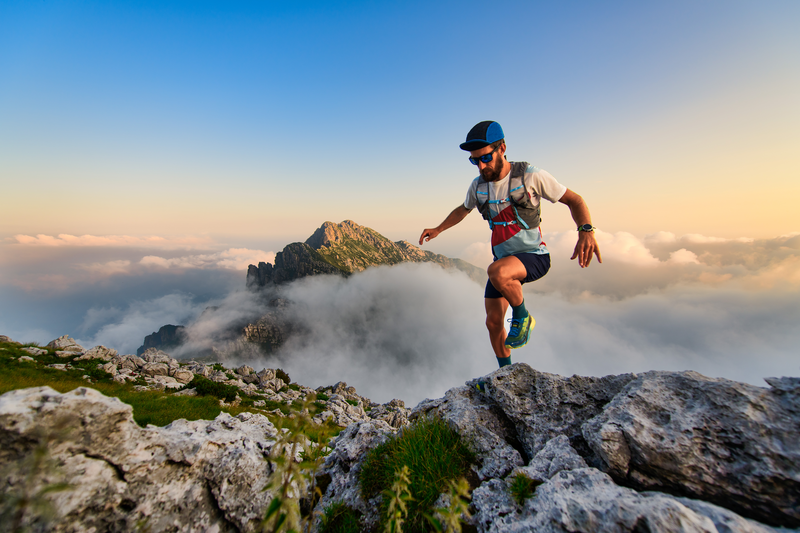
94% of researchers rate our articles as excellent or good
Learn more about the work of our research integrity team to safeguard the quality of each article we publish.
Find out more
REVIEW article
Front. Mol. Neurosci. , 11 January 2024
Sec. Molecular Signalling and Pathways
Volume 16 - 2023 | https://doi.org/10.3389/fnmol.2023.1340725
Five muscarinic acetylcholine (mACh) receptor subtypes are divided into two classes: the M1 class (M1, M3, and M5) and the M2 class (M2 and M4). The former is coupled to Gq proteins, while the latter is coupled to Gi/o proteins. Accumulating evidence indicates that mACh receptors play a significant role in the regulation of the Src family kinase (SFK), a subfamily of non-receptor tyrosine kinases. mACh receptors exert their roles in a subtype-dependent fashion and preferentially target Src and Fyn, two members of SFKs that are expressed in the brain and enriched at synaptic sites. While the M1 receptor positively modulates SFK activity, the M4 receptor inhibits it. By modulating SFKs, mACh receptors are actively involved in the regulation of expression and function of a variety of receptors, structural proteins, and signaling molecules. In particular, the M4 receptor and the dopamine D1 receptor are coexpressed in striatonigral projection neurons of the striatum. Gi/o-coupled M4 and Gq-coupled D1 receptors antagonistically regulate SFK activity, thereby forming a dynamic balance controlling glutamate receptor activity, excitability of neurons, and synaptic plasticity. In summary, mACh receptors play a crucial role in regulating SFK activity in heterologous cells and neurons.
The non-receptor tyrosine kinase (nRTK) family consists of a panel of kinases that tyrosine-phosphorylate proteins and thereby regulate a variety of cellular and signaling activities involving the phosphorylation-modified proteins. In the central nervous system, nRTKs play a pivotal role in the regulation of neuronal and synaptic activities and in the pathogenesis and symptomatology of various neurological and neuropsychiatric disorders (Neet and Hunter, 1996). The Src family kinase (SFK) is a subfamily of nRTKs. Several SFK members (Src, Fyn, Yes, Lyn, and Lck) are expressed in the brain (Omri et al., 1996; Kalia et al., 2004; Bongiorno-Borbone et al., 2005). Noticeably, Src and Fyn are enriched at synaptic sites (Ohnishi et al., 2011). In addition to their abundant postsynaptic presence, Src and Fyn reside and function presynaptically (Onofri et al., 1997; Nakamura et al., 2001). As such, both SFK members are implicated in the modulation of synaptic transmission and plasticity (Kalia et al., 2004; Ohnishi et al., 2011; Schenone et al., 2011). A large number of substrates of Src and/or Fyn have been identified in the cytoplasmic and synaptic compartments, including receptors, ion channels, enzymes, signaling molecules, etc. By binding to these substrates, SFKs phosphorylate specific tyrosine sites on them and dynamically modulate their expression and function.
Acetylcholine is an essential neurotransmitter in the mammalian brain. This transmitter interacts with nicotinic and muscarinic acetylcholine (mACh) receptors to achieve its action. While nicotinic receptors are ion channels, mACh receptors are G protein-coupled receptors (GPCR). Based on the type of G proteins that mACh receptors are connected to, five subtypes of mACh receptors (M1–M5) are divided into two classes: the M1 class and the M2 class (Caulfield and Birdsall, 1998). The M1 class of mACh receptors includes M1, M3, and M5 subtypes which are coupled to Gq proteins. The M2 class, i.e., M2 and M4 subtypes, is coupled to Gi/o proteins (Wess, 1996). As such, activation of the M1 class activates phospholipase Cβ1 (PLCβ1), yielding two downstream signaling molecules, diacylglycerol (DAG) and inositol-1,4,5-triphosphate (IP3). The former activates protein kinase C (PKC), whereas the latter induces Ca2+ release from the intracellular Ca2+ stores. Activation of the M2 class inhibits adenylyl cyclase, leading to the reduction of cAMP formation and inhibition of protein kinase A (PKA) activity. By triggering distinct signaling pathways, mACh receptors exert the receptor subtype-specific regulation of neuronal and synaptic activities.
SFK activity is regulated by changing cellular and synaptic input in a phosphorylation-dependent manner. The upregulated SFK activity is seen following an increase in autophosphorylation at a specific site. That is, phosphorylation of SFKs at a conserved residue, pan tyrosine 416 (Y416), within the activation loop results in activation of SFKs (Roskoski, 2005; Okada, 2012). Multiple neurotransmitters have been found to regulate SFKs through altering Y416 phosphorylation. Among these transmitters is acetylcholine. Accumulating evidence shows that stimulation of mACh receptors has a profound impact on SFK activity in transfected mammalian cells and in neurons at various brain regions. For instance, pharmacological stimulation of the M1 class of mACh receptors activates SFKs and thereby regulates a discrete set of downstream targets. Meanwhile, stimulation of the M2 class, especially the M4 subtype, has a significant impact on SFK activity, leading to changes in SFK-mediated tyrosine phosphorylation of a number of substrates. We in this review summarize the mACh receptor-mediated regulation of SFKs in heterologous cells and neurons. Of note, mACh receptors and SFKs are broadly distributed in the mammalian brain. Their expression and interactions in the hippocampus may play significant roles in memory, emotion, and cognitive function, while an active state of mACh-SFK coupling in the striatum may be implicated in the modulation of motivation, reinforcement, and reward perception.
