- Department of Biotechnology, Khalsa College, Amritsar, India
The biomedical properties of nanoparticles have been the area of focus for contemporary science; however, there are issues concerning their long-term toxicities. Recent trends in nanoparticle fabrication and surface manipulation, the use of distinctive biogenic capping agents, have allowed the preparation of nontoxic, surface-functionalized, and monodispersed nanoparticles for medical applications. These capping agents act as stabilizers or binding molecules that prevent agglomeration and steric hindrance, alter the biological activity and surface chemistry, and stabilize the interaction of nanoparticles within the preparation medium. Explicit features of nanoparticles are majorly ascribed to the capping present on their surface. The present review article is an attempt to compile distinctive biological capping agents deployed in the synthesis of metal nanoparticles along with the medical applications of these capped nanoparticles. First, this innovative review highlights the various biogenic capping agents, including biomolecules and biological extracts of plants and microorganisms. Next, the therapeutic applications of capped nanoparticles and the effect of biomolecules on the efficiency of the nanoparticles have been expounded. Finally, challenges and future directions on the use of biological capping agents have been concluded. The goal of the present review article is to provide a comprehensive report to researchers who are looking for alternative biological capping agents for the green synthesis of important metallic nanoparticles.
Introduction
Nanoparticles are generally defined as small-sized particles with core-shell structure falling in the nanoscale range of 1–100 nm with broad properties like catalytic, optical, magnetic, mechanical, superior reactivity, electric, and thermal conductivity (Agarwal et al., 2017; Salem and Fouda, 2021; Ting and Chin, 2020). Over the last decades, the chemical and physical methods have been widely used for the large-scale synthesis of nanoparticles, but due to the usage of toxic solvents, harmful chemical capping agents, high energy input, low output yield, larger size, and their catastrophic effect on the environment, recently, the green synthesis involving biological capping agents has fetched more attention in the nanoscience field (Javed et al., 2020; Kumar et al., 2020; Priya et al., 2021). In this era of the 21st century, nanoparticles have been extensively used in the biomedical sector, including bioimaging [magnetic resonance imaging (MRI), computed tomography (CT), and positron emission tomography (PET)], biomarkers, drug delivery, cell labeling, gene delivery, magnetic hyperthermia, photoablation therapy, tissue engineering, bone regeneration, wound healing and dressing, antipermeability agents (in diabetic retinopathy), intracellular analysis, catalysis (artificial enzymes), medical devices (catheter modifications, prostheses, and vascular grafts), treatment of inflammation, cancer, pathogenic infections, and dental caries (biofilm inhibition) (Figure 1) (Burdusel et al., 2018; Magro et al., 2018; McNamara and Tofail, 2017; Khan et al., 2019; Kravanja et al., 2019; Shivaramakrishnan et al., 2017; Singh et al., 2020). As the biocompatibility and stability of nanoparticles in the biological fluids are the crucial factors, the surface of nanoparticles can be modified with different functional groups for their potent use in the biomedicine field (Ocsoy et al., 2018). Capping agents are widely employed to functionalize and stabilize the synthesized nanoparticles. Myriad chemical capping agents are utilized in the controlled synthesis of nanoparticles. However, being chemical in nature, they leave no meaning to green synthesis. To address the problems associated with the chemical capping agents, size, shape, agglomeration, and monodispersity of nanoparticles in the chemical and physical methods, green synthesis methods are based upon the usage of biodegradable, well-dispersed, biosoluble, biologically acceptable reducing, stabilizing, or capping agents so that they can be suitable in the living systems ( Singh et al., 2015; Javed et al., 2020; Ocsoy et al., 2018). Capping agents are defined as “binding molecules” that are used in minute quantity for the synthesis of nanoparticles (Sharma et al., 2021). These molecules mainly modulate the surface chemistry, morphology, and size distribution of the nanoparticles and shield them to prevent agglomeration and enhance the reduction kinetics of nanoparticles by forming complex structures with the metallic ions in the precursor salts (Li et al., 2013; Niu and Li, 2014; Campisi et al., 2016) (Figure 2). Majority of nanoparticle synthesis methods, including the biological method, rely on the use of chemical capping agents like surfactants (CTAB and SDS), polymers (PVP, PEG, and PGA), and thiols (dodecanethiol and thioglycerol) (Niu and Li, 2014). These molecules show a strong interaction with the surface of metal nanoparticles and thus serve as good stabilizing agents. However, a point value considered here is that these chemical capping agents are tough to detach from the surface of the nanoparticles and are nonbiodegradable, besides some conjointly being toxic to the biological systems. Thus, there may be a pressing want to go looking and searching for green capping agents in order to secure the biological system and the environment (Sharma et al., 2019). Keeping this in mind, green synthesis nowadays is focusing more on the naturally occurring reducing and stabilizing agents like carbohydrates (glucose, lactose, sucrose, fructose, cellulose, starch, and chitosan), proteins (collagen, enzymes, and albumin), amino acids (protein and nonprotein), lipids, honey, nucleic acid (DNA), and biological extracts (plants, bacteria, viruses, and fungal extracts) (Al-Zaban et al., 2021; Caschera et al., 2020; Chowdhury et al., 2014; Javed et al., 2020; Nogueira et al., 2019; Ocsoy et al., 2018; Ogundare and Zyl, 2018; Rather et al., 2019; Verma and Kumar et al., 2019). These are the best candidates for the fabrication of nanoparticles, and the conjugation of nanoparticles with these biomolecules could bring many remarkable biofunctions and protracted their biomedical applications (Miao et al., 2018). In this review, we mainly highlight the use of biological capping agents in the synthesis of nanoparticles, their therapeutic potential, and the efficiency of the capped nanoparticles.
Biogenic Capping Agents
The use of capping agents for controlling growth, aggregation, and physical and chemical properties is the key area of concern in the synthesis of nanoparticles. An appropriate choice of capping agents is required to regulate the stability and functionalization of the nanoparticles in the medium in which they are suspended (Javed et al., 2020; Maris;ca and Leopold, 2019). Different classes of green capping agents have been enlisted below with their roles in the synthesis of nanoparticles (Figure 3).
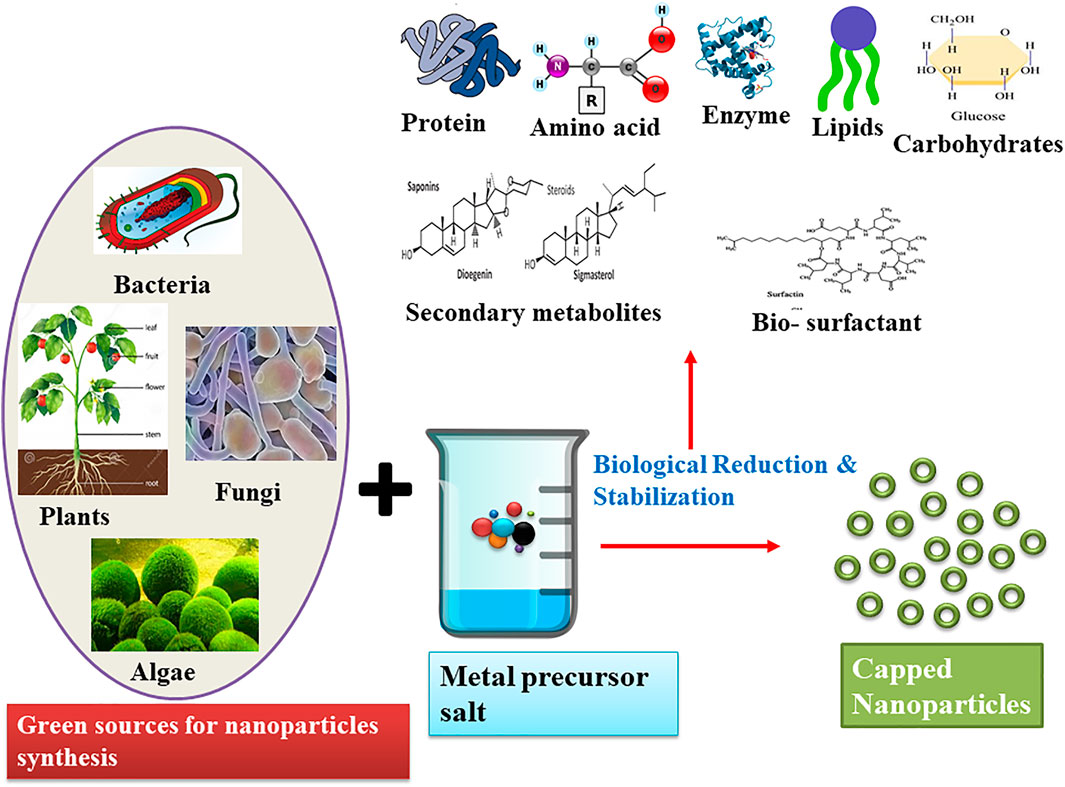
FIGURE 3. Schematic representation of the green synthesis of nanoparticles and their biological reduction and stabilization by various molecules.
Proteins
Proteins are an excellent source of distinctive functional groups that can be utilized in modulating the surface of the nanoparticles (Marișca and Leopold, 2019). From the literature study, it has been deduced that collagen is one of the most abundant natural animal proteins used for the capping of nanoparticles. Collagen is a widespread structural protein in mammals that is crucial for the formation of tissue and regeneration of organs and helps in maintaining the structural integrity of the extracellular matrix (Vijayakumar and Vaseeharan, 2018; Craciunescu et al., 2019; Municoy et al., 2021). It is the best material extensively used in nanoscience due to its biocompatibility, biodegradation, weak antigenicity, and the property of self-assembly and cross-linkage (Municoy et al., 2021). Using this ability of the collagen, Craciunescu et al. (2019) have explained the synthesis of silver nanoparticles via type 1 collagen as a reducing and stabilizing agent. In this method, three different concentrations of AgNO3 (0.1, 0.5, and 2.5 mM) were mixed with 0.3% (w/w) collagen gel in three variants. From the transmission electron microscopy (TEM) results, it has been observed that the intermediate concentration of AgNO3 (0.5 mM) yields the smallest-sized particles with an average diameter of 20 nm. Furthermore, the effect of collagen on the size and stability of AgNPs has also been compared with the suspension of silver nanoparticles in sodium citrate. The average diameter of AgNPs in collagen was ∼30 nm, whereas in the citrate suspension, the mean diameter of spherical NPs was 130.87 nm. Moreover, in the collagen-based suspension of AgNPs, no aggregation among the nanoparticles was observed, but in citrate suspension, the aggregates were seen during the storage at 4°C. This shows that nondenatured triple helical type 1 collagen molecules play a pivotal role in controlling the size and provide stability to the AgNPs by interacting with its amino acid residues and metal ions (Figure 4). In the same year, Vijayakumar and Vaseeharan highlighted the synthesis of collagen-coated ZnO nanoparticles by using the coprecipitation method. Electron microscopy results reveal that Cl–ZnO nanoparticles showed agglomerated hexagonal wurtzite shape with size in the range of 20–50 nm. Similarly, from the biomedical application point of view, this work also intensifies that biopolymer-capped zinc oxide nanoparticles had a greater ability for antimicrobial and anticancer activities. It has been studied that in the field of nanoscience, the physical properties (size, shape, and surface charge) of the nanoparticles influence their interactions with the living cells. Keeping this in mind, Marișca and Leopold (2019) put forward the novel simplified synthesis of gold nanoparticles by using hydrogen peroxide as a reducing agent and collagen as a stabilizing agent. Electron microscopy results demonstrate that Cl–AuNPs were anisotropic (raspberry-like), stable, and nonagglomerated and fall in the range of 60–80 nm.
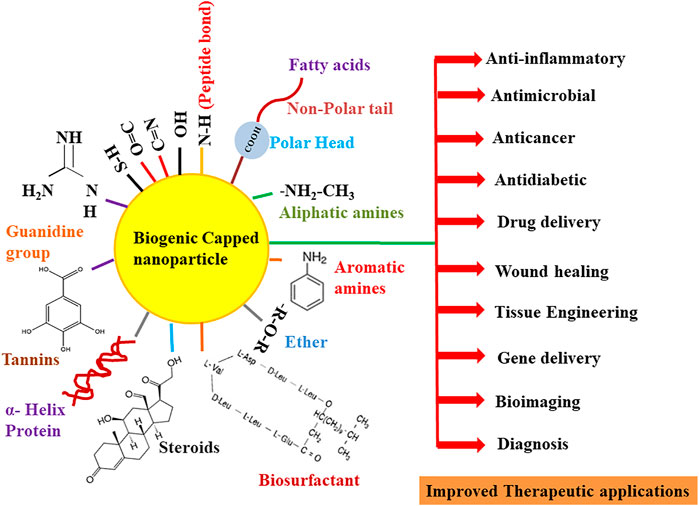
FIGURE 4. Overview of the role of various functional groups of biomolecules in the capping and therapeutic improvement of nanoparticles.
