- 1Department of Medicine, Weill Cornell Graduate School of Medical Sciences, Weill Cornell Medicine, New York, NY, United States
- 2Department of Biochemistry, Albert Einstein College of Medicine, Bronx, NY, United States
- 3Department of Psychiatry, Weill Cornell Medicine, New York, NY, United States
Despite structural similarity with other tumor necrosis factor receptor superfamily (TNFRSF) members, the p75 neurotrophin receptor (p75NTR, TNFR16) mediates pleiotropic biological functions not shared with other TNFRs. The high level of p75NTR expression in the nervous system instead of immune cells, its utilization of co-receptors, and its interaction with soluble dimeric, rather than soluble or cell-tethered trimeric ligands are all characteristics which distinguish it from most other TNFRs. Here, we compare these attributes to other members of the TNFR superfamily. In addition, we describe the recent evolutionary adaptation in B7-1 (CD80), an immunoglobulin (Ig) superfamily member, which allows engagement to neuronally-expressed p75NTR. B7-1-mediated binding to p75NTR occurs in humans and other primates, but not lower mammals due to specific sequence changes that evolved recently in primate B7-1. This discovery highlights an additional mechanism by which p75NTR can respond to inflammatory cues and trigger synaptic elimination in the brain through engagement of B7-1, which was considered to be immune-restricted. These observations suggest p75NTR does share commonality with other immune co-modulatory TNFR family members, by responding to immunoregulatory cues. The evolution of primate B7-1 to bind and elicit p75NTR-mediated effects on neuronal morphology and function are discussed in relationship to immune-driven modulation of synaptic actions during injury or inflammation.
Introduction
The p75 receptor (p75NTR) is classically defined as a neurotrophin receptor, activated by soluble, dimeric neurotrophin ligands to mediate a diverse range of functions. These include critical roles during development, such as patterning, survival and pruning of peripheral sympathetic and sensory neurons, and cell cycle regulation in the central nervous system. In the context of injury, aging or inflammation, p75NTR can induce neuronal and glial apoptosis, and negative remodeling of synapses. Engagement of p75NTR by secreted dimeric proneurotrophin ligands mediates apoptosis, long term synaptic depression and acute synaptic remodeling, all of which also require coexpression of sortilin family receptors (sortilin or SorCS2). Collectively these activities are distinct from the proinflammatory signaling associated with TNF:TNFR interactions typically occurring between antigen presenting cells and T-cells, although p75NTR is expressed at low levels in lymphoid tissues and specifically by plasmacytoid dendritic cells (Bandola et al., 2017) and reviewed in Minnone et al. (2017). A small subset of TNFRSF members, including p75NTR, DR6 (TNFRS21), and TROY (TNFRSF19) are distinct from other TNFRSF members as they recognize non-TNF-like ligands. HVEM (herpesvirus entry mediator) recognizes both conventional and non-conventional TNF-like ligands (Shrestha et al., 2020). These non-conventional TNFRs are all highly expressed in the nervous system, have atypical ligands, and mediate effects on neurite growth, survival and migration, but have not been considered mediators of neuroimmune signaling (Liu et al., 2019; Ding et al., 2020). However, recent identification of the human immune co-stimulatory protein, B7-1 (CD80), as a ligand of p75NTR provides another mechanism by which immune cells, specifically activated microglia, can mediate acute and deleterious actions on cells of the central nervous system (CNS) in the settings of injury, inflammation, and neurodegeneration. Here, we describe the functions of p75NTR that distinguish it from other members of the TNFRSF and consider the potential impact of human B7-1 as a pharmacological target in neuro-immune mediated synapse elimination and dysfunction.
TNFR superfamily and p75NTR
In the 1980s, tumor necrosis factors (TNFα, LTα) were identified, followed closely by the discovery of their TNFR1 and TNFR2 receptors (Aggarwal et al., 1985; Shirai et al., 1985; Loetscher et al., 1990; Schall et al., 1990; Smith et al., 1990). During the same period, p75 nerve growth factor receptor (p75NTR, TNFR16) was identified on the basis of binding to nerve growth factor (NGF) (Chao et al., 1986; Radeke et al., 1987). The TNFRSF now contains 29 members in humans, and is evolutionarily ancient, with TNFRSF members identified in Chordata, Arthropoda, and Cnidaria (Quistad et al., 2014; Quistad and Traylor-Knowles, 2016).
The TNFRs are type-I transmembrane proteins with extracellular ligand-binding cysteine-rich domains (CRD), a transmembrane domain and a cytoplasmic domain containing structural motifs that recruit signaling proteins (Figure 1). TNF ligands are type-II transmembrane proteins that form compact trimeric structures and mediate clustering of TNFRs into 3:3 receptor:ligand assemblies. All TNFRs, except the three unconventional receptors and HVEM, bind at least one TNF ligand, with each TNFR monomer binding the interface between TNF trimer subunits. Despite the high degree of structural similarity, the sequence homology between family members is limited, with TNFR1 and TNFR2 only sharing ∼50% homology, despite recognizing the same TNF ligands. This degree of diversity underlies the selectivity between TNF:TNFR pairs, the tuning of receptor affinities to shared ligands, differences in cell surface organization, and differences in signaling molecule recruitment. Conventional TNFR superfamily members are widely and differentially expressed across many tissues, including immune cells (T-cells, B-cells or NK-cells). TNF superfamily ligands are typically expressed by antigen presenting cells such as macrophages, monocytes and dendritic cells. Within the context of immune regulation, these interactions provide critical secondary signals that sustain T-cell activation post peptide major histocompatibility complex/T cell receptor (post pMHC/TCR) engagement and promote inflammatory responses. Their central role in immunity and inflammatory diseases has made them important targets for the development of therapeutic interventions (i.e., anti-TNF monoclonal antibodies, Fas-L monoclonal antibodies).
