95, 1.87, and 1.90 mJ/cm2 for τσ = 0.5, 1.0, 2.0, and 3.0 ps, respectively. The 1/e2 diameter of the σ (solid line) and π (dash line) pulses were 52.0 ± 0.8 and 68.2 ± 1.8 μm, respectively. The scale bar corresponds to 20 μm. (B) The switching efficiency as a function of number of pulse pairs for τσ = 0.5, 1.0, 2.0, and 3.0 ps.
We next checked if a circular polarization of the fs pulse has an impact on the dual-pulse AO-HDS. Snapshots in Figure 3 show the dual-pulse AO-HDS by a combination of 90-fs and 3.0-ps σ pulses with Δt = 5.0 ps. One cannot find any meaningful impact of the helicity of the fs pulses on the final magnetization state. The results show that the first process of the dual pulse AO-HDS is helicity independent, like multi-pulse AO-HDS. Hundreds of fs after the act of the short pulse, the spin temperature reaches the Curie temperature and magnetic textures should be thermally nucleated. Therefore, the helicity of the fs pulse does not largely boost or suppress the creation of magnetic textures, although the absorption of the fs σ pulse slightly changes according to the initial magnetization orientation and the light helicity due to magnetic circular dichroism (MCD) (Tsema, et al., 2016).
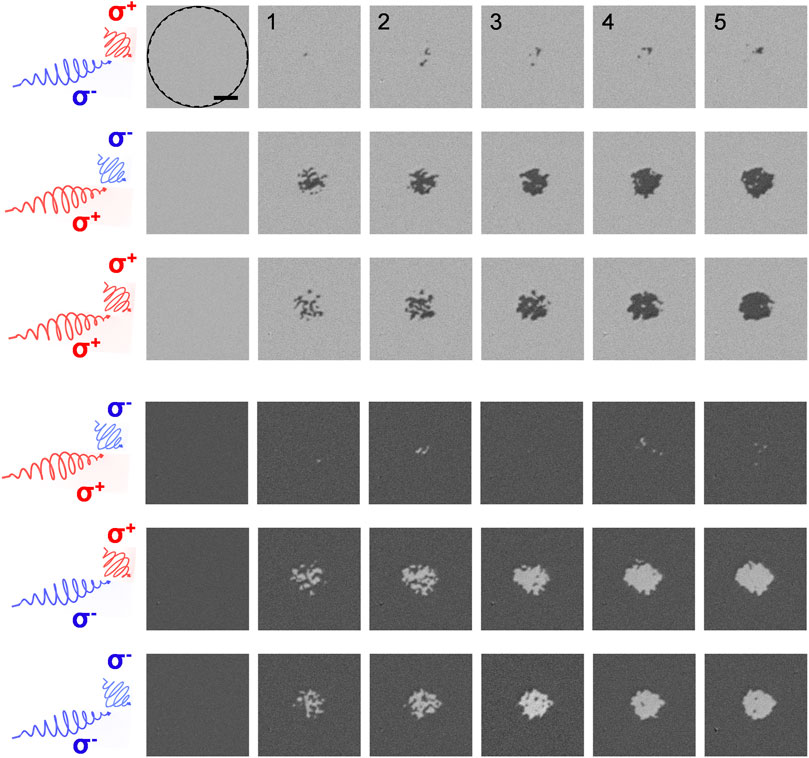
FIGURE 3. AO-HDS with a combination of 90-fs and 3.0-ps circularly polarized pulses. Snapshots before and after illuminating the uniformly magnetized sample with 1–5 pairs of pulses at Δt of 5.0 ps are displayed. The error bars were determined by repeating the same measurements three times. Here, we used fluences Fσ1 = 2.35 mJ/cm2 and Fσ2 = 1.32 mJ/cm2 for fs and ps circularly polarized pulses, respectively. The 1/e2 diameter of the ps (solid line) and fs (dash line) pulses were 84.2 ± 1.2 and 82.7 ± 1.0 μm, respectively. The scale bar corresponds to 20 μm.