GPCRs are linked to SFKs (Berndt and Liebschcer, 2021; Perez et al., 2022) and among these GPCRs is the mACh receptor. A large number of early studies have observed consistent results, establishing that mACh receptors can activate SFKs to regulate a variety of downstream targets. For instance, application of the mACh selective agonist muscarine potentiated N-methyl-D-aspartate (NMDA)-evoked currents in acutely isolated hippocampal CA1 pyramidal neurons (Lu et al., 1999; Tian et al., 2016). This muscarine-induced potentiation was blocked by the tyrosine kinase inhibitor lavendustin A, but not its inactive analog lavendustin B. The effect of muscarine was also blocked by a Src inhibitory peptide Src (40–58) but not the scrambled sequence control sSrc (40–58). Since Src (40–58) disrupts the Src interaction with NMDA receptor GluN2A subunits (Gingrich et al., 2004) and thereby selectively blocks the effect of Src but not Fyn on their substrates (Yang et al., 2012), Src was believed to be activated by muscarine to link mACh signals to NMDA receptors. In contrast to Src, Fyn seems insignificant in this event since a Fyn interfering peptide Fyn39–57 did not block the muscarine-induced NMDA current potentiation in hippocampal CA1 neurons (Tian et al., 2016).
In addition to the Src-NMDA receptor pathway (Rajani et al., 2021), mACh receptors have been found to engage SFKs to activate other proteins and signaling pathways. Pharmacological stimulation of mACh receptors with a non-selective agonist carbachol increased phosphorylation of extracellular signal-regulated kinases (ERK) in cultured cortical neurons (Rosenblum et al., 2000). The SFK inhibitor PP1 reduced this increase. Since carbachol retained its ability to activate ERK in cortical cultures from Fyn knockout mice, Fyn may not participate in processing the mACh regulation of ERK. Additionally, carbachol or muscarine stimulated (1) phosphorylation of PKCδ (Benes and Soltoff, 2001) and focal adhesion kinase (FAK) (Jope et al., 1999; Watcharasit et al., 2001), (2) expression of the activity-regulated cytoskeleton-associated gene (ARC) (Teber et al., 2004), (3) secretion of a soluble amyloid precursor protein in human neuroblastoma SH-SY5Y cells (Canet-Aviles et al., 2002), and (4) activity of ERK and/or the cAMP-responsive element-binding protein in rat neural precursor cells (Zhao et al., 2003), MCF-7 human breast cancer cells (Jimenez and Montiel, 2005), and oligodendrocytes progenitors (Cui et al., 2006). All of these carbachol- or muscarine-stimulated events were blocked by PP1 or another SFK inhibitor PP2, indicating that SFKs take part in forming a signaling pathway linking mACh receptors to these targets. In addition to carbachol and muscarine, donepezil (a selective acetylcholinesterase inhibitor) was used in exploring the cholinergic receptor-SFK coupling. Donepezil by inhibiting cholinesterase-catalyzed hydrolysis of acetylcholine increases acetylcholine concentrations at cholinergic synapses. Recent studies demonstrated that donepezil promoted stroke-induced neurogenesis in the rat and mouse subventricular zone (Wang et al., 2017; Man et al., 2020), while the mACh receptor antagonist atropine reduced it in the mouse subventricular zone (Wang et al., 2017). Since the effect of donepezil was abolished by the Src inhibitor KX-01 (Man et al., 2020), active Src is required for linking mACh receptors to enhanced neurogenesis.
Along with the above indirect data observed with SFK inhibitors, evidence for activation of SFKs in response to mACh receptor agonists was obtained by measuring SFK phosphorylation at Y416. Carbachol, for instance, increased SFK phosphorylation at Y416, i.e., activation of SFKs, in pyramidal neurons of rat prefrontal cortex (PFC) slices (Ma et al., 2003) and cultured rat neural precursor cells (Zhao et al., 2003). Activated Src may mediate the carbachol-induced potentiation of γ-aminobutyric acid (GABA)A receptor-mediated currents because (1) the Src inhibitory peptide Src (40–58) but not its control sSrc (40–58) prevented the potentiation of GABAA receptors induced by carbachol, and (2) injecting the active enzyme p60-cSrc into PFC neurons mimicked the effect of carbachol (Ma et al., 2003). Of note, carbachol also enhanced tyrosine phosphorylation of immunopurified Fyn but not Lyn from cultured rat oligodendrocyte progenitors (Cui et al., 2006). In pancreatic acinar cells, the SFK member Yes was activated by carbachol as demonstrated by an increase in Yes-Y416 phosphorylation in response to carbachol (Sancho et al., 2012).
While mACh receptor agonists, probably through activating the M1 class (see below), activate SFKs, mACh receptor antagonists also elevate SFK activity in a specific brain region. In a dopamine-innervated brain region, i.e., the striatum where SFKs (Src and Fyn) and M4 receptors are abundantly expressed (Levey et al., 1991; Pascoli et al., 2011), the non-subtype-selective mACh receptor antagonist scopolamine after a systemic injection markedly enhanced SFK Y416 phosphorylation in adult rats in vivo (Mao et al., 2018). The scopolamine stimulation of Y416 phosphorylation occurred in the two subdivisions of the striatum, the caudate putamen and nucleus accumbens. Another mACh antagonist atropine produced the similar increase in striatal Y416 phosphorylation. These findings indicate that mACh receptors in the striatum inhibit basal phosphorylation of SFK Y416 under normal conditions, probably via a subtype-specific mechanism involving M4 receptors (see below). Of note, scopolamine phosphorylated Fyn rather than Src immunopurified from the striatum, indicating a selective effect of scopolamine on Fyn (Mao et al., 2018). Additionally, coadministration of scopolamine and a dopamine D1 receptor agonist SFK81297 at their subthreshold doses induced a significant increase in SFK Y416 phosphorylation in the striatum (Mao et al., 2018). This suggests that the mACh receptor-mediated cholinergic transmission and the D1-mediated dopaminergic transmission antagonistically interact with each other to form an intrinsic balance within the striatum controlling SFK homeostasis (see below).
Application of carbachol enhanced Src autophosphorylation at Y418 (Y416 in chicken Src), an indicator of Src activation, in rat PFC slices (Ma et al., 2003). Given that M1 receptor mRNAs were most abundant in PFC pyramidal neurons (Ma et al., 2003), the M1 subtype may participate in mediating the effect of carbachol on Src in these neurons. In support of this notion, the M1 antagonist pirenzepine blocked the Src-dependent potentiation of GABAA receptors in rat PFC neurons in response to carbachol (Ma et al., 2003). Moreover, carbachol stimulated Gq-coupled mACh receptors to increase Y416 phosphorylation of immunopurified Src in HEK293 cells (Vazquez et al., 2004).
