- 1Department Health Kinesiology and Applied Physiology, Concordia University, Montreal, QC, Canada
- 2School of Health, Concordia University, Montreal, QC, Canada
- 3Centre de Recherche Interdisciplinaire en Réadaptation (CRIR), Montreal, QC, Canada
Introduction: Body composition is well known to affect sport performance and previous studies suggested that structural and functional lumbar multifidus (LM) impairments in athletes were associated with low back pain and lower leg injuries. However few studies have examined the relationship between LM characteristics and body composition in athletic populations.
Methods: This cross-sectional study included a total of 134 university varsity athletes (hockey, soccer, rugby, and football players). Ultrasound imaging was used to examined LM characteristics at the L5 bilaterally [e.g., size, thickness at rest, thickness during contraction, echo-intensity (EI) and % thickness change from rest to contraction] and body composition parameters (dual-energy x-ray absorptiometry). Pearson correlations were used to assess the relationship between LM characteristics and body composition parameters. One-way ANOVA assessed differences in LM characteristics and body composition between sports. All analyses were performed separately by sex.
Results: LM size and thickness were positively correlated with weight, height, lean body mass and total bone mass (male: r = 0.23–0.55, p < 0.01–0.05; female: r = 0.30–0.39, p < 0.01–0.05). LM EI was strongly correlated with % body fat (male: r = 0.62, female: r = 0.71, p < 0.01). LM thickness at rest (r = 0.42, p < 0.01) and contracted (r = 0.27, p < 0.05) were positively correlated and % thickness change was negatively correlated with % body fat in male athletes (r = −0.43, p < 0.01).
Discussion: The significant differences in body composition and LM characteristics between sports may be attributed to sport specific demands. Understanding connections between body composition and LM may aid in preseason screening for athletes at risk of low back pain or lower leg injuries during the season.
1. Introduction
Body composition provides insights into an individual's fat tissue, lean tissue, and bone density, and can be used to determine an individual's health status or establish a baseline, which is common in collegiate level athletes (1–5). Body composition parameters are associated with both performance and incidence of injury in collegiate athletes (6–8), with differences in body composition between sports (1–6, 9), between positions of the same sport (1, 8, 10, 11), and between competition levels of the same sport (12). While there are several ways to measure body composition, dual energy x-ray absorptiometry (DEXA) is the gold standard. DEXA is suitable for most athletes, is fast, non-invasive, and provides regional body composition (i.e., separation into arms, legs, trunk, etc.) for several measures including, but not limited to, bone mass, lean mass, and fat mass (13). As body composition is an important part of the overall health of an athlete, it can be monitored and provide useful information for coaches, trainers, and other health professionals involved in tailoring training programs to an athlete's specific needs.
Ultrasound (US) is a valid and reliable way to assess muscle characteristics (14, 15). Previously, it has been used to investigate muscle cross-sectional area (CSA), thickness, % thickness change, and echo intensity (EI) in athletes (16–19). CSA is a measure of muscle size, thickness is how thick the muscle is at rest or during contraction, % thickness change is the difference observed between a resting and contracted state of the muscle, and EI is the mean pixel intensity of a region of interest on an US image, which can be used as an indicator of muscle quality (e.g., degree of intramuscular fatty infiltration and connective tissue) (20, 21). In general, muscles that are larger, thicker, and have less intramuscular fat demonstrate greater strength and power (7, 22). Higher EI can decrease muscle quality as muscle fibres are replaced by fat (23). Thus, improvement in muscle EI and size may be important for performance and injury prevention. US imaging and body composition assessments may be a feasible way to monitor the effect of training adaptations in athletes.
The lumbar multifidus (LM) muscle provides stabilization and proprioceptive feedback of the lumbar spine and force transferal of the extremities through the kinetic chain (24, 25). Changes in the morphology and function of LM have been investigated in both athletic (17, 19, 26, 27) and non-athletic (28, 29) populations with and without low back pain (LBP) and lower limb injury (LLI). While athletes with LBP and LLI tend to have decreased LM CSA and thickness and increased LM CSA asymmetry, EI, and % thickness change (17, 18, 30, 31), results remain inconsistent (32, 33). LM morphology is also influenced by age, gender, height, and weight, and comprehensive studies evaluating the relationship between body composition and LM characteristics in athletes are limited (16, 17, 19, 26).
