- 1Division of Biochemistry, Faculty of Basic Sciences, SKUAST, Jammu, India
- 2School of Life Sciences, Jaipur National University, Jaipur, India
- 3Division of Fruit Science SKUAST-Kashmir, Srinagar, India
- 4Division of Basic Science and Humanities SKUAST-Kashmir, Srinagar, India
- 5Department of Biotechnology, University of Kashmir, Srinagar, India
- 6Department of Food Science and Technology, School of Agricultural Engineering and Food Science, Shandong University of Technology, Zibo, China
The use of silver nanoparticles (AgNPs) against various pathogens is now being well recognized in the agriculture and health sector. Nanoparticles have been shown to exhibit various novel properties and these properties, on other hand, rely upon the size, shape, and morphology of these particles. Moreover, these physical characteristics enable them to interact with microbes, plants, and animals. Smaller-sized particles have shown more toxicity than larger-sized nanoparticles. AgNPs have shown growth inhibition of many fungi like Aspergillus fumigates, A. niger, A. flavus, Trichophyton rubrum, Candida albicans, and Penicillium species. According to the current hypothesis, AgNPs act by producing reactive oxygen species and free radicals, which cause protein denaturation, nucleic acid and proton pump damage, lipid peroxidation, and cell wall damage. Therefore, they alter the cell membrane permeability, causing cell death. This mini-review summarizes the use of silver nanoparticles against fungal pathogens and fungal biofilm in the agricultural sector.
Introduction
Nanotechnology is an emerging area of scientific research that has been extremely well-known in recent decades (Mohseniazar et al., 2011). It has a wide range of applications in microbiology and biotechnology. It can assist in development, design, and manipulation of nanostructures to extremely small dimensions and with large surface area to volume ratio. As a result of their exceptional properties, nanoparticles attracted the attention of researchers in a variety of disciplines. Morphology, size, and chemical properties of NPs are used to classify nanoparticles and hence they are classified according to their physical and chemical properties; there are several well-known classes of nanoparticles (Khan et al., 2019) like the organic, inorganic, and carbon-based.
Nanoparticles or polymers of organic nature include dendrimers, micelles, liposomes, and ferritin. Micelles and liposomes have a hollow core, also known as a nanocapsule, and they are sensitive to thermal and electromagnetic radiation. Due to their efficiency, these are widely used in the biomedical field, for example, drug delivery systems (Tiwari et al., 2008). Metal- and metal oxide–based nanoparticles are generally categorized as inorganic nanoparticles. Metal-based nanoparticles are synthesized using destructive or constructive processes; the most commonly used metals are aluminum (Al), cadmium (Cd), cobalt (Co), copper (Cu), gold (Au), iron (Fe), lead (Pb), silver (Ag), and zinc (Zn). These nanoparticles have unique characteristics, including sizes ranging from 10 to 100 nm, high surface area to volume ratios, pore sizes, surface charge and surface charge density, crystalline and amorphous structures, spherical and cylindrical shapes, and environmental sensitivity (Salavati-Niasari et al., 2008). Metal oxide–based nanoparticles are made to alter the properties of metal-based nanoparticles. The primary reason for the development of metal oxide nanoparticles is to improve their reactivity and efficiency. The most often synthesized oxides are aluminum oxide (Al2O3), cerium oxide (CeO2), iron oxide (Fe2O3), magnetite (Fe3O4), silicon dioxide (SiO2), titanium oxide (TiO2), and zinc oxide (ZnO). These nanoparticles have superior properties when compared to their metal counterparts (Tai et al., 2007; Ealia and Saravanakumar, 2017). Carbon-based nanoparticles are made completely of carbon. They can be classified into fullerenes, graphene, carbon nanotubes (CNTs), carbon nanofibers and carbon black, and sometimes activated carbon in nano size (Bhaviripudi et al., 2007; Ealia and Saravanakumar, 2017).
Crop losses as results of phyto pathogenesis account for about 16 percent of the total crop production worldwide (Fontana et al., 2021). The losses as a result of these pathogens are undisputable as they may be amplified further depending upon the predisposing factors, pathogen virulence, and environment. Agricultural crops suffer significant losses owing to pest damage and plant diseases, despite the fact that weeds are the leading cause of crop loss on a global scale. The global yearly production tonnage percentage lost to various pests at the start of the twenty-first century has been approximated as follows in rounded (approximate) figures: Animal pests accounted for 18% of crop production tonnage losses; microbiological illnesses accounted for 16% (and fungi were responsible for 70–80% of these losses); and weeds accounted for 34%, for a total of 68 percent annual crop production tonnage loss (Moore et al., 2020).
Silver nanoparticles (AgNPs) due to their antimicrobial properties have got marvelous attention (Ahmad et al., 2003; Wong and Liu, 2010; Foldbjerg et al., 2015; Guilger-Casagrande and Lima, 2019). AgNPs have got a good number of applications in controlling development of microorganisms (Wright et al., 1999; Silver, 2003; Nithya and Ragunathan, 2012; Zhao et al., 2018; Feroze et al., 2020; Sathiyaseelan et al., 2020). There are still a large number of potential applications for silver nanoparticles in various fields of health and agriculture. AgNPs synthesized from fungi are well applied in agriculture, showing good potentials against plant-pathogenic insects, fungi, bacteria, and viruses. The novel properties of silver nanoparticles in shape, size, and morphology have helped them to interact with different microorganisms, animals, and plants. Nanoparticles are more toxic in smaller size and even at a lesser concentration (Singh et al., 2015; Moradi et al., 2021). Silver nanoparticles are the most commercialized medical nanomaterial, widely used in medical product coatings, medical diagnostics, and sensors (Wijnhoven et al., 2009; Lin et al., 2011; Li et al., 2012; Sintubin et al., 2012; Burdușel et al., 2018). In commercial products, nanoparticles are primarily used in goods and self-care products that contain metals and metal oxides. The majority of global production is made up of nanoparticles of zinc oxide, titanium oxide, and silicon dioxide, with annual production rates of 5.50 tonnes, 3.00 tonnes, and 5,500 tonnes, respectively. Several estimates of global AgNPs production have been published in recent years. As of 2025, it is expected that AgNPs production will reach approximately 800 tonnes. As compared to other nanoparticles, AgNPs are thought to have a higher marketing value, and they are widely advertised in consumer products. Nanoparticles have proven to offer fascinating, demanding, and promising features suitable for many biomedical applications; silver nanoparticles (AgNPs) have become one of the most examined and explored nanotechnology-derived nanostructures in recent years. Modern biomedical potential of AgNPs is focused on therapeutically enhanced personalized healthcare practice, which has attracted a great deal of attention. As a result of their unique properties, AgNPs are proving to be a valuable tool for the development of novel antimicrobial agents as well as drug delivery formulations, detection platforms, and diagnostic platforms (Burdușel et al., 2018). Synthesis of AgNPs by using microorganisms has gained huge attention over chemical and physical methods since the biological method is ecofriendly (Thakkar et al., 2010; Ghosh et al., 2012; Gaidhani et al., 2014). Various plant diseases caused by fungi, bacteria, virus, and pests can be tackled by use of nanotechnology, which provides a good management alternative (Alghuthaymi et al., 2015).
AgNPs are formulated according to a wide range of variables, such as temperature, pH, media, and AgNO3 concentration (Figure 1). Other considerations, such as methods of preparation, solvent types, and media, depend on the physical characteristics of nanoparticles (Husen and Siddiqi, 2014; Siddiqi and Husen, 2016; 2017). Effectiveness of AgNPs not only depends on their physical characteristics, like sizes, shapes, and coating agents, but also on the type of pathogen against which they are formulated. Many pathogens are more prone to AgNPs than other nanoparticles. Several studies reported that bacterial pathogens were more susceptible than fungal pathogens to AgNPs. It was studied that the differences in antimicrobial activity of bacterial species and fungal species were due to differences in organization of their cells and cell structure. The bacteria, being among prokaryotic organisms, have less complexity in cell structure, therefore incapable to fight against toxicity of AgNPs. The fungi, however, have much more complex cell structure and better detoxification system, to effectively withstand the action of higher AgNPs concentrations (Panáček et al., 2009; Jalal et al., 2018). AgNPs surround the pathogen and inhibit its pivotal functioning by entering the cell. AgNPs can be effective at cellular and subcellular levels (Jayasree et al., 2006; Brayner, 2008; Panda et al., 2011; Ibrahim et al., 2021). AgNPs have broad-spectrum modes of action against different microorganisms, with a significant inhibitory effect on both Gram-positive and Gram-negative bacteria (Gogoi et al., 2006; Jo et al., 2009; Kim and Ryu, 2013).