The M1 receptor-mediated upregulation of SFK activity affects several surface-expressed receptor activities. As aforementioned, muscarine potentiated NMDA receptor activity via activating Src in hippocampal CA1 neurons (Lu et al., 1999; Tian et al., 2016). This potentiation was likely mediated by the M1 class of mACh receptors since (1) the hippocampus is enriched with M1 receptors (Levey, 1996), (2) the muscarine-induced potentiation of NMDA receptors in this region was mimicked by the M1 agonist xanomeline and was blocked by the M1 antagonist pirenzepine (Tian et al., 2016), and (3) inhibition of SFKs with PP2 prevented the carbachol-triggered and M1-mediated phosphorylation of NMDA receptor subunits (GluN2B) at Y1472 in primary rat cortical cultures (Chen et al., 2016). In addition to NMDA receptors, M1 receptors are believed to engage active Src to potentiate GABAA receptor activity in rat PFC pyramidal neurons (Ma et al., 2003). Moreover, M1 receptors interacted with fibroblast growth factor receptors (FGFR) to form heteroreceptor complexes in hippocampus cultures, and as a result, M1 receptor signals could readily transactivate FGFRs via a Src-dependent manner (Di Liberto et al., 2017).
M1-activated SFKs may serve as a key transducer linking M1 receptors to many other downstream effectors. For example, mitogen-activated protein kinases (MAPK) form an essential intracellular signaling pathway. Carbachol via stimulating M1 receptors activated the MAPK/ERK pathway in COS-7 cells (Igishi and Gutkind, 1998; Rosenblum et al., 2000). In DT40 cells deficient in Lyn, M1 receptors failed to stimulate MAPKs, indicating the role of Lyn in linking M1 receptors to MAPK (Wan et al., 1996). The M1-regulated ERK phosphorylation was also SFK-dependent in SK-N-MC human brain neuroepithelioma cells (Chan et al., 2005). In addition to the MAPK/ERK pathway, carbachol stimulation of M1 and/or M3 receptors activated SFKs to elevate ARC expression in SH-SY5Y cells (Teber et al., 2004) and in primary rat cortical neurons (Chen et al., 2016). Activation of Gq-coupled mACh receptors obligated Src to activate transient receptor potential (TRP) channels in HEK293 cells (Vazquez et al., 2004). Stimulation of M1 receptors induced endocytosis of TWIK (tandem of P domains in a weak inwardly rectifying K+ channel)-related acid-sensitive K+ (TASK)1 channels in rat adrenal medullary cells (Matsuoka and Inoue, 2017).
The postreceptor signaling pathway(s) linking M1 receptors to SFKs have been studied in heterologous cells and neurons. M1 receptors are known to be coupled to pertussis toxin-insensitive heterotrimeric Gq proteins, including αq, β, and γ subunits. Evidence shows that both Gαq and Gβ/γ dimers play important roles in relaying M1 signals to Src. In HEK293 cells, a constitutively active mutant of Gαq proteins stimulated SFK activity, and active SFKs then tyrosine-phosphorylated multiple downstream proteins (Nagao et al., 1998). In COS-7 cells stably expressing recombinant M1 receptors, stimulating M1 receptors induced a PP1-sensitive phosphorylation of MAPKs (Igishi and Gutkind, 1998). Overexpression of Gβ/γ dimers in these cells also activated MAPKs, which was inhibited by the dominant-negative Src (Igishi and Gutkind, 1998). At the level downstream to G proteins, M1 receptors are known to activate PLCβ1 to produce DAG which sequentially activates PKC. After PKC activation, evidence shows that proline-rich tyrosine kinase 2 (Pyk2) (also known as cell adhesion kinase β, CAKβ), a member of FAK family of nRTKs, works as an intermediary protein between PKC and Src (Yang et al., 2014; Figure 1). Indeed, M1 receptors were found to activate Pyk2 (Felsch et al., 1998), and active Pyk2 then served as an effector to link PKC to Src in response to M1 stimulation in a stable cell line (Felsch et al., 1998) or mACh receptor stimulation in rat PFC slices (Ma et al., 2003). Similarly, in PC12 cells, muscarinic receptor stimulation activated Src through the PKC-Pyk2 pathway, which led to TASK1 channel endocytosis (Matsuoka et al., 2020).
Figure 1. The M1 receptor-associated signaling pathway in activation of intracellular Src proteins. Activation of Gq-coupled M1 receptors results in activation of PLC, which subsequently hydrolyzes phosphoinositide (PI) to yield DAG and IP3 molecules. DAG functions as an activator of downstream PKC. Active PKC then promotes Src activation through an intermediary protein Pyk2.
In addition to the M1 subtype, the M3 subtype appears to be linked to SFKs. In HEK cells stably expressing M3 receptors, carbachol showed the ability to activate MAPKs (Slack, 2000). The activation of MAPKs by M3 receptor stimulation was partially reduced by PP1, indicating that M3 receptors may in part activate PP1-sensitive SFKs to stimulate MAPKs. Similarly, stimulation of M3 receptors with the muscarinic receptor agonist pilocarpine caused activation of MAPK/ERK1/2 in mouse insulinoma cells, and the stimulatory effect of pilocarpine was blocked by PP2 (Pronin et al., 2017).
Beyond the MAPK/ERK pathway, M3 receptors regulate another downstream kinase via a SKF/Fyn-dependent mechanism. In SH-SY5Y cells expressing M3 receptors, the non-subtype-selective mACh agonist oxotremorine-M increased tyrosine phosphorylation of the activated Cdc42Hs-associated kinase-1 (ACK-1), which was blocked by the SFK inhibitor (Linseman et al., 2001). Since loading cells with the Fyn-SH2 or Fyn-SH3 domain that reduced the SH2- and SH3-mediated interactions between Fyn and ACK-1 attenuated the effect of oxotremorine-M, Fyn tyrosine kinase was likely activated by oxotremorine-M to link M3 receptors to ACK-1 (Linseman et al., 2001).
The M2 class of mACh receptors includes the M2 and M4 subtypes. Studies conducted in heterologous cell lines in vitro reveal the linkage of M2 receptors to SFKs. Pharmacological stimulation of M2 receptors increased Src but not Fyn activity in cultured colonic smooth muscle cells (Singer et al., 2002) and activated PP2-sensitive SFKs in gastric smooth muscle cells (Mahavadi et al., 2007). M2 receptors also activated Src in COS-7 cells (Igishi and Gutkind, 1998) and Fyn in SH-SY5Y cells (Stirnweiss et al., 2006). The M2-mediated upregulation of Src seems to be mediated through a signaling mechanism involving Gβ/γ (Igishi and Gutkind, 1998; Murthy, 2008). In a recent study, M2 receptors with the phosphorylated C-terminal tail were able to interact with Gβ -arrestin-1, which constitutes a necessary and sufficient step to allosterically activate a downstream effector (Src) by promoting Src autophosphorylation in vitro (Pakharukova et al., 2020).