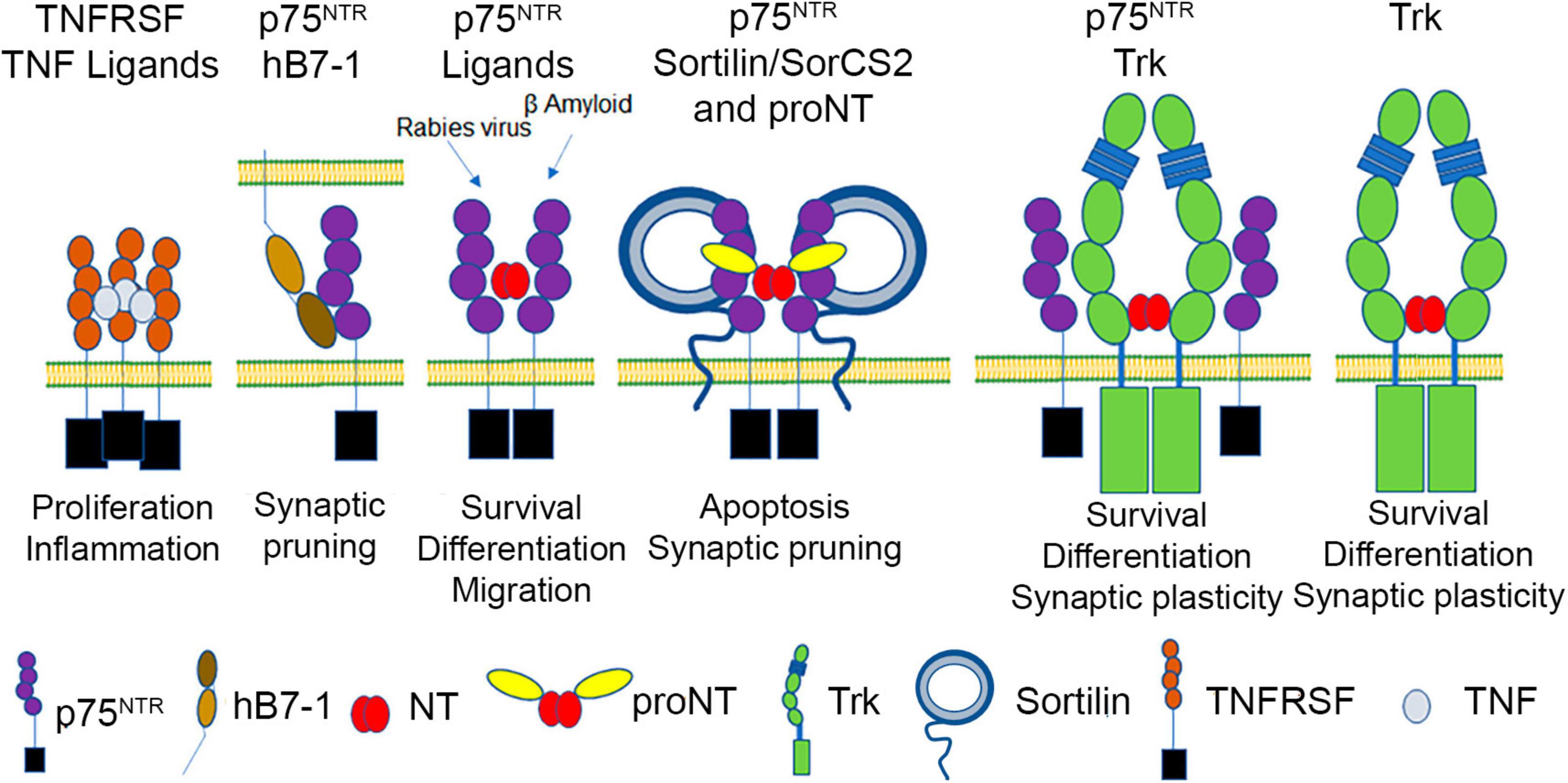
Figure 1. p75NTR, a TNFRSF member, binds to mature neurotrophins (NT), proneurotrophins (proNT) (using sortilin/SorCS2 as a co-receptor), human B7-1, β-amyloid, and rabies virus glycoprotein, and can interact with Trk receptors, which independently bind mature neurotrophins.
Broadly the TNFR family can be divided into three groups; (1) death receptors (2) TRAF-motif containing receptors or (3) decoy receptors. Death receptors contain a death domain (DD) that recruits the signaling adapter protein TRADD leading to NFκB activation and, after downstream caspase activation, apoptosis and inflammation. TNFR1 represents a primary example of a canonical death receptor and its balancing of cellular life and death signals has been extensively studied and reviewed (Dostert et al., 2019; Wajant and Siegmund, 2019; Kucka and Wajant, 2020; Vanamee and Faustman, 2020). The TRAF-motif containing receptors, such as TNFR2, do not contain a death domain and instead bind TRAFs directly, resulting in signaling that is unique but significantly overlaps and competes with that of death receptors (Xie, 2013). Decoy receptors are a small group of TNFRs that contain no signaling modality and act to sequester specific ligands away from other family members.
The three unconventional TNFRSF members, TROY, DR6, and p75NTR, possess all of the structural hallmarks of conventional TNFRSF members, but exhibit distinct functional attributes. Firstly, excluding anomalous expression by cancerous tissues, these receptors are most highly expressed by neurons and glia, rather than by immune cells. Additionally, none of these receptors bind a trimeric TNF ligand and are thus considered “outliers.” TROY was identified as a co-receptor for NOGO-receptor-1 (NgR1), which along with LINGO forms a heterotrimeric complex that triggers neurite growth, survival and migration (Park et al., 2005; Shao et al., 2005). DR6 has been reported to bind the amyloid precursor protein (APP) but interactions with other ligands are unknown, despite a role in animal models of multiple sclerosis (Ren et al., 2022). However, these unconventional receptors are largely restricted to the nervous system, suggesting they have diverged from other TNFR members to participate in neuronal patterning and degeneration.
Compared to TROY and DR6, p75NTR function is better characterized. Based on primary sequence, p75NTR is highly conserved among vertebrate species (murine p75NTR is 92% homologous to human p75NTR) and encodes a death domain (Chao et al., 1986; Johnson et al., 1986; Radeke et al., 1987; Lin et al., 2015). The extracellular domain of p75NTR consists of four CRD domains, each of which has three intradomain disulfide bonds (Yan and Chao, 1991; Baldwin et al., 1992; Figure 1). Structural and biochemical studies show that mature neurotrophins interact with two sites of the p75NTR receptor; site 1, consisting of residues within CRD1 and 2, and site 2, consisting of residues within CRD 3 and 4 (Yan and Chao, 1991; Baldwin et al., 1992; Chapman and Kuntz, 1995; Shamovsky et al., 1999; Gong et al., 2008). The 21 aa transmembrane segment is followed by an intracellular domain containing an intrinsically disordered juxtamembrane domain, a 29 aa Chopper sequence required for apoptotic signaling (Coulson et al., 2000) and a C-terminal death domain, which shares some features with canonical TNFR death domains, but does not self-associate (Liepinsh et al., 1997; Lin et al., 2015). Signaling cascades associated with other TNFRs are also employed in p75NTR-mediated signaling. Specifically, p75NTR utilizes TRAF6, RIP2 and the IKK complex to facilitate NFκB activation and inflammatory signaling (Ye et al., 1999; Charalampopoulos et al., 2012). In addition, p75NTR can utilize MAGE homologs (NRAGE) and TRADD, to promote apoptosis through JNK activation (Hempstead, 2002; Salehi et al., 2002; Gentry et al., 2004). Activation of the p75NTR also induces rapid reorganization of the actin cytoskeleton utilizing Rac, Rho, Trio (a guanine exchange factor) as well as MEK and MAPK (Yamashita et al., 1999; Lad and Neet, 2003; Gehler et al., 2004; Domeniconi et al., 2005; Deinhardt et al., 2011; Anastasia et al., 2013), which further distinguishes this receptor from other TNFRSF members.
In contrast to the expression of canonical TNFRSF members on immune cells, p75NTR is expressed in the peripheral and central nervous systems during development and becomes regionally restricted in the adult brain to the subiculum, basal forebrain and cerebellum, and to peripheral sensory and sympathetic neurons (Cheng et al., 2018). However, following injury, inflammation or with aging, p75NTR expression is rapidly induced (Dechant and Barde, 2002; Ibanez and Simi, 2012; Wong et al., 2021).
The best characterized p75NTR ligands are members of the neurotrophin family (Figure 1). Neurotrophins are initially synthesized as precursor forms, which possess an intrinsically disordered prodomain and a structured domain. Dimerization is promoted by mature domain interactions, and cleavage of the prodomain results in dimeric mature domains of NGF, BDNF, NT-3 or NT-4 which are secreted as cysteine knot proteins (Heymach and Shooter, 1995) [reviewed in Sun and Davies (1995)]. In the absence of prodomain cleavage, proneurotrophins, including proNGF, proBDNF and proNT-3, can also be secreted as dimers (Lee et al., 2001). The mature domain of proneurotrophins interact with p75NTR utilizing site 1 and site 2 as described above, although there are modest differences in the specific contacts made between p75NTR and mature NGF versus proNGF (He and Garcia, 2004; Feng et al., 2010).