Discussion
The results above clearly demonstrate the advantage of the dual-pulse AO-HDS and trigger the question of the mechanism behind it. It is known that the inverse Faraday effect (Kimel et al., 2005) is able to switch the magnetization. Atomistic spin simulations show however that the effective field of the inverse Faraday effect must be tens of Tesla to deterministically reverse the spins of FePt (Ellis, et al., 2016). To realize such large field amplitude in our sample, we must use a fluence much large than the damage threshold for our sample. Previous studies indicated that the inverse Faraday effect can induce coherent magnetization dynamics, but the magnetization precession angles are small with similar fluences (Choi et al., 2017). If the inverse Faraday of our sample is strong, it is strange that the helicity of the fs pulse has little impact on the dual-pulse AO-HDS. Therefore, the inverse Faraday effect is unlikely to drive the dual-pulse AO-HDS.
Another probable mechanism is helicity-dependent laser absorption via MCD (Gorchon et al., 2016). MCD can produce a heat gradient across a DW, and subsequently push a DW from the hotter (lower DW energy) to the colder (higher DW energy) area (Hadri et al., 2016a; Hadri et al., 2016b; Medapalli et al., 2017; Parlak et al., 2018; Quessab et al., 2018), as the up (down)-magnetized domain absorbs the left (right) circularly polarized light more than the down (up)- magnetized domain. During the cooling process, the DW will not move back to the initial position due to pinning. When a circularly polarized pulse with Fσ = 1.94 mJ/cm2 illuminates the Pt/Co/Pt stack, the heat gradient across a DW can be estimated to be
For the switching process of the dual-pulse AO-HDS, the fs pulse will contribute to the helicity independent creation of switched magnetic textures by causing ultrafast demagnetization. Simultaneously, the reduction of the magnetic properties by the fs pulse should facilitate the magnetization switching, like heat-assisted magnetic recording (Kryder et al., 2008), where the reduced coercivity field by laser heating enables the magnetization switching by a smaller magnetic field. The reduced magnetic properties may promote the nucleation of reversed domains in the overlapping area of the two pulses. While the demagnetization by the fs pulse proceeds within 500 fs to 1 ps, the remagnetization takes tens of ps after the act of the fs pulse (Borchert et al., 2008). The required pulse separation between 0 and 10 ps suggests that the helicity dependent absorption of the ps pulse may affect the remagnetizing system, resulting in efficient magnetization switching. Time-resolve studies and simulations on the dual-pulse AO-HDS should give crucial insights into the details of the actual switching dynamics of this new approach.
Conclusion
In conclusion, we have demonstrated that a dual-pulse method can drastically reduce the pulse number required for AO-HDS in a Pt/Co/Pt multilayer. Using a linearly polarized femtosecond pulse for the first pump and a circularly polarized picosecond pulse for the second pump, we successfully realized a record small number of eight pulses to fully switch a magnetic domain. The small impact of the helicity of the femtosecond pulse on the dual-pulse AO-HDS suggests that the nucleation of magnetic textures by the pulse is helicity independent. The dual-pulse AO-HDS is optimized at a pulse separation of about 5 ps. This implies that the switching might be completed on an ultrashort time scale by controlling the remagnetization in a helicity dependent way. The femtosecond pulse can forcibly create magnetic textures even in granular magnetic media (Takahashi et al., 2016; John et al., 2017) and nano devices (Brataas et al., 2012; Dieny et al., 2020) where a single domain state has been stabilized at room temperature. This means that the dual-pulse AO-HDS is practical for the application of opto-magnetic writing in magnetic recording media. The dual-pulse method is not only efficient but also a potential route towards implementation of all-optical magnetization switching to practical magnetic recording media, such as hard disc drives and magnetic random access memories.
Data Availability Statement
The original contributions presented in the study are included in the article/supplementary material, further inquiries can be directed to the corresponding authors.
Author Contributions
KTY, AVK, and TR conceived the experiments. KTY, KP, and SS designed and build the experimental set-up. KTY performed the measurements, and collected data. All the data were analyzed by KTY with the help of AVK and TR. The sample was fabricated and provided by TL, FA, and TO. KTY, AVK, and TR wrote the manuscript. All authors discussed the results and commented on the manuscript. This project was coordinated by AVK and TR.
