- 1Department of Women’s and Children’s Health, Karolinska Institutet, Stockholm, Sweden
- 2Astrid Lindgren Children’s Hospital, Karolinska University Hospital, Stockholm, Sweden
- 3Department of Sustainable Energy Technology, SINTEF, Oslo, Norway
The COVID-19 pandemic underlined that by investing in both basic and clinical life science research and if there are enough volunteers, it is feasible to have -validated by Phase III clinical trials- vaccines in less than a year. Regarding the treatment options for the people who were infected by COVID-19, we know that it was the large clinical trials - like SOLIDARITY (WHO) and RECOVERY (UK)- that gave the most valid results, and that although hundreds of drugs were repurposed, sadly, most proved to be unsuccessful. Repurposing drugs and compassionate use, were the only options for the first half of 2020. The same applied to the convalescent plasma (CP) approach; however, apart from CP, other cell derived therapeutics were deployed, such as synthetic monoclonal antibodies, which were also tested and given provisional licences by health authorities. Unfortunately, synthetic antibody production comes with problems related to low and slow yield that were not overcome, while SARS-CoV-2 viral mutations may possibly render them less effective. One approach that works and is currently assessed in several clinical trials, is mesenchymal stromal cell (MSCs) and extracellular vesicle (EV) administration for therapy. Interdisciplinarity may prove key here. Easy to produce nanomaterials and biomaterials should be further investigated to increase bioproduction of MSCs, both at the level of therapeutics, as the base substrate for EV production and to upscale synthetic antibody production for therapy.
Introduction
The global urgency of the Covid-19 pandemic coupled with the funding and the contribution of volunteers has advanced vaccine research and clinical trials with unprecedented speed. Although magnanimous efforts were put into repurposing drugs to fight the coronavirus infection, the side of pharmaceutical interventions failed to catch up with the speed of the prophylactic interventions, i.e., the vaccine R&D. These novel vaccine platforms, some of which had never been marketed before the pandemic, gained conditional authorization for use in humans (such as the Pfizer/BioNTech and Moderna vaccines), are essentially nanocarrier based delivery systems.
Irrespective of the advantages weighing on their side, the formulation or the adjuvants of the nanoparticle delivery platforms may induce immunogenic reactions and may not be consistent amongst different people. Keeping these limitations under consideration, other types of carriers have also been designed and even deployed for COVID-19 treatment. At the forefront of these mainly MSC derived (Li et al., 2020) cell-based therapeutics are extracellular vesicles (EVs) (Börger et al., 2020; O'Driscoll, 2020; Pocsfalvi et al., 2020; Machhi et al., 2021).
Broad Applications of Stem- and Mesenchymal Stromal- Cells and Extracellular Vesicles in Clinical Trials
Pluripotent stem cells, and mesenchymal stromal cells (MSCs) have great therapeutic potential (Naji et al., 2019; Robb et al., 2019), both due to their differentiation potential and hypoimmunogenicity, and their immunomodulatory, anti-inflammatory and cell-protective profile. Extracellular vesicles (exosomes and shedding vesicles; EVs) derive from and are released by the cells. They are considered a “storable,” cell-free therapeutic alternative, documented to comparable therapeutic properties to the cells they are generated from Phinney and Pittenger (2017), Qiu et al. (2019).
Breakthrough in COVID-19 Clinical Trials Using EXO-CD24 Exosomes
A biologic therapeutic agent based on exosomes carrying CD24 has successfully completed phase 1 trials (ClinicalTrials.gov Identifier: NCT04747574). CD24 is a protein located on the cell surface and has a well-known role in regulating the immune system. The rationale for its use is that in the case of COVID-19, it can fight off the cytokine storm, i.e., the potentially lethal immune overreaction to the infection caused by SARS-COV-2, that is believed to be responsible for much of the deaths associated with the disease. According to the description of the trial, exosomes overexpressing CD24, isolated and purified from T-REx™-293 cells engineered to express CD24 at high levels, can be delivered intranasally to the target organ i.e., the lung, and suppress the cytokine storm as a highly compatible delivery vehicle. The preparation is inhaled once a day for a few minutes, for 5 days, whereas the intranasal route of administration significantly reduces both the dose that would have been necessary for systemic administration, and the risk for side effects and thus adverse events. The Phase I clinical trial had recruited 30 patients whose conditions were moderate or worse; all 30 recovered, and 29 of them within three to 5 days. The results were confirmed by the phase II study and the therapeutic exosomes are now at phase III. However, as mentioned previously, the bioproduction of the cells might prove expensive to scale up and match an increasingly larger demand.
