- Key Laboratory of Neuroregeneration of Jiangsu and Ministry of Education, School of Medicine, Co-innovation Center of Neuroregeneration, Nantong University, Nantong, China
Nerve injuries significantly impact the quality of life for patients, with severe cases posing life-threatening risks. A comprehensive understanding of the pathophysiological mechanisms underlying nerve injury is crucial to the development of effective strategies to promote nerve regeneration. Circular RNAs (circRNAs), a recently characterized class of RNAs distinguished by their covalently closed-loop structures, have been shown to play an important role in various biological processes. Numerous studies have highlighted the pivotal role of circRNAs in nerve regeneration, identifying them as potential therapeutic targets. This review aims to succinctly outline the latest advances in the role of circRNAs related to nerve injury repair and the underlying mechanisms, including peripheral nerve injury, traumatic brain injury, spinal cord injury, and neuropathic pain. Finally, we discuss the potential applications of circRNAs in drug development and consider the potential directions for future research in this field to provide insights into circRNAs in nerve injury repair.
1 Introduction
In clinical practice, injuries to the central and peripheral nervous systems are a frequent occurrence. Such neurological injuries, encompassing peripheral nerve injury (PNI), traumatic brain injury (TBI), spinal cord injury (SCI), and neuropathic pain (NP), not only detrimentally impact patient quality of life, but bring substantial economic burdens to families and society at large. However, the current therapeutic options for these conditions are notably limited, largely due to an inadequate understanding of the molecular changes following these injuries.
Circular RNA (circRNA) is a type of covalently closed single-stranded RNA, generated through back-splicing events during canonical RNA splicing, and is ubiquitously present in eukaryotic cells (Aufiero et al., 2019; Liu and Chen, 2022). Remarkable progress in circRNA research have been witnessed in recent years, revealing its pivotal roles in both physiological and pathological processes (Kristensen et al., 2022). The expression and function of circRNAs are subject to stringent regulation, and their expression levels are closely tied to cell type, intracellular signaling cascades, and environmental cues. Furthermore, circRNAs exhibit tissue-specific and cell-specific expression patterns, and their biogenesis is governed by specific cis- and trans-acting elements (Yang et al., 2021). A growing body of evidence highlights the diverse regulatory functions of circRNAs, including the roles as miRNA sponges, anchors for circRNA binding proteins (cRBPs), transcriptional regulators, molecular scaffolds, and sources for translation of small proteins/peptides (Huang et al., 2020; Misir et al., 2022). Notably, dysregulated circRNA expression has been implicated in a variety of diseases, including central nervous system (CNS) disorders, tumors, cardiovascular pathologies, and metabolic disorders, etc. (Yang et al., 2021; Fan et al., 2022; Zhang et al., 2022). Furthermore, circRNAs exhibit remarkable stability in both intracellular and extracellular environments (Liu and Chen, 2022). Consequently, they have emerged as promising biomarkers for the diagnosis of various diseases. In recent years, there has been a growing interest in the study of circRNAs in the context of nerve injury. Evidence suggests that circRNAs may have the potential to ameliorate acute CNS injuries through diverse molecules and pathways. After peripheral nerve injuries, circRNAs have been shown to promote the repair of neurological injuries by facilitating axon regeneration, promoting Schwann cell (SC) proliferation, and regulating neuronal autophagy (Zhou et al., 2018; Sohn and Park, 2020; Liu et al., 2022). Thus, circRNAs hold promise for the treatment of nerve injuries. This review aims to summarize recent advances in circRNA expression profiles (Supplementary Table S1) and roles (Tables 1–4) in this field and present a prospective view of circRNA in the treatment of nerve injury.
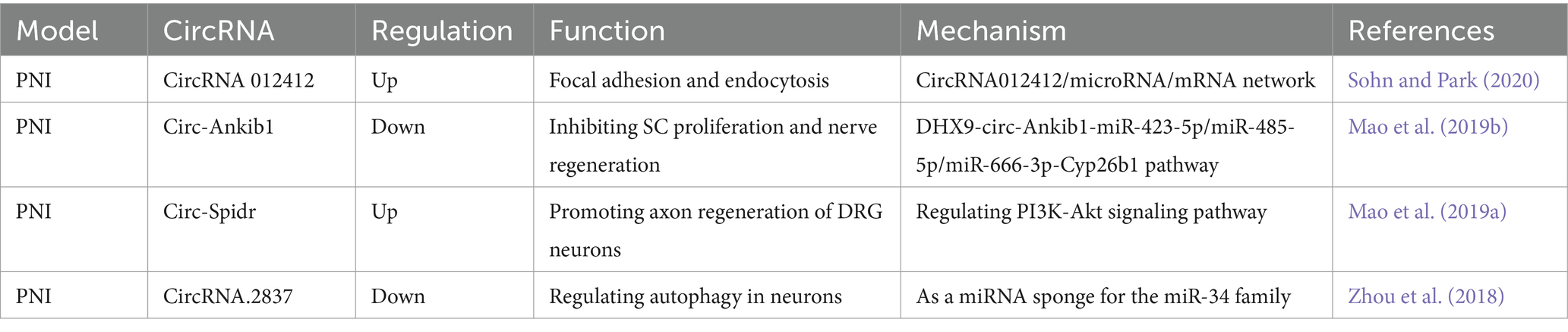
Table 1. Functional characterization of the circRNAs post peripheral nerve injury (PNI) and the underlying mechanisms.
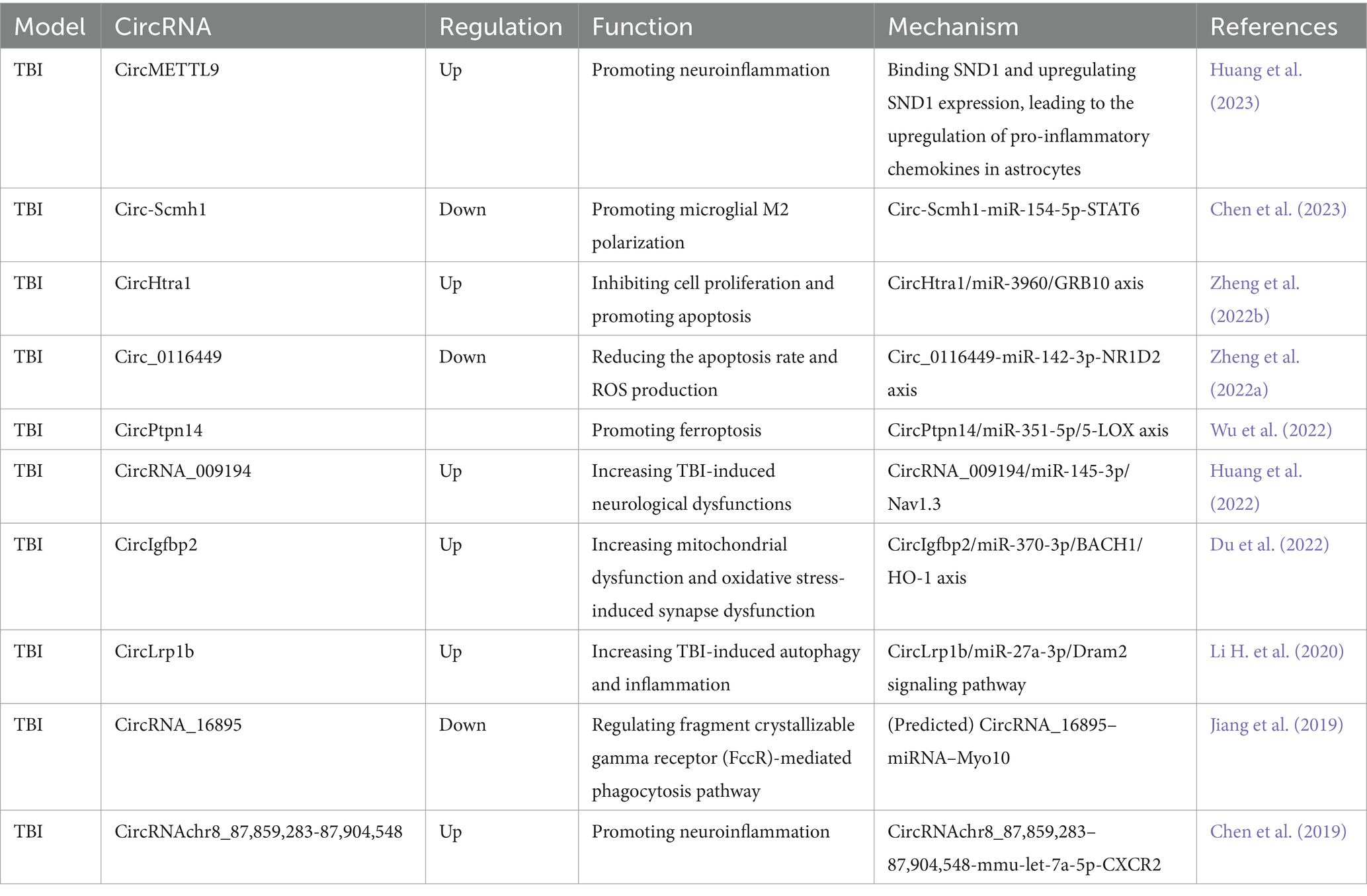
Table 2. Functional characterization of the circRNAs post traumatic brain injury (TBI) and the underlying mechanisms.