Neurotrophins are secreted by numerous cell types to regulate the targeting of peripheral innervation during development, and mediate survival effects by binding to the Trk family of receptor tyrosine kinases that are unrelated to p75NTR (Figure 1). The Trk receptor tyrosine kinases (Huang and Reichardt, 2003) are type-I membrane proteins activated by mature neurotrophins (Wehrman et al., 2007) independently of p75NTR; however, p75NTR activation by neurotrophins also plays important roles in sensory innervation and cerebellar development (Chen et al., 2017; Zanin et al., 2019; Zanin and Friedman, 2022). BDNF and NT-3 are also secreted by central neurons in an activity-dependent manner, with BDNF and NT-3 modulating synaptic plasticity utilizing TrkB and TrkC, respectively (Park and Poo, 2013; Chen et al., 2017). Proneurotrophins, including proNGF, proBDNF and proNT-3, utilize the mature domain to bind p75NTR, while using their prodomain to engage members of the sortilin family of type I transmembrane proteins (sortilin, SorCS2, SorCS3) (Nykjaer et al., 2004; Westergaard et al., 2005; Feng et al., 2010; Glerup et al., 2014). The binding of proNGF to p75NTR and a sortilin family member can induce cell death (Nykjaer et al., 2004) and proBDNF can mediate synaptic depression utilizing p75NTR and a sortilin family member (Woo et al., 2005; Yang et al., 2014). Amyloid-β oligomers have also been described as p75NTR ligands that induce cell death or synaptic remodeling (Yaar et al., 1997; Knowles et al., 2009; Patnaik et al., 2020). However, little is known about the specific p75NTR surfaces responsible for amyloid-β engagement and the multimerization of p75NTR required to elicit these effects. p75NTR also interacts with Ephrin A and the Nogo receptor complex (Wong et al., 2002; Lim et al., 2008). Lastly, the trimeric rabies virus glycoprotein was reported as a ligand for p75NTR, which may increase the rate of viral axonal transport; however, the specific mechanism has not been described (Tuffereau et al., 2007; Gluska et al., 2014).
In the unliganded state, p75NTR monomers, dimers and trimers were identified on the cell surface using crosslinking, FRET and super resolution microscopy, as well as structure:function analyses involving p75NTR mutants expressed in mice (Vilar et al., 2009; Anastasia et al., 2015; Marchetti et al., 2019). While there is consensus that monomers, dimers and trimers exist in dynamic equilibrium, the mechanisms that regulate p75NTR multimerization and recruitment of co-receptors are areas of active investigation. Indeed, p75NTR is distinct from most TNFR superfamily members, which form homomeric complexes, as it forms both homomeric complexes and heteromeric complexes with TrkA or Sortilin family members. The interaction of TrkA with p75NTR enhances its affinity for NGF. Structure function analysis confirmed that interactions between the transmembrane and intracellular domains of p75NTR and TrkA mediate allosteric changes that drive the increase in NGF affinity and that direct contact between NGF and p75NTR is not required (Hempstead et al., 1991; Esposito et al., 2001; Franco et al., 2021). In contrast, the formation of a heteromeric complex between p75NTR and a sortilin family member, which is required for proneurotrophin-induced activation, requires the direct binding of the proneurotrophin prodomain to a sortilin family member, and the mature domain to p75NTR (Feng et al., 2010). One study suggests that mature NGF interacts with SorCS2; however, the affinity of this interaction is much weaker than that involving proNGF, and the biological effects of the mature NGF:SorCS2 interaction are not established (Leloup et al., 2018).
Human B7.1:p75NTR, a newly identified protein-protein interaction
Using an unbiased cell-based, protein-protein interaction screen of immunoglobulin superfamily (IgSF) members and TNFRSF members, the interaction between human B7-1 and p75NTR was identified (Morano et al., 2022). B7-1, also known as Cluster of Differentiation 80 (CD80), is a member of the B7 family of type-I transmembrane glycoproteins within the IgSF, which includes B7-2 and eight other homologous proteins (Liu and Zang, 2019; Ye et al., 2019). The B7-1 extracellular domain consists of an N-terminal Ig-V domain and a membrane proximal Ig-C domain, followed by a transmembrane domain (21 aa), and a short cytoplasmic tail (25 aa) (Bhatia et al., 2005, 2010). B7-1 shares ∼25% sequence identity with B7-2 and both are located on chromosome 3 (3q13.3-q2.1), likely evolving from a gene duplication event (Hansen et al., 2009). Both B7 proteins are expressed by antigen presenting cells (APC), including macrophages, monocytes, and dendritic cells (DC). Basal expression of B7-1 by APCs is low but rapidly induced in response to a number of proinflammatory cytokines, as described in more detail below.
Engagement of constitutively expressed CD28 on T-cells with B7 drives T-cell proliferation, cytokine secretion, and prevents the induction of T-cell anergy (Satoh et al., 1995; Rawat and Spector, 2017). In contrast, CTLA-4 is induced upon T-cell activation and competes with CD28 for B7 ligand binding, attenuating T-cell activation and preventing unchecked T-cell expansion (De Simone et al., 1995; Windhagen et al., 1995; O’Keefe et al., 2002). As such, B7-1 is a critical contributor to the immunological synapse, the interface formed between antigen presenting cells and T-cells, and elicits both costimulatory signals by activating CD28, and co-inhibitory signals via CTLA-4. Growing evidence suggests that in addition to T-cell mediated effects, there is also reverse signaling into the APC upon receptor engagement, as CD28 binding to B7 ligands elicits increased secretion of IL-6 from dendritic cells, resulting in additional immunostimulatory activity (Orabona et al., 2004; Koorella et al., 2014). In contrast, CTLA-4-B7 engagement of B7 ligands upregulates IFNγ, which enhances IDO expression and leads to tryptophan catabolism and suppression of T-cell proliferation (Chao et al., 2006; Famenini et al., 2017). There is also evidence that the cytoplasmic tail of B7-1 may regulate the spatial organization of B7-1 on the cell surface and the localization of its receptors within the immunological synapse (Doty and Clark, 1998). Recently PD-L1, another B7-family member was shown to interact with B7-1 in cis, reducing the ability of PD-L1 to bind the PD-1 inhibitory receptor and preferentially promoting B7-1 engagement with CD28 and not CTLA-4 (Chaudhri et al., 2018; Sugiura et al., 2019; Garrett-Thomson et al., 2020).
Identification of p75NTR as an additional receptor for B7-1 expands the contributions of this pleiotropic ligand. Importantly, the interaction between B7-1 and p75NTR represents another example of crosstalk between the Ig and TNFR superfamilies. The first was the discovery that HVEM (TNFRSF16) interacts with two IgSF ligands, BTLA and CD160 in addition to its canonical TNF ligand, LIGHT (Sedy et al., 2005; Cai et al., 2008; Liu et al., 2021). Recently, ligand-selective HVEM mutants were designed to elucidate the in vivo contributions of each of these interactions, highlighting the myriad of complexities and promiscuities involved in tightly controlling the location, duration and intensity of immune responses to specific challenges (Liu et al., 2021). Similarly, the interaction between B7-1 and p75NTR suggests similar complexities and underscores a novel mechanism by which B7-1 can elicit neuroimmune regulatory effects.
In contrast to the highly conserved p75NTR, B7-1 is much less conserved, with human and murine B7-1 sharing only 44% sequence homology. This sequence divergence appears to be responsible for the lack of interaction between murine B7-1 and p75NTR orthologues, while the regions of p75NTR/CD28/CTLA4 that interact with the B7-1 Ig-V domain are sufficiently conserved to permit human B7-1 to recognize murine p75NTR/CD28/CTLA-4 (Morano et al., 2022). Alanine-scanning mutagenesis and competition binding experiments indicate that the binding surfaces for CTLA-4, CD28 and p75NTR on B7-1 overlap, though the interface with p75NTR is more extended. However, the divergence in amino acid sequence between rodent and primate B7-1 map to regions of B7-1 that mediate p75NTR binding, permitting binding of human and primate B7-1, but not rodent B7-1 to p75NTR. The restriction of the B7-1:p75NTR interaction to primates and cross-binding reactivity observed between human/primate B7-1 orthologues and human/mouse/rat p75NTR orthologues suggests that p75NTR binding is a property that has been recently acquired due to unique features present in human/primate B7-1.