Still Lacking Information on Safety and Efficacy
EVs are membrane-surrounded structures that are secreted by cells and involved in cell to cell communication, delivering signaling molecules. Furthermore, accumulating research accounts for the new and emerging therapeutic, wound healing and immunomodulatory roles of EVs beyond inflammation and lung infections. Groundbreaking work, however, has also shown that the numbers of EVs secreted by the cells that have been infected by a virus, increase immensely, demonstrating thus their role in disease pathogenesis (Yoshikawa et al., 2019). Encompassing these properties, and their drug delivery capacity, EVs are a promising antiviral therapeutic, such as those lacking in the COVID-19 pandemic. However, the heterogeneity of the inherent sources in physiology and disease, and the differences resulting from the age of the cells as seen in studies, may result in functional differences at the level of regenerative capacity. Moreover, it should be highlighted that no EV-based therapy has been approved to date.
All the above underline the need for further research, and optimization of EV isolation and advanced characterization techniques, will be fundamental to deconvolute both the safety and the efficacy of EV-based therapies against COVID-19 and beyond this pandemic.
One of the advantages of allogeneic cell therapeutics, such as MSCs and EVs, is that they can be prepared in advance to meet patient needs, as a potent on-demand, off-the-shelf treatment. This renders them highly significant, and not only in pandemics where we are facing huge health system burdens. Off the shelf therapeutics, and standardized SOPs to scale-up biocultures, are eminent in diseases such as COVID-19 and certain others, where the window to treat may be too short to manufacture and test EVs derived from the patient’s own MSCs. Thus, studies to develop biomaterials that can accelerate bioproduction and pave the way to the development of allogeneic clinical products for clinical trials, should be facilitated in the future.
Currently over 75 MSCs therapies - of different tissue origin- for COVID-19 are registered (clinicaltrials.gov/ct2/results?cond=Covid19&term=mesenchymal+stem+&cntry=&state=&city=&dist=). However, one of the challenges in cell therapy, which is also a challenge in MSC-EV biomanufacturing, is as mentioned, optimizing SOPs to be scalable while developing a platform for cGMP-compatible manufacturing. Thus, manipulation of culture conditions needs to be employed in future studies, to optimize the EV production by MSCs aiming to decrease scale and cost per manufactured EV therapeutic batch.
Interdisciplinarity Is Key to Study the Secretion of Extracellular Vesicles and Their Membrane Properties
EVs and shedding vesicles originate as bulges on the surface of the cells. However, increasing their turnover and how their formation can be stimulated depending on cell type, is under investigation. Thus, it is not only a matter of scientific breakthrough but also of utmost clinical importance to understand the mechanism that EVs are secreted from the cells, and more importantly the MSCs and stem cells that are used in clinical trials. Furthermore, it is equally or even more important to identify the effect of how different drugs and substances or even cell culture conditions may affect the yield of the production of EVs.
Enriching Culture Conditions With Graphene
Graphene oxide (GO) has several favourable features, including excellent bio-adaptability, high drug loading capacity, privileged electroconductivity, whereas both size and shape can be controlled. Furthermore, the numerous hydroxyl and carboxylic acid GO functional groups, endow GO with hydrophilic properties (Kim et al., 2014). These functional groups also facilitate conjugation of GO to other molecules and polymers, allowing it to be used in sophisticated drug delivery applications (Gao, 2015). Graphene oxide containing nanoscaffolds and EVs can potentially find high applicability in upscaling of biocultures, to use in tissue regeneration and drug delivery studies, as research is showing (Geetha Bai et al., 2019; Driscoll et al., 2021; Zhao et al., 2021). Approaches should however be comprehensively finetuned for human targeted applications, keeping in mind the potential cytotoxicity of GO (Wang et al., 2011). Recently, small graphene oxide (s-GO) nanoflakes were used to boost vesicle shedding from cells in cultures and the s-GO generated microvesicles were comparable to those generated by ATP (Musto et al., 2021).