At present, the M2-SFK relationship in neurons is less well characterized due to limited studies. One study demonstrated that stimulation of M2 receptors induced hyperpolarization of local GABAergic interneurons of the mouse thalamus by recruiting Gβγ, class-1A phosphatidylinositol-4,5-bisphosphate 3-kinase (PI3K), and c-Src, leading to activation of TASK-1 channels in these interneurons (Leist et al., 2017).
Meanwhile, initial studies investigated the potential role of M4 receptors in the regulation of SFKs in neurons from the striatum, one of brain regions known for its highest level of M4 receptors and its significance in mood, cognitive, and motor functions (Levey et al., 1991; Hersch et al., 1994; Chapman et al., 2011). Pharmacological blockade of mACh receptors by scopolamine readily increased SFK Y416 phosphorylation in the rat striatum (Mao et al., 2018). The scopolamine effect was likely mediated by blocking the M4 subtype as M4 receptors are inhibitory in nature (i.e., inhibiting the cAMP/PKA pathway, also see below) and represent a subtype from the M2 class expressed in striatonigral output neurons (Levey et al., 1991; Ince et al., 1997; Santiago and Potter, 2001). These data indicate that there exists a tonic M4 receptor-dependent inhibition of SKF activity in striatal neurons. As such, pharmacological blockade of these mACh receptors leads to upregulation of SFK activity in the region. Moreover, the M4 inhibition appears to function at a relatively high level under basal conditions, given that exogenous application of a systemically active positive allosteric modulator (PAM) selective for M4 receptors (VU0152100) exhibited a minimal impact on spontaneous SFK Y416 phosphorylation in the rat striatum (Mao and Wang, 2015).
The postreceptor signaling pathway linking M4 receptors to SFK/Fyn may involve cAMP and PKA. As a Gi/o-coupled receptor, the M4 receptor inhibits cAMP formation and thereby reduces PKA activity (Wess, 1996). Interestingly, Fyn contains a PKA recognition motif (RxxS) on its amino terminal SH4 domain. Within this motif, the S21 residue was phosphorylated by PKA, and S21A mutation (phosphorylation-deficient mutation) blocked PKA phosphorylation of Fyn (Yeo et al., 2011). The PKA-catalyzed Fyn S21 phosphorylation was critical for regulating Fyn activity as S21A mutation caused a deficit of the ability of Fyn in modulating cell mobility (Yeo et al., 2011). Thus, M4 receptors likely inhibit the cAMP-PKA pathway to suppress Fyn activity (Figure 2). Consistent with this notion, stimulating PKA with forskolin upregulated Fyn activity although not Src activity in spinal neurons (Yang et al., 2011).
Figure 2. The M4 receptor-associated signaling pathway in inhibition of cytoplasmic Fyn. Fyn activity is likely regulated by the adenylyl cyclase/cAMP/PKA pathway. Active adenylyl cyclase is known to increase cAMP formation and thereby activate PKA. Active PKA can then phosphorylate Fyn at a serine site (S21), which is a critical event for maintaining Fyn kinase activity. Gs-coupled D1 receptors and Gi/o-coupled M4 receptors are co-expressed in striatonigral projection neurons within the striatum. Since the two receptors have the opposite effects on adenylyl cyclase, they form a dynamic balance controlling the cAMP/PKA pathway and thus Fyn activity in these neurons.
In addition to M4 receptors, dopamine D1 and D2 receptors are expressed in the striatum. As the major dopamine receptor subtypes in this region, these two receptors are segregated into two subpopulations of medium spiny projection neurons: D1 receptors in striatonigral neuron and D2 receptors in striatopallidal neurons (Gerfen et al., 1990; Aubert et al., 2000; Bertran-Gonzalez et al., 2010). Consistent data show that D1 receptors stimulate Fyn in the striatum, while D2 receptors inhibit it. Specifically, D1 agonists and D2 antagonists enhanced Fyn although not Src phosphorylation in striatal neurons (Dunah et al., 2004; Hattori et al., 2006; Pascoli et al., 2011; Mao and Wang, 2015, 2016a) as well as hippocampal neurons (Yang et al., 2012). Remarkably, D1 and M4 receptors are coexpressed in striatonigral neurons (Ince et al., 1997; Santiago and Potter, 2001). This provides a basis for two receptors to crosstalk. It is possible that Gs-coupled D1 receptors and Gi/o-coupled M4 receptors work in concert to form a dynamic balance to regulate the cAMP-PKA pathway and thus Fyn in striatonigral neurons (Figure 2). Consistent with this model, the M4 PAM VU0152100 attenuated the D1 agonist SFK81297-stimulated SFK Y416 phosphorylation in the rat striatum (Mao and Wang, 2015). Coadministration of SKF81297 and scopolamine consistently induced a synergistic increase in striatal Y416 phosphorylation (Mao et al., 2018).
Early studies have evaluated the role of mACh receptors in the regulation of SFKs in transfected cells or stable cell lines expressing a specific subtype of mACh receptors. It was found that the M1 subtype exhibits a profound influence over SFK activity. Pharmacological stimulation of M1 receptors activates SFKs as evidenced by an increase in autophosphorylation of SFKs at a conserved residue (Y416) in the activation loop of SFKs. Activated SFKs then serve as an essential element in forming a signaling pathway relaying M1 signals to various downstream effectors, including receptors, ion channels, enzymes, etc. Recent studies attempted to define the role of mACh receptors in neurons. Remarkably, M4 receptors seem to show an inhibitory role in regulating SFKs in striatal neurons. The M4 receptor also works in concert with the D1 receptor to control SFK activity in striatonigral output neurons coexpressing M4 and D1 receptors. M4/D1-regulated SFKs are able to link integrated signals from these receptors to glutamate receptors, thereby determining the excitability of these neurons in relation to synaptic transmission and plasticity.
While evidence has shown the existence of the linkage of mACh receptors to SFKs, precise signaling pathway(s) linking the receptor to the kinase are less clear. The cAMP-PKA pathway has been implicated in connecting M4 receptors to Fyn in striatal neurons. More studies are needed to confirm the role of the cAMP-PKA pathway in this event and to determine whether the M4-mediated regulation of Fyn occurs in M4-bearing striatonigral output neurons. In addition, the selectivity of SFK members subjected to the regulation by mACh receptors needs to be explored and characterized in neurons. Five among nine members of SFKs are known to be present in the brain, including Src, Fyn, Yes, Lyn, and Lck (Omri et al., 1996; Kalia et al., 2004; Bongiorno-Borbone et al., 2005). These SFK members are also distributed at synaptic sites (Kalia and Salter, 2003). Thus, they are thought to constitute a set of regulators essential for the modulation of synaptic transmission and plasticity. Evidence has already been shown to support the contribution of Src and Fyn in this regard (Mao et al., 2017; Matrone et al., 2020; Rajani et al., 2021). Future studies will target other members of SFKs to elucidate their individual contributions.
