- 1Department of Medical Laboratory Technology, Faculty of Applied Medical Sciences, Northern Border University, Arar, Saudi Arabia
- 2National Probiotics Laboratory, National Institute for Biotechnology and Genetic Engineering, Faisalabad, Pakistan
- 3Department of Chemistry, COMSATS University Islamabad, Islamabad, Pakistan
- 4Lebanese Institute for Biomedical Research and Application (LIBRA), Lebanese International University, Beirut, Lebanon
Microemulsions, comprising oil, water and a surfactant, in association with some co-surfactant, are thermodynamically stable systems. They have found applications in a large number of chemical and pharmacological processes due to their unique properties such as large interfacial area, low interfacial tension, and most importantly, the ability to solubilize and deliver hydrophobic drugs. In addition to the oral and intravenous route, they are suitable for drug delivery through the ophthalmic, vaginal, pulmonary, dental, and topical routes. This review highlights the properties and several recent developments in the use of microemulsions for medical treatment purposes including targeted drug delivery.
Introduction
Microemulsions comprise a special class of “dispersion” that may be transparent or translucent in appearance (Asua, 2014; Dixit & Mathur, 2015). They were first discovered by Hoar and Schulman (1943) in their experimental study of titration of long-chain fatty acids (soapy milky emulsions) with medium-/short-chain alcohols producing translucent or transparent system of emulsions. A schematic representation of the titration method adopted to produce is given below, which highlights the formation of transparent emulsion from water-in-oil (W/O) emulsion stabilized by long-chain fatty acids (soap) (Edser, 2011).
A definition for microemulsion was provided by Danielsson and Lindman in 1981 as “a system of water, oil and amphiphile which is a single optically isotropic and thermodynamically stable liquid solution” (Danielsson & Lindman, 1981). Generally, microemulsions can be described as pseudo-homogeneous mixtures of water, water-insoluble organic compounds, and a mixture of surfactant/co-surfactant (Paveglio et al., 2021) (Figure 1). It is clearly evident from Figure 1 that microemulsions can be prepared by mixing water, oil, surfactant/co-surfactant in different mixing ratios, considering the kind of microemulsion of either oil/water or water/oil. The amphiphiles (surfactant/co-surfactant mixture) lower the oil–water interfacial tension by interfacial adsorption, thus minimizing the positive free energy change of dispersion associated with the formation of a surface (Sharma et al., 2016).
Microemulsions are merely alike emulsions that are a different class of colloidal systems. We can observe colloidal systems in all three possible states of matter—gas, liquid, and solid. Basically, the emulsions are formed via the emulsification of two or more immiscible liquids primarily.
The emulsions are basically formed from two or more immiscible liquid phases via emulsification, a profound characteristic of surface-active agents, making them versatile for a wide range of practical application, for instance, milk and cream, butter, margarine, mayonnaise, espresso, cutting fluid for metal working, and the photo-sensitizer (Kale & Deore, 2016).
Properties
There are two types of emulsions depending upon the size of the particles—emulsions having particle size in range of <0.5–50 µm are known as macroemulsions. These are easily visible under the microscope. The other type of emulsions is called microemulsion and has a particle size ranging from 10 to 200 nm (0.01–0.20 µm) (Schuster, 1996). Emulsions may be classified according to the structure of the system or the nature of the emulsifier (Table 1) (Schuster, 1996). Interestingly, the size of dispersed particles in an emulsion actually determines its appearance to the naked eye. If the diameter of the dispersed particle is 1 μm, the emulsion is milky white; 1–0.1 µm, blue white; 0.1–0.05 µm, gray, semitransparent; and <0.05 µm, transparent. Thus, macroemulsion is opaque, and microemulsion is transparent or semitransparent to visible light.
