- Center for Studies in Behavioral Neurobiology, Department of Psychology, Concordia University, Montreal, QC, Canada
Circadian dysfunction caused by exposure to aberrant light–dark conditions is associated with abnormal alcohol consumption in humans and animal models. Changes in drinking behavior have been linked to alterations in clock gene expression in reward-related brain areas, which could be attributed to either the effect of chronodisruption or alcohol. To date, however, the combinatory effect of circadian disruption and alcohol on brain functions is less understood. Moreover, despite known sex differences in alcohol drinking behavior, most research has been carried out on male subjects only, and therefore implications for females remain unclear. To address this gap, adult female rats housed under an 11 h/11 h light–dark cycle (LD22) or standard light conditions (LD24, 12 h/12 h light–dark) were given access to an intermittent alcohol drinking protocol (IA20%) to assess the impact on gene expression in brain areas implicated in alcohol consumption and reward: the prefrontal cortex (PFC), nucleus accumbens (NAc), and dorsal striatum (DS). mRNA expression of core clock genes (Bmal1, Clock, Per2), sex hormone receptors (ERβ, PR), glutamate receptors (mGluR5, GluN2B), a calcium-activated channel (Kcnn2), and an inflammatory cytokine (TNF-α) were measured at two-time points relative to the locomotor activity cycle. Housing under LD22 did not affect alcohol intake but significantly disrupted circadian activity rhythms and reduced locomotion. Significant changes in the expression of Bmal1, ERβ, and TNF-α were primarily related to the aberrant light conditions, whereas changes in Per2 and PR expression were associated with the effect of alcohol. Collectively, these results indicate that disruption of circadian rhythms and/or intermittent alcohol exposure have distinct effects on gene expression in the female brain, which may have implications for the regulation of alcohol drinking, addiction, and, ultimately, brain health.
Introduction
Alcohol use disorder is a global public health concern, contributing to millions of premature deaths each year (World Health Organization, 2018). Various factors influence alcohol consumption, including circadian rhythm disruption. Research has shown that working night shifts or rotating schedules can increase the risk of alcohol abuse (Richter et al., 2021), while genetic variations in clock genes are linked to alcohol dependence and increased alcohol intake (Kovanen et al., 2010). Long-term alcohol consumption also interferes with the circadian system, which can further promote alcohol intake (Spanagel et al., 2005b). To understand the interplay between circadian rhythm disruption and alcohol consumption, and their impact on health, it is crucial to address their sole and combined effects on the organism.
The circadian system comprises a network of biological clocks located in almost every cell of the body generating daily rhythms in metabolism, physiology, and behavior based on the interaction of so-called clock genes and their proteins. Transcriptional activators Brain and muscle arnt-like 1 (BMAL1) and Circadian locomotor output cycles kaput (CLOCK), and repressors Period (PERs) and Cryptochrome (CRYs) form the core of a transcription-translation feedback loop (TTFL). In brief, heterodimers of BMAL1/CLOCK induce the transcription of Per and Cry genes, which dimerize once translated into proteins and translocate back to the nucleus where they inhibit the transcriptional activity of BMAL1/CLOCK. Degradation of PER and CRY allows BMAL1/CLOCK to activate transcription again, thus starting a new cycle that repeats every 24 h. Chronodisruption caused by working in rotating shifts, amongst others, disorganizes the core loop and thus the expression of so-called clock-controlled genes, which mediate the clock output and regulate daily oscillations of physiological and behavioral programs (Bozek et al., 2009).
In the past, both environmental and genetic manipulations have been applied in animal models to study the effects of circadian disruption on alcohol-drinking behavior. Interestingly, exposure to experimental light–dark (LD) conditions aimed to induce misalignment of circadian rhythms, such as chronic jetlag paradigms, revealed inconsistent results. While some studies reported an increase in alcohol intake in male Sprague–Dawley and female HAD-1 rats (Clark et al., 2007; Gauvin et al., 1997), others observed no changes or reduced alcohol intake in female and male Lewis, Fisher, and Wistar rats (Clark et al., 2007; Meyer et al., 2022; Rosenwasser et al., 2010). The use of various animal models and experimental protocols may explain the degree of variability in the observed outcomes, specifically with regard to the level of circadian disruption. Although studies targeting components of the molecular clockwork revealed clear associations between the expression of single clock genes and alcohol consumption, responses are not uniform across different clock genes. While global knockouts of Per2 and Clock have been shown to increase alcohol consumption in mice (Ozburn et al., 2013; Spanagel et al., 2005a), deficiencies in Rev-erbα and Cry1/2 have been associated with reduced alcohol preference and consumption (Al-Sabagh et al., 2022; Hühne et al., 2022). As such, it is conceivable that the results of studies using environmentally induced chronodisruption vary depending on the level of disorganized clock gene expression.
The circadian system is sexually dimorphic (Yan and Silver, 2016) and sex differences in alcohol consumption in animals with disrupted circadian rhythms have been reported (Rizk et al., 2022; Rosenwasser et al., 2014). Moreover, there is emerging evidence that circadian clock genes in specific brain regions mediate sex differences in alcohol-drinking behavior (de Zavalia et al., 2022). However, because the impact of chronodisruption on alcohol consumption has been studied in males primarily, implications for females remain unclear.
Also, unknown to date is the impact of alcohol consumption on circadian clock function in brain regions regulating alcohol-drinking behavior. Alcohol has been found to affect the circadian system at the molecular and behavioral level (Brager et al., 2010; Chen et al., 2004; Logan et al., 2010; Rosenwasser et al., 2005), which may exacerbate the effects of chronodisruption, thus promoting abnormal alcohol consumption or the development of alcohol use disorder depending on sex.
To address this, we tested the distinct and combined effects of chronodisruption and alcohol consumption on gene expression in specific brain regions that have been identified to regulate drinking behavior in rodents. While female rats did not consume more alcohol when chronodisrupted, our results indicate that the combination of exposure to aberrant light conditions and alcohol consumption modified the expression of clock genes and genes associated with proinflammatory responses in the rats’ brains, whereas the isolated effects of chronodisruption or alcohol were less prominent. Our results contribute to the understanding of the molecular consequences of circadian disruption and/or alcohol use in female rats and may help in the development of targeted interventions and recommendations to treat alcohol abuse in females.
Results
Circadian rhythm parameter, locomotor activity, food intake and body weight
We first assessed the locomotor activity of rats kept under LD24, LD22, and in combination with the IA20% paradigm. Visual inspection of the actograms showed that exposure to aberrant light conditions affected locomotor activity rhythms, whereas alcohol did not (Figure 1).
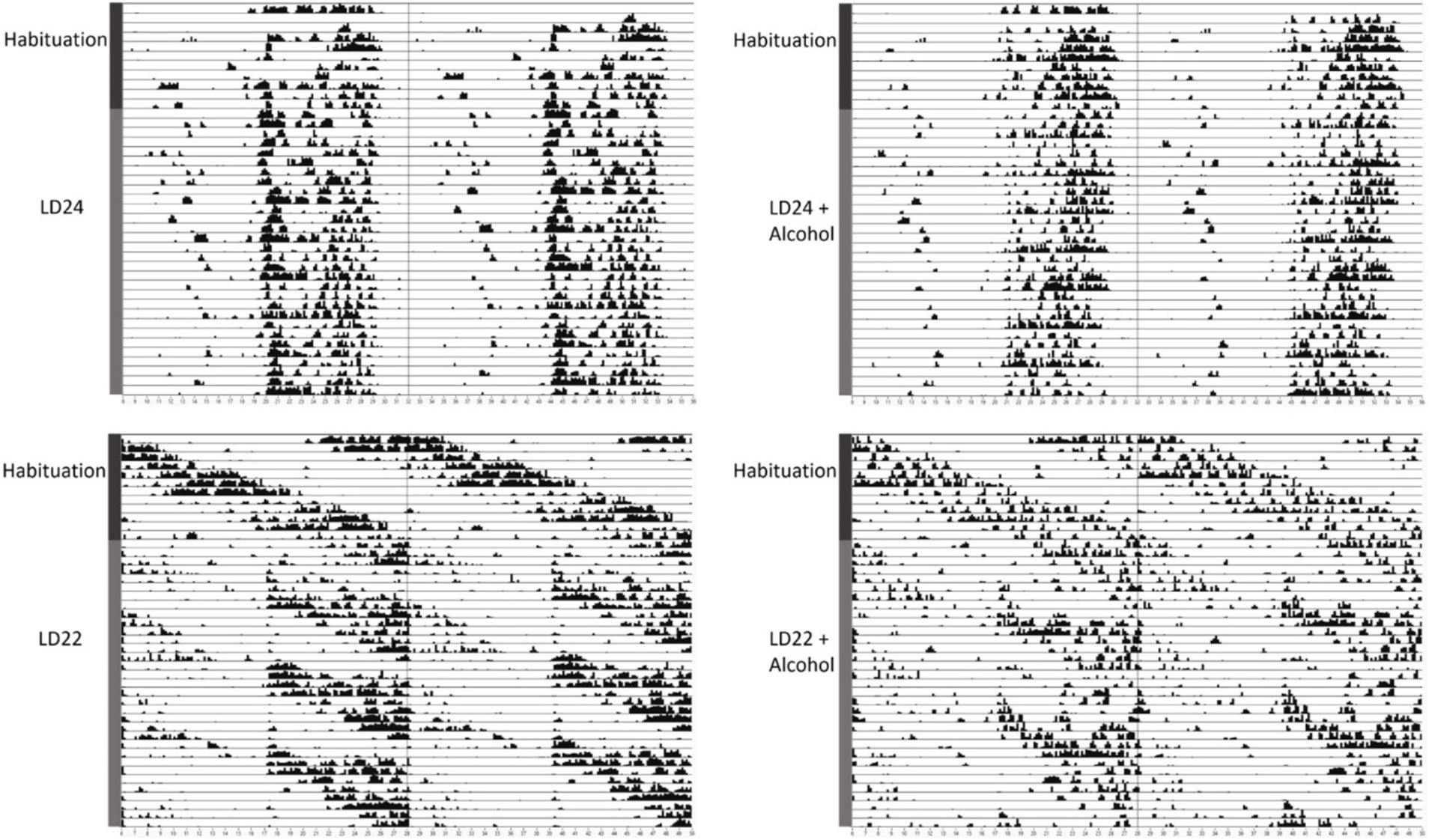
Figure 1. Representative double-plotted actograms of locomotor activity rhythms of female rats kept under various light conditions (standard LD24: LD12:12 h or aberrant LD22:LD11:11 h cycle) and intermittent access to alcohol solution (IA20%) over 40 days. The time of day is double-plotted along the x-axis for each LD paradigm respectively, and successive days are arranged from top to bottom along the y-axis.
As expected, the circadian parameters Interdaily Stability (IS) and Relative Amplitude (RA) differed between the LD groups (Figure 2; One-way ANOVA, IS: F(3,20) = 11.78, p = 0.0001; RA: F(3,20) = 10.13 p = 0.0003). However, Intradaily Variability (IV) did not vary between LD24 and LD22 (IV: F(3,20) = 0.6710, p = 0.5798). Alcohol consumption did not affect the assessed circadian parameters.