Ionotropic and metabotropic glutamate receptors have been identified to be biochemical substrates of Src/Fyn (Groveman et al., 2012; Mao and Wang, 2016b; Jin et al., 2017). SFKs bind to the intracellular domain of glutamate receptors and phosphorylate these receptors at specific residues to regulate trafficking, subcellular and subsynaptic distribution, and functions of modified receptors. Through a SFK-dependent pathway, mACh signals may modulate glutamate receptors. Indeed, the M1 agonist potentiated GluN2B-containing NMDA receptors in hippocampal CA1 neurons via Src (Ishibashi et al., 2014). The M4 PAM VU0152100 reduced the D1 agonist-stimulated GluN2B Y1472 phosphorylation in striatal neurons (Mao and Wang, 2015), indicating that the D1-regulated NMDA receptor phosphorylation is subject to the inhibitory modulation by M4 receptors. In addition to glutamate receptors, SFKs are shown to target other local synaptic proteins and coordinate their responses to changing synaptic input. Moreover, the mACh-SFK coupling in the hippocampus and striatum is thought to be critical for maintaining normal memory, cognitive behavior, mood, and movement. Dysfunction of this coupling is linked to pathogenesis of various neuropsychiatric and neurological illnesses (Ge et al., 2020; Guglietti et al., 2021; Rajani et al., 2021; Portugal et al., 2022; Wang et al., 2022).
L-MM: Conceptualization, Formal Analysis, Writing – original draft. LY: Validation, Writing – review and editing. X-PC: Validation, Writing – review and editing. JW: Conceptualization, Funding acquisition, Supervision, Validation, Writing – original draft.
The author(s) declare financial support was received for the research, authorship, and/or publication of this article. This work by the authors discussed in this article was supported by the NIH grant (R01 MH061469). JW holds the Westport Anesthesia/Missouri Endowed Chair.
We want to thank the NIH for the research grant that promotes the production of this and other publications.
The authors declare that the research was conducted in the absence of any commercial or financial relationships that could be construed as a potential conflict of interest.
All claims expressed in this article are solely those of the authors and do not necessarily represent those of their affiliated organizations, or those of the publisher, the editors and the reviewers. Any product that may be evaluated in this article, or claim that may be made by its manufacturer, is not guaranteed or endorsed by the publisher.
ACK-1, activated Cdc42Hs-associated kinase-1; ARC, activity-regulated cytoskeleton-associated gene; CAK β, cell adhesion kinase β; DAG, diacylglycerol; ERK, extracellular signal-regulated kinases; FAK, focal adhesion kinase; FGFR, fibroblast growth factor receptors; GABA, γ -aminobutyric acid; GPCR, G protein-coupled receptors; IP3, inositol-1,4,5-triphosphate; mACh, muscarinic acetylcholine; MAPK, mitogen-activated protein kinases; NMDA, N-methyl -D-aspartate; nRTK, non-receptor tyrosine kinase; PAM, positive allosteric modulator; PFC, prefrontal cortex; PI, phosphoinositide; PI3K, phosphatidylinositol-4,5-bisphosphate 3-kinase; PKA, protein kinase A; PKC, protein kinase C; PLC β 1, phospholipase C β 1; Pyk2, proline-rich tyrosine kinase 2; SFK, Src family kinase; TASK, TWIK-related acid-sensitive K+; TRP, transient receptor potential; TWIK, tandem of P domains in a weak inwardly rectifying K+ channel.
Aubert, I., Ghorayeb, I., Normand, E., and Bloch, B. (2000). Phenotypical characterization of the neurons expressing the D1 and D2 dopamine receptors in the monkey striatum. J. Comp. Neurol. 418, 22–32.
Benes, C., and Soltoff, S. P. (2001). Modulation of PKAdelta tyrosine phosphorylation and activity in salivary and PC-12 cells by Src kinases. Am. J. Physiol. Cell. Physiol. 280, C1498–C1510. doi: 10.1152/ajpcell.2001.280.6.C1498
Berndt, S., and Liebschcer, I. (2021). New structural perspectives in G protein-coupled receptor-mediated Src family kinase activation. Int. J. Mol. Sci. 22:6489. doi: 10.3390/ijms22126489
Bertran-Gonzalez, J., Herve, D., Girault, J. A., and Valjent, E. (2010). What is the degree of segregation between striatonigral and striatopallidal projections? Front. Neuroanat. 4:136. doi: 10.3389/fnana.2010.00136
Bongiorno-Borbone, L., Kadare, G., Benfenati, F., and Girault, J. A. (2005). FAK and PYK2 interact with SAP90/PSD-95-associated protein-3. Biochem. Biophys. Res. Commun. 337, 641–646. doi: 10.1016/j.bbrc.2005.09.099
Canet-Aviles, R. M., Anderton, M., Hooper, N. M., Turner, A. J., and Vaughan, P. F. (2002). Muscarine enhances soluble amyloid precursor protein secretion in human neuroblastoma SH-SY5Y by a pathway dependent on protein kinase C(alpha), src-tyrosine kinase and extracellular signal-regulated kinase but not phospholipase C. Mol. Brain Res. 102, 62–72. doi: 10.1016/s0169-328x(02)00184-5
Caulfield, M. P., and Birdsall, N. J. (1998). International union of pharmacology. XVII. Classification of muscarinic acetylcholine receptors. Pharmacol. Rev. 50, 279–290.
Chan, A. S., Yeung, W. W., and Wong, Y. H. (2005). Integration of G protein signals by extracellular signal-regulated protein kinases in SK-N-MC neuroepithelioma cells. J. Neurochem. 94, 1457–1470. doi: 10.1111/j.1471-4159.2005.03304.x
Chapman, K. L., Vaswani, D., Hendry, N., Langmead, C. J., Kew, J. N., and Watson, J. M. (2011). The muscarinic M(4) receptor is the functionally predominant subtype in rat and mouse striatum as demonstrated using [(35)S] GTPγS binding. Eur. J. Pharmacol. 652, 1–6.
Chen, T. J., Chen, S. S., Wang, D. C., and Hung, H. S. (2016). The cholinergic signaling responsible for the expression of a memory-related protein in primary rat cortical neurons. J. Cell. Physiol. 231, 2428–2438. doi: 10.1002/jcp.25347
Cui, Q. L., Fogle, E., and Almazan, G. (2006). Muscarinic acetylcholine receptors mediate oligodendrocyte progenitor survival through Src-like tyrosine kinases and PI3K/Akt pathways. Neurochem. Int. 48, 383–393. doi: 10.1016/j.neuint.2005.11.014
Di Liberto, V., Borroto-Escuela, D. O., Frinchi, M., Verdi, V., Fuxe, K., Belluardo, N., et al. (2017). Existence of muscarinic acetylcholine receptor (mAChR) and fibroblast growth factor receptor (FGFR) heteroreceptor complexes and their enhancement of neurite outgrowth in neural hippocampal cultures. Biochim. Biophys. Acta Gen. Subj. 1861, 235–245. doi: 10.1016/j.bbagen.2016.10.026
Dunah, A. W., Sirianni, A. C., Fienberg, A. A., Bastia, E., Schwarzschild, M. A., and Standaert, D. G. (2004). Dopamine D1-dependent trafficking of striatal N-methyl-D-aspartate glutamate receptors requires Fyn protein tyrosine kinase but not DARPP-32. Mol. Pharmacol. 65, 121–129. doi: 10.1124/mol.65.1.121
Felsch, J. S., Cachero, T. G., and Peralta, E. G. (1998). Activation of protein tyrosine kinase PYK2 by the m1 muscarinic acetylcholine receptor. Proc. Natl. Acad. Sci. U.S.A. 95, 5051–5056.
