- 1Department of Radiology, University of California San Diego, San Diego, CA, United States
- 2Biodesign Division, Department of Academia-Government-Industry Collaboration, University of Hiroshima, Hiroshima, Japan
- 3Department of Pathology, University of California San Diego, San Diego, CA, United States
- 4Department of Molecular and Cellular Biology, Shiley Center for Orthopedic Research and Education at Scripps Clinic, La Jolla, CA, United States
Introduction: The acetabulum labrum plays a critical role in hip function. Medical imaging techniques for measuring changes in labral properties due to its degeneration may help improve our knowledge of its role in hip osteoarthritis (OA). This study aimed to explore the correlation between the mechanical properties of the acetabulum labrum and ultrashort echo time (UTE) MRI properties.
Material and method: Acetabular labrum specimens were dissected from 12 fresh-frozen human cadaveric hip joints (64.6 ± 11.6 years old at the time of death, 7 female). UTE Cones sequences were used to measure apparent spin-spin (T2*) and spin-lattice (T1) relaxation times using a knee coil at 3 T in a clinical MR scanner. The stiffness and the elastic modulus (E) of the specimens were measured before MRI scans using uniaxial tensile tests. Spearman's rank correlation coefficients of Tendons' UTE-T2* and -T1 with their mechanical properties were calculated.
Results: The human labrum specimen elastic modulus showed a significant inverse correlation with UTE-T2* (R = −0.66, P < 0.01) and with UTE-T1 (R = −0.56, P = 0.05). The stiffness of the specimens showed significant inverse correlations with UTE-T2* (R = −0.53, P = 0.01) and UTE-T1 (R = −0.63, P = 0.02).
Conclusion: This study highlighted the potential of UTE-MRI techniques for the mechanical assessment of the acetabular labrum. UTE-MRI may improve labrum degeneration detection and monitoring, which requires further investigations.
1 Introduction
The acetabular labrum is a C-shaped fibrocartilaginous rim that outlines the bony acetabulum's ring in the hip joint (1). The acetabular labrum is crucial in maintaining normal hip joint biomechanics and function (2, 3). Labral damage can lead to abnormal joint mechanics, increasing the risk of osteoarthritis (OA) (4–6).
OA pathogenesis involves a complex interaction of factors, including the loss of articular cartilage, altered subchondral bone structure, and chronic low-grade inflammation. This condition not only significantly reduces patients' quality of life but also imposes a substantial burden on healthcare systems due to associated disability and costs of management (7, 8).
Mughal et al. observed an increased risk of developing early moderate osteoarthritis in young individuals with labral tears likely due to abnormal joint mechanics (9). Strong connections between mechanical symptoms, hip pain, reduced range of motion, and underlying labral tears and cartilage defects have been reported in the literature (6, 9, 10). Chondral lesions also have been shown to frequently occur in joints exhibiting labral fraying or tears, with the majority of cartilage damage happening near the labral injury (6). Therefore, a non-invasive assessment of the labrum quality and its mechanical status may help detect OA-related changes in the hip joint.
The acetabular labrum tear and large lesions can be detected semi-quantitatively using conventional magnetic resonance imaging (MRI). Conventional MRI has been used in several studies for morphological assessment of acetabular labrum (11–13). Fat-saturated T1- and T2-weighted images have been reported to be the most useful for qualitative assessment of the acetabular labrum lesions (14, 15). MRI signal intensity of an injured or recovering acetabular labrum is likely higher than a healthy acetabular labrum (16–18). However, quantitative assessment of the acetabular labrum using MR signal intensity is highly challenging as the signal intensity in MR images depends on various factors such as the scanner and coil specifications, subject positioning, acquisition parameters, reconstruction algorithms, and signal normalization.
Collagen and proteoglycan (PG) constitute a major portion of the dry weight of acetabular labrum which results in its short T2 values and low signal in conventional MR sequences (19). However, employing ultrashort echo time (UTE) MRI sequences can acquire high signals from short T2 musculoskeletal tissues enabling accurate non-invasive quantitative assessment (20–23). The feasibility of the UTE T2* on acetabular labrum has been evaluated by Wong et al. on the hip of eight asymptomatic volunteers (24). To the authors' knowledge, the relationship between UTE-MRI and acetabular labrum mechanical properties has not been reported in the literature.