Chemically vs Green-Synthesized Silver Nanoparticles
Chemical approach for synthesis of AgNPs is simple convenient and results in high yield. But this method is highly expensive and needs an additional step to prevent aggregation of particles (Zhang et al., 2016). Moreover, chemically synthesized AgNPs result in toxic effects of nanoparticles or by-products released to the environment (Gade et al., 2008). The chemical method requires involvement of toxic chemicals as reducing and capping agents, which leads to adsorption of these toxic chemicals on nanoparticles and results in adverse effects in application section (Kummara et al., 2016; Roy et al., 2019). On the contrary, biogenic synthesis of nanoparticles, especially using plant extract, utilizes less toxic reducing and stabilizing agents. The reaction can take place in ambient conditions without harsh or rigid reaction limitations. Plant extract–derived nanoparticles are of low or no cytotoxicity. Therefore, plant extract–mediated green synthesis of nanoparticles is considered ecofriendly, cost-effective, and safe and is a viable alternative for microbiological applications (Garibo et al., 2020). A previous study compared the antifungal activities of AgNPs synthesized by green and chemical methods (Tyagi et al., 2020). This study reported 100% inhibition against both Corynespora cassiicola and A. solani, and 85% inhibition against Fusarium spp., for chemically synthesized AgNPs; in contrast, green-synthesized AgNPs showed 90% inhibition against C. cassiicola, 95% inhibition against A. solani, and 90% inhibition against Fusarium spp. The antifungal activity for both types of nanoparticles depends on its dose and targeted fungal species. Moreover, toxicities for both types of AgNPs were analyzed against Drosophila melanogaster. Chemically synthesized AgNPs were toxic and their toxicity was dose-dependent, whereas green-synthesized AgNPs were not toxic. For four pathogens including C. albicans, the minimum inhibitory concentrations of green-synthesized AgNPs were from 0.06 μg/ml to 0.25 μg/ml, lower than those of chemically synthesized AgNPs from 2.5 μg/ml to 5.0 μg/ml, indicating a higher antifungal potency of green NPs than chemically synthesized NPs (Garibo et al., 2020).
Silver Nanoparticles Against Fungal Pathogens
As an alternative to conventional paths, a green synthesis of nanoparticles has evolved. Several green sources were identified, including microorganisms, viruses, natural polysaccharide reducing agents, polyols, and plant extracts. Green nanoparticle synthesis was done using a broad range of biological agents such as bacteria, fungi, algae, plant extracts, and a number of beneficial microorganisms (Narayanan and Sakthivel, 2010). Different plants for the formation of silver nanoparticles were previously investigated (Pulit-Prociak and Banach, 2016). Phyllanthus urinaria, Pouzolzia zeylanica, and Scoparia dulcis are herbs of great importance in the world, and have anti-bacteria, anti-inflammatory, anti-allergic, antioxidant, and many other potential activities (Hossain et al., 2017; Chen et al., 2018; Frezza et al., 2020). Under moderate conditions, three kinds of silver nanoparticles were actively biosynthesized (P.uri.AgNPs, P.zey.AgNPs, and S.dul.AgNPs), simply by applying silver (I) to these herbal plant minerals. P.uri.AgNPs, P.zey.AgNPs, and S.dul.AgNPs show antifungal capability against Aspergillus niger, A. flavus, and Fusarium oxysporum. This green approach is the most straightforward and widely scalable method for producing biomedical and agricultural antifungal silver nanoparticles (Nguyen et al., 2020). In addition, effective roles of AgNPs against Bipolaris and Magnapthe grisea have been reported previously (Morones et al., 2005; Panacek et al., 2006; Panáček et al., 2009). Therefore, silver nanoparticles have earned great attention in nanobiotechnological research, owing to their physical, chemical, and biological properties.
The leaf extract formulation of AgNPs using Acalphya indica have showed significant antifungal activity against pathogens such as Alternaria alternata, Botrytis cinerea, Curvularia lunata, Macrophomina phaseolina, Rhizoctonia solani, and Sclerotinia sclerotiorum (Krishnaraj et al., 2012; Medda et al., 2015). Green-synthesized AgNPs using leaf extract of Aloe vera showed a better antifungal activity against Aspergillus than Rhizopus (Medda et al., 2015). Microscopic observations showed that these synthesized nanoparticles had detrimental effects on fungal hyphae and conidial germination.
AgNPs synthesized using leaf extract of Brassica oleracea efficiently controlled the development of two pathogenic fungal pathogens, Aspergillus and Pneumocystis, at 50 μg/ml concentrations, with its inhibitory effect comparable to that of fluconazole (Kuppusamy et al., 2015). AgNPs synthesized from Cassia roxburghii leaf extract showed an excellent antifungal activity against five human fungal pathogens, A. fumigates, A. niger, A. flavus, Candida albicans, Penicillium sp., and three plant fungal pathogens, Curvularia sp., Rhizoctonia solani, and F. oxysporum (Balashanmugam et al., 2016). Amphotericin B was very effective against human fungal pathogens and moderately effective against plant fungal pathogens.
Some studies have reported antifungal action of AgNPs formulated using root extract of Diospyros sylvatica (Elumalai and Velmurugan, 2015; Pethakamsetty et al., 2017). These nanoparticles showed maximal activity against Pencillium notatum and A. niger, moderate activity toward Saccharomyces ceriviseae and A. flavus, and mild toward C. albicans. AgNPs synthesized from root extract of Diospyros paniculata showed significant antifungal potential against various pathogens with maximum activity toward A. flavus, P. notatum, and S. ceriviseae and moderate activity toward A. niger and C. albicans (Rao et al., 2016).
Silver Nanoparticles Against Fungal Biofilms
Many bacteria and fungi primarily emerge in cell crowds called biofilms. Biofilms can form on both biotic and abiotic surfaces, and have distinct properties from free-floating (planktonic) cells. Biofilms can be produced in a laboratory environment where they are commonly examined. More than one type of microorganisms (bacteria, protozoa, and fungi) in natural environments may grow in a synthrophic consortium (Lohse et al., 2018; Motaung et al., 2020). While fungal biofilms have been linked to animal and plant diseases, bacteria and yeast biofilms have received a lot of attention. It has been highlighted by mounting evidence that many fungal species are increasingly posing a threat to global food production, biodiversity, and human health. Pathogenic fungi cause significant yield losses in large calorie on commodity crops such as wheat, rice, sugarcane, corn, potato, and soybean around the world. There is a paucity of literature on plant-associated fungal biofilms, suggesting that the degree to which these consortia of microbial cells underpin plant health is underappreciated (Fanning and Mitchell, 2012; Motaung et al., 2020).
Biofilm formations are prevalent, virulent, or resistant factors of some opportunistic pathogens (bacteria or yeasts). Biofilm can be defined as a multidimensional assembly of microbes surrounded by an extracellular matrix (ECM) formed either on abiotic or biotic substrate (Fanning and Mitchell, 2012). The concept of plant-associated biofilms is mainly attributable to free-floating microbial cells, also known as planktonic cells, which are mainly responsible for commencing biofilm formation via initial attachment. Plant-associated fungal biofilms are generally thought to configure interactions across and among plant populations; butits-related research lags far behind as compared to the research on human-associated biofilms. Such deficit could limit the research avenues for plant improvement programs and disease management (Hassani et al., 2018; Motaung et al., 2020). The knowledge of plant-associated fungal biofilms could be pivotal for gaining information on various ecological roles such as medically important fungi, secondary metabolite production, plant beneficial functions, and climate change (Morris and Monier, 2003; Harding et al., 2017; Villa et al., 2017). Such functions could further be complimented with nanotechnology, wherein, nano-based approaches could be employed to plan tpathogens, thus giving a huge scope for agriculture exploration. One such directed approach is of silver nanoparticles (AgNPs), mainly attributable for their strong antimicrobial and anti-biofilm activity (Ali et al., 2020). As compared to plant-associated conditions, the use of AgNPs is highly widespread and multidirectional in oncology, nosocomial infections, and pulmonary diseases. The applicability of AgNPs to control plant-associated diseases is quite relative. Owing to their exceedingly small scale, AgNPs have tiny areas, high reactivity, and fast penetration of the biofilm materials and cell membranes with huge surface areas (Figure 2).
Jo et al. have examined the antifungal activity of various silver ions and nanoparticle on two plant-pathogenic fungi, Bipolaris sorokiniana and Magnaporthe grisea. They found that silver ions and nanoparticles had a significant inhibiting effect on the microbial colony formation of aforementioned pathogens via in vitro petri dish assays. In addition, growth chamber inoculation assays have concluded that silver ions and nanoparticles significantly reduce these two fungal diseases on perennial ryegrass (Lolium perenne) upon application at 3 h before spore inoculation (Jo et al., 2009). Ibrahim et al. have documented the use of green-synthesized AgNPs from garlic plants (Allium sativum) against Fusarium graminearum (head blight pathogen) on wheat crops. They have reported that AgNPs show strong inhibition on mycelium growth, spore germination, germ tube development, and mycotoxin production of F. graminearum (Ibrahim et al., 2020). Kaur et al. have reported the biosynthesis of silver nanoparticles (AgNPs) from isolated rhizospheric microflora of chickpea and evaluated their potential role in controlling wilt disease caused by F. oxysporum f. sp. ciceri (FOC) of chickpea. AgNPs showed a very high antifungal activity (95%) against FOC in vitro at the concentration of 100 μg/ml, and chickpea seeds coated with AgNPs showed high germinability (Kaur et al., 2018). Mishra et al. have obtained extracellularly synthesized AgNPs from the culture supernatant of the Serratia bacterium and demonstrated their efficacy for the management of Bipolaris sorokiniana, a causative agent of spot blotch disease on wheat. These AgNPs have exhibited strong antifungal activity with accountable inhibition of conidial germination as determined from a leaf bioassay (Fanning and Mitchell, 2012). Nanoparticles have gotten a lot of attention in the last decade because of their unusual properties. Since these particles are reactive, they can quickly penetrate the matrix. Non-toxic and environmentally safe methods are used in the synthesis of green NP. These AgNPs inhibit biofilm formation and cause the majority of P. aeruginosa biofilms to detach. Plant extracts are used to bio-reduce metal ions for NP biosynthesis, which is a cost-effective, fast, bacteria-free, and easy-to-scale-up operation (Qayyum and Khan, 2016). Although several studies have shown the anti-biofilm efficacy of metal NPs, their modes of action and toxicity remained to be elucidated.
