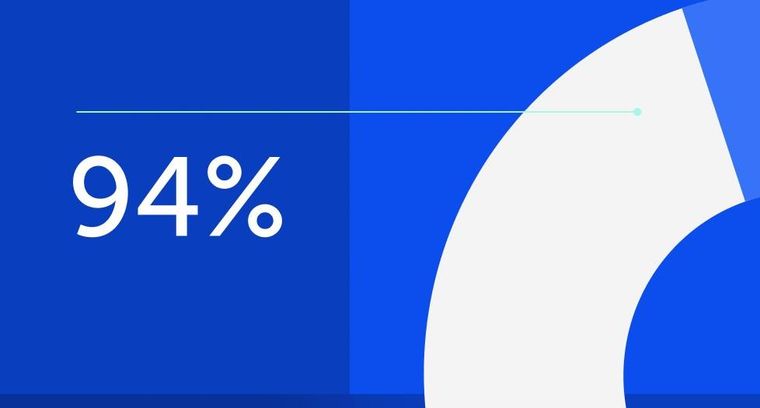
94% of researchers rate our articles as excellent or good
Learn more about the work of our research integrity team to safeguard the quality of each article we publish.
Find out more
MINI REVIEW article
Front. Mol. Neurosci., 21 March 2025
Sec. Pain Mechanisms and Modulators
Volume 18 - 2025 | https://doi.org/10.3389/fnmol.2025.1547647
Neuropathic pain causes tremendous biological and psychological suffering to patients worldwide. Environmental enrichment (EE) is a promising non-pharmacological strategy with high cost-effectiveness to reduce neuropathic pain and support rehabilitation therapy. Three researchers reviewed previous studies to determine the efficacy of EE for neuropathic pain to research how EE improves neuropathic pain through neuroinflammation. For this review, Embase, PubMed, and Cochran were searched. Three authors did study selection and data extraction. Out of 74 papers, 7 studies met the inclusion criteria. In the chronic constriction injury rats with acute or chronic detrimental stimulation, the change of pain behavior was influenced by environmental settings like start time, and cage size. Besides, physical EE has a larger effect than socially EE in inflammatory pain. These articles suggest employing various EE to regulate the release of pain-causing substances and changes in ion channels in the peripheral and central nerves to improve neuropathic pain behavior and depression and anxiety conditions. The existing proof provides important knowledge for upcoming preclinical investigations and the practical use of EE in clinical pain treatment. This analysis aids in the advancement of improved approaches for managing chronic pain, with a focus on internal mechanisms for controlling pain.
The International Association for the Study of Pain defines neuropathic pain as “pain caused by a lesion or disease of primary afferent neurons in the somatosensory nervous system.” This type of pain is associated with profound neuroinflammation in the central nervous system (CNS) and peripheral nervous system (PNS) regions (Finnerup et al., 2021). Neuropathic pain is closely linked to the activation of immune cells and the release of inflammatory mediators (Fiore et al., 2023). Following nerve injury or disease, microglia in the CNS and macrophages in the PNS become activated and release pro-inflammatory cytokines, such as TNF-α, IL-1β, and IL-6 (Fiore et al., 2023; Bhol et al., 2024). These inflammatory mediators contribute to pain hypersensitivity by sensitizing nociceptive pathways in the spinal cord and peripheral neurons, leading to central sensitization and persistent pain states (Smith, 2023; Monteiro et al., 2020). Additionally, chemokines such as CCL2 and CX3CL1 facilitate neuroimmune interactions, further exacerbating neuroinflammation and chronic pain (Zheng et al., 2024). In some cases, severe neuroinflammation can promote the continued development of nerve pain, leading to chronic persistent pain. Recent studies have demonstrated that sustained glial activation and neuroinflammatory signaling drive the transition from acute to chronic neuropathic pain, highlighting the need for effective anti-inflammatory interventions (Song et al., 2024; Echeverria-Villalobos et al., 2023). According to the global epidemiological survey (Zhao et al., 2023), neuropathic pain affects 7 to 10% of the general population; it is a major threat to patients’ lives, health, and quality of life and has brought a huge economic and public health burden to society. Neuropathic pain is a complicated disease to treat (Xing et al., 2024). At present, the main treatment is drug therapy, such as using antidepressants, anticonvulsants, and opioids (Salvemini and Doyle, 2023). However, these drugs often have limited efficacy and significant side effects, making alternative therapeutic approaches essential (Alaoui Mdarhri et al., 2022).
Environmental enrichment (EE) is a preclinical model of rehabilitation used to facilitate voluntary motor, sensory, social, and cognitive activities by providing a stimulating environment and avoiding the risk of exercise overload (Tai et al., 2021). Evidence suggests that EE can alleviate neuropathic pain by modulating neuroimmune responses and reducing neuroinflammation (Kimura et al., 2019; Kimura et al., 2020; Wang et al., 2019; Wang et al., 2019). EE is achieved mainly by improving psychosocial factors, environmental novelty, physical aspects (increased activity and exercise), and sensitization of animal mechanical sensitivity threshold (Yam et al., 2018). Moreover, EE has been shown to suppress glial activation, reduce inflammatory cytokine levels (TNF-α, IL-1β, IL-6), and enhance anti-inflammatory signaling (IL-10, TGF-β) in both the CNS and PNS, leading to reduced pain hypersensitivity and improved functional recovery (Kimura et al., 2022).
Previous reviews on EE and neuropathic pain have shown that rodents exposed to EE exhibit reduced pain sensitivity compared to those in standard environments. However, a comprehensive review of the specific mechanisms and pathways EE modulates neuropathic pain and neuroinflammation has not yet been conducted. Therefore, this review focuses on summarizing the current understanding of how EE exerts its neuroprotective and anti-inflammatory effects in neuropathic pain conditions.
Embase, PubMed, and Cochran were searched from December 1999 to December 2024 for relevant rat’s trials and neuropathic pain or neuroinflammation review. The following keywords are used as the primary targets in the search, either individually or jointly: T1 = “Environmental enrichment” OR “Enriched environment” OR “Swimming training” OR “Voluntary exercise” or “Wheel running” OR “Social activities.” T2 = “Neuroinflammation” OR “Neuropathic pain” OR “Neuroimmune” OR “Neurodegenerative dis-orders.” T3 = “Inflammatory factors” OR “Macrophage” OR “Inflammation factors” OR “Microglia.” When screening mice trials, the inclusion criteria are as follows: (1) Type of study. We included only published Randomized controlled trial articles that investigated the effects of EE on neuroinflammation and neuropathic pain in mice. The language of the article is limited to English; (2) Mice pain model. We included chronic constriction injury model mice, spinal nerve injury model mice and inflammatory pain model mice; (3) Interventions. When the mice are born or grow to a certain number of weeks, they will be divided into cages according to a certain number of cage breeding, intervention measures mainly include replacing larger cage, putting plastic cylinders, surgical caps, cardboard tubes, running wheels, tunnels of different styles and so on into the cage. After that, they change their toys every few days or weeks; (4) Types of outcome measures. The outcome measures mainly included mechanical sensitivity, temperature sensitivity, some of the experiments also measured depression, anxiety, motor capacity and inflammatory factors (for example TNF-α).
Three authors searched Embase, PubMed, and Cochran for all titles, abstracts, and text that matched the criteria, according to the screening criteria. For controversial papers, the two authors dis-cuss or let the corresponding author pass judgment. Papers that did not match the criteria for inclusion were omitted. The following information was extracted from the selected articles: (1) Published data (author, year); (2) Study design (mice pain model, sample size, control groups); (3) Intervention proto-col (including cage size, number of mice, type and number of toys, duration); (4) Outcome measures (mechanical stimulation, temperature stimulation, motor ability, etc.)
