- 1Department Health Kinesiology and Applied Physiology, Concordia University, Montreal, QC, Canada
- 2School of Health, Concordia University, Montreal, QC, Canada
- 3Centre de Recherche Interdisciplinaire en Réadaptation (CRIR), Montreal, QC, Canada
Objective: Chronic low-back pain (CLBP) is the leading cause of disability globally. CLBP is associated with a decrease in cross-sectional area (CSA) and an increase in fat infiltration (FI) of the lumbar paraspinal muscles, such as erector spinae (ES). Neuromuscular electrical stimulation (NMES) therapy showed promising outcomes in the treatment of CLBP, but its impact on ES morphology is unknown. Therefore, this study aimed to compare the effect of two NMES protocols on ES CSA and FI in a cohort of patients with CLBP.
Methods: Twenty participants with CLBP (aged 18–60 years old) were randomized into the phasic (n = 11) or the combined (n = 9) protocol groups. They completed a 10-week (20 sessions) NMES therapy using the StimaWELL 120MTRS device. Magnetic resonance imaging (MRI) examinations were acquired at baseline and post-intervention to assess ES CSA and FI at all spinal levels (e.g., L1–L2 to L5–S1). Statistical analyses were conducted to assess the effect of the intervention on ES morphology irrespective of groups, and between groups.
Results: Irrespective of groups, participants showed a decrease in left ES CSA (p = 0.005) at L2–L3 and left ES FI at L5–S1 (p = 0.040). We also observed a greater decrease in ES FI in the combined protocol compared to the phasic protocol on the right side at L3–L4 (p = 0.029) and L4–L5 (p = 0.015). No other changes in ES CSA or FI were observed (p > 0.05).
Conclusion: NMES therapy demonstrated minimal effect on ES morphology in patients with CLBP. Further research is needed to extend and confirm our findings.
1. Introduction
Low back pain is responsible for 60.1 million years lived with disability amongst working-age adults (1). The economic burden and drug programs for CLBP are similar to other high-cost conditions (2), such as cardiovascular diseases, cancer, mental health and autoimmune diseases, and 80%–90% of these cost are due to production losses (1). Chronic low-back pain (CLBP) is defined as pain lasting >3 months (with or without associated leg pain), located between the lower rib margins and the gluteal folds (1, 3). CLBP is classified as non-specific when no causative nociceptor factors can be identified. It can emerge intrinsically from the spine, intervertebral discs, or surrounding soft tissue (4). A recent study reported that the “Mo-fi-disc” scoring system, which considers the combined effect of paraspinal muscles, end-plates, and discs, is a significant predictor of the intensity of LBP (OR = 1.193, 95% CI 1.05–1.349, p = 0.005) (5).
In the lumbar spine, the erector spinae (ES) muscle is responsible for global stability and torque generation (6). At upper lumbar levels, the thoracic fibers of the lumbar erector spinae contribute between 70% and 86% of the total extensor moment (7). The ES controls spinal orientation and balances the external forces applied to the trunk (6) by producing a compressive and posterior shearing force at L1–L4 in the upright posture (7–9).
Lumbar ES muscle degeneration has been associated with non-specific CLBP. This degeneration is characterized by macroscopic or microscopic changes (10). Macroscopic changes can be observed via changes in muscle cross-sectional area (CSA) and intramuscular fatty infiltration (FI). Microscopic changes present as changes in muscle fiber type and as microtears in the tissue (10). Although the link between CLBP and morphological muscle changes of the lumbar multifidus (LM) muscle has been thoroughly examined in the literature (11), there is inconclusive evidence regarding a similar association for the ES. One study reported that elderly patients with CLBP had a smaller ES CSA compared to aged matched controls without CLBP (12). Another study showed increased paraspinal muscle FI in patients with continuous vs. recurrent CLBP, but no difference was observed in ES CSA (13). A third study found that patients with severe intervertebral disc degeneration (IDD) were more likely to have increased FI in the ES, and that female participants with symptomatic IDD had greater FI infiltration in the ES at L5–S1 than matched male participants (14). Indeed, a model to predict in vivo intervertebral disc function was recently developed, allowing for a better evaluation of the etiology of CLBP as it pertains to intervertebral disc function (15). Facet joint tropism at the upper lumbar levels has also been associated with higher levels of paraspinal muscles FI (both ES and LM) at lower lumbar levels (16). Overall, while atrophy of the paraspinal muscles may be more present in lower levels of the lumbar spine compared to the upper levels, evidence for ES-specific atrophy is lacking (10). Other factors such as age, sex, body mass index (BMI) and disuse have also been associated with paraspinal muscle FI. Microscopically, CLBP patients show a similar fiber type distribution to healthy controls with about 64% of type I fibers in paraspinal muscles (17).