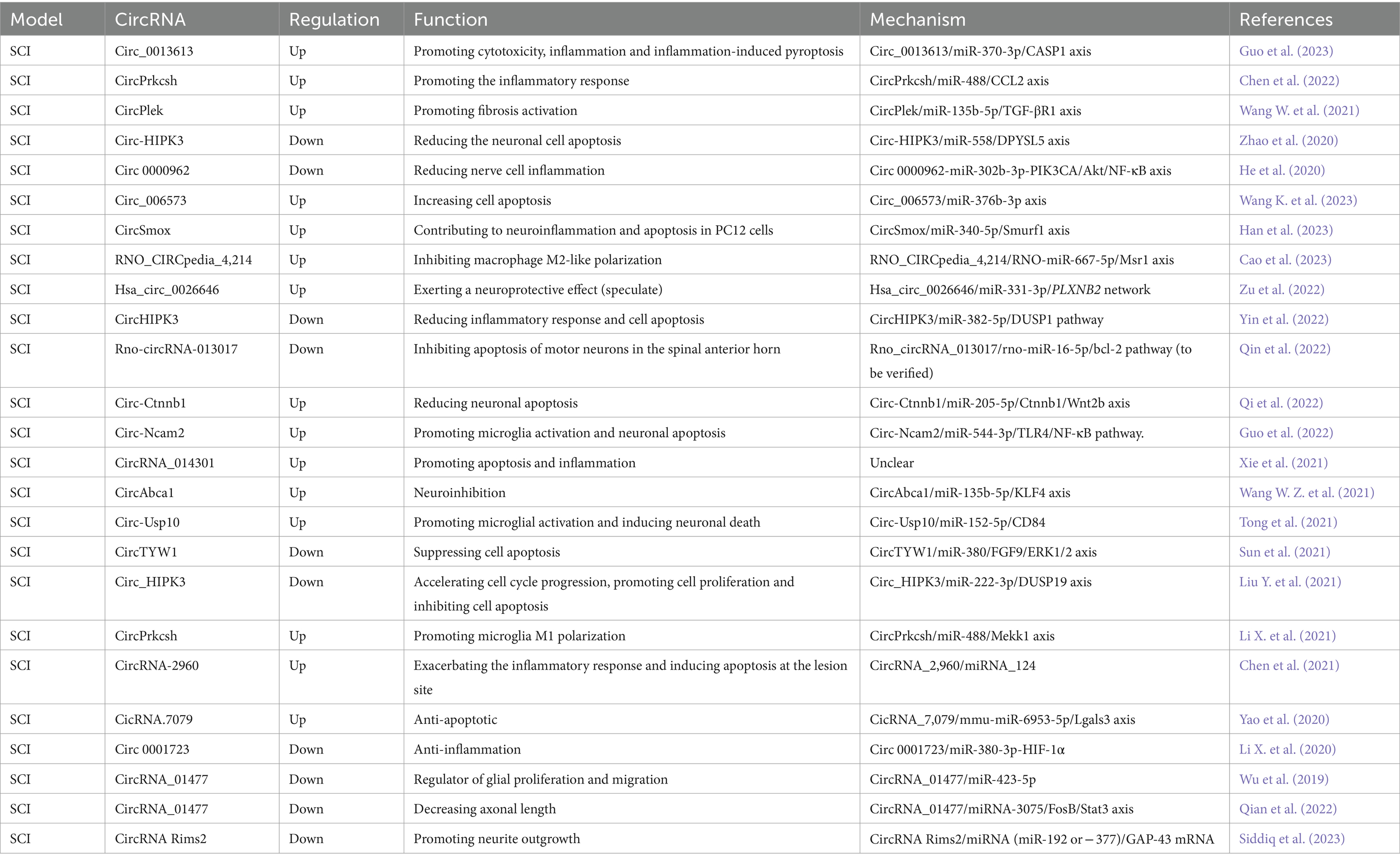
Table 3. Functional characterization of the circRNAs post spinal cord injury (SCI) and the underlying mechanisms.
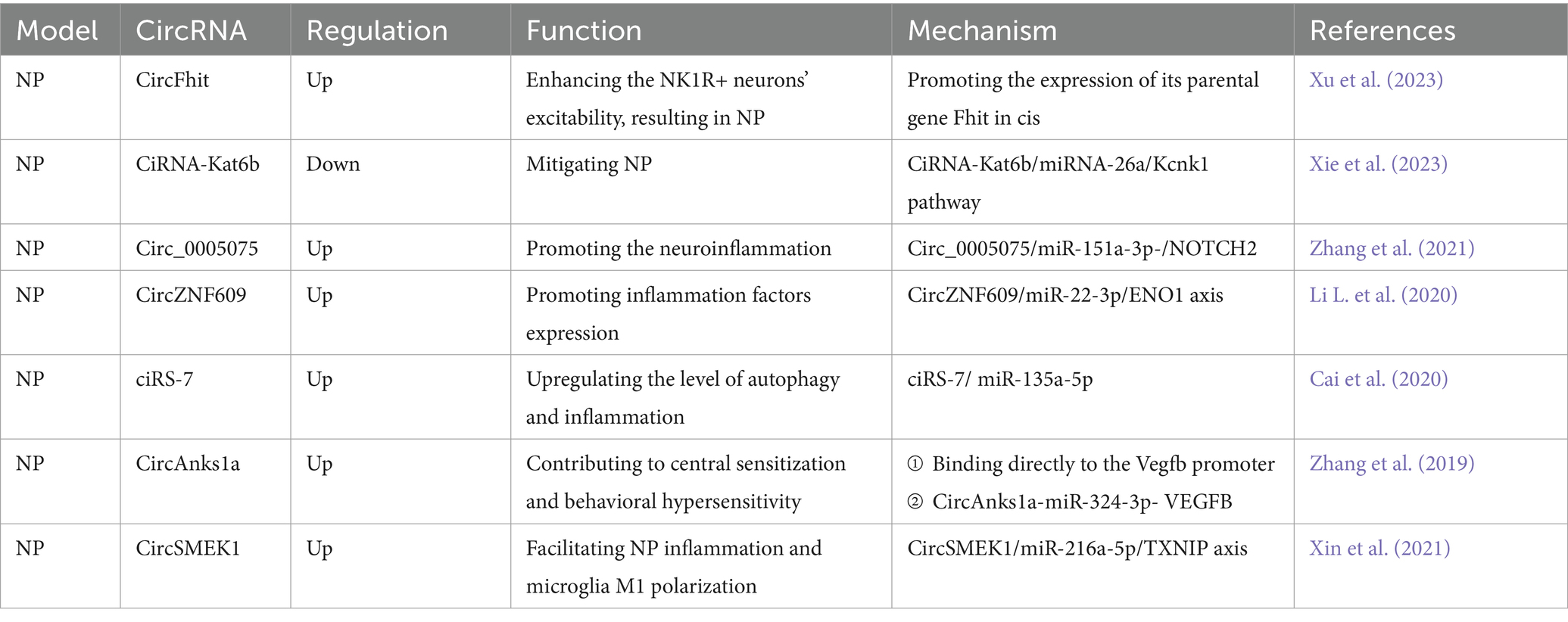
Table 4. Functional characterization of the circRNAs post neuropathic pain (NP) and the underlying mechanisms.
2 The roles of circRNAs in peripheral nerve injury
Peripheral nerve injury (PNI) is a condition that results from trauma or disease, affecting nerves outside the CNS, leading to sensory and motor dysfunction. In recent years, there has been an increasing focus on the role of circRNAs in peripheral nerve injury. CircRNAs have been shown to participate in various biological functions during axonal regeneration and degeneration through the circRNA/miRNA/mRNA network. This has been demonstrated by the up-regulation of circRNA 012412 following PNI and the identification of multiple biological functions of the circRNA 012412/microRNA/mRNA network (Sohn and Park, 2020). Our previous work has shown that SCs play a critical role in the repair of nerve injury, and the circRNA circ-Ankib1 is specifically enriched in SCs. After PNI, circ-Ankib1 is down-regulated through the DHX9-circ-Ankib1-miR-423-5p/miR-485-5p/miR-666-3p-Cyp26b1 pathway. This down-regulation induced SC cell proliferation and promoted nerve regeneration (Mao et al., 2019b). Consequently, the targeted modulation of circ-Ankib1 emerges as a promising therapeutic strategy for the enhancement of axonal regeneration after nerve injury. Another circRNA, circ-Spidr, is enriched in the cytoplasm of dorsal root ganglion (DRG) neurons, undergoes upregulation following PNI and contributes to axonal regeneration in these neurons, partially through its regulatory influence on the PI3K-Akt signaling pathway (Mao et al., 2019a). PI3K/Akt pathway was reported to be involved in both neuronal survival and regeneration (Ahn, 2014; Zhang et al., 2014). It suggests that overexpression of circ-Spidr may serve as a viable therapeutic approach for the promotion of axonal regeneration after nerve injury. Additionally, circRNA 2,837 is down-regulated after PNI, and its reduction has been shown to alleviate sciatic nerve injury by inducing autophagy in vivo. Knockdown of circRNA 2,837 has been shown to protect neurons from nerve injury by acting as a sponge for members of the miR-34 family members (Zhou et al., 2018). These discoveries underscore the intricate regulatory roles of circRNAs in peripheral nerve injury and hint at their potential therapeutic utility in the context of nerve regeneration (Figure 1).