Various preclinical studies have shown the clear effect of EE with different parameter settings (Table 1) for rodents with persistent neuropathic pain and inflammation. Pain models, including chronic constriction injury (CCI), spared nerve injury (SNI), and chronic compression of dorsal root ganglion, could reflect many facets of chronic pain in humans; in particular, hypersensitivity and presence of comorbidities, such as anxiety, depression, and cognitive deficit (Mouraux et al., 2021), have been investigated, and findings are translated into clinical pain management. The components of EE are physical enrichment (voluntary wheel running), social enrichment (group housing), and multifaceted stimulation through toy provision. A cage in different sizes is an indispensable physical component in EE and is more useful than regular-sized cages (26,656 cm3) and large cages (66,000 cm3) to improve pain behavior in neuropathic models (Kimura et al., 2019; Wang et al., 2019). The free-running wheel is one of the common physical environment modalities that facilitate the status of physical activity to promote exercise-induced hypoalgesia (Greenwood and Fleshner, 2019). Townsend et al. (2022) evaluated the effect of a 12-h voluntary running, 4 days a week for 5 weeks, on pain behavior. Sessions with high levels of exercise (within 3,300–9,600 m/day) before surgery demonstrated relief of ongoing pain in rats, but not in low runners (within 200–3,150 m/day). Compared with voluntary wheel running on the forced treadmill, high running with a peak rate of 16 m/min can block pain and reverse tactile hypersensitivity (Schmitt et al., 2020). Group housing consists of social enrichment. Stickney and Morgan (2021) conducted a trial and found that the social condition of as few as two rats could promote recovery by reducing pain and pain-evoked responses; however, the upper limit of the house group remains unknown. Toy provision could provide multifaceted stimulation in rat models of pain. Different combinations of climbing platforms, balls, tubes, cylinders, and caps have a positive effect on modifying chronic pain development (Kimura et al., 2019; Kimura et al., 2020; Wang et al., 2019; Wang et al., 2019; Chen et al., 2022).
EE with a variety of novel objects and large living spaces can weaken the reduction of pain threshold, depression-like phenotype, and memory deficits in mice with neuropathic pain after CCI (Wang et al., 2019). Kimura et al. (2019) established and tested two models of EE in a similar social environment; they found that compared with simple EE (sEE), in which enrichment started after weaning in regular-size cages with three different objections on regularly change (cardboard tubes, plastic cylinders, and disposable caps), pain behavior was reduced only by improved EE (iEE) condition, in which enrichment started at birth with five different objects weekly adding in order (like ping-pong balls, acrylic tubes, and cabin) in bigger cages (66,000 cm3). This research also argued that CCI rats could abolish acute and chronic neuropathic pain behavior after living under iEE conditions for 14 days; even after weaning, introducing sEE provided sufficient stimulation to reduce animal anxiety (Kimura et al., 2019). Current research using inflammatory pain models of mice found that living in EE 2 weeks with two running wheels, three different types of tunnels, a screw-type ladder, a swing, a teeterboard, a nest made of medical cotton, and a crib (8 per cage) improved pain behavior substantially (Estrázulas et al., 2022). Most studies reported that EE could improve neuropathic pain associated with physical activity (Wang et al., 2019; Leitzelar and Koltyn, 2021; Toloui et al., 2023); however, without sports environment, such as running wheels, social and inanimate interaction as analgesic and neuroimmune manipulator could prevent nerve degeneration upon CCI because exposure to EE impedes the development of pathological pain after chronic nerve injury (Kimura et al., 2020). A physically enriched environment (PE), such as living in large cages (±220,000 cm3) with various inanimate items, such as tunnels, running wheels, and extra nesting materials, has a larger effect on inflammatory pain than a socially enriched environment (SE), such as living in a group with four rats in the social environment and standard cages (±40,000 cm3) (Sadler et al., 2022). For psychological problems, EE (physically and socially enriched) can improve depression and anxiety-like behavior in the pain model of rats compared with the standard environment; nevertheless, no significant differences were found in SE, EE, and PE groups as well as in iEE and sEE groups (Kimura et al., 2020; Parent-Vachon and Vachon, 2018). In addition, many studies showed that EE can improve psychological problems, especially depression and anxiety-like behavior (Sparling et al., 2020; Ji and Xia, 2022; Smail et al., 2020).
According to anatomical studies, pain perception is caused by nociceptive neurons, which are activated in peripheral sensory nerves to produce nociceptive signals; the signals are then transmitted through dorsal root neurons and project onto the thalamus and the cerebral cortex (Figure 1) (Li et al., 2021; Chen et al., 2021). Neuropathic pain is associated with excessive inflammatory responses in the CNS and PNS (Fiore et al., 2023) and is caused by nerve damage (Ji et al., 2018). The neuropathic pain phenotype is characterized by the initiation and maintenance of the hypersensitivity of the CNS or PNS, neuroglia, activation of macrophages and white blood cells, recruitment of peripheral immune cells, and release of inflammatory mediators, such as cytokines (IL-1β, TNF-α, IL-6), chemokines (CCL2, CX3CL1), lipid mediators (PGE2, PGI2), and reactive oxygen species (Yu et al., 2020). These substances have the undesirable effect of sensitizing and irritating nociceptors, leading to persistent neuropathic pain (Hakim et al., 2024).
Figure 1. Mechanism of environmental enrichment therapy for neuropathic pain. Activating Transcription Factor 3 (ATF3); Arginase-1(Arg-1); Brain-Derived Neurotrophic Factor (BDNF); CX3C Chemokine Ligand 1 (CX3CL1); Chemokine Ligand 2 (CCL2); Calcitonin Gene-Related Peptide (CGRP); Cluster of Differentiation 11b (CD11b); Inducible Nitric Oxide Synthase (iNOS); Ionized Calcium Binding Adaptor Molecule 1 (IBA1); Interleukin-1β (IL-1β); Interleukin-6 (IL-6); Interleukin-10 (IL-10); Nerve Growth Factor (NGF); Substance P (SP); Tropomyosin Receptor Kinase A (TrkA); Tumor Necrosis Factor Receptor 1 (TNFR1); Tumor Necrosis Factor-α (TNF-α); Transforming Growth Factor-β (TGF-β); Dorsal Root Ganglion (DRG); Environmental Enrichment (EE). Created with BioRender.com.
The dorsal root ganglion (DRG) plays a crucial role in pain transmission by relaying nociceptive signals to the spinal cord (Jang and Garraway, 2024). When peripheral nerves are injured, white blood cells, macrophages, and neuroglia in DRG are involved in immune responses, leading to pain (Rios et al., 2021; Msheik et al., 2022). EE modulates neuroinflammation in the PNS primarily by regulating immune cell activity and inflammatory signaling pathways.
The macrophage chemokine CCL2 is associated with neuropathic pain and inflammation in DRG. CCL2 consistently induces mechano-nociceptive hypersensitivity (Dansereau et al., 2021) and activates microglia after peripheral nerve injury (Popiolek-Barczyk et al., 2020). Gu et al. (2021) found that EE can reduce the expression of inflammatory factors, such as macrophage marker IBA1, microglia CD11b, and chemokines CCL2 and ATF3 (a transcription factor considered as a marker of nerve damage) in DRG to achieve pain relief. Another study (Kimura et al., 2020) found that DRG attenuated the expression of ATF3 and iNOS and increased the expression of the anti-inflammatory marker Arg-1 in CCI injury after EE. Xu et al. (2025) studied chronic neuropathic pain in rats and found the enrichment of proteins related to “antioxidant activity” in ganglia. These proteins are involved in analgesia and act on protein-coupled receptors, causing the inactivation of calcium channels to reduce pain.