Besides collagen, different types of albumin (protein component of blood plasma) like ovalbumin (OVA), rat serum albumin (RSA), bovine serum albumin (BSA), and human serum albumin (HSA) are also considered eco-friendly proteins for the capping of the nanoparticles. Out of these proteins, BSA or HSA has been broadly used as a stabilizing agent due to its nontoxicity, low immunogenicity, biodegradability, high stability at 60°C temperature, high drug binding ability, selective drug delivery capability, and availability of natural binding sites (exposed ionic groups at C and N termini) (Nghiem et al., 2010; Pradeepa et al., 2017; Bolaños et al., 2019; Elsupikhe et al., 2020; Khodashenas et al., 2020). Pradeepa et al. (2017) elucidated the synthesis of silver nanoparticles in a foam matrix at room temperature by using HSA as a coating and stabilizing agent. The scanning electron microscopy (SEM) characterization of HSA–Ag nanoparticles indicates that particles were of face-centered cubic size, 20–30 nm in diameter, and without any aggregation. Similarly, Majeed et al. (2019) also demonstrated the anticancer property of BSA-capped and -uncapped AgNPs against bone, breast, and intestinal colon cell lines. The method of synthesis of silver nanoparticles was quite different from Pradeepa et al. (2017), as in this work, a completely green approach was used for the extracellular synthesis of silver nanoparticles by using the Bacillus cereus strain. TEM characterization results showed that uncapped AgNPs were spherical in shape and 5.37–17.19 nm in size, while on the contrary, BSA-capped AgNPs were larger in size (11.26–23.85 nm) but showed little agglomeration due to the interaction of AgNPs and BSA. This speculates that the coating of surface nanoparticles with biogenic capping agents prevents their aggregation among themselves. In the same year, Wang et al. (2019) synthesized in situ concentrated silver nanoparticles by using BSA as a stabilizer and costabilizer with other ligands (PVP and citrate). Interestingly, it has been found that though the PVP has been popularly used as a stabilizing agent for AgNPs, its capacity of stabilization was 40 times less than that of BSA. Various interactions like α-helix capping, peptide bond chelating, and different binding sites (thiol groups, guanidine side chains, and arginine and lysine residues) for functional groups, which are present in BSA (Figure 4), provide AgNPs more stability. In addition, BSA-capped AgNPs (10 ± 5 nm) were not only stable in the colloidal solution but also remained stable in the solid phase (after solvent water evaporation), 0.9% NaCl (physiological condition) without showing any agglomeration.
Amino Acids
Amino acids are the “building blocks of proteins” comprised of amine (–NH2), carboxyl functional groups (–COOH), and specific side chains in their structure. Due to the existence of side chains, these amino acid residues can interact with other compounds and hence act as eco-friendly, biocompatible capping agents (Figure 4) (Ghiyasiyan-Arani et al., 2018; Basnet et al., 2020; Hameed et al., 2020; Báez-Cruz et al., 2021). Maruyama et al. (2015) reported the synthesis of gold nanoparticles using 20 different types of amino acids (alanine, arginine, asparagine, aspartic acid, cysteine, glycine, glutamine, glutamic acid, histidine, isoleucine, leucine, lysine, methionine, phenylalanine, proline, serine, threonine, tryptophan, tyrosine, and valine). Out of these, histidine was considered the best reducing agent for gold chloride acid (HAuCl4, 0.5 mM) and capping agent for the synthesized Au nanoparticles. TEM results showed that with an increase in the concentration of His (25–100 mM), there is a direct decrease in the size of the nanoparticles (4–7.4 nm). This means that an increase in the histidine concentration prevents the aggregation of the Au nanoparticles. Another study based on the biological application of nonprotein amino acid as a stable, noncytotoxic capping agent for gold nanoparticles has been put forth by Verma and Kumar (2019). They, for the first time, utilized taurine (2-aminoethanesulfonic acid) as a capping agent in the synthesis of gold nanoparticles via an in situ wet chemical reduction method. They found that the average size of taurine-capped AuNPs with λmax 515 nm was 6.9 nm, whereas at λmax 545 nm, the size was 46 nm. These nanoparticles (108 and 1011 particles/ml) were further tested for a cytotoxicity study (MTT assay) on normal liver carcinoma cells (HepG2) (the major organ that accumulates nanoparticles during hepatotoxicity), and it was found that taurine-capped gold nanospheres were nontoxic, biocompatible, and safer as their size has no effect on cell death. This concluded that taurine is a harmless novel capping agent and is the best substitute to noxious chemical capping agents (CTAB and trisodium citrate) used in the synthesis of gold nanoparticles. Hameed et al. (2020) employed a biomimetic method for the synthesis of gold nanoparticles by using simply reducible aryldiazonium gold (III) salt AuCl4 with L-tyrosine, L-tryptophan, and L-cysteine amino acids as capping agents. TEM analysis showed that capped nanoparticles were spherical in shape and fall in the range of 27.2 ± 5.4 nm (for tyrosine AuNPs), 14.6 ± 7.7 nm (for tryptophan AuNPs), and 8.6 ± 2.6 nm (for cysteine AuNPs). The cytotoxicity study of amino acid-capped AuNPs (less than 6.25% concentration) was observed against normal human dermal fibroblast cells. This concentration of tyrosine-fabricated nanoparticles was considered safer, but in the case of cysteine and tryptophan nanoparticles, 53%–68% less viable cells were observed. Moreover, these capped nanoparticles also behaved as cell imaging agents as observed from a cellular uptake study in the viable HeLa cells.
Carbohydrates
Carbohydrates have also been used as fabricating agents for the large-scale production of nanoparticles with beneficial properties (Ghiyasiyan-Arani et al., 2018). Fructose, lactose, glucose, dextrose, starch, cellulose, sucrose, and chitosan are used as reducing and stabilizing agents due to their sustainability, abundance, low cost, harmless, renewability, biodegradable, and compatibility with biological systems (Ghiyasiyan-Arani et al., 2018; Caschera et al., 2020; Garza-Cervantes et al., 2020; Rather et al., 2019; Shanmuganathan et al., 2019). Maiti et al. (2019) illustrated the synthesis of silver nanoparticles by using carboxymethyl cellulose (CMC) as a primary reducing and capping agent, whereas dextrose was used as an additional reducing agent. The study showed that AgNPs were triangular in shape with 9.5 nm in size, and from the biological point of view, the antimicrobial activity of nanoparticles was also studied. It has been found that the antimicrobial property of CMC-capped AgNPs was 128–512 fold higher in comparison with native silver nitrate solution. Additionally, AgNPs were also employed as an ophthalmic medicine on a rat model, and it was concluded that CMC-capped nanoparticles were safer to use in treatment as no loss of vision, no opacity in the corona, and any corneal reflex change were observed in the rat after 7 days of application on the skin. Besides, Rather et al. (2019) explained the impact of capping agents (glucose, fructose, starch, and sucrose) on the bactericidal property, size, and absorption bands [surface plasmon resonance (SPR)] of Ag and Cu nanoparticles. It has been found that sugars showed a variable effect on the absorption peaks of silver and copper nanoparticles; as in the case of AgNPs, the maximum plasmonic band (λmax) (434 nm) was shown in sucrose-capped nanoparticles, but in CuNPs, λmax (641 nm) was observed in starch-mediated capped nanoparticles. Interestingly, the same type of sugars not only alters the surface plasmon resonance behavior but also alters the size of the nanoparticles. In the case of silver, the starch-mediated nanoparticles exhibited a size of 76 nm, whereas Cu fructose-mediated nanoparticles showed a maximum size of 164 nm. The main reason behind the variable size distribution of metallic nanoparticles by the same reducing agent is due to the structure and number of monomeric units present in the sugar molecules. Out of fructose, glucose, sucrose, and starch, both fructose and glucose showed easy stabilization of nanoparticles due to the presence of only one anomeric carbon atom; however, in the case of starch (polysaccharide) and sucrose (disaccharide, nonreducing sugar with no free anomeric carbon atom), strong alkali treatment was required for their fragmentation. Moreover, antimicrobial studies put on view that starch-based AgNPs were more effective than CuNPs using glucose, fructose, and sucrose. The larger zone of inhibition (1.82 cm) for Ag was observed for starch-based NPs, whereas for CuNPs, a larger zone of inhibition was seen in fructose-based nanoparticles (1.10 cm). In the end, we can conclude that different linkages of sugars affect the morphological, antimicrobial, and optical properties of the nanoparticles. From the literature studies, it has been inferred that silver nanoparticles are extensively used in the treatment of microbial infections, but how the capping agents modulate their antimicrobial activity has not been well illustrated (Nguyen et al., 2019; Radzig et al., 2013). Keeping this in view, Nguyen et al. (2019) compared the effect of chitosan and gelatin capping agents on the silver nanoparticle-based hydrogels and membranes. TEM characterization of GelAg and CsAg hydrogels showed that gelatin was the best stabilizer as nanoparticles were spherical, uniform, and monodispersed in gelatin hydrogels, whereas chitosan hydrogel particles were asymmetric and agglomerated in nature. Second, the average size of silver nanoparticles capped by chitosan was larger in size (20–40 nm) than gelatin-stabilized AgNPs (6–12 nm). The gelatin was considered a good stabilizer, but in antimicrobial assays, it has been found that chitosan-capped Ag hydrogels had lower MIC values and maximum antibacterial potency. On the other hand, in the case of Ag nanoparticle-coated membranes, GelAgNPs exhibited good antimicrobial properties than CsAgNPs. At last, we can infer that both stabilizing agents showed different behaviors in different application designs of AgNPs for wound treatment. Garza-Cervantes et al. (2020) explained the synthesis of the silver biocomposite using ascorbic acid as a reducing agent and microfibrillated cellulose (obtained from orange peels) as a capping agent and also studied the antimicrobial activity. TEM results showed that AgNPs were spherical in shape, capped with cellulose matrix, and fall in the average size of 140.79 ± 85.41 nm. Similarly, Caschera et al. (2020) also presented the fabrication of silver nanoparticles by using carboxylated nanocellulose (ONC, obtained from raw bagasse fibers) as a capping agent. The TEM, SEM, and atomic force microscopy (AFM) characterization of Ag–ONC nanocomposite showed that these exhibited spherical shape with diameter 4–10 nm with uniformly tight attachment to ONC fibrils. Accordingly, we can conclude that these molecules prevent the agglomeration of the nanoparticles due to the availability of hydroxyl and carbonyl groups in their structure that form hydrogen bonds with the surface of nanoparticles, also promote the nucleation of monodispersed nanoparticles, and hence provide stability (Figure 4) (Ghiyasiyan-Arani et al., 2018; Caschera et al., 2020; Garza-Cervantes et al., 2020).
Lipids
Any organic compound that is insoluble in water but soluble in organic solvents has been loosely classified as a “lipid.” These chemical characteristics can be found in fatty acids, phospholipids, sterols, sphingolipids, terpenes, and other compounds. Lipids are a complex and ubiquitous category of molecules that perform a variety of important biological tasks, including acting as structural components of cell membranes, storing energy, and engaging in signaling cascades (Fahy et al., 2011). Being the natural component of the cell membranes, lipids serve as the structural framework. Phospholipids make up the majority of cell membranes, which operate as a barrier to protect cell components from the outside world (Simons, 2016). Lipids have proved to be appropriate candidates for several biomedical applications where they form a biocompatible protective barrier on the nanomaterial surface, similar to their biological functions (Luchini and Vitiello, 2019).
Several fatty acids and edible oils have been tried and found to be excellent capping agents for the synthesis of a wide variety of metallic nanoparticles. Oleic acid is one of the most commonly employed fatty acids. In one study, Bi2WO6 nanoplates had been synthesized by a simple one-step hydrothermal technique by using oleic acid (OA) as a biological and cost-effective capping agent. From the SEM images, the particles were predicted to possess an average thickness of 90 nm along with an approximate width of 100–300 nm. It was determined from the FTIR research that oleic acid was chemically adsorbed via hydrogen-bonded carbonyl functional groups (Figure 4) on the Bi2WO6 surface, and also, there might be physical adsorption of oleic acid onto the nanotubes through van der Waals forces (Rahimi et al., 2016). In a similar study, Lu et al. (2008) compared the synthesis of magnetic Co nanoparticles using three long-chain acids—oleic, elaidic, and stearic acids—as capping agents. They discovered that elaidic acid, oleic acid, and a mixture of elaidic/stearic or oleic/stearic acids result in the narrow size distribution of synthesized nanoparticles with spherical shape. Overall, the oleic acid gave superior results, which is believed to stabilize the colloidal suspension, control particle growth, and prevent the final product from being oxidized by the air. The superior performance of oleic acid is mostly believed to be attributed to its nonlinear conformation. Oluwalana and Ajibade (2019) synthesized SnS nanocrystals at three different temperatures (150°C, 190°C, and 230°C) using bis(phenylpiperazine dithiocarbamate) tin (II) in oleic acid (OA) and octadecylamine (ODA). It was found that both the temperature and capping agent show a predominant effect on the size and shape of nanocrystals. The shape of the nanocrystals synthesized using oleic acid-capped SnS was spherical (OASnS), while the particles synthesized using octadecylamine (ODASnS) were cubic in nature. The size of the nanoparticles changes with the increase in temperature; monodispersed SnS with a size range of 10.67–17.74 nm was obtained at 150°C for OASnS, while the biggest-sized nanocrystals were obtained at 230°C.
