- 1The First Clinical Medical School, Yunnan University of Traditional Chinese Medicine, Kunming, Yunnan, China
- 2College of Acupuncture and Massage, Yunnan University of Traditional Chinese Medicine, Kunming, Yunnan, China
- 3Yunnan Provincial Hospital of Traditional Chinese Medicine, Kunming, Yunnan, China
Annually, more than 15 million people worldwide suffer from stroke, a condition linked to high mortality and disability rates. This disease significantly affects daily life, impairing everyday functioning, executive function, and cognition. Moreover, stroke severely restricts patients’ ability to perform daily activities, diminishing their overall quality of life. Recent scientific studies have identified cuproptosis, a newly discovered form of cell death, as a key factor in stroke development. However, the role of cuproptosis in stroke remains unclear to researchers. Therefore, it is crucial to investigate the mechanisms of cuproptosis in stroke’s pathogenesis. This review examines the physiological role of copper, the characteristics and mechanisms of cuproptosis, the differences and similarities between cuproptosis and other cell death types, and the pathophysiology of cuproptosis in stroke, focusing on mitochondrial dysfunction and immune infiltration. Further research is necessary to understand the relationship between previous strokes and cuproptosis and to clarify the mechanisms behind these associations.
1 Introduction
Annually, stroke affects approximately 15 million people globally, leading to high mortality and disability rates. Recent statistics rank stroke as the second leading cause of death worldwide (Campbell et al., 2020; Krishnamurthi et al., 2020). Stroke patients often suffer structural brain damage due to a combination of ischemia and bleeding (Zhang et al., 2021). Even a mild stroke can significantly impact various aspects of an individual’s daily functioning, including executive function and cognition (Fride et al., 2015). Various pathways have been identified in stroke-induced damage, such as mitochondrial dysfunction, ferroptosis, and apoptosis. Recently, a novel process called cuproptosis has been proposed as a significant contributor to stroke development. However, the specific factors involved in cuproptosis-related stroke are not yet fully understood. This review delves into the physiological role of copper, the characteristics and mechanisms of cuproptosis, the distinctions and parallels between cuproptosis and other cell death forms, and the pathophysiology of cuproptosis in stroke.
2 Fundamental aspects of cuproptosis
2.1 Physiological function of copper
Copper exists in two ionic states within living organisms: the reduced copper ion (Cu+) and the oxidized copper ion (Cu2+), due to its REDOX activity (Haitao et al., 2023). These ions can bind with various proteins or enzymes, serving as cofactors or structural components. They participate in regulating physiological processes including energy metabolism, mitochondrial respiration, and antioxidation (Rui et al., 2023). Additionally, copper plays a role in key biological processes, including angiogenesis, hypoxia response, and neuromodulation (Tianwen et al., 2023). It is involved in the generation of reactive oxygen species (ROS) and the oxidative modification of low-density lipoprotein cholesterol (Huiqin et al., 2023). Furthermore, copper impacts the nervous system by contributing to myelination, synaptic activity regulation, signaling cascades, and neuronal death modulation (Rui et al., 2023).
2.2 Role of trace metal elements in the central nervous system
Alterations in the levels of essential metal elements, such as iron, zinc, and copper, signal the onset of various neurological diseases (Ayton et al., 2013). Iron and its associated proteins, vital for brain development, are abundantly present in the brain. They regulate the synthesis and release of nerve myelin and dopamine neurotransmitters. Iron deficiency in the brain impairs ganglia function, inhibits nerve impulse conduction, and affects brain development and function, leading to decreased attention, memory, and motor coordination (Acevedo et al., 2019). Zinc, as the active center of many enzymes, participates in hormone secretion and bone calcification regulation. It is involved in the synthesis of enzymes and neurotransmitters in brain tissue and is associated with various neurodegenerative diseases. Both excessive and insufficient zinc levels can increase disease risk (Li et al., 2022). Copper, crucial for heme synthesis, immune function, and nerve conduction, is an essential transition metal in the human body. It is involved in oxygen metabolism, collagen synthesis, iron homeostasis, and antioxidant defense. In the nervous system, copper contributes to myelin formation, synaptic activity regulation, neuron death, and signal cascade reaction. Maintaining copper metabolism homeostasis is vital (Rui et al., 2023). Additionally, cerium plays a significant role in the nervous system. It can stimulate human growth, balance and regulate metabolism, and influence growth, reproductive function, brain development, and aging. The cerium content in the adult brain decreases with age (Roberts et al., 2016).
2.3 Characteristics of cuproptosis
Cuproptosis is a newly discovered form of cell death that occurs when there is an imbalance in the regulation of copper ions within cells. These ions can directly interact with fatty acylated components in the tricarboxylic acid cycle (TCA), leading to the aggregation and imbalance of certain proteins, which ultimately disrupts the TCA cycle. This disruption results in toxic stress on proteins and ultimately causes cell death (Tianwen et al., 2023). The process begins with a reduction in the lipoylation of DLAT and DLST, as well as the formation of oligomers of lipoylated proteins involved in the TCA cycle, due to the direct binding of copper. Additionally, there is a destabilization and overall decrease in iron–sulfur (Fe-S) cluster proteins. These effects combine to induce proteotoxic stress and impair mitochondrial function (Huo et al., 2023). Another critical process is the necessity of fatty acylation for binding copper ions. The binding of copper ions to fatty acylated DLAT and the subsequent induction of oligomerization are noted. Moreover, the harmful synthesis of fatty acylated proteins upon exposure to a copper ionophore is partly mediated by aberrant oligomerization. Additionally, the depletion of the endogenous intracellular copper chaperone glutathione leads to copper-dependent cell death (Huiqin et al., 2023). This phenomenon is associated with a reduction in fatty acylation due to the weakening of FDX1 and low-intensity aerobic exercise (LIAS), as well as an increase in DLAT oligomerization (Jia et al., 2023).