This study aimed to investigate the correlations of apparent spin-spin (T2*) and spin-lattice (T1) relaxation times with the tensile elastic modulus, a crucial index representing the tissue's quality, of human acetabular labrum specimens. T2* and T1 are common quantitative MRI techniques used for musculoskeletal (MSK) tissue assessment (25–27). T2* and T1 are hypothesized to show correlations with acetabular labrum mechanical properties at a similar level demonstrated for other MSK tissues such as anterior cruciate ligament (ACL) (28).
2 Materials and methods
2.1 Sample preparation
The anterolateral acetabular labrum specimens were dissected from 12 fresh-frozen human cadaveric hip joints (64.6 ± 11.6 years old at the time of death, 7 female). Frozen hip specimens were provided by a non-profit whole-body donation company (Anatomy Gifts Registry) and experiments were performed in the Scripps Research Institute. The average length and cross-sectional area of the specimens were 31 ± 4.7 mm and 23.7 ± 7.7 mm2, respectively. Figure 1A shows a representative harvested acetabular labrum specimen from a 60-year-old female donor.
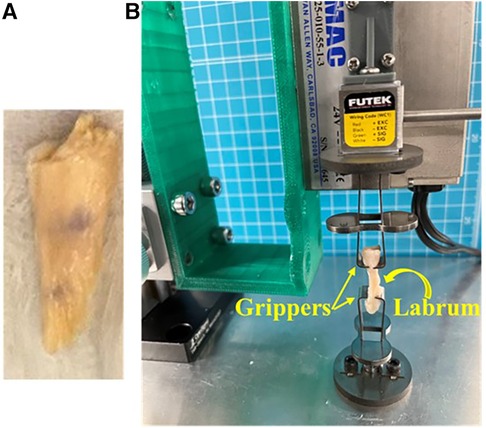
Figure 1. (A) A representative thawed acetabular labrum specimen harvested from the hip joint of a 60-year-old female. (B) A representative acetabular labrum specimen mounted on the grippers of an in-house developed benchtop uniaxial loading device for tensile mechanical tests.
2.2 Tensile mechanical test
The stiffness and the elastic modulus (E) of the specimens were measured before MRI scans. The mechanical setup for a representative acetabular labrum specimen is shown in Figure 1B. Both ends of the specimens were mounted on the stainless-steel grippers of an in-house developed benchtop uniaxial loading device. The initial distance between the fixed and actuated gripper was set to 10 mm. After initial alignment and preloading to straighten the specimens (steps of 0.005 N tension were applied for 5s periods up to the time the sample held 0.02 N as preload for the 20s), a 1 mm tensile displacement was applied at 0.3 mm/sec rate and the applied force was recorded. The average maximum mechanical stress was calculated as the maximum force (at 1 mm displacement) divided by the specimen's cross-sectional area, measured using a digital caliper after pausing the loading. The maximum strain for all specimens was 0.1 (i.e., 1 mm / 10 mm). The average stiffness was calculated as the maximum force at (1 mm displacement) divided by 10 mm (the maximum deformation). The average tensile elastic modulus was calculated as the average maximum stress divided by the average maximum strain.
2.3 Quantitative UTE-MRI
All specimens were placed separately in 5 ml syringes as shown in Figure 2A for MR imaging. The dissected specimens were soaked in phosphate-buffered saline (PBS) for 1 h before scanning to ensure similar levels of hydration in all specimens. All syringes were filled with perfluoropolyether (Fomblin, Ausimont, NJ, USA) to minimize dehydration and susceptibility artifacts during MRI scans.