Peripheral tissue or nerve injury increases the expression of BDNF in DRG (Smith, 2024). Wu et al. (2021) reported that BDNF at the level of DRG can contribute to pain transmission as well as pain hypersensitivity and central sensitization mainly because BDNF can enhance the excitability of the dorsal horn of the spinal cord and promote the transmission of pain after nerve injury mainly by regulating glutamatergic excitability and GABAergic/glycinergic to inhibit nerve conduction. Previous studies found (Thakkar and Acevedo, 2023; Huang et al., 2021) that BDNF can act as a factor that contributes to the onset and development of pain and a pain mediator that regulates pain. Thus, the release of BDNF in DRG is undesirable because it contributes to the development of nerve pain. A study reported that EE can reduce the expression of BDNF in DRG by reducing neuroglia activation, chemokine and cytokine expression, and ion channel activity to alleviate hyperalgesia after partial nerve injury to inhibit neuropathic pain (Tian et al., 2018).
Immune cells are related to the occurrence of inflammation after peripheral nerve injury (Vergne-Salle and Bertin, 2021). Inflammation drives microglia and inflammatory cells to release several proinflammatory cytokines and chemokines (such as TNF-α, IL-1β, CCL2, and CCL3). TNF-α is a key proinflammatory cytokine that binds to the receptor TNFR1 to activate the signal pathways of NF-κB and p38 MAPK and trigger inflammatory responses (Duan et al., 2022). One study found (Chu et al., 2020) that EE reduces TNF-α and IL-1β levels in the sciatic nerve, hinders the activation of inflammatory signaling pathways, and relieves peripheral neuropathic pain. Wallerian degeneration occurs after peripheral nerve injury and is an important degenerative process that occurs at the distal stump of the nerve in response to nerve fiber damage (Tian et al., 2025). Kimura et al. (2020) found that an EE could reduce the occurrence of Wallerian degeneration by significantly retaining myelinated nerve fibers in the proximal part of the sciatic nerve with CCI, thereby affecting the reduction of nerve pain in sciatic nerves. In another study (Kimura et al., 2020), researchers found that EE can promote the expression of Arg-1, an enzyme that initiates downstream pathways associated with cell proliferation and tissue repair. As such, changes in Wallerian degeneration might be related to Arg-1. Yu et al. (2020) found increased Arg-1 expression in the sciatic nerve after EE and decreased expression of the macrophage markers Iba-1 and CCL2 while significantly ameliorating neuropathic pain in CCI mice. Microglia activation is mainly divided into M1 and M2. The activation of M1 is a pro-inflammatory state, which releases cytokines and chemokines, while the activation of M2 is an anti-inflammatory state, which releases Arg-1 and IL-10. M2 activation promotes the release of anti-inflammatory cytokines, such as IL-10 and TGF-β, and induces Arg-1, which promotes the conversion of arginine into polyamines to ease the onset of inflammation (Arabpour et al., 2021).
In the peripheral nervous system, one major pathway involved is the NF-κB signaling pathway, which is crucial for the production of pro-inflammatory cytokines such as TNF-α and IL-1β (Guo et al., 2024). Peripheral nerve injury leads to NF-κB activation through the phosphorylation and degradation of IκBα, allowing p65/p50 nuclear translocation and subsequent transcription of inflammatory mediators (Giridharan and Srinivasan, 2018). Studies have shown that EE downregulates NF-κB activation in DRG by inhibiting the phosphorylation of IKK complex, reducing TNF-α and IL-1β expression (Wu et al., 2020). Additionally, the MAPK signaling pathway is another critical target of EE in the PNS. The activation of p38 MAPK in response to nerve injury enhances pro-inflammatory cytokine release, leading to nociceptor sensitization (Falcicchia et al., 2020). EE has been demonstrated to suppress p38 MAPK phosphorylation, thereby attenuating the inflammatory cascade in DRG neurons and associated macrophages (Martina et al., 2023). Furthermore, EE influences the JAK/STAT pathway, particularly by downregulating STAT3 phosphorylation, which is known to promote IL-6 expression and neuroinflammation (Lv et al., 2024). Another essential mechanism of EE in the PNS is its regulation of immune cell polarization (Udit et al., 2022). EE exposure has been linked to increased expression of Arg-1, a marker of M2 anti-inflammatory macrophages while reducing IBA-1 expression, which is associated with pro-inflammatory M1 macrophages (Assis et al., 2022). This shift toward an M2-dominant phenotype supports nerve regeneration and pain relief by enhancing anti-inflammatory cytokine production, such as IL-10 and TGF-β (Liang et al., 2024).
Pain signals from peripheral nerves are integrated and modulated in the spinal cord before being transmitted to higher brain regions (Gurba et al., 2022). CNS sensitization is caused by the abnormal activation of ion channels, spinal microglia, and astrocytes and by the release of pain signals, such as inflammatory cytokines, SP, and CGRP (Cui et al., 2023). EE plays a crucial role in mitigating central sensitization by regulating inflammatory signaling within microglia and astrocytes.
Gong et al. (2018) found that EE can reduce pain and decrease the release of IL-1β and TNF-α. SP neuropeptide and calcitonin gene-related peptides are important pain mediators released by nociceptive sensory nerve endings, which promote central nervous hypersensitivity mainly through the release of inflammatory cytokines and chemokines through MRGPRX2 activation (Green et al., 2019). He et al., 2022) found that SNI mice exposed to EE had significantly lower levels of SP and calcitonin genes in the spinal cord thereby improving pain in SNI mice. Another study (Hsieh et al., 2022) found that EE significantly reduced SP expression, improved microglial hyperactivation, and reduced IBA1 expression to restrain pain. For the calcitonin gene-related peptide, the EE may inhibit the release of TrkA or NGF to decrease the sprouting of CGRP+ fibers, which may be the reason for the reduction of calcitonin gene under EE (Sliwinski et al., 2018). During chronic pain, a decrease in serotonin from the rostral ventromedial medulla to the spinal cord helps to reduce pain. Wang et al. (Takeura et al., 2019) detected decreased serotonin and microglia macrophage markers in the spinal cord after EE in CCL pain model mice. Tai et al. (2021) found that using ketamine in combination with EE could improve spinal cord injury (SCI) pain models, decrease astrocytes, and suppress neurons and microglia NR2B subunits of NMDAR; the activation of NMDAR and NR2B is particularly associated with nerve pain at the lesion site of the spine. The activation of NF-κB and interleukin (IL -1β) is enhanced, resulting in hyperalgesia (Wang et al., 2022). The combination of ketamine and EE reduces inflammatory processes, such as interleukin (IL-1β) in the spinal cord, thereby reducing nerve pain and inflammation; this finding provides a novel scheme for the intervention of nerve pain in the future. In general, EE mainly reverses mechanical allergy in the spinal cord, reduces pro-inflammatory factors in nerve pain, increases anti-inflammatory substances in the spinal cord, and inhibits microglia and astrocyte activation to relieve pain, particularly CNS inflammation after SNI and SCI.