Edible VOs such as rapeseed, corn, castor, sunflower, olive, coconut, and palm oils have been used as inexpensive and eco-friendly capping agents for the synthesis of various nanoparticles.
Thermodynamically, nanoparticles demonstrate a tendency to agglomerate and form larger particles after synthesis. VO and FA are amphiphilic molecules used to effectively control the aggregation of nanoparticles. The polar or hydrophilic end (carboxylic group) of FAs interacts with the nanoparticles, while the nonpolar aliphatic tails or hydrophobic end sterically disperses the particles (Figure 4). However, in an evolving nanotechnology industry, the use of edible oils as solvents poses an inherent challenge that may be related to the pricing and raw material rivalry (Mensah et al., 2018). In a research by Sadrolhosseini et al. (2017), gold nanoparticles were fabricated using palm oil as a capping agent at different temperatures (40°C, 64°C, 75°C, 83°C, and 92°C) to investigate the effect on the overall size of the particles. The use of palm oil has resulted in the formation of spherical gold nanoparticles with a size range of 8.92–19.73 nm. The increase in temperature is considered to cause the agglomeration of nanoparticles resulting in the formation of larger-sized particles. Cahyana and Reza (2018) reported the synthesis of magnetic iron oxide nanoparticles by the thermal decomposition reaction between rust, solvent (n-octadecane), and palmitic acid (as a capping agent). Fe3O4 nanoparticles with a size range between 8 and 10 nm were synthesized successfully using palmitic acid as a capping agent. In another study, silver nanoparticles were synthesized deploying an epoxidized derivative of ricinoleic acid, sodium 9,10-epoxy-12-hydroxytetradecanoate (SEAR), as a reducing and stabilizing agent. The concentration of SEAR was found to be an important factor contributing to the size of nanoparticles. Concentrations below the critical micelle concentration (CMC) of SEAR produced AgNPs of 23 nm in size with a standard deviation of 10 nm, whereas AgNPs of 50 nm (σ = 21 nm) were obtained at a concentration of above SEAR CMC (Costa et al., 2017). Table 1 illustrates the various biogenic capping agents (proteins, amino acids, carbohydrates, and lipids) in the synthesis of nanoparticles.
Fungal Extract as a Capping Agent
Fungi are considered one of the attractive sources for the biological synthesis of nanoparticles due to the presence of a large quantity of proteins, high yield of nanoparticles, ease of handling, and production of less toxic effluents. Fungi act as both reducing and stabilizing agents in the synthesis of metal nanoparticles (Ninganagouda et al., 2013). The biomolecules present in the fungi confer a coating onto the nanoparticles, which increase their stability, inhibit agglomeration, and aid in enhancing the biological activity of nanoparticles (Guilger-Casagrande and Lima, 2019; Priya et al., 2021). These biomolecules pose a high affinity toward binding onto the surface of the nanoparticles, with mostly proteins and amino acid residues coating the nanoparticle surfaces (Basavaraja et al., 2008). The free amino groups or cysteine residues are believed to interact and bind the nanoparticle surfaces. The cell wall enzymes are also assumed to provide negative carboxyl groups adding to the electrostatic attraction between the nanoparticles and these biomolecules (Figure 4) (Gole et al., 2001; Durán et al., 2011; Husseiny et al., 2015). Furthermore, the nucleophilic (OH−) ions present on the surface of the nanoparticles are thought to supply electrons required for the reduction of the metal ions resulting in the production of nanoparticles demonstrating narrow size distribution (Gurunathan et al., 2009). Several studies have demonstrated the role of biomolecules from fungal extract, acting as a capping agent. Gudikandula et al. (2017) synthesized biogenic silver nanoparticles using two white-rot fungi Trametes ljubarskyi and Ganoderma enigmaticum. FTIR analysis revealed bands at 3,385 and 1,638 cm−1 representing primary amine (N–H) stretching and amide (N–H) bending vibration, respectively. C–N stretching vibrations of the aromatic and aliphatic amine were attributed to the bands observed around 1,383 and 1,032 cm−1, confirming the presence of proteins on the surface of nanoparticles for their stabilization (Figure 4). Elgorban et al. (2016) synthesized biological silver nanoparticles using Aspergillus versicolor and established the presence of a capping from SEM and EDS analysis. While noticing the strong signal at about 3 keV corresponding to Ag, the workers also reported signals for oxygen and carbon, indicating the presence of organic compounds getting adsorbed on the nanoparticles from the culture filtrate. Kadam et al. (2019) synthesized ZnO nanoparticles employing zinc-tolerant endophytic fungi identified as Cochliobolus geniculatus. To confirm the presence of proteins as capping agents on the surface of the synthesized nanoparticles, the particles were treated with 1% SDS for 10 min, followed by centrifugation at 8,500 g for 10 min. The obtained supernatant was further denatured using 3X Laemmli’s dye, followed by heating for 5 min and centrifugation for 1 min at 8,500 × g (4°C). The sample thus obtained was separated by SDS–PAGE, which demonstrated the presence of numerous protein bands in the range of 97–36 kDa. The occurrence of capping protein on the ZnO nanoparticle surface was evaluated by recording fluorescence spectra at an excitation wavelength of 280 and 325 nm, respectively. UV-vis spectroscopy analysis of nanoparticles exhibits a typical signature peak at 280 nm. The protein shows a strong absorption at 282 nm due to π → π transitions arising from aromatic amino acids tyrosine and tryptophan of proteins present on the surface of the particles. Further, FTIR analysis also revealed the presence of several bands specifying the protein coating on the surface of the nanoparticles. Cuevas et al. (2015) synthesized copper and copper oxide (Cu, Cu2O, and CuO) nanoparticles by a white-rot fungus Stereum hirsutum. The FTIR and XRD studies of all three synthesized nanoparticles portray the presence of proteins and carbohydrates on the surface of these nanoparticles. Besides, the typical FTIR bands corresponding to C–N stretching vibrations of aromatic and aliphatic amines, bending vibration movements in amides I, II, and III, bands at 900–1,105 cm−1 indicating the presence of pyranose ring stretching, glycosidic ethers, and beta-glycosidic linkages of some polycarbohydrates were also observed (Figure 4). The XRD analysis demonstrates the presence of peaks indicating the existence of polycarbohydrate as surface capping on the particles. Another study conducted by Das et al. (2009) for the synthesis of AuNPs by the viable biomass of Rhizopus oryzae postulated the possible involvement of phosphate bonds in the interaction of proteins on the nanoparticle surface. EDX studies indicate the C, N, and O signals representing the presence of biomolecules on the surface of nanoparticles along with strong peaks corresponding to Au. A shift in FTIR bands from 1,034 to 1,025 cm−1 observed could be assigned to the phosphate bond intervention in this interaction process of proteins on the surface of the nanoparticles. Majority of the studies involving the use of fungi as reducing and stabilizing agents put forward the fact that proteins along with carbohydrates to a certain extent are present in the extract acting as the sole capping agent. Yeast has also been employed widely as a reducing-cum-capping agent for the synthesis of monodispersed nanoparticles. In one such study, Shu et al. (2020) synthesized silver nanoparticles using yeast extract for the reduction of AgNO3 solution. The shape and size as revealed by the SEM image is spherical with an average particle size of 13.8 nm. Data obtained through EDX, FTIR, and zeta potential (helps in depicting the surface electrostatic potential at the junction between the diffuse and compact layers of the nanoparticles) measurement clarifies the presence of surface coating, dispersion ability of the colloidal solutions, and long-term storage stability of the synthesized particles. Proteins and amino acids are regarded as the main molecules acting as capping agents. The zeta potential of AgNPs decreased drastically from −12.1 mV at pH 7.0 to −24.4 mV at pH 11.0, which defines the presence of negatively charged groups on the surface of AgNPs. Overall, it is assumed that the surface amino acid carries a net negative charge at the alkaline pH providing storage stability for more than a year without the formation of any precipitate.
Algal Extract as a Capping Agent
Algae have been exploited industrially and commercially for ages as food, feed additives, fuels, fertilizers, pharmaceuticals, and cosmetics (Sharma and Sharma, 2017). Recently, a drastic shift has been noticed in this field, and now algae are looked at as one of the green resources for the synthesis of various nanoparticles. Algae are a rich source of proteins, peptides, secondary metabolites, and pigments, which make them an attractive nanofactory (Negi and Singh, 2018). Working with algae is advantageous as they are fast-growing, can grow in a simple, inexpensive medium, offer ease of scale-up, and are easy to culture and harvest (Fabris et al., 2020). Various classes of algae like red algae (Rhodophyceae), blue-green algae (Cyanophyceae), green algae (Chlorophyceae), and brown algae (Phaeophyceae) (Abdel-Raouf et al., 2017; Aboelfetoh et al., 2017; Khan et al., 2018; Fatima et al., 2020) have been widely employed for the synthesis of metallic nanoparticles. Like plants and fungi, algal extracts are expected to act as both reducing and capping agents in the synthesis of metallic nanoparticles (Chaudhary et al., 2020).
The biomolecules present in the extract reduce the metal ions and coat the surface of the nanoparticles. Proteins, amino acids, and secondary metabolites such as terpenoids, alkaloids, and phenolic compounds secreted extracellularly by algae are considered main candidates for surface capping (Figures 3 and 4). Moreover, the alkaline pH of the algal extract is also considered to play a crucial role in the stabilization of the synthesized nanoparticles. The higher pH enhances the reducing power of the surface functional groups preventing particle agglomeration. Alkaline pH is also believed to interact with the amino group of proteins and amino acids which aid the stabilization of the particles (Parial et al., 2012; Namvar et al., 2015). Gold nanoparticles were more stable in the pH range of 5–9, whereas their agglomeration was observed at pH 3 (Namvar et al., 2015). In a study carried out for the green synthesis of silver nanoparticles using green algae Caulerpa serrulata, the FTIR study reveals the existence of various bands depicting the stretching of (O–H) and C=O groups, (C–H) stretching of alkanes, stretching vibration of the alkene C=C group, and C≡C stretching. This strongly recommends the presence of organic biomolecules as capping agents on the surface of the synthesized AgNPs (Figure 4). These workers also investigated the effect of varied extract concentration, temperature, and pH on the size and shape of synthesized NPs. The results showed that AgNPs synthesized at higher temperature (95°C), at higher pH values (>6.65), and at higher extract concentration are superior being crystalline and spherical with an average particle size of 10 ± 2 nm (Aboelfetoh et al., 2017). Abdel-Raouf et al. (2017) synthesized the gold nanoparticles by using Galaxaura elongata. The results of the FTIR study showed the presence of three bands at about 332.49, 2,158.12, and 1,635.09 cm−1 for the ethanolic extract and at 3,414.96, 2,158.28, and 1,640.60 cm−1 for nanoparticles synthesized using algal powder, which could be assigned to amide bands of proteins, as well as carbonyl stretching and free N–H stretching vibrations, respectively, in the amide linkages of the proteins (Figure 4). The study thus indicates the role of proteins in the extract acting as capping and stabilizing agents. Another study conducted by the same researchers dealt with the synthesis of highly stable silver nanoparticles employing marine brown alga Padina pavonia. The synthesis was carried out using algal powder and organic extracts (ethanol and chloroform). The workers noticed the synthesis of the Ag nanoparticles using powder form in the stunningly shorter duration of 2 min as compared with the 3 h taken by ethanol and chloroform extracts. The HPLC and GC–MS analysis for the algal extracts proved the presence of high values of natural functional compounds, such as alkaloids, terpenes, amino acids, and fatty acids, which were considered to act as stabilizers and were responsible for preventing particle agglomeration (Abdel-Raouf et al., 2017). Sinha et al. (2015) carried out the biological synthesis of silver nanoparticles using freshwater green alga Pithophora oedogonia by the reduction of silver nitrate. It was observed that the synthesis process was extremely fast, and the reduction of silver ions to silver nanoparticles using algal extract happened within a few minutes. The preliminary phytochemical analysis of the extract revealed the presence of saponins, carbohydrates, steroids, proteins, tannins, and terpenoids. FTIR analysis of the synthesized nanoparticles demonstrated the presence of various bands concluding the involvement of long-chain fatty acids, terpenoids, and secondary amide derivatives to be possible biomolecules acting as capping and stabilizing agents (Figure 4).