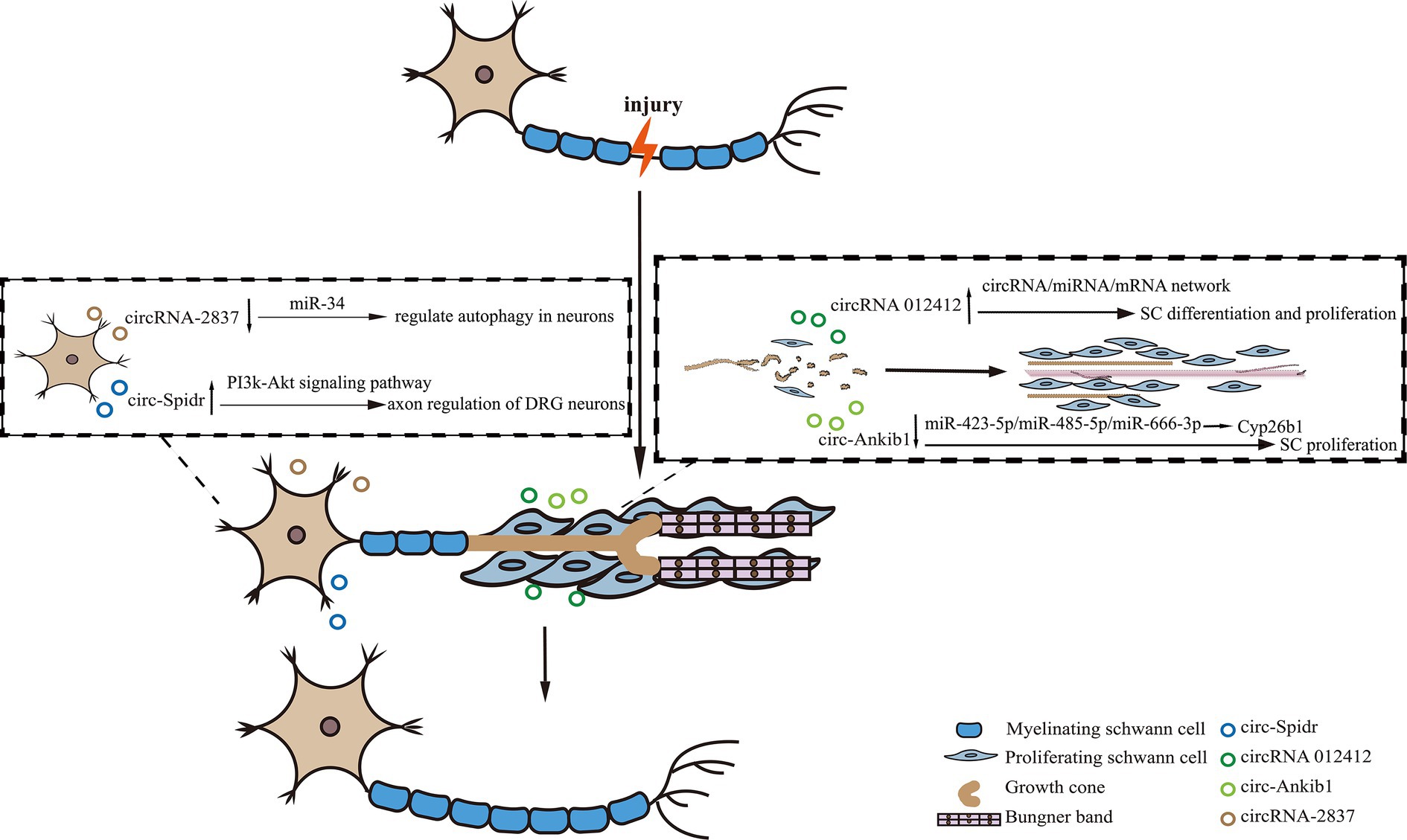
Figure 1. The diagram showing the role of circRNAs in peripheral nerve injury. In the process of peripheral nerve regeneration after injury, circRNAs play crucial roles in neurons or Schwann cells. For instance, circRNA-2837 regulates neuron autophagy, while circ-Spidr enhances axon regeneration. In Schwann cells, circRNA012142 and circ-Ankib1, respectively, regulate cell differentiation and/or proliferation to promote nerve injury repair.
3 The roles of circRNAs in traumatic brain injury
Traumatic brain injury (TBI) refers to brain damage caused by an external force, such as a blow or jolt to the head or penetration of the skull. The immediate aftermath of TBI can manifest as unconsciousness, confusion, memory loss, and neurological deficits, while the enduring effects may include cognitive impairment, motor dysfunction, and psychological disorders. Neuroinflammation plays a crucial role in the cellular response after TBI (Wu et al., 2021). In animal models of TBI, various circRNAs have been linked to neuroinflammation. For example, circMETTL9 has been found to bind to SND1, a multifunctional protein that is involved in regulating neuroinflammation, boosts SND1 expression and elevates the production of pro-inflammatory chemokines in astrocytes. This cascade of events triggers neuroinflammation and neurodegeneration (Huang et al., 2023). In another study, the circRNA circLrp1b was identified as a mediator in the inhibition of Dexmedetomidine in the inflammatory response and autophagy involved in TBI in vivo through its interaction with the miR-27a-3p/Dram2 axis (Li H. et al., 2020). Furthermore, the circRNA chr8_87,859,283-87,904,548 was found to be significantly upregulated following TBI, thereby promoting neuroinflammation by increasing CXCR2 protein levels via the sequestration of mmu-let-7a-5p (Chen et al., 2019). Notably, in the serum of mouse with TBI, a down-regulation of circ-Scmh1 was observed in exosomes derived from adipose-derived stem cells (ADSCs). The overexpression of circ-Scmh1 was shown to mitigate inflammation-induced hippocampal nerve injury by inducing the polarization of M2 microglia and suppressing inflammation (Chen et al., 2023). These findings underscore the crucial role of circRNA in the regulation of neuroinflammation after TBI. Moreover, lipid peroxidation has been established as a biomarker for secondary brain injury in both traumatic and non-traumatic contexts (Piastra et al., 2020). Investigating the contributions of circRNAs and lipid peroxidation to the sequelae of TBI could potentially lead to the development of more effective treatments and interventions for those afflicted with this condition. In a study by Zheng et al., 2022a, the down-regulation of circ_0116449 was observed and its role in regulating lipid metabolism in TBI through the circ_0116449-miR-142-3p-NR1D2 axis was elucidated. Another circRNA, circPtpn14, was negatively regulated after melatonin treatment, which exerted anti-ferroptotic and anti-endoplasmic reticulum (ER) stress effects in brain injury by alleviating lipid peroxidation via the circPtpn14/miR-351-5p/5-LOX axis (Wu et al., 2022).
After brain injury, a significant increase in CircHtra1 expression was noted, and this upregulation was correlated with the extent of injury severity. Research revealed that CircHtra1 contributed to neuronal loss by sponging miR-3960 and modulating GRB10 and apoptosis (Zheng et al., 2022b). Nav1.3, one of the large pore α subunit proteins of voltage-gated sodium channels, has been identified as a pivotal voltage sensor in the cell membrane. Notably, it exhibits a significant upregulation in rat’s cortex following TBI, with its expression levels closely linked to the severity of the TBI (Huang et al., 2013). A circRNA circRNA_009194 was observed to be upregulated after TBI. And its suppression, directly or indirectly, suppressed Nav1.3, thereby enhancing neurological outcomes in vivo (Huang et al., 2022). Mitochondrial dysfunction and oxidative stress represent pivotal pathological hallmarks in the early stages of TBI. Inhibition of circIgfbp2 was found to alleviate mitochondrial dysfunction and synaptic dysfunction induced by oxidative stress post TBI through the miR-370-3p/BACH1/HO-1 axis (Du et al., 2022). Furthermore, diminished levels of circRNA_16895 post TBI predicted the regulation of the regulatory fragment crystallizable γ receptor (FcγR)-mediated phagocytosis pathway, which is modulated by the circRNA_16895-miRNA-Myo10 axis (Jiang et al., 2019). These discoveries highlight the diverse roles of circRNAs in the pathogenesis of TBI and propose potential therapeutic targets.