Possible Modes of Action
Fungal cell wall plays an important role in maintaining cell homeostasis (Gow et al., 2017; Latgé, 2007). The surrounding environment of the fungal cell can play a positive or negative role that determines the survival rate of fungal cell. This cell wall contains a hard, durable, and tensile scaffold that is 40 percent of the total cell volume. Multitudes of proteins and carbohydrates support this system. The outer layer of the fungal cell wall contains mannosylated glycoproteins with modified N- and O-linked oligosaccharides (Shibata et al., 1995; Hobson et al., 2004). The inner layer contains chitin and glucan with 50–60% dry weight being β-(1–3)-glucan. The inner cell wall is pivotal in maintaining the cell environment by resisting the pressure created by the cell membrane and cytoplasm (Gow et al., 2017; Ghosh et al., 2012). Advances in recent studies on fungal cell wall composition, structure, and role in drug resistance have opened doors for new targets against fungal pathogens, and also helped in better understanding of mechanism by which antifungal resistance is developed. AgNPs might play a crucial role in breaking down such resistance. AgNPs cause cell wall disintegration, surface protein damage, nucleic acid damage by production and accumulation of ROS and free radicals, and blockage of proton pumps (Figure 3). It has hypothesized that AgNPs lead to accumulation of silver ions, which blocks respiration by efflux of intracellular ions and thus damages the electron transport system (Du et al., 2012). Smaller size to large surface ratio of nanoparticles is attributed for antifungal activity. AgNPs with smaller size can penetrate easily through cell boundaries. The toxicity of AgNPs is partially attributed through production of reactive oxygen species (ROS), which leads to apoptosis. It has been hypothesized that the in vitro toxicity of AgNPs is owing to either the mutual effect of Ag ions and AgNPs or their separate effect (Beer et al., 2012; Cronholm et al., 2013; Kim and Ryu, 2013). The exact mechanisms and modes of action of AgNPs need to be further explored.
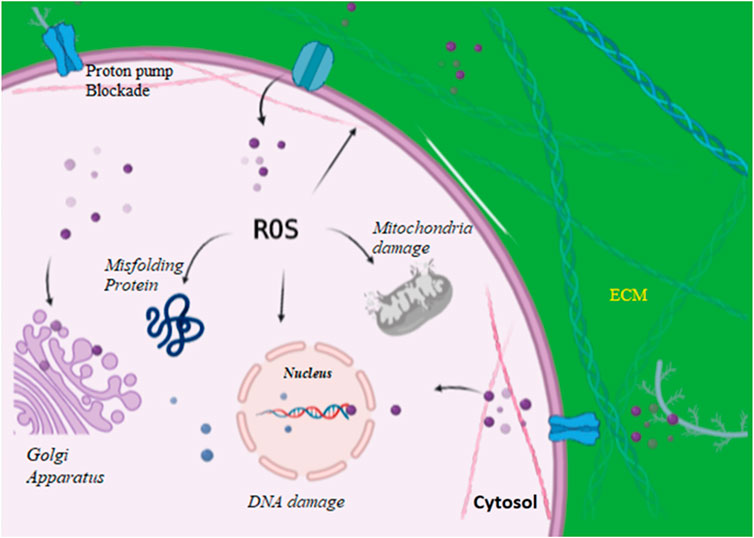
FIGURE 3. AgNPs enter into the cell via proton pumps and increasing reactive oxygen species thereby damaging intracellular organelles, blocking proton pumps, and causing damage to cell wall.
Potentials of Silver Nanoparticle in Agriculture
In agriculture, there is huge loss annually due to bacterial and fungal pathogens. One way to control such loss is the use of fungicides and pesticides, but these chemicals have detrimental effects on the environment. A large number of companies produce water-soluble nano-fertilizers, nano-pesticides, and nano herbicides of 100–250 nm and intelligent plant-nutrient delivery systems in agriculture. Nano-fertilizers should synch with the production of biomass to prevent a nutrient shortage (Prasad, 2014). The alternative to this is the synthesis and use of AgNPs as antifungal and antimicrobial agents, which is safer than the synthetic fungicides.
Silver nanoparticles have widely been reported to cause disease control in many agricultural crops; their efficiency has been seen to be controlled by the particle size and concentrations in the formulation (Steinfeld et al., 2015). In many cases, the silver nanoparticles have been seen to be more effective against the phytopathogens in the plant tissues itself than the in vitro conditions (Villamizar-Gallardo et al., 2016). Abdelmalek and Salaheldin (2016) reported promising fungicidal activity of AgNPs against Alternaria alternata, Penicillium digitatum, and Alternaria citri at 150 ppm compared to synthetic fungicides, difenoconazole, and iprodione at the same concentration (Abdelmalek and Salaheldin, 2016). Human health on exposure to silver nanoparticles and other nanomaterials as agriculture products is of major concerns. The direct contact of these nanomaterials used as nutritional ingredients may risk human health. The ROS (reactive oxidative species) production serves as the toxicological mechanisms leading to cellular damage and even death. ROS overproduction can cause damage to neuron, DNA damage, autophagy, severe age-related diseases, mutagenesis, and carcinogenesis in humans. Allergic reactions are also unfortunate outcomes upon the food nano-products exposure. Furthermore, the nanoparticles accumulation in human body and plant seeds (edible parts) may cause serious problems at long-term interactions and high concentrations. Legislation and regulation are most important in order to regulate the manufacturing of nanomaterials, their processing, application, and disposal (He et al., 2019). In agriculture and other sectors, the use of silver-based nano-emulsions have widely been questioned because of the apprehensions of lethal health effects on humans and other biological entities, the deliberate flow of nanoparticles into the different ecological niches could bring about the irreversible environmental degradation, and accumulation of these substances in the food chain (Kah, 2015). These emulsions not only target the non-parasitic/friendly entities but accumulate in the foods itself. The apprehensions in context to agricultural laborers getting into contact with these harmful xenobiotics could put their life at risk (Iavicoli et al., 2017). Although large work is carried on effects of AgNPs on bacteria, not much attention has been yet received on their role on antifungal effects (Kim et al., 2008; Roe et al., 2008). Antifungal potential of Ag-SiO2 has been reported against Botrytis cinereal (Oh et al., 2006). The mutual effect of fluconazole and AgNPs were tested for their antifungal activity against Phoma glomerata, P. herbarum, F. semitectum, Trichoderma sp., and C. albicans (Gajbhiye et al., 2009). AgNPs were also reported to decrease disease development of plant fungal pathogens including B. sorokiniana, Magnaportheas as well as sclerotium-forming phytopathogenic fungi Grisea, gloeosporioides, A. niger, and Sclerotium cepivorum (Park et al., 2006; Kim et al., 2009; Min et al., 2009; Choudhury et al., 2010). AgNPs also exhibit a significant antifungal activity against F. oxysporum and the unknown ambrosia fungus Raffaelea sp. that causes death of large oak trees in Korea (Wijnhoven et al., 2009; Musarrat et al., 2010). AgNPs were also demonstrated to be effective against plant fungal pathogens, A. alternata, S. sclerotiorum, Macrophomina phaseolina, Rhizoctonia solani, B. cinerea, and Curvularia lunata (Krishnaraj et al., 2012; Alghuthaymi et al., 2015). AgNPs prepared by using Fusarium solani from wheat proved to be very effective against different pathogens of wheat, barley, and maize (Abd El-Aziz et al., 2015). AgNPs synthesized from the fungus Aspergilus versicolor showed concentration-dependent activity against S. sclerotiorum and B. cinereaon strawberry plant (Elgorban A. et al., 2016). The fungus Epicoccum nigrumcan can be used to synthesize AgNPs against C. albicans and F. solani (Qian et al., 2013). Potential roles of the fungus Guignardia mangiferae-derived AgNPs were tested to control plant pathogens Colletotrichum sp., R. solani, and Curvularia lunata (Balakumaran et al., 2015). A combination of A. alternata-derivedAgNPs and fluconazole was evaluated against the phytopathogenic fungi P. glomerata, P. herbarum, and F. semitectum, as well as the human pathogenic fungus C. albicans, with the highest activity to C. albicans (Gajbhiye et al., 2009).
Silver nanoparticles play a significant role in control of fungal pathogens in many crops (Al-Zubaidi et al., 2019). Antifungal activity of silver nanoparticles derived from Agaricus bisporus against various fungal strains like Sclerotium rolfsi, A. niger, and Rhizoctonia solani which are known to be the causal agents of stem, collar, and root rot disease, respectively, in groundnut (Roy et al., 2013). Rao and Savitramma examined the effectiveness of naturally orchestrated silver nanoparticles from Svensonia hyderabadensis leaf extracts against F. oxysporum, Curvularia lunata, A. niger, and Rhizopus arrhizus (Rao and Savithramma, 2011).
Silver nanoparticles synthesized from turnip leaf extraction demonstrated a wide range of antifungal action against wood-degrading fungi including Gloeophyllum abietinum, G. trabeum, Chaetomium globosum, and Phanerochaete sordida (Narayanan and Park, 2014). AgNPs were also synthesized successfully with leaf extracts from C. roxburghii and could be used as an antifungal agent to treat various pathogenic diseases of humans and plants (Rai et al., 2021; Balashanmugam et al., 2016; Table 1).