Araya-Castro et al. (2021) attempted a novel protein-assisted method for the synthesis of biogenic CuO NPs using separated protein fractions from an aqueous extract of Macrocystis pyrifera (brown algae) through size exclusion chromatography (HPLC–SEC). The study was carried out to prove the potential of proteins present in the extract acting as reducing and capping agents in the synthesis process. TEM images inferred the synthesis of spherical nanoparticles ranging from 2 to 50 nm in diameter in all the protein fractions. EDX analysis of nanoparticles depicted a strong signal for copper (Cu) and carbon (C) which is assumed to be from the surface coating of proteins in the synthesized nanoparticles. Another interesting study was conducted by Xie et al. (2007) to synthesize single-crystalline gold nanoplates by the reduction of chloroauric acid with the extract of green alga Chlorella vulgaris. The study suggests proteins as the principal biomolecules involved in providing the dual function of Au reduction and the size- and shape-controlled synthesis of the nanogold crystals. Further, a protein with a molecular weight of around 28 kDa was extracted and purified by using reversed-phase HPLC; the isolated protein was examined for the specific reduction of chloroauric acid in an aqueous solution. The sole use of this protein resulted in the production of triangular nanoplates with hexagonal shapes, demonstrating a yield of 90%. Later, this protein was named as gold shape-directing protein (GSP).
Bacterial Extract as a capping Agent
Bacteria depict an extraordinary ability to reduce a variety of metal ions and hence are emerging as one of the best candidates for the synthesis of nanoparticles. Some bacterial species have developed the mechanism to tolerate metal stress, including heavy metal toxicity. For instance, many bacterial species can survive and grow at extremely high metal ion concentrations (Iravani, 2014). Bacteria can not only tolerate high concentrations of metals but can also convert these metal ions into nontoxic metal nanoparticles. Due to this reason, bacteria are looked at as alternative nanofactories for the synthesis of metal nanoparticles. The use of bacteria offers certain obvious advantages like a high degree of adaptability to extreme conditions, fast growth rate, low energy consumption, and superior process controllability (Tsekhmistrenko et al., 2020). Nanoparticle formation in bacteria can be intracellular or extracellular. Extracellular synthesis is more convenient because of the easy separation of the nanoparticles. In addition to this, these particles are observed to be more resistant to environmental oxidation after their synthesis (Gahlawat and Choudhury, 2019). Bacterial extracts like other organisms are considered to act as both reducing and stabilizing agents in the synthesis of nanoparticles. Out of the biomolecules present in the bacterial extract, biosurfactants have been explored widely for nanoparticle synthesis. Biosurfactants are amphiphilic molecules that are capable of playing a vital role in the process of nanoparticle stabilization (Kumar et al., 2010). These molecules get adsorbed on the surface of the metallic nanoparticles, preventing their aggregation and leading to their stabilization (Figure 4). One of the modes of action is through adsorption onto metallic nanoparticles. The mechanism of surfactant adsorption is determined by the type of surfactant (polymeric, ionic, nonionic, etc.) and the thickness of the layer adsorbed on the surface of the particles (Płaza et al., 2014).
Joanna et al. (2018) synthesized biogenic silver nanoparticles using Bacillus subtilis grown on agro-industrial wastes, such as brewery effluents and molasses. The synthesized nanoparticles were evaluated for their antimicrobial activity and were compared with the chemically synthesized silver nanoparticles. Biosurfactants present in the cell-free extract had assumed to cap the nanoparticles. The presence of biosurfactant coating considerably increased the stability of biogenic AgNPs and improved their antimicrobial activities. These nanoparticles have demonstrated broad-spectrum activity against bacteria and fungi. Salazar-Bryam et al. (2021) investigated the effect of pH on the synthesis of silver nanoparticles stabilized by glycolipid surfactants: rhamnolipids. A comparison of physical properties and nanoparticle stability was carried out for the particles synthesized at pH 5, 7, and 9. Dynamic light scattering techniques were studied to evaluate particle size—larger particles were formed at pH 5 (78–190 nm), whereas smaller-sized particles were formed at pH 9 (5.6–28.1 nm). Similar results were obtained for nanoparticle stability (analyzed based on the zeta potential), where the highest potential was noticed at pH 9, demonstrating enhanced particle stability. Spherical morphology of the particles was confirmed using field emission gun scanning electron microscopy. More particle aggregation was observed at low pH of 5, which could be because of the protonation of the carboxylic acid group of the rhamnolipids, which overweigh the electrostatic repulsion forces resulting in increased particle size. The rhamnolipids acted as capping agents, reducing the formation of aggregates, determining the particle size, and also stabilizing the colloidal suspension. Lipopeptide biosurfactants have also been reported in nanoparticle synthesis. Similar results were obtained by Reddy et al. (2009) while synthesizing Ag and Au nanoparticles deploying lipopeptide biosurfactants: surfactin produced by Bacillus subtilis. While comparing the effect of pH 5, 7, and 9 on the particle size and stability, they too inferred that the nanoparticles synthesized at pH 9 were smaller in size and were stable for 2 months. The silver nanoparticles synthesized by Brevibacterium casei using a glycolipid biosurfactant were more stable than the nanoparticles synthesized without a surfactant. These workers checked the aggregation of the nanoparticles by placing them at room temperature for different time intervals. The use of surfactants enhanced the stability up to 2 months, whereas control particles synthesized in the absence of surfactants aggregated within 7 days. The data concludes that the surfactant acts as a stabilizing agent and prevents particle agglomeration (Kiran et al., 2010). Xu et al. (2018) confirmed the presence of protein and polysaccharide capping onto selenium nanoparticles synthesized by Lactobacillus casei. SEM, TEM, and EDX images of the prepared SeNPs showed the presence of spherical particles consisting of carbon (C), nitrogen (N), oxygen (O), phosphorus (P), sulfur (S), and Se elements. Furthermore, the FTIR analysis demonstrates the stretching vibration peak of O–H and N–H at 3,323 cm−1, corresponding to polysaccharide and protein residues. Stretching vibration of C–H in the sugar ring or peptide chain could be assigned to the band observed at 2,923 cm−1. The bands at 1726 and 1,467 cm−1 can be related to the stretching vibrations of C=O and COO–, respectively, while the bands at 1,631 cm−1 could be associated with the stretching vibrations of C=C and C=N. The concentration of Se, protein, and polysaccharide in the purified SeNPs was 7.6%, 70.88%, and 14.17%, respectively. This data denoted the presence of protein and carbohydrate coating on the surface of the prepared nanoparticles.
Plant Extract as a Capping Agent
Plants have gained immense importance in the field of nanotechnology due to their cost-effectiveness, less toxicity, rapidity, availability, and nonpathogenicity; the economic protocol includes mild conditions for biosynthesis, large-scale production, and a broad range of biological activities (Jadoun et al., 2021; Sivakumar, 2021; Tadele et al., 2021). Different parts of plants, including the leaf, stem, root, fruit, bark, flowers, rhizomes, callus, seed, bud, and latex, have been extensively utilized in the green synthesis of nanoparticles due to the presence of various biomolecules. The plant-based bioconstituents like steroids, sapogenins, tannins, terpenoids, polyols, alkaloids, polysaccharides, flavonoids, phenolics, proteins, amino acids, enzymes, and vitamins (Figures 3 and 4) function as strong chelating, reducing, and stabilizing agents, which provide stability, prevent agglomeration, and help in tuning the shape and size of the nanoparticles (Adelere and Lateef, 2016; Nayak et al., 2020; Din et al., 2021; Jadoun et al., 2021; Restrepo and villa, 2021; Sivakumar, 2021; Tadele et al., 2021). A research study carried out by Shaheen and Ahmad (2020) identified the different biogenic capping agents [phenols (ascorbic acid, resorcinol, 4-nitrophenol, umbelliferone, vanillic acid, tannic acid, phenol, rutin, and ellagic acid), flavonoids, alkaloids, acetic acid, D-alanine, and aromatic and aliphatic amines (octodrine)] by HPLC and GC–MS from the leaves of Nasturtium officinale for the synthesis of Mo3 nanoparticles. The data concluded by this report confirmed the presence of phytochemicals on the surface of Mo3 nanoparticles. SEM results of synthesized nanoparticles showed different shapes of particles with C and O elements at different magnifications. Moreover, FTIR analysis speculates that vibration peaks at 461.5 cm−1 represent Mo3 particles along with stabilizing agents at 1,637.62, 1,484.94, and 1,021.6 cm−1, which correspond to the primary amine, aromatic, and aliphatic amine functional groups. This work concluded that phytochemicals not only help in the reduction process but also minimize the aggregation of nanoparticles. Furthermore, Nayak and his coworkers highlight the contribution of bioactive compounds in the synthesis of silver nanoparticles by using Dillenia indica L. leaf extract. In this study, the FTIR study showed the various peaks at 541, 668, 778, 862, 1,044, 1,119, 1,266, 1,318, 1,384, 1,427, 1,459, 1,597, 2,853, 2,923, and 3,416 cm−1. The peaks at 3,416, 1,597, 668, 778, and 862 cm−1 correspond to free –COOH groups, as well as stretching modes of nitro (containing oxygen molecules) and functional groups of esters. These stretching vibrational peaks clearly indicate the presence of biomolecules as stabilizing agents. TEM results demonstrate that AgNPs were spherical in shape with size in the range of 10.01–23.24 nm. Moreover, zeta potential measurements exhibit that exterior parts of nanoparticles were negatively charged with a sharp peak at −39.5 mV zeta potential. This negative value states the repulsion among the synthesized nanoparticles, which in turn were stable for a long time. An interesting work done by Yulizar et al. (2020) for the first time showed the synthesis of cerium nanoparticles (CeO2) by using Datura metel leaf extract (DME). Various literature studies showed that secondary metabolites play a key role in the reduction of metal ions and redox reactions for the formation of a nanosized mass of metal oxide nanoparticles (Nayak et al., 2020), so these workers initially performed phytochemical screening to confirm the presence of secondary metabolites (alkaloids and flavonoids). FTIR analysis of DME showed the strong absorption bands at 1,030, 1,408, and 1,610 cm−1 that corresponded to C–N stretching vibrations (indicates the presence of aliphatic amine groups of alkaloids), C=O carbonyl groups, and C–C aromatic groups (indicates flavonoids). In addition, SEM and TEM characterization of CeO2 nanoparticles showed that particles were spherical in shape, agglomerated, and were 5–15 nm in size. This result rendered that secondary metabolites play a multifunctional role of a weak base source, as well as oxidizing, capping, and chelating agents without the addition of any base chemical compound in the preparation of nanoparticles. Similarly, Sahayaraj et al. (2020) also showed that the presence of secondary metabolites in the plant extract not only contributes as fabricating agents but also enhances the dispersity and stability of the nanoparticles. These workers synthesized AgNPs by using the dry leaf aqueous extract of the Pongamia glabra tree, and FTIR characterization of silver bionanoparticles demonstrates that amines (vibration band at 771 cm−1) and amides (peak at 1,630 cm−1) were responsible for the reduction of metal ions and capping agents. SEM results exhibit that synthesized silver nanoparticles were well dispersed, with largely spherical shape (67.8%) and few squares (38.2%) with 29.4 nm in size, and were highly dense in nature due to the electrostatic interactions between the capping molecules on the surface of AgNPs. Further, nanosilver ions were also stabilized as there was no direct contact among them. Interestingly, a fascinating study has been conducted by Kambale et al. (2020) that showed the effect of different plant leaf extracts [Brillantaisia patula (BR-PA), Crossopteryx febrifuga (CR-FE), and Senna siamea (SE-SI)] on the optical, structural, surface morphology, and biological properties of the silver nanoparticles. It has been observed that Ag nanoparticles synthesized from the aqueous extract of BR-PA, CR-FE, and SE-SI showed different UV-vis absorption bands at 434, 489, and 522 nm due to the surface plasmon resonance (SPR). The main reason for distinctive absorption peaks has been correlated with the interaction of phytoconstituents with the surface of the nanoparticles. EDX analysis showed the existence of carbon and oxygen atoms on the surface of AgNPs, supporting the difference in the SPR absorption bands of three types of AgNPs from BR-PA, CR-FE, and SE-SI extracts due to the difference in the phytochemical composition of the extracts that has been validated by FTIR analysis. The sharp round bands at around 3,325–3,198 cm−1, 2,917–2,833 cm−1, 1,380–1,360 cm−1, and 1,050–1,025 cm−1 correspond to alcoholic (O–H), alkane (C–H), phenolic (O–H), and (C–O) stretching vibrations, whereas 1,640–1,620 cm−1 stretching vibrations were with respect to the carbonyl group (C=O) from carboxylic, aldehyde, ketone, or ester-containing compounds. The particle size distribution from TEM results indicates that AgNPs were 45 nm, 115 nm, and 47 nm, which correspond to BR-PA, SE-SI, and CR-FE extracts. This confirms that the difference in the size, UV-vis, and FTIR behavior of the synthesized nanoparticles was due to the presence of various types of phytoconstituents in the extract.