We have also used GO nanoflakes and atomic force microscopy (AFM) to follow up vesicles on the membranes of fixed human embryonic stem cells (Figure 1).
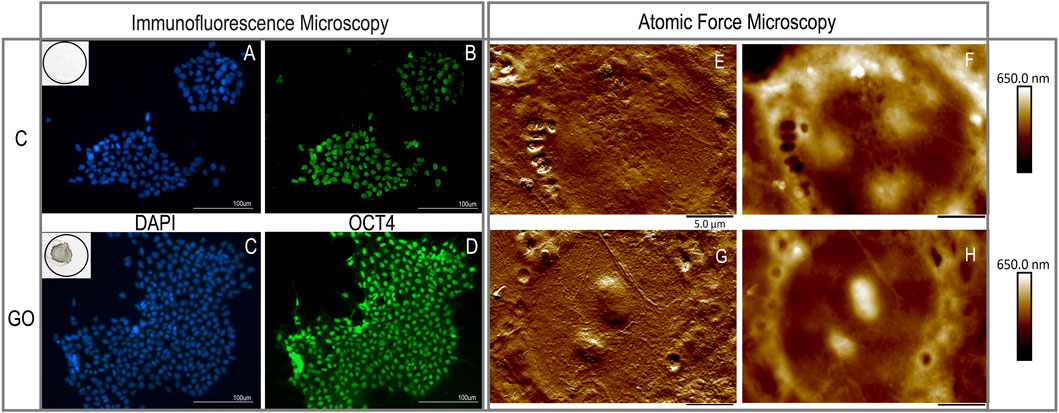
FIGURE 1. Micrographs of fixed hESCs grown for 2 days on Geltrex base matrix [top row; control (C)] or on the Geltrex/ graphene oxide compound substrate [bottom row; (GO)]. Parallel cultures of hESCs were processed for immunofluorescence (left panel) or atomic force microscopy (AFM; right panel) analysis. Under the culture conditions described, there were no signs of substrate induced toxicity and cell death, as demonstrated by the intact cell nuclei stained with DAPI (in blue; (A,C)) or loss of pluripotency and differentiation, as demonstrated by the pluripotency marker OCT4 evidently present in all hESCs (images (B,D)). The topography and the peak force error signal of a single cell is imaged in each of the AFM images (G top, GO bottom row; (E,G) for peak force error, (F,H) for surface topography showing the surface topographical properties of the cells).
AFM is an underused tool to study the surface of both fixed and living cells. With a resolution power well beyond that of optic microscopy and capacity for quantification (e.g., height and membrane stiffness) it is an excellent instrument to employ for the nanometric-sized extracellular vesicle studies described. We therefore used AFM topographic imaging to analyse the possible effect of GO nanoflakes on vesicle formation.
Graphene Oxide (GO) flakes (100 nm–5 µm) were obtained from Abalonyx AS, washed and diluted to remove excess contaminants from the production process and to increase the pH. We used GO (50 µg/ml) in combination with Geltrex matrix (ThermoFisher, diluted according to the manufacturer’s instructions) to coat 13 mm round coated glass coverslips. HS360 hESCs (30.000 cells per coverslip) were seeded either on Geltrex (control, C) or Geltrex-GO (GO) coated glass coverslips and grown for 2 days in Essential 8 medium (ThermoFisher) for 2 days, where they reached 60–70% confluency. The cells were then fixed with a 4% paraformaldehyde/PBS solution, washed 3X with PBS and permeabilized with 0.1% Triton X-100, following standard cell fixation and preparation for immunocytochemistry protocols.