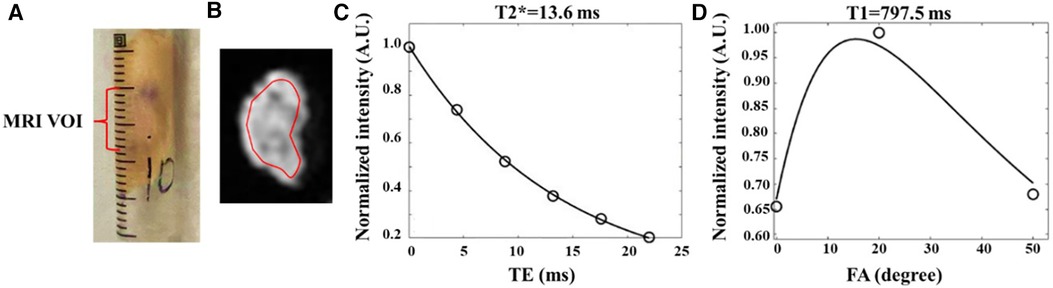
Figure 2. Quantitative UTE-MRI analyses in a representative human acetabular labrum specimen (from a 68-year-old male donor). (A) each specimen was placed in a standard 5 ml syringe, then they were fixed with tape together before the MRI scan. The middle third of the specimen is highlighted as the volume interest region (VOI) analyzed in MRI. (B) selected region of interest (ROI) for the same sample depicted schematically on the UTE-MRI image. (C) Single-component exponential fitting of T2* decay, and (D) T1 recovery within the selected ROI.
The UTE-MRI scans were performed on a 3 T MRI scanner (MR750, GE Healthcare Technologies, WI, USA) using a standard transmit/receive knee coil (GE Healthcare Technologies, Transmit/Receive, 8 channels). The grouped syringes were positioned horizontally inside the coil to ensure acetabular labrum alignment parallel to B0. All MRI images were acquired in the axial plane.
Two following sets of 3D UTE Cones MRI sequences were performed, (1) T2* sequence with differing echo times (TEs) to measure T2* values and (2) actual flip angle imaging (AFI) with variable flip angle (VFA) sequences (AFI-VFA) to measure T1 values (29). Data acquisition parameters for the two quantitative UTE-MRI protocols are presented in Table 1. Features of the 3D UTE Cones sequence have been described in previous studies (30).
UTE-MRI biomarkers were calculated in three representative slices at the middle of each specimen (volume of interest, VOI is indicated in Figure 2A) within global regions of interest (ROIs) covering the entire cross-sectional area. For each slice, average signal values within the ROI were used for T2* and T1 measurements using single-component exponential fitting models. Then the values for three slices were averaged to obtain the average value for each specimen.
All UTE-MRI measurements and models were performed using in-house developed codes in MATLAB (version 2021, The Mathworks Inc., Natick, MA, USA).
2.4 Statistical analysis
The normal distribution of all MRI and mechanical variables was examined using the Kolmogorov-Smirnov test. All variables were found to have non-normal distributions as expected because of the limited number of specimens. Thus, Spearman's rank correlations were calculated between average UTE-MRI quantifications and the acetabular labrum mechanical properties. Statistical analyses were performed in MATLAB. P values below 0.05 were considered significant.
3 Results
Figures 2C,D show single-component exponential T2* and T1 fittings, respectively, for a representative acetabular labrum specimen. The high signal obtained by UTE MRI leads to a well-fitting of the actual data points.
Table 2 summarizes the mean, standard deviation (SD) of the donor age and body mass index (BMI), UTE-T2* and -T1 values, and mechanical properties of the studied acetabular labrum specimens.

Table 2. Mean, standard deviation (SD), and range of the UTE-MRI, mechanical in the studied acetabular labrum specimens.
The scatter plots, linear regression trendlines, and Spearman's rank correlation coefficients of the elastic modulus and stiffness on UTE- T2* and -T1 values are presented in Figure 3. The elastic modulus showed a significant inverse correlation with T2* (R = −0.66, P < 0.01) and with UTE-T1 (R = −0.56, P = 0.05). The stiffness of the specimens showed significant inverse correlations with UTE-T2* (R = −0.53, P = 0.01) and UTE-T1 (R = −0.63, P = 0.02).