Biomedical Applications of Capped Nanoparticles
Antimicrobial agent: It is a known fact that different metallic nanoparticles like Au, Ag, ZnO, Pd, Cu, Pt, Ti, and Fe possessed antimicrobial activity against bacteria, fungi, viruses, etc. (Puja and Kumar, 2019). Generally, the basic mechanism for the antimicrobial action of these nanoparticles is based upon the oxidative and nonoxidative stress, metal ion release, electrostatic interaction between the metal ion and the plasma membrane, and inhibition of ATP-dependent protein synthesis, and inducing DNA damage will in turn lead to ROS production, lipid peroxidation, and protein oxidation (Singh et al., 2018; Puja and Kumar, 2019). Various studies elucidate the antimicrobial property of biogenic-capped nanoparticles against pathogenic microorganisms. For instance, the research of Vijayakumar and Vaseeharan on zinc oxide showed that 75 μg/ml concentration of Cl–ZnO NPs exhibited greater antibacterial and antibiofilm activity against Gram-positive (Streptococcus mutans) and Gram-negative (Proteus vulgaris) bacteria and fungi (Candida albicans). Additionally, Garza-Cervantes et al. (2020) also showed that cellulose-capped AgNPs were effectively used as antimicrobial agents for killing antibiotic microbial-resistant and -sensitive bacteria. It has been found that Ag–cellulose composite showed an antimicrobial efficiency five times higher than the optimum clear zone value obtained for Gram-negative strains, whereas in the case of Gram-positive strains, these showed 10 times more efficiency. Moreover, Caschera et al. (2020) also reported the antibacterial activity of nanocomposites against Gram-positive (Bacillus subtilis and Staphylococcus aureus) and Gram-negative bacterial strains (Escherichia coli and Pseudomonas aeruginosa). Antibacterial assay results speculate that Ag–ONC nanocomposites have low solubility in the agar media but had a good antibacterial activity (12–14-mm inhibition zone) against both types of bacteria.
Anticancer: In a similar trend, biologically synthesized nanoparticles also exhibited antitumor and cytotoxic activity. Besides an antibacterial study, Vijayakumar and Vaseeharan also highlighted the cytotoxicity activity of collagen-capped ZnO NPs (75 μg/ml) against normal murine macrophages (RAW 264.7) and human liver cancer cells (HepG2). This intensifies that biopolymer-capped zinc oxide nanoparticles had a greater ability for anticancer activities. Furthermore, the research work of Pradeepa et al. (2017) for the first time showed the antiproliferative effect of silver nanoparticles against breast cancer cell lines (MCF-7). MTT assay results speculate the cytotoxic effect of synthesized AgNPs in MCF-7 cell lines; moreover, apoptosis (cell shrinkage, impaction of nuclei, and fragmentation of chromatin) was also observed in the treated cell lines. This clearly indicates that AgNPs inhibit the growth of cancer cells and can be used as therapeutic agents for cancer treatment. Similarly, Majeed et al. (2019) comparatively showed the cytotoxic analysis of both the capped and uncapped nanoparticles against MCF-7, HCT-116, and MG-63 cell lines. It has been inferred that a low concentration of capped AgNPs (80 μg/ml) showed half-maximal inhibition (IC50), whereas uncapped AgNPs showed IC50 at 100 μg/ml against MCF-7 and MG-63 cells. In the case of HCT-116 cell lines, 60 μg/ml of BSA–AgNPs and 80 μg/ml of AgNPs showed IC50. Besides this, capped AgNP-treated cell lines also showed maximum release of lactate dehydrogenase (LDH) enzyme in comparison with uncapped nanoparticle-treated cancerous cell lines. This confirmed that cAgNPs were used as antitumor agents as they kill the cancer cells by increasing programmed cell death (apoptosis).
Anti-inflammatory: Due to the increase in the risk of chronic inflammatory diseases like fibromyalgia, gastrointestinal, kidney, cardiovascular, diabetes, neurodegenerative, musculoskeletal, or rheumatic diseases, there has been a paradigm shift from the usage of anti-inflammatory drugs (aspirin, acetylsalicylic acid, diclofenac, ibuprofen, etc.) to the nanoparticulate drug delivery systems which facilitate the solubility, bioavailability, and targeted delivery of drugs (NDDS) (Oyinloye et al., 2015; Placha and Jampilek, 2021). Various nanoformulations of drugs include hydrocortisone, budesonide, methylprednisolone-encapsulated chitosan and BSA nanoparticles, dexamethasone incorporated into solid lipid nanoparticles, celecoxib-loaded silk fibroin nanoparticles, and hyaluronan nanocapsules; experimental evaluation on treated mouse models and cell cultures showed that nanoformulated drugs have more anti-inflammatory activity [decrease in immune markers (histamine, IgE, helper T cells, prostaglandin E2, and EGFα), IL-1, TNF-α, IL-10, NO, and IL-6; increase in antioxidant activity (ROS scavenging)] than normal drugs (Gai et al., 2018; Crivelli et al., 2019; Choi et al., 2020; Ramos Campos et al., 2020; Nedelcu et al., 2021).
Biosensors, medical diagnosis, and imaging: Different nanoparticles like silver, gold, superparamagnetic iron oxide, titanium dioxide, manganese dioxide, cerium, zinc oxide, liposome, and solid lipid nanoparticles are widely used in diagnostic, biomedicine, and medical imaging like MRI, ultrasound imaging, computed tomography, positron emission tomography, and single-photon emission computed tomography as highly sensitive probes for targeting and imaging small molecules like DNA, proteins, cell tissue, and tumors (Carrasco-Esteban et al., 2021; Lee and Jun, 2019; Mirahadi et al., 2018; Silva et al., 2021).
Wound healing: Wound dressing is usually required for the maintenance of the environment, eliminating the growth of other invading microorganisms, and the removal of excess fluid and dead cells around the wound. To overcome the problems (narrow antimicrobial spectrum, skin allergies, irritation, and cytotoxicity) related to the use of conventional drugs [glucocorticoid steroids and nonsteroid anti-inflammatory drugs (ibuprofen, naproxen, diclofenac, ketoprofen, indomethacin, and acetylsalicylic acid)], various types of metallic nanoparticles have been used in the healing process (Chugh et al., 2021). It has been found that silver, zinc oxide, and gold nanoparticles coated with biomolecules like polysaccharides and biological extracts help in the wound healing mechanism. These nanoparticles trigger apoptosis, tissue necrosis, platelet and stem cell activation, angiogenesis, tissue scar formation, and bacterial clearance and reduce cytokines (IL-6 and IL-10) and reepithelialization (Wang et al., 2017; Ovais et al., 2018; Batool et al., 2021; Chugh et al., 2021).
Drug delivery: Chemotherapeutic agents have been widely accepted for the treatment of diseases, but these have some limitations like high toxicity, short lifespan in the body, low solubility, and large volume of distributions (Wang et al., 2017). Nowadays, nanoparticles are utilized in targeted drug delivery systems in order to increase the efficiency of chemical agents (antineoplastic drugs). Silver, gold, zinc, copper, iron oxide, titanium dioxide, cerium dioxide, and iron–platinum have achieved considerable attention in this field due to their small size and ability to cross biological membranes (Andleeb et al., 2021; Desai et al., 2021; Nikzamir et al., 2021; Roy et al., 2021).
Concluding Remarks
Capping agents play a crucial role in controlling particle size, morphology, agglomeration, surface energy, grain growth, dispersion, and electrostatic and steric hindrance by providing specific functional groups on the surface of nanoparticles. Keeping in mind the limitations of chemical capping agents, green chemistry has been applied for reducing, stabilizing, and fabricating synthesized nanoparticles. In this review, functions of various biomolecules like proteins, amino acids, lipids, carbohydrates, and biosurfactants (as shown in Table 1) and biological extracts along with their medical applications have been emphasized. Natural substances used to synthesize and/or cap the nanoparticles offer various advantages over synthetic medications, including fewer adverse effects; therefore, biological materials have long piqued the interest of researchers. Biological molecules (collagen, BSA, amino acids, lipids, and carbohydrates) coating onto the nanoparticles not only reduce their side effects but also improve their characteristics like small size, long-term stability, and biocompatibility (speed up the uptake and retention of nanoparticles) and enhance antimicrobial and anticancer activity All the capping agents discussed in this paper provide surface functionalization and reduce the size of nanoparticles by preventing agglomeration in addition to increasing their storage stability. Comparison between these agents to determine the best one is difficult. Thus, the choice of the suitable green capping agent can be made by considering the surface functional groups introduced by them and their availability and cost. However, these capping agents offer several advantages; storage stability (in the case of biological extracts), homogenous fabrication, toxicity levels, and somehow agglomeration of nanoparticles based upon biogenic capping agents are the biggest issues that need considerable attention. With the pace of time, the demand for nanoparticles in the biomedical sector has been increasing day by day; therefore, for large-scale production, further research is urgently required to address the issues especially related to yield, stability, uniformity, reproducibility, and kinetics. Moreover, intensive research is also mandatory for exploring the mechanism of biomolecules as capping agents in the green way-mediated biosynthesis processes.
Author Contributions
AS, NV, and PK designed and wrote the manuscript and revised and finalized the draft. AS and PK supervised the whole project and reviewed the manuscript. All authors contributed to the article and approved the submitted version.
Conflict of Interest
The authors declare that the research was conducted in the absence of any commercial or financial relationships that could be construed as a potential conflict of interest.
Publisher’s Note
All claims expressed in this article are solely those of the authors and do not necessarily represent those of their affiliated organizations, or those of the publisher, the editors, and the reviewers. Any product that may be evaluated in this article, or claim that may be made by its manufacturer, is not guaranteed or endorsed by the publisher.