2.4 Mechanism of cuproptosis
Cuproptosis disrupts mitochondrial respiration and protein lipidation, leading to membrane permeability, cellular damage, and the initiation of apoptotic pathways under various conditions (Chen et al., 2022). Firstly, copper induces harm through oxidative stress and facilitates cell death, with oxidative stress being a primary inducer of apoptosis by impairing fundamental biomolecules such as proteins, lipids, and DNA. Additionally, the presence of copper may amplify the effects of oxidative stress (Acilan et al., 2016). Secondly, protein acylation triggered by copper ultimately leads to cell death. High concentrations of copper ions can interfere with the normal configuration and operational mechanisms of proteins due to their affinity for protein binding sites. Copper targeting and binding to fatty acylated constituents of the TCA cycle result in the aggregation of these Cu-bound fatty acylated proteins within the mitochondria, leading to a reduction of iron–sulfur (Fe-S) clusters and triggering proteotoxic stress, ultimately causing cell death. Thirdly, the breakdown of copper homeostasis mediates cell death (Cobine and Brady, 2022). It is crucial to maintain an adequate concentration of copper ions within the cell for normal physiological metabolism and to implement precautionary measures to prevent excessive uptake of copper ions, whose toxicity can lead to cell death. Lastly, cell death triggered by copper ionophores occurs following an accumulation phase of intracellular copper ions, facilitating the anomalous oligomerization of lipoacylated proteins in the TCA cycle and leading to a decrease in iron–sulfur cluster levels. Copper ionophores can transport copper ions into cells, thereby elevating the intracellular Cu2+ concentration, promoting the generation of reactive oxygen species (ROS), impeding the activity of the proteasome, and ultimately triggering cell death (Figure 1).
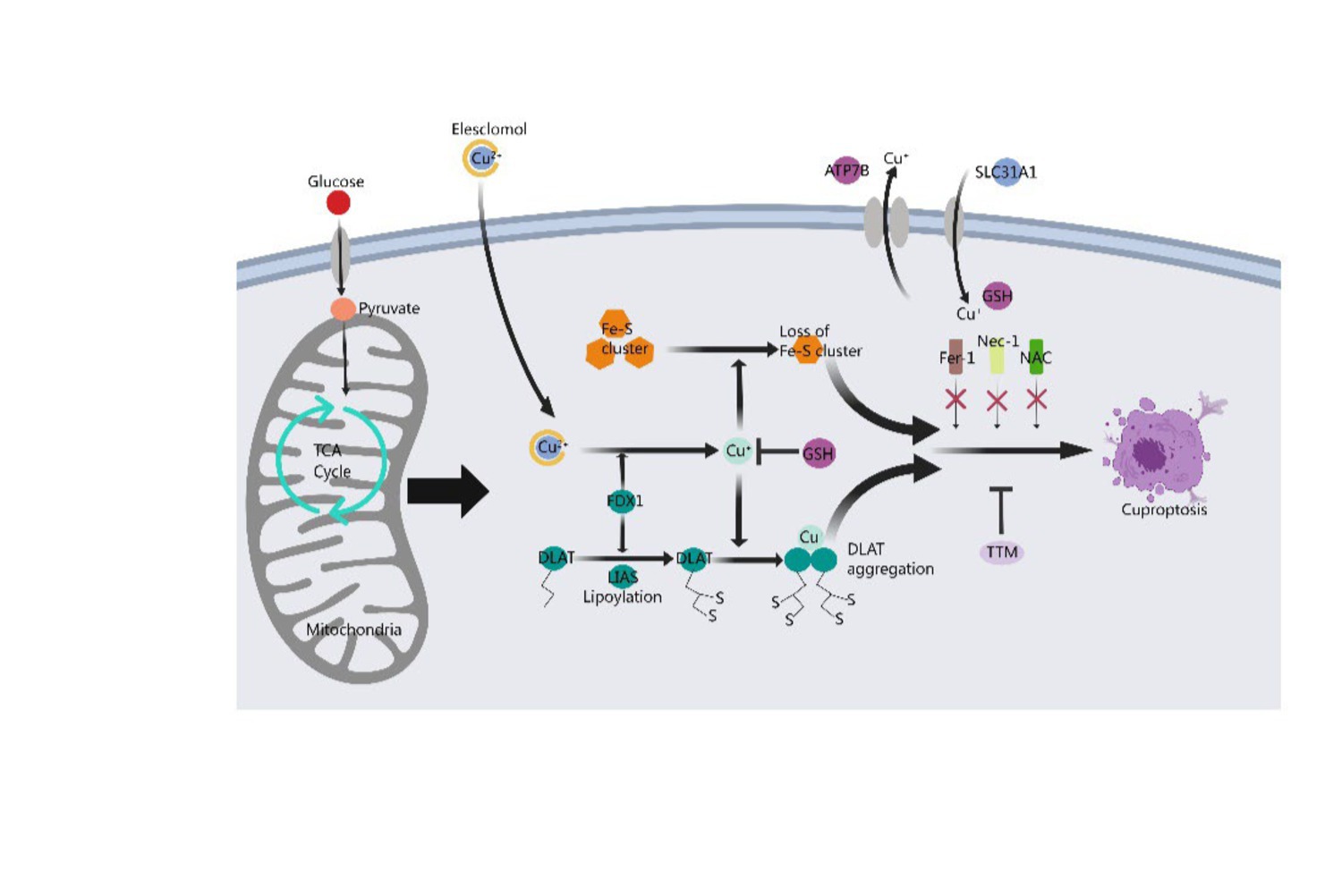
Figure 1. Mechanism of cuproptosis. A novel cell death process called cuproptosis provides strong evidence for the concept that copper-induced cell death occurs through a well-established mechanism involving protein lipoacylation. Cuproptosis begins with copper directly interacting with the fatty acylated parts of the tricarboxylic acid (TCA) cycle. This interaction causes the accumulation of fatty acylated proteins and eventually disrupts iron–sulfur cluster proteins, leading to proteotoxic stress and resulting in cell death. Copper chelator TTM inhibited cuproptosis, while ferroptosis inhibitor (Fer-1), necrotizing apoptosis inhibitor (Nec-1) and oxidative stress inhibitor (NAC) had no effect on cuproptosis. FDX1 acts as a key regulator of protein lipoacylation, which is essential for controlling cuproptosis. Both FDX1 and protein lipoacylation are critical in managing this cell death process.