We performed confocal microscopy and the micrographs shown in Figure 1 depict representative immunofluorescent images of the C and GO cultures. The nuclei of the cells that are stained blue with DAPI were intact (A and C correspond to DAPI in C and GO cultures, respectively). We did not observe any signs of cell death or differentiation induced by the substrate. Moreover, growing hESC cells for 2 days on the compound base matrix substrate containing GO, did not have an effect on the pluripotency of the hESCs, as demonstrated by the pluripotency marker OCT4 (STEMCELL Technologies, #60093AD, used 1:250) by immunocytochemistry. The images shown on B and D, similarly, correspond to the same C and GO cultures illustrated by the DAPI images above them.
The atomic force microscopy images were obtained using a state-of-the-art Bruker Multimode 8 microscope, operated in peak force tapping mode with ScanAsyst-air probes (0.4 N/m). All images obtained were 512 × 512 pixels. Both the surface topography and the peak force error signal of single cell is imaged in each of the AFM images. As before, an isolated cell of a colony growing on Geltrex is depicted on the top row (E for surface topography and F for peak force error), and a cell from a colony growing on Geltrex-GO is shown on the bottom row of the right panel (G and H, for the respective imaging modes)). In the case of the cells growing on Geltrex-GO their nuclear regions seem pre-dominantly raised, but a preliminary quantification using the AFM analysis software showed no significance. Further studies at different timepoints and culture confluence will delineate whether this finding is important to cell adherence due to the substrate’s effect.
Zooming out and imaging a larger size of the hESC colonies (as seen on the left panel of Figure 2), we observe that the cell packing, and colony formation were not affected (control on the top row and GO on the lower row. A and C represent surface topography and B and D represent images taken in peak force error mode, respectively. The yellow arrows were placed to show the fine edges of a GO nanoflake the cells are growing on; yet one more proof that the nanoflakes on the coating substrate were not toxic to the hESC cell culture. The surface of the membrane as demonstrated by the AFM revealed small and larger protrusions and pits with clear, raised rims. On the right panel (2, of Figure 2), a magnified image of the control (A) and Geltrex-GO (B) cell culture showing possible sites of release shedding vesicles, are framed in light blue rectangles. The craters/pits resemble the membrane fused multivesicular bodies forming vesicles for release from the cell membrane and are framed in yellow rectangles.
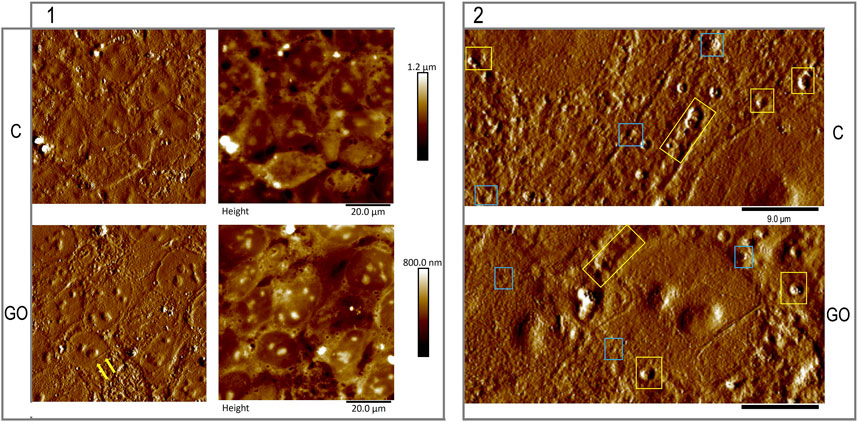
FIGURE 2. Representative larger areas of the hESCs grown for 2 days on Geltrex base matrix [top row; control (C)] or on the Geltrex/graphene oxide compound substrate [bottom row; (GO)]. In the left panel (1), we observe that the close cell packing, and colony formation were not affected by the graphene oxide addition to the base matrix. The yellow arrows were placed to show the fine edges of a GO nanoflake the cells are growing on. The surface of the membrane as demonstrated by the AFM revealed small and larger protrusions and pits with clear, raised rims. On the right panel (2), a magnified image of the control (A) and Geltrex-GO (B) cell culture, possibly showing sites of shedding vesicles are framed in light blue rectangles. The framed in yellow rectangles craters/ pits resemble membrane fused multivesicular bodies forming vesicles for release from the cell membrane.