4 The roles of circRNAs in spinal cord injury
Spinal cord injury (SCI) involves a cascade of biological processes that lead to tissue impairment and neurological dysfunction. The primary injury is precipitated by mechanical trauma, resulting in immediate disruption of neural pathways. Secondary injury mechanisms, including inflammation, excitotoxicity, and oxidative stress, further exacerbate tissue damage and lead to neuronal death. A comprehensive understanding of these processes is imperative for the development of effective therapies to mitigate secondary injury and promote neural repair in SCI. While the functions of certain circRNAs in SCI have been summarized in a previous review (Xu et al., 2021), this review integrates the latest insights into the function of circRNAs in SCI.
4.1 CircRNAs in inflammation
In the realm of SCI, inflammation is one of the predominant secondary injuries. The inflammatory cascade post SCI encompasses the accumulation of inflammatory cytokines, activation of microglia, recruitment of macrophages, and invasion of neutrophils (Freyermuth-Trujillo et al., 2022). Various circRNAs have been identified to modulate distinct inflammatory events to promote recovery after SCI, with details provided below.
4.1.1 Inflammatory cytokines
The interplay between inflammatory cytokines and circRNAs has emerged as a pivotal factor in the repair process for SCI. While certain inflammatory cytokines are conducive to spinal cord repair, others can exacerbate damage (Ren et al., 2018). Post-SCI, the surge in pro-inflammatory cytokines can lead to neuronal injury. Notably, specific circRNAs have been implicated in the release of pro-inflammatory cytokines. For instance, the expression of circ_0013613 is elevated following SCI. By knocking down circ_0013613, the cytotoxicity, inflammation, and inflammation-induced cell pyroptosis are mitigated via the miR-370-3p/caspase1 pathway, thus promoting SCI recovery (Guo et al., 2023). In a study by Chen et al. (2022), it was found that inhibiting circPrkcsh reduced the inflammatory response following SCI by upregulating miR-488, which in turn decreased CCL2 level and curtailed inflammatory cytokine secretion in vitro. Following SCI, circHecw1 is down-regulated, and its knockdown has been observed to attenuate inflammation in neuronal cells by modulating the miR-3551-3p/LRRTM1 signaling pathway (Ban et al., 2022). Additionally, circ0000962 is down-regulated after SCI, and its overexpression can reduce the inflammatory response through the miR-302b-3p-mediated PI3K/Akt/NF-κB signaling (He et al., 2020). CircSmox expression was also increased after SCI, and its knockdown alleviates LPS-induced neuroinflammation and apoptosis in PC12 cells via the miR-12-340p/Smurf5 axis, exerting a neuroprotective effect (Han et al., 2023). Furthermore, circRNA_014301 is associated with neuroinflammation and its silencing has been shown to attenuate apoptosis and inflammation in PC12 cells (Xie et al., 2021). These intricate interplays between circRNAs and inflammatory pathways highlight the potential for novel therapeutic interventions in the context of SCI.
4.1.2 Microglia and macrophages
After SCI, activated M1-like microglia initiate a cascade of neurotoxic responses, leading to cell apoptosis and necrosis (Freyermuth-Trujillo et al., 2022). In a mouse model of SCI, circ-Ncam2 is found to be up-regulated. The silence of circ-Ncam2 has demonstrated a reduction in LPS-induced microglia activation and neuronal apoptosis by blocking the TLR4/NF-κB pathway, functioning as a miR-544-3p sponge (Guo et al., 2022). Moreover, circ-Usp10, which is up-regulated after SCI, promotes microglia activation by targeting miR-152-5p/CD84 and induces neuronal death (Tong et al., 2021). Another study revealed that circPrkcsh promotes microglia M1 polarization through the miR-488/MEKK1/JNK/p38 MAPK signaling pathway, thus aggravating SCI injury. Consequently, down-regulating circPrkcsh can reduce inflammation and promote recovery from SCI (Li X. et al., 2021).
Macrophages can exert either beneficial or detrimental effects after SCI, depending on their phenotype, corresponding to the classes M1 (pro-inflammatory) and M2 (anti-inflammatory) (Shechter et al., 2013; Van Broeckhoven et al., 2021). Preventing pro-inflammatory macrophage/microglia polarization can promote damage repair. A study has constructed a ceRNA network closely related to SCI immunoinflammation, and the rno_CIRCpedia_4,214/rno-miR-667-5p/Msr1 axis may potentially promote macrophage M2-like polarization in SCI, serving as an effective strategy to mitigate the severity of secondary SCI injury (Cao et al., 2023). During the tissue repair process after SCI, a transmembrane receptor PLXNB2 was reported to induce microglia motility, steer injury-activated microglia/macrophages away from colliding cells, and facilitate matrix compaction (Zhou et al., 2020). Subsequently, Zu et al. proved that hsa_circ_0026646 was upregulated in SCI, which correlates with PLXNB2 by targeting miR-331-3p, providing novel insights into the regulatory mechanisms associated with SCI and potential therapeutic targets for its management (Zu et al., 2022).
4.2 CircRNAs in cell apoptosis
Secondary injury following SCI is considered to be due to the continuation of cellular destruction through cell apoptosis. Therefore, the identification of methods to inhibit cell apoptosis after SCI holds significant value in repairing nerve damage (Shi et al., 2021). Circ-HIPK3 has been shown to regulate the biological behavior of neuronal cells. After SCI, the expression of circ-HIPK3 was negatively regulated. However, up-regulation of circ-HIPK3 expression alleviated neuronal cell apoptosis in SCI by regulating the miR-588/DPYSL5 axis (Zhao et al., 2020). Furthermore, it has been shown that overexpression of circHIPK3 reduced the inflammatory response and neuronal apoptosis in AGE1.HN cells induced by oxygen–glucose deprivation, which is achieved by regulating the miR-382-5p/DUSP1 axis (Yin et al., 2022). In another study, circ_HIPK3 was found to alleviate CoCl2-induced apoptotic injury in neuronal cells by modulating the miR-222-3p/DUSP19 axis (Liu Y. et al., 2021). SCI inflicts injury on vascular endothelial cells, leading to the leakage of vascular material and accumulation of various inflammatory cytokines, which aggravate the inflammatory reaction (Freyermuth-Trujillo et al., 2022). In a study, circ_006573 was found to be significantly up-regulated after SCI, and the circ_006573/miR-376b-3p axis promoted the recovery of spinal cord function after injury by regulating vascular remodeling (Wang K. et al., 2023). Moreover, rno-circRNA-013017 was significantly down-regulated in rats after injury. Overexpression of rno-circRNA-013017 was found to inhibit apoptosis of motor neurons in the spinal anterior horn 3 days after SCI, thereby contributing to motor function in rats after SCI (Qin et al., 2022). Circ-Ctnnb1 exhibits high expression in both the rat model of SCI and neuronal cells challenged by hypoxia. Its deletion has been observed to elevate the apoptosis rate in neuronal cells challenged by hypoxia. Mechanistically, circ-Ctnnb1 activates the Wnt/β-catenin pathway, a well-known pathway that inhibits apoptosis of neuronal cells (Gao et al., 2016), reversing SCI by sponging miR-205-5p to up-regulate Ctnnb1 and Wnt2b (Qi et al., 2022). Additionally, CircTYW1 expression is negatively regulated after SCI, and circTYW1 has been found to promote neurological recovery in SCI of rats and inhibit apoptosis in spinal cord tissues. CircTYW1 can promote neurological recovery after SCI through the miR-380/FGF9/ERK1/2 regulatory axis. Thus, overexpression of circTYW1 may serve as a novel therapeutic approach to SCI (Sun et al., 2021).
4.3 CircRNAs in axonal regeneration
The axonal regeneration of mature neurons is inhibited, while long-distance regeneration of axons plays a critical role in functional recovery after SCI (Alilain et al., 2011; Hilton et al., 2022). Certain circRNAs have been identified as potential players in axonal elongation and repair of SCI. CircRNA_01477 was down-regulated after SCI, and its knockdown significantly increased the axonal length by regulating the miRNA-3075/FosB/Stat3 axis (Qian et al., 2022). Axonal translation is believed to enable neurorepair after SCI. CircRNA Rims2 has been shown to regulate axonal regeneration through miRNAs that modulate the GAP-43 axonal translation, highlighting a crucial role of circRNA in axonal translation in nerve repair (Siddiq et al., 2023). In an inflammatory environment, fibroblasts and astrocytes activation leads to scar formation, which is a significant barrier to axonal regeneration after SCI. CircPlek has been demonstrated to promote fibrotic activation by modulating the miR-135b-5p/TGF-βR1 axis following SCI (Wang W. et al., 2021). In a separate investigation concerning traumatic SCI, silencing circAbca1 is believed to have a neuroprotective effect via the circAbca1/miR-135b-5p/KLF4 network. Within this network, the zinc finger-containing transcription factor KLF4 is implicated in the inhibition of axon regeneration (Qin et al., 2013; Wang W. Z. et al., 2021).