2.5 Similarities and differences between cuproptosis and other cell death modes
Ferroptosis is a form of cell death dependent on iron, where an excessive iron accumulation leads to the buildup of lipid peroxides in cellular membranes, resulting in programmed cell death (Jiang et al., 2021). When comparing ferroptosis and cuproptosis, it is clear that mitochondria play a critical role in both types of cell death. Dihydrolipamide S-acetyltransferase (DLAT) (Liu et al., 2024) is central to this process. Copper directly binds to DLAT, promoting the formation of disulfide bonds in fatty acylated DLAT, which triggers cell death. This process can be mitigated by mitochondrial glutathione (GSH), which inhibits lipid acylation of the enzyme and promotes DLAT oligomerization. In ferroptosis, using mitoquinone (MitoQ), a mitochondria-targeting compound, has been shown to increase GSH levels, maintaining mitochondrial integrity and protecting cells from lipid peroxide accumulation, thus preventing cell death. Contrarily, ferroptosis involves mitochondrial morphological changes, such as shrinkage, increased membrane density, and mitochondrial fragmentation (Li J. et al., 2020; Li X. et al., 2020), not observed in cuproptosis. Further research is needed to identify the specific morphological features of cuproptosis and its potential link to iron homeostasis disruption and ferroptosis induction.
Apoptosis is primarily triggered by physiological or minor pathogenic stimuli and is an active cellular demise mechanism (Elmore, 2007), occurring without any inflammatory response. It is characterized by distinct cellular and tissue alterations, different from necrosis.
Necrosis is caused by pathogenic stimuli or severe injury, leading to the release of cellular contents outside the cell and subsequent inflammation (Nikoletopoulou et al., 2013). It features cell swelling, membrane disruption, content leakage, nuclear changes, partial DNA destruction, and significant local inflammatory responses. In the context of pyroptosis (Fang et al., 2020), activated cysteine aspartate proteases cleave GSDMD/GSDME into fragments that form pores in the cellular membrane, releasing inflammatory cytokines and other cell components. Apoptosis, pyroptosis, and necrosis are distinct cell death mechanisms that together create a cohesive system, where pathways can compensate for each other. These pathways might be activated sequentially in a single cell, depending on time and environmental factors. It is acknowledged that cell death is not caused by a single mechanism. Instead, there is a reciprocal interaction between pathways, where modulation of one can significantly affect the others (Ketelut-Carneiro and Fitzgerald, 2022).
Autophagy is a cellular breakdown mechanism conserved across evolution and tightly regulated in eukaryotic cells. It activates in response to metabolic stimuli, either internal or external, such as nutrient deprivation or low oxygen levels. This mechanism involves the sequestration and delivery of cellular components including aggregates, excessive or damaged organelles, and pathogens, to specialized compartments called lysosomes. Within the lysosomes, these components undergo hydrolysis, breaking down into smaller molecules, such as nucleotides, which are then recycled back into the cytoplasm for utilization by the cell (Li J. et al., 2020; Li X. et al., 2020).
3 Pathogenesis of cuproptosis in stroke
3.1 Cuproptosis is associated with brain damage
Cuproptosis, a relatively new concept in cellular death, has recently been explored in several scholarly studies, particularly for its link to cerebral impairment. Patel’s research provides experimental evidence of a correlation between brain copper imbalance, neurotoxicity, and the development of Alzheimer’s disease (Patel and Aschner, 2021). However, other studies suggest that copper presence in the brain might protect against cognitive decline in individuals with Alzheimer’s disease (Agarwal et al., 2022). Research has also indicated that dietary copper restriction, the use of copper chelators, and genetic manipulation of copper transporters could slow Huntington’s disease progression in animal models (Hands et al., 2010). In amyotrophic lateral sclerosis (ALS) (Gil-Bea et al., 2017), difficulties arise in the interaction between the copper chaperone of superoxide dismutase (CCS) and mutant copper-zinc superoxide dismutase (SOD1). This impairment in interaction leads to reduced copper delivery to mitochondria, accumulation of unstable SOD1, and increased susceptibility to pro-oxidants. These molecular events result in toxic gain-of-function effects, particularly in motor neurons. The study also indicates that SOD1 mitigates neuronal damage following cerebral ischemia by decreasing the release of mitochondrial cytochrome C and inhibiting caspase activation. Gliomas, originating from abnormal glial cells or their precursors, represent a common and deadly type of primary intracranial malignancy (Xu et al., 2020). Cell death within tumors often occurs due to metabolic stressors like hypoxia or glucose deprivation (Qin et al., 2019). Consequently, cuproptosis could be a uniquenovel therapeutic approach for cancer treatment (Wang T. et al., 2022; Wang W. et al., 2022). Studies suggest that prolonged occupational exposure to copper increases the risk of developing Parkinson’s disease, with excess copper inducing neuronal cell death and α-synuclein aggregation (Montes et al., 2014). Parkinson’s disease (PD) progression may also be linked to mitochondrial malfunction and oxidative stress (Vallée et al., 2020).