In the spinal cord, a key mechanism is the inhibition of NF-κB and p38 MAPK pathways, which are highly active following nerve injury and contribute to chronic pain states (Guo et al., 2024). EE reduces TNF-α and IL-1β expression in the spinal cord by downregulating NF-κB nuclear translocation and suppressing p38 MAPK activation in spinal microglia (Su et al., 2021). Furthermore, EE modulates the JAK/STAT pathway, particularly through the inhibition of STAT3 phosphorylation, which has been implicated in astrocyte-mediated inflammation and neuroimmune interactions (Lv et al., 2024). Differently, EE differentially affects IBA-1 expression in the spinal cord compared to the PNS (Strath et al., 2021). While EE significantly downregulates IBA-1-positive microglia in the dorsal horn, it also reduces astrocyte activation, indicated by decreased GFAP expression (Han et al., 2024). This suggests that EE exerts broader anti-inflammatory effects in the spinal cord by targeting both microglia and astrocytes, whereas in the PNS, its primary action is on macrophage polarization (Liao et al., 2024). Moreover, EE modulates neurotransmitter signaling to counteract central sensitization (Wang et al., 2024). It increases GABAergic inhibitory transmission while reducing excessive glutamate release, thus balancing excitatory and inhibitory inputs in the spinal cord (Welle and Smith, 2025). Exposure to EE has been shown to enhance CX3CL1 (fractalkine) expression, which promotes microglial homeostasis and reduces neuroinflammation (Subbarayan et al., 2022; Angelopoulou et al., 2020).
The brain plays a central role in processing and modulating pain perception. EE exerts significant neuroprotective effects in the thalamus, cerebral cortex, and hippocampus, primarily by reducing neuroinflammation and oxidative stress (Usrey and Sherman, 2023).
When inflammation occurs in the brain, it can induce oxidative stress and release pro-inflammatory factors, such as IL-1β, TNF-α, and NO (Bai et al., 2023). BDNF is a major CNS factor that regulates many different cellular processes by binding and activating TrkB, which is involved in the development and maintenance of normal brain function (Colucci-D'Amato et al., 2020). Neuropathic pain reduces the complexity of excitatory synapse junctions and BDNF expression in the hippocampus; this finding is due to the signal augment and microglia activation and the release of TNF-α and the receptor TNFR1 after neuropathic pain, leading to the dysregulation of the BDNF/TrkB pathway (Tao et al., 2022). Reducing the expression of pro-inflammatory cytokines in the brain is key to improving neuroinflammation and analgesia (Bhol et al., 2024; Karavis et al., 2023). Kalb et al. (2021) and Qian and Huang (2022) found that the EE attenuated the expression of IL-1 β and TNF-α, regulated the levels of BDNF in the CNS, and increased the expression of CX3CL1, which is a chemokine constitutively expressing in the brain and playing immunomodulatory and anti-inflammatory roles during acute and chronic neuroinflammation. Wang et al. (2019) demonstrated that EE attenuates TNF-α levels in the hippocampus, thereby reducing neuroinflammation in the hippocampus and reducing the pain threshold in mice with neuropathic pain after CCI. Camuso et al. (2022) also demonstrated that BDNF levels in the hippocampus and frontal cortex were significantly increased, and neuropathic pain was improved in mice exposed to 8 weeks of EE stimulation. Lee et al. (2023) found that long-term, EE stimulation reduced astrocyte and microglia activation in mice with neuropathic pain; the expression levels of TNF-α, IL-1β, IL-6, and iNOS in the brain of mice were becoming normal (NO is a highly unstable free radical gas associated with neurosensitization; iNOS is an enzyme produced by NO (Tewari et al., 2021). In general, EEs improve inflammation and pain by reducing the expression of pro-inflammatory factors and increasing the levels of BDNF.
One primary target of EE is BDNF/TrkB signaling, which is crucial for neuroplasticity and neuroimmune regulation (Cappoli et al., 2020). Neuropathic pain reduces BDNF levels in the hippocampus due to chronic inflammation and microglia activation (Lv et al., 2024). EE reverses this effect by upregulating BDNF expression, thereby restoring synaptic function and reducing pain-related emotional disturbances (Li et al., 2025). Furthermore, EE suppresses NF-κB-mediated inflammatory pathways in the hippocampus and cortex, reducing levels of TNF-α, IL-1β, and nitric oxide synthase (iNOS) (Gu et al., 2021). These effects help alleviate neuroinflammation and improve cognitive function in chronic pain states. Unlike in the PNS and spinal cord, where EE mainly affects microglia and macrophages, its effects in the brain extend to astrocytes (Bennett and Bennett, 2020). EE reduces GFAP expression, indicating decreased astrocyte reactivity, which is crucial for long-term pain modulation (Enbar et al., 2024). Another distinguishing feature of EE’s action in the brain is its impact on oxidative stress pathways (Ramos et al., 2024). EE enhances the expression of antioxidant enzymes, such as superoxide dismutase and catalase, which mitigate oxidative stress-induced neuronal damage in neuropathic pain conditions (Kapoor et al., 2019). Additionally, EE upregulates CX3CL1 signaling, which further suppresses microglial activation and promotes anti-inflammatory responses (Subbarayan et al., 2022).
This review summarizes the potential mechanisms by which EE can alleviate neuropathic pain via modulation of neuroinflammation. EE can relieve neuropathic pain by reducing inflammatory mediators, such as proinflammatory cytokines (IL-1β, TNF-α, IL-6) and chemokines (CCL2, CCL3), among others, increasing anti-inflammatory substances (IL-10, Arg-1, CX3CL1) in the periphery and CNS. Specifically, BDNF has an active role in the brain, but the opposite is true in the dorsal root ganglion. Although many studies found that EE can alleviate neuropathic pain, there is still a long way to understand the full mechanism. EE can be used as a non-pharmacological tool to ameliorate pain, anxiety, and depression in neurorehabilitation programs. A more in-depth investigation is required to optimize the integration of EE interventions with other medical approaches to enhance treatment outcomes.
J-DZ: Writing – original draft, Data curation, Investigation. Z-AZ: Conceptualization, Supervision, Methodology, Writing – review & editing. W-YX: Conceptualization, Project administration, Supervision, Methodology, Writing – review & editing.
The author(s) declare that no financial support was received for the research and/or publication of this article.
The authors thank all the participants and clinical researchers involved in the publications cited in this review and peer reviewers who contributed to the continuous improvement of this article.
The authors declare that the research was conducted in the absence of any commercial or financial relationships that could be construed as a potential conflict of interest.
The authors declare that no Generative AI was used in the creation of this manuscript.
All claims expressed in this article are solely those of the authors and do not necessarily represent those of their affiliated organizations, or those of the publisher, the editors and the reviewers. Any product that may be evaluated in this article, or claim that may be made by its manufacturer, is not guaranteed or endorsed by the publisher.