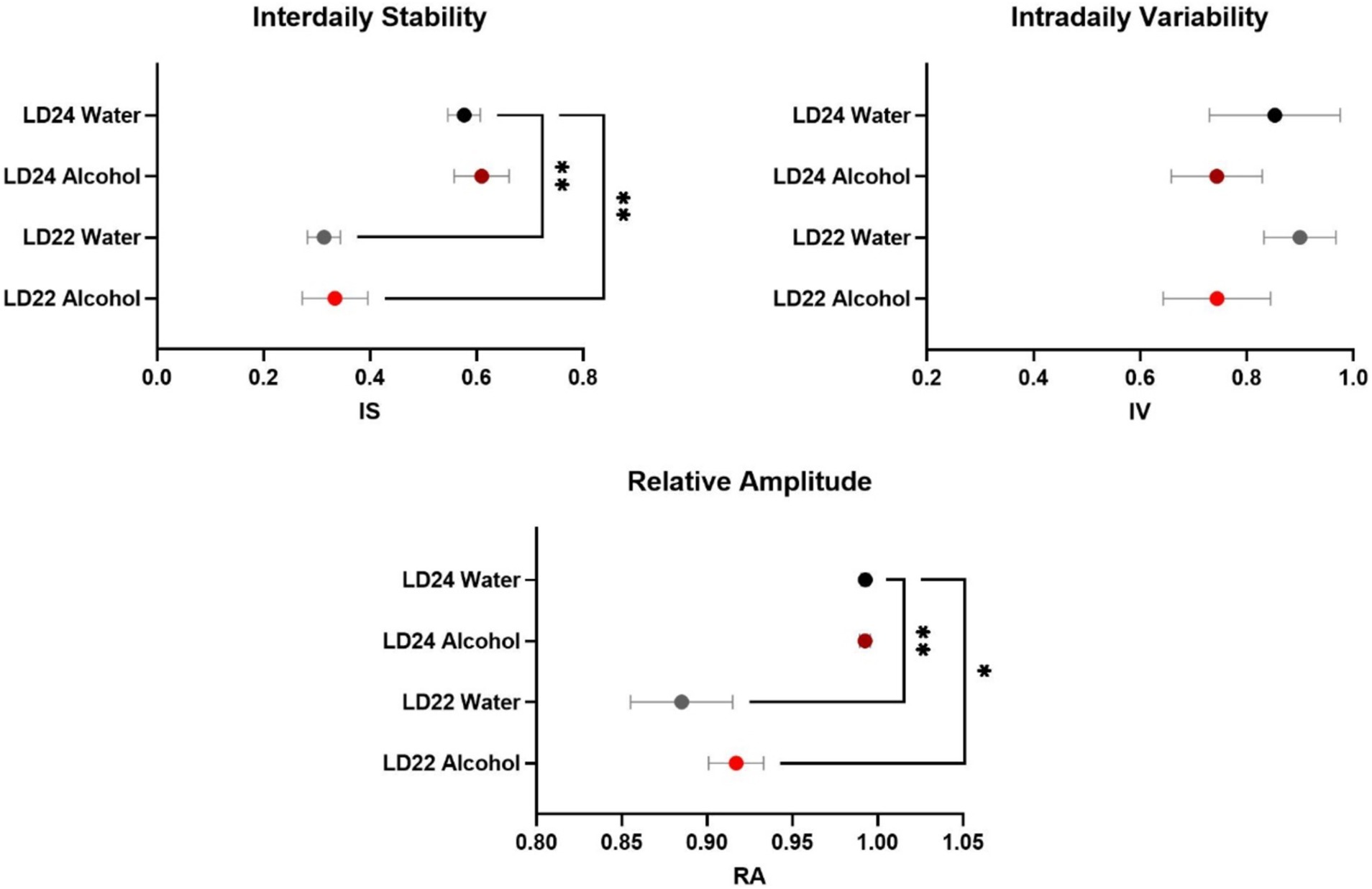
Figure 2. Circadian rhythm parameters during the last 10 days of the experiment. The Interdaily Stability (IS) and Relative Amplitude (RA) of the circadian rhythms were significantly affected by the LD condition, while Intradaily Variability (IV) was unchanged. Data are presented as mean ± SEM, with n = 6 animals per LD and fluid condition. One-way ANOVA followed by Tukey’s multiple comparisons test: *p < 0.05, **p < 0.01.
We further assessed locomotor activity, food intake, and body weight to provide a comprehensive understanding of the physiological effects of chronodisruption and alcohol exposure, as these parameters are critical indicators of overall health and metabolic status. Locomotor activity analysis revealed a significant effect of photoperiod, with rats under LD22 conditions exhibiting suppressed activity compared to those under LD24 (Figure 3; Two-way ANOVA: F(1,20) = 10.00, p = 0.0049). Neither alcohol access nor the interaction between photoperiod and fluid condition significantly affected locomotor activity (Fluid Condition: F(1,20) = 0.6332, p = 0.4355; Interaction: F(1,20) = 0.1309, p = 0.7213). Food intake was measured weekly over a 28-day period, resulting in 4 assessments. Daily food intake was then calculated based on these weekly measurements. The Two-way ANOVA revealed that food intake was significantly affected by fluid conditions but not by photoperiod (Fluid Condition: F(1,12) = 82.18, p < 0.0001; Photoperiod: F(1,12) = 2.494, p = 0.1403). The interaction between photoperiod and fluid condition was not significant (F(1,12) = 2.909, p = 0.1138). Lastly, body weight was not significantly influenced by either photoperiod or fluid condition, but showed a significant progression over time (Three-way ANOVA: Photoperiod: F(1,20) = 0.01244, p = 0.9123; Fluid Condition: F(1,20) = 0.001900, p = 0.9657; Progression: F(2.898, 57.95) = 81.52, p < 0.0001).
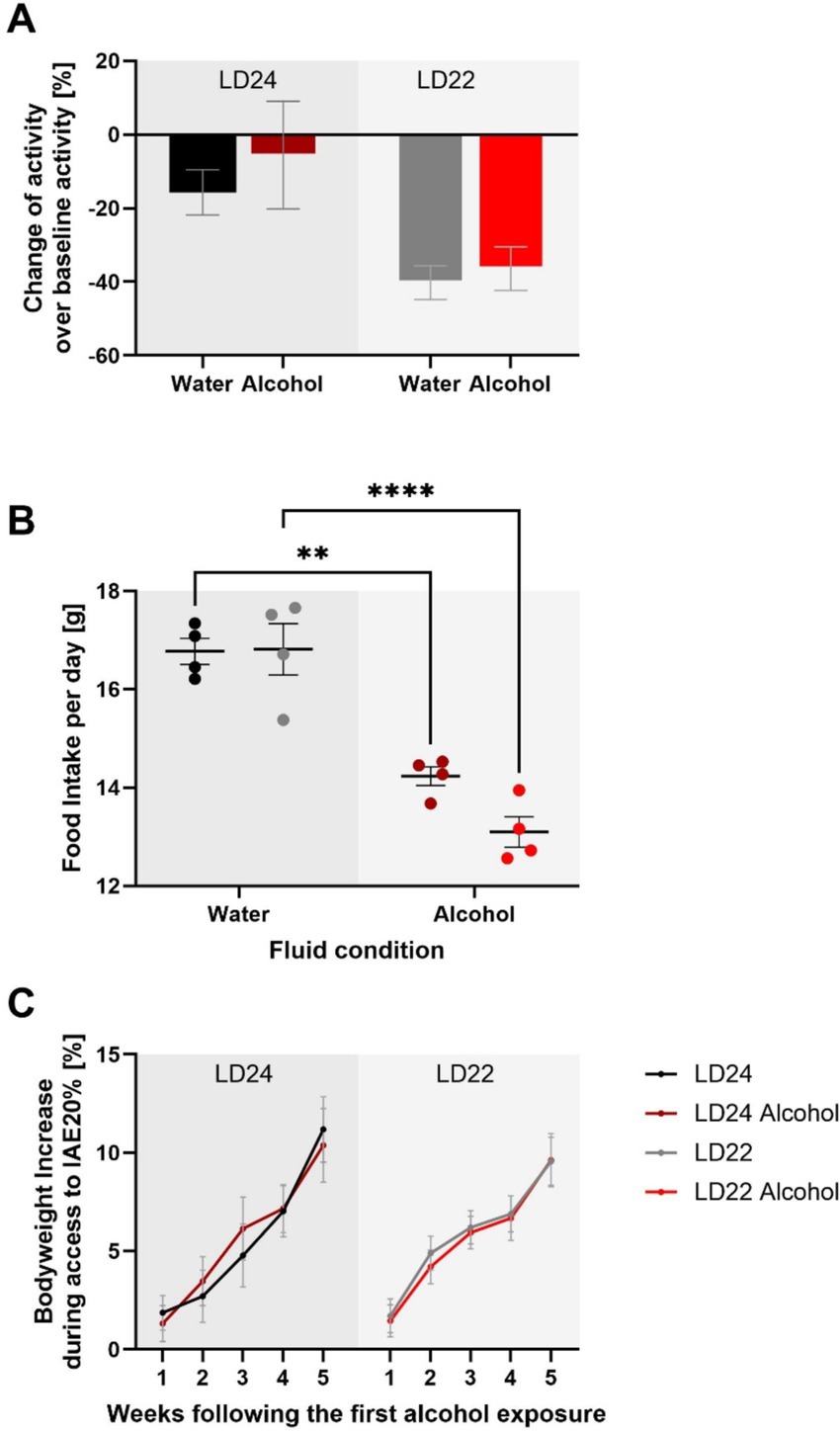
Figure 3. Impact of aberrant light conditions and alcohol consumption on locomotor activity, food intake, and body weight in female rats. (A) Locomotor activity under different light–dark (LD) and fluid conditions. Data represent the sum of activity counts recorded in 10-min intervals over eight consecutive days. Rats exposed to aberrant light conditions (LD22) exhibited significantly suppressed locomotion compared to those under standard conditions (LD24), regardless of fluid type, while alcohol consumption did not significantly affect activity levels. (B) Average daily food intake across experimental conditions. Food consumption was measured weekly over a 28-day period, with daily intake estimated by dividing the total weekly intake by 7. There is a significant effect of fluid condition but not photoperiod on food intake. (C) Body weight gain (%) relative to baseline across different LD paradigms and fluid conditions. No significant effects of photoperiod or fluid condition on body weight gain were observed, but a significant effect of time. Data are presented as mean ± SEM, with n = 6 animals per LD and fluid condition. Two-way ANOVA followed by Tukey’s multiple comparisons test: **p < 0.01, ****p < 0.0001.
These results suggest that while chronodisruption affects locomotor activity, it does not significantly impact food intake or body weight. Alcohol exposure, on the other hand, influences food intake without affecting locomotor activity or body weight.
Alcohol drinking behavior
Having established that the LD22 condition successfully disrupted circadian rhythms, we next examined whether this disruption affected alcohol consumption patterns. All rats, regardless of LD condition, significantly increased their alcohol intake and preference over the course of the intermittent alcohol exposure sessions (Figure 4, Alcohol consumption: Session F(3.975, 39.75) = 6.383 p = 0.0005; Alcohol preference: Session F(4.898, 48.98) = 4.566 p = 0.0018). However, we did not observe any differences in alcohol intake or preference between the LD24 and LD22 groups (Two-way ANOVA; Photoperiod: F(1, 10) = 1.160, p = 0.3068) and alcohol preference (Two-way ANOVA: Photoperiod: F(1, 10) = 0.3119, p = 0.5888).