3.2 Stroke and cuproptosis related mitochondrial dysfunction
Fang Huiqin hypothesized that mitochondria are vital for cellular homeostasis, with disruptions often resulting from reduced mitochondrial DNA copy number and oxygen consumption (Huiqin et al., 2023). Therefore, treating AS involves regulating copper ion homeostasis and improving mitochondrial dysfunction to affect vascular endothelial function and macrophage polarization, reducing stroke risk. Previous research supports the link between mitochondrial dysfunction and decreased adenosine triphosphate (ATP) synthesis in ischemic strokes, leading to cerebral cell death due to ATP deficiency. However, given cuproptosis depends on mitochondrial respiration and the tricarboxylic acid cycle, it’s hypothesized to be a key mechanism in ischemia and hypoxic nerve cell and oligodendrocyte death. Still, conclusive evidence for this is currently lacking (Wang T. et al., 2022; Wang W. et al., 2022). Huo et al. (2023) and Jia et al. (2023) described cuproptosis as initiated by the nonspecific interaction between copper and fatty acylation proteins in mitochondria. This interaction reduces the expression of proteins in the mitochondrial oxidative respiratory chain, decreasing the activity of mitochondrial complexes I and III, and lowering ATP content. This leads to cellular energy deficits and cardiomyocyte death. Considering the high oxygen and ATP demands of brain tissue, it is worth exploring whether cuproptosis, known to cause cardiac cell death, could also play a role in stroke development. However, this theory needs further validation.
3.3 Stroke and cuproptosis-related immune infiltration
The importance of copper homeostasis in immune system infiltration has been shown in recent studies. TAN found that copper chelation in macrophages leads to the removal of lysyl oxidase-like 4-mediated programmed death ligand I presentation. This prevents cellular immune evasion. CHOI’s research indicated that clodoxoline, a common copper chelator, significantly reduces immune cell infiltration, including CD4 and CD8 cells, which are linked to encephalitis development (Huiqin et al., 2023). Xue suggested that the transcription factor Nrf2 and the NLRP3 inflammasome might contribute to cerebral ischemia–reperfusion injury (Fan et al., 2023). Li′s proposal was that LIAS, a protein containing Fe-S clusters, is associated with oxidative stress and inflammation (Rui et al., 2023). Overexpression of LIAS can reduce pro-inflammatory cytokines/chemokines and suppress NF-κB activity. Additionally, LIAS overexpression reduces oxidative stress and enhances antioxidant defense mechanisms, leading to an increase in the synthesis of NRF2 and LIAS. Therefore, this has the potential to safeguard mitochondrial function in diabetic nephropathy. LIAS could improve the health of stroke survivors by protecting brain mitochondria and reducing the immune response (Figure 2).
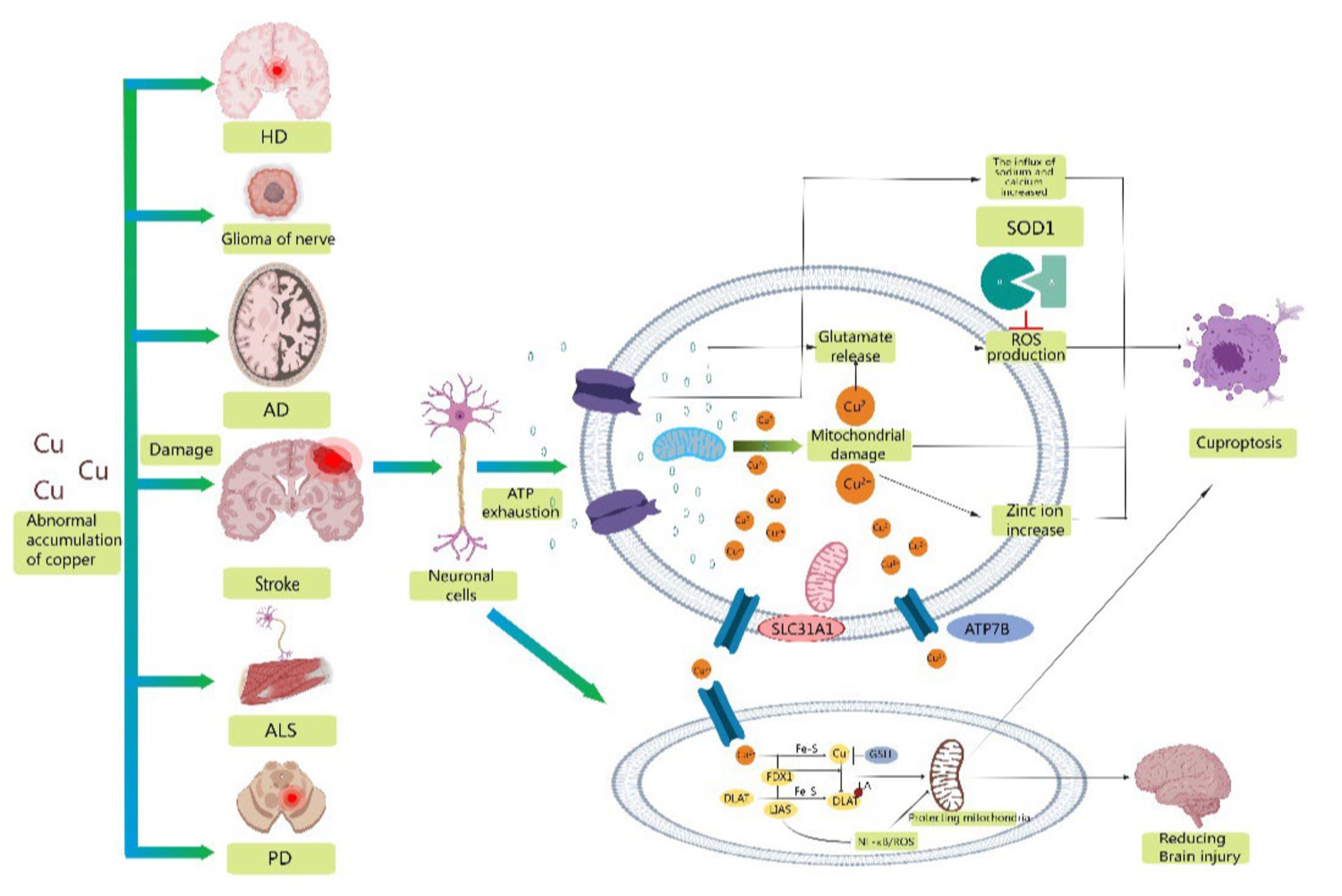
Figure 2. Schematic representation of possible mechanisms of cuproptosis in stroke. Abnormal accumulation of copper contributes to the development of a variety of neurodegenerative diseases, including stroke, Alzheimer's disease, amyotrophic lateral sclerosis, Parkinson's disease, glioma, and Huntington's disease. Mitochondrial oxidative stress injury is involved in the progression of stroke, providing conditions for the occurrence of cuproptosis. Therefore, reducing mitochondrial ROS production, excluding damaged mitochondria and refusing damaged mitochondria to reenter neuronal cells may be important measures to reduce neuronal oxidative stress injury and cuproptosis. Secondly, overexpression of LIAS reduced proinflammatory cytokines/chemokines and inhibited NF-κB activity. In addition, overexpression of LIAS can reduce oxidative stress and enhance antioxidant defense mechanisms, which may have some protective effect on mitochondria and reduce damage after stroke.