The current recommendations for the treatment of CLBP suggest that first-line care should be non-pharmacological (18). Indeed, a large clinical trial found that cognitive functional therapy was more effective in the treatment of CLBP and more cost effective than usual care (19). Another treatment option is neuromuscular electrical stimulation (NMES), which uses surface electrodes to transmit electrical current and depolarize nerves, leading to muscle contraction. There is limited evidence that NMES therapy is effective in treating the muscular and functional deficits seen with CLBP. NMES paired with trunk muscle training increased function (20, 21), and decreased pain and low-back pain-related disability in patients with CLBP (22). NMES therapy of the lumbar ES improved trunk balance (e.g., static and dynamic), postural control, recovery of ambulation, and health-related quality of life in stroke patients (21). NMES therapy also increased LM CSA in patients ≥65 years old (23). On the other hand, a 4-week NMES intervention showed no change in function and no pain reduction in patients with CLBP (24).
To our knowledge, no studies have investigated the impact of NMES on ES CSA and FI in CLBP patients. Therefore, the aim of this study was to examine the effect of a 10-week NMES intervention on ES CSA and FI, from L1–L2 to L5–S1, in a cohort of CLBP patients. We hypothesized that NMES therapy would increase CSA and reduce FI of the ES from pre- to post-intervention.
2. Materials and methods
2.1. Study design
This study was a preliminary analysis of a broader ongoing randomized controlled trial (RCT) with two groups (e.g., test-retest design) evaluating the effect of NMES on multifidus muscle morphology and function in CLBP patients. This randomized controlled trial was approved by the Central Ethics Research Committee of the Quebec Minister of Health and Social Services (#CCER-20-21-07) and the protocol was published in BMC Musculoskeletal Disorders (25).
2.2. Study sample
Twenty participants (12 women, 8 men), aged 18–60 years old, were recruited by students and clinicians affiliated with the Quebec Low Back Pain Consortium through the School of Health website and mailing list, over social media, and through word-of-mouth.
Participants included in the research met the following criteria:
• Chronic non-specific LBP (>3 months), defined as pain in the region between the lower ribs and gluteal folds, with or without leg pain.
• Aged between 18 and 60 years old.
• English or French speakers.
• Have at least a score of “moderate” on the Modified Oswestry Disability Index (ODI) (26, 27).
• Able to undergo magnetic resonance imaging (MRI) examination.
Participants were excluded if they met any of the following criteria:
• Currently undergoing or having received physical therapy treatment in the previous month.
• Consistent motor control training for the low back and/or consistent weightlifting, powerlifting, bodybuilding, or strongman training in the previous 6 weeks [resistance training is a potential confounding variable; however, muscle strength and size decrease 2–6 weeks following the cessation of resistance training in both trained and untrained individuals (28, 29)].
• History of lumbar surgery or minimally invasive technique such as radiography ablation.
• Presence of positive lumbosacral dermatomes or myotomes.
• Presence of disease that could affect the stiffness of muscle tissue (collagen tissue disease, hemiplegia, multiple sclerosis, blood clots).
• Presence of systemic disease (cancer, metabolic syndrome).
• Presence of spinal abnormality (spinal stenosis, fracture, infection, tumor, or lumbar scoliosis greater than 10 degrees).
• BMI > 30 [subcutaneous fat attenuates ultrasound signal and can invalidate measurements in obese individuals (30)].
• Presence of cardiac arrhythmia.
• Pregnant and breastfeeding women.
• Individuals with epilepsy.
• Individuals at risk for serious bleeding.
• Individuals with pacemakers or metal implants.
• Individuals with aneurysms or heart valve clips.
• Individuals who have taken prescribed muscle relaxants more than once a week in the previous month.
2.3. Procedures
All potential participants were screened for eligibility via phone interview. Individuals who passed the initial phone screening were invited to participate in a trial visit. Upon arrival, the participants were asked to sign a consent form and a physical exam was performed to rule out signs of nerve root compression. Afterward, participants completed a sociodemographic questionnaire and outcomes questionnaires. Finally, participants received a 10-minute familiarization treatment with the StimaWELL 120MTRS system, set for “combined” lumbar muscle therapy. Subsequently, participants were randomized into two groups (1:1), the phasic intervention group and the combined (tonic and phasic) intervention group, using consecutively sealed opaque envelopes created by a third party. Participants in both groups received therapy with one of the StimaWELL 120 MTRS system's (Figure 1) pre-set muscle therapy programs. The phasic group received phasic muscle stimulation of the lumbar spine (3 kHz, modulation 50 Hz). The combined group received phasic and tonic muscle stimulation of the lumbar spine (3 kHz, modulation 4 and 50 Hz).
In-person activities, including neurological testing and intervention administration, were carried out by a PhD student who is a certified athletic therapist. A certified technician from the School of Health (Concordia University) performed the MRI examinations. Due to methodological constraints, the PhD student was not blinded to group allocation.
2.3.1. Calibration
The StimaWELL 120MTRS system can deliver current through up to 12 channels, however, the current may not be felt equally across all channels in cases of pain or injury. Therefore, the wave-mat was calibrated before the trial to ensure equal current distribution across all channels. Participants were asked to lie on a towel that had been sprayed with warm water and positioned on top of the wave-mat, with their knees bent and their upper clothing removed (including their bra, if applicable). A blanket was provided for privacy. Participants were positioned on the wave-mat so that the base of their lumbar spine was in contact with the inferior-most channel. During the calibration process, the examiner increased the intensity until participants felt a strong but non-painful sensation. Then, the intensity was adjusted channel by channel, from bottom to top, to make sure each lumbar channel was set to the appropriate intensity. Finally, the current was adjusted from side to side in groups of two channels, from bottom to top, to ensure equal intensity from one side to the other. This process was repeated before participants' 5th, 9th, 13th, and 17th treatments to account for unilateral and segmental changes and ensure proper stimulation of the ES.