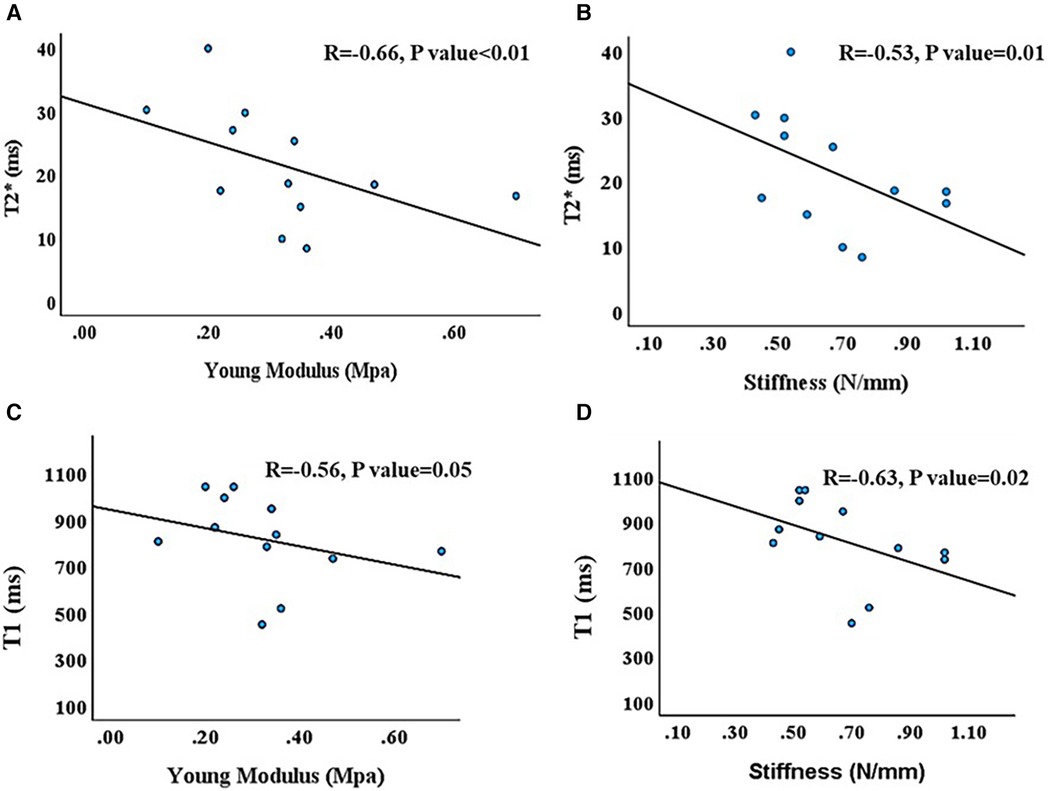
Figure 3. Scatter plot and linear regressions of mechanical characteristics on quantitative UTE-MRI analyses in human labrum specimen specimens. (A,B) T2* (ms) values which showed a significant negative correlation with Young Modulus (Mpa) and Stiffness (N/mm) of the acetabular labrum, respectively, (C,D) T1 (ms) values with a significant negative correlation with Young Modulus (Mpa) and Stiffness (N/mm) of the acetabular labrum, respectively. Significance levels for these correlations were below 0.05.
4 Discussion
This study was the first to focus on the assessment of human acetabulum labrum's mechanical properties using quantitative UTE MRI. UTE-T2* and -T1 were selected as the most common and less sophisticated techniques which are closer to being translated into clinical applications (25–27). Significant correlations between the mechanical properties and quantitative UTE techniques highlight their potential capabilities to non-invasively assess acetabular labrum tissue quality.
UTE-T2* has been used previously to assess human femoral condyle specimens from volunteers undergoing total knee arthroplasty (TKA). Significantly lower T2* values were observed in the moderate to severe OA group than in the normal group (31). In a similar study on the tibial cartilage, UTE-T2* was significantly higher in the early OA group vs. the control group (32). Lower UTE-T1 values have been reported for degenerated lumber discs as compared with normal ones in another study (33). UTE-T2* has shown correlations with mechanical and histological properties of other MSK tissues, such as ACL (28, 34), articular cartilage (35, 36), and bone (37). UTE-T1 also has shown correlations with the mechanical properties of the ACL (28).
UTE T2* in the normal human acetabulum volunteers values was reported 16.73–19.37 ms (24) which is in the range of the values presented in our study. In another Ex vivo study, histologically confirmed degenerated glenoid labra had increased UTE-T2* and decreased UTE-T1 values as compared with normal labra (38).