Therefore, the purpose of this study was to evaluate the relationship between body composition and LM muscle characteristics in a large sample of male and female university level varsity athletes. A secondary objective was to examine differences in body composition and LM characteristics between sports according to sex. We hypothesized that LM CSA and thickness will have a negative correlation with fat mass and % body fat and a positive correlation with lean tissue mass. We also hypothesized that increased LM EI and % thickness change will have a positive correlation with fat mass and % body fat and a negative correlation with lean mass. Finally, we hypothesized that LM CSA would be larger in football and hockey players and that soccer athletes would have the lowest fat mass and % body fat compared to the other sports investigated.
2. Materials and methods
2.1. Participants
This retrospective cross-sectional study (e.g., secondary analysis) included pre-season measurements from 134 participants (50 female, 84 male) from four varsity teams at Concordia University, Montreal, Quebec (ice hockey players (32; 18 female, 14 male), football players (41; all male), soccer players (27; 12 female, 15 male), and rugby players (34; 20 female, 14 male). Specific training load for each sport was not asked, however on average, the varsity teams practice 5–6 times per week with additional lifting sessions approximately 2–3 times per week. All available players from each team were invited to participate to maximize the sample size. The exclusion criteria included previous severe trauma or spinal fracture, previous spinal surgery, observable spinal abnormalities, and pregnancy. The study was approved by the Central Ethics Committee of the Quebec Minister of Health and Social Services. All players provided written informed consent.
2.2. Design and procedures
2.2.1. DEXA
All participants had a full body DEXA scan (Lunar Prodigy Advance, GE, USA) performed by a certified medical imaging technologist. Participants wore loose fitting clothing and removed any metal to avoid interference with the scan. Age, height, weight, and ethnicity were entered into the computer program prior to imaging. Participants were supine with their arms held slightly away from the body with thumbs pointed upwards and legs slightly apart with toes pointed upwards. Total lean mass, arm lean mass, leg lean mass, total bone mass, total fat mass, and total % body fat were obtained.
2.2.2. Ultrasound
US B-mode images of LM were acquired using a LOGIQ e ultrasound machine (GE Healthcare, Milwaukee, WI) with a 5 MHz curvilinear transducer. The imaging parameters were consistent for all acquisitions (frequency: 5 MHz, gain: 60, depth: 8.0 cm).
2.2.3. LM CSA & thickness measurements
Bilateral transverse images of the right and left LM CSA at L5 were obtained in prone position. In athletes with larger muscles, the right and left sides were imaged separately (Figure 1). Parasagittal images of the right and left were used to assess L5 LM thickness at rest and during a submaximal contraction via contralateral arm lift (CAL) in prone position (Figure 2). The handheld weight used for the CAL was based on the participant's body weight (<68.2 kg = 0.68 kg, 68.2–90.9 kg = 0.9 kg, > 90.0 kg = 1.36 kg). The region of interest (ROI) for LM CSA was traced using the following borders: spinous process, lamina, longissimus muscle, and thoracolumbar fascia. EI was also acquired from this ROI. LM thickness was measured from the tip of the spinous process to the thoracolumbar fascia. The measurement techniques used are described in detail elsewhere (16).
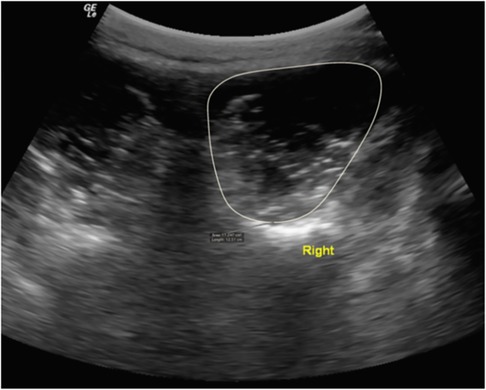
Figure 1. LM CSA measurement in a male soccer player at the L5 vertebral level in prone. Permission has been acquired for use of this photo (17).
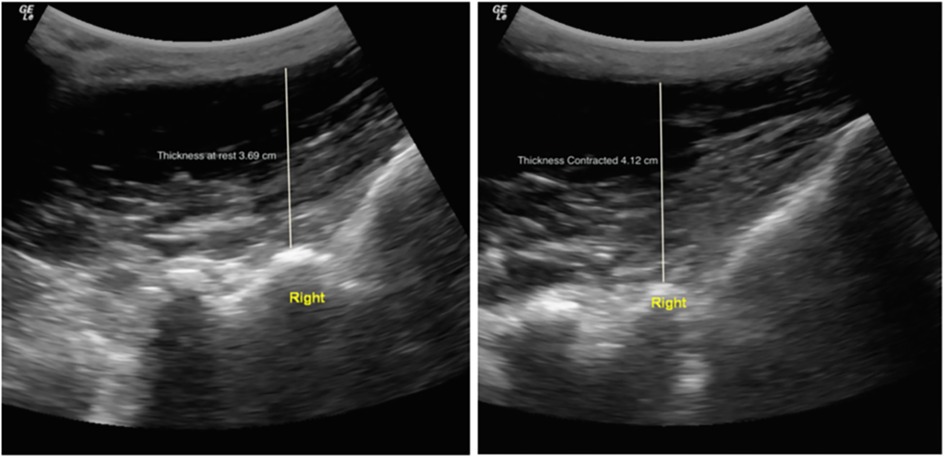
Figure 2. LM thickness measurement in a male soccer player at L5-S1 at rest (left) and during contraction (right) via a CAL in prone. Permission has been acquired for use of this photo (17).