2.3.2. Treatment
Treatments with both the phasic and combined settings of the StimaWELL 120MTRS activate the 4 inferior-most channels of the wave-mat. Since the length of each channel spans 1–2 vertebral segments (depending on individual anatomy), the entirety of the lumbar ES (from L1–L2 to L5–S1) is stimulated by the treatment. However, since participants lie supine with the lumbar region in direct contact with the wave-mat, it is not possible to known the precise points of NMES application. To ensure that the ES was appropriately stimulated, current intensity was increased to tolerance (“strong but comfortable”) for each treatment, and the operator asked participants if they felt contractions or pulsations in their lower back. The intensity of stimulation was meant to feel similar to patients across all four lumbar channels. There was no deliberate attempt to induce a higher stimulation intensity in the upper lumbar spine. If participants reported that the stimulation intensity felt uneven, this was adjusted immediately; otherwise, channel parameters were adjusted, if needed, during the next calibration session.
2.3.3. Timeline
The participants in both groups received treatment twice a week for a period of 10 weeks. The treatment lasted 20 min for weeks 1–3, 25 min for weeks 4–6, and 30 min for weeks 7–10. After completion of the therapy program, participants were scheduled for a post-intervention visit to complete the follow-up MRI evaluation.
2.3.4. MRI assessment
All participants underwent lumbosacral MRI evaluation at baseline and post-intervention using the School of Health 3-T GE machine to determine ES muscle CSA and FI. MR images were collected using a standard phased-array body coil with 4-mm slice thickness, 180-mm2 field of view, and 512 × 512 matrix. Bilateral axial T2 weighted images were used to obtain quantitative ES muscle measurements at the L1–L2 to L5–S1 spinal levels. The ES muscle CSA was measured manually at mid-disc for each spinal level. To determine ES muscle composition (e.g., FI), we used IDEAL (Lava-flex, 2 echo sequence) fat and water images to calculate the percent-fat signal fraction at each spinal level using the following equation: %FSF = (Signalfat/[Signalwater + SignalFat] × 100) (31). All CSA and FI measurements were performed by an Athletic Therapist honours student, who was trained by an experienced researcher with more than 14 years of experience in spine imaging analysis.
2.4. Statistical analysis
Intra-rater reliability for ES measurements (CSA and FI) was investigated using interclass correlation coefficient (ICC) with a two-way mixed model and absolute agreement. A random sample of 10 participants was used and ES measurements were acquired bilaterally at all spinal levels. The ICCs were interpreted based on Portney and Watkins using a poor (<0.50), moderate (0.50–0.75), good (0.75–0.90) or excellent (>0.90) reliability scale (32). Descriptive statistics, including means and standard deviations, were calculated for bilateral CSA and FI at each spinal level as well as for baseline demographic and clinical characteristics. Paired t-tests were used to assess possible changes in ES muscle CSA and FI following the 10-week NMES intervention (irrespective of groups), and independent t-tests were used to assess between-group changes (e.g., % change of phasic vs. combined). When the data was not normally distributed, Wilcoxon Signed Rank Tests and the Mann-Whitney U-Tests were used. In all cases, the accepted level of significance was 5%. Statistical analysis was performed using Statistical Package for the Social Sciences version 23.0 (SPSS Inc, Chicago, Illinois).
3. Results
3.1. Reliability of erector spinae muscle measurements
The ICCs for erector spinae measurements (both CSA and FI) at all spinal levels were excellent and varied between 0.928 and 0.999.
3.2. Baseline demographic and clinical characteristics
Means and standard deviations are presented for all baseline demographic and clinical characteristics in Table 1. Both groups were comparable for BMI, sex and age (Table 1). Statistical analyses showed no significant difference in baseline demographic data between the phasic and combined groups (p > 0.05), allowing intergroup comparison.
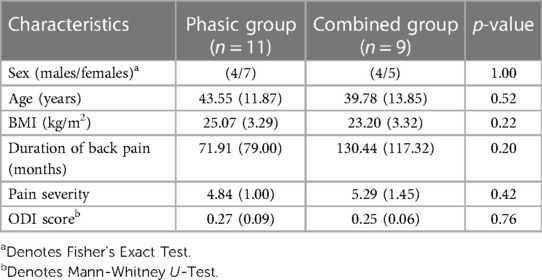
Table 1. Mean and standard deviation of participants’ baseline demographic and clinical characteristics.