The limitations of this study can be summarized in fifth aspects. First, the number of samples was relatively small as is the nature of pilot studies. Moreover, the acetabular labrum specimens were from twelve donors and were without any gross visible abnormality such as degeneration or tearing. However, the presence of minor defects or microscopic tears in the specimens was not examined in this study, which often exists in most tissues from old donors. The inclusion of more specimens with some degree of degeneration, which can be confirmed by histopathology evaluation, is expected to increase the generalizability of this study. Future well-designed studies comparing quantitative UTE-MRI techniques with histology measures of the acetabular labrum are also required to understand such techniques' capability to localize the tissue degeneration. Second, the acetabular labrum specimens were not scanned in their precise anatomical direction in the body. Nevertheless, as the specimens were all in the same direction, the orientation impact was assumed to be the same on all specimens and did not impact the correlation study presented here. Third, the performance of the UTE MRI techniques for in vivo evaluation of acetabular labrum has not been investigated in this study, which will be the focus of future investigations. It should be noted that the MR imaging of the acetabular labrum in vivo is highly challenging. For example, the anatomical misidentification of the sub-labral sulcus as a labral lesion in hip MRI images (39, 40). Moreover, studies have also reported the complexity of evaluating the morphology of postoperative labra to align with preoperative labral tears, posing difficulties for radiological assessments (41). Fourth, this study was performed on ex vivo specimens after a single freeze-thaw cycle, which may have influenced the results. However, studies have shown that a single freeze-thaw cycle does not significantly affect the biomechanical properties of other MSK tissues (42). Fifth, the elastic modulus measurement on the studied specimens was performed using tensile tests. However, this does not rigorously mimic the in vivo forces over most regions of the acetabular labrum, which is typically exposed to a combination of compressive, compact, bending, and tensile loading.
5 Conclusions
Two common quantitative UTE-MRI biomarkers, UTE-T2* and UTE-T1 were investigated for their correlations with the mechanical properties of human acetabulum labrum specimens. Mechanical properties of the tissues showed significant inverse correlations with UTE-T2* and UTE-T1. This study highlighted the potential of UTE-MRI techniques for the acetabular labrum mechanical assessment which can help improve labrum degeneration detection and monitoring which requires further investigations.
Data availability statement
The original contributions presented in the study are included in the article/Supplementary Material, further inquiries can be directed to the corresponding authors.
Ethics statement
The studies involving humans were approved by Scripps Research Institute institutional review board. The studies were conducted in accordance with the local legislation and institutional requirements. The human samples used in this study were acquired from non-profit whole-body donation company (Anatomy Gifts Registry). Written informed consent for participation was not required from the participants or the participants’ legal guardians/next of kin in accordance with the national legislation and institutional requirements.
Author contributions
DM: Formal Analysis, Investigation, Methodology, Writing – original draft, Writing – review & editing. TH: Conceptualization, Data curation, Investigation, Methodology, Writing – review & editing, Funding acquisition. HS: Formal Analysis, Investigation, Writing – review & editing. AT: Data curation, Formal Analysis, Investigation, Writing – review & editing. ED: Methodology, Resources, Validation, Writing – review & editing. DL: Conceptualization, Investigation, Methodology, Resources, Supervision, Writing – review & editing. SJ: Conceptualization, Funding acquisition, Investigation, Methodology, Project administration, Resources, Supervision, Validation, Writing – original draft, Writing – review & editing.
Funding
The author(s) declare that financial support was received for the research, authorship, and/or publication of this article.
The authors acknowledge grant support from NIH (K01AR080257) and JSPS KAKENHI (JP19K09658, JP18KK0104).
Conflict of interest
The authors declare that the research was conducted in the absence of any commercial or financial relationships that could be construed as a potential conflict of interest.
Publisher's note
All claims expressed in this article are solely those of the authors and do not necessarily represent those of their affiliated organizations, or those of the publisher, the editors and the reviewers. Any product that may be evaluated in this article, or claim that may be made by its manufacturer, is not guaranteed or endorsed by the publisher.