Ge, M. M., Zhou, Y. Q., Tian, X. B., Manyande, A., Tian, Y. K., Ye, D. W., et al. (2020). Src-family protein tyrosine kinases: A promising target for treating chronic pain. Biomed. Pharmacother. 125:110017. doi: 10.1016/j.biopha.2020.110017
Gerfen, C. R., Engber, T. M., Mahan, L. C., Susel, Z., Chase, T. N., and Monsma, F. J. Jr., et al. (1990). D1 and D2 dopamine receptor-regulated gene expression of striatonigral and striatopallidal neurons. Science 250, 1429–1432.
Gingrich, J. R., Pelkey, K. A., Fam, S. R., Huang, Y., Petralia, R. S., Wenthold, R. J., et al. (2004). Unique domain anchoring of Src to synaptic NMDA receptors via the mitochondrial protein NADH dehydrogenase subunit 2. Proc. Natl. Acad. Sci. U.S.A. 101, 6237–6242. doi: 10.1073/pnas.0401413101
Groveman, B. R., Feng, S., Fang, X. Q., Plueger, M., Lin, S. X., Bienkiewicz, E. A., et al. (2012). The regulation of N-methyl-D-aspartate receptors by Src kinase. FEBS J. 279, 20–28.
Guglietti, B., Sivasankar, S., Mustafa, S., Corrigan, F., and Collins-Praino, L. E. (2021). Fyn kinase activity and its role in neurodegenerative disease pathology: A potential universal target? Mol. Neurobiol. 58, 5986–6005. doi: 10.1007/s12035-021-02518-3
Hattori, K., Uchino, S., Isosaka, T., Maekawa, M., Iyo, M., Sato, T., et al. (2006). Fyn is required for haloperidol-induced catalepsy in mice. J. Biol. Chem. 281, 7129–7135. doi: 10.1074/jbc.M511608200
Hersch, S. M., Gutekunst, C. A., Rees, H. D., Heilman, C. J., and Levey, A. I. (1994). Distribution of m1-m4 muscarinic receptor proteins in the rat striatum: Light and electron microscopic immunocytochemistry using subtype-specific antibodies. J. Neurosci. 14, 3351–3363. doi: 10.1523/JNEUROSCI.14-05-03351.1994
Igishi, T., and Gutkind, J. S. (1998). Tyrosine kinases of the Src family participate in signaling to MAP kinase from both Gq and Gi-coupled receptors. Biochem. Biophys. Res. Commun. 244, 5–10. doi: 10.1006/bbrc.1998.8208
Ince, E., Ciliax, B. J., and Levey, A. I. (1997). Differential expression of D1 and D2 dopamine and m4 muscarinic acetylcholine receptor proteins in identified striatonigral neurons. Synapse 27, 357–366. doi: 10.1002/(SICI)1098-2396(199712)27:4<357::AID-SYN9>3.0.CO;2-B
Ishibashi, M., Yamazaki, Y., Miledi, R., and Sumikawa, K. (2014). Nicotinic and muscarinic agonists and acetylcholinesterase inhibitors stimulate a common pathway to enhance GluN2B-NMDAR responses. Proc. Natl. Acad. Sci. U.S.A. 111, 12538–12543. doi: 10.1073/pnas.1408805111
Jimenez, E., and Montiel, M. (2005). Activation of MAP kinase by muscarinic cholinergic receptors induces cell proliferation and protein synthesis in human breast cancer cells. J. Cell. Physiol. 204, 678–686. doi: 10.1002/jcp.20326
Jin, D. Z., Mao, L. M., and Wang, J. Q. (2017). An essential role of Fyn in the modulation of metabotropic glutamate receptor 1 in neurons. eNeuro 4, ENEURO.96–ENEURO.17. doi: 10.1523/ENEURO.0096-17.2017
Jope, R. S., Song, L., Grimes, C. A., and Zhang, L. (1999). Oxidative stress oppositely modulates protein tyrosine phosphorylation stimulated by muscarinic G protein-coupled and epidermal growth factor receptors. J. Neurosci. Res. 55, 329–340. doi: 10.1002/(SICI)1097-4547(19990201)55:3<329::AID-JNR8>3.0.CO;2-K
Kalia, L. V., and Salter, M. W. (2003). Interactions between Src family protein tyrosine kinases and PSD-95. Neuropharmacology 45, 720–728.
Kalia, L. V., Gingrich, J. R., and Salter, M. W. (2004). Src in synaptic transmission and plasticity. Oncogene 23, 8007–8016.
Leist, M., Rinne, S., Datunashvili, M., Aissaoui, A., Pape, H. C., Decher, N., et al. (2017). Acetylcholine-dependent upregulation of TASK-1 channels in thalamic interneurons by a smooth muscle-like signaling pathway. J. Physiol. 595, 5875–5893. doi: 10.1113/JP274527
Levey, A. I. (1996). Muscarinic acetylcholine receptor expression in memory circuits: Implications for treatment of Alzheimer disease. Proc. Natl. Acad. Sci. U.S.A. 93, 13541–13546. doi: 10.1073/pnas.93.24.13541
Levey, A. I., Kitt, C. A., Simonds, W. F., Price, D. L., and Brann, M. R. (1991). Identification and localization of muscarinic acetylcholine receptor proteins in brain with subtype-specific antibodies. J. Neurosci. 11, 3218–3226.