3.3. Effect of NMES treatment on ES CSA and FI irrespective of group
Tables 2, 3 show means and standard deviations for CSA and FI changes from pre- to post-intervention of the entire sample (n = 20). Except for a significant decrease in the left ES CSA at L2–L3 [mean difference = −0.35 (−0.60, −0.10); p = 0.005] and a decrease in the left ES FI at L5–S1 [mean difference = −1.65 (−3.21, −0.09); p = 0.040], no other significant changes were found. Figures 2, 3 also illustrate the mean CSA and FI changes from pre- to post-intervention of the entire sample.
3.4. Between-group changes in ES CSA and FI
Tables 4, 5 show the mean percentage change (e.g., pre- to post-intervention) and standard deviations for CSA and FI changes between the phasic and the combined group. Except for an observed increased effectiveness in the combined group compared to the phasic group in the right L3–L4 (p = 0.020) and L4–L5 [mean difference = 18.17 (2.61, 33.73); p = 0.015] ES FI, no other significant changes were found.
4. Discussion
Our findings suggest that a 10-week StimaWELL 120MTRS NMES therapy led to minimal changes in ES CSA and FI in patients with CLBP. Only a significant decrease in CSA at the right L2–L3 ES was observed, which highlights the lack of consistency of the effect of NMES between levels and sides. These results are inconsistent with previous reports stating that NMES increases CSA in healthy and diseased populations (33). These discrepancies could be due to various methodological factors described below. In terms of muscle composition, a significant decrease in FI was only observable at the left ES at L5–S1. The latter findings may be due to the higher levels of FI pre-intervention on this side as compared to the right side. Higher paraspinal muscle FI values on the left side in CLBP was also reported in a recent study (34) Our findings regarding the reversibility of FI is aligned with a recent systematic review which investigated the effect of exercise therapy on FI in CLBP patients (35). This systematic review, however, was based on a small number of available studies, and highlighted several methodological concerns (e.g., study design) and clinical heterogeneity (e.g., imaging acquisition methods) (35). To our knowledge, no other study has measured the effect of NMES therapy on FI in any muscle.
Our results did not show major differences in CSA or FI changes between the phasic and combined stimulation of the StimaWELL 120MTRS device to participants with CLBP. A greater decrease in FI was observed in the combined group at the right L3–L4 and L4–L5 ES. The minimal detectable change at 95% confidence for LM CSA post-treatment is 100 mm2 in patients with CLBP (36). This relatively large amount of perceivable change could explain why no significant difference in ES CSA was observed. There is no known value for ES FI minimal detectable change, however the presence of FI in paraspinal muscle has been associated with inflammatory dysregulation (37). While some animal models have studied the anti-inflammatory properties of omega-3 fatty acids in reducing inflammation and promoting neurodegeneration in spinal cord injury (38), the therapeutic effect of such intervention in patients remains to be investigated.
Both the phasic and the combined group included a frequency of 50 Hz, which could explain the similarities in the outcome between the groups. A frequency between 25 and 75 Hz is considered optimal for increasing muscle force—thus promoting hypertrophy—as it creates full tetanic contraction of the muscle (33). Lower non-tetanic frequencies between 2 and 10 Hz lead to low-force muscle twitches but high metabolic demand, which can in turn lead to an increase in oxidative capacity and a shift from type IIx fibers to type IIa fibers (33). In our study, the protocols were differentiated by the presence of a low-frequency signal in the combined group, but no difference in CSA or FI change scores was observed between the protocols.
To the best of our knowledge, only one study assessed the effect of NMES on paraspinal CSA. Jandova et al. (2020) used ultrasound to assess paraspinal muscle morphology and reported a 5.6% (left) and 7.1% (right) increase in LM CSA following 8-weeks of NMES muscle training in healthy individuals aged ≥65 years-old (23). Two other ultrasound studies with similar protocols measured the LM muscle thickness of women (aged 18–30) with CLBP (39, 40). They both noted an increase in the L4 and L5 LM muscle segments after 12 sessions of NMES therapy over 4 weeks (39, 40). Batistella et al. (2020) reported a lower baseline CS thickness in their NMES group compared to their control group at baseline (p = 0.031). But the difference resolved in the subsequent evaluations (week 4: p = 0.229; week 8: p = 0.195), suggesting that NMES increased LM thickness (39). Additionally, Pelegrini et al. (2019) reported no difference in LM thickness between their NMES and control groups at baseline (p = 0.110) but significant differences in the subsequent evaluations (week 4: p = 0.005; week 8: p = 0.016), also suggesting an increase in thickness following NMES therapy (40). Thickness correlates with CSA in the LM at L4 and L5 in males and females with CLBP (41). In comparison to our study, Pelegrini et al. (2019) used a frequency of 1 kHz, modulated at 50 Hz, with a burst duration of 4 milliseconds whereas Batistella et al. (2020) used a frequency of 2,500 Hz, modulated at 50 Hz, 50% cycle, at rise/one-decay/off times—respectively 2, 8, 2, 12 s (40). Both used an Ibramed® device (Amparo, Sao Paulo, Brazil). This difference in NMES parameters could have impacted the outcome. The difference in imaging modalities (e.g., ultrasound vs. MRI) may have also contributed to the inconsistent findings. Our study used MRI, considered the gold standard to assess skeletal muscle morphology (11).