Exposure of murine hippocampal neurons that express p75NTR to soluble or cell surface-presented human B7-1 alters dendritic morphology with loss of postsynaptic proteins and fragmentation of the dendritic cytoskeleton. Injection of human B7-1 into the dorsal subiculum of live mice, results in the rapid pruning of p75NTR-expressing dendritic spines (Morano et al., 2022). While these studies suggest that induction of B7-1 in activated microglia may act to negatively impact neuronal synapses, the finding that this behavior is restricted to human B7-1 will require the development of a humanized mouse model of B7-1 to better understand the pathophysiological roles of this interaction.
Immune:neuronal interactions which promote synaptic elimination
While synaptic elimination during development and in disease was initially described as a neuronal-intrinsic phenomenon, it is now recognized that antigen presenting cells, APCs (i.e., microglia), regulate neuronal structure and function, often in the context of inflammatory stimuli or neurodegenerative conditions [reviewed in Wilton et al. (2019)]. In response to injury or inflammatory signals, microglia extend processes to survey the surrounding environment and engulf neuronal synapses to regulate synaptic activity (Nimmerjahn et al., 2005), (Wake et al., 2009; Tremblay et al., 2010). The ligand:receptor complexes that regulate microglial:neuronal interactions are incompletely understood; however, as microglia function as mediators of innate immunity, induction of immune ligands on microglia and cognate receptors on synaptic structures would be anticipated (Figure 2). One of the first classes of mediators to be described are components of the complement fixation process, specifically C1q and C3, and the CR3 complement receptor expressed by activated microglia. In mouse models of Alzheimer’s Disease and frontotemporal dementia (FTD), C3 and C1q associate with synapses, and microglia recognize and engulf these synapses in a CR3 (complement receptor 3)-dependent manner (Hong et al., 2016). While C1q can “tag” synapses for degradation to mediate proper synaptic pruning during development (Stevens et al., 2007), the mechanisms utilized in neurodegenerative models are incompletely characterized. Recent reports suggest a role for neuronally expressed pentraxin (Zhou et al., 2023), and other candidates include the TREM2 receptor, which may protect against synaptic loss by associating with C1q (Zhong et al., 2023). While microglial products can promote a neuroinflammatory response, other microglial-expressed proteins may promote an anti-inflammatory and neuroprotective environment. For example, the neuronally-expressed proteins CD200 and fractalkine (Eyo and Wu, 2013) and CD200 receptor (CD200R) expressed on macrophages and microglia may induce CD200-CD200R signaling to maintain a resting or non-activated microglial state (Wright et al., 2001; Hernangómez et al., 2012). Defects in CD200 signaling promote microglial activation and are associated with neuro-inflammatory conditions such as multiple sclerosis (MS) and Alzheimer’s disease (AD) (Walker et al., 2009).
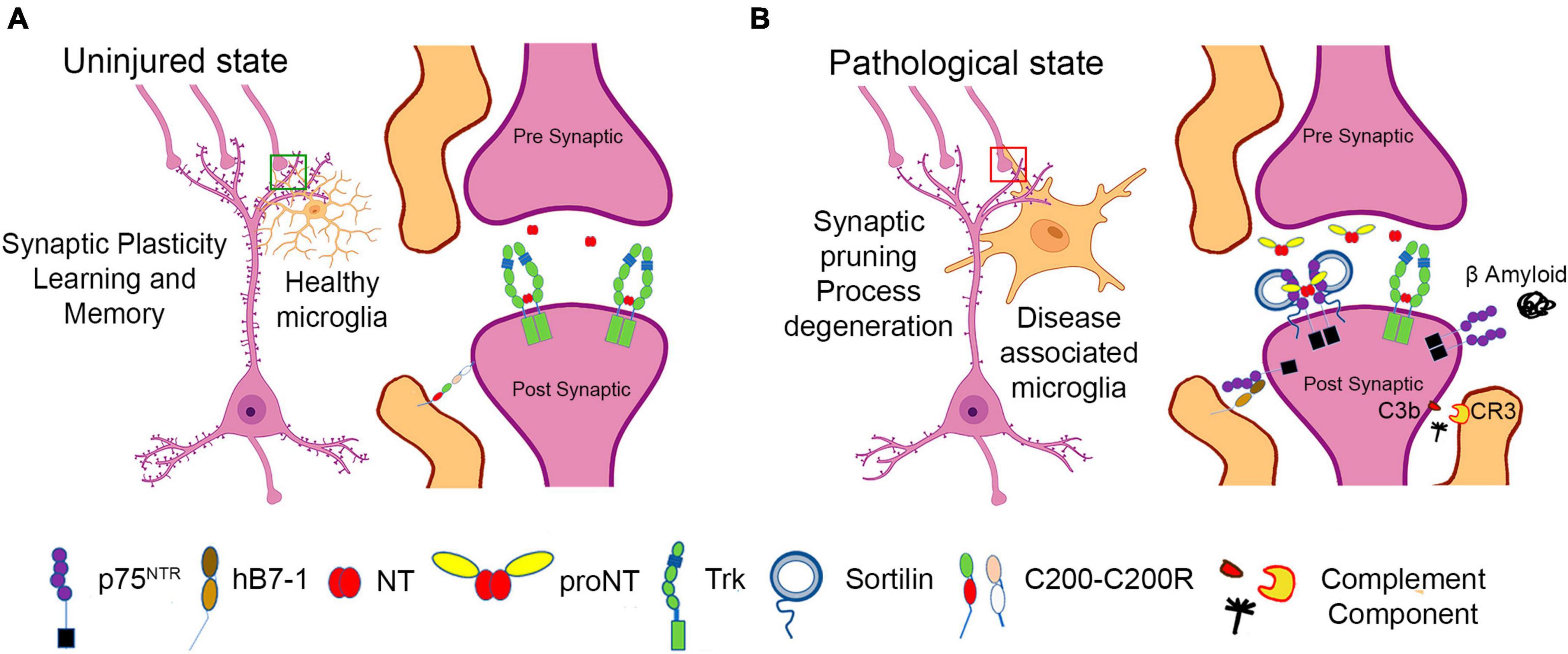
Figure 2. Schematic representation of neuronal:microglial interactions. (A) In the uninjured state, microglia support synaptic structure and function. (B) In the setting of inflammation, injury, and neurodegenerative states, disease associated microglia express numerous immunomodulatory factors, including B7-1, which may lead to negative effects on neuronal function, inducing synaptic pruning and process degeneration.
In humans, B7-1 may also be considered a mediator of synaptic elimination by targeting synapses that express p75NTR. B7-1 is minimally expressed by microglia from uninjured adult brain (Dangond et al., 1997). However, B7-1 expression is upregulated in the CNS in response to infection, and neurodegenerative diseases such as Multiple Sclerosis, and in Alzheimer’s Disease, specifically in microglia and immune cells that populate peri-venule cuffs (Williams et al., 1994; De Simone et al., 1995; Satoh et al., 1995, 2016; Dangond et al., 1997; Svenningsson et al., 1997; Magnus et al., 2005; Hussain et al., 2007; Durafourt et al., 2012; Busse et al., 2015; Peferoen et al., 2015; Fraussen et al., 2016). Recent RNAseq data from murine models and human tissue indicate that in the brain, B7-1 expression is restricted to microglia that also exhibit upregulation of genes associated with an activated phenotype observed in amyloid pathology (Olah et al., 2020). B7-1 is induced in numerous pathological settings, including microglia of MS lesions (Williams et al., 1994, De Simone et al., 1995), in human embryonic tissue in response to IFN-gamma (Satoh et al., 1995) and in B cells and monocytes from MS patients (Svenningsson et al., 1997). CNS-associated macrophages (CAMs) may also be a source of B7-1. These cells are found at the interface of the cortex with the meninges and choroid plexus (Prinz et al., 2021). CAMs and other immune cells, including T and B cells, monocytes, natural killer (NK) cells are found at the CNS borders, and readily cross the blood brain barrier in inflammatory states (Goldmann et al., 2016). Brain infiltrating macrophages are also a source of B7-1 in response to exposure to LPS and proinflammatory cytokines (Satoh et al., 1995; Awada et al., 2014), traumatic brain injury (Awada et al., 2014; Donat et al., 2017) and infections of the brain (Olson et al., 2001; Rawat and Spector, 2017). Further studies will be required to dissect the specific roles of macrophage, monocyte and microglia gene products in mediating synaptic elimination in the setting of inflammation or injury and in particular to define the contributions of B7-1.