References
1. Seldes RM, Tan V, Hunt J, Katz M, Winiarsky R, Fitzgerald RH. Anatomy, histologic features, and vascularity of the adult acetabular labrum. Clin Orthop. (2001) 382:232–40. doi: 10.1097/00003086-200101000-00031
2. Crawford MJ, Dy CJ, Alexander JW, Thompson M, Schroder SJ, Vega CE, et al. The 2007 frank stinchfield award. The biomechanics of the hip labrum and the stability of the hip. Clin Orthop. (2007) 465:16–22. doi: 10.1097/BLO.0b013e31815b181f
3. Dienst M, Seil R, Gödde S, Brang M, Becker K, Georg T, et al. Effects of traction, distension, and joint position on distraction of the hip joint: an experimental study in cadavers. Arthroscopy. (2002) 18(8):865–71. doi: 10.1053/jars.2002.36120
4. Roemer FW, Hunter DJ, Winterstein A, Li L, Kim YJ, Cibere J, et al. Hip osteoarthritis MRI scoring system (HOAMS): reliability and associations with radiographic and clinical findings. Osteoarthritis Cartilage. (2011) 19(8):946–62. doi: 10.1016/j.joca.2011.04.003
5. Beck M, Kalhor M, Leunig M, Ganz R. Hip morphology influences the pattern of damage to the acetabular cartilage: femoroacetabular impingement as a cause of early osteoarthritis of the hip. J Bone Joint Surg Br. (2005) 87(7):1012–8. doi: 10.1302/0301-620X.87B7.15203
6. McCarthy JC, Noble PC, Schuck MR, Wright J, Lee J. The role of labral lesions to development of early degenerative hip disease. Clin Orthop. (2001) 393:25–37. doi: 10.1097/00003086-200112000-00004
7. Motta F, Barone E, Sica A, Selmi C. Inflammaging and osteoarthritis. Clin Rev Allergy Immunol. (2023) 64(2):222–38. doi: 10.1007/s12016-022-08941-1
8. Wojdasiewicz P, Poniatowski ŁA, Kotela A, Skoda M, Pyzlak M, Stangret A, et al. Comparative analysis of the occurrence and role of CX3CL1 (fractalkine) and its receptor CX3CR1 in hemophilic arthropathy and osteoarthritis. J Immunol Res. (2020) 2020:2932696. doi: 10.1155/2020/2932696
9. Mughal A, Vukanic D, Murphy EP, Crozier-Shaw G, Mahapatra A. Labral tear is associated with increased risk of osteoarthritis—a cross-sectional study. J Orthop Rep. (2023) 2(4):100185. doi: 10.1016/j.jorep.2023.100185
10. McCarthy JC, Busconi B. The role of hip arthroscopy in the diagnosis and treatment of hip disease. Orthopedics. (2013) 18(8):753–6. doi: 10.3928/0147-7447-19950801-12
11. Cotten A, Boutry N, Demondion X, Paret C, Dewatre F, Liesse A, et al. Acetabular labrum: MRI in asymptomatic volunteers. J Comput Assist Tomogr. (1998) 22(1):1. doi: 10.1097/00004728-199801000-00001
12. Sundberg TP, Toomayan GA, Major NM. Evaluation of the acetabular labrum at 3.0-T MR imaging compared with 1.5-T MR arthrography: preliminary experience. Radiology. (2006) 238(2):706–11. doi: 10.1148/radiol.2382050165
13. Thomas JD, Li Z, Agur AM, Robinson P. Imaging of the acetabular labrum. Semin Musculoskelet Radiol. (2013) 17(3):248–57. doi: 10.1055/s-0033-1348091
14. Fu Q, Gao GY, Xu Y, Lin ZH, Sun YJ, Cui LG. Comparative study of ultrasound and magnetic resonance imaging in the diagnosis of asymptomatic anterosuperior acetabular labrum tears. Beijing Da Xue Xue Bao. (2023) 55(4):665–9. doi: 10.19723/j.issn.1671-167x.2023.04.016
15. Huang Z, Liu W, Li T, Liu Z, Zhao P. Diagnostic value of magnetic resonance imaging and magnetic resonance arthrography for assessing acetabular labral tears: a systematic review and meta-analysis. Medicine (Baltimore). 2023;102(9):e32963. doi: 10.1097/MD.0000000000032963
17. Horii M, Kubo T, Hirasawa Y. Radial MRI of the hip with moderate osteoarthritis. J Bone Joint Surg Br. 2000;82-B(3):364–8. doi: 10.1302/0301-620x.82b3.0820364