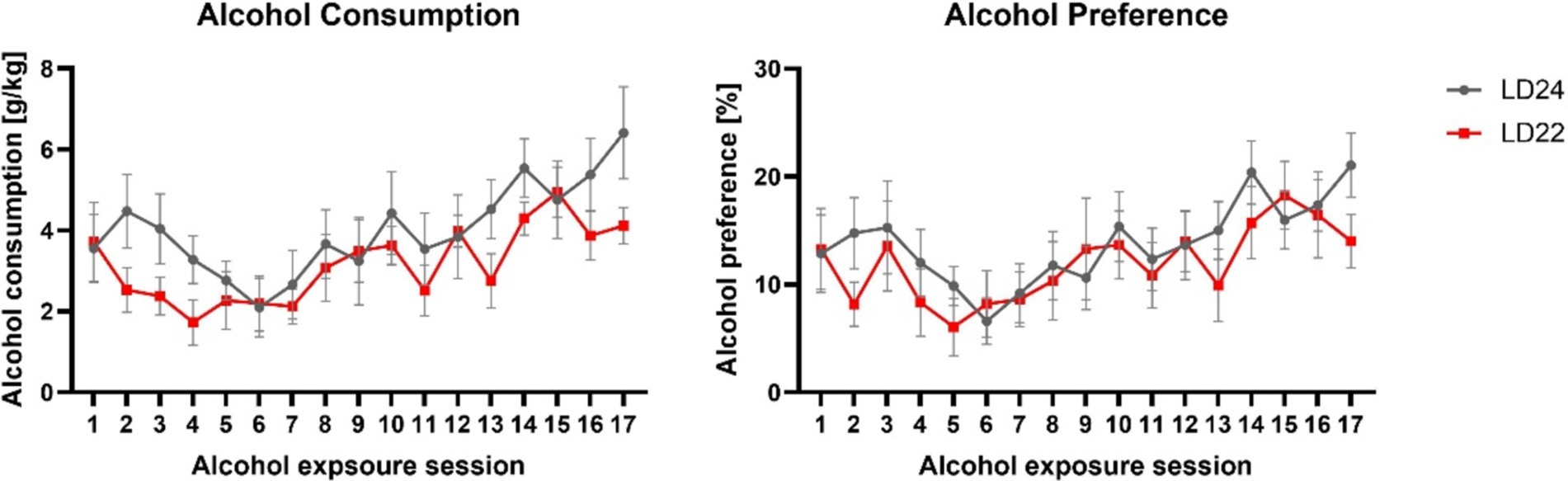
Figure 4. Alcohol consumption and preference were unaffected in female rats exposed to aberrant light conditions. Rats in both the LD24 and LD22 groups showed similar drinking patterns that changed significantly as the experiment progressed. Data are presented as mean ± SEM, with n = 6 animals per LD condition.
Gene expression
Following behavioral characterization, we investigated the impact of chronodisruption and alcohol exposure on gene expression in reward-related brain regions. We selected genes representing key systems potentially affected by these conditions: core clock genes (Bmal1, Per2, and Clock), sex hormone receptors (ERβ, PR), glutamate signaling components (mGluR5, GluN2B, Kcnn2), and an inflammatory marker (TNF-α). These targets were chosen based on their established roles in circadian rhythms, reward processing, and alcohol-related neuroadaptations. Using quantitative real-time PCR, we examined expression changes in the prefrontal cortex (PFC), nucleus accumbens (NAc), and dorsal striatum (DS) of rats exposed to different light–dark cycles and fluid conditions. mRNA was extracted from tissue collected at two-time points relative to each animal’s activity cycle: 2 h after activity onset (HAON2) and 2 h after activity offset (HAOFF2) for both LD24 and LD22 groups. These internal phase markers were chosen to achieve comparable sampling times between groups, as external markers like the light:dark cycle differ between conditions. For consistency and clarity, we refer to these time points as ZT14 and ZT2, respectively, throughout the study, as they correspond to these zeitgeber times in LD24 animals. Statistical analyses were performed using three-way ANOVAs (Photoperiod/LD × fluid condition × time point) for each gene and brain region, followed by Tukey’s post-hoc tests where appropriate.
Expression patterns of clock genes Bmal1 and Per2 in the PFC, NAc, and DS were significantly affected by time of day, whereas the effects of photoperiods (LD24 vs. LD22) and alcohol vary depending on the gene (Figure 5 and Table 1). A notable reduction in the amplitude of Bmal1 expression measured at ZT2 and ZT14 was observed in the striatum of animals kept under LD22 cycles, while alcohol consumption did not change levels of Bmal1 expression in either brain region. Interestingly, the photoperiod did not affect Per2 expression levels, demonstrated by noticeable differences in Per2 levels at ZT2 and ZT14 that were present under both LD22 and LD24 photoperiods (Figure 5 center panel, Table 1). Per2 expression in the PFC, DS, and most notably in the NAc, however, was affected by the fluid condition animals were exposed to (Figure 5 center panel, Table 1). In contrast, no effects of photoperiod, time of day, or alcohol exposure on Clock gene expression were found, except for the PFC where Clock appears marginally upregulated under the LD22 light cycle (Figure 5 right panel, Table 1).
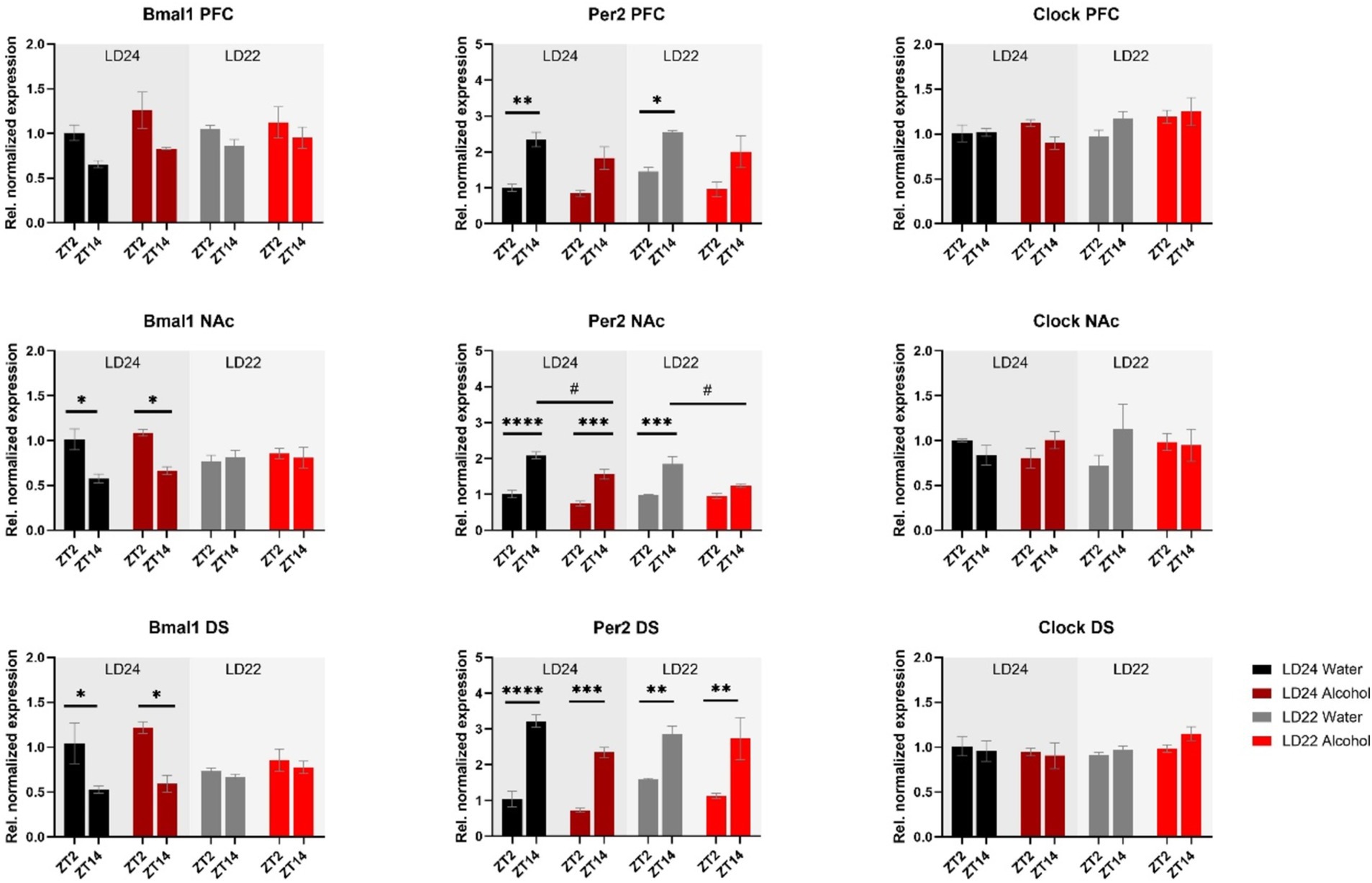
Figure 5. mRNA expression of clock genes Bmal1, Per2, and Clock in the PFC, NAc, and DS at ZT2 and ZT14. The expression of Bmal1 and Per2, but not Clock was affected by the light or fluid condition differently (see Results section and Table 1 for details). Data are presented as mean ± SEM, with n = 3 animals per LD condition and fluid condition. Three-way ANOVA with Šídák’s multiple comparisons test: *p < 0.05, **p < 0.01, ***p < 0.001, ****p < 0.0001.
mRNA expression of the hormone receptors ERβ and PR was further examined (Figure 6). While expression of both receptors was not markedly affected by the experimental conditions, three-way ANOVA revealed changes in ERβ levels in the PFC depending on the time of day, while photoperiod affected ERβ in the PFC and DS (Table 1). PR mRNA expression in the PFC was marginally but significantly affected by the fluid condition (Table 1) while remaining unchanged in the dorsal and ventral striatum irrespective of time of day, photoperiod, or drinking paradigm.
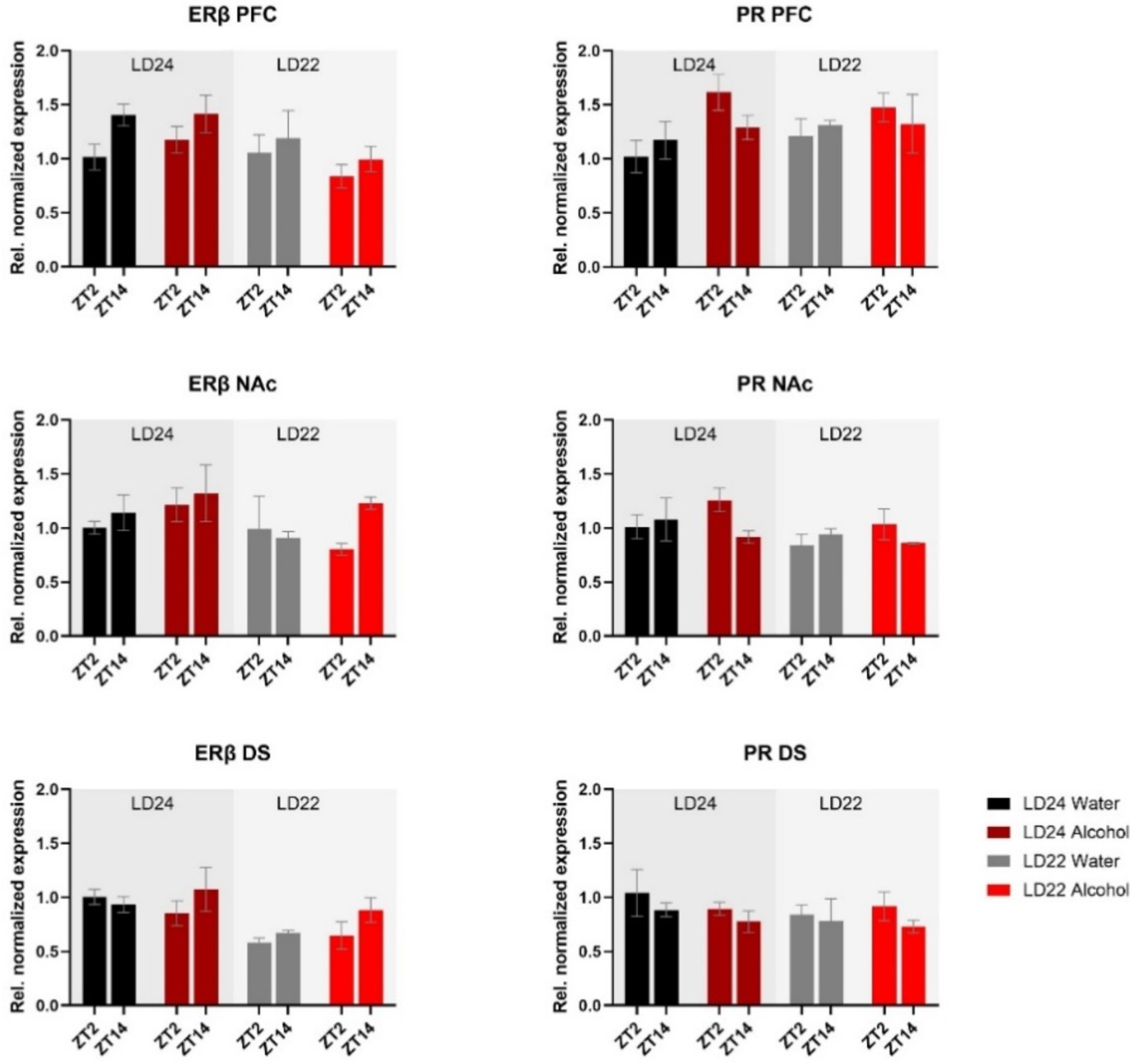
Figure 6. Expression of Estrogen Receptor β (ERβ) and Progesterone Receptor (PR) in the PFC, NAc, and DS at ZT2 and ZT14. The mRNA expression of ERβ and PR was only mildly affected by the LD condition or fluid condition (see Results section and Table 1 for details). Results are presented as mean ± SEM, with n = 3 animals per LD and fluid condition.