2.2.4. Imaging assessment
US images were stored and analyzed offline using OsiriX imaging software (OsiriXLiteVersion 9.0, Geneva, Switzerland). LM CSA was obtained by tracing the muscle borders on both sides (LM borders: paraspinals, laminae, and thoracolumbar fascia). The relative % asymmetry in CSA between right and left sides was calculated using the following formula: [(larger side—smaller side)/larger side]x100%. LM thickness at rest and contracted in both in prone and standing positions were obtained using linear measurements from the tip of the L5/S1 zygoapophyseal joint to the inside edge of the superior muscle border. The following formula was used to assess LM contraction: [(thicknesscontraction—thicknessrest)/thicknessrest]×100. LM EI was measured using grayscale analysis imaging (ImageJ, National Institute of Health, USA, Version 1.49) by tracing a region of interest representing LM CSA in prone. A standard histogram function of pixels was used (0 = black, 255 = white). All measurements were performed 3 times on 3 different images and the average was used in subsequent analyses.
The intra-class correlation coefficients (ICC) for LM characteristics was investigated in a related study and ranged from 0.96–0.99 with a standard error of measurement (SEM) of 0.04–0.14 cm2 and LM EI measurements had a reliability of 0.99 with SEM of 1.97 (16).
2.3. Statistical analysis
All statistical analyses were performed with SPSS (IBM SPSS Statistics, New York, USA, v.29.0.0.0). Means and standard deviations were calculated for athletes' body composition measurements and LM characteristics. Pearson's correlation coefficients were used to examine the correlation between body composition and LM measurements in female and male athletes, separately. A one-way ANOVA was performed to examine the differences of body composition and LM characteristics between sports in male and female athletes separately. Post-hoc tests were only completed for variables with a p < 0.05. All significant female variables were run through a Tukey post-hoc test. In males, a Games-Howell post-hoc test was used for significant variables without equal variance and a Hochberg post-hoc test was used for significant variables with equal variance, as the sample size differed between groups.
3. Results
3.1. Demographics
The average age of female and male athletes were 21.2 ± 1.8 years and 20.9 ± 1.4 years, respectively. The average BMI of female and male athletes were 24.6 ± 2.7 and 26.8 ± 4.6, respectively. Female athletes played at the competitive level for an average of 7.3 ± 3.7 years and at the university level for an average of 2.2 ± 1.5 years. Male athletes played at the competitive level for an average of 8.6 ± 3.9 years and at the university level for an average of 1.5 ± 1.6 years.
3.2. Correlation analyses
The correlation matrix between body composition values and LM characteristics in females is presented in Table 1. The correlation matrix between body composition values and LM characteristics in males is presented in Table 2.
3.3. Body composition differences between sports
The results of the ANOVA for body composition and LM measurements in female players by sport are presented in Table 3. Female soccer players had significantly less total lean mass (F2,47 = 3.918, p = 0.027) compared to rugby and hockey players with an average of 4.94 kg less lean mass (95% CI: −9.56, −0.32; p = 0.034) and 4.73 kg less lean mass (95% CI: −9.45, −0.02; p = 0.049), respectively. Hockey players had significantly greater LM CSA (F2,46 = 6.665, p = 0.003) compared to rugby and soccer with an average increase of 1.36 cm2 (95% CI: 0.42, 2.30; p = 0.003) and 1.12 cm2 (95% CI: 0.05, 2.18; p = 0.038), respectively. Hockey players also had significantly thicker LM at rest (F2,47 = 3.778, p = 0.03) compared to rugby with an average increase of 0.33 cm (95% CI: 0.03, 0.63; p = 0.025). There was no significant difference in weight, height, total fat mass, % body fat, % thickness change or EI between sports.