4 Predictions of treatment on stroke by cuproptosis
Currently, no specific drug therapy has been identified for stroke treatment through cuproptosis. However, based on the pathology of cuproptosis in stroke, certain treatment predictions have been made, summarized in Table 1.
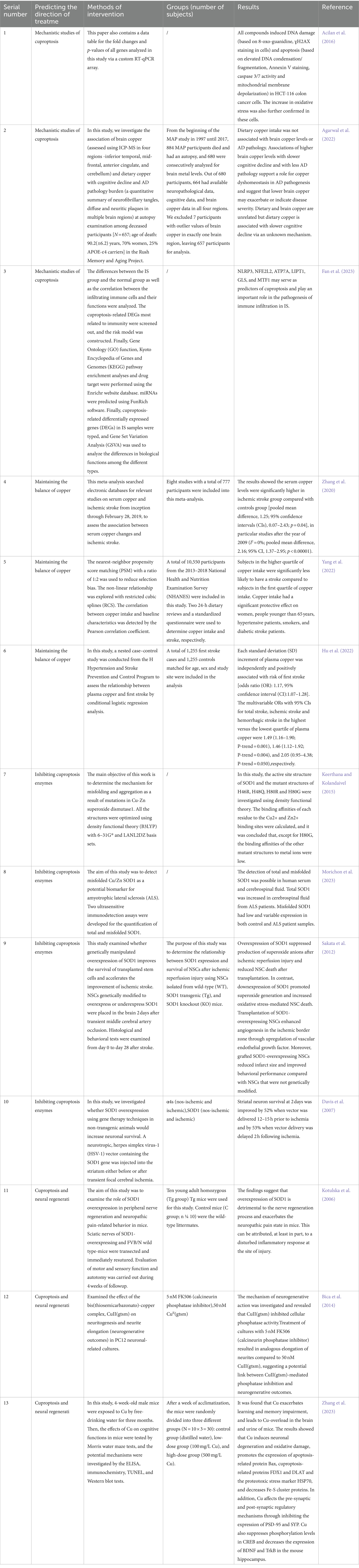
Table 1. Mechanistic studies of cuproptosis and randomized controlled trials on predictions of treatment on stroke by cuproptosis.
4.1 Maintenance of the balance of copper ions in the brain
Copper ions play a crucial role in the process of cuproptosis, and impeding their cellular uptake could serve as a potential preventive measure against cuproptosis. Moreover, a previous study has demonstrated that the entry of copper (Cu) ions into cells can lead to their accumulation and subsequent induction of cell death (Xiong et al., 2023). Another study indicated that serum copper levels were significantly elevated in individuals with ischemic stroke compared with the control group (Zhang et al., 2020). However, a separate case–control study showed an inverse relationship between daily dietary copper intake and stroke risk (Yang et al., 2022). Hu’s research on the plasma copper levels of 1,255 individuals who experienced their first stroke found a positive association that followed a linear trend between plasma copper levels and the risk of first ischemic stroke (Hu et al., 2022). While no definitive study has yet established the efficacy of maintaining copper ion balance in the brain as a treatment for stroke, multiple sources suggest that copper ions play a significant part in the stroke process.
4.2 Enzymes that inhibit cuproptosis
Cuproptosis involves several distinct enzymes that are promising therapeutic targets for stroke management (Robinson and Winge, 2010). The enzyme Cu/Zn-SOD1 is a dimeric cytosolic enzyme (Keerthana and Kolandaivel, 2015). A study investigated the potential of misfolded Cu/Zn-SOD1 as a biomarker for ALS by detecting total SOD1 and misfolded SOD1 in human serum and cerebrospinal fluid (Morichon et al., 2023). ALS, a neurodegenerative disorder, shares some pathophysiological similarities with those of stroke (Mohr, 2004). Sakata’s study concluded that the overexpression of SOD1 could decrease ROS levels, enhance neural stem cell survival, and protect the mouse brain during temporary focal cerebral ischemia episodes (Sakata et al., 2012). Alexis’s research showed that upregulating SOD1 using a herpes simplex virus vector could provide neuroprotection to striatal neurons during temporary focal cerebral ischemia (Davis et al., 2007) episodes. Thus, it is plausible to hypothesize that inhibiting these enzymes could help prevent or mitigate cuproptosis, potentially improving the prognosis for individuals affected by stroke.