Funding
This work was partly supported by the European Research Council Grant Agreement No. 856538 (3D-MAGiC), by the FOM programme Exciting Exchange, de Nederlandse Organisatie voor Wetenschappelijk Onderzoek (NWO), by the EU H2020 Program Grant Agreement No. 713481 (SPICE) and No. 737093 (FEMTOTERABYTE), by JSPS KAKENHI No. 15H05702, No. 20K22327, No. 20H00332, No. 20H05665, No. 17J07326, and No. 18J22219, and by the Collaborative Research Program of the Institute for Chemical Research, Kyoto University.
Conflict of Interest
The authors declare that the research was conducted in the absence of any commercial or financial relationships that could be construed as a potential conflict of interest.
Publisher’s Note
All claims expressed in this article are solely those of the authors and do not necessarily represent those of their affiliated organizations, or those of the publisher, the editors and the reviewers. Any product that may be evaluated in this article, or claim that may be made by its manufacturer, is not guaranteed or endorsed by the publisher.
Acknowledgments
We acknowledge T. Toonen and C. Berkhout for the continuous technical supports, J. Mentink and T. Satoh for fruitful discussions, and T. Taniguchi for valuable comments on sample fabrication.
References
Apalkov, D., Dieny, B., and Slaughter, J. M. (2016). Magnetoresistive Random Access Memory. Proc. IEEE 104, 1796–1830. doi:10.1109/jproc.2016.2590142
Beyond CMOS (2018). The Future of Semiconductors – IEEE International Roadmap for Devices and Systems. Available at: https://irds.ieee.org/home/what-is-beyond-cmos (Accessed August 27, 2018).
Borchert, M., Von Korff Schmising, C., Schick, D., Engel, D., Sharma, S., and Eisebitt, S. (2008). Manipulation of Ultrafast Demagnetization Dynamics by Optically Induced Intersite Spin Transfer in Magnetic Compounds with Distinct Density of States.
Brataas, A., Kent, A. D., and Ohno, H. (2012). Current-induced Torques in Magnetic Materials. Nat. Mater 11, 372–381. doi:10.1038/nmat3311
Chakravarty, A., Mentink, J. H., Davies, C. S., Yamada, K. T., Kimel, A. V., and Rasing, Th. (2019). Supervised Learning of an Opto-Magnetic Neural Network with Ultrashort Laser Pulses. Appl. Phys. Lett. 114, 192407. doi:10.1063/1.5087648
Cheng, F., Du, Z., Wang, X., Cai, Z., Li, L., Wang, C., et al. (2020). All‐Optical Helicity‐Dependent Switching in Hybrid Metal-Ferromagnet Thin Films. Adv. Opt. Mater. 8, 2000379. doi:10.1002/adom.202000379
Choi, G.-M., Schleife, A., and Cahill, D. G. (2017). Optical-Helicity-Driven Magnetization Dynamics in Metallic Ferromagnets. Nat. Commun. 8, 15085. doi:10.1038/ncomms15085
Dieny, B., Prejbeanu, I. L., Garello, K., Gambardella, P., Freitas, P., Lehndorff, R., et al. (2020). Opportunities and Challenges for Spintronics in the Microelectronics Industry. Nat. Electron. 3, 446–459. doi:10.1038/s41928-020-0461-5
Ellis, M. O. A., Fullerton, E. E., and Chantrell, R. W. (2016). All-optical Switching in Granular Ferromagnets Caused by Magnetic Circular Dichroism. Sci. Rep. 6, 30522. doi:10.1038/srep30522
Gorchon, J., Yang, Y., and Bokor, J. (2016). Model for Multi-Shot All-thermal All-Optical Switching in Ferromagnets. Phys. Rev. B 94, 020409. doi:10.1103/physrevb.94.020409