The results of the ANOVA for body composition and LM characteristics in males by sport are presented in Table 4. Overall, football players had significantly larger values in all body composition measurements compared to soccer players, except for height. There was a statistically significant different in weight between groups (F3,80 = 9.120, p < 0.001). Football players were significantly heavier than rugby and soccer players, with an average increase of 13.94 kg (95% CI: 3.19, 24.69; p = 0.006) and 22.13 kg (95% CI: 12.49, 31.77; p < 0.001), respectively. Hockey players were also significantly heavier than soccer players, with an average increase of 14.62 kg (95% CI: 7.24, 22.00; p < 0.001). Total fat mass also had a significant difference between groups (F3,80 = 4.460, p = 0.006). Soccer players had significantly less fat mass than rugby, hockey, and football players, with an average of 5.50 kg less (95% CI: −9.96,−1.05; p = 0.013), 4.69 kg less (95% CI: −8.15, −1.23; p = 0.006), and 10.28 kg less (95% CI: −16.00, −4.56; p < 0.001), respectively. There was also a significant difference in % body fat between groups (F3,80 = 3.518, p = 0.019). Soccer players also had significantly less % body fat compared to rugby and football players, with an average of 5.38% less (95% CI: −9.86, −0.90; p = 0.015) and 6.36% less (95% CI: −10.44, −2.27; p < 0.001), respectively There was a significant difference in LM thickness at rest between groups (F3,79 = 4.582, p = 0.005). Football players had significantly thicker LM at rest compared to hockey players with an average increase of 0.50 cm (95% CI: 0.09, 0.92; p = 0.009). Furthermore, there was a statistically significant difference in LM thickness contracted between groups (F3,78 = 3.293, p = 0.025). Football players also had significantly thicker LM during a contracted state compared to hockey players, with an average increase of 0.45 cm (95% CI: 0.03, 0.87; p = 0.29). There was no significant difference in height, LM CSA, EI, or % thickness change between sports.
4. Discussion
4.1. Correlation analysis
Our results showed a significant positive correlation between weight and LM CSA and thickness at rest in female athletes. LM CSA was also significantly positively correlated with total lean mass, total bone mass, and height in females. Compared to previously published normative data in an older group of general population participants, our LM CSA was larger in both males and females, which is expected when comparing general population to an athletic population with greater physical activity levels (23, 34, 35). Our LM CSA results are comparable to both male and female elite weightlifters of similar age (36). In male athletes, weight was significantly positively correlated with all LM measurements, except % thickness change. A significant positive correlation in males was observed between LM CSA and thickness at rest and contracted with all body composition measurements, except for thickness at rest and height, which were not significant. Previous single sport investigations also found similar positive correlations between LM CSA and thickness, height and weight (16–19), however it was not indicated if male and female analyses were completed separately. Heavier and taller individuals may require larger and thicker muscles to support the additional weight carried and to traverse the distance from segment to segment. Males are naturally taller, heavier, and have higher lean body mass and less body fat than females, which correspond to larger and leaner muscles and may help explain the stronger correlations observed in males compared to females. Total bone mass was also significantly correlated with thickness at rest and contracted in both females and males, which was previously observed in rugby and soccer players (17, 18). Increased force production may be required for stabilization of heavier bones during movement, thus a thicker LM may be necessary to produce a stronger contraction during stabilization. However, the increased total bone mass observed with a thicker LM may be the result of Wolff's Law, whereby bone is laid down to adapt to the forces being placed on it (37).
EI is used as an indicator of intramuscular fat, using a grayscale distribution of pixel range within a selected ROI (e.g., lean muscle tissue appears darker (or hypoechoic) and adipose tissue appears whiter (or hyperechoic)). Recent work revealed moderate to strong correlations between LM intramuscular fat measured via ultrasound EI and MRI (e.g., % fat signal fraction) (38). While MRI remains the gold-standard to assess skeletal muscle intramuscular fat, US provides is a non-invasive, inexpensive, and easily accessible alternative to estimate muscle composition. Previously, EI was also strongly positively correlated with total % body fat (16, 17, 18, 19) and total fat mass (16, 19). In this study, total fat mass and % body fat were significantly positively correlated with EI in both female and male athletes. A larger amount of fat present in the body may lead to increased intramuscular fat, resulting in a greater EI. The significant negative correlation between % thickness change and total fat mass, % body fat, and weight may suggest that less fat resulted in a greater contraction, indicating the function of LM may be affected by the presence of fat in the body. EI and % thickness change were also negatively correlated, however it was not significant. In single sport research, a significant negative correlation between LM EI and % thickness change was also observed in football (19), while hockey had no association (16). Previous research in rugby athletes found a weak positive correlation between LM % thickness change and % body fat (18) and a study investigating elite athletes at the international level found a negative correlation between % body fat and fat mass and torso muscle strength (7). These results may also explain why football athletes, who had decreased LM % thickness change, had the greatest % body fat, total fat mass, and EI. Interestingly, previous studies with football and cross-country collegiate athletes found a significant negative correlation between vastus lateralis (VL) muscle CSA and EI, yet no correlation between EI and % body fat and fat mass (10, 39). This may indicate the association between EI and % body fat and fat mass may be muscle specific. Previously, paraspinal muscles have been found to be more susceptible to age-related increases in fat fraction compared to thigh muscles (40). In addition, fat typically deposits around the mid-section which may explain why LM EI and % body fat are more closely related compared to VL EI. Furthermore, LM is a small muscle compared to VL, thus the presence of intramuscular fat may have a greater impact on muscle function than would be observed in VL.