Biosafety of AgNPs
Despite the numerous benefits of nanoscale materials, their possible health risks must be considered due to their uncontrollable use, discharge into the natural environment, and hazardous consequences. Aside from their numerous commercial and medical applications, NPs and other nanomaterials are linked to a number of toxicities. To deal with these hazardous effects appropriately, you will need some basic understanding. NPs enter the environment through water, soil, and the air during a variety of human activities. Instead, NPs are actively injected or dumped into soil and aquatic systems. Small size, high reactivity, and high capacity are advantages of magnetic nanoparticles (NPs), but they can also be disadvantages because they can have toxic and detrimental effects on cells, which are rare in micron-sized counterparts (Xu et al., 2020). Commercial and biomedical applications of nanosilver include its use in cosmetics and textile engineering, as well as a bactericidal agent in dressings and disinfectants for wounds or surgical instruments. As a result, AgNPs interactions with terrestrial and aquatic environments, as well as human exposure and toxicity, have increased. A number of factors influence the cytotoxic effects of AgNPs, including size, shape, coating, dose, and cell type (Tang and Xi, 2008; Khlebtsov and Dykman, 2011; El-Sheikh et al., 2013; Xu et al., 2020).
There is a lack of appropriate and standard characterization procedures that may be adopted for research evaluating the toxicity of AgNPs in order to compare the results of different investigations utilizing similar NPs. The data on the impact of AgNPs exposure on animal models of increased vulnerability, such as hypertension, diabetes, and asthma, are scarce. The cytotoxicity of AgNPs is determined by their size, shape, and concentration. Wang et al. (2015) employed TEM and SR-TXM combined with 3D tomographic imaging to explore AgNPs uptake, accumulation, degradation, chemical change, and elimination at the cellular level. According to the findings, the chemical transformation of AgNPs (Ag0-to-AgO-to-AgS) may produce cellular metabolic alterations. The possible cytotoxicity, long-term health impacts, and particular mechanisms of AgNPs are still unknown. Furthermore, AgNPs can cause acute lung injury, the severity of which is determined by particle accumulation and clearance. Akinori et al. employed mouse models to investigate the lung toxicity of nanoparticles. Ultrafine particles may pass across the air–blood barrier due to the narrow space between alveolar epithelial cells, producing bronchiolar epithelial cell vacuolation and necrosis, resulting in transitory acute lung inflammation and tissue damage. Because ultrafine particles promote oxidative stress and cell death, this is the case. Size-dependent lung toxicity was also observed for nanoparticles, meaning that smaller particles were more capable of causing lung inflammation and tissue damage than larger particles (Kaewamatawong et al., 2005; Kaewamatawong et al., 2006). There has been some discussion of AgNPs’ potential toxicities in multiple organ systems including the skin, kidneys, respiratory system, hepatobiliary, and immune systems. AgNPs’ biocompatibility and potential cytotoxicity must be evaluated in more detail, which could lead to the development of safer and biocompatible AgNPs-based agents.
Before mass manufacture and use of NPs in the food business, precise and standard tests should be used to assess the long-term consequences of acute and chronic exposure to various NPs found in food systems.
Conclusion and Future Prospective
Agriculture forms the backbone of economy in most of the countries. AgNPs have been studied comprehensively from the last few decades, and use of AgNPs in agriculture provides alternative protection mean against pests, which could reduce our dependence on environmentally detrimental fungicides and pesticides. Extracts from fruits and some medicinal plants serve as an easy and cheap means for AgNPs biosynthesis. Yet, for large-scale production of AgNPs, there is a need for a more reliable route, which is environmentally sound as well as economically viable. Although the mechanism of AgNPs synthesis from plant extracts is still not clear, but some studies mention the formation and mechanism of nanoparticles from a diverse range of plant species successfully (Simchi et al., 2007; Mittal et al., 2013; Al‐Rashed et al., 2019). AgNPs are extensively used as antimicrobial agents and biosensors in medicine, healthcare, and agriculture sectors. Precise modes of action of AgNPs against fungal pathogens need to be elucidated with experiments. AgNPs can be modified and engineered in order to enhance its stability, efficacy, and biosafety. Nanoparticles fabricated and coated with cappings derived from fungi have exhibited great biological activity. Mycosynthesized AgNPs have been reported to disintegrate membrane integrity in fungal cells. Different formulations of mycosynthesized AgNPs must be tested for their biological activities in various fields. Although the role of silver nanoparticles against various fungal pathogens has been established, there are large number of fungal pathogens responsible for huge loss in agricultural productivity which need to be tested against AgNPs. Future research should focus on the discovery of potential candidates and mechanisms involved in their actions, taking into consideration their effects on the living and non-living components of the environment before their large-scale production. Keeping in view of their toxic effects, rational strategies with minimum risk of toxicity need to be designed. Work must be done to promote the development of nanobiosensors and nano-based delivery systems, which are user friendly and easy to carry out for precise analysis of soil, plants, and water. Efforts should be made for their use in plant disease management on account of being a cost-effective and ecofriendly alternative. There are many studies that endorse NPs as anti-biofilm agents, but there are still some know-hows about their modes of operation. Using NPs as anti-biofilm agents to manage biofilm-mediated pathogens can help increase plant life and human health in the future.
Author Contributions
Conceptualization, SM and LJ; software SM; validation, formal analysis, investigation, data curation; SM, IZ, TRB, SAP, ZAB, AMK, and LJ; writing—original draft preparation, SM, IZ, and LJ; review and editing TRB, SAP, ZAB, AMK, and LJ. supervision LJ.
Conflict of Interest
The authors declare that the research was conducted in the absence of any commercial or financial relationships that could be construed as a potential conflict of interest.
Publisher’s Note
All claims expressed in this article are solely those of the authors and do not necessarily represent those of their affiliated organizations, or those of the publisher, the editors and the reviewers. Any product that may be evaluated in this article, or claim that may be made by its manufacturer, is not guaranteed or endorsed by the publisher.
References
Abd El-Aziz, A., Al-Othman, M., Mahmoud, M., and Metwaly, H. (2015). Biosynthesis of Silver Nanoparticles Using Fusarium Solani and its Impact on Grain Borne Fungi. Dig. J. Nanomater. Biostructures 10, 655–662.
Abdelmalek, G., and Salaheldin, T. (2016). Silver Nanoparticles as a Potent Fungicide for Citrus Phytopathogenic Fungi. J. Nanomedicine Res. 3, 00065. doi:10.15406/jnmr.2016.03.00065
Ahmad, A., Mukherjee, P., Senapati, S., Mandal, D., Khan, M. I., Kumar, R., et al. (2003). Extracellular Biosynthesis of Silver Nanoparticles Using the Fungus Fusarium Oxysporum. Colloids Surf. B: Biointerfaces 28, 313–318. doi:10.1016/s0927-7765(02)00174-1
Al‐Rashed, M. M., Niknezhad, S., and Jana, S. C. (2019). Mechanism and Factors Influencing Formation and Stability of Chitosan/lignosulfonate Nanoparticles. Macromolecular Chem. Phys. 220, 1800338. doi:10.1002/macp.201800338
Al-Zubaidi, S., Al-Ayafi, A., and Abdelkader, H. (2019). Biosynthesis, Characterization and Antifungal Activity of Silver Nanoparticles by Aspergillus niger Isolate. J. Nanotechnology Res. 1, 23–36. doi:10.26502/jnr.2688-8521002
Alghuthaymi, M. A., Almoammar, H., Rai, M., Said-Galiev, E., and Abd-Elsalam, K. A. (2015). Myconanoparticles: Synthesis and Their Role in Phytopathogens Management. Biotechnol. Biotechnological Equipment 29, 221–236. doi:10.1080/13102818.2015.1008194