Linseman, D. A., Heidenreich, K. A., and Fisher, S. K. (2001). Stimulation of M3 muscarinic receptors induced phosphorylation of the Cdc42 effector activated Cdc42Hs-associated kinase-1 via a Fyn tyrosine kinase signaling pathway. J. Biol. Chem. 276, 5622–5628. doi: 10.1074/jbc.M006812200
Lu, W. Y., Xiong, Z. G., Lei, S., Orser, B. A., Dudek, E., Browning, M. D., et al. (1999). G-protein-coupled receptors act via protein kinase C and Src to regulate NMDA receptors. Nat. Neurosci. 2, 331–338. doi: 10.1038/7243
Ma, X. H., Zhong, P., Gu, Z., Feng, J., and Yan, Z. (2003). Muscarinic potentiation of GABA(A) receptor currents is gated by insulin signaling in the prefrontal cortex. J. Neurosci. 23, 1159–1168. doi: 10.1523/JNEUROSCI.23-04-01159.2003
Mahavadi, S., Huang, J., Sriwai, W., Rao, K. R., and Murthy, K. S. (2007). Cross-regulation of VPAC2 receptor internalization by m2 receptors via c-Src-mediated phosphorylation of GRK2. Regul. Pept. 139, 109–114. doi: 10.1016/j.regpep.2006.10.013
Man, J., Cui, K., Fu, X., Zhang, D., Lu, Z., Gao, Y., et al. (2020). Donepezil promotes neurogenesis via Src signaling pathway in a rat model of chronic cerebral hypoperfusion. Brain Res. 1736, 146782. doi: 10.1016/j.brainres.2020.146782
Mao, L. M., and Wang, J. Q. (2015). Dopaminergic and cholinergic regulation of Fyn tyrosine kinase phosphorylation in the rat striatum in vivo. Neuropharmacology 99, 491–499. doi: 10.1016/j.neuropharm.2015.08.017
Mao, L. M., and Wang, J. Q. (2016a). Dopamine D2 receptors are involved in the regulation of Fyn and metabotropic glutamate receptor 5 phosphorylation in the rat striatum in vivo. J. Neurosci. Res. 94, 329–338. doi: 10.1002/jnr.23713
Mao, L. M., and Wang, J. Q. (2016b). Tyrosine phosphorylation of glutamate receptors by non-receptor tyrosine kinases: Roles in depression-like behavior. Neurotransmitter 3:e1118.
Mao, L. M., Faris, H. J., and Wang, J. Q. (2018). Muscarinic acetylcholine receptors inhibit Fyn activity in the rat striatum in vivo. J. Mol. Neurosci. 64, 523–532. doi: 10.1007/s12031-018-1053-y
Mao, L. M., Geosling, R., Penman, B., and Wang, J. Q. (2017). Local substrates of non-receptor tyrosine kinases at synaptic sites in neurons. Sheng Li Xue Bao 69, 657–665.
Matrone, C., Petrillo, F., Nasso, R., and Ferretti, G. (2020). Fyn tyrosine kinase as harmonizing factor in neuronal functions and dysfunctions. Int. J. Mol. Sci. 21:4444. doi: 10.3390/ijms21124444
Matsuoka, H., and Inoue, M. (2017). Molecular mechanism for muscarinic M1 receptor-mediated endocytosis of TWIK-related acid-sensitive K+ 1 channels in rat adrenal medullary cells. J. Physiol. 595, 6851–6867. doi: 10.1113/JP275039
Matsuoka, H., Harada, K., Mashima, K., and Inoue, M. (2020). Muscarinic receptors stimulation induces TASK1 channel endocytosis through a PKC-Pyk2-Src pathway in PC12 cells. Cell Signal. 65:109434. doi: 10.1016/j.cellsig.2019.109434
Murthy, K. S. (2008). Inhibitory phosphorylation of soluble guanylyl cyclase by muscarinic m2 receptors via Gbetagamma-dependent activation of c-Src kinase. J. Pharmacol. Exp. Ther. 325, 183–189. doi: 10.1124/jpet.107.132928
Nagao, M., Yamauchi, J., Kaziro, Y., and Itoh, H. (1998). Involvement of protein kinase C and Src family tyrosine kinase in Galphaq/11-induced activation of c-Jun N-terminal kinase and p38 mitogen-activated protein kinase. J. Biol. Chem. 273, 22892–22898. doi: 10.1074/jbc.273.36.22892
Nakamura, T., Yamashita, H., Takahashi, T., and Nakamura, S. (2001). Activated Fyn phosphorylates alpha-synuclein at tyrosine residue 125. Biochem. Biophys. Res. Commun. 280, 1085–1092. doi: 10.1006/bbrc.2000.4253
Neet, K., and Hunter, T. (1996). Vertebrate non-receptor protein-tyrosine kinase families. Genes Cell 1, 147–169.
Ohnishi, H., Murata, Y., Okazawa, H., and Matozaki, T. (2011). Src family kinases: Modulators of neurotransmitter receptor function and behavior. Trends Neurosci. 34, 629–637.
Omri, B., Crisanti, P., Marty, M. C., Alliot, F., Fagard, R., Molina, T., et al. (1996). The Lck tyrosine kinase is expressed in brain neurons. J. Neurochem. 67, 1360–1364.
Onofri, F., Giovedi, S., Vaccaro, P., Czernik, A. J., Valtorta, F., De Camilli, P., et al. (1997). Synapsin I interacts with c-Src and stimulates its tyrosine kinase activity. Proc. Natl. Acad. Sci. U.S.A. 94, 12168–12173. doi: 10.1073/pnas.94.22.12168
Pakharukova, N., Masoudi, A., Pani, B., Staus, D. P., and Lefkowitz, R. J. (2020). Allosteric activation of proto-oncogene kinase Src by GPCR-beta-arrestin complex. J. Biol. Chem. 295, 16773–16784.
Pascoli, V., Besnard, A., Herve, D., Pages, C., Heck, N., Girault, J. A., et al. (2011). Cyclic adenosine monophosphate-independent tyrosine phosphorylation of NR2B mediates cocaine-induced extracellular signal-regulated kinase activation. Biol. Psychiatry 69, 218–227. doi: 10.1016/j.biopsych.2010.08.031
Perez, I., Berndt, S., Agarwal, R., Castro, M. A., Vishnivetskiy, S. A., Smith, J. C., et al. (2022). A model for the signal initiation complex between arrestin-3 and the Src family kinase Fgr. J. Mol. Biol. 434:167400. doi: 10.1016/j.jmb.2021.167400
Portugal, C. C., Almeida, T. O., Socodato, R., and Relvas, J. B. (2022). Src family kinases (SFKs): Critical regulators of microglial homeostatic functions and neurodegeneration in Parkinson’s and Alzheimer’s diseases. FEBS J. 289, 7760–7775. doi: 10.1111/febs.16197
Pronin, A. N., Wang, Q., and Slepak, V. Z. (2017). Teaching an old drug new tricks: Agonism, antagonism, and biased signaling of pilocarpine through M3 muscarinic acetylcholine receptor. Mol. Pharmacol. 92, 601–612. doi: 10.1124/mol.117.109678
Rajani, V., Sengar, A. S., and Salter, M. W. (2021). Src and Fyn regulation of NMDA receptors in health and disease. Neuropharmacology 193:108615. doi: 10.1016/j.neuropharm.2021.108615
Rosenblum, K., Futter, M., Jones, M., Hulme, E. C., and Bliss, T. V. P. (2000). ERKI/II regulation by the muscarinic acetylcholine receptors in neurons. J. Neurosci. 20, 977–985. doi: 10.1523/JNEUROSCI.20-03-00977.2000
Roskoski, R. Jr. (2005). Src kinase regulation by phosphorylation and dephosphorylation. Biochem. Biophys. Res. Commun. 331, 1–14.