4.2. Body composition differences between sports
Overall, body composition values observed in our study for male rugby and soccer athletes were comparable to references values from a previous study that investigated body composition in collegiate athletes (4). That same study did not include football athletes and had a sample of two male hockey athletes, which may explain why values observed in the present study differed for hockey athletes (4). Recently, a study compared body composition in male football, hockey, and rugby athletes (1). Football and male rugby athletes had similar total fat mass and % body fat values to those observed in our study, however our football and male rugby athletes had less total lean mass (1). Hockey was the opposite with similar total lean mass values in males and our male hockey athletes having greater total fat mass and % body fat compared to Currier and colleagues (2019).
Our results showed that female soccer players had the smallest values for all lean mass measurements when compared to other varsity sports, which is in accordance with previous investigations (2). In males, soccer players had significantly lower fat mass compared to the other sports investigated and significantly less % body fat than rugby and football players, in addition to having the smallest body composition values overall. Soccer is an endurance sport that requires longer and more frequent bouts of running compared to power-focused sports like football, rugby and hockey, which tends to result in leaner and lighter musculature. Our results are comparable to other endurance sports that require large amounts of running with minimal stoppage, such as cross country (39) and sprinters in track and field (2, 9, 41), and other studies with soccer athletes (2, 4).
While reference values for a variety of sports have been obtained, there has been a lack of comparison between female hockey and rugby athletes to other sports in the literature when it comes to lean mass, fat mass, and % body fat (4, 9). In our study, female hockey players had a larger and thicker LM than both rugby and soccer players. The increased muscle size and thickness may be explained by the explosive nature of the sport. Increased forces through the kinetic chain during powerful, short shifts on the ice will require increased stability through the core and trunk, potentially resulting in a larger and thicker LM. Furthermore, hockey requires the athlete to maintain a stooped position for both skating and defensive stances, as compared to rugby and soccer where athletes spend more time in upright running positions. One study showed LM CSA increased as the trunk stoops forward in labourers, indicating the LM may be more activated in this position for stabilization (42). Interestingly, while female hockey players had the largest and thickest LM of the sports investigated, male hockey players had the smallest and thinnest, and thickness at rest and contracted was significantly different from football players. The differences observed in sex may be the result of sport specific biomechanics due to pelvic differences, however there is currently a lack of research investigating biomechanical sex differences in hockey players.
Our results in male athletes revealed that football players had significantly greater values in almost all body composition measurements compared to rugby and soccer players and had significantly thicker LM compared to hockey players. The greater size and stature may allow football athletes to handle the forces they experience during tackling from other large opponents as well as to have greater strength and power for the explosive movements required for quick bursts, change of direction, and blocking. The varying demands of each sport requires the body to adapt and is also reflected in position specific changes, which are most evident in football. Offensive and defensive linemen had higher fat mass, lean mass, and % body fat than other positions (10, 43), with other offensive and defensive positions that mirror each other having similar body compositions (43). However, offensive and defensive lineman only accounted for 12% of our total male sample and likely did not play a major role in our results.
4.3. Limitations
While this is the first study investigating the connection between body composition values and LM characteristics across a general sample of male and female university varsity athletes, only four sports were included (rugby, hockey, soccer, and football). Sampling from a single university limited the availability of other varsity sports not found at that university. Furthermore, no reference group was included in this study for sport comparison, preventing inferring differences between the general population and athletes. Although DEXA is the gold standard for body composition measurements, it is not commonly used in practice and a secondary body composition measurement (i.e., bioimpedance, skinfold, etc.) may have been beneficial for practical application comparisons. Lastly, we did not include MRI evaluation for fatty infiltration, which is the gold-standard for evaluating intramuscular fat in skeletal muscle.