References
Abdel-Raouf, N., Al-Enazi, N. M., and Ibraheem, I. B. M. (2017). Green Biosynthesis of Gold Nanoparticles Using Galaxaura Elongata and Characterization of Their Antibacterial Activity. Arabian J. Chem. 10, S3029–S3039. doi:10.1016/j.arabjc.2013.11.044
Aboelfetoh, E. F., El-Shenody, R. A., and Ghobara, M. M. (2017). Eco-friendly Synthesis of Silver Nanoparticles Using green Algae (Caulerpa Serrulata): Reaction Optimization, Catalytic and Antibacterial Activities. Environ. Monit. Assess. 189 (7), 1–15. doi:10.1007/s10661-017-6033-0
Adelere, I. A., and Lateef, A. (2016). A Novel Approach to the green Synthesis of Metallic Nanoparticles: the Use of Agro-Wastes, Enzymes, and Pigments. Nanotechnology Rev. 5 (6), 567–587. doi:10.1515/ntrev-2016-0024
Agarwal, H., Venkat Kumar, S., and Rajeshkumar, S. (2017). A Review on green Synthesis of Zinc Oxide Nanoparticles - an Eco-Friendly Approach. Resource-Efficient Tech. 3 (4), 406–413. doi:10.1016/j.reffit.2017.03.002
Al-Zaban, M. I., Mahmoud, M. A., and AlHarbi, M. A. (2021). Catalytic Degradation of Methylene Blue Using Silver Nanoparticles Synthesized by Honey. Saudi J. Biol. Sci. 28 (3), 2007–2013. doi:10.1016/j.sjbs.2021.01.003
Andleeb, A., Andleeb, A., Asghar, S., Zaman, G., Tariq, M., Mehmood, A., et al. (2021). A Systematic Review of Biosynthesized Metallic Nanoparticles as a Promising Anti-cancer-strategy. Cancers 13 (11), 2818. doi:10.3390/cancers13112818
Araya-Castro, K., Chao, T. C., Durán-Vinet, B., Cisternas, C., and Rubilar, O. (2021). Green Synthesis of Copper Oxide Nanoparticles Using Protein Fractions from an Aqueous Extract of Brown Algae Macrocystis Pyrifera. Processes 9 (1), 78. doi:10.3390/pr9010078
Báez-Cruz, R., Baptista, L. A., Ntim, S., Manidurai, P., Espinoza, S., Ramanan, C., et al. (2021). Role of pH in the Synthesis and Growth of Gold Nanoparticles Using L-Asparagine: a Combined Experimental and Simulation Study. J. Phys. Condensed Matter 33 (25), 254005. doi:10.1088/1361-648X/abf6e3
Basavaraja, S., Balaji, S. D., Lagashetty, A., Rajasab, A. H., and Venkataraman, A. (2008). Extracellular Biosynthesis of Silver Nanoparticles Using the Fungus Fusarium Semitectum. Mater. Res. Bull. 43 (5), 1164–1170. doi:10.1016/j.materresbull.2007.06.020
Basnet, P., Samanta, D., Chanu, T. I., Jha, S., and Chatterjee, S. (2020). Glycine-A Bio-Capping Agent for the Bioinspired Synthesis of Nano-Zinc Oxide Photocatalyst. J. Mater. Sci. Mater. Elect. 31 (4), 2949–2966. doi:10.1007/s10854-019-02839-z
Bastami, T. R., and Entezari, M. H. (2010). Sono-synthesis of Mn3O4 Nanoparticles in Different media without Additives. Chem. Eng. J. 164 (1), 261–266. doi:10.1016/j.cej.2010.08.030
Batool, M., Khurshid, S., Qureshi, Z., and Daoush, W. M. (2021). Adsorption, Antimicrobial and Wound Healing Activities of Biosynthesised Zinc Oxide Nanoparticles. Chem. Pap. 75 (3), 893–907. doi:10.1007/s11696-020-01343-7
Bolaños, K., Kogan, M. J., and Araya, E. (2019). Capping Gold Nanoparticles with Albumin to Improve Their Biomedical Properties. Int. J. nanomedicine 14, 6387. doi:10.2147/IJN.S210992
Burdușel, A. C., Gherasim, O., Grumezescu, A. M., Mogoantă, L., Ficai, A., and Andronescu, E. (2018). Biomedical Applications of Silver Nanoparticles: an Up-To-Date Overview. Nanomaterials 8 (9), 681. doi:10.3390/nano8090681
Cahyana, A. H., and Reza, A. I. (2018).Synthesis and Characterization of Fe3O4 Nanoparticles Dispersed in Paraffin as Solvent. In AIP Conference Proceedings, 2049. Depok, Indonesia: AIP Publishing LLC, 020047. doi:10.1063/1.5082452
Campisi, S., Schiavoni, M., Chan-Thaw, C. E., and Villa, A. (2016). Untangling the Role of the Capping Agent in Nanocatalysis: Recent Advances and Perspectives. Catalysts 6 (12), 185. doi:10.3390/catal6120185
Carrasco-Esteban, E., Domínguez-Rullán, J. A., Barrionuevo-Castillo, P., Pelari-Mici, L., Leaman, O., Sastre-Gallego, S., et al. (2021). Current Role of Nanoparticles in the Treatment of Lung Cancer. J. Clin. Translational Res. 7 (2), 140. doi:10.18053/jctres.07.202102.005
Caschera, D., Toro, R. G., Federici, F., Montanari, R., de Caro, T., Al-Shemy, M. T., et al. (2020). Green Approach for the Fabrication of Silver-Oxidized Cellulose Nanocomposite with Antibacterial Properties. Cellulose 27 (14), 8059–8073. doi:10.1007/s10570-020-03364-7
Chaudhary, R., Nawaz, K., Khan, A. K., Hano, C., Abbasi, B. H., and Anjum, S. (2020). An Overview of the Algae-Mediated Biosynthesis of Nanoparticles and Their Biomedical Applications. Biomolecules 10 (11), 1498. doi:10.3390/biom10111498
Choi, J. S., Lee, D. H., Ahn, J. B., Sim, S., Heo, K. S., Myung, C. S., et al. (2020). Therapeutic Effects of Celecoxib Polymeric Systems in Rat Models of Inflammation and Adjuvant-Induced Rheumatoid Arthritis. Mater. Sci. Eng. C 114, 111042. doi:10.1016/j.msec.2020.111042
Chowdhury, S., Basu, A., and Kundu, S. (2014). Green Synthesis of Protein Capped Silver Nanoparticles from Phytopathogenic Fungus Macrophomina Phaseolina (Tassi) Goid with Antimicrobial Properties against Multidrug-Resistant Bacteria. Nanoscale Res. Lett. 9 (1), 1–11. doi:10.1186/1556-276X-9-365
Chugh, D., Viswamalya, V. S., and Das, B. (2021). Green Synthesis of Silver Nanoparticles with Algae and the Importance of Capping Agents in the Process. J. Genet. Eng. Biotechnol. 19 (1), 1–21. doi:10.1186/s43141-021-00228-w
Costa, I. D., Wanderley Neto, A. D. O., Da Silva, H. F. O., Moraes, E. P., Damascena Nóbrega, E. T., Sant’Anna, C., et al. (2017). Dual Role of a Ricinoleic Acid Derivative in the Aqueous Synthesis of Silver Nanoparticles. J. Nanomater. doi:10.1155/2017/1230467
Craciunescu, O., Seciu, A. M., Manoiu, V. S., Trif, M., Moisei, M., Nicu, A. I., et al. (2019). Biosynthesis of Silver Nanoparticles in Collagen Gel Improves Their Medical Use in Periodontitis Treatment. Particulate Sci. Tech. 37 (6), 757–763. doi:10.1080/02726351.2018.1455780
Crivelli, B., Bari, E., Perteghella, S., Catenacci, L., Sorrenti, M., Mocchi, M., et al. (2019). Silk Fibroin Nanoparticles for Celecoxib and Curcumin Delivery: ROS-Scavenging and Anti-inflammatory Activities in an In Vitro Model of Osteoarthritis. Eur. J. Pharmaceutics Biopharmaceutics 137, 37–45. doi:10.1016/j.ejpb.2019.02.008
Cuevas, R., Durán, N., Diez, M. C., Tortella, G. R., and Rubilar, O. (2015). Extracellular Biosynthesis of Copper and Copper Oxide Nanoparticles by Stereum Hirsutum, a Native white-rot Fungus from Chilean Forests. J. Nanomater. doi:10.1155/2015/789089
Das, S. K., Das, A. R., and Guha, A. K. (2009). Gold Nanoparticles: Microbial Synthesis and Application in Water hygiene Management. Langmuir 25 (14), 8192–8199. doi:10.1021/la900585p
Din, M. I., Rani, A., Hussain, Z., Khalid, R., Aihetasham, A., and Mukhtar, M. (2021). Biofabrication of Size-Controlled ZnO Nanoparticles Using Various Capping Agents and Their Cytotoxic and Antitermite Activity. Int. J. Environ. Anal. Chem. 101 (6), 821–837. doi:10.1080/03067319.2019.1672671
Durán, N., Marcato, P. D., Durán, M., Yadav, A., Gade, A., and Rai, M. (2011). Mechanistic Aspects in the Biogenic Synthesis of Extracellular Metal Nanoparticles by Peptides, Bacteria, Fungi, and Plants. Appl. Microbiol. Biotechnol. 90 (5), 1609–1624. doi:10.1007/s00253-011-3249-8
Elgorban, A. M., Aref, S. M., Seham, S. M., Elhindi, K. M., Bahkali, A. H., Sayed, S. R., et al. (2016). Extracellular Synthesis of Silver Nanoparticles Using Aspergillus versicolor and Evaluation of Their Activity on Plant Pathogenic Fungi. Mycosphere 7 (6), 844–852. doi:10.5943/mycosphere/7/6/15
Elsupikhe, R. F., Alfazani, T. S., Al-Jazwia, F. M., Abo-Eisa, H., Hammad, I., and Sheltami, R. M. (2020). Albumin/Ag Nanoparticles Synthesized by Using UV-Irradiation and Estimation of Their Antibacterial Activity. Int. Res. J. Pure Appl. Chem., 36–45. doi:10.9734/irjpac/2020/v21i130148
Fabris, M., Abbriano, R. M., Pernice, M., Sutherland, D. L., Commault, A. S., Hall, C. C., et al. (2020). Emerging Technologies in Algal Biotechnology: Toward the Establishment of a Sustainable, Algae-Based Bioeconomy. Front. Plant Sci. 11, 279. doi:10.3389/fpls.2020.00279
Fahy, E., Cotter, D., Sud, M., and Subramaniam, S. (2011). Lipid Classification, Structures and Tools. Biochim. Biophys. Acta (BBA)-Molecular Cel Biol. Lipids 1811 (11), 637–647. doi:10.1016/j.bbalip.2011.06.009
Fatima, R., Priya, M., Indurthi, L., Radhakrishnan, V., and Sudhakaran, R. (2020). Biosynthesis of Silver Nanoparticles Using Red Algae Portieria Hornemannii and its Antibacterial Activity against Fish Pathogens. Microb. pathogenesis 138, 103780. doi:10.1016/j.micpath.2019.103780
Gahlawat, G., and Choudhury, A. R. (2019). A Review on the Biosynthesis of Metal and Metal Salt Nanoparticles by Microbes. RSC Adv. 9 (23), 12944–12967. doi:10.1039/C8RA10483B
Gai, X., Jiang, Z., Liu, M., Li, Q., Wang, S., Li, T., et al. (2018). Therapeutic Effect of a Novel Nano-Drug Delivery System on Membranous Glomerulonephritis Rat Model Induced by Cationic Bovine Serum. AAPS PharmSciTech 19 (5), 2195–2202. doi:10.1208/s12249-018-1034-z
Garza-Cervantes, J. A., Mendiola-Garza, G., de Melo, E. M., Dugmore, T. I., Matharu, A. S., and Morones-Ramirez, J. R. (2020). Antimicrobial Activity of a Silver-Microfibrillated Cellulose Biocomposite against Susceptible and Resistant Bacteria. Scientific Rep. 10 (1), 1–7. doi:10.1038/s41598-020-64127-9
Ghiyasiyan-Arani, M., Salavati-Niasari, M., Masjedi-Arani, M., and Mazloom, F. (2018). An Easy Sonochemical Route for Synthesis, Characterization and Photocatalytic Performance of Nanosized FeVO 4 in the Presence of Aminoacids as green Capping Agents. J. Mater. Sci. Mater. Elect. 29 (1), 474–485. doi:10.1007/s10854-017-7936-9
Gole, A., Dash, C., Ramakrishnan, V., Sainkar, S. R., Mandale, A. B., Rao, M., et al. (2001). Pepsin− Gold Colloid Conjugates: Preparation, Characterization, and Enzymatic Activity. Langmuir 17 (5), 1674–1679. doi:10.1021/la001164w