The p75NTR receptor is also an established component of the inflammatory response in the brain. p75NTR expression is upregulated in the setting of traumatic brain and spinal cord injury (Shi et al., 2013; Tep et al., 2013), viral infection (Meeker et al., 2012), stroke (Kokaia et al., 1998), and in the setting of complex neurological disorders, including Alzheimer’s disease (Coulson, 2006) and experimental autoimmune encephalitis (Dechant and Barde, 2002; Delivanoglou et al., 2020). Induction of p75NTR occurs by both transcriptional and translational mechanisms (Ramos et al., 2007; Irmady et al., 2014) can adversely impact neuronal function. However, despite the similarities of induction of p75NTR and B7-1 in pathological states, additional studies are needed to evaluate if this results from common mechanisms.
Outstanding questions
Despite its identification nearly 40 years, there is still much to discover about p75NTR biology. Several characteristics distinguish p75NTR from canonical TNFRSF members. First, its ability to interact with receptor tyrosine kinases (for example TrkA) to alter the affinity and selectivity of ligands (Mischel et al., 2001) is unique amongst the TNFRSF members. In addition, its prominent neuroprotective activities during development, its direct activation of cytoskeletal dynamics to promote cell migration during development and migration and invasive phenotype of tumors that overexpress p75NTR are also atypical of TNFRSF members (Wislet et al., 2018).
The large number of ligands that bind to p75NTR suggests multiple independent mechanisms may contribute to its activation. Does p75NTR respond to multiple local ligands in the setting of neuroinflammation or injury? Is the induction of numerous ligands in the setting of injury or neurodegeneration a coordinated response to optimize p75NTR effects? Prior studies have established the coordinate induction of proneurotrophins and p75NTR following axotomy, traumatic brain injury, AD, and retinitis, resulting in synaptic elimination, neuronal degeneration and apoptosis. Based on these deleterious effects, many studies have evaluated approaches to blunt p75NTR activation using small molecules and antibody-mediated strategies (Shi et al., 2013). However, as proneurotrophin-mediated effects require both p75NTR and sortilin or SorCS2, approaches that limit binding to sortilin or SorCS2 have also been considered (Jakobsen et al., 2023).
The discovery that recent evolutionary changes in the human/primate B7-1 ectodomain permit binding and activation of p75NTR, raises many questions. Unlike proneurotrophins, which require that p75NTR is co-expressed with a sortilin family member, B7-1 does not interact with sortilin or SorCS2 (Morano et al., 2022). Furthermore, the potential effects of B7-1 sequestration or clearance from the surface of antigen presenting cells following interaction with p75NTR remains to be determined. Does this process limit B7-1 effects on its typical T-cell targets? Lastly, it is important to recognize that the high degree of species homology of both neurotrophins and p75NTR facilitates the translation of results from murine models to human pathological states. This opportunity is not available for B7-1, as murine models will fail to recapitulate the potential functions of human B7-1 in the diseased brain, and modeling of the effects of human B7-1 in mouse models of neurodegeneration will require the development of mice which express human B7-1. Nonetheless, the development of specific therapeutics that selectively impair activation of p75NTR by distinct ligands, including B7-1, while not modulating other critical interactions (i.e., its role as a costimulatory mediator of immune function) are challenging yet exciting prospects for the future.
Data availability statement
The original contributions presented in this study are included in this article/supplementary material, further inquiries can be directed to the corresponding authors.
Author contributions
VD: Writing – original draft, Writing – review & editing. SG-T: Writing – original draft, Writing – review & editing. SA: Writing – original draft, Writing – review & editing. FL: Writing – original draft, Writing – review & editing. BH: Writing – original draft, Writing – review & editing.
Funding
The authors declare financial support was received for the research, authorship, and/or publication of this article. This work was supported by the NIH grants 6R01HG008325 and 3R01CA198090 to SA, 5R01NS052819 and 3R01NS05281913 to FL and BH, and 1RF1AG078613 to BH, FL, and SA.
Acknowledgments
We acknowledge the Macromolecular Therapeutic Development Facility at Albert Einstein College of Medicine.
Conflict of interest
SG-T, VD, FL, BH, and SA were inventors on a patent application (PCT/US222/25730) that includes data regarding the interaction of human B7-1 with p75NTR. The intellectual property of the pending patent was held by Albert Einstein College of Medicine and Cornell University.
Publisher’s note
All claims expressed in this article are solely those of the authors and do not necessarily represent those of their affiliated organizations, or those of the publisher, the editors and the reviewers. Any product that may be evaluated in this article, or claim that may be made by its manufacturer, is not guaranteed or endorsed by the publisher.
References
Aggarwal, B. B., Eessalu, T. E., and Hass, P. E. (1985). Characterization of receptors for human tumour necrosis factor and their regulation by gamma-interferon. Nature 318, 665–667.
Anastasia, A., Barker, P. A., Chao, M. V., and Hempstead, B. L. (2015). Detection of p75NTR Trimers: Implications for receptor stoichiometry and activation. J. Neurosci. 35, 11911–11920. doi: 10.1523/JNEUROSCI.0591-15.2015
Anastasia, A., Deinhardt, K., Chao, M. V., Will, N. E., Irmady, K., Lee, F. S., et al. (2013). Val66Met polymorphism of BDNF alters prodomain structure to induce neuronal growth cone retraction. Nat. Commun. 4:2490. doi: 10.1038/ncomms3490
Awada, R., Saulnier-Blache, J. S., Gres, S., Bourdon, E., Rondeau, P., Parimisetty, A., et al. (2014). Autotaxin downregulates LPS-induced microglia activation and pro-inflammatory cytokines production. J. Cell Biochem. 115, 2123–2132. doi: 10.1002/jcb.24889
Baldwin, A. N., Bitler, C. M., Welcher, A. A., and Shooter, E. M. (1992). Studies on the structure and binding properties of the cysteine-rich domain of rat low affinity nerve growth factor receptor (p75NGFR). J. Biol. Chem. 267, 8352–8359.
Bandola, J., Richter, C., Ryser, M., Jamal, A., Ashton, M. P., von Bonin, M., et al. (2017). Neurotrophin receptor p75NTR regulates immune function of plasmacytoid dendritic cells. Front. Immunol. 8:981. doi: 10.3389/fimmu.2017.00981
Bhatia, S., Edidin, M., Almo, S. C., and Nathenson, S. G. (2005). Different cell surface oligomeric states of B7-1 and B7-2: Implications for signaling. Proc. Natl. Acad. Sci. U.S.A. 102, 15569–15574. doi: 10.1073/pnas.0507257102
Bhatia, S., Sun, K., Almo, S. C., Nathenson, S. G., and Hodes, R. J. (2010). Dynamic equilibrium of B7-1 dimers and monomers differentially affects immunological synapse formation and T cell activation in response to TCR/CD28 stimulation. J. Immunol. 184, 1821–1828. doi: 10.4049/jimmunol.0902869
Busse, S., Steiner, J., Alter, J., Dobrowolny, H., Mawrin, C., Bogerts, B., et al. (2015). Expression of HLA-DR, CD80, and CD86 in healthy aging and Alzheimer’s disease. J. Alzheimers Dis. 47, 177–184. doi: 10.3233/JAD-150217
Cai, G., Anumanthan, A., Brown, J. A., Greenfield, E. A., Zhu, B., and Freeman, G. J. (2008). CD160 inhibits activation of human CD4+ T cells through interaction with herpesvirus entry mediator. Nat. Immunol. 9, 176–185.