5. Conclusions
Our study provides novel insights into connections between LM characteristics and body composition, emphasizing the importance of assessing body composition in athletic populations and presents normative data of LM characteristics for direct comparison between sports. Overall, our findings revealed differences in body composition and LM characteristics between sports in males and females, which are likely due to sport specific requirements. The results of the current study further support that body composition measures likely play a role in LM morphology and function and should be considered when investigating paraspinal musculature and exploring links with the presence of spinal pathologies. Through body composition and LM preseason screening, coaches and clinicians may be able to identify athletes with higher risk of injury, as a growing body of evidence revealed that LM characteristics are associated with low back pain and lower limb injury (16–19, 26, 27, 30, 33). Future studies should investigate if targeting athletes at risk through specific exercise intervention or dietary programs can assist in the prevention of injury during the season.
Data availability statement
The raw data supporting the conclusions of this article will be made available by the authors, without undue reservation.
Ethics statement
The studies involving humans were approved by Central Ethics Committee of the Quebec Minister of Health and Social Services. The studies were conducted in accordance with the local legislation and institutional requirements. The participants provided their written informed consent to participate in this study.
Author contributions
MF contributed to conception and design of the study. AR and SF contributed to participant recruitment and measurements. MA organized the database, performed the statistical analysis, and wrote the first draft of the manuscript. All authors contributed to manuscript revision, read, and approved the submitted version. All authors contributed to the article and approved the submitted version.
Acknowledgments
The authors would like to sincerely thank the players and coaches for taking part in this study and Sean Christensen for his help with participant recruitment.
Conflict of interest
The authors declare that the research was conducted in the absence of any commercial or financial relationships that could be construed as a potential conflict of interest.
The author(s) MF declared that they were an editorial board member of Frontiers, at the time of submission. This had no impact on the peer review process and the final decision.
Publisher's note
All claims expressed in this article are solely those of the authors and do not necessarily represent those of their affiliated organizations, or those of the publisher, the editors and the reviewers. Any product that may be evaluated in this article, or claim that may be made by its manufacturer, is not guaranteed or endorsed by the publisher.
References
1. Currier BS, Harty PS, Zabriskie HA, Stecker RA, Moon JM, Jagim AR, et al. Fat-free mass index in a diverse sample of male collegiate athletes. J Strength Cond Res. (2019) 33:1474–9. doi: 10.1519/JSC.0000000000003158
2. Stanforth PR, Crim BN, Stanforth D, Stults-Kolehmainen MA. Body composition changes among female NCAA division 1 athletes across the competitive season and over a multiyear time frame. J Strength Cond Res. (2014) 28:300–7. doi: 10.1519/JSC.0b013e3182a20f06
3. Blue MNM, Hirsch KR, Pihoker AA, Trexle ET, Smith-Ryan AE. Normative fat-free mass index values for a diverse sample of collegiate female athletes. J Sports Sci. (2019) 37:1741–5. doi: 10.1080/02640414.2019.1591575
4. Santos DA, Dawson JA, Matias CN, Rocha PM, Minderico CS, Allison DB, et al. Reference values for body composition and anthropometric measurements in athletes. PLoS ONE. (2014) 9:e97846. doi: 10.1371/journal.pone.0097846
5. Mudd LM, Fornetti W, Pivarnik JM. Bone mineral density in collegiate female athletes: comparisons among sports. J Athl Train Natl Athl Train Assoc. (2007) 42:403–8.
6. Calatayud J, Borreani S, Moya D, Colado JC, Triplett NT. Exercise to improve bone mineral density. Strength Cond J. (2013) 35:70–4. doi: 10.1519/SSC.0b013e3182980d57
7. Akınoğlu B, Kocahan T. Body composition and torso muscle strength relationship in athletes. Prog Nutr. (2019) 21:1019–28.