18. Kubo T, Horii M, Yamaguchi J, Inoue S, Fujioka M, Ueshima K, et al. Acetabular labrum in hip dysplasia evaluated by radial magnetic resonance imaging. J Rheumatol. (2000) 27(8):1955–60.10955338
19. Groh MM, Herrera J. A comprehensive review of hip labral tears. Curr Rev Musculoskelet Med. (2009 Jun) 2(2):105–17. doi: 10.1007/s12178-009-9052-9
20. Chang EY, Du J, Chung CB. UTE imaging in the musculoskeletal system. J Magn Reson Imaging. (2015) 41(4):870–83. doi: 10.1002/jmri.24713
21. Du J, Bydder GM. Qualitative and quantitative ultrashort-TE MRI of cortical bone. NMR Biomed. (2013) 26(5):489–506. doi: 10.1002/nbm.2906
22. Ma Y, Jang H, Jerban S, Chang EY, Chung CB, Bydder GM, et al. Making the invisible visible-ultrashort echo time magnetic resonance imaging: technical developments and applications. Appl Phys Rev. (2022) 9(4):041303. doi: 10.1063/5.0086459
23. Ma YJ, Jerban S, Jang H, Chang D, Chang EY, Du J. Quantitative ultrashort echo time (UTE) magnetic resonance imaging of bone: an update. Front Endocrinol (Lausanne). (2020 Sep 18) 11:567417. doi: 10.3389/fendo.2020.567417
24. Wong TT, Quarterman P, Lynch TS, Rasiej MJ, Jaramillo D, Jambawalikar SR. Feasibility of ultrashort echo time (UTE) T2* cartilage mapping in the hip: a pilot study. Acta Radiol. (2022) 63(6):760–6. doi: 10.1177/02841851211011563
25. Bydder GM, Young IR. Clinical use of the partial saturation and saturation recovery sequences in MR imaging. J Comput Assist Tomogr. (1985) 9(6):1020–32. doi: 10.1097/00004728-198511000-00004
26. Du J, Carl M, Bydder M, Takahashi A, Chung CB, Bydder GM. Qualitative and quantitative ultrashort echo time (UTE) imaging of cortical bone. J Magn Reson San Diego Calif 1997. (2010) 207(2):304–11. doi: 10.1016/j.jmr.2010.09.013
27. Afsahi AM, Sedaghat S, Moazamian D, Afsahi G, Athertya JS, Jang H, et al. Articular cartilage assessment using ultrashort echo time MRI: a review. Front Endocrinol (Lausanne). (2022) 13:892961. doi: 10.3389/fendo.2022.892961
28. Jerban S, Hananouchi T, Ma Y, Namiranian B, Dorthe EW, Wong JH, et al. Correlation between the elastic modulus of anterior cruciate ligament (ACL) and quantitative ultrashort echo time (UTE) magnetic resonance imaging. J Orthop Res Off Publ Orthop Res Soc. (2022) 40(10):2330–9. doi: 10.1002/jor.25266
29. Ma YJ, Zhao W, Wan L, Guo T, Searleman A, Jang H, et al. Whole knee joint T1 values measured in vivo at 3T by combined 3D ultrashort echo time cones actual flip angle and variable flip angle methods. Magn Reson Med. (2019 Mar) 81(3):1634–44. doi: 10.1002/mrm.27510
30. Ma YJ, Chang EY, Carl M, Du J. Quantitative magnetization transfer ultrashort Echo time imaging using a time-efficient 3D multispoke cones sequence. Magn Reson Med. (2018) 79(2):692–700. doi: 10.1002/mrm.26716
31. Yang J, Shao H, Ma Y, Wan L, Zhang Y, Jiang J, et al. Quantitative ultrashort echo time magnetization transfer (UTE-MT) for diagnosis of early cartilage degeneration: comparison with UTE-T2* and T2 mapping. Quant Imaging Med Surg. (2020) 10(1):171–83. doi: 10.21037/qims.2019.12.04
32. Imamura R, Teramoto A, Murahashi Y, Okada Y, Okimura S, Akatsuka Y, et al. Ultra-short echo time-MRI T2* mapping of articular cartilage layers is associated with histological early degeneration. Cartilage. (2023):19476035231205685. doi: 10.1177/19476035231205685. [Epub ahead of print]37846091
33. Jenkins JP, Hickey DS, Zhu XP, Machin M, Isherwood I. MR Imaging of the intervertebral disc: a quantitative study. Br J Radiol. (1985) 58(692):705–9. doi: 10.1259/0007-1285-58-692-705
34. Biercevicz AM, Akelman MR, Rubin LE, Walsh EG, Merck D, Fleming BC. The uncertainty of predicting intact anterior cruciate ligament degeneration in terms of structural properties using T(2)(*) relaxometry in a human cadaveric model. J Biomech. (2015) 48(6):1188–92. doi: 10.1016/j.jbiomech.2015.02.021