Chao, M. V., Bothwell, M. A., Ross, A. H., Koprowski, H., Lanahan, A. A., Buck, C. R., et al. (1986). Gene transfer and molecular cloning of the human NGF receptor. Science 232, 518–521.
Chao, M. V., Rajagopal, R., and Lee, F. S. (2006). Neurotrophin signalling in health and disease. Clin. Sci. 110, 167–173.
Chapman, B. S., and Kuntz, I. D. (1995). Modeled structure of the 75-kDa neurotrophin receptor. Protein Sci. 4, 1696–1707.
Charalampopoulos, I., Vicario, A., Pediaditakis, I., Gravanis, A., Simi, A., and Ibanez, C. F. (2012). Genetic dissection of neurotrophin signaling through the p75 neurotrophin receptor. Cell Rep. 2, 1563–1570.
Chaudhri, A., Xiao, Y., Klee, A. N., Wang, X., Zhu, B., and Freeman, G. J. (2018). PD-L1 binds to B7-1 only in Cis on the same cell surface. Cancer Immunol. Res. 6, 921–929. doi: 10.1158/2326-6066.CIR-17-0316
Chen, Z., Donnelly, C. R., Dominguez, B., Harada, Y., Lin, W., Halim, A. S., et al. (2017). p75 is required for the establishment of postnatal sensory neuron diversity by potentiating ret signaling. Cell Rep. 21, 707–720. doi: 10.1016/j.celrep.2017.09.037
Cheng, I., Jin, L., Rose, L. C., and Deppmann, C. D. (2018). Temporally restricted death and the role of p75NTR as a survival receptor in the developing sensory nervous system. Dev. Neurobiol. 78, 701–717. doi: 10.1002/dneu.22591
Coulson, E. J. (2006). Does the p75 neurotrophin receptor mediate Aβ-induced toxicity in Alzheimer’s disease? J. Neurochem. 98, 654–660.
Coulson, E. J., Reid, K., Baca, M., Shipham, K. A., Hulett, S. M., Kilpatrick, T. J., et al. (2000). Chopper, a new death domain of the p75 neurotrophin receptor that mediates rapid neuronal cell death. J. Biol. Chem. 275, 30537–30545. doi: 10.1074/jbc.M005214200
Dangond, F., Windhagen, A., Groves, C. J., and Hafler, D. A. (1997). Constitutive expression of costimulatory molecules by human microglia and its relevance to CNS autoimmunity. J. Neuroimmunol. 76, 132–138. doi: 10.1016/s0165-5728(97)00043-x
De Simone, R., Giampaolo, A., Giometto, B., Gallo, P., Levi, G., Peschle, C., et al. (1995). The costimulatory molecule B7 is expressed on human microglia in culture and in multiple sclerosis acute lesions. J. Neuropathol. Exp. Neurol. 54, 175–187. doi: 10.1097/00005072-199503000-00004
Dechant, G., and Barde, Y. A. (2002). The neurotrophin receptor p75(NTR): Novel functions and implications for diseases of the nervous system. Nat. Neurosci. 5, 1131–1136.
Deinhardt, K., Kim, T., Spellman, D. S., Mains, R. E., Eipper, B. A., Neubert, T. A., et al. (2011). Neuronal growth cone retraction relies on proneurotrophin receptor signaling through Rac. Sci. Signal. 4:ra82. doi: 10.1126/scisignal.2002060
Delivanoglou, N., Boziki, M., Theotokis, P., Kesidou, E., Touloumi, O., Dafi, N., et al. (2020). Spatio-temporal expression profile of NGF and the two-receptor system. TrkA and p75NTR, in experimental autoimmune encephalomyelitis. J. Neuroinflamm. 17:41. doi: 10.1186/s12974-020-1708-9
Ding, Z., Kloss, J. M., Tuncali, S., Tran, N. L., and Loftus, J. C. (2020). TROY signals through JAK1-STAT3 to promote glioblastoma cell migration and resistance. Neoplasia 22, 352–364. doi: 10.1016/j.neo.2020.06.005
Domeniconi, M., Zampieri, N., Spencer, T., Hilaire, M., Mellado, W., Chao, M. V., et al. (2005). MAG induces regulated intramembrane proteolysis of the p75 neurotrophin receptor to inhibit neurite outgrowth. Neuron 46, 849–855.
Donat, C. K., Scott, G., Gentleman, S. M., and Sastre, M. (2017). Microglial activation in traumatic brain injury. Front. Aging Neurosci. 9:208. doi: 10.3389/fnagi.2017.00208
Dostert, C., Grusdat, M., Letellier, E., and Brenner, D. (2019). The TNF family of ligands and receptors: Communication modules in the immune system and beyond. Physiol. Rev. 99, 115–160. doi: 10.1152/physrev.00045.2017
Doty, R. T., and Clark, E. A. (1998). Two regions in the CD80 cytoplasmic tail regulate CD80 redistribution and T cell costimulation. J. Immunol. 161, 2700–2707.
Durafourt, B. A., Moore, C. S., Zammit, D. A., Johnson, T. A., Zaguia, F., Guiot, M. C., et al. (2012). Comparison of polarization properties of human adult microglia and blood-derived macrophages. Glia 60, 717–727. doi: 10.1002/glia.22298
Esposito, D., Patel, P., Stephens, R. M., Perez, P., Chao, M. V., Kaplan, D. R., et al. (2001). The cytoplasmic and transmembrane domains of the p75 and Trk A receptors regulate high affinity binding to nerve growth factor. J. Biol. Chem. 276, 32687–32695. doi: 10.1074/jbc.M011674200
Eyo, U. B., and Wu, L. J. (2013). Bidirectional microglia-neuron communication in the healthy brain. Neural Plast 2013:456857.
Famenini, S., Rigali, E. A., Olivera-Perez, H. M., Dang, J., Chang, M. T., Halder, R., et al. (2017). Increased intermediate M1-M2 macrophage polarization and improved cognition in mild cognitive impairment patients on omega-3 supplementation. FASEB J. 31, 148–160. doi: 10.1096/fj.201600677RR
Feng, D., Kim, T., Ozkan, E., Light, M., Torkin, R., Teng, K. K., et al. (2010). Molecular and structural insight into proNGF engagement of p75NTR and sortilin. J. Mol. Biol. 396, 967–984. doi: 10.1016/j.jmb.2009.12.030
Franco, M. L., Nadezhdin, K. D., Light, T. P., Goncharuk, S. A., Soler-Lopez, A., Ahmed, F., et al. (2021). Interaction between the transmembrane domains of neurotrophin receptors p75 and TrkA mediates their reciprocal activation. J. Biol. Chem. 297:100926. doi: 10.1016/j.jbc.2021.100926
Fraussen, J., Claes, N., Van Wijmeersch, B., van Horssen, J., Stinissen, P., Hupperts, R., et al. (2016). B cells of multiple sclerosis patients induce autoreactive proinflammatory T cell responses. Clin. Immunol. 173, 124–132.
Garrett-Thomson, S. C., Massimi, A., Fedorov, E. V., Bonanno, J. B., Scandiuzzi, L., Hillerich, B., et al. (2020). Mechanistic dissection of the PD-L1:B7-1 co-inhibitory immune complex. PLoS One 15:e0233578. doi: 10.1371/journal.pone.0233578
Gehler, S., Gallo, G., Veien, E., and Letourneau, P. C. (2004). p75 neurotrophin receptor signaling regulates growth cone filopodial dynamics through modulating RhoA activity. J. Neurosci. 24, 4363–4372. doi: 10.1523/JNEUROSCI.0404-04.2004
Gentry, J. J., Barker, P. A., and Carter, B. D. (2004). The p75 neurotrophin receptor: Multiple interactors and numerous functions. Prog. Brain Res. 146, 25–39. doi: 10.1016/S0079-6123(03)46002-0
Glerup, S., Nykjaer, A., and Vaegter, C. B. (2014). Sortilins in neurotrophic factor signaling. Handb. Exp. Pharmacol. 220, 165–189.