Furthermore, we investigated genes related to glutamate signaling, including the glutamate receptors mGluR5 and GluN2B, and the small conductance calcium-activated potassium channel protein 2 (Kcnn2) (Figure 7). No main effects of time of day, photoperiod, or drinking paradigm were observed in either brain region on mGluR5 and GluN2B, while Kcnn2 expression in the PFC and NAc was marginally affected by the light cycle or time of day, respectively (Table 1).
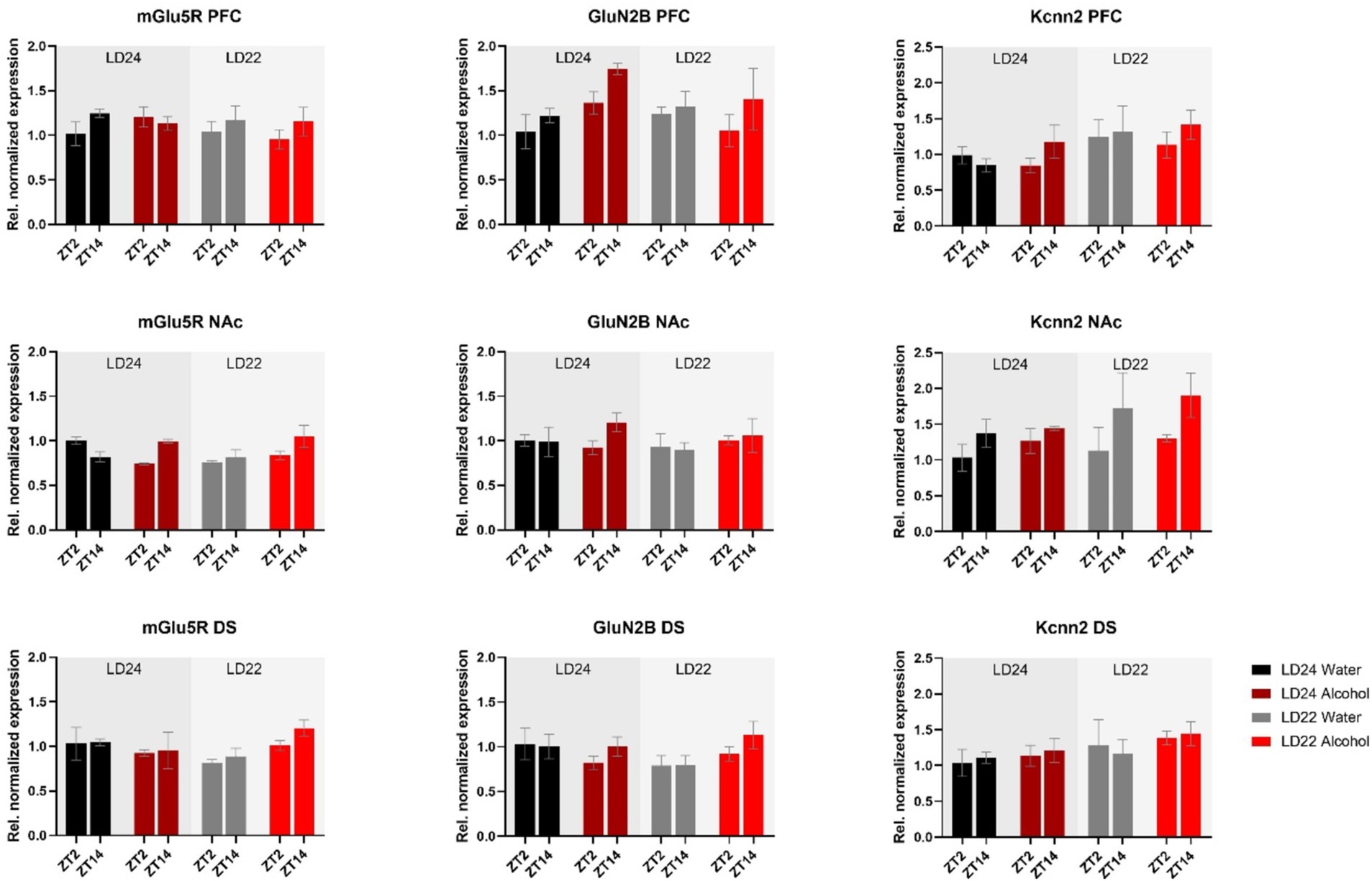
Figure 7. Expression of glutamate receptors (mGluR5, GluN2B) and the Calcium-Activated Potassium Channel (Kcnn2) in the PFC, NAc, and DS at ZT2 and ZT14. The mRNA expression of the glutamate receptor mGluR5 was affected by the interaction of LD and fluid conditions, while no changes were observed in the GluN2B receptor. mRNA levels of Kcnn2 were slightly increased under the LD22 condition in the PFC and NAc (see Results section and Table 1 for details). Results are presented as mean ± SEM, with n = 3 animals per LD condition and fluid condition.
The expression of inflammatory marker TNF-α was also examined (Figure 8). Statistical analysis revealed significant main effects of photoperiod on expression patterns in all three brain regions (Table 1), suggesting that aberrant light conditions led to an increase in TNF-α expression. While time of day had a significant effect on striatal TNF-α expression as well, alcohol consumption appears to exacerbate the difference although no significant interaction was found (Table 1).
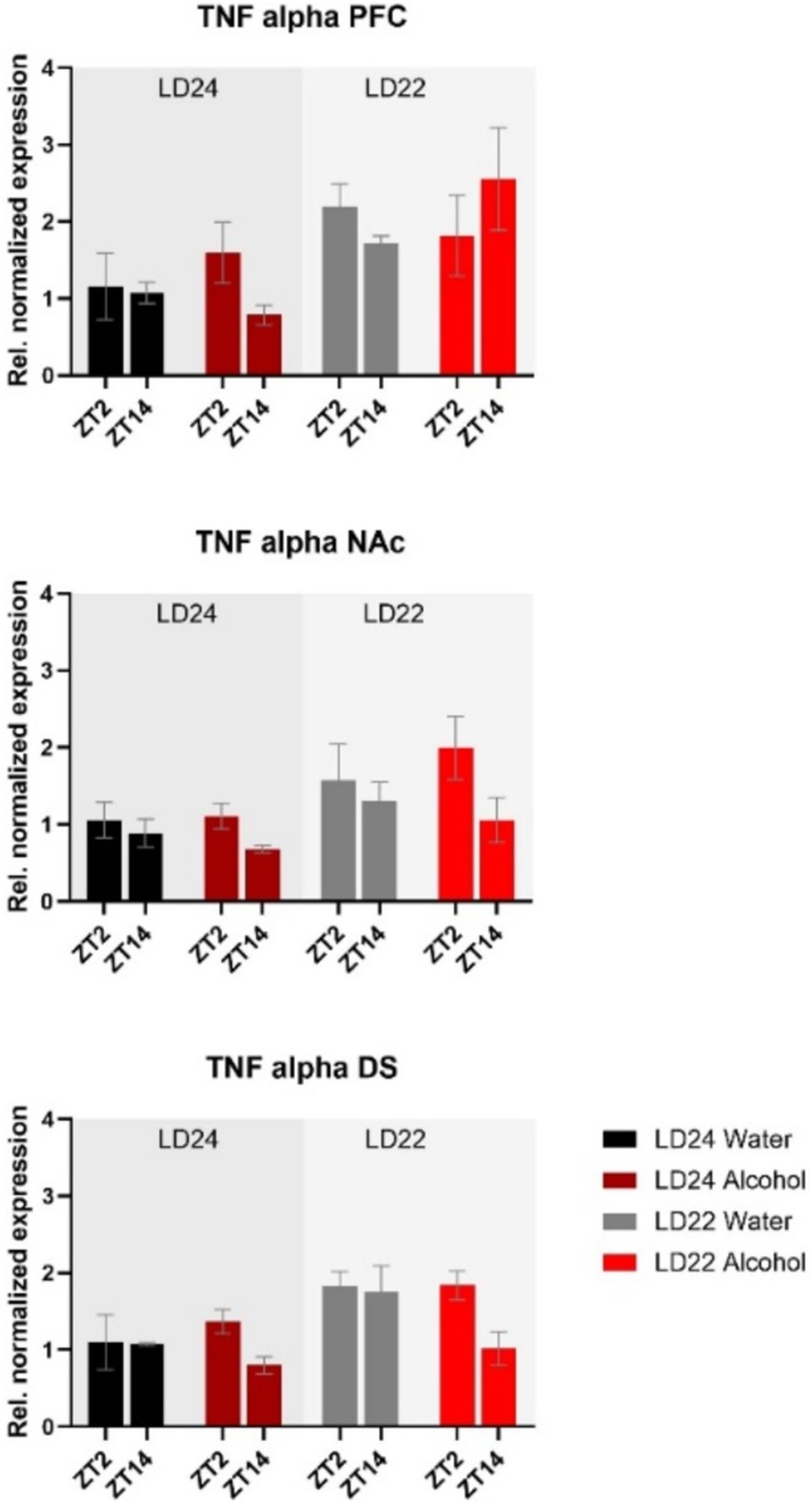
Figure 8. Expression of the inflammatory marker TNF-α in the PFC, NAc, and DS at ZT2 and ZT14. The mRNA expression of TNF-α was affected by both the LD22 condition and time of day. Results are presented as mean ± SEM, with n = 3 animals per LD condition and fluid condition.
Discussion
Disruption of circadian rhythms caused by exposure to abnormal light–dark (LD) conditions is considered a risk factor for alcohol abuse (Barko et al., 2019). However, the neurobiological mechanisms underlying this relationship, particularly in females, are not well understood. In this study, we examined the impact of circadian disruption and intermittent alcohol consumption on the expression of genes in brain areas involved in alcohol-drinking behavior.
Consistent with previous findings, housing female rats under LD22 conditions resulted in the desynchronization of locomotor activity rhythms, but it had no significant effect on alcohol consumption or preference (Meyer et al., 2022). At the molecular level, expression patterns of Bmal1 and TNF-α in the striatum were mostly affected by the aberrant light conditions, whereas alcohol significantly affected the expression of Per2 in the PFC, NAc, and DS only.
Overall, the results indicate that in females, aberrant LD conditions and/or alcohol consumption have only mild effects on the expression of clock and cell-signaling genes within reward-related brain areas. This may explain why alcohol-drinking behavior remains similar under both standard and altered LD conditions in female rats. Based on these findings, it can be concluded that, in female rats, chronodisruption is not a main factor leading to abnormal alcohol-drinking behavior.
Circadian rhythmicity, locomotor activity, food intake and body weight
Changes in circadian rhythm parameters have been associated with neurodegenerative diseases (Anderson et al., 2009; Hatfield et al., 2004). Consistent with previous findings in male rats (De La Iglesia et al., 2004), our study confirmed that the LD22 schedule exhibits chronodisruptive characteristics in females. However, despite a significant decline in daily activity and consolidated rest-activity patterns, rhythm fragmentation remained unchanged irrespective of photoperiod and fluid conditions. The reduced rhythmicity resulted from impaired day-to-day coordination, evidenced by decreased interdaily stability (IS) and dampened robustness of activity rhythms (RA), rather than a fragmentation within each day (unchanged intradaily variability, IV) (Banks et al., 2022).