Alaoui Mdarhri, H., Benmessaoud, R., Yacoubi, H., Seffar, L., Guennouni Assimi, H., Hamam, M., et al. (2022). Alternatives therapeutic approaches to conventional antibiotics: advantages, limitations and potential application in medicine. Antibiotics 11:1826. doi: 10.3390/antibiotics11121826
Angelopoulou, E., Paudel, Y. N., Shaikh, M. F., and Piperi, C. (2020). Fractalkine (CX3CL1) signaling and neuroinflammation in Parkinson's disease: potential clinical and therapeutic implications. Pharmacol. Res. 158:104930. doi: 10.1016/j.phrs.2020.104930
Arabpour, M., Saghazadeh, A., and Rezaei, N. (2021). Anti-inflammatory and M2 macrophage polarization-promoting effect of mesenchymal stem cell-derived exosomes. Int. Immunopharmacol. 97:107823. doi: 10.1016/j.intimp.2021.107823
Assis, L. H. P., Dorighello, G. G., and Oliveira, H. C. F. (2022). Pro-inflammatory polarization of macrophages is associated with reduced endoplasmic reticulum-mitochondria interaction. Biochem. Biophys. Res. Commun. 606, 61–67. doi: 10.1016/j.bbrc.2022.03.086
Bai, Y. W., Yang, Q. H., Chen, P. J., and Wang, X. Q. (2023). Repetitive transcranial magnetic stimulation regulates neuroinflammation in neuropathic pain. Front. Immunol. 14:1172293. doi: 10.3389/fimmu.2023.1172293
Bennett, M. L., and Bennett, F. C. (2020). The influence of environment and origin on brain resident macrophages and implications for therapy. Nat. Neurosci. 23, 157–166. doi: 10.1038/s41593-019-0545-6
Bhol, N. K., Bhanjadeo, M. M., Singh, A. K., Dash, U. C., Ojha, R. R., Majhi, S., et al. (2024). The interplay between cytokines, inflammation, and antioxidants: mechanistic insights and therapeutic potentials of various antioxidants and anti-cytokine compounds. Biomed. Pharmacother. 178:117177. doi: 10.1016/j.biopha.2024.117177
Camuso, S., La Rosa, P., Fiorenza, M. T., and Canterini, S. (2022). Pleiotropic effects of BDNF on the cerebellum and hippocampus: implications for neurodevelopmental disorders. Neurobiol. Dis. 163:105606. doi: 10.1016/j.nbd.2021.105606
Cappoli, N., Tabolacci, E., Aceto, P., and Dello Russo, C. (2020). The emerging role of the BDNF-TrkB signaling pathway in the modulation of pain perception. J. Neuroimmunol. 349:577406. doi: 10.1016/j.jneuroim.2020.577406
Chen, Q., Zhang, W., Sadana, N., and Chen, X. (2021). Estrogen receptors in pain modulation: cellular signaling. Biol. Sex Differ. 12:22. doi: 10.1186/s13293-021-00364-5
Chen, Y. S., Zhang, S. M., Yue, C. X., Xiang, P., Li, J. Q., Wei, Z., et al. (2022). Early environmental enrichment for autism spectrum disorder Fmr1 mice models has positive behavioral and molecular effects. Exp. Neurol. 352:114033. doi: 10.1016/j.expneurol.2022.114033
Chu, L. W., Cheng, K. I., Chen, J. Y., Cheng, Y. C., Chang, Y. C., Yeh, J. L., et al. (2020). Loganin prevents chronic constriction injury-provoked neuropathic pain by reducing TNF-α/IL-1β-mediated NF-κB activation and Schwann cell demyelination. Phytomedicine 67:153166. doi: 10.1016/j.phymed.2019.153166
Colucci-D'Amato, L., Speranza, L., and Volpicelli, F. (2020). Neurotrophic Factor BDNF, Physiological functions and therapeutic potential in depression, neurodegeneration and brain Cancer. Int. J. Mol. Sci. 21:7777. doi: 10.3390/ijms21207777
Cui, C. X., Liu, H. Y., Yue, N., Du, Y. R., Che, L. M., and Yu, J. S. (2023). Research progress on the mechanism of chronic neuropathic pain. IBRO Neurosci. Rep. 14, 80–85. doi: 10.1016/j.ibneur.2022.12.007
Dansereau, M. A., Midavaine, E., Begin-Lavallee, V., Belkouch, M., Beaudet, N., Longpre, J. M., et al. (2021). Mechanistic insights into the role of the chemokine CCL2/CCR2 axis in dorsal root ganglia to peripheral inflammation and pain hypersensitivity. J. Neuroinflammation 18:79. doi: 10.1186/s12974-021-02125-y
Duan, Y. W., Chen, S. X., Li, Q. Y., and Zang, Y. (2022). Neuroimmune mechanisms underlying neuropathic pain: the potential role of TNF-alpha-necroptosis pathway. Int. J. Mol. Sci. 23:7191. doi: 10.3390/ijms23137191
Echeverria-Villalobos, M., Tortorici, V., Brito, B. E., Ryskamp, D., Uribe, A., and Weaver, T. (2023). The role of neuroinflammation in the transition of acute to chronic pain and the opioid-induced hyperalgesia and tolerance. Front. Pharmacol. 14:1297931. doi: 10.3389/fphar.2023.1297931
Enbar, T., Hickmott, J. W., Siu, R., Gao, D., Garcia-Flores, E., Smart, J., et al. (2024). Regionally distinct GFAP promoter expression plays a role in off-target neuron expression following AAV5 transduction. Sci. Rep. 14:31583. doi: 10.1038/s41598-024-79124-5
Estrázulas, M., Freitas, R. D. S., Käfer, E. T., Dagnino, A. P. A., and Campos, M. M. (2022). Central and peripheral effects of environmental enrichment in a mouse model of arthritis. Int. Immunopharmacol. 102:108386. doi: 10.1016/j.intimp.2021.108386
Falcicchia, C., Tozzi, F., Arancio, O., Watterson, D. M., and Origlia, N. (2020). Involvement of p38 MAPK in synaptic function and dysfunction. Int. J. Mol. Sci. 21:5624. doi: 10.3390/ijms21165624
Finnerup, N. B., Kuner, R., and Jensen, T. S. (2021). Neuropathic pain: from mechanisms to treatment. Physiol. Rev. 101, 259–301. doi: 10.1152/physrev.00045.2019
Fiore, N. T., Debs, S. R., Hayes, J. P., Duffy, S. S., and Moalem-Taylor, G. (2023). Pain-resolving immune mechanisms in neuropathic pain. Nat. Rev. Neurol. 19, 199–220. doi: 10.1038/s41582-023-00777-3
Giridharan, S., and Srinivasan, M. (2018). Mechanisms of NF-κB p65 and strategies for therapeutic manipulation. J. Inflamm. Res. 11, 407–419. doi: 10.2147/JIR.S140188
Gong, X., Chen, Y., Chang, J., Huang, Y., Cai, M., and Zhang, M. (2018). Environmental enrichment reduces adolescent anxiety- and depression-like behaviors of rats subjected to infant nerve injury. J. Neuroinflammation 15:262. doi: 10.1186/s12974-018-1301-7
Green, D. P., Limjunyawong, N., Gour, N., Pundir, P., and Dong, X. (2019). A mast-cell-specific receptor mediates neurogenic inflammation and pain. Neuron 101, 412–420.e3. doi: 10.1016/j.neuron.2019.01.012
Greenwood, B. N., and Fleshner, M. (2019). Voluntary wheel running: a useful rodent model for investigating the mechanisms of stress robustness and neural circuits of exercise motivation. Curr. Opin. Behav. Sci. 28, 78–84. doi: 10.1016/j.cobeha.2019.02.001
Gu, J. Y., Xu, Y. W., Feng, L. P., Dong, J., Zhao, L. Q., Liu, C., et al. (2021). Enriched environment mitigates depressive behavior by changing the inflammatory activation phenotype of microglia in the hippocampus of depression model rats. Brain Res. Bull. 177, 252–262. doi: 10.1016/j.brainresbull.2021.10.005
Guo, Q., Jin, Y., Chen, X., Ye, X., Shen, X., Lin, M., et al. (2024). NF-κB in biology and targeted therapy: new insights and translational implications. Signal Transduct. Target. Ther. 9:53. doi: 10.1038/s41392-024-01757-9