4.4 CircRNAs in exosomes
Exosomes derived from mesenchymal stem cells hold great promise for the treatment of SCI (Liu W. Z. et al., 2021). Specifically, hypoxia-induced expression of exosomal circular RNA OXNAD1 (circOXNAD1), derived from human umbilical cord mesenchymal stem cells (HucMSCs), has been demonstrated to alleviate spinal cord ischemia–reperfusion injury in SCI through the circOXNAD1/miR-29a-3p/FOXO3a axis (Wang X. et al., 2023). Moreover, bone marrow mesenchymal stem cells (BMSCs) have exhibited certain therapeutic effects on SCI (Cofano et al., 2019), and BMSC-derived exosomes have been shown to improve recovery from SCI by alleviating inflammasome-associated sepsis by delivering circ_003564 (Zhao Y. et al., 2022). CircZFHX3, also derived from exosomes, has been observed to counteract LPS-induced damage in BV-2 cells, partially by regulating the miR-16-5p/IGF-1 axis, suggesting its potential as a therapeutic strategy for SCI (Tian et al., 2022).
5 The roles of circRNAs in neuropathic pain
Neuropathic pain (NP) typically arises from nerve injury, where damage or dysfunction disrupts normal nerve signaling. This chronic pain state can be precipitated by a variety of factors, including trauma, disease, or surgical intervention. Nerve injury triggers abnormal nerve firing, heightened sensitivity, and altered nociceptive perceptions, all of which contribute to enduring discomfort. Additionally, inflammatory responses and biochemical changes further exacerbate pain sensations. Notably, NP frequently persists even after the initial injury has healed, significantly impacting the quality of life. Deciphering the interplay between these factors is crucial for the development of targeted interventions aimed at alleviating NP and improving patient outcomes. A recent study has identified circFhit, an exon-intron circRNA expressed in GABAergic neurons, as a significant contributor to NP by increasing NK1R neuronal hyperexcitation through the regulation of Fhit in cis (Xu et al., 2023). Central sensitization, particularly in the dorsal spinal cord, is considered a central role player in pain hypersensitivity. In NP models, down-regulation of ciRNA-Kat6b in the spinal dorsal horn exacerbated pain hypersensitivity, while blocking this down-regulation attenuated NP through the ciRNA-Kat6b/miRNA-26a/Kcnk1 pathway (Xie et al., 2023). Post-nerve injury, the up-regulation of inflammation-associated circ_0005075 exacerbated pain progression, which can be alleviated by circ_0005075 deletion-induced miR-151a-3p up-regulation and NOTCH2 signaling inactivation (Zhang et al., 2021). Similarly, in a CCI rat model, circZNF609 exacerbated NP via the miR-22-3p/ENO1 axis (Li L. et al., 2020). Moreover, the upregulation of ciRS-7 has been observed to promote NP through interaction with miR-135a-5p, enhancing inflammation and autophagy (Cai et al., 2020). Another up-regulated circRNA in dorsal horn neurons during NP is circAnks1a. The interaction between circAnks1a and YBX1 promotes YBX1 translocation into the nucleus and enhances its binding to the Vegfb promoter, thus promoting Vegfb transcription in dorsal horn neurons after nerve injury. Increased levels of circAnks1a also act as a sponge for miR-324-3p to modulate the translation of Vegfb mRNA in the dorsal horn. Down-regulation of circAnks1a attenuates pain-like behaviors induced by nerve injury (Zhang et al., 2019). Thioredoxin interacting protein (TXNIP) is highly expressed in NP, and usually binds to the NLRP3 inflammasome to affect inflammation, oxidative phosphorylation, and apoptosis to modulate pain. A study found that the expression of TXNIP is mediated by circSMEK1, which is upregulated in NP and induces NP in rats by acting as a sponge for microRNA-216a-5p (Xin et al., 2021). In summary, these findings highlight the multifaceted roles of circRNAs in NP pathways, providing valuable information on potential therapeutic targets for pain management.
6 Summary and perspective
This review article offers a comprehensive summary of recent advancements in the roles of circRNAs in nerve injury and repair. The majority of current studies focus on elucidating the involvement of circRNAs in signaling pathways and gene regulatory networks pertinent to nerve injury. Two primary mechanisms have been identified: acting as sponge RNAs and directly binding to proteins. However, circRNAs exhibit multiple other functions, including translating polypeptides, forming pseudogenes, and so on (Misir et al., 2022). Future studies should explore the functions and mechanisms of circRNAs in nerve injury repair based on these alternative functions.
Exosomes derived from mesenchymal stem cells play a crucial role in nerve regeneration after injury. This review also highlights several exosomal circRNAs that contribute to nerve injury repair, particularly in TBI and SCI. However, the role of exosomal circRNAs in peripheral nerve injury and NP requires further investigation. Several studies have shown that Schwann cell-derived exosomes can treat peripheral nerve injury by inhibiting inflammation and promoting axonal regeneration (Qing et al., 2018; Sun et al., 2023). Additionally, exosomes derived from Schwann cell-like cells can also promote nerve injury recovery by facilitating myelin formation and angiogenesis (Hu et al., 2023). However, it remains to be determined whether these exosomes contain circRNAs that promote nerve injury repair, serving as a direction for future research on peripheral nerve injury repair.
Given their greater stability in comparison to linear RNAs, circRNAs are expected to emerge as the preferred RNA platform in the pharmaceutical industry. CircRNA vaccines share numerous similarities with linear mRNA vaccines and hold immense potential as the next generation of RNA-based vaccine platforms (Niu et al., 2023). Compared to mRNA vaccines, circRNA vaccines have the advantage of continuous expression and are less susceptible to degradation (Zhao X. et al., 2022). Consequently, they can generate more durable antigens, thereby eliciting a more robust immune response. Currently, several circRNA-based drugs or vaccines have emerged, such as the SARS-CoV-2 circRNA vaccine, which expresses the spike protein trimer RBD and can effectively stimulate the production of neutralizing antibodies and T-cell responses, providing substantial protection for the body (Qu et al., 2022). Currently, there is no circRNA-based drug reported in repairing human nerve injuries. However, several studies have found that the expression of certain circRNAs is significantly increased in patients with acute ischemic stroke, and their levels positively correlate with infarct volume, suggesting their potential as biomarkers for the diagnosis and prognosis of acute ischemic stroke (Zuo et al., 2020; Li S. et al., 2021). This highlights the important roles circRNAs play in human pathophysiology in the nervous system. Regarding the function of circRNA in nerve injury repair, current research is primarily focused on rodent models. The translational potential of circRNAs identified in these models to human clinical applications remains an important direction for future research. Due to the high conservation of circRNA across species, it is possible that the targets identified in rodents may have significant clinical effects in human as well. Studies have shown that circRNAs exhibit a very high degree of conservation among primates (Santos-Rodriguez et al., 2021). Therefore, further clinical studies or trials of circRNA-based therapeutics could be conducted in non-human primates before advancing to human clinical trials. However, two challenges must be addressed for the future applications of circular RNA drugs or vaccines: the development of efficient production methods and the optimization of delivery systems. Purification of circular RNA products using existing technology is extremely challenging, and effective isolation and purification represent indispensable steps in the production of circular RNA products. The advent of delivery vehicles such as lipid nanoparticles offers hope for the successful delivery of circular RNA (Loan Young et al., 2023). Further investigation addressing these two challenges is elementary to fully explore the potential of applying circRNA-based strategies in clinics. Hopefully, circRNA-based drugs targeting nerve damage repair can be developed soon.
7 Conclusion
In conclusion, the investigation of circRNAs in nerve injury remains highly valuable, and more research is needed to uncover new functions and mechanisms of circRNAs in the repair of nerve injury. Furthermore, the development of drugs based on circRNAs holds great promise in paving the way for novel breakthroughs in the treatment of nerve injury and merits further attention.
Author contributions
YZ: Writing – original draft, Writing – review & editing, Investigation. YD: Investigation, Writing – original draft, Writing – review & editing, Visualization. JY: Investigation, Writing – review & editing. BY: Writing – review & editing, Funding acquisition. DW: Writing – review & editing, Project administration, Supervision. SM: Project administration, Supervision, Writing – review & editing, Conceptualization, Funding acquisition, Writing – original draft.
Funding
The author(s) declare that financial support was received for the research, authorship, and/or publication of this article. This work was supported by the National Key R&D Program of China (2021YFA1201400, BY and SM); the National Natural Science Foundation of China (31700925, SM); the Collegiate Natural Science Fund of Jiangsu Province (21KJA180002, SM); and the Training Program of Innovation and Entrepreneurship for Graduates (202310304045Z, YZ).
Conflict of interest
The authors declare that the research was conducted in the absence of any commercial or financial relationships that could be construed as a potential conflict of interest.
Publisher’s note
All claims expressed in this article are solely those of the authors and do not necessarily represent those of their affiliated organizations, or those of the publisher, the editors and the reviewers. Any product that may be evaluated in this article, or claim that may be made by its manufacturer, is not guaranteed or endorsed by the publisher.