4.3 Cuproptosis and nerve regeneration
A study suggests that copper ions may inhibit neuron growth and repair, adversely impacting nerve regeneration (Kotulska et al., 2006). Conversely, copper ions could have a beneficial effect on this process. Various studies indicate that copper ions aid axon growth and synapse formation, contributing to brain function restoration (Bica et al., 2014). The CREB/BDNF pathway plays a crucial role in nerve regeneration. Research indicates that (Zhang et al., 2023) copper can disrupt synaptic plasticity through the CREB/BDNF pathway, trigger cuproptosis, and promote cell death, suggesting a strong link between copper-induced cell death and nerve regeneration. Additionally, cuproptosis’s influence on nerve regeneration is complex, as it can either support or hinder repair. This effect is similar to neuroinflammation post-stroke, where neuroinflammation can either aid recovery or intensify brain damage (Stuckey et al., 2021). Thus, determining the optimal timing for intervening in neuroinflammation is vital. This insight leads to the hypothesis that modulating cuproptosis timing in nerve regeneration could allow control over the regenerative process.
5 Summary and outlook
Given the accumulation of copper in various neurodegenerative diseases, it’s plausible that cuproptosis plays a pathogenic role, potentially offering a new therapeutic target. Strategies such as using copper chelators, reducing dietary copper intake, and genetically modifying copper transporters can mitigate copper-induced cell death by lowering intracellular copper levels. However, further research is needed to fully understand how cuproptosis contributes to disease progression.
The lipoic acid pathway is key in mediating cuproptosis, but the exact mechanisms of this cell death form remain unclear, including the role of other metabolic pathways and how lipoylated mitochondrial enzyme aggregation triggers copper-dependent cell death. Further investigation is necessary to uncover these mechanisms and explore clinical applications. Recent studies highlight the significant role of cuproptosis in stroke progression and provide new opportunities for clinical applications.
Despite the ongoing uncertainty surrounding the exact mechanism of cuproptosis in relation to stroke, a growing body of experimental research has highlighted the significant role of cuproptosis in stroke progression (Fan et al., 2023). At present, the main approaches to stroke management include pharmacotherapy, interventional procedures, surgical interventions, and rehabilitation. However, these treatments have inherent limitations. The link between cuproptosis and stroke offers promising opportunities for improving stroke treatments, emphasizing the need for targeted research into the effects of cuproptosis on stroke.
Author contributions
LX: Writing – original draft, Writing – review & editing. ZW: Data curation, Methodology, Writing – original draft. ZH: Data curation, Writing – original draft. PP: Conceptualization, Investigation, Writing – review & editing. AY: Methodology, Writing – review & editing. JW: Conceptualization, Writing – review & editing.
Funding
The author(s) declare that financial support was received for the research, authorship, and/or publication of this article. This work was supported by the General Project of Yunnan Applied Basic Research Program (202201AT070214); Yunnan Provincial Science and Technology Department-Applied Basic Research Joint Special Funds of Chinese Medicine (202001AZ070001-020, 202301AZ070001-016); Two types of talent projects in Yunnan Province (202205 AD160024).
Conflict of interest
The authors declare that the research was conducted in the absence of any commercial or financial relationships that could be construed as a potential conflict of interest.
Publisher’s note
All claims expressed in this article are solely those of the authors and do not necessarily represent those of their affiliated organizations, or those of the publisher, the editors and the reviewers. Any product that may be evaluated in this article, or claim that may be made by its manufacturer, is not guaranteed or endorsed by the publisher.
References
Acevedo, K., Masaldan, S., Opazo, C. M., and Bush, A. I. (2019). Redox active metals in neurodegenerative diseases. J. Biol. Inorg. Chem. 24, 1141–1157. doi: 10.1007/s00775-019-01731-9
Acilan, C., Cevatemre, B., Adiguzel, Z., Karakas, D., Ulukaya, E., Ribeiro, N., et al. (2016). Validation data supporting the characterization of novel copper complexes as anticancer agents. Data Brief 9, 1160–1174. doi: 10.1016/j.dib.2016.11.063
Agarwal, P., Ayton, S., Agrawal, S., Dhana, K., Bennett, D. A., Barnes, L. L., et al. (2022). Brain copper may protect from cognitive decline and Alzheimer’s disease pathology: a community-based study. Mol. Psychiatry 27, 4307–4313. doi: 10.1038/s41380-022-01802-5
Ayton, S., Lei, P., and Bush, A. I. (2013). Metallostasis in Alzheimer’s disease. Free Radic. Biol. Med. 62, 76–89. doi: 10.1016/j.freeradbiomed.2012.10.558
Bica, L., Liddell, J. R., Donnelly, P. S., Duncan, C., Caragounis, A., Volitakis, I., et al. (2014). Neuroprotective copper bis(thiosemicarbazonato) complexes promote neurite elongation. PLoS One 9:e90070. doi: 10.1371/journal.pone.0090070