Gurba, K. N., Chaudhry, R., and Haroutounian, S. (2022). Central neuropathic pain syndromes: current and emerging pharmacological strategies. CNS Drugs 36, 483–516. doi: 10.1007/s40263-022-00914-4
Hakim, S., Jain, A., and Woolf, C. J. (2024). Immune drivers of pain resolution and protection. Nat. Immunol. 25, 2200–2208. doi: 10.1038/s41590-024-02002-9
Han, Y., Shen, X., Gao, Z., Han, P., and Bi, X. (2024). Enriched environment treatment promotes neural functional recovery together with microglia polarization and remyelination after cerebral ischemia in rats. Brain Res. Bull. 209:110912. doi: 10.1016/j.brainresbull.2024.110912
He, L., Zhao, W., Zhang, L., Ilango, M., Zhao, N., Yang, L., et al. (2022). Modified spared nerve injury surgery model of neuropathic pain in mice. J. Visual. Experiment. 179. doi: 10.3791/63362-v
Hsieh, Y. L., Yang, N. P., Chen, S. F., Lu, Y. L., and Yang, C. C. (2022). Early intervention of cold-water swimming on functional recovery and spinal pain modulation following brachial plexus avulsion in rats. Int. J. Mol. Sci. 23:1178. doi: 10.3390/ijms23031178
Huang, L., Jin, J., Chen, K., You, S., Zhang, H., Sideris, A., et al. (2021). BDNF produced by cerebral microglia promotes cortical plasticity and pain hypersensitivity after peripheral nerve injury. PLoS Biol. 19:e3001337. doi: 10.1371/journal.pbio.3001337
Jang, K., and Garraway, S. M. (2024). A review of dorsal root ganglia and primary sensory neuron plasticity mediating inflammatory and chronic neuropathic pain. Neurobiol. Pain 15:100151. doi: 10.1016/j.ynpai.2024.100151
Ji, R. R., Nackley, A., Huh, Y., Terrando, N., and Maixner, W. (2018). Neuroinflammation and central sensitization in chronic and widespread pain. Anesthesiology 129, 343–366. doi: 10.1097/ALN.0000000000002130
Ji, N. N., and Xia, M. (2022). Enriched environment alleviates adolescent visceral pain, anxiety- and depression-like behaviors induced by neonatal maternal separation. Transl Pediatr. 11, 1398–1407. doi: 10.21037/tp-22-410
Kalb, D., Vo, H. D., Adikari, S., Hong-Geller, E., Munsky, B., and Werner, J. (2021). Visualization and modeling of inhibition of IL-1β and TNF-α mRNA transcription at the single-cell level. Sci. Rep. 11:13692. doi: 10.1038/s41598-021-92846-0
Kapoor, D., Singh, S., Kumar, V., Romero, R., Prasad, R., and Singh, J. (2019). Antioxidant enzymes regulation in plants about reactive oxygen species (ROS) and reactive nitrogen species (RNS). Plant Gene. 19:100182. doi: 10.1016/j.plgene.2019.100182
Karavis, M. Y., Siafaka, I., Vadalouca, A., and Georgoudis, G. (2023). Role of microglia in neuropathic pain. Cureus 15:e43555. doi: 10.7759/cureus.43555
Kimura, L. F., Mattaraia, V. G. M., and Picolo, G. (2019). Distinct environmental enrichment protocols reduce anxiety but differentially modulate pain sensitivity in rats. Behav. Brain Res. 364, 442–446. doi: 10.1016/j.bbr.2017.11.012
Kimura, L. F., Novaes, L. S., Picolo, G., Munhoz, C. D., Cheung, C. W., and Camarini, R. (2022). How environmental enrichment balances out neuroinflammation in chronic pain and comorbid depression and anxiety disorders. Br. J. Pharmacol. 179, 1640–1660. doi: 10.1111/bph.15584
Kimura, L. F., Sant'Anna, M. B., Zambelli, V. O., Giardini, A. C., Jared, S. G. S., Antoniazzi, M. M., et al. (2020). Early exposure to environmental enrichment protects male rats against neuropathic pain development after nerve injury. Exp. Neurol. 332:113390. doi: 10.1016/j.expneurol.2020.113390
Lee, J. Y., Park, C. S., Seo, K. J., Kim, I. Y., Han, S., Youn, I., et al. (2023). IL-6/JAK2/STAT3 axis mediates neuropathic pain by regulating astrocyte and microglia activation after spinal cord injury. Exp. Neurol. 370:114576. doi: 10.1016/j.expneurol.2023.114576
Leitzelar, B. N., and Koltyn, K. F. (2021). Exercise and neuropathic pain: a general overview of preclinical and clinical research. Sports Med Open. 7:21. doi: 10.1186/s40798-021-00307-9
Li, H. N., Yang, Q. Q., Wang, W. T., Tian, X., Feng, F., Zhang, S. T., et al. (2021). Red nucleus IL-33 facilitates the early development of mononeuropathic pain in male rats by inducing TNF-alpha through activating ERK, p38 MAPK, and JAK2/STAT3. J. Neuroinflammation 18:150. doi: 10.1186/s12974-021-02198-9
Li, J., Yu, G., Wang, L., Zhang, W., Ke, W., Li, Y., et al. (2025). Enriched environment rescues bisphenol a induced anxiety-like behavior and cognitive impairment by modulating synaptic plasticity. Ecotoxicol. Environ. Saf. 289:117427. doi: 10.1016/j.ecoenv.2024.117427
Liang, J.-H., Zheng, Y.-H., Guo, A.-H., Yu, H., Xia, C.-p., Zhang, Z.-Y., et al. (2024). Astaxanthin promotes nerve repair by regulating the M1/M2 ratio of microglia and promoting angiogenesis. J. Funct. Foods 121:106424. doi: 10.1016/j.jff.2024.106424
Liao, J.-x., Huang, Q.-m., Pan, Z.-c., Wu, J., and Zhang, W.-j. (2024). The anti-inflammatory and immunomodulatory effects of olfactory ensheathing cells transplantation in spinal cord injury and concomitant pathological pain. Eur. J. Pharmacol. 982:176950. doi: 10.1016/j.ejphar.2024.176950
Lv, X. J., Lv, S. S., Wang, G. H., Chang, Y., Cai, Y. Q., Liu, H. Z., et al. (2024). Glia-derived adenosine in the ventral hippocampus drives pain-related anxiodepression in a mouse model resembling trigeminal neuralgia. Brain Behav. Immun. 117, 224–241. doi: 10.1016/j.bbi.2024.01.012
Lv, Y., Qi, J., Babon, J. J., Cao, L., Fan, G., Lang, J., et al. (2024). The JAK-STAT pathway: from structural biology to cytokine engineering. Signal Transduct. Target. Ther. 9:221. doi: 10.1038/s41392-024-01934-w
Martina, J. A., Jeong, E., and Puertollano, R. (2023). p38 MAPK-dependent phosphorylation of TFEB promotes monocyte-to-macrophage differentiation. EMBO Rep. 24:e55472. doi: 10.15252/embr.202255472
Monteiro, S., de Araujo, D., Nassini, R., Geppetti, P., and De Logu, F. (2020). TRPA1 as a therapeutic target for nociceptive pain. Expert Opin. Ther. Targets 24, 997–1008. doi: 10.1080/14728222.2020.1815191
Mouraux, A., Bannister, K., Becker, S., Finn, D. P., Pickering, G., Pogatzki-Zahn, E., et al. (2021). Challenges and opportunities in translational pain research - an opinion paper of the working group on translational pain research of the European pain federation (EFIC). Eur J. Pain 25, 731–756. doi: 10.1002/ejp.1730
Msheik, Z., El Massry, M., Rovini, A., Billet, F., and Desmoulière, A. (2022). The macrophage: a key player in the pathophysiology of peripheral neuropathies. J. Neuroinflammation 19:97. doi: 10.1186/s12974-022-02454-6
Parent-Vachon, M., Beaudry, F., Carrier, D., Di Cristo, G., and Vachon, P. (2019). The effects of exercise on pain and reproductive performance in female pregnant mice with neuropathic pain. Biol. Res. Nurs. 21, 500–509. doi: 10.1177/1099800419857812