We further assessed the levels of wheel running activity, as previous research has demonstrated that changes in circadian photoperiod and alcohol exposure can influence locomotor activity (Benstaali et al., 2002; Perreau-Lenz and Spanagel, 2015). Our findings revealed a significant decline in activity under LD22 conditions, while alcohol access did not affect the levels of wheel running. Prior studies have reported conflicting effects of non-standard LD conditions on locomotion, with both increases and decreases observed in male rodents (Bartoszewicz et al., 2009; Delorme et al., 2022; Okuliarova et al., 2016). In female mice, both forward and inverted (shift work-like) photoperiod shifts were found to decrease locomotion (Banks et al., 2022). Notably, the reported changes in locomotor activity were not associated with any significant alterations in alcohol-drinking behavior under experimental LD conditions (Gamsby and Gulick, 2015; Rosenwasser et al., 2010). Hence, the employed experimental LD condition in this study may have a more pronounced impact on locomotor behavior compared to the alcohol exposure paradigm, and the altered locomotor behavior does not appear to affect alcohol intake.
Assessing locomotor activity, food intake, and body weight provided insights into the physiological effects of chronodisruption and alcohol exposure in female rats. Food intake was significantly affected by fluid conditions (alcohol vs. water) but not by photoperiod. It is known that alcoholic solutions serve as a caloric source and may alter feeding behavior independently of circadian disruption. Despite these changes, overall body weight remained unaffected by both photoperiod and fluid conditions, indicating that the observed alterations in locomotor activity and food intake were insufficient to impact overall body mass. These findings suggest that while chronodisruption affects locomotor behavior, it does not significantly alter metabolic outcomes such as food intake or body weight in female rats.
Alcohol drinking behavior
The intermittent alcohol exposure (IAE20%) paradigm used in this study involves daily cycles of alcohol drinking and abstinence, which mimics the repetitive pattern of excessive intake, abstinence, and relapse observed in individuals with alcohol abuse and dependence (Crabbe et al., 2011; Koob and Volkow, 2016), which induces pharmacologically relevant blood alcohol concentrations in rats (Carnicella et al., 2014).
Consistent with earlier studies using the IA20% paradigm in male rats (Mill et al., 2013; Simms et al., 2008), the female rats in our study also exhibited increased alcohol consumption over time. However, we did not observe an effect of an aberrant light cycle on alcohol intake behavior, thus confirming the results of previous studies (Meyer et al., 2022; Rizk et al., 2022).
Gene expression
Non-standard light conditions have been shown to disrupt rhythms of clock gene expression in various tissues (Oishi et al., 2015; Szántóová et al., 2011) and affect the diurnal regulation of reward-related processes (Depoy et al., 2017). Furthermore, alcohol exposure has been reported to alter molecular and behavioral rhythms (Guo et al., 2016) and to affect the expression of genes regulating reward-related processes in the brain (Logan et al., 2014). Through gene expression analysis, we aimed to investigate the interplay of environmental circadian disruption and alcohol consumption on genes involved in circadian function and cell signaling in brain areas mediating reward processes.
Gene association studies in humans have linked the core clock genes Bmal1, Per2, and Clock to abnormal alcohol consumption (Dong et al., 2011; Kovanen et al., 2010), and animal studies have supported these findings. Mutations of Per1, Per2, and Clock in mice have been shown to enhance alcohol consumption, likely through their impact on brain reward processes (Gamsby et al., 2013; Ozburn et al., 2013; Rizk et al., 2022; Spanagel et al., 2005a), whereas Cry1/2-deficient mice exhibited decreased alcohol preference (Hühne et al., 2022). Notably, a targeted knockout of Bmal1 in medium spiny neurons (MSNs) of the striatum decreased alcohol consumption in female mice (de Zavalia et al., 2021), while Bmal1 ablation in the NAc resulted in higher alcohol consumption (Herrera et al., 2023). Moreover, the selective deletion of Per2 in either the entire striatum or the NAc did not affect alcohol consumption in female mice (de Zavalia et al., 2021; Herrera et al., 2023). The results suggest that clock genes may have inhibitory or stimulatory effects on alcohol consumption in females, depending on the specific clock gene and brain region, highlighting the intricate regulation of alcohol consumption by these genes.
Our data indicated differential effects of aberrant light conditions and alcohol consumption on the mRNA expression of the clock genes Clock, Bmal1, and Per2 in female rats. Interestingly, Clock expression remained unaffected by photoperiod and alcohol exposure when compared to control animals, which may explain the similar drinking patterns in both groups. Previous findings by Ozburn et al. (2013) showed that mice bearing a Clock knockout exhibited significantly increased alcohol intake, particularly in females, while others reported that female Clock-deficient and WT female mice consumed similar amounts of alcohol, unlike males (Rizk et al., 2022). Notably, these drinking phenotypes remained unchanged when animals were kept under aberrant LD schedules (Rizk et al., 2022). Despite the contradictory outcomes in studies on Clock knockout mice that may be attributed to variations in drinking paradigms or the use of different mouse strains, our results confirm the view that robust expression of Clock, particularly in the NAc (Ozburn et al., 2013), may confer protection from abnormal drinking, among other factors.
The expression of Bmal1 appeared to be dysregulated in the striatum and prefrontal cortex in animals exposed to aberrant light conditions but their drinking remained unchanged compared to control animals. This suggests that, in females, the sole abundance of Bmal1 in the striatum rather than its oscillation plays a role in the regulation of alcohol consumption as demonstrated by experiments in mice with ablation of Bmal1 from neurons of the entire striatum or just the NAc (de Zavalia et al., 2021; Herrera et al., 2023).
It could also be suggested that certain clock-controlled processes may remain largely unaffected by the dysregulation of Bmal1 expression, as indicated by the levels of Per2 mRNA in the mPFC, NAc, and DS in females kept under LD22 photoperiods, potentially mitigating the effect of chronodisruption on alcohol consumption. Although the persistence of differences in Per2 mRNA levels at ZT2 and ZT14 under LD22 conditions were unexpected, they align with trends observed in other studies. For example, male and female mice with a striatum-specific deletion of Bmal1 show only mild changes in Per2 expression in the dorsal striatum (Schoettner et al., 2022). Additionally, male mice exposed to aberrant LD conditions display disrupted Per2 expression in the SCN, while robust expression profiles of Per2 were observed in the striatum (Ikeno and Yan, 2016). These findings suggest that other factors, such as dopamine signaling, may influence the expression of clock genes in the mesolimbic system, as demonstrated by Hood et al. (2010). Despite recent advances, further studies are needed to better understand the interaction of cell signaling pathways, such as the dopaminergic circuit, the circadian clock, or individual clock genes in the reward system, and how they influence drug-related behaviors.
Previous studies have associated Per2 expression with alcohol consumption in rodents, but recent findings in mice suggest that these effects are sex-dependent. For example, a global or striatum-specific knockout of Per2 increased alcohol consumption in male mice (de Zavalia et al., 2021; Herrera et al., 2023; Spanagel et al., 2005a). In contrast, female mice with striatum-specific Per2 knockout display no change in alcohol consumption, indicating that other sex-specific factors must be considered in the regulation of alcohol-drinking behavior in females.
Sex hormones could potentially be the link between sex-dependent alcohol consumption and clock gene expression in reward-regulating brain areas. Previous studies have shown that CLOCK-BMAL1 complexes bind to the E-box element in the promoter region of estrogen receptor β (ERβ), thereby influencing its expression and subsequently altering cell signaling pathways, such as glutamatergic signaling in the striatum of female rats (Grove-Strawser et al., 2010), which may indirectly affect alcohol consumption (Pandey et al., 2004). In our study, we found that the levels of ERβ mRNA remained unchanged irrespective of LD conditions, levels of Bmal1 expression, or access to alcohol. To determine, whether estrogen signaling is affected by exposure to aberrant LD conditions and/or alcohol exposure, future investigations should include a functional analysis and explore the levels of fluctuating sex hormones.
It is also widely recognized that changes in glutamatergic circuits have an impact on reward-related behaviors, including alcohol consumption, and vice versa (Chandrasekar, 2013; Eisenhardt et al., 2015; Gass and Olive, 2008). Numerous studies have established a connection between the metabotropic glutamate receptor subtype-5 (mGluR5) and the regulation of various behavioral pathologies associated with alcohol misuse. Previous research has demonstrated that alcohol consumption modulates mGluR5 in a brain-region-specific manner (Cozzoli et al., 2009; Simonyi et al., 2004) and that mGluR5 receptor manipulations affect alcohol intake (Cozzoli et al., 2014; Goodwani et al., 2017).
Furthermore, the inotropic NMDA receptor serves as a key target for the actions of alcohol within the central nervous system, and behavioral and cellular investigations have pointed to the importance of the GluN2B subunit in mediating the effects of alcohol (Allgaier, 2002; Nagy et al., 2005). NMDA receptors are implicated in synaptic plasticity and are involved in various aspects of drug and alcohol addiction (Abrahao et al., 2013; Kroener et al., 2012). However, the effects of alcohol on NMDA receptors are inconsistent, as acute alcohol exposure has been shown to inhibit, while chronic exposure promotes the function of the GluN2B subunit (Nagy et al., 2003; Sheela Rani and Ticku, 2006; Wang et al., 2007).
Additionally, small-conductance calcium-activated potassium channels (SKs) modulate NMDA receptor-dependent synaptic plasticity (Faber et al., 2005; Lin et al., 2008) and facilitate learning and memory acquisition (Hammond et al., 2006; Sun et al., 2020). Previous research has shown that chronic alcohol exposure in mice led to a reduction in the expression of Kcnn2 (KCa2.2) and a significant increase in the expression of GluN2B (Mulholland et al., 2011).
Overall, our study did not reveal substantial changes in mRNA expression of the selected neurotransmitter receptors mentioned above following chronic alcohol consumption and/or chronodisruption. While this aligns with the observed lack of changes in alcohol-drinking behavior, further work needs to evaluate their functions using electrophysiological approaches to draw final conclusions, as gene expression analysis may not directly relate to receptor density and function.
In addition to their influence on cell signaling, a growing body of evidence supports the pivotal role of the circadian clock genes in the regulation of inflammatory processes (Carter et al., 2015), which may contribute to alterations in alcohol consumption. Studies have shown that the deletion of Bmal1 and Rev-erbα leads to widespread glial activation, inflammation, and oxidative stress in the brain (Griffin et al., 2019; Musiek et al., 2013). Likewise, chronic alcohol intake has been reported to induce neuroinflammation characterized by increased expression of proinflammatory cytokines, such as tumor necrosis factor-alpha (TNF-α) (Blednov et al., 2005, 2011; Cooper et al., 2020; Kelley and Dantzer, 2011). Activation of TNF-α has been associated with impaired neuronal functioning and increased risk of alcohol abuse and relapse (Obad et al., 2018). Our findings demonstrate that aberrant light cycles, but not chronic alcohol consumption, amplify TNF-α mRNA levels in all examined brain regions. However, TNF-α is a nonspecific inflammatory marker, and future studies should investigate the complex relationship between circadian disruption, clock genes, and neuroinflammatory processes in greater detail.
While our study focused on mRNA expression analysis, we acknowledge that future protein-level analyses would provide valuable insights into post-transcriptional regulation. The primary goal of the study was to capture gene expression changes related to circadian rhythms, reward processing, and alcohol-related neuroadaptations. Future studies employing Western blotting or immunohistochemistry would complement our findings and elucidate the molecular consequences of chronodisruption and alcohol exposure. Such analyses could reveal important post-transcriptional mechanisms, potential mRNA-protein discrepancies, and insights into protein localization, modifications, and interactions, enhancing our understanding of how chronodisruption and alcohol consumption affect cellular processes in female rat brains.