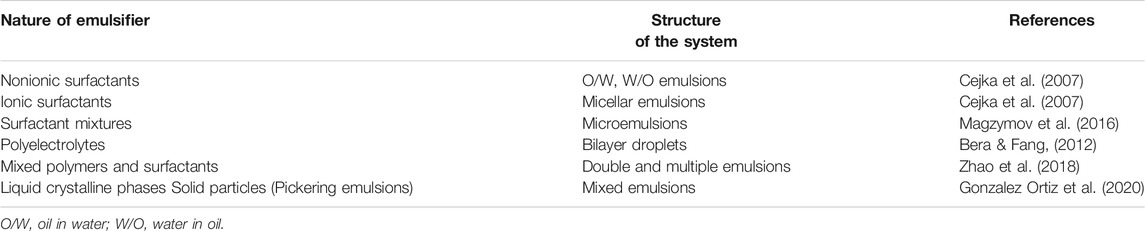
TABLE 1. Classification of emulsion types (Sweeta and Abdurahman, 2018).
Depending upon the nature of the dispersed particles, microemulsions are classified into the following types:
1) Water-in-oil microemulsion
The W/O type microemulsion is a dispersion of water or an aqueous solution in a water-immiscible liquid. The water is, in this case, the “discontinuous” (inner) phase, and the oil is the “continuous” (outer) phase (Figure 2).
2) Oil-in-water microemulsion
The oil-in-water type is a dispersion of a water-immiscible liquid (always called the oil) in an aqueous phase. The oil is, in this case, the “discontinuous” (inner) phase, and the aqueous phase is the “continuous” (outer) phase (Figure 2).
3) Bicontinuous microemulsion
It consists of an infinite bilayer bent everywhere with a saddle-like curvature so that it is multiconnected to itself (by many random “passages” similar to tubular connections in the sponge) isotropically over macroscopic distances and divides space into two independent solvents. Bicontinuous structures are present in the systems where the amount of water and oil is similar. Both the oil and water exist as a continuous phase in the presence of a continuously fluctuating surfactant-stabilized interface with a net curvature of zero (Figure 3) (Montalvo et al., 2001).
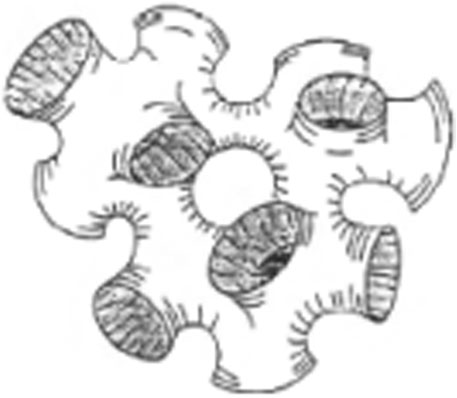
FIGURE 3. Schematic diagram of random connected bilayer network (Schuster, 1996).
Characterization of Microemulsions
The characterization techniques of microemulsions generally probe in at the macroscopic level, and microemulsions are generally evaluated at the macroscopic level via viscosity, conductivity, and dielectric measurement methods. The viscosity measurements probe in the micellar structure (Gukelberger et al., 2020; Majolino et al., 1990), the composition of emulsion is determined by conductivity measurements (Laguës & Sauterey, 1980; Pereira et al., 2016), and both the dynamic and detailed structural features of the emulsions are elucidated by dielectric measurements (Lian & Zhao, 2011; Weber & Stühn, 2016).
On the other hand, at the microscopic level, the optical clarity and isotropic nature of the emulsions are determined by spectroscopic techniques (Gao et al., 2006; Paul & Panda, 2014). A wide range of scattering techniques such as dynamic light scattering (DLS), static light scattering, small-angle X-ray scattering (SAXS), and small-angle neutron scattering (SANS) may provide detailed insight of the microstructure (Kaler et al., 1991; Lawrence & Rees, 2012; Paul & Panda, 2014). Nevertheless, the characterization of microemulsions is challenging due to inter particle-particle interactions due to the thickness of the solution, which needs statistical correction while measuring the droplet size accurately.
Pharmacological Applications
Microemulsions have been studied extensively as potential drug delivery vehicles for poorly water-soluble drugs (Barot et al., 2012; Chudasama et al., 2011; Formariz et al., 2006; Hegde et al., 2014; Hu et al., 2011, 2014; Vinarov et al., 2018; Vyas et al., 2006) (Table 2). They are extensively being used as drug carrier systems for topical, oral, and parenteral administration of drugs, offering a variety of advantages such as ease of preparation, spontaneous formation and scale-up, thermodynamic stability, enhanced drug solubilization, and bioavailability. Microemulsions dramatically enhance the therapeutic efficacy of drugs and reduce the volume of the drug delivery vehicle, thus minimizing toxic side effects. The presence of surfactant as a major component of the microemulsion facilitates the drug absorption by elevating the permeability of cell membrane. Besides, in case of lipophilic drug administration, the ability of cell membrane to solubilize lipophilic component tremendously aids its absorption.