Parent-Vachon, M., and Vachon, P. (2018). Environmental enrichment alleviates chronic pain in rats following a spared nerve injury to induce neuropathic pain. A preliminary study. Vet Med 9, 69–72. doi: 10.2147/VMRR.S181531
Popiolek-Barczyk, K., Ciechanowska, A., Ciapała, K., Pawlik, K., Oggioni, M., Mercurio, D., et al. (2020). The CCL2/CCL7/CCL12/CCR2 pathway is substantially and persistently upregulated in mice after traumatic brain injury, and CCL2 modulates the complement system in microglia. Mol. Cell. Probes 54:101671. doi: 10.1016/j.mcp.2020.101671
Qian, J., and Huang, Y. (2022). Expression of TNF-α and IL-1β in peripheral blood of patients with T2DM retinopathy. Comput. Math. Methods Med. 2022, 1–7. doi: 10.1155/2022/9073372
Ramos, T. L., de Sousa Fernandes, M. S., da Silva Fidélis, D. E., Jurema Santos, G. C., Albuquerque, R. B., Ferreira, D. J. S., et al. (2024). The impact of enriched environments on cerebral oxidative balance in rodents: a systematic review of environmental variability effects. Front. Neurosci. 18:1366747. doi: 10.3389/fnins.2024.1366747
Rios, R., Jablonka-Shariff, A., Broberg, C., and Snyder-Warwick, A. K. (2021). Macrophage roles in peripheral nervous system injury and pathology: allies in neuromuscular junction recovery. Mol. Cell. Neurosci. 111:103590. doi: 10.1016/j.mcn.2021.103590
Sadler, K. E., Mogil, J. S., and Stucky, C. L. (2022). Innovations and advances in modelling and measuring pain in animals. Nat. Rev. Neurosci. 23, 70–85. doi: 10.1038/s41583-021-00536-7
Salvemini, D., and Doyle, T. M. (2023). Targeting neuroinflammation in neuropathic pain and opioid use. J. Exp. Med. 220:e20221244. doi: 10.1084/jem.20221244
Schmitt, A., Herzog, P., Röchner, F., Brändle, A. L., Fragasso, A., and Munz, B. (2020). Skeletal muscle effects of two different 10-week exercise regimens, voluntary wheel running, and forced treadmill running, in mice: a pilot study. Physiol. Rep. 8:e14609. doi: 10.14814/phy2.14609
Sliwinski, C., Nees, T. A., Puttagunta, R., Weidner, N., and Blesch, A. (2018). Sensorimotor activity partially ameliorates pain and reduces nociceptive Fiber density in the chronically injured spinal cord. J. Neurotrauma 35, 2222–2238. doi: 10.1089/neu.2017.5431
Smail, M. A., Smith, B. L., Nawreen, N., and Herman, J. P. (2020). Differential impact of stress and environmental enrichment on corticolimbic circuits. Pharmacol. Biochem. Behav. 197:172993. doi: 10.1016/j.pbb.2020.172993
Smith, P. A. (2023). The known biology of neuropathic pain and its relevance to pain management. Can. J. Neurol. Sci. 51, 32–39. doi: 10.1017/cjn.2023.10
Smith, P. A. (2024). BDNF in neuropathic pain; the culprit that cannot be apprehended. Neuroscience 543, 49–64. doi: 10.1016/j.neuroscience.2024.02.020
Song, Q. E. S., Zhang, Z., and Liang, Y. (2024). Neuroplasticity in the transition from acute to chronic pain. Neurotherapeutics 21:e00464. doi: 10.1016/j.neurot.2024.e00464
Sparling, J. E., Barbeau, K., Boileau, K., and Konkle, A. T. M. (2020). Environmental enrichment and its influence on rodent offspring and maternal behaviours, a scoping style review of indices of depression and anxiety. Pharmacol. Biochem. Behav. 197:172997. doi: 10.1016/j.pbb.2020.172997
Stickney, J. D., and Morgan, M. M. (2021). Social housing promotes recovery of wheel running depressed by inflammatory pain and morphine withdrawal in male rats. Behav. Brain Res. 396:112912. doi: 10.1016/j.bbr.2020.112912
Strath, L. J., Totsch, S. K., Quinn, T. L., Menard, M., Philip George, A., Lukens, S. L., et al. (2021). The effect of the standard American diet on Iba-1 immunoreactivity in the spinal cord before and after peripheral inflammatory injury in rats. PharmaNutrition. 18:100278. doi: 10.1016/j.phanu.2021.100278
Su, C.-H., Lin, C.-Y., Tsai, C.-H., Lee, H.-P., Lo, L.-C., Huang, W.-C., et al. (2021). Betulin suppresses TNF-α and IL-1β production in osteoarthritis synovial fibroblasts by inhibiting the MEK/ERK/NF-κB pathway. J. Funct. Foods 86:104729. doi: 10.1016/j.jff.2021.104729
Subbarayan, M. S., Joly-Amado, A., Bickford, P. C., and Nash, K. R. (2022). CX3CL1/CX3CR1 signaling targets for the treatment of neurodegenerative diseases. Pharmacol. Ther. 231:107989. doi: 10.1016/j.pharmthera.2021.107989
Tai, W. L., Sun, L., Li, H., Gu, P., Joosten, E. A., and Cheung, C. W. (2021). Additive effects of environmental enrichment and ketamine on neuropathic pain relief by reducing glutamatergic activation in spinal cord injury in rats. Front. Neurosci. 15:635187. doi: 10.3389/fnins.2021.635187
Takeura, N., Nakajima, H., Watanabe, S., Honjoh, K., Takahashi, A., and Matsumine, A. (2019). Role of macrophages and activated microglia in neuropathic pain associated with chronic progressive spinal cord compression. Sci. Rep. 9:15656. doi: 10.1038/s41598-019-52234-1
Tao, W., Zhang, X., Ding, J., Yu, S., Ge, P., Han, J., et al. (2022). The effect of propofol on hypoxia- and TNF-alpha-mediated BDNF/TrkB pathway dysregulation in primary rat hippocampal neurons. CNS Neurosci. Ther. 28, 761–774. doi: 10.1111/cns.13809
Tewari, D., Sah, A. N., Bawari, S., Nabavi, S. F., Dehpour, A. R., Shirooie, S., et al. (2021). Role of nitric oxide in neurodegeneration: function, regulation, and inhibition. Curr. Neuropharmacol. 19, 114–126. doi: 10.2174/18756190MTA2pMjApy
Thakkar, B., and Acevedo, E. O. (2023). BDNF as a biomarker for neuropathic pain: consideration of mechanisms of action and associated measurement challenges. Brain Behav. 13:e2903. doi: 10.1002/brb3.2903
Tian, J., Yu, T., Xu, Y., Pu, S., Lv, Y., Zhang, X., et al. (2018). Swimming training reduces neuroma pain by regulating Neurotrophins. Med. Sci. Sports Exerc. 50, 54–61. doi: 10.1249/MSS.0000000000001411
Tian, R., Zhou, Y., Ren, Y., Zhang, Y., and Tang, W. (2025). Wallerian degeneration: from mechanism to disease to imaging. Heliyon 11:e40729. doi: 10.1016/j.heliyon.2024.e40729
Toloui, A., Ramawad, H. A., Gharin, P., Vaccaro, A. R., Zarei, H., Hosseini, M., et al. (2023). The role of exercise in the alleviation of neuropathic pain following traumatic spinal cord injuries: a systematic review and Meta-analysis. Neurospine 20, 1073–1087. doi: 10.14245/ns.2346588.294
Townsend, K., Imbert, I., Eaton, V., Stevenson, G. W., and King, T. (2022). Voluntary exercise blocks ongoing pain and diminishes bone remodeling while sparing protective mechanical pain in a rat model of advanced osteoarthritis pain. Pain 163, e476–e487. doi: 10.1097/j.pain.0000000000002392
Udit, S., Blake, K., and Chiu, I. M. (2022). Somatosensory and autonomic neuronal regulation of the immune response. Nat. Rev. Neurosci. 23, 157–171. doi: 10.1038/s41583-021-00555-4
Usrey, W. M., and Sherman, S. M. (eds), (2023). The Cerebral Cortex and Thalamus (New York: Oxford Academic ). Available at: https://doi.org/10.1093/med/9780197676158.001.0001 (Accessed March 12, 2025).