Both, this study, and previous work in mice indicate that female rodents may exhibit greater resilience to aberrant light conditions concerning alcohol consumption. However, a confounding factor in these findings may be the use of running wheels. Exercise is known to influence alcohol-drinking behavior (Buhr et al., 2021; Gallego et al., 2015; Werme et al., 2002). It may be interesting for future studies to compare the effect of aberrant light conditions on alcohol intake and gene expression in the presence and absence of running wheels.
Conclusion
The results of this study show that environmentally induced circadian rhythm disruptions did not affect alcohol consumption in female rats, despite dysregulation of Bmal1 expression in several brain regions associated with alcohol-drinking behavior. It is possible that other clock genes, such as Per2, maintain rhythmicity in brain areas important for reward processing, or that the expression of genes like Clock remains unchanged, contributing to compensatory mechanisms to counteract the loss of Bmal1 rhythmicity.
Our data indicated a more intricate pattern of clock gene expression in the striatum, a brain region that governs alcohol drinking in a sex-dependent manner in rodents, regardless of the presence or absence of specific clock genes. Future studies should focus on investigating specific neural circuits to elucidate the relations between molecular changes and behavioral phenotypes in females.
Materials and methods
Ethics statement
All experimental protocols were approved by the Concordia University Animal Care Committee (certificate number: 30000256).
Animals and light–dark conditions
Adult female Wistar rats were purchased from Charles River (St. Constant, QC, Canada). Animals were singly housed in clear plexiglass cages (9.5″ × 8″ × 16″) equipped with running wheels in light- (standardized illuminance of ∼200–300 lux during the light period, 0 lux during the dark period) and temperature-and humidity-controlled rooms (temperature: 21 ± 1°C, relative humidity: 65 ± 5%). Wheel-running activity of each animal was continuously recorded with the VitalView system (Mini-Mitter, Starr Life Sciences Corp., Oakmont, USA). Food and water were available ad libitum. Only animals with a regular estrous cycle were included in the study.
Following a 3-week habituation to the housing and experimental setting, half of the animals were placed under LD22 (11:11 h light–dark, n = 12), and the other half remained under standard LD24 (12:12 h light–dark, n = 12) conditions. The LD22 light paradigm has been shown to disrupt circadian rhythms and to affect temperature, sleep, corticosterone secretion, and mood (Ben-Hamo et al., 2016; Cambras et al., 2007; De La Iglesia et al., 2004; Wotus et al., 2013).
Intermittent alcohol drinking paradigm
Female rats kept under LD24 or LD22 were randomly assigned into two groups (n = 6) and given access to alcohol (20% v/v, in tap water) every second day for 17 days (IAE20%) as previously described (Wise, 1973). On alcohol days, animals were given access to one bottle of 20% alcohol solution and one bottle of water. After 24 h, the bottles were replaced with two water bottles that were available for the next 24 h. This pattern was repeated each Monday, Wednesday, and Friday. Over the weekend, animals had access to water for 48 h. To control side preferences, the position of the alcohol and water bottles was switched for each alcohol exposure session. Alcohol intake (g/kg body weight/day) and alcohol preference (g of alcohol solution consumed/g of total fluid intake) were measured and calculated at the end of each daily alcohol session. The remaining animals continued drinking water only throughout the experiment.
Gene expression
This study focused on mRNA expression analysis as an initial step to identify transcriptional changes induced by chronodisruption and alcohol exposure. Gene expression was assessed in tissue punches collected from the prefrontal cortex (PFC), nucleus accumbens (NAc), and dorsal striatum (DS). Animals were euthanized using pentobarbital (0.4 mL/kg i.p.; Euthanyl, Bimeda-MTC Animal Health Inc., Cambridge, ON, Canada) followed by decapitation, 24 h to 72 h after the last alcohol exposure at ZT2 (n = 3) and ZT14 (n = 3) (ZT-Zeitgeber time; ZT0 represents the time of light onset). For all animals, regardless of their light–dark cycle, tissue collection times were determined relative to their activity patterns. ZT2 corresponded to 2 h after activity offset (HAOFF2), and ZT14 to 2 h after activity onset (HAON2). These time points were determined by visual inspection of each animal’s actogram on the day of tissue collection. For consistency, we used ZT-terminology throughout the study, as HAON2 and HAOFF2 in LD22 animals correspond to ZT14 and ZT2 in LD24 animals, respectively. Animals kept under LD24 were euthanized at the stage of proestrus. Animals exposed to LD22 did not show a regular estrous cycle (Meyer et al., 2022). The estrous cycle stage was visually determined by the locomotor activity pattern over 4 days, as previously described (Wollnik and Turek, 1988).
After euthanasia, brains were flash-frozen and stored at −80°C until further processing. 200 μm thick coronal sections were obtained using a cryostat (Microm HM505 E, Microm International GmbH, Walldorf, Germany), and tissue punches (2 mm in diameter) were collected from each hemisphere following the rat brain atlas (Paxinos and Watson, 2006).
Total RNA isolation was conducted following a standard Trizol extraction protocol according to the manufacturer’s instructions (Invitrogen™, Life Technologies Corporation, Carlsbad, CA, United States), and total RNA yield was measured using spectrophotometry (Nanodrop 2000; Thermo Scientific™, Wilmington, DE, United States). Next, the RNA integrity was determined by the “bleach gel” method (Aranda et al., 2012) cDNA was synthesized from 1 μg of RNA using the iScriptTMReverse Transcription Supermix following the manufacturer’s instructions (Biorad, Hercules, CA, United States). Besides the standard cDNA samples, a no reverse transcriptase (no-RT) control was prepared.
Realtime PCR was performed with SYBR®Green Supermix SYPR (Biorad, Hercules, CA, United States) and amplification was done using the CFX96TM Real-Time PCR system (Biorad, Hercules, CA, United States). Quantification of target genes was calculated by using the delta–delta Ct (ΔΔCt) method (Pfaffl, 2001) by the CFX Maestro qPCR Software (Biorad, Hercules, CA, United States). The Ct values were normalized to those of β-actin and Gapdh. The primers for the used genes are shown in Table 2.
Data analysis and statistics
All data from locomotor activity, circadian measurements, and gene expression were analyzed using Prism 9 (GraphPad Software, San Diego, CA, United States). Results are expressed as mean ± standard error of the mean (SEM) and were examined for normality and homogeneity of variance. The significance level was set at p < 0.05.
Circadian rhythmicity was analyzed by continuous registration of locomotor activity using running wheels. Summed wheel revolutions were recorded over 10-min periods using the VitalView system (Mini-Mitter, Starr Life Sciences Corp., Oakmont, USA). Wheel running activities were visualized as double-plotted actograms using ClockLab 6 (Actimetrics Software, Evanston, United States). The circadian rhythm parameters, Relative Amplitude (RA), Intradaily Variability (IV), and Interdaily Stability (IS), were calculated using ClockLab 6 over the last 10 days of the experiment. Differences between the treatment groups were calculated by a one-way ANOVA, followed by Tukey’s multiple comparisons tests.
Locomotor activity counts were determined over 8 days during habituation and 8 days at the end of the experiment. As locomotor activity varies in female rodents depending on the stage of the estrous cycle, we assessed the activity counts over 8 days as a multiple of the four-day estrous cycle. Changes in locomotor activity during treatment compared to baseline were calculated as a percentage.
Alcohol consumption and alcohol preference over water were assessed by a two-way repeated measures ANOVA (LD and alcohol session effect). A three-way ANOVA (LD, fluid condition, and ZT effect) followed by Šídák’s multiple comparisons test was used to analyze the gene expression data.
Data availability statement
The data supporting the findings of this study will be made available from the corresponding author upon reasonable request.
Ethics statement
All experimental protocols were approved by the Concordia University Animal Care Committee (certificate number: 30000256). The study was conducted in accordance with the local legislation and institutional requirements.
Author contributions
CM: Conceptualization, Formal analysis, Investigation, Methodology, Visualization, Writing – original draft, Writing – review & editing. KS: Conceptualization, Writing – review & editing, Methodology, Validation. SA: Conceptualization, Funding acquisition, Project administration, Supervision, Writing – review & editing.
Funding
The author(s) declare financial support was received for the research, authorship, and/or publication of this article. This work was supported by a grant from the Canadian Institutes of Health Research to SA.
Conflict of interest
The authors declare that the research was conducted in the absence of any commercial or financial relationships that could be construed as a potential conflict of interest.
Publisher’s note
All claims expressed in this article are solely those of the authors and do not necessarily represent those of their affiliated organizations, or those of the publisher, the editors and the reviewers. Any product that may be evaluated in this article, or claim that may be made by its manufacturer, is not guaranteed or endorsed by the publisher.