Sancho, V., Nuche-Berenguer, B., and Jensen, R. T. (2012). The Src kinase Yes is activated in pancreatic acinar cells by gastrointestinal hormones/neurotransmitters, but not pancreatic growth factors, which stimulate its association with numerous other signaling molecules. Biochim. Biophys. Acta 1823, 1285–1294. doi: 10.1016/j.bbamcr.2012.05.015
Santiago, M. P., and Potter, L. T. (2001). Biotinylated m4-toxin demonstrates more M4 muscarinic receptor protein on direct than indirect striatal projection neurons. Brain Res. 894, 12–20. doi: 10.1016/s0006-8993(00)03170-x
Schenone, S., Brullo, C., Musumeci, F., Biava, M., Falchi, F., and Botta, M. (2011). Fyn kinase in brain diseases and cancer: The search for inhibitors. Curr. Med. Chem. 18, 2921–2942. doi: 10.2174/092986711796150531
Singer, C. A., Vang, S., and Gerthoffer, W. T. (2002). Coupling of M(2) muscarinic receptors to Src activation in cultured canine colonic smooth muscle cells. Am. J. Physiol. 282, G61–G68. doi: 10.1152/ajpgi.00100.2002
Slack, B. E. (2000). The m3 muscarinic acetylcholine receptor is coupled to mitogen-activated protein kinase via protein kinase C and epidermal growth factor receptor kinase. Biochem. J. 348, 381–387.
Stirnweiss, J., Valkova, C., Ziesche, E., Drube, S., and Liebmann, C. (2006). Muscarinic M2 receptors mediate transactivation of EGF receptor through Fyn kinase and without matrix metalloproteases. Cell Signal. 18, 1338–1349. doi: 10.1016/j.cellsig.2005.10.018
Teber, I., Kohling, R., Speckmann, E. J., Barnekow, A., and Kremerskothen, J. (2004). Muscarinic acetylcholine receptor stimulation induces expression of the activity-regulated cytoskeleton-associated gene (ARC). Mol. Brain Res. 121, 131–136.
Tian, M., Xu, J., Lei, G., Lombroso, P. J., Jackson, M. F., and MacDonald, J. F. (2016). STEP activation by Gαq coupled GPCRs opposes Src regulation of NMDA receptors containing the GluN2A subunit. Sci. Rep. 6:36684.
Vazquez, G., Wedel, B. J., Kawasaki, B. T., Bird, G. S., and Putney, J. W. Jr. (2004). Obligatory role of Src kinase in the signaling mechanism for TRPC3 cation channels. J. Biol. Chem. 279, 40521–40528. doi: 10.1074/jbc.M405280200
Wan, Y., Kurosaki, T., and Huang, X. Y. (1996). Tyrosine kinases in activation of the MAP kinase cascade by G-protein-coupled receptors. Nature 380, 541–544. doi: 10.1038/380541a0
Wang, J. Q., Derges, J. D., Bodepudi, A., Pokala, N., and Mao, L. M. (2022). Roles of non-receptor tyrosine kinases in pathogenesis and treatment of depression. J. Integr. Neurosci. 21:25.
Wang, J., Fu, X., Zhang, D., Yu, L., Li, N., Lu, Z., et al. (2017). ChAT-positive neurons participate in subventricular zone neurogenesis after middle cerebral artery occlusion in mice. Behav. Brain Res. 316, 145–151.
Watcharasit, P., Tucholski, J., and Jope, R. S. (2001). Src family kinase involvement in muscarinic receptor-induced tyrosine phosphorylation in differentiated SH-SY5Y cells. Neurochem. Res. 26, 809–816. doi: 10.1023/a:1011612118779
Wess, J. (1996). Molecular biology of muscarinic acetylcholine receptors. Crit. Rev. Neurobiol. 10, 69–99.
Yang, H. B., Yang, X., Cao, J., Li, S., Liu, Y. N., Suo, Z. W., et al. (2011). cAMP-dependent protein kinase activated Fyn in spinal dorsal horn to regulate NMDA receptor function during inflammatory pain. J. Neurochem. 116, 93–104. doi: 10.1111/j.1471-4159.2010.07088.x
Yang, K., Jackson, M. F., and McDonald, J. F. (2014). Recent progress in understanding subtype specific regulation of NMDA receptors by G protein-coupled receptors (GPCRs). Int. J. Mol. Sci. 15, 3003–3024. doi: 10.3390/ijms15023003
Yang, K., Trepanier, C., Sidhu, B., Xie, Y. F., Li, H., Lei, G., et al. (2012). Metaplasticity gated through differential regulation of GluN2A versus GluN2B receptors by Src family kinases. EMBO J. 31, 805–816. doi: 10.1038/emboj.2011.453
Yeo, M. G., Oh, H. J., Cho, H. S., Chun, J. S., Marcantonio, E. E., and Song, W. K. (2011). Phosphorylation of Ser 21 in Fyn regulates its kinase activity, focal adhesion targeting, and is required for cell migration. J. Cell. Physiol. 226, 236–247. doi: 10.1002/jcp.22335
Zhao, W. Q., Alkon, D. L., and Ma, W. (2003). c-Src protein tyrosine kinase activity is required for muscarinic receptor-mediated DNA synthesis and neurogenesis via ERK1/2 and c-AMP-responsive element-binding protein signaling in neural precursor cells. J. Neurosci. Res. 72, 334–342. doi: 10.1002/jnr.10591
Keywords: Src, Fyn, tyrosine kinase, M1 receptor, M4 receptor, striatum
Citation: Mao L-M, Young L, Chu X-P and Wang JQ (2024) Regulation of Src family kinases by muscarinic acetylcholine receptors in heterologous cells and neurons. Front. Mol. Neurosci. 16:1340725. doi: 10.3389/fnmol.2023.1340725
Received: 21 November 2023; Accepted: 27 December 2023;
Published: 11 January 2024.
Edited by:
Manel M. Santafe, University of Rovira i Virgili, SpainReviewed by:
George Leondaritis, University of Ioannina, GreeceCopyright © 2024 Mao, Young, Chu and Wang. This is an open-access article distributed under the terms of the Creative Commons Attribution License (CC BY). The use, distribution or reproduction in other forums is permitted, provided the original author(s) and the copyright owner(s) are credited and that the original publication in this journal is cited, in accordance with accepted academic practice. No use, distribution or reproduction is permitted which does not comply with these terms.
*Correspondence: John Q. Wang, d2FuZ2pxQHVta2MuZWR1
Disclaimer: All claims expressed in this article are solely those of the authors and do not necessarily represent those of their affiliated organizations, or those of the publisher, the editors and the reviewers. Any product that may be evaluated in this article or claim that may be made by its manufacturer is not guaranteed or endorsed by the publisher.
Research integrity at Frontiers
Learn more about the work of our research integrity team to safeguard the quality of each article we publish.