Vergne-Salle, P., and Bertin, P. (2021). Chronic pain and neuroinflammation. Joint Bone Spine 88:105222. doi: 10.1016/j.jbspin.2021.105222
Wang, L., Liu, X., Zhu, C., Wu, S., Li, Z., Jing, L., et al. (2024). Environmental enrichment alleviates hyperalgesia by modulating central sensitization in a nitroglycerin-induced chronic migraine model of mice. J. Headache Pain 25:74. doi: 10.1186/s10194-024-01779-2
Wang, X. M., Pan, W., Xu, N., Zhou, Z. Q., Zhang, G. F., and Shen, J. C. (2019). Environmental enrichment improves long-term memory impairment and aberrant synaptic plasticity by BDNF/TrkB signaling in nerve-injured mice. Neurosci. Lett. 694, 93–98. doi: 10.1016/j.neulet.2018.11.049
Wang, Y., Zhang, J., Chang, H., Wang, H., Xu, W., Cong, H., et al. (2022). NMO-IgG induce Interleukin-6 release via activation of the NF-κB signaling pathway in astrocytes. Neuroscience 496, 96–104. doi: 10.1016/j.neuroscience.2022.05.038
Wang, X. M., Zhang, G. F., Jia, M., Xie, Z. M., Yang, J. J., Shen, J. C., et al. (2019). Environmental enrichment improves pain sensitivity, depression-like phenotype, and memory deficit in mice with neuropathic pain: role of NPAS4. Psychopharmacology 236, 1999–2014. doi: 10.1007/s00213-019-5187-6
Welle, T. M., and Smith, K. R. (2025). Release your inhibitions: the cell biology of GABAergic postsynaptic plasticity. Curr. Opin. Neurobiol. 90:102952. doi: 10.1016/j.conb.2024.102952
Wu, H., Liu, H., Zhao, X., Zheng, Y., Liu, B., Zhang, L., et al. (2020). IKIP negatively regulates NF-κB activation and inflammation through inhibition of IKKα/β phosphorylation. J. Immunol. 204, 418–427. doi: 10.4049/jimmunol.1900626
Wu, Y., Shen, Z., Xu, H., Zhang, K., Guo, M., Wang, F., et al. (2021). BDNF participates in chronic constriction injury-induced neuropathic pain via transcriptionally activating P2X(7) in primary sensory neurons. Mol. Neurobiol. 58, 4226–4236. doi: 10.1007/s12035-021-02410-0
Xing, W. Y., Zhang, Y. H., Yang, Q. H., and Wang, X. Q. (2024). Prevalence and risk factors of low back pain in military personnel: a systematic review. EFORT Open Rev. 9, 1002–1012. doi: 10.1530/EOR-22-0113
Xu, Y. Q., Chen, Y., Xing, J. X., and Yao, J. (2025). Relationship between enriched environment and neurodegeneration: a review from mechanism to therapy. Clin. Epigenetics 17:13. doi: 10.1186/s13148-025-01820-4
Yam, M. F., Loh, Y. C., Tan, C. S., Khadijah Adam, S., Abdul Manan, N., and Basir, R. (2018). General pathways of pain sensation and the major neurotransmitters involved in pain regulation. Int. J. Mol. Sci. 19:2164. doi: 10.3390/ijms19082164
Yu, X., Liu, H., Hamel, K. A., Morvan, M. G., Yu, S., Leff, J., et al. (2020). Dorsal root ganglion macrophages contribute to both the initiation and persistence of neuropathic pain. Nat. Commun. 11:264. doi: 10.1038/s41467-019-13839-2
Zhao, Y. Y., Wu, Z. J., Zhu, L. J., Niu, T. X., Liu, B., and Li, J. (2023). Emerging roles of miRNAs in neuropathic pain: from new findings to novel mechanisms. Front. Mol. Neurosci. 16:1110975. doi: 10.3389/fnmol.2023.1110975
Zheng, K., Chen, M., Xu, X., Li, P., Yin, C., Wang, J., et al. (2024). Chemokine CXCL13-CXCR5 signaling in neuroinflammation and pathogenesis of chronic pain and neurological diseases. Cell. Mol. Biol. Lett. 29:134. doi: 10.1186/s11658-024-00653-y
Zheng, J., Jiang, Y. Y., Xu, L. C., Ma, L. Y., Liu, F. Y., Cui, S., et al. (2017). Adult hippocampal neurogenesis along the Dorsoventral Axis contributes differentially to environmental enrichment combined with voluntary exercise in alleviating chronic inflammatory pain in mice. J. Neurosci. 37, 4145–4157. doi: 10.1523/JNEUROSCI.3333-16.2017
ATF3 - activating transcription factor 3
Arg-1 - arginase-1
BDNF - brain-derived neurotrophic factor
CCI - chronic constriction injury
CX3CL1 - CX3C chemokine ligand 1
CCL2 - chemokine ligand 2
CCL3 - chemokine ligand 3
CGRP - calcitonin gene-related peptide
CD11b - cluster of differentiation 11b
iNOS - inducible nitric oxide synthase
IBA1 - ionized calcium binding adaptor molecule 1
IL-1β - interleukin-1β
IL-6 - interleukin-6
IL-10 - interleukin-10
NGF - nerve growth factor
SP - substance P
TrkA - tropomyosin receptor kinase A
TrkB - tropomyosin receptor kinase B
TNFR1 - tumor necrosis factor receptor 1
TGF-β - transforming growth factor-β
TNF-α - tumor necrosis factor-α
PGI2 - prostacyclin I2
PGE2 - prostaglandin E2
NF-κB - nuclear factor kappa-B
MAPK - mitogen-activated protein kinase
MRGPRX2 - Mas-related G-protein coupled receptor member X2
NMDA - N-methyl-D-aspartic acid
NO - nitric oxide
JAK/STAT - Janus kinase/signal transducer and activator of transcription
Keywords: environmental enrichment, neuropathic pain, neuroinflammation, cytokines, chemokines activating transcription factor 3, arginase-1, brain-derived neurotrophic factor, chronic constriction injury
Citation: Zhang J-D, Zhong Z-A and Xing W-Y (2025) Environmental enrichment for neuropathic pain via modulation of neuroinflammation. Front. Mol. Neurosci. 18:1547647. doi: 10.3389/fnmol.2025.1547647
Received: 20 December 2024; Accepted: 06 March 2025;
Published: 21 March 2025.
Edited by:
Ildikó Rácz, University Hospital Bonn, GermanyReviewed by:
Guang-Yin Xu, Soochow University, ChinaCopyright © 2025 Zhang, Zhong and Xing. This is an open-access article distributed under the terms of the Creative Commons Attribution License (CC BY). The use, distribution or reproduction in other forums is permitted, provided the original author(s) and the copyright owner(s) are credited and that the original publication in this journal is cited, in accordance with accepted academic practice. No use, distribution or reproduction is permitted which does not comply with these terms.
*Correspondence: Zi-An Zhong, bW95b29kZUAxNjMuY29t; Wen-Yuan Xing, d3l4aW5nMjAwMDc5QDE2My5jb20=
Disclaimer: All claims expressed in this article are solely those of the authors and do not necessarily represent those of their affiliated organizations, or those of the publisher, the editors and the reviewers. Any product that may be evaluated in this article or claim that may be made by its manufacturer is not guaranteed or endorsed by the publisher.
Research integrity at Frontiers
Learn more about the work of our research integrity team to safeguard the quality of each article we publish.