Supplementary material
The Supplementary material for this article can be found online at: https://www.frontiersin.org/articles/10.3389/fnmol.2024.1419520/full#supplementary-material
References
Ahn, J. Y. (2014). Neuroprotection signaling of nuclear akt in neuronal cells. Exp. Neurobiol. 23, 200–206. doi: 10.5607/en.2014.23.3.200
Alilain, W. J., Horn, K. P., Hu, H., Dick, T. E., and Silver, J. (2011). Functional regeneration of respiratory pathways after spinal cord injury. Nature 475, 196–200. doi: 10.1038/nature10199
Aufiero, S., Reckman, Y. J., Pinto, Y. M., and Creemers, E. E. (2019). Circular RNAs open a new chapter in cardiovascular biology. Nat. Rev. Cardiol. 16, 503–514. doi: 10.1038/s41569-019-0185-2
Ban, D., Xiang, Z., Yu, P., and Liu, Y. (2022). Circular RNA Hecw1 regulates the inflammatory imbalance in spinal cord injury via miR-3551-3p/Lrrtm1 Axis. Appl. Biochem. Biotechnol. 194, 5151–5166. doi: 10.1007/s12010-022-03999-1
Cai, W., Zhang, Y., and Su, Z. (2020). ciRS-7 targeting miR-135a-5p promotes neuropathic pain in CCI rats via inflammation and autophagy. Gene 736:144386. doi: 10.1016/j.gene.2020.144386
Cao, J., Pan, C., Zhang, J., Chen, Q., Li, T., He, D., et al. (2023). Analysis and verification of the circRNA regulatory network RNO_CIRCpedia_ 4214/RNO-miR-667-5p/Msr1 axis as a potential ceRNA promoting macrophage M2-like polarization in spinal cord injury. BMC Genomics 24:181. doi: 10.1186/s12864-023-09273-w
Chen, J., Fu, B., Bao, J., Su, R., Zhao, H., and Liu, Z. (2021). Novel circular RNA 2960 contributes to secondary damage of spinal cord injury by sponging miRNA-124. J. Comp. Neurol. 529, 1456–1464. doi: 10.1002/cne.25030
Chen, S., Wang, X., Qian, Z., Wang, M., Zhang, F., Zeng, T., et al. (2023). Exosomes from ADSCs ameliorate nerve damage in the hippocampus caused by post traumatic brain injury via the delivery of circ-Scmh1 promoting microglial M2 polarization. Injury 54:110927. doi: 10.1016/j.injury.2023.110927
Chen, Z., Wang, H., Zhong, J., Yang, J., Darwazeh, R., Tian, X., et al. (2019). Significant changes in circular RNA in the mouse cerebral cortex around an injury site after traumatic brain injury. Exp. Neurol. 313, 37–48. doi: 10.1016/j.expneurol.2018.12.003
Chen, J. N., Zhang, Y. N., Tian, L. G., Zhang, Y., Li, X. Y., and Ning, B. (2022). Down-regulating circular RNA Prkcsh suppresses the inflammatory response after spinal cord injury. Neural Regen. Res. 17, 144–151. doi: 10.4103/1673-5374.314114
Cofano, F., Boido, M., Monticelli, M., Zenga, F., Ducati, A., Vercelli, A., et al. (2019). Mesenchymal stem cells for spinal cord injury: current options, limitations, and future of cell therapy. Int. J. Mol. Sci. 20:2698. doi: 10.3390/ijms20112698
Du, M., Wu, C., Yu, R., Cheng, Y., Tang, Z., Wu, B., et al. (2022). A novel circular RNA, circIgfbp2, links neural plasticity and anxiety through targeting mitochondrial dysfunction and oxidative stress-induced synapse dysfunction after traumatic brain injury. Mol. Psychiatry 27, 4575–4589. doi: 10.1038/s41380-022-01711-7
Fan, W., Pang, H., Xie, Z., Huang, G., and Zhou, Z. (2022). Circular RNAs in diabetes mellitus and its complications. Front. Endocrinol. 13:885650. doi: 10.3389/fendo.2022.885650
Freyermuth-Trujillo, X., Segura-Uribe, J. J., Salgado-Ceballos, H., Orozco-Barrios, C. E., and Coyoy-Salgado, A. (2022). Inflammation: a target for treatment in spinal cord injury. Cells 11:2692. doi: 10.3390/cells11172692
Gao, K., Shen, Z., Yuan, Y., Han, D., Song, C., Guo, Y., et al. (2016). Simvastatin inhibits neural cell apoptosis and promotes locomotor recovery via activation of Wnt/β-catenin signaling pathway after spinal cord injury. J. Neurochem. 138, 139–149. doi: 10.1111/jnc.13382
Guo, K., Chang, Y., Jin, Y., Yuan, H., and Che, P. (2022). Circ-Ncam2 (mmu_circ_0006413) participates in LPS-induced microglia activation and neuronal apoptosis via the TLR4/NF-κB pathway. J. Mol. Neurosci. 72, 1738–1748. doi: 10.1007/s12031-022-02018-6
Guo, Y., Ma, L., and Zhang, Z. (2023). circ_0013613 promotes the recovery of spinal cord injury by regulating mir-370-3p/caspase1 to regulate neuronal pyroptosis. Acta Biochim. Pol. 70, 91–97. doi: 10.18388/abp.2020_6244
Han, Z., Mou, Z., Jing, Y., Jiang, R., and Sun, T. (2023). CircSmox knockdown alleviates PC12 cell apoptosis and inflammation in spinal cord injury by miR-340-5p/Smurf1 axis. Immun. Inflamm. Dis. 11:e824. doi: 10.1002/iid3.824
He, R., Tang, G. L., Niu, L., Ge, C., Zhang, X. Q., Ji, X. F., et al. (2020). Quietness Circ 0000962 promoted nerve cell inflammation through PIK3CA/AKT/Nf-κB signaling by miR-302b-3p in spinal cord injury. Ann. Palliat. Med. 9, 190–198. doi: 10.21037/apm.2020.02.13
Hilton, B. J., Husch, A., Schaffran, B., Lin, T. C., Burnside, E. R., Dupraz, S., et al. (2022). An active vesicle priming machinery suppresses axon regeneration upon adult CNS injury. Neuron 110, 51–69.e7. doi: 10.1016/j.neuron.2021.10.007
Hu, T., Chang, S., Qi, F., Zhang, Z., Chen, J., Jiang, L., et al. (2023). Neural grafts containing exosomes derived from Schwann cell-like cells promote peripheral nerve regeneration in rats. Burns Trauma 11:tkad013. doi: 10.1093/burnst/tkad013
Huang, X. J., Mao, Q., Lin, Y., Feng, J. F., and Jiang, J. Y. (2013). Expression of voltage-gated sodium channel Nav1.3 is associated with severity of traumatic brain injury in adult rats. J. Neurotrauma 30, 39–46. doi: 10.1089/neu.2012.2508
Huang, X. J., Su, G. J., Wu, C. W., Sha, X. S., Zou, J. F., Liu, X. S., et al. (2022). Knockdown of rno_circRNA_009194 improves outcomes in traumatic brain injury rats through inhibiting voltage-gated Sodium Channel Nav1.3. J. Neurotrauma 39, 196–210. doi: 10.1089/neu.2020.7520
Huang, C., Sun, L., Xiao, C., You, W., Sun, L., Wang, S., et al. (2023). Circular RNA METTL9 contributes to neuroinflammation following traumatic brain injury by complexing with astrocytic SND1. J. Neuroinflammation 20:39. doi: 10.1186/s12974-023-02716-x
Huang, A., Zheng, H., Wu, Z., Chen, M., and Huang, Y. (2020). Circular RNA-protein interactions: functions, mechanisms, and identification. Theranostics 10, 3503–3517. doi: 10.7150/thno.42174
Jiang, Y. J., Cao, S. Q., Gao, L. B., Wang, Y. Y., Zhou, B., Hu, X., et al. (2019). Circular ribonucleic acid expression profile in mouse cortex after traumatic brain injury. J. Neurotrauma 36, 1018–1028. doi: 10.1089/neu.2018.5647
Kristensen, L. S., Jakobsen, T., Hager, H., and Kjems, J. (2022). The emerging roles of circRNAs in cancer and oncology. Nat. Rev. Clin. Oncol. 19, 188–206. doi: 10.1038/s41571-021-00585-y
Li, S., Hu, W., Deng, F., Chen, S., Zhu, P., Wang, M., et al. (2021). Identification of circular RNA hsa_circ_0001599 as a novel biomarker for large-artery atherosclerotic stroke. DNA Cell Biol. 40, 457–468. doi: 10.1089/dna.2020.5662
Li, X., Kang, J., Lv, H., Liu, R., Chen, J., Zhang, Y., et al. (2021). CircPrkcsh, a circular RNA, contributes to the polarization of microglia towards the M1 phenotype induced by spinal cord injury and acts via the JNK/p38 MAPK pathway. FASEB J. 35:e22014. doi: 10.1096/fj.202100993R
Li, X., Lou, X., Xu, S., Du, J., and Wu, J. (2020). Hypoxia inducible factor-1 (Hif-1α) reduced inflammation in spinal cord injury via miR-380-3p/ NLRP3 by Circ 0001723. Biol. Res. 53:35. doi: 10.1186/s40659-020-00302-6