References
Abrahao, K. P., Ariwodola, O. J., Butler, T. R., Rau, A. R., Skelly, M. J., Carter, E., et al. (2013). Locomotor sensitization to ethanol impairs NMDA receptor-dependent synaptic plasticity in the nucleus accumbens and increases ethanol self-administration. J. Neurosci. Off. J. Soc. Neurosci. 33, 4834–4842. doi: 10.1523/JNEUROSCI.5839-11.2013
Allgaier, C. (2002). Ethanol sensitivity of NMDA receptors. Neurochem. Int. 41, 377–382. doi: 10.1016/S0197-0186(02)00046-3
Al-Sabagh, Y., Thorpe, H. H. A., Jenkins, B. W., Hamidullah, S., Talhat, M. A., Suggett, C. B., et al. (2022). Rev-erbα knockout reduces ethanol consumption and preference in male and female mice. Int. J. Mol. Sci. 23:5197. doi: 10.3390/ijms23095197
Anderson, K. N., Hatfield, C., Kipps, C., Hastings, M., and Hodges, J. R. (2009). Disrupted sleep and circadian patterns in frontotemporal dementia. Eur. J. Neurol. 16, 317–323. doi: 10.1111/J.1468-1331.2008.02414.X
Aranda, P. S., Lajoie, D. M., and Jorcyk, C. L. (2012). Bleach gel: a simple agarose gel for analyzing RNA quality. Electrophoresis 33, 366–369. doi: 10.1002/elps.201100335
Banks, G., Nolan, P. M., and Bourbia, N. (2022). Shift work-like patterns effect on female and male mouse behavior. Neurobiol. Sleep Circadian Rhythms 13:100082. doi: 10.1016/J.NBSCR.2022.100082
Barko, K., Shelton, M. A., Seggio, J. A., and Logan, R. W. (2019). Circadian Rhythms and Addiction. Neural Mechanisms of Addiction. 189–212. doi: 10.1016/B978-0-12-812202-0.00013-0
Bartoszewicz, R., Chmielewska, D., Domoń, M., and Barbacka-Surowiak, G. (2009). Influence of short-term constant light on phase shift of mouse circadian locomotor activity rhythm induced by agonist and antagonist of serotonin. Biological Rhythm Research, 41, 279–288. doi: 10.1080/09291010903018016
Ben-Hamo, M., Larson, T. A., Duge, L. S., Sikkema, C., Wilkinson, C. W., De La Iglesia, H. O., et al. (2016). Circadian forced desynchrony of the master clock leads to phenotypic manifestation of depression in rats. eNeuro 3:ENEURO.0237-16. 2016. doi: 10.1523/ENEURO.0237-16.2016
Benstaali, C., Bogdan, A., and Touitou, Y. (2002). Effect of a short photoperiod on circadian rhythms of body temperature and motor activity in old rats. Pflugers Arch. Eur. J. Physiol. 444, 73–79. doi: 10.1007/s00424-002-0795-z
Blednov, Y. A., Benavidez, J. M., Geil, C., Perra, S., Morikawa, H., and Harris, R. A. (2011). Activation of inflammatory signaling by lipopolysaccharide produces a prolonged increase of voluntary alcohol intake in mice. Brain Behav. Immun. 25, S92–S105. doi: 10.1016/j.bbi.2011.01.008
Blednov, Y. A., Bergeson, S. E., Walker, D., Ferreira, V. M. M., Kuziel, W. A., and Harris, R. A. (2005). Perturbation of chemokine networks by gene deletion alters the reinforcing actions of ethanol. Behav. Brain Res. 165, 110–125. doi: 10.1016/j.bbr.2005.06.026
Bozek, K., Relógio, A., Kielbasa, S. M., Heine, M., Dame, C., Kramer, A., et al. (2009). Regulation of clock-controlled genes in mammals. PLoS One 4:e4882. doi: 10.1371/journal.pone.0004882
Brager, A. J., Ruby, C. L., Prosser, R. A., and Glass, J. D. (2010). Chronic ethanol disrupts circadian photic entrainment and daily locomotor activity in the mouse. Alcohol. Clin. Exp. Res. 34, 1266–1273. doi: 10.1111/J.1530-0277.2010.01204.X
Buhr, T. J., Reed, C. H., Shoeman, A., Bauer, E. E., Valentine, R. J., and Clark, P. J. (2021). The influence of moderate physical activity on brain monoaminergic responses to binge-patterned alcohol ingestion in female mice. Front. Behav. Neurosci. 15:780189. doi: 10.3389/FNBEH.2021.639790
Cambras, T., Weller, J. R., Anglès-Pujoràs, M., Lee, M. L., Christopher, A., Díez-Noguera, A., et al. (2007). Circadian desynchronization of core body temperature and sleep stages in the rat. Proc. Natl. Acad. Sci. U. S. A. 104, 7634–7639. doi: 10.1073/pnas.0702424104
Carnicella, S., Ron, D., and Barak, S. (2014). Intermittent ethanol access schedule in rats as a preclinical model of alcohol abuse. Alcohol 48, 243–252. doi: 10.1016/j.alcohol.2014.01.006
Carter, S. J., Durrington, H. J., Gibbs, J. E., Blaikley, J., Loudon, A. S., Ray, D. W., et al. (2015). A matter of time: study of circadian clocks and their role in inflammation. J. Leukoc. Biol. 99, 549–560. doi: 10.1189/jlb.3RU1015-451R
Chandrasekar, R. (2013). Alcohol and NMDA receptor: current research and future direction. Front. Mol. Neurosci. 6:14. doi: 10.3389/FNMOL.2013.00014
Chen, C. P., Kuhn, P., Advis, J. P., and Sarkar, D. K. (2004). Chronic ethanol consumption impairs the circadian rhythm of pro-opiomelanocortin and period genes mRNA expression in the hypothalamus of the male rat. J. Neurochem. 88, 1547–1554. doi: 10.1046/J.1471-4159.2003.02300.X
Clark, J. W., Fixaris, M. C., Belanger, G. V., and Rosenwasser, A. M. (2007). Repeated light-dark phase shifts modulate voluntary ethanol intake in male and female high alcohol-drinking (HAD1) rats. Alcohol. Clin. Exp. Res. 31, 1699–1706. doi: 10.1111/j.1530-0277.2007.00476.x
Cooper, I. A., Beecher, K., Chehrehasa, F., Belmer, A., and Bartlett, S. E. (2020). Tumour necrosis factor in neuroplasticity, neurogenesis and alcohol use disorder. Brain Plasticity 6, 47–66. doi: 10.3233/BPL-190095
Cozzoli, D. K., Goulding, S. P., Zhang, P. W., Xiao, B., Hu, J.-H., Ary, A. W., et al. (2009). Behavioral/systems/cognitive binge drinking upregulates accumbens mGluR5-Homer2-PI3K signaling: functional implications for alcoholism. J Neurosci 29, 8655–8668. doi: 10.1523/JNEUROSCI.5900-08.2009
Cozzoli, D. K., Strong-Kaufman, M. N., Tanchuck, M. A., Hashimoto, J. G., Wiren, K. M., and Finn, D. A. (2014). The effect of mGluR5 antagonism during binge Drinkingon subsequent ethanol intake in C57BL/6J mice: sex-and age-induced differences. Alcohol. Clin. Exp. Res. 38, 730–738. doi: 10.1111/ACER.12292
Crabbe, J. C., Harris, R. A., and Koob, G. F. (2011). Preclinical studies of alcohol binge drinking. Ann. N. Y. Acad. Sci. 1216, 24–40. doi: 10.1111/J.1749-6632.2010.05895.X
De La Iglesia, H. O., Cambras, T., Schwartz, W. J., and Díez-Noguera, A. (2004). Forced desynchronization of dual circadian oscillators within the rat suprachiasmatic nucleus. Curr. Biol. 14, 796–800. doi: 10.1016/j.cub.2004.04.034
de Zavalia, N., Ferraro, S., and Amir, S. (2022). Sexually dimorphic role of circadian clock genes in alcohol drinking behavior. Psychopharmacology 240, 431–440. doi: 10.1007/S00213-022-06247-W
de Zavalia, N., Schoettner, K., Goldsmith, J. A., Solis, P., Ferraro, S., Parent, G., et al. (2021). Bmal1 in the striatum influences alcohol intake in a sexually dimorphic manner. Commun. Biol. 4, 1227–1213. doi: 10.1038/s42003-021-02715-9
Delorme, T. C., Srikanta, S. B., Fisk, A. S., Cloutier, M. E., Sato, M., Pothecary, C. A., et al. (2022). Chronic exposure to dim light at night or irregular lighting conditions impact circadian behavior, motor coordination, and neuronal morphology. Front. Neurosci. 16:855154. doi: 10.3389/fnins.2022.855154
Depoy, L. M., McClung, C. A., and Logan, R. W. (2017). Neural Mechanisms of Circadian Regulation of Natural and Drug Reward. Neural Plasticity, 5720842. doi: 10.1155/2017/5720842
Dong, L., Bilbao, A., Laucht, M., Henriksson, R., Yakovleva, T., Ridinger, M., et al. (2011). Effects of the circadian rhythm gene period 1 (Per1) on psychosocial stress-induced alcohol drinking. Am. J. Psychiatry 168, 1090–1098. doi: 10.1176/appi.ajp.2011.10111579
Eisenhardt, M., Leixner, S., Luján, R., Spanagel, R., and Bilbao, A. (2015). Glutamate receptors within the mesolimbic dopamine system mediate alcohol relapse behavior. J. Neurosci. 35, 15523–15538. doi: 10.1523/JNEUROSCI.2970-15.2015
Faber, E. S. L., Delaney, A. J., and Sah, P. (2005). SK channels regulate excitatory synaptic transmission and plasticity in the lateral amygdala. Nat. Neurosci. 8, 635–641. doi: 10.1038/nn1450
Gallego, X., Cox, R. J., Funk, E., Foster, R. A., and Ehringer, M. A. (2015). Voluntary exercise decreases ethanol preference and consumption in C57BL/6 adolescent mice: sex differences and hippocampal BDNF expression. Physiol. Behav. 138, 28–36. doi: 10.1016/j.physbeh.2014.10.008
Gamsby, J. J., and Gulick, D. (2015). Chronic shifts in the length and phase of the light cycle increase intermittent alcohol drinking in C57BL/6J mice. Front. Behav. Neurosci. 9:9. doi: 10.3389/fnbeh.2015.00009
Gamsby, J. J., Templeton, E. L., Bonvini, L. A., Wang, W., Loros, J. J., Dunlap, J. C., et al. (2013). The circadian Per1 and Per2 genes influence alcohol intake, reinforcement, and blood alcohol levels. Behav. Brain Res. 249, 15–21. doi: 10.1016/j.bbr.2013.04.016
Gass, J. T., and Olive, M. F. (2008). Glutamatergic substrates of drug addiction and alcoholism. Biochem. Pharmacol. 75, 218–265. doi: 10.1016/J.BCP.2007.06.039
Gauvin, D. V., Baird, T. J., Vanecek, S. A., Briscoe, R. J., Vallett, M., and Holloway, F. A. (1997). Effects of time-of-day and photoperiod phase shifts on voluntary ethanol consumption in rats. Alcohol. Clin. Exp. Res. 21, 817–825. doi: 10.1111/j.1530-0277.1997.tb03845.x
Goodwani, S., Saternos, H., Alasmari, F., and Sari, Y. (2017). Metabotropic and ionotropic glutamate receptors as potential targets for the treatment of alcohol use disorder. Neurosci. Biobehav. Rev. 77, 14–31. doi: 10.1016/J.NEUBIOREV.2017.02.024
Griffin, P., Dimitry, J. M., Sheehan, P. W., Lananna, B. V., Guo, C., Robinette, M. L., et al. (2019). Circadian clock protein Rev-erbα regulates neuroinflammation. Proc. Natl. Acad. Sci. U. S. A. 116, 5102–5107. doi: 10.1073/pnas.1812405116
Grove-Strawser, D., Boulware, M. I., and Mermelstein, P. G. (2010). Membrane estrogen receptors activate the metabotropic glutamate receptors mGluR5 and mGluR3 to bidirectionally regulate CREB phosphorylation in female rat striatal neurons. Neuroscience 170, 1045–1055. doi: 10.1016/J.NEUROSCIENCE.2010.08.012
Guo, R., Simasko, S. M., and Jansen, H. T. (2016). Chronic alcohol consumption in rats leads to desynchrony in diurnal rhythms and molecular clocks. Alcohol. Clin. Exp. Res. 40, 291–300. doi: 10.1111/acer.12944
Hammond, R. S., Bond, C. T., Strassmaier, T., Ngo-Anh, T. J., Adelman, J. P., Maylie, J., et al. (2006). Small-conductance Ca2+-activated K+ channel type 2 (SK2) modulates hippocampal learning, memory, and synaptic plasticity. J. Neurosci. 26, 1844–1853. doi: 10.1523/JNEUROSCI.4106-05.2006
Hatfield, C. F., Herbert, J., Van Someren, E. J. W., Hodges, J. R., and Hastings, M. H. (2004). Disrupted daily activity/rest cycles in relation to daily cortisol rhythms of home-dwelling patients with early Alzheimer’s dementia. Brain 127, 1061–1074. doi: 10.1093/BRAIN/AWH129
Herrera, J., Button, M., Doherty-Haigh,, Goldfarb, P., and Amir, N. (2023). Circadian clock genes Bmal1 and Per2 in the nucleus accumbens are negative regulators of alcohol-drinking behavior in mice.
Hood, S., Cassidy, P., Cossette, M.-P., Weigl, Y., Verwey, M., Robinson, B., et al. (2010). Endogenous dopamine regulates the rhythm of expression of the clock protein PER2 in the rat dorsal striatum via daily activation of D2 dopamine receptors. J. Neurosci. 30, 14046–14058. doi: 10.1523/JNEUROSCI.2128-10.2010
Hühne, A., Echtler, L., Kling, C., Stephan, M., Schmidt, M. V., Rossner, M. J., et al. (2022). Circadian gene × environment perturbations influence alcohol drinking in Cryptochrome-deficient mice. Addict. Biol. 27:e13105. doi: 10.1111/adb.13105
Ikeno, T., and Yan, L. (2016). Chronic light exposure in the middle of the night disturbs the circadian system and emotional regulation. J. Biol. Rhythm. 31, 352–364. doi: 10.1177/0748730416642065
Kelley, K. W., and Dantzer, R. (2011). “Alcoholism and inflammation: neuroimmunology of behavioral and mood disorders” in Brain, behavior, and immunity, vol. 25.