Gluska, S., Zahavi, E. E., Chein, M., Gradus, T., Bauer, A., Finke, S., et al. (2014). Rabies virus hijacks and accelerates the p75NTR retrograde axonal transport machinery. PLoS Pathog. 10:e1004348. doi: 10.1371/journal.ppat.1004348
Goldmann, T., Wieghofer, P., Jordao, M. J., Prutek, F., Hagemeyer, N., Frenzel, K., et al. (2016). Origin, fate and dynamics of macrophages at central nervous system interfaces. Nat. Immunol. 17, 797–805. doi: 10.1038/ni.3423
Gong, Y., Cao, P., Yu, H. J., and Jiang, T. (2008). Crystal structure of the neurotrophin-3 and p75NTR symmetrical complex. Nature 454, 789–793.
Hansen, J. D., Du Pasquier, L., Lefranc, M. P., Lopez, V., Benmansour, A., and Boudinot, P. (2009). The B7 family of immunoregulatory receptors: A comparative and evolutionary perspective. Mol. Immunol. 46, 457–472. doi: 10.1016/j.molimm.2008.10.007
He, X. L., and Garcia, K. C. (2004). Structure of nerve growth factor complexed with the shared neurotrophin receptor p75. Science 304, 870–875.
Hempstead, B. L., Martin-Zanca, D., Kaplan, D. R., Parada, L. F., and Chao, M. V. (1991). High-affinity NGF binding requires coexpression of the trk proto-oncogene and the low-affinity NGF receptor. Nature 350, 678–683. doi: 10.1038/350678a0
Hernangómez, M., Mestre, L., Correa, F. G., Loría, F., Mecha, M., Iñigo, P. M., et al. (2012). CD200-CD200R1 interaction contributes to neuroprotective effects of anandamide on experimentally induced inflammation. Glia 60, 1437–1450. doi: 10.1002/glia.22366
Heymach, J. V. Jr., and Shooter, E. M. (1995). The biosynthesis of neurotrophin heterodimers by transfected mammalian cells. J. Biol. Chem. 270, 12297–12304.
Hong, S., Beja-Glasser, V. F., Nfonoyim, B. M., Frouin, A., Li, S., Ramakrishnan, S., et al. (2016). Complement and microglia mediate early synapse loss in Alzheimer mouse models. Science 352, 712–716.
Huang, E. J., and Reichardt, L. F. (2003). Trk receptors: roles in neuronal signal transduction. Annu. Rev. Biochem. 72, 609–42. doi: 10.1146/annurev.biochem.72.121801.161629
Hussain, S. F., Kong, L. Y., Jordan, J., Conrad, C., Madden, T., Fokt, I., et al. (2007). A novel small molecule inhibitor of signal transducers and activators of transcription 3 reverses immune tolerance in malignant glioma patients. Cancer Res. 67, 9630–9636. doi: 10.1158/0008-5472.CAN-07-1243
Ibanez, C. F., and Simi, A. (2012). p75 neurotrophin receptor signaling in nervous system injury and degeneration: Paradox and opportunity. Trends Neurosci. 35, 431–440. doi: 10.1016/j.tins.2012.03.007
Irmady, K., Jackman, K. A., Padow, V. A., Shahani, N., Martin, L. A., Cerchietti, L., et al. (2014). Mir-592 regulates the induction and cell death-promoting activity of p75NTR in neuronal ischemic injury. J. Neurosci. 34, 3419–3428. doi: 10.1523/JNEUROSCI.1982-13.2014
Jakobsen, T. S., Østergaard, J. A., Kjolby, M., Birch, E. L., Bek, T., Nykjaer, A., et al. (2023). Sortilin inhibition protects neurons from degeneration in the diabetic retina. Invest. Ophthalmol. Vis. Sci. 64:8. doi: 10.1167/iovs.64.7.8
Johnson, D., Lanahan, A., Buck, C. R., Sehgal, A., Morgan, C., Mercer, E., et al. (1986). Expression and structure of the human NGF receptor. Cell 47, 545–554.
Knowles, J. K., Rajadas, J., Nguyen, T. V., Yang, T., LeMieux, M. C., Vander Griend, L., et al. (2009). The p75 neurotrophin receptor promotes amyloid-beta(1-42)-induced neuritic dystrophy in vitro and in vivo. J. Neurosci. 29, 10627–10637. doi: 10.1523/JNEUROSCI.0620-09.2009
Koorella, C, Nair, J. R., Murray, M. E., Carlson, L. M., Watkins, S. K., and Lee, K. P. (2014). Novel regulation of CD80/CD86-induced phosphatidylinositol 3-kinase signaling by NOTCH1 protein in interleukin-6 and indoleamine 2,3-dioxygenase production by dentdritic cells. J. Biol. Chem. 289, 7747–62. doi: 10.1074/jbc.M113.519686
Kokaia, Z., Andsberg, G., Martinez-Serrano, A., and Lindvall, O. (1998). Focal cerebral ischemia in rats induces expression of P75 neurotrophin receptor in resistant striatal cholinergic neurons. Neuroscience 84, 1113–1125. doi: 10.1016/s0306-4522(97)00579-4
Kucka, K., and Wajant, H. (2020). Receptor oligomerization and its relevance for signaling by receptors of the tumor necrosis factor receptor superfamily. Front. Cell Dev. Biol. 8:615141. doi: 10.3389/fcell.2020.615141
Lad, S. P., and Neet, K. E. (2003). Activation of the mitogen-activated protein kinase pathway through p75NTR: A common mechanism for the neurotrophin family. J. Neurosci. Res. 73, 614–626. doi: 10.1002/jnr.10695
Lee, R., Kermani, P., Teng, K. K., and Hempstead, B. L. (2001). Regulation of cell survival by secreted proneurotrophins. Science 294, 1945–1948.
Leloup, N., Chataigner, L. M. P., and Janssen, B. J. C. (2018). Structural insights into SorCS2-Nerve Growth Factor complex formation. Nat. Commun. 9:2979. doi: 10.1038/s41467-018-05405-z
Liepinsh, E., Ilag, L. L., Otting, G., and Ibanez, C. F. (1997). NMR structure of the death domain of the p75 neurotrophin receptor. EMBO J. 16, 4999–5005.
Lim, Y. S., McLaughlin, T., Sung, T. C., Santiago, A., Lee, K. F., and O’Leary, D. D. (2008). p75(NTR) mediates ephrin-A reverse signaling required for axon repulsion and mapping. Neuron 59, 746–758. doi: 10.1016/j.neuron.2008.07.032
Lin, Z., Tann, J. Y., Goh, E. T., Kelly, C., Lim, K. B., Gao, J. F., et al. (2015). Structural basis of death domain signaling in the p75 neurotrophin receptor. eLife 4:e11692.
Liu, W., Chou, T. F., Garrett-Thomson, S. C., Seo, G. Y., Fedorov, E., Ramagopal, U. A., et al. (2021). HVEM structures and mutants reveal distinct functions of binding to LIGHT and BTLA/CD160. J. Exp. Med. 218:e20211112. doi: 10.1084/jem.20211112
Liu, W., and Zang, X. (2019). Structures of immune checkpoints: An overview on the CD28-B7 family. Adv. Exp. Med. Biol. 1172, 63–78. doi: 10.1007/978-981-13-9367-9_3
Liu, X., Bao, Y., Meng, W., Yang, P., An, Y., Ma, J., et al. (2019). TROY interacts with RKIP to promote glioma development. Oncogene 38, 1544–1559. doi: 10.1038/s41388-018-0503-x
Loetscher, H., Pan, Y. C., Lahm, H. W., Gentz, R., Brockhaus, M., Tabuchi, H., et al. (1990). Molecular cloning and expression of the human 55 kd tumor necrosis factor receptor. Cell 61, 351–359.