Li, H., Lu, C., Yao, W., Xu, L., Zhou, J., and Zheng, B. (2020). Dexmedetomidine inhibits inflammatory response and autophagy through the circLrp1b/miR-27a-3p/Dram2 pathway in a rat model of traumatic brain injury. Aging (Albany NY) 12, 21687–21705. doi: 10.18632/aging.103975
Li, L., Luo, Y., Zhang, Y., Wei, M., Zhang, M., Liu, H., et al. (2020). Circznf609 aggravates neuropathic pain via miR-22-3p/ENO1 axis in CCI rat models. Gene 763:145069. doi: 10.1016/j.gene.2020.145069
Liu, Y., Ao, S., Zhang, H., Zhang, Y., Wang, Y., Yang, X., et al. (2021). Circ_HIPK3 alleviates CoCl(2)-induced apoptotic injury in neuronal cells by depending on the regulation of the miR-222-3p/DUSP19 axis. Biochem. Biophys. Res. Commun. 553, 126–133. doi: 10.1016/j.bbrc.2021.03.070
Liu, C. X., and Chen, L. L. (2022). Circular RNAs: characterization, cellular roles, and applications. Cell 185, 2016–2034. doi: 10.1016/j.cell.2022.04.021
Liu, M., Li, P., Jia, Y., Cui, Q., Zhang, K., and Jiang, J. (2022). Role of non-coding RNAs in axon regeneration after peripheral nerve injury. Int. J. Biol. Sci. 18, 3435–3446. doi: 10.7150/ijbs.70290
Liu, W. Z., Ma, Z. J., Li, J. R., and Kang, X. W. (2021). Mesenchymal stem cell-derived exosomes: therapeutic opportunities and challenges for spinal cord injury. Stem Cell Res Ther 12:102. doi: 10.1186/s13287-021-02153-8
Loan Young, T., Chang Wang, K., James Varley, A., and Li, B. (2023). Clinical delivery of circular RNA: lessons learned from RNA drug development. Adv. Drug Deliv. Rev. 197:114826. doi: 10.1016/j.addr.2023.114826
Mao, S., Huang, T., Chen, Y., Shen, L., Zhou, S., Zhang, S., et al. (2019a). Circ-Spidr enhances axon regeneration after peripheral nerve injury. Cell Death Dis. 10:787. doi: 10.1038/s41419-019-2027-x
Mao, S., Zhang, S., Zhou, S., Huang, T., Feng, W., Gu, X., et al. (2019b). A Schwann cell-enriched circular RNA circ-Ankib1 regulates Schwann cell proliferation following peripheral nerve injury. FASEB J. 33, 12409–12424. doi: 10.1096/fj.201900965R
Misir, S., Wu, N., and Yang, B. B. (2022). Specific expression and functions of circular RNAs. Cell Death Differ. 29, 481–491. doi: 10.1038/s41418-022-00948-7
Niu, D., Wu, Y., and Lian, J. (2023). Circular RNA vaccine in disease prevention and treatment. Signal Transduct. Target. Ther. 8:341. doi: 10.1038/s41392-023-01561-x
Piastra, M., Caresta, E., Massimi, L., Picconi, E., Luca, E., Morena, T. C., et al. (2020). Lipid peroxidation and antioxidant consumption as early markers of neurosurgery-related brain injury in children. Neurocrit. Care. 33, 124–131. doi: 10.1007/s12028-019-00870-w
Qi, J., Wang, T., Zhang, Z., Yin, Z., Liu, Y., Ma, L., et al. (2022). Circ-Ctnnb1 regulates neuronal injury in spinal cord injury through the Wnt/β-catenin signaling pathway. Dev. Neurosci. 44, 131–141. doi: 10.1159/000521172
Qian, X., Lin, G., Wang, J., Zhang, S., Ma, J., Yu, B., et al. (2022). CircRNA_01477 influences axonal growth via regulating miR-3075/FosB/Stat3 axis. Exp. Neurol. 347:113905. doi: 10.1016/j.expneurol.2021.113905
Qin, C., Liu, Y., Xu, P. P., Zhang, X., Talifu, Z., Liu, J. Y., et al. (2022). Inhibition by rno-circRNA-013017 of the apoptosis of motor neurons in anterior horn and descending axonal degeneration in rats after traumatic spinal cord injury. Front. Neurosci. 16:1065897. doi: 10.3389/fnins.2022.1065897
Qin, S., Zou, Y. H., and Zhang, C. L. (2013). Cross-talk between KLF4 and STAT3 regulates axon regeneration. Nat. Commun. 4:2633. doi: 10.1038/ncomms3633
Qing, L. M., Chen, H. W., Tang, J. Y., and Jia, X. F. (2018). Exosomes and their MicroRNA cargo: new players in peripheral nerve regeneration. Neurorehabil. Neural Repair 32, 765–776. doi: 10.1177/1545968318798955
Qu, L., Yi, Z., Shen, Y., Lin, L., Chen, F., Xu, Y., et al. (2022). Circular RNA vaccines against Sars-CoV-2 and emerging variants. Cell 185, 1728–1744.e16. doi: 10.1016/j.cell.2022.03.044
Ren, H., Chen, X., Tian, M., Zhou, J., Ouyang, H., and Zhang, Z. (2018). Regulation of inflammatory cytokines for spinal cord injury repair through local delivery of therapeutic agents. Adv. Sci. 5:1800529. doi: 10.1002/advs.201800529
Santos-Rodriguez, G., Voineagu, I., and Weatheritt, R. J. (2021). Evolutionary dynamics of circular RNAs in primates. eLife 10:e69148. doi: 10.7554/eLife.69148
Shechter, R., Miller, O., Yovel, G., Rosenzweig, N., London, A., Ruckh, J., et al. (2013). Recruitment of beneficial M2 macrophages to injured spinal cord is orchestrated by remote brain choroid plexus. Immunity 38, 555–569. doi: 10.1016/j.immuni.2013.02.012
Shi, Z., Yuan, S., Shi, L., Li, J., Ning, G., Kong, X., et al. (2021). Programmed cell death in spinal cord injury pathogenesis and therapy. Cell Prolif. 54:e12992. doi: 10.1111/cpr.12992
Siddiq, M. M., Toro, C. A., Johnson, N. P., Hansen, J., Xiong, Y., Mellado, W., et al. (2023). Spinal cord injury regulates circular RNA expression in axons. Front. Mol. Neurosci. 16:1183315. doi: 10.3389/fnmol.2023.1183315
Sohn, E. J., and Park, H. T. (2020). Differential expression of circular RNAs in the proximal and distal segments of the sciatic nerve after injury. Neuroreport 31, 76–84. doi: 10.1097/WNR.0000000000001371
Sun, J., Liao, Z., Li, Z., Li, H., Wu, Z., Chen, C., et al. (2023). Down-regulation miR-146a-5p in Schwann cell-derived exosomes induced macrophage M1 polarization by impairing the inhibition on TRAF6/Nf-kappaB pathway after peripheral nerve injury. Exp. Neurol. 362:114295. doi: 10.1016/j.expneurol.2022.114295
Sun, Y., Zhou, Y., Shi, X., Ma, X., Peng, X., Xie, Y., et al. (2021). Circtyw1 serves as a sponge for microRNA-380 in accelerating neurological recovery following spinal cord injury via regulating FGF9. Cell Cycle 20, 1828–1844. doi: 10.1080/15384101.2021.1962634
Tian, F., Yang, J., and Xia, R. (2022). Exosomes secreted from circZFHX3-modified mesenchymal stem cells repaired spinal cord injury through mir-16-5p/IGF-1 in mice. Neurochem. Res. 47, 2076–2089. doi: 10.1007/s11064-022-03607-y
Tong, D., Zhao, Y., Tang, Y., Ma, J., Wang, Z., and Li, C. (2021). Circ-Usp10 promotes microglial activation and induces neuronal death by targeting miRNA-152-5p/CD84. Bioengineered 12, 10812–10822. doi: 10.1080/21655979.2021.2004362
Van Broeckhoven, J., Sommer, D., Dooley, D., Hendrix, S., and Franssen, A. (2021). Macrophage phagocytosis after spinal cord injury: when friends become foes. Brain 144, 2933–2945. doi: 10.1093/brain/awab250
Wang, W., He, D., Chen, J., Zhang, Z., Wang, S., Jiang, Y., et al. (2021). Circular RNA Plek promotes fibrogenic activation by regulating the miR-135b-5p/TGF-βR1 axis after spinal cord injury. Aging (Albany NY) 13, 13211–13224. doi: 10.18632/aging.203002
Wang, X., Li, W., Hao, M., Yang, Y., and Xu, Y. (2023). Hypoxia-treated umbilical mesenchymal stem cell alleviates spinal cord ischemia-reperfusion injury in SCI by circular RNA circOXNAD1/ miR-29a-3p/ FOXO3a axis. Biochem. Biophys. Rep. 34:101458. doi: 10.1016/j.bbrep.2023.101458
Wang, W. Z., Li, J., Liu, L., Zhang, Z. D., Li, M. X., Li, Q., et al. (2021). Role of circular RNA expression in the pathological progression after spinal cord injury. Neural Regen. Res. 16, 2048–2055. doi: 10.4103/1673-5374.308100