Ali, M. A., Ahmed, T., Wu, W., Hossain, A., Hafeez, R., Islam Masum, M. M., et al. (2020). Advancements in Plant and Microbe-Based Synthesis of Metallic Nanoparticles and Their Antimicrobial Activity against Plant Pathogens. Nanomaterials 10, 1146. doi:10.3390/nano10061146
Bahrami-Teimoori, B., Nikparast, Y., Hojatianfar, M., Akhlaghi, M., Ghorbani, R., and Pourianfar, H. R. (2017). Characterisation and Antifungal Activity of Silver Nanoparticles Biologically Synthesised by Amaranthus Retroflexus Leaf Extract. J. Exp. Nanoscience 12, 129–139. doi:10.1080/17458080.2017.1279355
Balakumaran, M. D., Ramachandran, R., and Kalaichelvan, P. T. (2015). Exploitation of Endophytic Fungus, Guignardia Mangiferae for Extracellular Synthesis of Silver Nanoparticles and Their In Vitro Biological Activities. Microbiol. Res. 178, 9–17. doi:10.1016/j.micres.2015.05.009
Balashanmugam, P., Balakumaran, M. D., Murugan, R., Dhanapal, K., and Kalaichelvan, P. T. (2016). Phytogenic Synthesis of Silver Nanoparticles, Optimization and Evaluation of In Vitro Antifungal Activity against Human and Plant Pathogens. Microbiol. Res. 192, 52–64. doi:10.1016/j.micres.2016.06.004
Beer, C., Foldbjerg, R., Hayashi, Y., Sutherland, D. S., and Autrup, H. (2012). Toxicity of Silver Nanoparticles-Nanoparticle or Silver Ion? Toxicol. Lett. 208, 286–292. doi:10.1016/j.toxlet.2011.11.002
Bhaviripudi, S., Mile, E., Steiner, S. A., Zare, A. T., Dresselhaus, M. S., Belcher, A. M., et al. (2007). CVD Synthesis of Single-Walled Carbon Nanotubes from Gold Nanoparticle Catalysts. J. Am. Chem. Soc. 129, 1516–1517. doi:10.1021/ja0673332
Brayner, R. (2008). The Toxicological Impact of Nanoparticles. Nano Today 3, 48–55. doi:10.1016/s1748-0132(08)70015-x
Burdușel, A.-C., Gherasim, O., Grumezescu, A. M., Mogoantă, L., Ficai, A., and Andronescu, E. (2018). Biomedical Applications of Silver Nanoparticles: an Up-To-Date Overview. Nanomaterials 8, 681. doi:10.3390/nano8090681
Chen, X.-M., Li, Z.-H., Tao, S.-H., Chen, Y.-F., Chen, Z.-H., and Guo, L.-B. (2018). Effect of FPZ, a Total Flavonoids Ointment Topical Application from Pouzolzia Zeylanica Var. Microphylla, on Mice Skin Infections. Revista Brasileira de Farmacognosia 28, 732–737. doi:10.1016/j.bjp.2018.08.009
Choudhury, S. R., Nair, K. K., Kumar, R., Gogoi, R., Srivastava, C., Gopal, M., et al. (2010). Nanosulfur: a Potent Fungicide against Food Pathogen, Aspergillus niger. AIP Conf. Proc. 1276, 154–157. doi:10.1063/1.3504287
Cronholm, P., Karlsson, H. L., Hedberg, J., Lowe, T. A., Winnberg, L., Elihn, K., et al. (2013). Intracellular Uptake and Toxicity of Ag and CuO Nanoparticles: a Comparison between Nanoparticles and Their Corresponding Metal Ions. Small 9, 970–982. doi:10.1002/smll.201201069
Du, H., Lo, T.-M., Sitompul, J., and Chang, M. W. (2012). Systems-level Analysis of Escherichia coli Response to Silver Nanoparticles: the Roles of Anaerobic Respiration in Microbial Resistance. Biochem. biophysical Res. Commun. 424, 657–662. doi:10.1016/j.bbrc.2012.06.134
Ealia, S. a. M., and Saravanakumar, M. (2017). A Review on the Classification, Characterisation, Synthesis of Nanoparticles and Their Application. IOP Conf. Ser. Mater. Sci. Eng. 263, 032019. doi:10.1088/1757-899X/263/3/032019
El-Moslamy, S. H., Elkady, M. F., Rezk, A. H., and Abdel-Fattah, Y. R. (2017). Applying Taguchi Design and Large-Scale Strategy for Mycosynthesis of Nano-Silver from Endophytic Trichoderma harzianum SYA.F4 and its Application against Phytopathogens. Sci. Rep. 7, 45297. doi:10.1038/srep45297
El-Sheikh, M., El Gabry, L., and Ibrahim, H. (2013). Photosynthesis of Carboxymethyl Starch-Stabilized Silver Nanoparticles and Utilization to Impart Antibacterial Finishing for Wool and Acrylic Fabrics. J. Polym. 2013, 792035. doi:10.1155/2013/792035
Elgorban, A., Aref, S., Seham, S., Elhindi, K., Bahkali, A., Sayed, S., et al. (2016a). Extracellular Synthesis of Silver Nanoparticles Using Aspergillus versicolor and Evaluation of Their Activity on Plant Pathogenic Fungi. Mycosphere 7, 844–852. doi:10.5943/mycosphere/7/6/15
Elgorban, A. M., Al-Rahmah, A. N., Sayed, S. R., Hirad, A., Mostafa, A. A.-F., and Bahkali, A. H. (2016b). Antimicrobial Activity and green Synthesis of Silver Nanoparticles usingTrichoderma Viride. Biotechnol. Biotechnological Equipment 30, 299–304. doi:10.1080/13102818.2015.1133255
Elumalai, K., and Velmurugan, S. (2015). Green Synthesis, Characterization and Antimicrobial Activities of Zinc Oxide Nanoparticles from the Leaf Extract of Azadirachta indica (L.). Appl. Surf. Sci. 345, 329–336. doi:10.1016/j.apsusc.2015.03.176
Fanning, S., and Mitchell, A. P. (2012). Fungal Biofilms. Plos Pathog. 8, e1002585. doi:10.1371/journal.ppat.1002585
Feroze, N., Arshad, B., Younas, M., Afridi, M. I., Saqib, S., and Ayaz, A. (2020). Fungal Mediated Synthesis of Silver Nanoparticles and Evaluation of Antibacterial Activity. Microsc. Res. Tech. 83, 72–80. doi:10.1002/jemt.23390
Foldbjerg, R., Jiang, X., Miclăuş, T., Chen, C., Autrup, H., and Beer, C. (2015). Silver Nanoparticles - Wolves in Sheep's Clothing? Toxicol. Res. 4, 563–575. doi:10.1039/c4tx00110a
Fontana, D. C., De Paula, S., Torres, A. G., De Souza, V. H. M., Pascholati, S. F., Schmidt, D., et al. (2021). Endophytic Fungi: Biological Control and Induced Resistance to Phytopathogens and Abiotic Stresses. Pathogens 10, 570. doi:10.3390/pathogens10050570
Frezza, C., Venditti, A., Toniolo, C., De Vita, D., Franceschin, M., Ventrone, A., et al. (2020). Nor-Lignans: Occurrence in Plants and Biological Activities-A Review. Molecules 25, 197. doi:10.3390/molecules25010197
Gade, A. K., Bonde, P., Ingle, A. P., Marcato, P. D., Durán, N., and Rai, M. K. (2008). Exploitation of Aspergillus niger for Synthesis of Silver Nanoparticles. J. Biobased Mat Bioenergy 2, 243–247. doi:10.1166/jbmb.2008.401
Gaidhani, S. V., Raskar, A. V., Poddar, S., Gosavi, S., Sahu, P. K., Pardesi, K. R., et al. (2014). Time Dependent Enhanced Resistance against Antibiotics & Metal Salts by Planktonic & Biofilm Form of Acinetobacter Haemolyticus MMC 8 Clinical Isolate. Indian J. Med. Res. 140, 665–671.
Gajbhiye, M., Kesharwani, J., Ingle, A., Gade, A., and Rai, M. (2009). Fungus-mediated Synthesis of Silver Nanoparticles and Their Activity against Pathogenic Fungi in Combination with Fluconazole. Nanomedicine: Nanotechnology, Biol. Med. 5, 382–386. doi:10.1016/j.nano.2009.06.005
Garibo, D., Borbón-Nuñez, H. A., de León, J. N. D., García Mendoza, E., Estrada, I., Toledano-Magaña, Y., et al. (2020). Green Synthesis of Silver Nanoparticles Using Lysiloma Acapulcensis Exhibit High-Antimicrobial Activity. Sci. Rep. 10, 12805–12811. doi:10.1038/s41598-020-69606-7
Ghojavand, S., Madani, M., and Karimi, J. (2020). Green Synthesis, Characterization and Antifungal Activity of Silver Nanoparticles Using Stems and Flowers of Felty Germander. J. Inorg. Organomet. Polym. 30, 2987–2997. doi:10.1007/s10904-020-01449-1
Ghosh, S., Patil, S., Ahire, M., Kitture, R., Kale, S., Pardesi, K., et al. (2012). Synthesis of Silver Nanoparticles Using Dioscorea Bulbifera Tuber Extract and Evaluation of its Synergistic Potential in Combination with Antimicrobial Agents. Int. J. Nanomedicine 7, 483–496. doi:10.2147/IJN.S24793
Gogoi, S. K., Gopinath, P., Paul, A., Ramesh, A., Ghosh, S. S., and Chattopadhyay, A. (2006). Green Fluorescent Protein-ExpressingEscherichiacolias a Model System for Investigating the Antimicrobial Activities of Silver Nanoparticles. Langmuir 22, 9322–9328. doi:10.1021/la060661v
Gow, N. R., Latge, J.-P., and Munro, C. A. (2017). The Fungal Cell wall: Structure, Biosynthesis, and Function. Microbiol. Spectr. 5. doi:10.1128/microbiolspec.FUNK-0035-2016
Guilger, M., Pasquoto-Stigliani, T., Bilesky-Jose, N., Grillo, R., Abhilash, P. C., Fraceto, L. F., et al. (2017). Biogenic Silver Nanoparticles Based on Trichoderma harzianum: Synthesis, Characterization, Toxicity Evaluation and Biological Activity. Sci. Rep. 7, 44421. doi:10.1038/srep44421
Guilger-Casagrande, M., and Lima, R. d. (2019). Synthesis of Silver Nanoparticles Mediated by Fungi: a Review. Front. Bioeng. Biotechnol. 7, 287. doi:10.3389/fbioe.2019.00287
Harding, M. W., Marques, L. L., Shore, B., and Daniels, G. (2017). The Significance of Fungal Biofilms in Association with Plants and Soils. Chichester, UK: John Wiley & Sons.