Compared to our intervention which involved 2 NMES sessions per week, prior protocols have consisted of 3 or more NMES sessions per week (39, 40). Greater improvement in quadriceps femoris strength was reported with 3 NMES sessions / week compared to 2 NMES sessions / week (42), and some NMES protocols recommend up to 2 sessions per day with portable units (33). Which is not feasible with the StimaWELL 120MTRS device. Although improvements in muscle size have been found after only 6, and 8 weeks of NMES therapy (33), these protocols included 3–5 treatments per week (43–48).
4.1. Study strengths
To our knowledge, this study was the first to investigate the impact of NMES on ES CSA. It was also the first to document the impact of NMES on the FI of skeletal muscle. Another strength of this study was the utilization of MRI, which is considered the gold standard for imaging spinal muscles (11).
4.2. Study limitations and future considerations
A major limitation of this study was the absence of a control group, which hindered our ability to assess the effectiveness of our NMES intervention in slowing ES atrophy in CLBP patients. The absence of a sham group also prevented assessment of the placebo effect, which is present when assessing treatment effects in clinical practice (49). Unlike other NMES devices, the StimaWELL 120MTRS wave-mat does not allow for targeted activation of specific muscles, which may have affected the specificity of our intervention. Additionally, the small number weekly treatments may have limited our results. A total of 2 NMES treatments per week was used to maximize the feasibility and favor patients' compliance. Individuals with a BMI > 30 were excluded because excessive fat can impact the validity of ultrasound measurements, which were taken for our larger RCT (25). We also excluded individuals over 60 years old, as intramuscular fat naturally increases with age. Another limitation is that sample size calculation was not performed for the current study and only for the broader ongoing RCT (25), as the present study was a preliminary analysis. Lastly, only the ES was investigated in the current study. The effect of this NMES intervention on the multifidus muscle is currently being investigated in our broader RCT (25). Due to the location of the NMES application with the wave-mat, it is unlikely that the treatment had an appreciated effect on the psoas muscle, and thus this muscle was not investigated.
Although no change in the morphology of the ES muscle was observed, our participants may have benefited from the treatment in other ways. Similarly to voluntary exercise, NMES has been shown to induce neural adaptations at the spinal and supraspinal levels by modifying the excitability of specific neural pathways, thereby increasing strength (50). The intervention may have improved our participants' muscle function or strength despite the lack of morphological changes to the ES, but this was not directly assessed. In future studies, we recommend pairing the testing of CSA and FI with functional outcomes to better understand the benefit of NMES therapy in CLBP patients.
4.3. Clinical significance
The results of this study are important as they show the need for greater knowledge of the effects of NMES on muscle morphology, especially in patients with CLBP. The effect of NMES on paraspinal muscle morphology remains conflicting. Given our findings and the paucity of other research into the effect of NMES on ES morphology in CLBP patients, clinicians may want to prioritize the use of volitional exercise to affect change in ES morphology. However, more research is needed on optimal NMES parameters for various health conditions to build effective NMES therapy programs. Such an undertaking could have major impact on patients' quality of life and reduce the medical and financial burden caused by CLBP.
4.4. Conclusion
Following a 10-week NMES intervention, we could not find consistent differences in ES muscle CSA and FI in CLBP patients. Further research is needed to confirm our findings, and to ascertain the clinical effectiveness of NMES therapy for CLBP patients.
Data availability statement
The raw data supporting the conclusions of this article will be made available by the authors, without undue reservation.
Ethics statement
The studies involving humans were approved by Central Ethics Research Committee of the Quebec Minister of Health and Social Services. The studies were conducted in accordance with the local legislation and institutional requirements. The participants provided their written informed consent to participate in this study.
Author contributions
Conception and design: MF, DW. Data collection: DW and LB. Data analysis: LB, DW. Drafting of the manuscript: LB. Critical revision of the manuscript: DW, MF. All authors contributed to the article and approved the submitted version.
Funding
This project was funded by a MITACS Accelerate grant; the sponsor had no role in the design or execution of this study. MF is supported by the Fond de la Recherche en Santé du Québec (FRQS—chercheur boursier Junior 1).
Acknowledgments
The authors would like to acknowledge the contributions of the study participants who generously gave their time and insights to make this research possible.
Conflict of interest
The authors declare that the research was conducted in the absence of any commercial or financial relationships that could be construed as a potential conflict of interest.
The author MF declared that they were an editorial board member of Frontiers, at the time of submission. This had no impact on the peer review process and the final decision.
Publisher's note
All claims expressed in this article are solely those of the authors and do not necessarily represent those of their affiliated organizations, or those of the publisher, the editors and the reviewers. Any product that may be evaluated in this article, or claim that may be made by its manufacturer, is not guaranteed or endorsed by the publisher.