Campbell, P., Rutten, F. H., Lee, M. M., Hawkins, N. M., and Petrie, M. C. (2020). Stroke. Lancet 396, 129–142. doi: 10.1016/S0140-6736(20)31179-X
Chen, L., Min, J., and Wang, F. (2022). Copper homeostasis and cuproptosis in health and disease. Signal Transduct. Target. Ther. 7:378. doi: 10.1038/s41392-022-01229-y
Cobine, P. A., and Brady, D. C. (2022). Cuproptosis: cellular and molecular mechanisms underlying copper-induced cell death. Mol. Cell 82, 1786–1787. doi: 10.1016/j.molcel.2022.05.001
Davis, A. S., Zhao, H., Sun, G. H., Sapolsky, R. M., and Steinberg, G. K. (2007). Gene therapy using SOD1 protects striatal neurons from experimental stroke. Neurosci. Lett. 411, 32–36. doi: 10.1016/j.neulet.2006.08.089
Elmore, S. (2007). Apoptosis: a review of programmed cell death. Toxicol. Pathol. 35, 495–516. doi: 10.1080/01926230701320337
Fan, X., Chen, H., Jiang, F., Xu, C., Wang, Y., Wang, H., et al. (2023). Comprehensive analysis of cuproptosis-related genes in immune infiltration in ischemic stroke. Front. Neurol. 13:1077178. doi: 10.3389/fneur.2022.1077178
Fang, Y., Tian, S., Pan, Y., Li, W., Wang, Q., Tang, Y., et al. (2020). Pyroptosis: a new frontier in cancer. Biomed. Pharmacother. 121:109595. doi: 10.1016/j.biopha.2019.109595
Fride, Y., Adamit, T., Maeir, A., Ben Assayag, E., Bornstein, N. M., Korczyn, A. D., et al. (2015). What are the correlates of cognition and participation to return to work after first ever mild stroke? Top. Stroke Rehabil. 22, 317–325. doi: 10.1179/1074935714Z.0000000013
Gil-Bea, F. J., Aldanondo, G., Lasa-Fernández, H., López de Munain, A., and Vallejo-Illarramendi, A. (2017). Insights into the mechanisms of copper dyshomeostasis in amyotrophic lateral sclerosis. Expert Rev. Mol. Med. 19:e7. doi: 10.1017/erm.2017.9
Haitao, L., Haidong, W., Jinhai, W., Xiaojun, S., Weiqing, L., Fanghong, N., et al. (2023). Progress in regulation mechanism of copper death. Chin. J. Pathophysiol. 39, 1491–1498. doi: 10.3969/j.issn.1000-4718.2023.08.018
Hands, S. L., Mason, R., Sajjad, M. U., Giorgini, F., and Wyttenbach, A. (2010). Metallothioneins and copper metabolism are candidate therapeutic targets in Huntington’s disease. Biochem. Soc. Trans. 38, 552–558. doi: 10.1042/BST0380552
Hu, L., Bi, C., Lin, T., Liu, L., Song, Y., Wang, P., et al. (2022). Association between plasma copper levels and first stroke: a community-based nested case-control study. Nutr. Neurosci. 25, 1524–1533. doi: 10.1080/1028415X.2021.1875299
Huiqin, F., Xiaowei, M., Qiu, C., Shufei, C., Junji, Z., Zongqin, Z., et al. (2023). Study on differential genes and potential biomarkers of early and advanced atherosclerosis related to cuproptosis based on bioinformatics. Chin. J. Arterioscler. 31, 1–11. doi: 10.20039/j.cnki.1007-3949.2023.11.003
Huo, S., Wang, Q., Shi, W., Peng, L., Jiang, Y., Zhu, M., et al. (2023). ATF3/SPI1/SLC31A1 signaling promotes cuproptosis induced by advanced glycosylation end products in diabetic myocardial injury. Int. J. Mol. Sci. 24:1667. doi: 10.3390/ijms24021667
Jia, W., Tian, H., Jiang, J., Zhou, L., Li, L., Luo, M., et al. (2023). Brain-targeted HFn-cu-REGO nanoplatform for site-specific delivery and manipulation of autophagy and cuproptosis in glioblastoma. Small 19:e2205354. doi: 10.1002/smll.202205354
Jiang, X., Stockwell, B. R., and Conrad, M. (2021). Ferroptosis: mechanisms, biology and role in disease. Nat. Rev. Mol. Cell Biol. 22, 266–282. doi: 10.1038/s41580-020-00324-8
Keerthana, S. P., and Kolandaivel, P. (2015). Study of mutation and misfolding of cu-Zn SOD1 protein. J. Biomol. Struct. Dyn. 33, 167–183. doi: 10.1080/07391102.2013.865104
Ketelut-Carneiro, N., and Fitzgerald, K. A. (2022). Apoptosis, pyroptosis, and necroptosis-oh my! The many ways a cell can die. J. Mol. Biol. 434:167378. doi: 10.1016/j.jmb.2021.167378
Kotulska, K., LePecheur, M., Marcol, W., Lewin-Kowalik, J., Larysz-Brysz, M., Paly, E., et al. (2006). Overexpression of copper/zinc-superoxide dismutase in transgenic mice markedly impairs regeneration and increases development of neuropathic pain after sciatic nerve injury. J. Neurosci. Res. 84, 1091–1097. doi: 10.1002/jnr.21000
Krishnamurthi, R. V., Ikeda, T., and Feigin, V. L. (2020). Global, regional and country-specific burden of ischaemic stroke, intracerebral haemorrhage and subarachnoid haemorrhage: a systematic analysis of the global burden of disease study 2017. Neuroepidemiology 54, 171–179. doi: 10.1159/000506396
Li, J., Cao, F., Yin, H. L., Huang, Z. J., Lin, Z. T., Mao, N., et al. (2020). Ferroptosis: past, present and future. Cell Death Dis. 11:88. doi: 10.1038/s41419-020-2298-2
Li, X., He, S., and Ma, B. (2020). Autophagy and autophagy-related proteins in cancer. Mol. Cancer 19:12. doi: 10.1186/s12943-020-1138-4
Li, Z., Liu, Y., Wei, R., Yong, V. W., and Xue, M. (2022). The important role of zinc in neurological diseases. Biomol. Ther. 13:28. doi: 10.3390/biom13010028
Liu, W. Q., Lin, W. R., Yan, L., Xu, W. H., and Yang, J. (2024). Copper homeostasis and cuproptosis in cancer immunity and therapy. Immunol. Res. 321, 211–227. doi: 10.1111/imr.13276