Hadri, M. S. El., Hehn, M., Pirro, P., Lambert, C.-H., Malinowski, G., Fullerton, E. E., et al. (2016a). Domain Size Criterion for the Observation of All-Optical Helicity-dependent Switching in Magnetic Thin Films. Phys. Rev. B 94, 064419. doi:10.1103/physrevb.94.064419
Hadri, M. S. El., Pirro, P., Lambert, C.-H., Petit-Watelot, C.-H., Quessab, Y., Hehn, M., et al. (2016b). Two Types of All-Optical Magnetization Switching Mechanisms Using Femtosecond Laser Pulses. Phys. Rev. B 94, 064412. doi:10.1103/physrevb.94.064412
Jiang, W., Upadhyaya, P., Fan, Y., Zhao, J., Wang, M., Chang, L.-T., et al. (2013). Direct Imaging of Thermally Driven Domain Wall Motion in Magnetic Insulators. Phys. Rev. Lett. 110, 177202. doi:10.1103/physrevlett.110.177202
John, R., Berritta, M., Hinzke, D., Müller, C., Santos, T., Ulrichs, H., et al. (2017). Magnetisation Switching of FePt Nanoparticle Recording Medium by Femtosecond Laser Pulses. Sci. Rep. 7, 4114. doi:10.1038/s41598-017-04167-w
Khorsand, A. R., Savoini, M., Kirilyuk, A., Kimel, A. V., Tsukamoto, A., Itoh, A., et al. (2012). Role of Magnetic Circular Dichroism in All-Optical Magnetic Recording. Phys. Rev. Lett. 108, 127205. doi:10.1103/physrevlett.108.127205
Kichin, G., Hehn, M., Gorchon, J., Malinowski, G., Hohlfeld, J., and Mangin, S. (2019). From Multiple- to Single-Pulse All-Optical Helicity-dependent Switching in Ferromagnetic Co/Pt Multilayers. Phys. Rev. Appl. 12, 024019. doi:10.1103/physrevapplied.12.024019
Kimel, A. V., Kirilyuk, A., Usachev, P. A., Pisarev, R. V., Balbashov, A. M., and Rasing, Th. (2005). Ultrafast Non-thermal Control of Magnetization by Instantaneous Photomagnetic Pulses. Nature 435, 655–657. doi:10.1038/nature03564
Kimel, A. V., and Li, M. (2019). Writing Magnetic Memory with Ultrashort Light Pulses. Nat. Rev. Mater. 4, 189–200. doi:10.1038/s41578-019-0086-3
Kirilyuk, A., Kimel, A. V., and Rasing, Th. (2010). Ultrafast Optical Manipulation of Magnetic Order. Rev. Mod. Phys. 82, 2731–2784. doi:10.1103/revmodphys.82.2731
Kryder, M. H., Gage, E. C., McDaniel, T. W., Challener, W. A., Rottmayer, R. E., Ganping Ju, G., et al. (2008). Heat Assisted Magnetic Recording. Proc. IEEE 96, 1810–1835. doi:10.1109/jproc.2008.2004315
Lambert, C.-H., Mangin, S., Varaprasad, B. S. D. C. S. B. S. D., Takahashi, Y. K., Hehn, M., Cinchetti, M., et al. (2014). All-optical Control of Ferromagnetic Thin Films and Nanostructures. Science 345, 1337–1340. doi:10.1126/science.1253493
Liu, J. M. (1982). Simple Technique for Measurements of Pulsed Gaussian-Beam Spot Sizes. Opt. Lett. 7, 196–198. doi:10.1364/ol.7.000196
Mangin, S., Gottwald, M., Lambert, C.-H., Steil, D., Uhlíř, V., Pang, L., et al. (2014). Engineered Materials for All-Optical Helicity-dependent Magnetic Switching. Nat. Mater 13, 286–292. doi:10.1038/nmat3864
Manipatruni, S., Nikonov, D. E., and Young, I. A. (2018). Beyond CMOS Computing with Spin and Polarization. Nat. Phys. 14, 338–343. doi:10.1038/s41567-018-0101-4
Medapalli, R., Afanasiev, D., Kim, D. K., Quessab, Y., Manna, S., Montoya, S. A., et al. (2017). Multiscale Dynamics of Helicity-Dependent All-Optical Magnetization Reversal in Ferromagnetic Co/Pt Multilayers. Phys. Rev. B 96, 224421. doi:10.1103/physrevb.96.224421
Metaxas, P. J., Jamet, J. P., Mougin, A., Cormier, M., Ferré, J., Baltz, V., et al. (2007). Creep and Flow Regimes of Magnetic Domain-Wall Motion in Ultrathin Pt/Co/Pt Films with Perpendicular Anisotropy. Phys. Rev. Lett. 99, 217208. doi:10.1103/physrevlett.99.217208