A new microemulsion-based system for a poorly water-soluble drug myricetin has been developed. Microemulsions protect the incorporated drugs from oxidative and enzymatic degradation. Examples include commercially available microemulsion formulations of cyclosporin A, saquinavir, and ritonavir (Fricker et al., 2010).
Microemulsions exhibit a high solubilization capacity for both lipophilic and hydrophilic drugs; thus, more drugs can be loaded into the microemulsion, which increases the concentration gradient across the skin without depletion. The reservoir effect of the internal phase maintains a constant driving force of drugs from the external phase to the skin and prolongs absorption. Since the diffusion of the drug into the skin only occurs from the external phase of the microemulsion, the internal phase continually supplies drugs to the external phase so that it remains saturated with the drugs (Fricker et al., 2010).
Oil-in-water (O/W) microemulsion systems are being used in the pharmaceutical industry with hydrophobic fluorocarbons (as oils) to produce short-time blood plasma substitutes to maintain the supply of oxygen in the living systems. The components that have been used include lecithins and non-ionic surfactants (Brijs, Arlacel 186, Spans, Tweens and AOT).
Targeted Drug Delivery
Various strategies have been employed to increase the pharmacokinetic properties via promoting the lymphatic transport; thereby, hepatic first pass metabolism can be surmounted. These strategies include complexation, pH modification, and use of lipid-based delivery systems. Among lipid-based formulations, self-microemulsifying formulations (droplet size <100 nm) have been shown to improve the pharmacokinetic properties of hydrophobic drugs primarily due to their efficiency in facilitating solubilization and in presenting the hydrophobic drug in solubilized form whereby the dissolution process can be circumvented (Akula et al., 2014; Alwadei et al., 2019; Atef & Belmonte, 2008; Kazi et al., 2021; Shahba et al., 2018).
The self-emulsifying system has gained exposure of its ability to increase solubility and pharmacokinetic properties of poorly soluble drug. Self-emulsifying drug delivery system (SEDDS) is an isotropic mixture of oil, surfactant, and co-solvent. SEDDS produces fine oil in water emulsion when introduced in aqueous media under gentle agitation. SEDDS-bound drug forms have been prepared for oral delivery for improved intestinal absorption of drugs. Forty percent of the newly discovered drugs possess little or low water solubility and hence minimal pharmacokinetic properties. These drugs are good candidates to be formulated in the form of self-microemulsifying drug delivery system (SMEDDS) (Gibaud & Attivi, 2012).
Influence of Physicochemical Properties on Clinical Stability and Efficiency of Microemulsions
The stability of parenteral emulsion is mandatory for administering them to the body. In contrast, instability of emulsions results in droplet cohesion and their separation, whereby the stability of the emulsions is strongly influenced by its physicochemical properties (Washington et al., 1993; McClements, 2007; Ishii & Sakurai, 2012; Schuh et al., 2014; Jacob et al., 2020). The physicochemical properties of parenteral emulsions directly depend upon a range of inherent factors such as composition and concentration of both hydrophilic and hydrophobic area, surface tension, pH of the medium, extent of dissociation, droplet size, electrical charge of the droplet surface, and their mutual interaction (Han et al., 2004; Hasan, 2019; Jacob et al., 2020). The surface electrical charge (zeta potential) of the emulsifier indicates its extent of dissociation. The higher the zeta potential, the higher would be the electrostatic repulsion between adjacent emulsion droplets leading to enhanced stability and vice versa (Fontana et al., 2016). Further, high surface tension is another desirable parameter of emulsions, which implies the homogenous dispersion of oil droplets in the emulsion leading to its stability. Among all of these properties, the mean diameter of the droplet is crucial to the parenteral emulsions, because large droplet size not only is prone to the hazard of emboli formation but also does not provide stability for longer circulation in the blood stream and, hence, is not desirable (Kawaguchi et al., 2008).