References
1. Hartvigsen J, Hancock MJ, Kongsted A, Louw Q, Ferreira ML, Genevay S, et al. What low back pain is and why we need to pay attention. Lancet. (2018) 391(10137):2356–67. doi: 10.1016/S0140-6736(18)30480-X
2. Mela A, Poniatowski ŁA, Drop B, Furtak-Niczyporuk M, Jaroszyński J, Wrona W, et al. Overview and analysis of the cost of drug programs in Poland: public payer expenditures and coverage of cancer and non-neoplastic diseases related drug therapies from 2015 to 2018 years. Front Pharmacol. (2020) 11:1123. doi: 10.3389/fphar.2020.01123
3. Patrick N, Emanski E, Knaub MA. Acute and chronic low back pain. Med Clin North Am. (2014) 98(4):777–89. doi: 10.1016/j.mcna.2014.03.005
4. Will JS, Bury DC, Miller JA. Mechanical low back pain. Am Fam Physician. (2018) 98(7):421–8. PMID: 30252425
5. MŞ E, Özcan-Ekşi EE, Orhun Ö, Turgut VU, Pamir MN. Proposal for a new scoring system for spinal degeneration: MO-FI-disc. Clin Neurol Neurosurg. (2020) 198:106120. doi: 10.1016/j.clineuro.2020.106120
6. Sakai Y, Matsui H, Ito S, Hida T, Ito K, Koshimizu H, et al. Electrophysiological function of the lumbar multifidus and erector spinae muscles in elderly patients with chronic low back pain. Clin Spine Surg. (2019) 32(1):E13–9. doi: 10.1097/BSD.0000000000000709
7. Bogduk N, Macintosh JE, Pearcy MJ. A universal model of the lumbar back muscles in the upright position. Spine. (1992) 17(8):897–913. doi: 10.1097/00007632-199208000-00007
8. Harriss AB, Brown SHM. Effects of changes in muscle activation level and spine and hip posture on erector spinae fiber orientation: muscle fiber orientation. Muscle Nerve. (2015) 51(3):426–33. doi: 10.1002/mus.24309
9. Sung PS, Lammers AR, Danial P. Different parts of erector spinae muscle fatigability in subjects with and without low back pain. Spine J. (2009) 9(2):115–20. doi: 10.1016/j.spinee.2007.11.011
10. Goubert D, Oosterwijck JV, Meeus M, Danneels L. Structural changes of lumbar muscles in non-specific low back pain: a systematic review. Pain Physician. (2016) 19(7):E985–1000. PMID: 27676689
11. Seyedhoseinpoor T, Taghipour M, Dadgoo M, Sanjari MA, Takamjani IE, Kazemnejad A, et al. Alteration of lumbar muscle morphology and composition in relation to low back pain: a systematic review and meta-analysis. Spine J. (2022) 22(4):660–76. doi: 10.1016/j.spinee.2021.10.018
12. Sions JM, Elliott JM, Pohlig RT, Hicks GE. Trunk muscle characteristics of the multifidi, erector spinae, psoas, and quadratus lumborum in older adults with and without chronic low back pain. J Orthop Sports Phys Ther. (2017) 47(3):173–9. doi: 10.2519/jospt.2017.7002
13. Goubert D, De Pauw R, Meeus M, Willems T, Cagnie B, Schouppe S, et al. Lumbar muscle structure and function in chronic versus recurrent low back pain: a cross-sectional study. Spine J. (2017) 17(9):1285–96. doi: 10.1016/j.spinee.2017.04.025
14. Özcan-Ekşi EE, Ekşi MŞ, Akçal MA. Severe lumbar intervertebral disc degeneration is associated with modic changes and fatty infiltration in the paraspinal muscles at all lumbar levels, except for L1-L2: a cross-sectional analysis of 50 symptomatic women and 50 age-matched symptomatic men. World Neurosurg. (2019) 122:e1069–77. doi: 10.1016/j.wneu.2018.10.229
15. Coppock JA, Zimmer NE, Spritzer CE, Goode AP, DeFrate LE. Automated segmentation and prediction of intervertebral disc morphology and uniaxial deformations from MRI. Osteoarthr Cartil Open. (2023) 5(3):100378. doi: 10.1016/j.ocarto.2023.100378
16. Özcan-Ekşi EE, Börekci A, MŞ E. Facet joint orientation/tropism could be associated with fatty infiltration in the lumbar paraspinal muscles. World Neurosurg. (2023) 173:e606–15. doi: 10.1016/j.wneu.2023.02.111
17. Crossman K, Mahon M, Watson PJ, Oldham JA, Cooper RG. Chronic low back pain-associated paraspinal muscle dysfunction is not the result of a constitutionally determined “adverse” fiber-type composition. Spine. (2004) 29(6):628–34. doi: 10.1097/01.BRS.0000115133.97216.EC
18. Foster NE, Anema JR, Cherkin D, Chou R, Cohen SP, Gross DP, et al. Prevention and treatment of low back pain: evidence, challenges, and promising directions. Lancet. (2018) 391(10137):2368–83. doi: 10.1016/S0140-6736(18)30489-6
19. Meziat-Filho N, Fernandez J, Castro J. Cognitive functional therapy for chronic disabling low back pain. Lancet. (2023) 401(10391):1828–9. doi: 10.1016/S0140-6736(23)00571-8