Wang, K., Su, X., Song, Q., Chen, Z., Chen, H., Han, Y., et al. (2023). The circ_006573/miR-376b-3p Axis advances spinal cord functional recovery after injury by modulating vascular regeneration. Mol. Neurobiol. 60, 4983–4999. doi: 10.1007/s12035-023-03357-0
Wu, C., Du, M., Yu, R., Cheng, Y., Wu, B., Fu, J., et al. (2022). A novel mechanism linking ferroptosis and endoplasmic reticulum stress via the circPtpn14/miR-351-5p/5-LOX signaling in melatonin-mediated treatment of traumatic brain injury. Free Radic. Biol. Med. 178, 271–294. doi: 10.1016/j.freeradbiomed.2021.12.007
Wu, R., Mao, S., Wang, Y., Zhou, S., Liu, Y., Liu, M., et al. (2019). Differential circular RNA expression profiles following spinal cord injury in rats: a temporal and experimental analysis. Front. Neurosci. 13:1303. doi: 10.3389/fnins.2019.01303
Wu, H., Zheng, J., Xu, S., Fang, Y., Wu, Y., Zeng, J., et al. (2021). Mer regulates microglial/macrophage M1/M2 polarization and alleviates neuroinflammation following traumatic brain injury. J. Neuroinflammation 18:2. doi: 10.1186/s12974-020-02041-7
Xie, X., Xiao, Y., and Xu, K. (2021). Mechanism underlying circularRNA_014301-mediated regulation of neuronal cell inflammation and apoptosis. Exp. Ther. Med. 22:1432. doi: 10.3892/etm.2021.10867
Xie, L., Zhang, M., Liu, Q., Wei, R., Sun, M., Zhang, Q., et al. (2023). Downregulation of ciRNA-Kat6b in dorsal spinal horn is required for neuropathic pain by regulating Kcnk1 in miRNA-26a-dependent manner. CNS Neurosci. Ther. 29, 2955–2971. doi: 10.1111/cns.14235
Xin, Y., Song, X., and Ge, Q. (2021). Circular RNA Smek1 promotes neuropathic pain in rats through targeting microRNA-216a-5p to mediate Thioredoxin interacting protein (TXNIP) expression. Bioengineered 12, 5540–5551. doi: 10.1080/21655979.2021.1965811
Xu, T., Li, Z. Y., Liu, M., Zhang, S. B., Ding, H. H., Wu, J. Y., et al. (2023). CircFhit modulates GABAergic synaptic transmission via regulating the parental gene Fhit expression in the spinal dorsal Horn in a rat model of neuropathic pain. Neurosci. Bull. 39, 947–961. doi: 10.1007/s12264-022-01014-5
Xu, L., Ye, X., Zhong, J., Chen, Y. Y., and Wang, L. L. (2021). New insight of circular RNAs' roles in central nervous system post-traumatic injury. Front. Neurosci. 15:644239. doi: 10.3389/fnins.2021.644239
Yang, Q., Li, F., He, A. T., and Yang, B. B. (2021). Circular RNAs: expression, localization, and therapeutic potentials. Mol. Ther. 29, 1683–1702. doi: 10.1016/j.ymthe.2021.01.018
Yao, Y., Wang, J., He, T., Li, H., Hu, J., Zheng, M., et al. (2020). Microarray assay of circular RNAs reveals cicRNA.7079 as a new anti-apoptotic molecule in spinal cord injury in mice. Brain Res. Bull. 164, 157–171. doi: 10.1016/j.brainresbull.2020.08.004
Yin, X., Zheng, W., He, L., Mu, S., Shen, Y., and Wang, J. (2022). CircHIPK3 alleviates inflammatory response and neuronal apoptosis via regulating miR-382-5p/DUSP1 axis in spinal cord injury. Transpl. Immunol. 73:101612. doi: 10.1016/j.trim.2022.101612
Zhang, Y., Gao, T., Li, X., Wen, C. C., Yan, X. T., Peng, C., et al. (2021). Circ_0005075 targeting miR-151a-3p promotes neuropathic pain in CCI rats via inducing NOTCH2 expression. Gene 767:145079. doi: 10.1016/j.gene.2020.145079
Zhang, L., Li, Z., Mao, L., and Wang, H. (2022). Circular RNA in acute central nervous system injuries: a new target for therapeutic intervention. Front. Mol. Neurosci. 15:816182. doi: 10.3389/fnmol.2022.816182
Zhang, S. B., Lin, S. Y., Liu, M., Liu, C. C., Ding, H. H., Sun, Y., et al. (2019). CircAnks1a in the spinal cord regulates hypersensitivity in a rodent model of neuropathic pain. Nat. Commun. 10:4119. doi: 10.1038/s41467-019-12049-0
Zhang, B. Y., Saijilafu, L., Liu, C.-M., Wang, R.-Y., Zhu, Q., Jiao, Z., et al. (2014). Akt-independent GSK3 inactivation downstream of PI3K signaling regulates mammalian axon regeneration. Biochem. Biophys. Res. Commun. 443, 743–748. doi: 10.1016/j.bbrc.2013.12.037
Zhao, Y., Chen, Y., Wang, Z., Xu, C., Qiao, S., Liu, T., et al. (2022). Bone marrow mesenchymal stem cell exosome attenuates Inflammasome-related Pyroptosis via delivering circ_003564 to improve the recovery of spinal cord injury. Mol. Neurobiol. 59, 6771–6789. doi: 10.1007/s12035-022-03006-y
Zhao, J., Qi, X., Bai, J., Gao, X., and Cheng, L. (2020). A circRNA derived from linear Hipk3 relieves the neuronal cell apoptosis in spinal cord injury via ceRNA pattern. Biochem. Biophys. Res. Commun. 528, 359–367. doi: 10.1016/j.bbrc.2020.02.108
Zhao, X., Zhong, Y., Wang, X., Shen, J., and An, W. (2022). Advances in circular RNA and its applications. Int. J. Med. Sci. 19, 975–985. doi: 10.7150/ijms.71840
Zheng, P., Ren, D., Yu, C., Zhang, X., and Zhang, Y. (2022a). DNA methylation-related circRNA_0116449 is involved in lipid peroxidation in traumatic brain injury. Front. Mol. Neurosci. 15:904913. doi: 10.3389/fnmol.2022.904913
Zheng, P., Shu, L., Ren, D., Kuang, Z., Zhang, Y., and Wan, J. (2022b). circHtra1/miR-3960/GRB10 Axis promotes neuronal loss and immune deficiency in traumatic brain injury. Oxidative Med. Cell. Longev. 2022:3522492. doi: 10.1155/2022/3522492
Zhou, Z. B., Niu, Y. L., Huang, G. X., Lu, J. J., Chen, A., and Zhu, L. (2018). Silencing of circRNA.2837 plays a protective role in sciatic nerve injury by sponging the miR-34 family via regulating neuronal autophagy. Mol. Ther. Nucleic Acids 12, 718–729. doi: 10.1016/j.omtn.2018.07.011
Zhou, X., Wahane, S., Friedl, M. S., Kluge, M., Friedel, C. C., Avrampou, K., et al. (2020). Microglia and macrophages promote corralling, wound compaction and recovery after spinal cord injury via Plexin-B2. Nat. Neurosci. 23, 337–350. doi: 10.1038/s41593-020-0597-7
Zu, C., Li, J., He, X., Ji, L., and Li, X. (2022). Identification of a circRNA-mediated comprehensive ceRNA network in spinal cord injury pathogenesis. Exp. Biol. Med. (Maywood) 247, 931–944. doi: 10.1177/15353702221082929
Keywords: circular RNA, nerve regeneration, peripheral nerve injury, traumatic brain injury, spinal cord injury, neuropathic pain
Citation: Zong Y, Dai Y, Yan J, Yu B, Wang D and Mao S (2024) The roles of circular RNAs in nerve injury and repair. Front. Mol. Neurosci. 17:1419520. doi: 10.3389/fnmol.2024.1419520
Edited by:
Wenzhao Wang, Shandong University, ChinaReviewed by:
Zhengdong Zhang, First Affiliated Hospital of Chengdu Medical College, ChinaMingjie Sun, Chongqing Medical University, China
Qi Wang, Swiss Federal Institute of Technology Lausanne, Switzerland
Copyright © 2024 Zong, Dai, Yan, Yu, Wang and Mao. This is an open-access article distributed under the terms of the Creative Commons Attribution License (CC BY). The use, distribution or reproduction in other forums is permitted, provided the original author(s) and the copyright owner(s) are credited and that the original publication in this journal is cited, in accordance with accepted academic practice. No use, distribution or reproduction is permitted which does not comply with these terms.
*Correspondence: Dong Wang, wangdong2018@ntu.edu.cn; Susu Mao, maosusu@ntu.edu.cn
†These authors have contributed equally to this work