Ostler, T. A., Barker, J., Evans, R. F., Chantrell, R. W., Atxitia, U., Chubykalo-Fesenko, O., et al. (2011). Ultrafast Heating as a Sufficient Stimulus for Magnetization Reversal in a Ferrimagnet. Nat. Commun. 3, 666. doi:10.1038/ncomms1666
Parlak, U., Adam, R., Bürgler, D. E., Gang, S., and Schneider, C. M. (2018). Optically Induced Magnetization Reversal in [Co/Pt]N Multilayers: Role of Domain wall Dynamics. Phys. Rev. B 98, 214443. doi:10.1103/physrevb.98.214443
Quessab, Y., Medapalli, R., Hadri, M. S. El., Hehn, M., Malinowski, G., Fullerton, E. E., et al. (2018). Helicity-Dependent All-Optical Domain wall Motion in Ferromagnetic Thin Films. Phys. Rev. B 97, 054419. doi:10.1103/physrevb.97.054419
Radu, I., Vahaplar, K., Stamm, C., Kachel, T., Pontius, N., Dürr, H. A., et al. (2011). Transient Ferromagnetic-Like State Mediating Ultrafast Reversal of Antiferromagnetically Coupled Spins. Nature 472, 205–208. doi:10.1038/nature09901
Stanciu, C. D., Hansteen, F., Kimel, A. V., Kirilyuk, A., Tsukamoto, A., Itoh, A., et al. (2007). All-Optical Magnetic Recording with Circularly Polarized Light. Phys. Rev. Lett. 99, 047601. doi:10.1103/PhysRevLett.99.047601
Takahashi, Y. K., Medapalli, R., Kasai, S., Wang, J., Ishioka, K., Wee, S.-H., et al. (2016). Accumulative Magnetic Switching of Ultrahigh-Density Recording media by Circularly Polarized Light. Phys. Rev. Appl. 6, 054004. doi:10.1103/physrevapplied.6.054004
Tsema, Y., Kichin, G., Hellwig, O., Mehta, V., Kimel, A. V., Kirilyuk, A., et al. (2016). Helicity and Field Dependent Magnetization Dynamics of Ferromagnetic Co/Pt Multilayers. Appl. Phys. Lett. 109, 072405. doi:10.1063/1.4961246
Vahaplar, K., Kalashnikova, A. M., Kimel, A. V., Hinzke, D., Nowak, U., Chantrell, R., et al. (2009). Ultrafast Path for Optical Magnetization Reversal via a Strongly Nonequilibrium State. Phys. Rev. Lett. 103, 117201. doi:10.1103/physrevlett.103.117201
Keywords: optical control of magnetism, ultrafast magnetism, opto-spintronics, all-optical helicity dependent magnetization switching, ferromagnetic thin film
Citation: Yamada KT, Kimel AV, Prabhakara KH, Ruta S, Li T, Ando F, Semin S, Ono T, Kirilyuk A and Rasing T (2022) Efficient All-Optical Helicity Dependent Switching of Spins in a Pt/Co/Pt Film by a Dual-Pulse Excitation. Front. Nanotechnol. 4:765848. doi: 10.3389/fnano.2022.765848
Received: 27 August 2021; Accepted: 18 January 2022;
Published: 23 February 2022.
Edited by:
Wen Zhang, Northwestern Polytechnical University, ChinaReviewed by:
Yong Xu, Beihang University, ChinaKai Chen, University of Science and Technology of China, China
Copyright © 2022 Yamada, Kimel, Prabhakara, Ruta, Li, Ando, Semin, Ono, Kirilyuk and Rasing. This is an open-access article distributed under the terms of the Creative Commons Attribution License (CC BY). The use, distribution or reproduction in other forums is permitted, provided the original author(s) and the copyright owner(s) are credited and that the original publication in this journal is cited, in accordance with accepted academic practice. No use, distribution or reproduction is permitted which does not comply with these terms.
*Correspondence: Kihiro T. Yamada, yamada@phys.titech.ac.jp; Alexey V. Kimel, aleksei.kimel@ru.nl