Koob, G. F., and Volkow, N. D. (2016). Neurobiology of addiction: a neurocircuitry analysis. Lancet Psychiatry 3, 760–773. doi: 10.1016/S2215-0366(16)00104-8
Kovanen, L., Saarikoski, S. T., Haukka, J., Pirkola, S., Aromaa, A., Lönnqvist, J., et al. (2010). Circadian clock gene polymorphisms in alcohol use disorders and alcohol consumption. Alcohol Alcohol. 45, 303–311. doi: 10.1093/alcalc/agq035
Kroener, S., Mulholland, P. J., New, N. N., Gass, J. T., Becker, H. C., and Chandler, L. J. (2012). Chronic alcohol exposure alters behavioral and synaptic plasticity of the rodent prefrontal cortex. PLoS One 7:e37541. doi: 10.1371/JOURNAL.PONE.0037541
Lin, M. T., Luján, R., Watanabe, M., Adelman, J. P., and Maylie, J. (2008). SK2 channel plasticity contributes to LTP at Schaffer collateral-CA1 synapses. Nat. Neurosci. 11, 170–177. doi: 10.1038/NN2041
Logan, R. W., Seggio, J. A., Robinson, S. L., Richard, G. R., and Rosenwasser, A. M. (2010). Circadian wheel-running activity during withdrawal from chronic intermittent ethanol exposure in mice. Alcohol 44, 239–244. doi: 10.1016/j.alcohol.2010.02.011
Logan, R. W., Williams, W. P., and McClung, C. A. (2014). Circadian rhythms and addiction: mechanistic insights and future directions. Behav. Neurosci. 13, S263–S264. doi: 10.1016/j.jtho.2018.08.138
Meyer, C., Schoettner, K., and Amir, S. (2022). The effects of circadian desynchronization on alcohol consumption and affective behavior during alcohol abstinence in female rats. Front. Behav. Neurosci. 16:1044783. doi: 10.3389/fnbeh.2022.1044783
Mill, D. J., Bito-Onon, J. J., Simms, J. A., Li, R., and Bartlett, S. E. (2013). Fischer rats consume 20% ethanol in a long-term intermittent-access two-bottle-choice paradigm. PLoS One 8:e79824. doi: 10.1371/journal.pone.0079824
Mulholland, P. J., Becker, H. C., Woodward, J. J., and Chandler, L. J. (2011). Small conductance calcium-activated potassium type 2 channels regulate alcohol-associated plasticity of glutamatergic synapses. Biol. Psychiatry 69, 625–632. doi: 10.1016/j.biopsych.2010.09.025
Musiek, E. S., Lim, M. M., Yang, G., Bauer, A. Q., Qi, L., Lee, Y., et al. (2013). Circadian clock proteins regulate neuronal redox homeostasis and neurodegeneration. J. Clin. Invest. 123, 5389–5400. doi: 10.1172/JCI70317
Nagy, J., Kolok, S., Boros, A., and Dezső, P. (2005). Role of altered structure and function of NMDA receptors in development of alcohol dependence. Curr. Neuropharmacol. 3, 281–297. doi: 10.2174/157015905774322499
Nagy, J., Kolok, S., Dezso, P., Boros, A., and Szombathelyi, Z. (2003). Differential alterations in the expression of NMDA receptor subunits following chronic ethanol treatment in primary cultures of rat cortical and hippocampal neurones. Neurochem. Int. 42, 35–43. doi: 10.1016/S0197-0186(02)00062-1
Obad, A., Peeran, A., Little, J. I., Haddad, G. E., and Tarzami, S. T. (2018). Alcohol-mediated organ damages: heart and brain. Front. Pharmacol. 9:81. doi: 10.3389/fphar.2018.00081
Oishi, K., Higo-Yamamoto, S., Yamamoto, S., and Yasumoto, Y. (2015). Disrupted light–dark cycle abolishes circadian expression of peripheral clock genes without inducing behavioral arrhythmicity in mice. Biochem. Biophys. Res. Commun. 458, 256–261. doi: 10.1016/J.BBRC.2015.01.095
Okuliarova, M., Molcan, L., and Zeman, M. (2016). Decreased emotional reactivity of rats exposed to repeated phase shifts of light-dark cycle. Physiol. Behav. 156, 16–23. doi: 10.1016/j.physbeh.2016.01.003
Ozburn, A. R., Falcon, E., Mukherjee, S., Gillman, A., Arey, R., Spencer, S., et al. (2013). The role of clock in ethanol-related behaviors. Neuropsychopharmacology 38, 2393–2400. doi: 10.1038/npp.2013.138
Pandey, S. C., Roy, A., Zhang, H., and Xu, T. (2004). Partial deletion of the cAMP response element-binding protein gene promotes alcohol-drinking behaviors. J. Neurosci. 24, 5022–5030. doi: 10.1523/JNEUROSCI.5557-03.2004
Paxinos, G., and Watson, C. (2006). The rat brain in stereotaxic coordinates: Hard cover edition-George Paxinos, Charles Watson-Google Книги. Available at: https://books.google.ca/books?hl=de&lr=&id=0prYfdDbh58C&oi=fnd&pg=PP1&dq=paxinos+g+watson+c+rat+brain&ots=-9juH1_IDn&sig=wETS1365dFZ3etLIXmOvfb3ocWg#v=onepage&q=paxinosgwatsoncratbrain&f=false
Perreau-Lenz, S., and Spanagel, R. (2015). Clock genes×stress×reward interactions in alcohol and substance use disorders. Alcohol 49, 351–357. doi: 10.1016/j.alcohol.2015.04.003
Pfaffl, M. W. (2001). A new mathematical model for relative quantification in real-time RT–PCR. Nucleic Acids Res. 29, 45e–445e. doi: 10.1093/nar/29.9.e45
Richter, K., Peter, L., Rodenbeck, A., Weess, H. G., Riedel-Heller, S. G., and Hillemacher, T. (2021). Shiftwork and alcohol consumption: a systematic review of the literature. Eur. Addict. Res. 27, 9–15. doi: 10.1159/000507573
Rizk, A. A., Jenkins, B. W., Al-Sabagh, Y., Hamidullah, S., Reitz, C. J., Rasouli, M., et al. (2022). The impact of sex, circadian disruption, and the clock∆19/∆19 genotype on alcohol drinking in mice. Genes 13:701. doi: 10.3390/genes13040701
Rosenwasser, A. M., Clark, J. W., Fixaris, M. C., Belanger, G. V., and Foster, J. A. (2010). Effects of repeated light-dark phase shifts on voluntary ethanol and water intake in male and female Fischer and Lewis rats. Alcohol 44, 229–237. doi: 10.1016/j.alcohol.2010.03.002
Rosenwasser, A. M., Fecteau, M. E., and Logan, R. W. (2005). Effects of ethanol intake and ethanol withdrawal on free-running circadian activity rhythms in rats. Physiol. Behav. 84, 537–542. doi: 10.1016/j.physbeh.2005.01.016
Rosenwasser, A. M., McCulley, W. D., and Fecteau, M. (2014). Circadian activity rhythms and voluntary ethanol intake in male and female ethanol-preferring rats: effects of long-term ethanol access. Alcohol 48, 647–655. doi: 10.1016/j.alcohol.2014.07.010
Schoettner, K., Alonso, M., Button, M., Goldfarb, C., Herrera, J., Quteishat, N., et al. (2022). Characterization of affective behaviors and motor functions in mice with a striatal-specific deletion of Bmal1 and Per2. Front. Physiol. 13:922080. doi: 10.3389/fphys.2022.922080
Sheela Rani, C. S., and Ticku, M. K. (2006). Comparison of chronic ethanol and chronic intermittent ethanol treatments on the expression of GABA (A) and NMDA receptor subunits. Alcohol 38, 89–97. doi: 10.1016/J.ALCOHOL.2006.05.002
Simms, J. A., Steensland, P., Medina, B., Abernathy, K. E., Chandler, L. J., Wise, R., et al. (2008). Intermittent access to 20% ethanol induces high ethanol consumption in long-Evans and Wistar rats. Alcohol. Clin. Exp. Res. 32, 1816–1823. doi: 10.1111/j.1530-0277.2008.00753.x
Simonyi, A., Christian, M. R., Sun, A. Y., and Sun, G. Y. (2004). Chronic ethanol-induced subtype-and subregion-specific decrease in the mRNA expression of metabotropic glutamate receptors in rat Hippocampus. Alcohol. Clin. Exp. Res. 28, 1419–1423. doi: 10.1097/01.ALC.0000139825.35438.A4
Spanagel, R., Pendyala, G., Abarca, C., Zghoul, T., Sanchis-Segura, C., Magnone, M. C., et al. (2005a). The clock gene Per2 influences the glutamatergic system and modulates alcohol consumption. Nat. Med. 11, 35–42. doi: 10.1038/nm1163
Spanagel, R., Rosenwasser, A. M., Schumann, G., and Sarkar, D. K. (2005b). Alcohol consumption and the body’s biological clock. Alcohol. Clin. Exp. Res. 29, 1550–1557. doi: 10.1097/01.alc.0000175074.70807.fd
Sun, J., Liu, Y., Baudry, M., and Bi, X. (2020). SK2 channel regulation of neuronal excitability, synaptic transmission, and brain rhythmic activity in health and diseases. Biochim. Biophys. Acta Molecular Cell Res. 1867:118834. doi: 10.1016/J.BBAMCR.2020.118834
Szántóová, K., Zeman, M., Veselá, A., and Herichová, I. (2011). Effect of phase delay lighting rotation schedule on daily expression of per2, bmal1, rev-erbα, pparα, and pdk4 genes in the heart and liver of Wistar rats. Mol. Cell. Biochem. 348, 53–60. doi: 10.1007/s11010-010-0636-x
Wang, J., Carnicella, S., Phamluong, K., Jeanblanc, J., Ronesi, J. A., Chaudhri, N., et al. (2007). Ethanol induces long-term facilitation of NR2B-NMDA receptor activity in the dorsal striatum: implications for alcohol drinking behavior. J. Neurosci. Off. J. Soc. Neurosci. 27, 3593–3602. doi: 10.1523/JNEUROSCI.4749-06.2007
Werme, M., Lindholm, S., Thorén, P., Franck, J., and Brené, S. (2002). Running increases ethanol preference. Behav. Brain Res. 133, 301–308. doi: 10.1016/S0166-4328(02)00027-X
Wise, R. A. (1973). Voluntary ethanol intake in rats following exposure to ethanol on various schedules. Psychopharmacologia 29, 203–210. doi: 10.1007/BF00414034
Wollnik, F., and Turek, F. W. (1988). Estrous correlated modulations of circadian and ultradian wheel-running activity rhythms in LEW/Ztm rats. Physiol. Behav. 43, 389–396. doi: 10.1016/0031-9384(88)90204-1
World Health Organization . (2018). Global status report on alcohol and health 2018. Available at: https://www.who.int/publications/i/item/9789241565639
Wotus, C., Lilley, T. R., Neal, A. S., Suleiman, N. L., Schmuck, S. C., Smarr, B. L., et al. (2013). Forced Desynchrony reveals independent contributions of Suprachiasmatic oscillators to the daily plasma corticosterone rhythm in male rats. PLoS One 8:e68793. doi: 10.1371/journal.pone.0068793
Keywords: clock genes, alcohol, females, gene expression, neuroinflammation
Citation: Meyer C, Schoettner K and Amir S (2024) Effects of chronodisruption and alcohol consumption on gene expression in reward-related brain areas in female rats. Front. Mol. Neurosci. 17:1493862. doi: 10.3389/fnmol.2024.1493862
Edited by:
Hiroyuki Aizawa, Aizawa Science Museum, JapanReviewed by:
Akihito Yasuoka, Seitoku University, JapanWoosung Ahn, Cedars Sinai Medical Center, United States
Copyright © 2024 Meyer, Schoettner and Amir. This is an open-access article distributed under the terms of the Creative Commons Attribution License (CC BY). The use, distribution or reproduction in other forums is permitted, provided the original author(s) and the copyright owner(s) are credited and that the original publication in this journal is cited, in accordance with accepted academic practice. No use, distribution or reproduction is permitted which does not comply with these terms.
*Correspondence: Shimon Amir, c2hpbW9uLmFtaXJAY29uY29yZGlhLmNh; Christiane Meyer, Y2hyaXN0aWFuZS5tZXllckBjb25jb3JkaWEuY2E=