35. Namiranian B, Jerban S, Ma Y, Dorthe EW, Afsahi AM, Wong J, et al. Assessment of mechanical properties of articular cartilage with quantitative three-dimensional ultrashort echo time (UTE) cones magnetic resonance imaging. J Biomech. (2020) 113:110085. doi: 10.1016/j.jbiomech.2020.110085
36. Hananouchi T, Chen Y, Jerban S, Teramoto M, Ma Y, Dorthe EW, et al. A useful combination of quantitative ultrashort Echo time MR imaging and a probing device for biomechanical evaluation of articular cartilage. Biosensors. (2021) 11(2):52. doi: 10.3390/bios11020052
37. Bae WC, Chen PC, Chung CB, Masuda K, D’Lima D, Du J. Quantitative ultrashort echo time (UTE) MRI of human cortical bone: correlation with porosity and biomechanical properties. J Bone Miner Res. (2012) 27(4):848–57. doi: 10.1002/jbmr.1535
38. Iwasaki K, Tafur M, Chang EY, Statum S, Biswas R, Tran B, et al. High resolution qualitative and quantitative MR evaluation of the glenoid labrum. J Comput Assist Tomogr. (2015) 39(6):936–44. doi: 10.1097/RCT.0000000000000307
39. Nguyen MS, Kheyfits V, Giordano BD, Dieudonne G, Monu JUV. Hip anatomic variants that may mimic abnormalities at MRI: labral variants. AJR Am J Roentgenol. (2013) 201(3):W394–400. doi: 10.2214/AJR.12.9860
40. Liu Y, Lu W, Ouyang K, Deng Z. The imaging evaluation of acetabular labral lesions. J Orthop Traumatol Off J Ital Soc Orthop Traumatol. (2021) 22:34. doi: 10.1186/s10195-021-00595-7
41. Foreman SC, Zhang AL, Neumann J, von Schacky CE, Souza RB, Majumdar S, et al. Postoperative MRI findings and associated pain changes after arthroscopic surgery for femoroacetabular impingement. AJR Am J Roentgenol. (2020) 214(1):177–84. doi: 10.2214/AJR.19.21421
Keywords: acetabular labrum, osteoarthritis, quantitative MRI, UTE, mechanical properties
Citation: Moazamian D, Hananouchi T, Shaterian Mohammadi H, Tayarani A, Dorthé EW, D'lima D and Jerban S (2024) Elastic modulus of cadaveric acetabular labrum correlates with ultrashort echo time (UTE) apparent spin-spin (T2*) and spin-lattice (T1) relaxation times. Front. Musculoskelet. Disord. 2:1359296. doi: 10.3389/fmscd.2024.1359296
Received: 21 December 2023; Accepted: 16 February 2024;
Published: 1 March 2024.
Edited by:
Jian Hou, The Chinese University of Hong Kong, ChinaReviewed by:
Łukasz A. Poniatowski, Medical University of Warsaw, PolandAntónio Proença Caetano, NOVA University of Lisbon, Portugal
© 2024 Moazamian, Hananouchi, Shaterian Mohammadi, Tayarani, Dorthé, D'lima and Jerban. This is an open-access article distributed under the terms of the Creative Commons Attribution License (CC BY). The use, distribution or reproduction in other forums is permitted, provided the original author(s) and the copyright owner(s) are credited and that the original publication in this journal is cited, in accordance with accepted academic practice. No use, distribution or reproduction is permitted which does not comply with these terms.
Abbreviations MR, magnetic resonance; MRI, magnetic resonance imaging; 3D, three-dimensional; UTE, ultrashort echo time imaging; RF, radio frequency; FOV, field of view; ROI, region of interest; TE, echo time; TR, repetition time; FA, flip angle; PBS, phosphate-buffered saline.
*Correspondence: Dina Moazamian ZG1vYXphbWlhbkBoZWFsdGgudWNzZC5lZHU= Takehito Hananouchi dGFrZWhpdG9AaGlyb3NoaW1hLXUuYWMuanA= Darryl D’Lima ZGRsaW1hQHNjcmlwcHMuZWR1 Saeed Jerban c2plcmJhbkBoZWFsdGgudWNzZC5lZHU=
†These authors have contributed equally to this work