Gudikandula, K., Vadapally, P., and Charya, M. S. (2017). Biogenic Synthesis of Silver Nanoparticles from white Rot Fungi: Their Characterization and Antibacterial Studies. OpenNano 2, 64–78. doi:10.1016/j.onano.2017.07.002
Guilger-Casagrande, M., and Lima, R. D. (2019). Synthesis of Silver Nanoparticles Mediated by Fungi: a Review. Front. Bioeng. Biotechnol. 7, 287. doi:10.3389/fbioe.2019.00287
Gurunathan, S., Kalishwaralal, K., Vaidyanathan, R., Venkataraman, D., Pandian, S. R. K., Muniyandi, J., et al. (2009). Biosynthesis, Purification and Characterization of Silver Nanoparticles Using Escherichia coli. Colloids Surf. B: Biointerfaces 74 (1), 328–335. doi:10.1016/j.colsurfb.2009.07.048
Hameed, M. K., Ahmady, I. M., Han, C., and Mohamed, A. A. (2020). Efficient Synthesis of Amino Acids Capped Gold Nanoparticles from Easily Reducible Aryldiazonium Tetrachloroaurate (III) Salts for Cellular Uptake Study. Amino Acids 52 (6), 941–953. doi:10.1007/s00726-020-02862-z
Husseiny, S. M., Salah, T. A., and Anter, H. A. (2015). Biosynthesis of Size Controlled Silver Nanoparticles by Fusarium Oxysporum, Their Antibacterial and Antitumor Activities. Beni-Suef Univ. J. Basic Appl. Sci. 4 (3), 225–231. doi:10.1016/j.bjbas.2015.07.004
Iravani, S. (2014). Bacteria in Nanoparticle Synthesis: Current Status and Future Prospects. Int. scholarly Res. notices. doi:10.1155/2014/359316
Jadoun, S., Arif, R., Jangid, N. K., and Meena, R. K. (2021). Green Synthesis of Nanoparticles Using Plant Extracts: A Review. Environ. Chem. Lett. 19 (1), 355–374. doi:10.1007/s10311-020-01074-x
Javed, R., Zia, M., Naz, S., Aisida, S. O., ul Ain, N., and Ao, Q. (2020). Role of Capping Agents in the Application of Nanoparticles in Biomedicine and Environmental Remediation: Recent Trends and Future Prospects. J. Nanobiotechnology 18 (1), 1–15. doi:10.1186/s12951-020-00704-4
Joanna, C., Marcin, L., Ewa, K., and Grażyna, P. (2018). A Nonspecific Synergistic Effect of Biogenic Silver Nanoparticles and Biosurfactant towards Environmental Bacteria and Fungi. Ecotoxicology 27 (3), 352–359. doi:10.1007/s10646-018-1899-3
Kadam, V. V., Ettiyappan, J. P., and Balakrishnan, R. M. (2019). Mechanistic Insight into the Endophytic Fungus Mediated Synthesis of Protein Capped ZnO Nanoparticles. Mater. Sci. Eng. B 243, 214–221. doi:10.1016/j.mseb.2019.04.017
Kambale, E. K., Nkanga, C. I., Mutonkole, B. P. I., Bapolisi, A. M., Tassa, D. O., Liesse, J. M. I., et al. (2020). Green Synthesis of Antimicrobial Silver Nanoparticles Using Aqueous Leaf Extracts from Three Congolese Plant Species (Brillantaisia Patula, Crossopteryx Febrifuga and Senna Siamea). Heliyon 6 (8), e04493. doi:10.1016/j.heliyon.2020.e04493
Khan, A. U., Khan, M., Malik, N., Cho, M. H., and Khan, M. M. (2019). Recent Progress of Algae and Blue–green Algae-Assisted Synthesis of Gold Nanoparticles for Various Applications. Bioproc. Biosyst. Eng. 42 (1), 1–15. doi:10.1007/s00449-018-2012-2
Khan, M. I., Shin, J. H., and Kim, J. D. (2018). The Promising Future of Microalgae: Current Status, Challenges, and Optimization of a Sustainable and Renewable Industry for Biofuels, Feed, and Other Products. Microb. Cel. factories 17 (1), 1–21. doi:10.1186/s12934-018-0879-x
Khodashenas, B., Ardjmand, M., Sharifzadeh Baei, M., Shokuhi Rad, A., and Akbarzadeh Khiyavi, A. (2020). Bovine Serum Albumin/gold Nanoparticles as a Drug Delivery System for Curcumin: Experimental and Computational Studies. J. Biomol. Struct. Dyn. 38 (15), 4644–4654. doi:10.1080/07391102.2019.1683073
Kiran, G. S., Sabu, A., and Selvin, J. (2010). Synthesis of Silver Nanoparticles by Glycolipid Biosurfactant Produced from marine Brevibacterium Casei MSA19. J. Biotechnol. 148 (4), 221–225. doi:10.1016/j.jbiotec.2010.06.012
Koltypin, Y., Perkas, N., and Gedanken, A. (2004). Commercial Edible Oils as New Solvents for Ultrasonic Synthesis of Nanoparticles: the Preparation of Air Stable Nanocrystalline Iron Particles. J. Mater. Chem. 14 (20), 2975–2977. doi:10.1039/B411983E
Kravanja, G., Primožič, M., Knez, Ž., and Leitgeb, M. (2019). Chitosan-based (Nano) Materials for Novel Biomedical Applications. Molecules 24 (10), 1960. doi:10.3390/molecules24101960
Kumar, A., Das, N., Satija, N. K., Mandrah, K., Roy, S. K., and Rayavarapu, R. G. (2020). A Novel Approach towards Synthesis and Characterization of Non-cytotoxic Gold Nanoparticles Using Taurine as Capping Agent. Nanomaterials 10 (1), 45. doi:10.3390/nano10010045
Kumar, C. G., Mamidyala, S. K., Das, B., Sridhar, B., Devi, G. S., and Karuna, M. S. (2010). Synthesis of Biosurfactant-Based Silver Nanoparticles with Purified Rhamnolipids Isolated from Pseudomonas aeruginosa BS-161R. J. Microbiol. Biotechnol. 20 (7), 1061–1068. doi:10.4014/jmb.1001.01018
Kumari, M. M., and Philip, D. (2013). Facile One-Pot Synthesis of Gold and Silver Nanocatalysts Using Edible Coconut Oil. Spectrochimica Acta A: Mol. Biomol. Spectrosc. 111, 154–160. doi:10.1016/j.saa.2013.03.076
Lee, S. H., and Jun, B. H. (2019). Silver Nanoparticles: Synthesis and Application for Nanomedicine. Int. J. Mol. Sci. 20 (4), 865. doi:10.3390/ijms20040865
Li, C. C., Chang, S. J., Su, F. J., Lin, S. W., and Chou, Y. C. (2013). Effects of Capping Agents on the Dispersion of Silver Nanoparticles. Colloids Surf. A: Physicochemical Eng. Aspects 419, 209–215. doi:10.1016/j.colsurfa.2012.11.077
Lu, Y., Lu, X., Mayers, B. T., Herricks, T., and Xia, Y. (2008). Synthesis and Characterization of Magnetic Co Nanoparticles: a Comparison Study of Three Different Capping Surfactants. J. Solid State. Chem. 181 (7), 1530–1538. doi:10.1016/j.jssc.2008.02.016
Luchini, A., and Vitiello, G. (2019). Understanding the Nano-Bio Interfaces: Lipid-Coatings for Inorganic Nanoparticles as Promising Strategy for Biomedical Applications. Front. Chem. 7, 343. doi:10.3389/fchem.2019.00343
Magro, M., Baratella, D., Bonaiuto, E., de A Roger, J., and Vianello, F. (2018). New Perspectives on Biomedical Applications of Iron Oxide Nanoparticles. Curr. Med. Chem. 25 (4), 540–555. doi:10.2174/0929867324666170616102922
Maiti, P. K., Ghosh, A., Parveen, R., Saha, A., and Choudhury, M. G. (2019). Preparation of Carboxy-Methyl Cellulose-Capped Nanosilver Particles and Their Antimicrobial Evaluation by an Automated Device. Appl. Nanoscience 9 (1), 105–111. doi:10.1007/s13204-018-0914-6
Majeed, S., Aripin, F. H. B., Shoeb, N. S. B., Danish, M., Ibrahim, M. M., and Hashim, R. (2019). Bioengineered Silver Nanoparticles Capped with Bovine Serum Albumin and its Anticancer and Apoptotic Activity against Breast, Bone and Intestinal colon Cancer Cell Lines. Mater. Sci. Eng. C 102, 254–263. doi:10.1016/j.msec.2019.04.041
Marișca, O. T., and Leopold, N. (2019). Anisotropic Gold Nanoparticle-Cell Interactions Mediated by Collagen. Materials 12 (7), 1131. doi:10.3390/ma12071131
Maruyama, T., Fujimoto, Y., and Maekawa, T. (2015). Synthesis of Gold Nanoparticles Using Various Amino Acids. J. Colloid Interf. Sci. 447, 254–257. doi:10.1016/j.jcis.2014.12.046
McNamara, K., and Tofail, S. A. (2017). Nanoparticles in Biomedical Applications. Adv. Phys. X 2 (1), 54–88. doi:10.1080/23746149.2016.1254570
Mensah, M. B., Awudza, J. A. M., and O'Brien, P. (2018). Castor Oil: a Suitable green Source of Capping Agent for Nanoparticle Syntheses and Facile Surface Functionalization. R. Soc. open Sci. 5 (8), 180824. doi:10.1098/rsos.180824
Miao, Z., Gao, Z., Chen, R., Yu, X., Su, Z., and Wei, G. (2018). Surface-bioengineered Gold Nanoparticles for Biomedical Applications. Curr. Med. Chem. 25 (16), 1920–1944. doi:10.2174/0929867325666180117111404
Mirahadi, M., Ghanbarzadeh, S., Ghorbani, M., Gholizadeh, A., and Hamishehkar, H. (2018). A Review on the Role of Lipid-Based Nanoparticles in Medical Diagnosis and Imaging. Ther. Deliv. 9 (8), 557–569. doi:10.4155/tde-2018-0020
Municoy, S., Antezana, P. E., Pérez, C. J., Bellino, M. G., and Desimone, M. F. (2021). Tuning the Antimicrobial Activity of Collagen Biomaterials through a Liposomal Approach. J. Appl. Polym. Sci. 138 (18), 50330. doi:10.1002/app.50330
Namvar, F., Azizi, S., Ahmad, M. B., Shameli, K., Mohamad, R., Mahdavi, M., et al. (2015). Green Synthesis and Characterization of Gold Nanoparticles Using the marine Macroalgae Sargassum Muticum. Res. Chem. Intermediates 41 (8), 5723–5730. doi:10.1007/s11164-014-1696-4
Nayak, S., Bhat, M. P., Udayashankar, A. C., Lakshmeesha, T. R., Geetha, N., and Jogaiah, S. (2020). Biosynthesis and Characterization of Dillenia Indica-Mediated Silver Nanoparticles and Their Biological Activity. Appl. Organomet. Chem. 34 (4), e5567. doi:10.1002/aoc.5567
Nedelcu, A., Mosteanu, O., Pop, T., Mocan, T., and Mocan, L. (2021). Recent Advances in Nanoparticle-Mediated Treatment of Inflammatory Bowel Diseases. Appl. Sci. 11 (1), 438. doi:10.3390/app11010438
Negi, S., and Singh, V. (2018). Algae: A Potential Source for Nanoparticle Synthesis. J. Appl. Nat. Sci. 10 (4), 1134–1140. doi:10.31018/jans.v10i4.1878
Nghiem, T. H. L., La, T. H., Vu, X. H., Chu, V. H., Nguyen, T. H., Le, Q. H., et al. (2010). Synthesis, Capping and Binding of Colloidal Gold Nanoparticles to Proteins. Adv. Nat. Sci. nanoscience nanotechnology 1 (2), 025009. doi:10.1088/2043-6254/1/2/025009
Nguyen, N. T. P., Nguyen, L. V. H., Thanh, N. T., Van Toi, V., Quyen, T. N., Tran, P. A., et al. (2019). Stabilization of Silver Nanoparticles in Chitosan and Gelatin Hydrogel and its Applications. Mater. Lett. 248, 241–245. doi:10.1016/j.matlet.2019.03.103
Nikzamir, M., Akbarzadeh, A., and Panahi, Y. (2021). An Overview on Nanoparticles Used in Biomedicine and Their Cytotoxicity. J. Drug Deliv. Sci. Tech. 61, 102316. doi:10.1016/j.jddst.2020.102316
Ninganagouda, S., Rathod, V., Jyoti, H., Singh, D., Prema, K., and Haq, M. U. (2013). Extracellular Biosynthesis of Silver Nanoparticles Using Aspergillus flavus and Their Antimicrobial Activity against Gram Negative MDR Strains. Int. J. Pharma Bio Sci. 4 (2), 222–229.