Hassani, M. A., Durán, P., and Hacquard, S. (2018). Microbial Interactions within the Plant Holobiont. Microbiome 6, 58–17. doi:10.1186/s40168-018-0445-0
He, X., Deng, H., and Hwang, H.-M. (2019). The Current Application of Nanotechnology in Food and Agriculture. J. Food Drug Anal. 27, 1–21. doi:10.1016/j.jfda.2018.12.002
Hobson, R. P., Munro, C. A., Bates, S., Maccallum, D. M., Cutler, J. E., Heinsbroek, S. E. M., et al. (2004). Loss of Cell wall Mannosylphosphate in Candida Albicans Does Not Influence Macrophage Recognition. J. Biol. Chem. 279, 39628–39635. doi:10.1074/jbc.m405003200
Hossain, M. S., Rahman, M. S., Imon, A. R., Zaman, S., Siddiky, A. B. A., Mondal, M., et al. (2017). Ethnopharmacological Investigations of Methanolic Extract of Pouzolzia Zeylanica (L.) Benn. Clin. Phytoscience 2, 1–10. doi:10.1186/s40816-016-0022-7
Husen, A., and Siddiqi, K. S. (2014). Phytosynthesis of Nanoparticles: Concept, Controversy and Application. Nanoscale Res. Lett. 9, 229. doi:10.1186/1556-276x-9-229
Iavicoli, I., Leso, V., Beezhold, D. H., and Shvedova, A. A. (2017). Nanotechnology in Agriculture: Opportunities, Toxicological Implications, and Occupational Risks. Toxicol. Appl. Pharmacol. 329, 96–111. doi:10.1016/j.taap.2017.05.025
Ibrahim, E., Zhang, M., Zhang, Y., Hossain, A., Qiu, W., Chen, Y., et al. (2020). Green-synthesization of Silver Nanoparticles Using Endophytic Bacteria Isolated from Garlic and its Antifungal Activity against Wheat Fusarium Head Blight Pathogen Fusarium Graminearum. Nanomaterials 10, 219. doi:10.3390/nano10020219
Ibrahim, S., Ahmad, Z., Manzoor, M. Z., Mujahid, M., Faheem, Z., and Adnan, A. (2021). Optimization for Biogenic Microbial Synthesis of Silver Nanoparticles through Response Surface Methodology, Characterization, Their Antimicrobial, Antioxidant, and Catalytic Potential. Scientific Rep. 11, 1–18. doi:10.1038/s41598-020-80805-0
Ishida, K., Cipriano, T. F., Rocha, G. M., Weissmüller, G., Gomes, F., Miranda, K., et al. (2014). Silver Nanoparticle Production by the Fungus Fusarium Oxysporum: Nanoparticle Characterisation and Analysis of Antifungal Activity against Pathogenic Yeasts. Mem. Inst. Oswaldo Cruz 109, 220–228. doi:10.1590/0074-0276130269
Jalal, M., Ansari, M., Alzohairy, M., Ali, S., Khan, H., Almatroudi, A., et al. (2018). Biosynthesis of Silver Nanoparticles from Oropharyngeal Candida Glabrata Isolates and Their Antimicrobial Activity against Clinical Strains of Bacteria and Fungi. Nanomaterials 8, 586. doi:10.3390/nano8080586
Jayasree, L., Janakiram, P., and Madhavi, R. (2006). Characterization of Vibrio Spp. Associated with Diseased Shrimp from Culture Ponds of Andhra Pradesh (India). J. World Aquacult. Soc 37, 523–532. doi:10.1111/j.1749-7345.2006.00066.x
Jo, Y.-K., Kim, B. H., and Jung, G. (2009). Antifungal Activity of Silver Ions and Nanoparticles on Phytopathogenic Fungi. Plant Dis. 93, 1037–1043. doi:10.1094/pdis-93-10-1037
Kaewamatawong, T., Kawamura, N., Okajima, M., Sawada, M., Morita, T., and Shimada, A. (2005). Acute Pulmonary Toxicity Caused by Exposure to Colloidal Silica: Particle Size Dependent Pathological Changes in Mice. Toxicol. Pathol. 33, 745–751. doi:10.1080/01926230500416302
Kaewamatawong, T., Shimada, A., Okajima, M., Inoue, H., Morita, T., Inoue, K., et al. (2006). Acute and Subacute Pulmonary Toxicity of Low Dose of Ultrafine Colloidal Silica Particles in Mice after Intratracheal Instillation. Toxicol. Pathol. 34, 958–965. doi:10.1080/01926230601094552
Kah, M. (2015). Nanopesticides and Nanofertilizers: Emerging Contaminants or Opportunities for Risk Mitigation? Front. Chem. 3, 64. doi:10.3389/fchem.2015.00064
Kaur, P., Thakur, R., Duhan, J. S., and Chaudhury, A. (2018). Management of Wilt Disease of Chickpea In Vivo by Silver Nanoparticles Biosynthesized by Rhizospheric Microflora of Chickpea (Cicer Arietinum ). J. Chem. Technol. Biotechnol. 93, 3233–3243. doi:10.1002/jctb.5680
Khan, I., Saeed, K., and Khan, I. (2019). Nanoparticles: Properties, Applications and Toxicities. Arabian J. Chem. 12, 908–931. doi:10.1016/j.arabjc.2017.05.011
Khan, T., Yasmin, A., and Townley, H. E. (2020). An Evaluation of the Activity of Biologically Synthesized Silver Nanoparticles against Bacteria, Fungi and Mammalian Cell Lines. Colloids Surf. B: Biointerfaces 194, 111156. doi:10.1016/j.colsurfb.2020.111156
Khlebtsov, N., and Dykman, L. (2011). Biodistribution and Toxicity of Engineered Gold Nanoparticles: a Review of In Vitro and In Vivo Studies. Chem. Soc. Rev. 40, 1647–1671. doi:10.1039/c0cs00018c
Kim, H. S., Kang, H. S., Chu, G. J., and Byun, H. S. (2008). Antifungal Effectiveness of Nanosilver Colloid against Rose Powdery Mildew in Greenhouses. Solid State Phenomena 135, 15–18. doi:10.4028/www.scientific.net/SSP.135.15
Kim, S., and Ryu, D.-Y. (2013). Silver Nanoparticle-Induced Oxidative Stress, Genotoxicity and Apoptosis in Cultured Cells and Animal Tissues. J. Appl. Toxicol. 33, 78–89. doi:10.1002/jat.2792
Kim, S. W., Kim, K. S., Lamsal, K., Kim, Y. J., Kim, S. B., Jung, M., et al. (2009). An In Vitro Study of the Antifungal Effect of Silver Nanoparticles on Oak Wilt Pathogen Raffaelea Sp. J. Microbiol. Biotechnol. 19, 760–764. doi:10.4014/jmb.0812.649
Kischkel, B., Castilho, P. F. d., De Oliveira, K. M., Rezende, P. S., Bruschi, M. L., Svidzinski, T. I., et al. (2020). Silver Nanoparticles Stabilized with Propolis Show Reduced Toxicity and Potential Activity against Fungal Infections. Future Microbiol. 15, 521–539. doi:10.2217/fmb-2019-0173
Krishnaraj, C., Ramachandran, R., Mohan, K., and Kalaichelvan, P. T. (2012). Optimization for Rapid Synthesis of Silver Nanoparticles and its Effect on Phytopathogenic Fungi. Spectrochimica Acta A: Mol. Biomol. Spectrosc. 93, 95–99. doi:10.1016/j.saa.2012.03.002
Kummara, S., Patil, M. B., and Uriah, T. (2016). Synthesis, Characterization, Biocompatible and Anticancer Activity of green and Chemically Synthesized Silver Nanoparticles - A Comparative Study. Biomed. Pharmacother. 84, 10–21. doi:10.1016/j.biopha.2016.09.003
Kuppusamy, P., Ichwan, S. J. A., Parine, N. R., Yusoff, M. M., Maniam, G. P., and Govindan, N. (2015). Intracellular Biosynthesis of Au and Ag Nanoparticles Using Ethanolic Extract of Brassica oleracea L. And Studies on Their Physicochemical and Biological Properties. J. Environ. Sci. 29, 151–157. doi:10.1016/j.jes.2014.06.050
Latgé, J. P. (2007). The Cell wall: a Carbohydrate armour for the Fungal Cell. Mol. Microbiol. 66, 279–290. doi:10.1111/j.1365-2958.2007.05872.x
Li, L., Sun, J., Li, X., Zhang, Y., Wang, Z., Wang, C., et al. (2012). Controllable Synthesis of Monodispersed Silver Nanoparticles as Standards for Quantitative Assessment of Their Cytotoxicity. Biomaterials 33, 1714–1721. doi:10.1016/j.biomaterials.2011.11.030
Lin, J., Chen, R., Feng, S., Pan, J., Li, Y., Chen, G., et al. (2011). A Novel Blood Plasma Analysis Technique Combining Membrane Electrophoresis with Silver Nanoparticle-Based SERS Spectroscopy for Potential Applications in Noninvasive Cancer Detection. Nanomedicine: Nanotechnology, Biol. Med. 7, 655–663. doi:10.1016/j.nano.2011.01.012
Lohse, M. B., Gulati, M., Johnson, A. D., and Nobile, C. J. (2018). Development and Regulation of Single- and Multi-Species Candida Albicans Biofilms. Nat. Rev. Microbiol. 16, 19–31. doi:10.1038/nrmicro.2017.107
Medda, S., Hajra, A., Dey, U., Bose, P., and Mondal, N. K. (2015). Biosynthesis of Silver Nanoparticles from Aloe Vera Leaf Extract and Antifungal Activity against Rhizopus Sp. And Aspergillus Sp. Appl. Nanosci 5, 875–880. doi:10.1007/s13204-014-0387-1
Min, J.-S., Kim, K.-S., Kim, S.-W., Jung, J.-H., Lamsal, K., Kim, S.-B., et al. (2009). Effects of Colloidal Silver Nanoparticles on Sclerotium-Forming Phytopathogenic Fungi. Plant Pathol. J. 25, 376–380. doi:10.5423/ppj.2009.25.4.376
Mittal, A. K., Chisti, Y., and Banerjee, U. C. (2013). Synthesis of Metallic Nanoparticles Using Plant Extracts. Biotechnol. Adv. 31, 346–356. doi:10.1016/j.biotechadv.2013.01.003
Moazeni, M., Rashidi, N., Shahverdi, A. R., Noorbakhsh, F., and Rezaie, S. (2012). Extracellular Production of Silver Nanoparticles by Using Three Common Species of Dermatophytes: Trichophyton Rubrum, Trichophyton Mentagrophytes and Microsporum canis. Iran Biomed. J. 16, 52–58. doi:10.6091/ibj.1001.2012
Mohseniazar, M., Barin, M., Zarredar, H., Alizadeh, S., and Shanehbandi, D. (2011). Potential of Microalgae and Lactobacilli in Biosynthesis of Silver Nanoparticles. Bioimpacts 1, 149–152. doi:10.5681/bi.2011.020
Moore, D., Robson, G. D., and Trinci, A. P. (2020). 21st century Guidebook to Fungi. Cambridge: Cambridge University Press.