Mohr, J. P. (2004). ALS lessons learned from other neurological diseases. Stroke. Amyotroph. Lateral Scler. Other Motor Neuron Disord. 5 Suppl 1, 31–33. doi: 10.1080/17434470410019870
Montes, S., Rivera-Mancia, S., Diaz-Ruiz, A., Tristan-Lopez, L., and Rios, C. (2014). Copper and copper proteins in Parkinson’s disease. Oxidative Med. Cell. Longev. 2014:147251. doi: 10.1155/2014/147251
Morichon, L., Hirtz, C., Tiers, L., Mezghrani, A., Raoul, C., Esselin, F., et al. (2023). Ultrasensitive digital immunoassays for SOD1 conformation in amyotrophic lateral sclerosis. Bioanalysis 15, 927–936. doi: 10.4155/bio-2023-0103
Nikoletopoulou, V., Markaki, M., Palikaras, K., and Tavernarakis, N. (2013). Crosstalk between apoptosis, necrosis and autophagy. Biochim. Biophys. Acta 1833, 3448–3459. doi: 10.1016/j.bbamcr.2013.06.001
Patel, R., and Aschner, M. (2021). Commonalities between copper neurotoxicity and Alzheimer’s disease. Toxics 9:4. doi: 10.3390/toxics9010004
Qin, X., Ma, D., Tan, Y. X., Wang, H. Y., and Cai, Z. (2019). The role of necroptosis in cancer: a double-edged sword? Biochim. Biophys. Acta Rev. Cancer 1871, 259–266. doi: 10.1016/j.bbcan.2019.01.006
Roberts, B. R., Doecke, J. D., Rembach, A., Yévenes, L. F., Fowler, C. J., McLean, C. A., et al. (2016). Rubidium and potassium levels are altered in Alzheimer’s disease brain and blood but not in cerebrospinal fluid. Acta Neuropathol. Commun. 4:119. doi: 10.1186/s40478-016-0390-8
Robinson, N. J., and Winge, D. R. (2010). Copper metallochaperones. Annu. Rev. Biochem. 79, 537–562. doi: 10.1146/annurev-biochem-030409-143539
Rui, G., Ying, K., Limin, P., Jinlang, T., Qian, Z., Yi, Z., et al. (2023). Intervention mechanism of acupuncture in treating VD based on cuproptosis and angiogenesis theory. J. Clin. Acupunct. Moxibustion 39, 1–6. doi: 10.19917/j.cnki.1005-0779.023107
Sakata, H., Niizuma, K., Wakai, T., Narasimhan, P., Maier, C. M., and Chan, P. H. (2012). Neural stem cells genetically modified to overexpress cu/zn-superoxide dismutase enhance amelioration of ischemic stroke in mice. Stroke 43, 2423–2429. doi: 10.1161/STROKEAHA.112.656900
Stuckey, S. M., Ong, L. K., Collins-Praino, L. E., and Turner, R. J. (2021). Neuroinflammation as a key driver of secondary neurodegeneration following stroke? Int. J. Mol. Sci. 22:13101. doi: 10.3390/ijms222313101
Tianwen, H., Haoyan, Z., and Zhibing, L. (2023). Identification of cuproptosis-related characteristic genes in unstable atherosclerotic plaque. Med. J. Wuhan Univ. 2023, 1–7. doi: 10.14188/j.1671-8852.2023.0195
Vallée, A., Lecarpentier, Y., Guillevin, R., and Vallée, J. N. (2020). Circadian rhythms, neuroinflammation and oxidative stress in the story of Parkinson’s disease. Cells 9:314. doi: 10.3390/cells9020314
Wang, T., Hu, L., and Fan, H. (2022). Cuproptosis and its possible role in neural cell death in ischemic stroke. Eur. J. Med. 31, 1724–1729. doi: 10.3760/cma.j.issn.1671-0282.2022.12.031
Wang, W., Lu, Z., Wang, M., Liu, Z., Wu, B., Yang, C., et al. (2022). The cuproptosis-related signature associated with the tumor environment and prognosis of patients with glioma. Front. Immunol. 13:998236. doi: 10.3389/fimmu.2022.998236
Xiong, C., Ling, H., Hao, Q., and Zhou, X. (2023). Cuproptosis: p53-regulated metabolic cell death? Cell Death Differ. 30, 876–884. doi: 10.1038/s41418-023-01125-0
Xu, S., Tang, L., Li, X., Fan, F., and Liu, Z. (2020). Immunotherapy for glioma: current management and future application. Cancer Lett. 476, 1–12. doi: 10.1016/j.canlet.2020.02.002
Yang, L., Chen, X., Cheng, H., and Zhang, L. (2022). Dietary copper intake and risk of stroke in adults: a case-control study based on national health and nutrition examination survey 2013-2018. Nutrients 14:409. doi: 10.3390/nu14030409
Zhang, M., Li, W., Wang, Y., Wang, T., Ma, M., and Tian, C. (2020). Association between the change of serum copper and ischemic stroke: a systematic review and meta-analysis. J. Mol. Neurosci. 70, 475–480. doi: 10.1007/s12031-019-01441-6
Zhang, P., Wang, C., Wu, J., and Zhang, S. (2021). A systematic review of the predictive value of plasma D-dimer levels for predicting stroke outcome. Front. Neurol. 12:693524. doi: 10.3389/fneur.2021.693524
Keywords: stroke, cuproptosis, pathogenesis, treatment, mechanism
Citation: Xing L, Wang Z, Hao Z, Pan P, Yang A and Wang J (2024) Cuproptosis in stroke: focusing on pathogenesis and treatment. Front. Mol. Neurosci. 17:1349123. doi: 10.3389/fnmol.2024.1349123
Edited by:
Jingqi Yan, Cleveland State University, United StatesReviewed by:
Ajay Ashok, Schepens Eye Research Institute and Harvard Medical School, United StatesCopyright © 2024 Xing, Wang, Hao, Pan, Yang and Wang. This is an open-access article distributed under the terms of the Creative Commons Attribution License (CC BY). The use, distribution or reproduction in other forums is permitted, provided the original author(s) and the copyright owner(s) are credited and that the original publication in this journal is cited, in accordance with accepted academic practice. No use, distribution or reproduction is permitted which does not comply with these terms.
*Correspondence: Jian Wang, bGFvaGFvZGlhb0AxMjYuY29t
†These authors have contributed equally to this work