20. Hicks GE, Sions JM, Velasco TO, Manal TJ. Trunk muscle training augmented with neuromuscular electrical stimulation appears to improve function in older adults with chronic low back pain: a randomized preliminary trial. Clin J Pain. (2016) 32(10):898–906. doi: 10.1097/AJP.0000000000000348
21. Pugliese JM, Sions JM, Velasco TO, Hicks GE. Use of trunk muscle training and neuromuscular electrical stimulation to reduce pain and disability in an older adult with chronic low back pain: a case report. Physiother Theory Pract. (2019) 35(8):797–804. doi: 10.1080/09593985.2018.1457745
22. Bilek F, Deniz G, Ercan Z, Cetisli Korkmaz N, Alkan G. The effect of additional neuromuscular electrical stimulation applied to erector spinae muscles on functional capacity, balance and mobility in post-stroke patients. NeuroRehabilitation. (2020) 47(2):181–9. doi: 10.3233/NRE-203114
23. Jandova T, Narici MV, Steffl M, Bondi D, D’Amico M, Pavlu D, et al. Muscle hypertrophy and architectural changes in response to eight-week neuromuscular electrical stimulation training in healthy older people. Life. (2020) 10(9):184. doi: 10.3390/life10090184
24. Guo P, Wang JW, Tong A. Therapeutic effectiveness of neuromuscular electrical stimulation for treating patients with chronic low back pain. Medicine. (2018) 97(48):e13197. doi: 10.1097/MD.0000000000013197
25. Fortin M, Wolfe D, Dover G, Boily M. The effect of phasic versus combined neuromuscular electrical stimulation using the StimaWELL 120MTRS system on multifidus muscle morphology and function in patients with chronic low back pain: a randomized controlled trial protocol. BMC Musculoskelet Disord. (2022) 23:627. doi: 10.1186/s12891-022-05578-1
26. Vogler D, Paillex R, Norberg M, Goumoëns P, Cabri J. Cross-cultural validation of the Oswestry disability index in French. Ann Readapt Med Phys. (2008) 51(5):379–85. doi: 10.1016/j.annrmp.2008.03.006
27. Saltychev M, Mattie R, McCormick Z, Bärlund E, Laimi K. Psychometric properties of the Oswestry disability index. Int J Rehabil Res. (2017) 40:202–8. doi: 10.1097/MRR.0000000000000226
28. McMaster DT, Gill N, Cronin J, McGuigan M. The development, retention and decay rates of strength and power in elite rugby union, rugby league and American football. Sports Med. (2013) 43(5):367–84. doi: 10.1007/s40279-013-0031-3
29. Ochi E, Maruo M, Tsuchiya Y, Ishii N, Miura K, Sasaki K. Higher training frequency is important for gaining muscular strength under volume-matched training. Front Physiol. (2018) 9:744. doi: 10.3389/fphys.2018.00744
30. Sigrist RMS, Liau J, Kaffas AE, Chammas MC, Willmann JK. Ultrasound elastography: review of techniques and clinical applications. Theranostics. (2017) 7(5):1303–29. doi: 10.7150/thno.18650
31. Fortin M, Omidyeganeh M, Battié MC, Ahmad O, Rivaz H. Evaluation of an automated thresholding algorithm for the quantification of paraspinal muscle composition from MRI images. Biomed Eng Online. (2017) 16:61. doi: 10.1186/s12938-017-0350-y
32. Koo TK, Li MY. A guideline of selecting and reporting intraclass correlation coefficients for reliability research. J Chiropr Med. (2016) 15(2):155–63. doi: 10.1016/j.jcm.2016.02.012
33. Herzig D, Maffiuletti NA, Eser P. The application of neuromuscular electrical stimulation training in Various non-neurologic patient populations: a narrative review. PM R. (2015) 7(11):1167–78. doi: 10.1016/j.pmrj.2015.03.022
34. Mandelli F, Nüesch C, Zhang Y, Halbeisen F, Schären S, Mündermann A, et al. Assessing fally infiltration of paraspinal muscle in patients with lumbar spinal stenosis: Goutallier classifiation and quantiative MRI measurements. Front Neurol. (2021) 12:656487. doi: 10.3389/fneur.2021.656487
35. Wesselink EO, Pool JJM, Mollema J, Weber KA, Elliott JM, Coppieters MW, et al. Is fatty infiltration in paraspinal muscles reversible with exercise in people with low back pain? A systematic review. Eur Spine J. (2023) 32(3):787–96. doi: 10.1007/s00586-022-07471-w
36. Pinto SM, Boghra SB, Macedo LG, Zheng YP, Pang MYC, Cheung JPY, et al. Does motor control exercise restore normal morphology of lumbar multifidus muscle in people with low back pain? a systematic review. J Pain Res. (2021) 14:2543–62. doi: 10.2147/JPR.S314971
37. James G, Chen X, Diwan A, Hodges PW. Fat infiltration in the multifidus muscle is related to inflammatory cytokine expression in the muscle and epidural adipose tissue in individuals undergoing surgery for intervertebral disc herniation. Eur Spine J. (2021) 30(4):837–45. doi: 10.1007/s00586-020-06514-4