Moradi, F., Sedaghat, S., Moradi, O., and Arab Salmanabadi, S. (2021). Review on green Nano-Biosynthesis of Silver Nanoparticles and Their Biological Activities: with an Emphasis on Medicinal Plants. Inorg. Nano-Metal Chem. 51, 133–142. doi:10.1080/24701556.2020.1769662
Morones, J. R., Elechiguerra, J. L., Camacho, A., Holt, K., Kouri, J. B., Ramírez, J. T., et al. (2005). The Bactericidal Effect of Silver Nanoparticles. Nanotechnology 16, 2346–2353. doi:10.1088/0957-4484/16/10/059
Morris, C. E., and Monier, J.-M. (2003). Theecologicalsignificance Ofbiofilmformation Byplant-Associatedbacteria. Annu. Rev. Phytopathol. 41, 429–453. doi:10.1146/annurev.phyto.41.022103.134521
Mosselhy, D. A., Abd El-Aziz, M., Hanna, M., Ahmed, M. A., Husien, M. M., and Feng, Q. (2015). Comparative Synthesis and Antimicrobial Action of Silver Nanoparticles and Silver Nitrate. J. Nanoparticle Res. 17, 1–10. doi:10.1007/s11051-015-3279-8
Motaung, T. E., Peremore, C., Wingfield, B., and Steenkamp, E. (2020). Plant-associated Fungal Biofilms-Knowns and Unknowns. FEMS Microbiol. Ecol. 96, fiaa224. doi:10.1093/femsec/fiaa224
Musarrat, J., Dwivedi, S., Singh, B. R., Al-Khedhairy, A. A., Azam, A., and Naqvi, A. (2010). Production of Antimicrobial Silver Nanoparticles in Water Extracts of the Fungus Amylomyces Rouxii Strain KSU-09. Bioresour. Technol. 101, 8772–8776. doi:10.1016/j.biortech.2010.06.065
Narayanan, K. B., and Park, H. H. (2014). Antifungal Activity of Silver Nanoparticles Synthesized Using Turnip Leaf Extract (Brassica Rapa L.) against wood Rotting Pathogens. Eur. J. Plant Pathol. 140, 185–192. doi:10.1007/s10658-014-0399-4
Narayanan, K. B., and Sakthivel, N. (2010). Biological Synthesis of Metal Nanoparticles by Microbes. Adv. Colloid Interf. Sci. 156, 1–13. doi:10.1016/j.cis.2010.02.001
Nayak, B. K., Nanda, A., and Prabhakar, V. (2018). Biogenic Synthesis of Silver Nanoparticle from Wasp Nest Soil Fungus, Penicillium italicum and its Analysis against Multi Drug Resistance Pathogens. Biocatal. Agric. Biotechnol. 16, 412–418. doi:10.1016/j.bcab.2018.09.014
Nguyen, D., Lee, J., Park, K., Ching, Y., Nguyen, X., Phan, V. H., et al. (2020). Green Silver Nanoparticles Formed by Phyllanthus Urinaria, Pouzolzia Zeylanica, and Scoparia Dulcis Leaf Extracts and the Antifungal Activity. Nanomaterials 10, 542. doi:10.3390/nano10030542
Nithya, R., and Ragunathan, R. (2012). Synthesis of Silver Nanoparticles Using a Probiotic Microbe and its Antibacterial Effect against Multidrug Resistant Bacteria. Afr. J. Biotechnol. 11, 11013–11021. doi:10.5897/ajb12.439
Oh, S.-D., Lee, S., Choi, S.-H., Lee, I.-S., Lee, Y.-M., Chun, J.-H., et al. (2006). Synthesis of Ag and Ag-SiO2 Nanoparticles by γ-irradiation and Their Antibacterial and Antifungal Efficiency against Salmonella enterica Serovar Typhimurium and Botrytis Cinerea. Colloids Surf. A: Physicochemical Eng. Aspects 275, 228–233. doi:10.1016/j.colsurfa.2005.11.039
Owaid, M. N., Raman, J., Lakshmanan, H., Al-Saeedi, S. S. S., Sabaratnam, V., and Abed, I. A. (2015). Mycosynthesis of Silver Nanoparticles by Pleurotus Cornucopiae Var. Citrinopileatus and its Inhibitory Effects against Candida Sp. Mater. Lett. 153, 186–190. doi:10.1016/j.matlet.2015.04.023
Panáček, A., Kolář, M., Večeřová, R., Prucek, R., Soukupova, J., Kryštof, V., et al. (2009). Antifungal Activity of Silver Nanoparticles against Candida Spp. Biomaterials 30, 6333–6340. doi:10.1016/j.biomaterials.2009.07.065
Panacek, A., Kvitek, L., Prucek, R., Kolář, M., VečEřová, R., Pizúrová, N., et al. (2006). Silver Colloid Nanoparticles: Synthesis, Characterization, and Their Antibacterial Activity. The J. Phys. Chem. B 110, 16248–16253. doi:10.1021/jp063826h
Panda, K. K., Achary, V. M. M., Krishnaveni, R., Padhi, B. K., Sarangi, S. N., Sahu, S. N., et al. (2011). In Vitro biosynthesis and Genotoxicity Bioassay of Silver Nanoparticles Using Plants. Toxicol. vitro 25, 1097–1105. doi:10.1016/j.tiv.2011.03.008
Park, H.-J., Kim, S.-H., Kim, H.-J., and Choi, S.-H. (2006). A New Composition of Nanosized Silica-Silver for Control of Various Plant Diseases. Plant Pathol. J. 22, 295–302. doi:10.5423/ppj.2006.22.3.295
Pereira, L., Dias, N., Carvalho, J., Fernandes, S., Santos, C., and Lima, N. (2014). Synthesis, Characterization and Antifungal Activity of Chemically and Fungal-Produced Silver Nanoparticles against Trichophyton Rubrum. J. Appl. Microbiol. 117, 1601–1613. doi:10.1111/jam.12652
Pethakamsetty, L., Kothapenta, K., Nammi, H. R., Ruddaraju, L. K., Kollu, P., Yoon, S. G., et al. (2017). Green Synthesis, Characterization and Antimicrobial Activity of Silver Nanoparticles Using Methanolic Root Extracts of Diospyros Sylvatica. J. Environ. Sci. 55, 157–163. doi:10.1016/j.jes.2016.04.027
Prasad, R. (2014). Synthesis of Silver Nanoparticles in Photosynthetic Plants. J. Nanoparticles 2014, 963961. doi:10.1155/2014/963961
Pulit-Prociak, J., and Banach, M. (2016). The Use of Plant Materials in the Process of Obtaining Silver and Gold Nanoparticles. J Comput. Theor. Nanosci 13, 2697–2704. doi:10.1166/jctn.2016.4904
Qayyum, S., and Khan, A. U. (2016). Nanoparticles vs. Biofilms: a Battle against Another Paradigm of Antibiotic Resistance. Med. Chem. Commun. 7, 1479–1498. doi:10.1039/c6md00124f
Qian, Y., Yu, H., He, D., Yang, H., Wang, W., Wan, X., et al. (2013). Biosynthesis of Silver Nanoparticles by the Endophytic Fungus Epicoccum Nigrum and Their Activity against Pathogenic Fungi. Bioproc. Biosyst Eng 36, 1613–1619. doi:10.1007/s00449-013-0937-z
Rai, M., Bonde, S., Golinska, P., Trzcińska-Wencel, J., Gade, A., Abd-Elsalam, K. A., et al. (2021). Fusarium as a Novel Fungus for the Synthesis of Nanoparticles: Mechanism and Applications. J. Fungi 7, 139. doi:10.3390/jof7020139
Rao, M. L., and Savithramma, N. (2011). Biological Synthesis of Silver Nanoparticles Using Svensonia Hyderabadensis Leaf Extract and Evaluation of Their Antimicrobial Efficacy. J. Pharm. Sci. Res. 3, 1117.