Niu, Z., and Li, Y. (2014). Removal and Utilization of Capping Agents in Nanocatalysis. Chem. Mater. 26 (1), 72–83. doi:10.1021/cm4022479
Nogueira, S. S., de Araujo-Nobre, A. R., Mafud, A. C., Guimarães, M. A., Alves, M. M. M., Plácido, A., et al. (2019). Silver Nanoparticle Stabilized by Hydrolyzed Collagen and Natural Polymers: Synthesis, Characterization and Antibacterial-Antifungal Evaluation. Int. J. Biol. macromolecules 135, 808–814. doi:10.1016/j.ijbiomac.2019.05.214
Nyamen, L. D., Revaprasadu, N., and Ndifon, P. T. (2014). Low Temperature Synthesis of PbS and CdS Nanoparticles in Olive Oil. Mater. Sci. semiconductor Process. 27, 191–196. doi:10.1016/j.mssp.2014.06.010
Ocsoy, I., Tasdemir, D., Mazicioglu, S., Celik, C., Katı, A., and Ulgen, F. (2018). Biomolecules Incorporated Metallic Nanoparticles Synthesis and Their Biomedical Applications. Mater. Lett. 212, 45–50. doi:10.1016/j.matlet.2017.10.068
Ogundare, S. A., and van Zyl, W. E. (2018). Nanocrystalline Cellulose as Reducing-And Stabilizing Agent in the Synthesis of Silver Nanoparticles: Application as a Surface-Enhanced Raman Scattering (SERS) Substrate. Surf. Inter. 13, 1–10. doi:10.1016/j.surÞn.2018.06.00410.1016/j.surfin.2018.06.004
Oluwalana, A. E., and Ajibade, P. A. (2019). Effect of Temperature and Capping Agents on Structural and Optical Properties of Tin Sulphide Nanocrystals. J. Nanotechnology. doi:10.1155/2019/8235816
Ovais, M., Ahmad, I., Khalil, A. T., Mukherjee, S., Javed, R., Ayaz, M., et al. (2018). Wound Healing Applications of Biogenic Colloidal Silver and Gold Nanoparticles: Recent Trends and Future Prospects. Appl. Microbiol. Biotechnol. 102 (10), 4305–4318. doi:10.1007/s00253-018-8939-z
Oyinloye, B. E., Adenowo, A. F., and Kappo, A. P. (2015). Reactive Oxygen Species, Apoptosis, Antimicrobial Peptides and Human Inflammatory Diseases. Pharmaceuticals 8 (2), 151–175. doi:10.3390/ph8020151
Parial, D., Patra, H. K., Roychoudhury, P., Dasgupta, A. K., and Pal, R. (2012). Gold Nanorod Production by Cyanobacteria—A green Chemistry Approach. J. Appl. phycology 24 (1), 55–60. doi:10.1007/s10811-010-9645-0
Pereira, A. S., Silva, N. J., Trindade, T., and Pereira, S. (2012). A Single-Source Route for the Synthesis of Metal Oxide Nanoparticles Using Vegetable Oil Solvents. J. nanoscience nanotechnology 12 (12), 8963–8968. doi:10.1166/jnn.2012.6700
Placha, D., and Jampilek, J. (2021). Chronic Inflammatory Diseases, Anti-inflammatory Agents and Their Delivery Nanosystems. Pharmaceutics 13 (1), 64. doi:10.3390/pharmaceutics13010064
Płaza, G. A., Chojniak, J., and Banat, I. M. (2014). Biosurfactant Mediated Biosynthesis of Selected Metallic Nanoparticles. Int. J. Mol. Sci. 15 (8), 13720–13737. doi:10.3390/ijms150813720
Pradeepa, V., Sujatha, M., and Sriram, T. (2017). Anti Proliferative Effect of Silver Nanoparticles Synthesized Using Human Serum Albumin on Mcf-7 Cell Line. Int. J. Pharma Bio Sci. 8 (2), 126–133. doi:10.22376/ijpbs.2017.8.2.b126
Priya, N., Kaur, K., and Sidhu, A. K. (2021). Green Synthesis: An Eco-Friendly Route for the Synthesis of Iron Oxide Nanoparticles. Front. Nanotechnology 3, 47. doi:10.3389/fnano.2021.655062
Puja, P., and Kumar, P. (2019). A Perspective on Biogenic Synthesis of Platinum Nanoparticles and Their Biomedical Applications. Spectrochimica Acta Part A: Mol. Biomol. Spectrosc. 211, 94–99. doi:10.1016/j.saa.2018.11.047
Qu, L., and Peng, X. (2002). Control of Photoluminescence Properties of CdSe Nanocrystals in Growth. J. Am. Chem. Soc. 124 (9), 2049–2055. doi:10.1021/ja017002j
Radzig, M. A., Nadtochenko, V. A., Koksharova, O. A., Kiwi, J., Lipasova, V. A., and Khmel, I. A. (2013). Antibacterial Effects of Silver Nanoparticles on Gram-Negative Bacteria: Influence on the Growth and Biofilms Formation, Mechanisms of Action. Colloids Surf. B: Biointerfaces 102, 300–306. doi:10.1016/j.colsurfb.2012.07.039
Rahimi, R., Pordel, S., and Rabbani, M. (2016). Synthesis of Bi 2 WO 6 Nanoplates Using Oleic Acid as a green Capping Agent and its Application for Thiols Oxidation. J. Nanostructure Chem. 6 (2), 191–196. doi:10.1007/s40097-016-0195-0
Ramos Campos, E. V., Proença, P. L. D. F., Doretto-Silva, L., Andrade-Oliveira, V., Fraceto, L. F., and de Araujo, D. R. (2020). Trends in Nanoformulations for Atopic Dermatitis Treatment. Expert Opin. Drug Deliv. 17 (11), 1615–1630. doi:10.1080/17425247.2020.1813107
Rather, R. A., Sarwara, R. K., Das, N., and Pal, B. (2019). Impact of Reducing and Capping Agents on Carbohydrates for the Growth of Ag and Cu Nanostructures and Their Antibacterial Activities. Particuology 43, 219–226. doi:10.1016/j.partic.2018.01.004
Reddy, A. S., Chen, C. Y., Baker, S. C., Chen, C. C., Jean, J. S., Fan, C. W., et al. (2009). Synthesis of Silver Nanoparticles Using Surfactin: A Biosurfactant as Stabilizing Agent. Mater. Lett. 63 (15), 1227–1230. doi:10.1016/j.matlet.2009.02.028
Restrepo, C. V., and Villa, C. C. (2021). Synthesis of Silver Nanoparticles, Influence of Capping Agents, and Dependence on Size and Shape: A Review. Quindío, Colombia: Environmental Nanotechnology, Monitoring & Management. doi:10.1016/j.enmm.2021.100428
Roy, A. D., Varghese, K. K., Ghosh, S., Paul, P., and Mitra, I. (2021). Metallic Nanoparticles from Natural Products as a Potential Anti-cancer. Int. J. Pharm. Sci. Rev. Res. 67 (1), 171–177. doi:10.47583/ijpsrr.2021.v67i01.028
Sadrolhosseini, A. R., Abdul Rashid, S., and Zakaria, A. (2017). Synthesis of Gold Nanoparticles Dispersed in palm Oil Using Laser Ablation Technique. J. Nanomater. doi:10.1155/2017/6496390
Sahayaraj, K., Balasubramanyam, G., and Chavali, M. (2020). Green Synthesis of Silver Nanoparticles Using Dry Leaf Aqueous Extract of Pongamia Glabra Vent (Fab.), Characterization and Phytofungicidal Activity. Environ. Nanotechnology, Monit. Manag. 14, 100349. doi:10.1016/j.enmm.2020.100349
Salazar-Bryam, A. M., Yoshimura, I., Santos, L. P., Moura, C. C., Santos, C. C., Silva, V. L., et al. (2021). Silver Nanoparticles Stabilized by Ramnolipids: Effect of pH. Colloids Surf. B: Biointerfaces 205, 111883. doi:10.1016/j.colsurfb.2021.111883
Salem, S. S., and Fouda, A. (2021). Green Synthesis of Metallic Nanoparticles and Their Prospective Biotechnological Applications: an Overview. Biol. trace Elem. Res. 199 (1), 344–370. doi:10.1007/s12011-020-02138-3
Shaheen, I., and Ahmad, K. S. (2020). Chromatographic Identification of “green Capping Agents” Extracted from Nasturtium Officinale (Brassicaceae) Leaves for the Synthesis of MoO3 Nanoparticles. J. Separat. Sci. 43 (3), 598–605. doi:10.1002/jssc.201900840
Shanmuganathan, R., Edison, T. N. J. I., LewisOscar, F., Kumar, P., Shanmugam, S., and Pugazhendhi, A. (2019). Chitosan Nanopolymers: an Overview of Drug Delivery against Cancer. Int. J. Biol. macromolecules 130, 727–736. doi:10.1016/j.ijbiomac.2019.02.060
Sharma, D., Kanchi, S., and Bisetty, K. (2019). Biogenic Synthesis of Nanoparticles: a Review. Arabian J. Chem. 12 (8), 3576–3600. doi:10.1016/j.arabjc.2015.11.002
Sharma, N., and Sharma, P. (2017). Industrial and Biotechnological Applications of Algae: A Review. J. Adv. Plant Biol. 1 (1), 1–25. doi:10.14302/issn.2638-4469.japb-17-1534
Sharma, P., Kumari, S., Ghosh, D., Yadav, V., Vij, A., Rawat, P., et al. (2021). Capping Agent-Induced Variation of Physicochemical and Biological Properties of α-Fe2O3 Nanoparticles. Mater. Chem. Phys. 258, 123899. doi:10.1016/j.matchemphys.2020.123899
Shivaramakrishnan, B., Gurumurthy, B., and Balasubramanian, A. (2017). Potential Biomedical Applications of Metallic Nanobiomaterials: a Review. Int. J. Pharm. Sci. Res. 8 (3), 985. doi:10.13040/IJPSR.0975-8232.8(3).985-00
Shu, M., He, F., Li, Z., Zhu, X., Ma, Y., Zhou, Z., et al. (2020). Biosynthesis and Antibacterial Activity of Silver Nanoparticles Using Yeast Extract as Reducing and Capping Agents. Nanoscale Res. Lett. 15 (1), 1–9. doi:10.1186/s11671-019-3244-z
Silva, F., Cabral Campello, M. P., and Paulo, A. (2021). Radiolabeled Gold Nanoparticles for Imaging and Therapy of Cancer. Materials 14 (1), 4. doi:10.3390/ma14010004
Simons, K. (2016). Cell Membranes: A Subjective Perspective. Biochim. Biophys. Acta (BBA)-Biomembranes 1858 (10), 2569–2572. doi:10.1016/j.bbamem.2016.01.023
Singh, A., Gautam, P. K., Verma, A., Singh, V., Shivapriya, P. M., Shivalkar, S., et al. (2020). Green Synthesis of Metallic Nanoparticles as Effective Alternatives to Treat Antibiotics Resistant Bacterial Infections: A Review. Biotechnol. Rep. 25, e00427. doi:10.1016/j.btre.2020.e00427
Singh, P., Garg, A., Pandit, S., Mokkapati, V. R. S. S., and Mijakovic, I. (2018). Antimicrobial Effects of Biogenic Nanoparticles. Nanomaterials 8 (12), 1009. doi:10.3390/nano8121009
Singh, R., Shedbalkar, U. U., Wadhwani, S. A., and Chopade, B. A. (2015). Bacteriagenic Silver Nanoparticles: Synthesis, Mechanism, and Applications. Appl. Microbiol. Biotechnol. 99 (11), 4579–4593. doi:10.1007/s00253-015-6622-1
Sinha, S. N., Paul, D., Halder, N., Sengupta, D., and Patra, S. K. (2015). Green Synthesis of Silver Nanoparticles Using Fresh Water green Alga Pithophora Oedogonia (Mont.) Wittrock and Evaluation of Their Antibacterial Activity. Appl. Nanoscience 5 (6), 703–709. doi:10.1007/s13204-014-0366-6
Sivakumar, T. (2021). A Modern Review of Silver Nanoparticles Mediated Plant Extracts and its Potential Bioapplications. Int. J. Bot. Stud. 6 (3), 170–175.
Tadele, K. T., Abire, T. O., and Feyisa, T. Y. (2021). Green Synthesized Silver Nanoparticles Using Plant Extracts as Promising Prospect for Cancer Therapy: A Review of Recent Findings. J. Nanomed 4, 1040.
Ting, A. S. Y., and Chin, J. E. (2020). Biogenic Synthesis of Iron Nanoparticles from Apple Peel Extracts for Decolorization of Malachite green Dye. Water Air Soil Pollut. 231, 1–10. doi:10.1007/s11270-020-04658-z
Tsekhmistrenko, S. I., Bityutskyy, V. S., Tsekhmistrenko, O. S., Horalskyi, L. P., Tymoshok, N. O., and Spivak, M. Y. (2020). Bacterial Synthesis of Nanoparticles: A green Approach. Biosyst. Divers. 28 (1), 9–17. doi:10.15421/012002
Verma, N., and Kumar, N. (2019). Synthesis and Biomedical Applications of Copper Oxide Nanoparticles: an Expanding Horizon. ACS Biomater. Sci. Eng. 5 (3), 1170–1188. doi:10.1021/acsbiomaterials.8b01092
Vijayakumar, S., and Vaseeharan, B. (2018). Antibiofilm, Anti Cancer and Ecotoxicity Properties of Collagen Based ZnO Nanoparticles. Adv. Powder Tech. 29 (10), 2331–2345. doi:10.1016/j.apt.2018.06.013
Wang, C., Gao, X., Chen, Z., Chen, Y., and Chen, H. (2017). Preparation, Characterization and Application of Polysaccharide-Based Metallic Nanoparticles: a Review. Polymers 9 (12), 689. doi:10.3390/polym9120689
Wang, J., Zhao, J., and Ma, G. (2019). Extremely Concentrated Silver Nanoparticles Stabilized in Aqueous Solution by Bovine Serum Albumin (BSA). Nano-Structures & Nano-Objects 19, 100349. doi:10.1016/j.nanoso.2019.100349
Xie, J., Lee, J. Y., Wang, D. I., and Ting, Y. P. (2007). Identification of Active Biomolecules in the High-Yield Synthesis of Single-Crystalline Gold Nanoplates in Algal Solutions. small 3 (4), 672–682. doi:10.1002/smll.200600612
Xu, C., Qiao, L., Guo, Y., Ma, L., and Cheng, Y. (2018). Preparation, Characteristics and Antioxidant Activity of Polysaccharides and Proteins-Capped Selenium Nanoparticles Synthesized by Lactobacillus Casei ATCC 393. Carbohydr. Polym. 195, 576–585. doi:10.1016/j.carbpol.2018.04.110
Yulizar, Y., Kusrini, E., Apriandanu, D. O. B., and Nurdini, N. (2020). Datura Metel L. Leaves Extract Mediated CeO2 Nanoparticles: Synthesis, Characterizations, and Degradation Activity of DPPH Radical. Surf. Inter. 19, 100437. doi:10.1016/j.surfin.2020.100437
Keywords: biomolecules, capping agents, green synthesis, nanoparticles, therapeutic applications
Citation: Sidhu AK, Verma N and Kaushal P (2022) Role of Biogenic Capping Agents in the Synthesis of Metallic Nanoparticles and Evaluation of Their Therapeutic Potential. Front. Nanotechnol. 3:801620. doi: 10.3389/fnano.2021.801620
Received: 25 October 2021; Accepted: 20 December 2021;
Published: 31 January 2022.
Edited by:
Vijayakumar Sekar, Shandong University, ChinaReviewed by:
Sheng-Song Yu, University of Science and Technology of China, ChinaJyotsana Mehta, Guru Jambheshwar University of Science and Technology Hisar, India
Periasamy Anbu, Inha University, South Korea
Copyright © 2022 Sidhu, Verma and Kaushal. This is an open-access article distributed under the terms of the Creative Commons Attribution License (CC BY). The use, distribution or reproduction in other forums is permitted, provided the original author(s) and the copyright owner(s) are credited and that the original publication in this journal is cited, in accordance with accepted academic practice. No use, distribution or reproduction is permitted which does not comply with these terms.
*Correspondence: Priya Kaushal, priyakaushal159@gmail.com
†These authors have contributed equally to this work