Several reports have been published to enunciate the correlation of physicochemical properties of microemulsion to their clinical stability and efficiency. For instance, Han et al. (Han et al., 2004) reported the successful preparation of stable emulsions of 2.5 mg/ml of paclitaxel using lecithin–sodium deoxycholate as an emulsifier mixture. The found excellent stability of the emulsion in plasma in the presence of polyethylene glycol as a stabilizer. Ishii et al. (Ishii & Sakurai, 2012) presented that 1.2% (w/w) lecithin emulsifier resulted in improved stability of emulsion in terms of zeta potential and mean diameter of the droplet. Shimokawa et al. (2017) observed a clear dependence of droplet sizes of emulsions using three different kinds of emulsifiers (egg yolk lecithin, egg yolk lysolecithin, and a mixture of both) in relation to the pH of the medium. Kawaguchi et al. (2008) studied the effect of emulsifier on the stability of parenteral emulsion of phosphatidylcholine (PC-LM) in the presence of purified egg yolk lecithin (PEL) and observed desirable mean diameter of the droplet (250 nm) with good stability.
Future Directions
Microemulsions offer a wide range of applications such as targeted drug delivery, sustained drug delivery, controlled drug delivery, enzyme immobilization, enhancing bioavailability, and masking taste. Since orally delivered hydrophilic drugs are unstable in the gastrointestinal tract (GIT), new approaches must be found consisting of the use of biocompatible moieties for active targeting in clinical trials. Additionally, W/O microemulsions hinder the water-soluble drug molecules from being metabolized. W/O microemulsions, in addition to aqueous fluids, are converted to O/W microemulsions and, hence, release the active pharmaceutical ingredient (API), allowing the microemulsions to selectively release API to the targeted regions of GIT.
Author Contributions
All authors listed have made a substantial, direct, and intellectual contribution to the work and approved it for publication.
Conflict of Interest
The authors declare that the research was conducted in the absence of any commercial or financial relationships that could be construed as a potential conflict of interest.
Publisher’s Note
All claims expressed in this article are solely those of the authors and do not necessarily represent those of their affiliated organizations, or those of the publisher, the editors and the reviewers. Any product that may be evaluated in this article, or claim that may be made by its manufacturer, is not guaranteed or endorsed by the publisher.
References
Akula, S., Gurram, A. K., and Devireddy, S. R. (2014). Self-Microemulsifying Drug Delivery Systems: An Attractive Strategy for Enhanced Therapeutic Profile. Int. Scholarly Res. Notices 2014, 1–11. doi:10.1155/2014/964051
Aliberti, A. L. M., de Queiroz, A. C., Praça, F. S. G., Eloy, J. O., Bentley, M. V. L. B., and Medina, W. S. G. (2017). Ketoprofen Microemulsion for Improved Skin Delivery and In Vivo Anti-inflammatory Effect. AAPS PharmSciTech 18, 2783–2791. doi:10.1208/s12249-017-0749-6
Alwadei, M., Kazi, M., and Alanazi, F. K. (2019). Novel Oral Dosage Regimen Based on Self-Nanoemulsifying Drug Delivery Systems for Codelivery of Phytochemicals - Curcumin and Thymoquinone. Saudi Pharm. J. 27, 866–876. doi:10.1016/j.jsps.2019.05.008
Asua, J. M. (2014). Challenges for Industrialization of Miniemulsion Polymerization. Prog. Polym. Sci. 39, 1797–1826. doi:10.1016/j.progpolymsci.2014.02.009
Atef, E., and Belmonte, A. A. (2008). Formulation and In Vitro and In Vivo Characterization of a Phenytoin Self-Emulsifying Drug Delivery System (SEDDS). Eur. J. Pharm. Sci. 35, 257–263. doi:10.1016/j.ejps.2008.07.004
Barot, B. S., Parejiya, P. B., Patel, H. K., Gohel, M. C., and Shelat, P. K. (2012). Microemulsion-based Gel of Terbinafine for the Treatment of Onychomycosis: Optimization of Formulation Using D-Optimal Design. AAPS PharmSciTech. 13, 184–192. doi:10.1208/s12249-011-9742-7
Bera, T., and Fang, J. (2012). Polyelectrolyte-coated Liquid crystal Droplets for Detecting Charged Macromolecules. J. Mater. Chem. 22, 6807. doi:10.1039/c2jm00038e
Cejka, J., Bekkum, H. Van., Corma, A., and Schüth, F. (2007). Introduction to Zeolite Science and Practice. Stud. Surf. Sci. Catal. 168, 1–1058.