8. Lukaski H, Raymond-Pope CJ. New frontiers of body composition in sport. Int J Sports Med. (2021) 42:588–601. doi: 10.1055/a-1373-5881
9. Carbuhn AF, Fernandez TE, Bragg AF, Green JS, Crouse SF. Sport and training influence bone and body composition in women collegiate athletes. J Strength Cond Res. (2010) 24:1710–7. doi: 10.1519/JSC.0b013e3181d09eb3
10. Melvin MN, Smith-Ryan AE, Wingfield HL, Ryan ED, Trexler ET, Roelofs EJ. Muscle characteristics and body composition of NCAA division I football players. J Strength Cond Res. (2014) 28:3320–9. doi: 10.1519/JSC.0000000000000651
11. Czeck MA, Raymond-Pope CJ, Stanforth PR, Carbuhn A, Bosch TA, Bach CW, et al. Total and regional body composition of NCAA division I collegiate female softball athletes. Int J Sports Med. (2019) 40:645–9. doi: 10.1055/a-0962-1283
12. Vitale JA, Caumo A, Roveda E, Montaruli A, La Torre A, Battaglini CL, et al. Physical attributes and NFL combine performance tests between Italian national league and American football players: a comparative study. J Strength Cond Res. (2016) 30:2802–8. doi: 10.1519/JSC.0000000000001377
13. Nana A, Slater GJ, Stewart AD, Burke LM. Methodology review: using dual-energy x-ray absorptiometry (DXA) for the assessment of body composition in athletes and active people. Int J Sport Nutr Exerc Metab. (2015) 25:198–215. doi: 10.1123/ijsnem.2013-0228
14. Belavý DL, Armbrecht G, Felsenberg D. Real-time ultrasound measures of lumbar erector spinae and multifidus: reliability and comparison to magnetic resonance imaging. Physiol Meas. (2015) 36:2285–99. doi: 10.1088/0967-3334/36/11/2285
15. Hides JA, Richardson CA, Jull GA. Magnetic resonance imaging and ultrasonography of the lumbar multifidus muscle: comparison of two different modalities. Spine. (1995) 20:54–8. doi: 10.1097/00007632-199501000-00010
16. Fortin M, Rizk A, Frenette S, Boily M, Rivaz H. Ultrasonography of multifidus muscle morphology and function in ice hockey players with and without low back pain. Phys Ther Sport. (2019) 37:77–85. doi: 10.1016/j.ptsp.2019.03.004
17. Nandlall N, Rivaz H, Rizk A, Frenette S, Boily M, Fortin M. The effect of low back pain and lower limb injury on lumbar multifidus muscle morphology and function in university soccer players. BMC Musculoskelet Disord. (2020) 21:96. doi: 10.1186/s12891-020-3119-6
18. Lévesque J, Rivaz H, Rizk A, Frenette S, Boily M, Fortin M. Lumbar multifidus muscle characteristics, body composition, and injury in university rugby players. J Athl Train. (2020) 55:1116–23. doi: 10.4085/1062-6050-304-19
19. Schryver A, Rivaz H, Rizk A, Frenette S, Boily M, Fortin M. Ultrasonography of lumbar multifidus muscle in university American football players. Med Sci Sports Exerc. (2020) 52:1495–501. doi: 10.1249/MSS.0000000000002292
20. Young H-J, Jenkins NT, Zhao Q, Mccully KK. Measurement of intramuscular fat by muscle echo intensity: muscle echo intensity and fat. Muscle Nerve. (2015) 52:963–71. doi: 10.1002/mus.24656
21. Rummens S, Robben E, Groef AD, Wambeke PV, Janssens L, Brumagne S, et al. Factors associated with the ultrasound characteristics of the lumbar multifidus: a systematic review. PM&R. (2020) 12:82–100. doi: 10.1002/pmrj.12212
22. Seo YG, Park WH, Lee CS, Kang KC. Lumbar extensor muscle size and isometric muscle strength in women with symptomatic lumbar degenerative diseases. Asian Spine J. (2018) 12:943–50. doi: 10.31616/asj.2018.12.5.943
23. Stokes M, Hides J, Elliott J, Kiesel K, Hodges P. Rehabilitative ultrasound imaging of the posterior paraspinal muscles. J Orthop Sports Phys Ther. (2007) 37:581–95. doi: 10.2519/jospt.2007.2599
24. Hodges PW, Danneels L. Changes in structure and function of the back muscles in low back pain: different time points, observations, and mechanisms. J Orthop Sports Phys Ther. (2019) 49:464–76. doi: 10.2519/jospt.2019.8827
25. Cheung WK, Cheung JPY, Lee W-N. Role of ultrasound in low back pain: a review. Ultrasound Med Biol. (2020) 46:1344–58. doi: 10.1016/j.ultrasmedbio.2020.02.004
26. Roy A, Rivaz H, Rizk A, Frenette S, Boily M, Fortin M. Seasonal changes in lumbar multifidus muscle in university rugby players. Med Sci Sports Exerc. (2021) 53:749–55. doi: 10.1249/MSS.0000000000002514
27. Hides J, Stanton W. Muscle imbalance among elite Australian rules football players: a longitudinal study of changes in trunk muscle size. J Athl Train. (2012) 47:314–9. doi: 10.4085/1062-6050-47.3.03