Our results confirm that graphene oxide nanoflakes should be further investigated as a coating biomaterial for hESC cultures, aiming to identify whether GO enhances the secretion of EVs. As discussed, the small size of EVs, falls below the resolving power of optical instruments, and most of the EV morphological studies use electron microscopy. In this report, we show that AFM topographic imaging is an advanced tool to study the EVs that are budding out to be secreted and the pits they leave on the surface of the membrane of fixed the hESCs. Moreover, we suggest that the use of nanomaterials supported by AFM should be exploited in future drug studies regarding release of extracellular vesicles.
Conclusion
Advances in nanomaterial sciences contributed vastly to the vaccine development, as we saw in the COVID-19 pandemic. The need for universal, off-the-shelf immunomodulatory therapeutics such as MSCs and EVs was also identified. Taking into consideration the difficulties in the development of therapeutics, interdisciplinary approaches that go beyond “all-hands-on-board” should be facilitated. Moreover, we can greatly benefit by the tools routinely used by material sciences, to develop and comprehensively characterize novel potent nanomaterials, aiming to standardize and upscale bioproduction of cell and cell derived therapeutics. Real interdisciplinarity is eminent in order to start preparing now for future global threats, like the COVID-19 pandemic.
Data Availability Statement
The original contributions presented in the study are included in the article/Supplementary Material, further inquiries can be directed to the corresponding author.
Author Contributions
AS: cell culture preparation, ICC, confocal microscopy, BB: GO nanoflake preparation, AFM microscopy. AS wrote the manuscript draft, BB and AS edited and approved of the final draft.
Conflict of Interest
The authors declare that the research was conducted in the absence of any commercial or financial relationships that could be construed as a potential conflict of interest.
Publisher’s Note
All claims expressed in this article are solely those of the authors and do not necessarily represent those of their affiliated organizations, or those of the publisher, the editors and the reviewers. Any product that may be evaluated in this article, or claim that may be made by its manufacturer, is not guaranteed or endorsed by the publisher.
References
Börger, V., Weiss, D. J., Anderson, J. D., Borràs, F. E., Bussolati, B., Carter, D. R. F., et al. (2020). International Society for Extracellular Vesicles and International Society for Cell and Gene Therapy Statement on Extracellular Vesicles from Mesenchymal Stromal Cells and Other Cells: Considerations for Potential Therapeutic Agents to Suppress Coronavirus Disease-19. Cytotherapy 22 (9), 482–485. Sep. doi:10.1016/j.jcyt.2020.05.002
Driscoll, J., Moirangthem, A., Yan, I. K., and Patel, T. (2021). Fabrication and Characterization of a Biomaterial Based on Extracellular-Vesicle Functionalized Graphene Oxide. Front. Bioeng. Biotechnol. 9, 686510. Jun 9. doi:10.3389/fbioe.2021.686510
Gao, W. (2015). Graphene Oxide: Reduction Recipes, Spectroscopy, and Applications. doi:10.1007/978-3-319-15500-5
Geetha Bai, R., Muthoosamy, K., Manickam, S., and Hilal-Alnaqbi, A. (2019). Graphene-based 3D Scaffolds in Tissue Engineering: Fabrication, Applications, and Future Scope in Liver Tissue Engineering. Int. J. Nanomedicine 14, 5753–5783. Jul 24. doi:10.2147/IJN.S192779
Kim, J., Jeon, J. ‐H., Kim, H. ‐J., Lim, H., and Oh, I. ‐K. (2014). Durable and Water-Floatable Ionic Polymer Actuator with Hydrophobic and Asymmetrically Laser-Scribed Reduced Graphene Oxide Paper Electrodes. ACS Nano 8 (3), 2986–2997. doi:10.1021/nn500283q