Danielsson, I., and Lindman, B. (1981). The Definition of Microemulsion. Colloids Surf. 3 (4): 391–392. doi:10.1016/0166-6622(81)80064-9
Dixit, G. R., and Mathur, V. B. (2015). Microemulsions: Platform for Improvement of Solubility and Dissolution of Poorly Soluble Drugs. Asian J. Pharm. Clin. Res. 8, 7–17.
Edser, C. (2011). Microemulsions: Putting Theory into Practice. Focus on Surfactants 2011, 2. doi:10.1016/s1351-4210(11)70304-3
Fontana, F., Ferreira, M. P. A., Correia, A., Hirvonen, J., and Santos, H. A. (2016). Microfluidics as a Cutting-Edge Technique for Drug Delivery Applications. J. Drug Deliv. Sci. Tech. 34, 76–87. doi:10.1016/j.jddst.2016.01.010
Formariz, T. P., Sarmento, V. H. V., Silva-Junior, A. A., Scarpa, M. V., Santilli, C. V., and Oliveira, A. G. (2006). Doxorubicin Biocompatible O/W Microemulsion Stabilized by Mixed Surfactant Containing Soya Phosphatidylcholine. Colloids Surf. B: Biointerfaces 51, 54–61. doi:10.1016/j.colsurfb.2006.05.005
Fricker, G., Kromp, T., Wendel, A., Blume, A., Zirkel, J., Rebmann, H., et al. (2010). Phospholipids and Lipid-Based Formulations in Oral Drug Delivery. Pharm. Res. 27, 1469–1486. doi:10.1007/s11095-010-0130-x
Gao, Y. a., Zhang, J., Xu, H., Zhao, X., Zheng, L., Li, X., et al. (2006). Structural Studies of 1-Butyl-3-Methylimidazolium Tetrafluoroborate/TX-100/p-Xylene Ionic Liquid Microemulsions. Chem. Eur. J. Chem. Phys. 7, 1554–1561. doi:10.1002/cphc.200600120
Gibaud, S., and Attivi, D. (2012). Microemulsions for Oral Administration and Their Therapeutic Applications. Expert Opin. Drug Deliv. 9, 937–951. doi:10.1517/17425247.2012.694865
Gonzalez Ortiz, D., Pochat-Bohatier, C., Cambedouzou, J., Bechelany, M., and Miele, P. (2020). Current Trends in Pickering Emulsions: Particle Morphology and Applications. Engineering 6, 468–482. doi:10.1016/j.eng.2019.08.017
Gukelberger, E., Hitzel, C., Mancuso, R., Galiano, F., Bruno, M., Simonutti, R., et al. (2020). Viscosity Modification of Polymerizable Bicontinuous Microemulsion by Controlled Radical Polymerization for Membrane Coating Applications. Membranes 10, 246. doi:10.3390/membranes10090246
Han, J., Davis, S. S., Papandreou, C., Melia, C. D., and Washington, C. (2004). Design and Evaluation of an Emulsion Vehicle for Paclitaxel. I. Physicochemical Properties and Plasma Stability. Pharm. Res. 21, 1573–1580. doi:10.1023/B:PHAM.0000041451.70367.21
Hasan, N. M. Y. (2019). Effect of Physicochemical Properties of Emulsions Formed by Self-Emulsifying Drug Delivery Systems (Sedds) on the Solubilization State of Drug: In Vitro Study. Int. J. App Pharm. 11, 61. doi:10.22159/ijap.2019v11i1.28664