Rao, N. H., N., L., Pammi, S. V. N., Kollu, P., S., G., and P., L. (2016). Green Synthesis of Silver Nanoparticles Using Methanolic Root Extracts of Diospyros Paniculata and Their Antimicrobial Activities. Mater. Sci. Eng. C 62, 553–557. doi:10.1016/j.msec.2016.01.072
Roe, D., Karandikar, B., Bonn-Savage, N., Gibbins, B., and Roullet, J.-B. (2008). Antimicrobial Surface Functionalization of Plastic Catheters by Silver Nanoparticles. J. Antimicrob. Chemother. 61, 869–876. doi:10.1093/jac/dkn034
Roy, A., Bulut, O., Some, S., Mandal, A. K., and Yilmaz, M. D. (2019). Green Synthesis of Silver Nanoparticles: Biomolecule-Nanoparticle Organizations Targeting Antimicrobial Activity. RSC Adv. 9, 2673–2702. doi:10.1039/c8ra08982e
Roy, S., Mukherjee, T., Chakraborty, S., and Das, T. K. (2013). Biosynthesis, Characterisation & Antifungal Activity of Silver Nanoparticles Synthesized by the Fungus Aspergillus Foetidus Mtcc8876. Dig. J. Nanomater. Biostructures 8, 197–205.
Salavati-Niasari, M., Davar, F., and Mir, N. (2008). Synthesis and Characterization of Metallic Copper Nanoparticles via thermal Decomposition. Polyhedron 27, 3514–3518. doi:10.1016/j.poly.2008.08.020
Sathiyaseelan, A., Saravanakumar, K., Mariadoss, A. V. A., and Wang, M.-H. (2020). Biocompatible Fungal Chitosan Encapsulated Phytogenic Silver Nanoparticles Enhanced Antidiabetic, Antioxidant and Antibacterial Activity. Int. J. Biol. macromolecules 153, 63–71. doi:10.1016/j.ijbiomac.2020.02.291
Shen, T., Wang, Q., Li, C., Zhou, B., Li, Y., and Liu, Y. (2020). Transcriptome Sequencing Analysis Reveals Silver Nanoparticles Antifungal Molecular Mechanism of the Soil Fungi Fusarium Solani Species Complex. J. Hazard. Mater. 388, 122063. doi:10.1016/j.jhazmat.2020.122063
Shibata, N., Ikuta, K., Imai, T., Satoh, Y., Satoh, R., Suzuki, A., et al. (1995). Existence of Branched Side Chains in the Cell Wall Mannan of Pathogenic Yeast, Candida Albicans. J. Biol. Chem. 270, 1113–1122. doi:10.1074/jbc.270.3.1113
Siddiqi, K. S., and Husen, A. (2016). Fabrication of Metal Nanoparticles from Fungi and Metal Salts: Scope and Application. Nanoscale Res. Lett. 11, 98. doi:10.1186/s11671-016-1311-2
Siddiqi, K. S., and Husen, A. (2017). Recent Advances in Plant-Mediated Engineered Gold Nanoparticles and Their Application in Biological System. J. Trace Elem. Med. Biol. 40, 10–23. doi:10.1016/j.jtemb.2016.11.012
Silver, S. (2003). Bacterial Silver Resistance: Molecular Biology and Uses and Misuses of Silver Compounds. FEMS Microbiol. Rev. 27, 341–353. doi:10.1016/s0168-6445(03)00047-0
Simchi, A., Ahmadi, R., Reihani, S. M. S., and Mahdavi, A. (2007). Kinetics and Mechanisms of Nanoparticle Formation and Growth in Vapor Phase Condensation Process. Mater. Des. 28, 850–856. doi:10.1016/j.matdes.2005.10.017
Singh, R., Shedbalkar, U. U., Wadhwani, S. A., and Chopade, B. A. (2015). Bacteriagenic Silver Nanoparticles: Synthesis, Mechanism, and Applications. Appl. Microbiol. Biotechnol. 99, 4579–4593. doi:10.1007/s00253-015-6622-1
Sintubin, L., Verstraete, W., and Boon, N. (2012). Biologically Produced Nanosilver: Current State and Future Perspectives. Biotechnol. Bioeng. 109, 2422–2436. doi:10.1002/bit.24570
Steinfeld, B., Scott, J., Vilander, G., Marx, L., Quirk, M., Lindberg, J., et al. (2015). The Role of Lean Process Improvement in Implementation of Evidence-Based Practices in Behavioral Health Care. J. Behav. Health Serv. Res. 42, 504–518. doi:10.1007/s11414-013-9386-3
Tai, C. Y., Tai, C.-T., Chang, M.-H., and Liu, H.-S. (2007). Synthesis of Magnesium Hydroxide and Oxide Nanoparticles Using a Spinning Disk Reactor. Ind. Eng. Chem. Res. 46, 5536–5541. doi:10.1021/ie060869b
Tang, J., and Xi, T. (2008). Status of Biological Evaluation on Silver Nanoparticles. Sheng Wu Yi Xue Gong Cheng Xue Za Zhi 25, 958–961.
Thakkar, K. N., Mhatre, S. S., and Parikh, R. Y. (2010). Biological Synthesis of Metallic Nanoparticles. Nanomedicine: Nanotechnology, Biol. Med. 6, 257–262. doi:10.1016/j.nano.2009.07.002
Tiwari, D. K., Behari, J., and Sen, P. (2008). Application of Nanoparticles in Waste Water Treatment. World Appl. Sci. J. 3 (3), 417–433.
Tyagi, P. K., Mishra, R., Khan, F., Gupta, D., and Gola, D. (2020). Antifungal Effects of Silver Nanoparticles against Various Plant Pathogenic Fungi and its Safety Evaluation on Drosophila melanogaster. Biointerface Res. Appl. Chem. 10, 6587–6596. doi:10.33263/briac106.65876596
Villa, F., Cappitelli, F., Cortesi, P., and Kunova, A. (2017). Fungal Biofilms: Targets for the Development of Novel Strategies in Plant Disease Management. Front. Microbiol. 8, 654. doi:10.3389/fmicb.2017.00654
Villamizar-Gallardo, R., Cruz, J. F. O., and Ortíz-Rodriguez, O. O. (2016). Fungicidal Effect of Silver Nanoparticles on Toxigenic Fungi in cocoa. Pesq. Agropec. Bras. 51, 1929–1936. doi:10.1590/s0100-204x2016001200003
Wang, L., Zhang, T., Li, P., Huang, W., Tang, J., Wang, P., et al. (2015). Use of Synchrotron Radiation-Analytical Techniques to Reveal Chemical Origin of Silver-Nanoparticle Cytotoxicity. ACS nano 9, 6532–6547. doi:10.1021/acsnano.5b02483
Wijnhoven, S. W. P., Peijnenburg, W. J. G. M., Herberts, C. A., Hagens, W. I., Oomen, A. G., Heugens, E. H. W., et al. (2009). Nano-silver - a Review of Available Data and Knowledge Gaps in Human and Environmental Risk Assessment. Nanotoxicology 3, 109–138. doi:10.1080/17435390902725914
Wong, K. K. Y., and Liu, X. (2010). Silver Nanoparticles-The Real "silver Bullet" in Clinical Medicine? Med. Chem. Commun. 1, 125–131. doi:10.1039/c0md00069h
Wright, J. B., Lam, K., Hansen, D., and Burrell, R. E. (1999). Efficacy of Topical Silver against Fungal Burn Wound Pathogens. Am. J. Infect. Control 27, 344–350. doi:10.1016/s0196-6553(99)70055-6
Xu, L., Wang, Y.-Y., Huang, J., Chen, C.-Y., Wang, Z.-X., and Xie, H. (2020). Silver Nanoparticles: Synthesis, Medical Applications and Biosafety. Theranostics 10, 8996–9031. doi:10.7150/thno.45413
Xue, B., He, D., Gao, S., Wang, D., Yokoyama, K., and Wang, L. (2016). Biosynthesis of Silver Nanoparticles by the Fungus Arthroderma Fulvum and its Antifungal Activity against Genera of Candida, Aspergillus and Fusarium. Int. J. Nanomedicine 11, 1899–1906. doi:10.2147/IJN.S98339
Yilmaz, M. T., İspirli, H., Taylan, O., and Dertli, E. (2020). Synthesis and Characterisation of Alternan-Stabilised Silver Nanoparticles and Determination of Their Antibacterial and Antifungal Activities against Foodborne Pathogens and Fungi. LWT 128, 109497. doi:10.1016/j.lwt.2020.109497
Zhang, X.-F., Liu, Z.-G., Shen, W., and Gurunathan, S. (2016). Silver Nanoparticles: Synthesis, Characterization, Properties, Applications, and Therapeutic Approaches. Int. J. Mol. Sci. 17, 1534. doi:10.3390/ijms17091534
Keywords: silver nanoparticles, antifungal activity, denaturation, proton pumps, cell death
Citation: Mansoor S, Zahoor I, Baba TR, Padder SA, Bhat ZA, Koul AM and Jiang L (2021) Fabrication of Silver Nanoparticles Against Fungal Pathogens. Front. Nanotechnol. 3:679358. doi: 10.3389/fnano.2021.679358
Received: 12 March 2021; Accepted: 17 August 2021;
Published: 08 October 2021.
Edited by:
Bingqing Wei, University of Delaware, United StatesCopyright © 2021 Mansoor, Zahoor, Baba, Padder, Bhat, Koul and Jiang. This is an open-access article distributed under the terms of the Creative Commons Attribution License (CC BY). The use, distribution or reproduction in other forums is permitted, provided the original author(s) and the copyright owner(s) are credited and that the original publication in this journal is cited, in accordance with accepted academic practice. No use, distribution or reproduction is permitted which does not comply with these terms.
*Correspondence: Linghuo Jiang, bGluZ2hvdWppYW5nQGhvdG1haWwuY29t