Li, Z., Niu, S., Guo, B., Gao, T., Wang, L., Wang, Y., et al. (2020). Stem Cell Therapy for COVID‐19, ARDS and Pulmonary Fibrosis. Cell Prolif 53 (12), e12939.Dec. doi:10.1111/cpr.12939
Machhi, J., Shahjin, F., Das, S., Patel, M., Abdelmoaty, M. M., Cohen, J. D., et al. (2021). A Role for Extracellular Vesicles in SARS-CoV-2 Therapeutics and Prevention. J. Neuroimmune Pharmacol. 16 (2), 270–288. doi:10.1007/s11481-020-09981-0
Musto, M., Parisse, P., Pachetti, M., Memo, C., Di Mauro, G., Ballesteros, B., et al. (2021). Corrigendum to Shedding Plasma Membrane Vesicles Induced by Graphene Oxide Nanoflakes in Brain Cultured Astrocytes [CARBON 176 (2021) 458–469]. Carbon 178, 824. doi:10.1016/j.carbon.2021.01.142
Naji, A., Eitoku, M., Favier, B., Deschaseaux, F., Rouas-Freiss, N., and Suganuma, N. (2019). Biological Functions of Mesenchymal Stem Cells and Clinical Implications. Cell Mol. Life Sci. 76 (17), 3323–3348. SepEpub 2019 May 4. doi:10.1007/s00018-019-03125-1
O'Driscoll, L. (2020). Extracellular Vesicles from Mesenchymal Stem Cells as a Covid-19 Treatment. Drug Discov. Today 25 (7), 1124–1125. Jul. doi:10.1016/j.drudis.2020.04.022
Phinney, D. G., and Pittenger, M. F. (2017). Concise Review: MSC-Derived Exosomes for Cell-free TherapyEpub 2017 Mar 10. Erratum in: Stem Cells. Stem Cells 3535 (49), 851–858. Apr2017 SepPMID: 28294454. doi:10.1002/stem.2575
Pocsfalvi, G., Mammadova, R., Ramos Juarez, A. P., Bokka, R., Trepiccione, F., and Capasso, G. (2020). COVID-19 and Extracellular Vesicles: An Intriguing Interplay. Kidney Blood Press. Res. 45 (5), 661–670. doi:10.1159/000511402
Qiu, G., Zheng, G., Ge, M., Wang, J., Huang, R., Shu, Q., et al. (2019). Functional Proteins of Mesenchymal Stem Cell-Derived Extracellular Vesicles. Stem. Cel. Res. Ther. 10 (1), 359. Nov 28. doi:10.1186/s13287-019-1484-6
Robb, K. P., Fitzgerald, J. C., Barry, F., and Viswanathan, S. (2019). Mesenchymal Stromal Cell Therapy: Progress in Manufacturing and Assessments of Potency. Cytotherapy 21 (3), 289–306. MarEpub 2018 Dec 6. doi:10.1016/j.jcyt.2018.10.014
Wang, K., Ruan, J., Song, H., Zhang, J., Wo, Y., Guo, S., et al. (2011). Biocompatibility of Graphene Oxide. Nanoscale Res. Lett. 6 (1), 1–8. Dec. doi:10.1007/s11671-010-9751-6
Yoshikawa, F. S., Teixeira, F. M., Sato, M. N., and Oliveira, L. M. (2019). Delivery of microRNAs by Extracellular Vesicles in Viral Infections: Could the News Be Packaged. Cells 8 (6), 611. Jun. doi:10.3390/cells8060611
Keywords: human embryonic stem cell, graphene oxide, interdisciplinaiy, exosomes, stem cells, MSCs, EVs, AFM
Citation: Samara A and Belle B (2021) Nanomaterials Upscaling Cell Production and Advancing Exosome-Based Stem Cell Therapies. Front. Nanotechnol. 3:714824. doi: 10.3389/fnano.2021.714824
Received: 25 May 2021; Accepted: 21 September 2021;
Published: 14 October 2021.
Edited by:
Francis Verpoort, Wuhan University of Technology, ChinaReviewed by:
Jonathan M. Zuidema, University of California, San Diego, United StatesVijayakumar Sekar, Shandong University, Weihai, China
Copyright © 2021 Samara and Belle. This is an open-access article distributed under the terms of the Creative Commons Attribution License (CC BY). The use, distribution or reproduction in other forums is permitted, provided the original author(s) and the copyright owner(s) are credited and that the original publication in this journal is cited, in accordance with accepted academic practice. No use, distribution or reproduction is permitted which does not comply with these terms.
*Correspondence: Athina Samara, athina.samara@ki.se