Hegde, R. R., Bhattacharya, S. S., Verma, A., and Ghosh, A. (2014). Physicochemical and Pharmacological Investigation of Water/oil Microemulsion of Non-selective Beta Blocker for Treatment of Glaucoma. Curr. Eye Res. 39, 155–163. doi:10.3109/02713683.2013.833630
Hoar, T. P., and Schulman, J. H. (1943). Transparent Water-In-Oil Dispersions: the Oleopathic Hydro-Micelle. Nature 152, 102–103. doi:10.1038/152102a0
Hu, L., Hu, Q., and Yang, J. (2014). Enhancement of Transdermal Delivery of Ibuprofen Using Microemulsion Vehicle. Iranian J. Basic Med. Sci. 17, 760. doi:10.22038/ijbms.2014.3450
Hu, L., Wu, H., Niu, F., Yan, C., Yang, X., and Jia, Y. (2011). Design of Fenofibrate Microemulsion for Improved Bioavailability. Int. J. Pharmaceutics 420, 251–255. doi:10.1016/j.ijpharm.2011.08.043
Ishii, T., and Sakurai, Y. (2012). Challenges and New Directions for Next-Generation Drug Delivery System (DDS) Research Based on Nano-Technology. Yakugaku Zasshi 132, 1345–1346. doi:10.1248/yakushi.12-00234-F
Jacob, S., Nair, A. B., and Shah, J. (2020). Emerging Role of Nanosuspensions in Drug Delivery Systems. Biomater. Res. 24, 3. doi:10.1186/s40824-020-0184-8
Jadhav, K., Ambade, K., Jadhav, S., Gambhire, M., Kurmi, S., and Kadam, V. (2008). Formulation and Evaluation of Flurbiprofen Microemulsion. Cdd 5, 32–41. doi:10.2174/156720108783331032
Kale, S. N., and Deore, S. L. (2016). Emulsion Micro Emulsion and Nano Emulsion: A Review. Syst. Rev. Pharm. 8, 39–47. doi:10.5530/srp.2017.1.8
Kaler, E. W., Billman, J. F., Fulton, J. L., and Smith, R. D. (1991). A Small-Angle Neutron Scattering Study of Intermicellar Interactions in Microemulsions of AOT, Water, and Near-Critical Propane. J. Phys. Chem. 95, 458–462. doi:10.1021/j100154a080
Kawaguchi, E., Shimokawa, K.-i., and Ishii, F. (2008). Physicochemical Properties of Structured Phosphatidylcholine in Drug Carrier Lipid Emulsions for Drug Delivery Systems. Colloids Surf. B: Biointerfaces 62, 130–135. doi:10.1016/j.colsurfb.2007.09.027
Kazi, M., Alqahtani, A., Ahmad, A., Noman, O. M., Aldughaim, M. S., Alqahtani, A. S., et al. (2021). Development and Optimization of Sitagliptin and Dapagliflozin Loaded Oral Self-Nanoemulsifying Formulation against Type 2 Diabetes Mellitus. Drug Deliv. 28, 100–114. doi:10.1080/10717544.2020.1859001
Kizilbash, N. A., Asif, S., Nazar, M. F., Shah, S. S., and Alenizi, D. (2011). Design of a Microemulsion-Based Drug Delivery System for Diclofenac Sodium. J. Chem. Soc. Pakistan 33, 1–6.