38. Turczyn P, Wojdasiewicz P, Poniatowski ŁA, Purrahman D, Maślińska M, Żurek G, et al. Omega-3 fatty acids in the treatment of spinal cord injury: untapped potential for therapeutic intervention? Mol Biol Rep. (2022) 49(11):10797–809. doi: 10.1007/s11033-022-07762-x
39. Batistella CE, Bidin F, Giacomelli I, Nunez MA, Gasoto E, de Albuquerque CE, et al. Effects of the Russian current in the treatment of low back pain in women: a randomized clinical trial. J Bodyw Mov Ther. (2020) 24(2):118–22. doi: 10.1016/j.jbmt.2019.10.009
40. Pelegrini ACA, Gasoto E, Bussolaro JM, Segatti G, de Albuquerque CE, Bertolini GRF. The analgesic action of Aussie current in women with non-specific chronic lumbar pain. Int J Ther Rehabil. (2019) 26(7):1–10. doi: 10.12968/ijtr.2018.0063
41. Stokes M, Hides J, Elliott J, Kiesel K, Hodges P. Rehabilitative ultrasound imaging of the posterior paraspinal muscles. J Orthop Sports Phys Ther. (2007) 37(10):581–95. doi: 10.2519/jospt.2007.2599
42. Parker MG, Bennett MJ, Hieb MA, Hollar AC, Roe AA. Strength response in human quadriceps femoris muscle during 2 neuromuscular electrical stimulation programs. J Orthop Sports Phys Ther. (2003) 33(12):719–26. doi: 10.2519/jospt.2003.33.12.719
43. Gondin J, Guette M, Ballay Y, Martin A. Electromyostimulation training effects on neural drive and muscle architecture. Med Sci Sports Exerc. (2005) 37(8):1291–9. doi: 10.1249/01.mss.0000175090.49048.41
44. Herrero JA, Izquierdo M, Maffiuletti NA, García-López J. Electromyostimulation and plyometric training effects on jumping and sprint time. Int J Sports Med. (2006) 27(7):533–9. doi: 10.1055/s-2005-865845
45. Maillefert JF, Eicher JC, Walker P, Dulieu V, Rouhier-Marcer I, Branly F, et al. Effects of low-frequency electrical stimulation of quadriceps and calf muscles in patients with chronic heart failure. J Cardiopulm Rehabil Prev. (1998) 18(4):277. doi: 10.1097/00008483-199807000-00004
46. Martin L, Cometti G, Pousson M, Morlon B. The influence of electrostimulation on mechanical and morphological characteristics of the triceps surae. J Sports Sci. (1994) 12(4):377–81. doi: 10.1080/02640419408732184
47. Quittan M, Wiesinger GF, Sturm B, Puig S, Mayr W, Sochor A, et al. Improvement of thigh muscles by neuromuscular electrical stimulation in patients with refractory heart failure: a single-blind, randomized, controlled trial. Am J Phys Med Rehabil. (2001) 80(3):206–14. doi: 10.1097/00002060-200103000-00011
48. Vivodtzev I, Debigaré R, Gagnon P, Mainguy V, Saey D, Dubé A, et al. Functional and muscular effects of neuromuscular electrical stimulation in patients with severe COPD: a randomized clinical trial. Chest. (2012) 141(3):716–25. doi: 10.1378/chest.11-0839
49. Hafliðadóttir SH, Juhl CB, Nielsen SM, Henriksen M, Harris IA, Bliddal H, et al. Placebo response and effect in randomized clinical trials: meta-research with focus on contextual effects. Trials. (2021) 22(1):493. doi: 10.1186/s13063-021-05454-8
Keywords: paraspinal muscle, erector spinae muscle, cross-sectional area, fatty infiltration, magnetic resonance imaging
Citation: Balthazard L, Wolfe D and Fortin M (2023) Effect of neuromuscular electrical stimulation therapy using the StimaWELL 120MTRS system on erector spinae morphology in patients with chronic low back pain. Front. Musculoskelet. Disord. 1:1240331. doi: 10.3389/fmscd.2023.1240331
Received: 14 June 2023; Accepted: 28 August 2023;
Published: 13 December 2023.
Edited by:
Emel Ece Özcan Ekşi, Bahçeşehir University, TürkiyeReviewed by:
Marco Ulises Martínez-Martinez, Mexican Social Security Institute (IMSS), MexicoŁukasz A. Poniatowski, Medical University of Warsaw, Poland
© 2023 Balthazard, Wolfe and Fortin. This is an open-access article distributed under the terms of the Creative Commons Attribution License (CC BY). The use, distribution or reproduction in other forums is permitted, provided the original author(s) and the copyright owner(s) are credited and that the original publication in this journal is cited, in accordance with accepted academic practice. No use, distribution or reproduction is permitted which does not comply with these terms.
*Correspondence: Maryse Fortin maryse.fortin@concordia.ca
Abbreviations CLBP, chronic low-back pain; ES, erector spinae; CSA, cross-sectional area; FI, fat infiltration; LM, lumbar multifidus; BMI, body mass index; RCT, randomized controlled trial; MRI, magnetic resonance imaging.