28. Ranger TA, Cicuttini FM, Jensen TS, Peiris WL, Hussain SM, Fairley J, et al. Are the size and composition of the paraspinal muscles associated with low back pain? A systematic review. Spine J. (2017) 17:1729–48. doi: 10.1016/j.spinee.2017.07.002
29. Danneels L. Structural changes of lumbar muscles in non-specific low back pain. Pain Physician. (2016) 7(19):E985–E1000. doi: 10.36076/ppj/2016.19.E985
30. Hides JA, Stanton WR. Predicting football injuries using size and ratio of the multifidus and quadratus lumborum muscles. Scand J Med Sci Sports. (2017) 27:440–7. doi: 10.1111/sms.12643
31. Hides JA, Brown CT, Penfold L, Stanton WR. Screening the lumbopelvic muscles for a relationship to injury of the quadriceps, hamstrings, and adductor muscles among elite Australian football league players. J Orthop Sports Phys Ther. (2011) 41:767–75. doi: 10.2519/jospt.2011.3755
32. McGregor AH. The trunk muscles of elite oarsmen. Br J Sports Med. (2002) 36:214–6. doi: 10.1136/bjsm.36.3.214
33. Smyers Evanson A, Myrer J, Eggett D, Mitchell U, Johnson A. Multifidus muscle size and symmetry in ballroom dancers with and without low back pain. Int J Sports Med. (2018) 39:630–5. doi: 10.1055/a-0631-3111
34. Stokes M, Rankin G, Newham DJ. Ultrasound imaging of lumbar multifidus muscle: normal reference ranges for measurements and practical guidance on the technique. Man Ther. (2005) 10:116–26. doi: 10.1016/j.math.2004.08.013
35. Watson T, McPherson S, Starr K. The association of nutritional status and gender with cross-sectional area of the multifidus muscle in establishing normative data. J Man Manip Ther. (2008) 16:93E–8E. doi: 10.1179/jmt.2008.16.4.93E
36. Sitilertpisan P, Hides J, Stanton W, Paungmali A, Pirunsan U. Multifidus muscle size and symmetry among elite weightlifters. Phys Ther Sport. (2012) 13:11–5. doi: 10.1016/j.ptsp.2011.04.005
37. Frost HM. A 2003 update of bone physiology and wolff’s law for clinicians. Angle Orthod. (2004) 74:13. doi: 10.1043/0003-3219(2004)074%3C0003:AUOBPA%3E2.0.CO;2
38. Crook J, Masi S, Naghdi N, Roussac A, Rye M, Rosenstein B, et al. Comparison of multifidus muscle intramuscular fat by ultrasound echo intensity and fat-water based MR images in individuals with chronic low back pain. Musculoskelet Sci Pract. (2023) 63:102717. doi: 10.1016/j.msksp.2023.102717
39. Roelofs EJ, Smith-Ryan AE, Melvin MN, Wingfield HL, Trexler ET, Walker N. Muscle size, quality, and body composition: characteristics of division I cross-country runners. J Strength Cond Res. (2015) 29:290–6. doi: 10.1519/JSC.0000000000000729
40. Dahlqvist JR, Vissing CR, Hedermann G, Thomsen C, Vissing J. Fat replacement of paraspinal muscles with aging in healthy adults. Med Sci Sports Exerc. (2017) 49:595–601. doi: 10.1249/MSS.0000000000001119
41. Hirsch KR, Smith-Ryan AE, Trexler ET, Roelofs EJ. Body composition and muscle characteristics of division I track and field athletes. J Strength Cond Res. (2016) 30:1231–8. doi: 10.1519/JSC.0000000000001203
42. Lee S, Chan CK, Lam T, Lam C, Lau N, Lau RW, et al. Relationship between low back pain and lumbar multifidus size at different postures. Spine. (2006) 31:2258–62. doi: 10.1097/01.brs.0000232807.76033.33
Keywords: lumbar multifidus, ultrasound imaging, athletes, sports, sex differences, DEXA
Citation: Anstruther M, Rizk A, Frenette S and Fortin M (2023) Lumbar multifidus characteristics and body composition in university level athletes. Front. Musculoskelet. Disord. 1:1235114. doi: 10.3389/fmscd.2023.1235114
Received: 5 June 2023; Accepted: 8 September 2023;
Published: 30 November 2023.
Edited by:
P. Bryant Chase, Florida State University, United StatesReviewed by:
Michael Fröhlich, Technical University of Kaiserslautern, GermanyJacopo Vitale, Schulthess Klinik, Switzerland
© 2023 Anstruther, Rizk, Frenette and Fortin. This is an open-access article distributed under the terms of the Creative Commons Attribution License (CC BY). The use, distribution or reproduction in other forums is permitted, provided the original author(s) and the copyright owner(s) are credited and that the original publication in this journal is cited, in accordance with accepted academic practice. No use, distribution or reproduction is permitted which does not comply with these terms.
*Correspondence: Maryse Fortin maryse.fortin@concordia.ca