Laguës, M., and Sauterey, C. (1980). Percolation Transition in Water in Oil Microemulsions. Electrical Conductivity Measurements. J. Phys. Chem. 84, 3503–3508. doi:10.1021/j100463a003
Lawrence, M. J., and Rees, G. D. (2012). Microemulsion-based media as Novel Drug Delivery Systems. Adv. Drug Deliv. Rev. 64, 175–193. doi:10.1016/j.addr.2012.09.018
Lian, Y., and Zhao, K. (2011). Dielectric Analysis of Micelles and Microemulsions Formed in a Hydrophilic Ionic Liquid. I. Interaction and Percolation. J. Phys. Chem. B 115, 11368–11374. doi:10.1021/jp205493r
Lv, F., Li, N., Zheng, L., and Tung, C. (2006). Studies on the Stability of the Chloramphenicol in the Microemulsion Free of Alcohols. Eur. J. Pharmaceutics Biopharmaceutics 62, 288–294. doi:10.1016/j.ejpb.2005.09.006
Magzymov, D., Qiao, C., and Johns, R. T. (2016). Impact of Surfactant Mixtures on Microemulsion Phase Behavior. SPE Annual Technical Conference and Exhibition. SPE-181651-MS. Dubai, UAE. doi:10.2118/181651-MS
Majolino, D., Mallamace, F., Venuto, S., and Micali, N. (1990). Viscosity Measurements in Dense Microemulsions. Phys. Rev. A. 42, 7330–7339. doi:10.1103/PhysRevA.42.7330
McClements, D. J. (2007). Critical Review of Techniques and Methodologies for Characterization of Emulsion Stability. Crit. Rev. Food Sci. Nutr. 47, 611–649. doi:10.1080/10408390701289292
Moghimipour, E., Salimi, A., Karami, M., and Isazadeh, S. (2013). Preparation and Characterization of Dexamethasone Microemulsion Based on Pseudoternary Phase Diagram. Jundishapur J. Nat. Pharm. Prod. 8, 105–112. doi:10.17795/jjnpp-9373
Montalvo, G., Valiente, M., Mortensen, K., and Gradzielski, M. (2001). Structural Changes Induced in the Surfactant System C12E4/benzyl Alcohol/water by the Admixture of the Cationic Surfactant Cetylpyridinium Chloride. J. Colloid Interf. Sci. 238, 251–258. doi:10.1006/jcis.2001.7482
Nazar, M. F., Khan, A. M., and Shah, S. S. (2009). Microemulsion System with Improved Loading of Piroxicam: A Study of Microstructure. AAPS PharmSciTech. 10, 1286. doi:10.1208/s12249-009-9328-9
Paul, S., and Panda, A. K. (2014). Combined Phase Behavior, Dynamic Light Scattering, Viscosity and Spectroscopic Investigations of a Pyridinium-Based Ionic Liquid-In-Oil Microemulsion. RSC Adv. 4, 32383–32390. doi:10.1039/c4ra01209g
Paveglio, G., Milani, F., Sauer, A., Roman, D., Meyer, A., and Pizzuti, L. (2021). Structure-physical Properties Relationship of Eutectic Solvents Prepared from Benzyltriethylammonium Chloride and Carboxylic Acids. J. Braz. Chem. Soc. 15, 12. doi:10.21577/0103-5053.20200208
Peira, E., Scolari, P., and Gasco, M. R. (2001). Transdermal Permeation of Apomorphine through Hairless Mouse Skin from Microemulsions. Int. J. Pharmaceutics 226, 47–51. doi:10.1016/S0378-5173(01)00759-1
Peltola, S., Saarinen-Savolainen, P., Kiesvaara, J., Suhonen, T. M., and Urtti, A. (2003). Microemulsions for Topical Delivery of Estradiol. Int. J. Pharmaceutics 254, 99–107. doi:10.1016/S0378-5173(02)00632-4
Pereira, T. C., Conceição, C. A. F., Khan, A., Fernandes, R. M. T., Ferreira, M. S., Marques, E. P., et al. (2016). Application of Electrochemical Impedance Spectroscopy: A Phase Behavior Study of Babassu Biodiesel-Based Microemulsions. Spectrochimica Acta A: Mol. Biomol. Spectrosc. 168, 60–64. doi:10.1016/j.saa.2016.05.034
Schuh, R. S., Bruxel, F., and Teixeira, H. F. (2014). Physicochemical Properties of Lecithin-Based Nanoemulsions Obtained by Spontaneous Emulsification or High-Pressure Homogenization. Quimica Nova. 37(7): 1193–1198. doi:10.5935/0100-4042.20140186
Schuster, D. (1996). “Encyclopedia of Emulsion Technology: Volume 4,” in Journal of Dispersion Science and Technology.
Shahba, A. A.-W., Alanazi, F. K., and Abdel-Rahman, S. I. (2018). Stabilization Benefits of Single and Multi-Layer Self-Nanoemulsifying Pellets: A Poorly-Water Soluble Model Drug with Hydrolytic Susceptibility. PLoS ONE 13, e0198469. doi:10.1371/journal.pone.0198469
Sharma, A. K., Garg, T., Goyal, A. K., and Rath, G. (2016). Role of Microemuslsions in Advanced Drug Delivery. Artif. Cell Nanomedicine, Biotechnol. 44, 1177–1185. doi:10.3109/21691401.2015.1012261
Shimokawa, K.-i., Nagasaka, Y., Sawa, M., Wada, Y., and Ishii, F. (2017). Physicochemical Properties and Stability of the Emulsion Prepared with Various Emulsifiers for Enteral Nutrition Preparations. J. Dispersion Sci. Tech. 38, 1221–1226. doi:10.1080/01932691.2016.1230066
Shishoo, C., Chudasama, A., Patel, V., Nivsarkar, M., and Vasu, K. (2011). Investigation of Microemulsion System for Transdermal Delivery of Itraconazole. J. Adv. Pharm. Tech. Res. 2, 30. doi:10.4103/2231-4040.79802
Sintov, A. C., and Shapiro, L. (2004). New Microemulsion Vehicle Facilitates Percutaneous Penetration In Vitro and Cutaneous Drug Bioavailability In Vivo. J. Controlled Release 95, 173–183. doi:10.1016/j.jconrel.2003.11.004
Sweeta, A., and Abdurahman, H. N. (2018). Emulsion Types, Stability Mechanisms and Rheology: A Review. Int. J. Innovative Res. Scientific Stud. 1, 14–21. doi:10.53894/ijirss.v1i1.4
Vinarov, Z., Dobreva, P., and Tcholakova, S. (2018). Effect of Surfactant Molecular Structure on Progesterone Solubilization. J. Drug Deliv. Sci. Tech. 43, 44–49. doi:10.1016/j.jddst.2017.09.014
Vyas, T. K., Babbar, A. K., Sharma, R. K., Singh, S., and Misra, A. (2006). Preliminary Brain-Targeting Studies on Intranasal Mucoadhesive Microemulsions of Sumatriptan. AAPS PharmSciTech. 7, E49–E57. doi:10.1208/pt070108
Washington, C., Koosha, F., and Davis, S. S. (1993). Physicochemical Properties of Parenteral Fat Emulsions Containing 20% Triglyceride; Intralipid and Ivelip. J. Clin. Pharm. Ther. 18, 123–131. doi:10.1111/j.1365-2710.1993.tb00578.x
Weber, A., and Stühn, B. (2016). Structure and Phase Behavior of Polymer Loaded Non-ionic and Anionic Microemulsions. J. Chem. Phys. 144, 144903. doi:10.1063/1.4945610
Yang, J.-H., Kim, Y.-l., and Kim, K.-M. (2002). Preparation and Evaluation of Aceclofenac Microemulsion for Transdermal Delivery System. Arch. Pharm. Res. 25, 534–540. doi:10.1007/BF02976614
Keywords: microemulsions, targeted drug delivery, biocompatible nanostructures, surfactants, self-emulsifying agents
Citation: Suhail N, Alzahrani AK, Basha WJ, Kizilbash N, Zaidi A, Ambreen J and Khachfe HM (2021) Microemulsions: Unique Properties, Pharmacological Applications, and Targeted Drug Delivery. Front. Nanotechnol. 3:754889. doi: 10.3389/fnano.2021.754889
Received: 07 August 2021; Accepted: 16 September 2021;
Published: 12 November 2021.
Edited by:
Ioannis Liakos, Independent researcher, Volos, GreeceReviewed by:
Eskandar Moghimipour, Ahvaz Jundishapur University of Medical Sciences, IranBapu Surnar, University of Miami Hospital, United States
Monzer Fanun, Al-Quds University, Palestine
Copyright © 2021 Suhail, Alzahrani, Basha, Kizilbash, Zaidi, Ambreen and Khachfe. This is an open-access article distributed under the terms of the Creative Commons Attribution License (CC BY). The use, distribution or reproduction in other forums is permitted, provided the original author(s) and the copyright owner(s) are credited and that the original publication in this journal is cited, in accordance with accepted academic practice. No use, distribution or reproduction is permitted which does not comply with these terms.
*Correspondence: Nadeem Kizilbash, fsd707@gmail.com