Magnus, T., Schreiner, B., Korn, T., Jack, C., Guo, H., Antel, J., et al. (2005). Microglial expression of the B7 family member B7 homolog 1 confers strong immune inhibition: Implications for immune responses and autoimmunity in the CNS. J. Neurosci. 25, 2537–2546. doi: 10.1523/JNEUROSCI.4794-04.2005
Marchetti, L., Bonsignore, F., Gobbo, F., Amodeo, R., Calvello, M., Jacob, A., et al. (2019). Fast-diffusing p75(NTR) monomers support apoptosis and growth cone collapse by neurotrophin ligands. Proc. Natl. Acad. Sci. U.S.A. 116, 21563–21572. doi: 10.1073/pnas.1902790116
Meeker, R. B., Poulton, W., Feng, W.-H., Hudson, L., and Longo, F. M. (2012). Suppression of immunodeficiency virus-associated neural damage by the p75 neurotrophin receptor ligand, LM11A-31, in an in vitro feline model. J. Neuroimmune Pharmacol. 7, 388–400. doi: 10.1007/s11481-011-9325-0
Minnone, G., De Benedetti, F., and Bracci-Laudiero, L. (2017). NGF and its receptors in the regulation of inflammatory response. Int. J. Mol. Sci. 18:1028.
Mischel, P. S., Smith, S. G., Vining, E. R., Valletta, J. S., Mobley, W. C., and Reichardt, L. F. (2001). The extracellular domain of p75NTR is necessary to inhibit neurotrophin-3 signaling through TrkA. J. Biol. Chem. 276, 11294–11301. doi: 10.1074/jbc.M005132200
Morano, N. C., Smith, R. S., Danelon, V., Schreiner, R., Patel, U., Herrera, N. G., et al. (2022). Human immunomodulatory ligand B7-1 mediates synaptic remodeling via the p75 neurotrophin receptor. J. Clin. Invest. 132:e157002. doi: 10.1172/JCI157002
Nimmerjahn, A., Kirchhoff, F., and Helmchen, F. (2005). Resting microglial cells are highly dynamic surveillants of brain parenchyma in vivo. Science 308, 1314–1318.
Nykjaer, A., Lee, R., Teng, K. K., Jansen, P., Madsen, P., Nielsen, M. S., et al. (2004). Sortilin is essential for proNGF-induced neuronal cell death. Nature 427, 843–848. doi: 10.1038/nature02319
O’Keefe, G. M., Nguyen, V. T., and Benveniste, E. N. (2002). Regulation and function of class II major histocompatibility complex. CD40, and B7 expression in macrophages and microglia: Implications in neurological diseases. J. Neurovirol. 8, 496–512. doi: 10.1080/13550280290100941
Olah, M., Menon, V., Habib, N., Taga, M. F., Ma, Y., Yung, C. J., et al. (2020). Single cell RNA sequencing of human microglia uncovers a subset associated with Alzheimer’s disease. Nat. Commun. 11:6129. doi: 10.1038/s41467-020-19737-2
Olson, J. K., Girvin, A. M., and Miller, S. D. (2001). Direct activation of innate and antigen-presenting functions of microglia following infection with Theiler’s virus. J. Virol. 75, 9780–9789. doi: 10.1128/JVI.75.20.9780-9789.2001
Orabona, C., Grohmann, U., Belladonna, M. L., Fallarino, F., Vacca, C., Bianchi, R., et al. (2004). CD28 induces immunostimulatory signals in dendritic cells via CD80 and CD86. Nat. Immunol. 5, 1134–42. doi: 10.1038/ni1124
Park, H., and Poo, M. M. (2013). Neurotrophin regulation of neural circuit development and function. Nat. Rev. Neurosci. 14, 7–23.
Park, J. B., Yiu, G., Kaneko, S., Wang, J., Chang, J., He, X. L., et al. (2005). A TNF receptor family member, TROY, is a coreceptor with Nogo receptor in mediating the inhibitory activity of myelin inhibitors. Neuron 45, 345–351. doi: 10.1016/j.neuron.2004.12.040
Patnaik, A., Zagrebelsky, M., Korte, M., and Holz, A. (2020). Signaling via the p75 neurotrophin receptor facilitates amyloid-beta-induced dendritic spine pathology. Sci. Rep. 10:13322. doi: 10.1038/s41598-020-70153-4
Peferoen, L. A., Vogel, D. Y., Ummenthum, K., Breur, M., Heijnen, P. D., Gerritsen, W. H., et al. (2015). Activation status of human microglia is dependent on lesion formation stage and remyelination in multiple sclerosis. J. Neuropathol. Exp. Neurol. 74, 48–63. doi: 10.1097/NEN.0000000000000149
Prinz, M., Masuda, T., Wheeler, M. A., and Quintana, F. J. (2021). Microglia and central nervous system-associated macrophages-from origin to disease modulation. Annu. Rev. Immunol. 39, 251–277. doi: 10.1146/annurev-immunol-093019-110159
Quistad, S. D., Stotland, A., Barott, K. L., Smurthwaite, C. A., Hilton, B. J., Grasis, J. A., et al. (2014). Evolution of TNF-induced apoptosis reveals 550 My of functional conservation. Proc. Natl. Acad. Sci. U.S.A. 111, 9567–9572. doi: 10.1073/pnas.1405912111
Quistad, S. D., and Traylor-Knowles, N. (2016). Precambrian origins of the TNFR superfamily. Cell Death Discov. 2:16058. doi: 10.1038/cddiscovery.2016.58
Radeke, M. J., Misko, T. P., Hsu, C., Herzenberg, L. A., and Shooter, E. M. (1987). Gene transfer and molecular cloning of the rat nerve growth factor receptor. Nature 325, 593–597.
Ramos, A., Ho, W. C., Forte, S., Dickson, K., Boutilier, J., Favell, K., et al. (2007). Hypo-osmolar stress induces p75NTR expression by activating Sp1-dependent transcription. J. Neurosci. 27, 1498–1506. doi: 10.1523/JNEUROSCI.4806-06.2007
Rawat, P., and Spector, S. A. (2017). Development and characterization of a human microglia cell model of HIV-1 infection. J. Neurovirol. 23, 33–46.
Ren, X., Lin, Z., and Yuan, W. (2022). A structural and functional perspective of death receptor 6. Front. Pharmacol. 13:836614. doi: 10.3389/fphar.2022.836614
Salehi, A. H., Xanthoudakis, S., and Barker, P. A. (2002). NRAGE, a p75 neurotrophin receptor-interacting protein, induces caspase activation and cell death through a JNK-dependent mitochondrial pathway. J. Biol. Chem. 277, 48043–48050. doi: 10.1074/jbc.M205324200
Satoh, J., Kino, Y., Asahina, N., Takitani, M., Miyoshi, J., Ishida, T., et al. (2016). TMEM119 marks a subset of microglia in the human brain. Neuropathology 36, 39–49. doi: 10.1111/neup.12235
Satoh, J., Lee, Y. B., and Kim, S. U. (1995). T-cell costimulatory molecules B7-1 (CD80) and B7-2 (CD86) are expressed in human microglia but not in astrocytes in culture. Brain Res. 704, 92–96.
Schall, T. J., Lewis, M., Koller, K. J., Lee, A., Rice, G. C., Wong, G. H., et al. (1990). Molecular cloning and expression of a receptor for human tumor necrosis factor. Cell 61, 361–370.
Sedy, J. R., Gavrieli, M., Potter, K. G., Hurchla, M. A., Lindsley, R. C., Hildner, K., et al. (2005). B and T lymphocyte attenuator regulates T cell activation through interaction with herpesvirus entry mediator. Nat. Immunol. 6, 90–98. doi: 10.1038/ni1144
Shamovsky, I. L., Ross, G. M., Riopelle, R. J., and Weaver, D. F. (1999). The interaction of neurotrophins with the p75NTR common neurotrophin receptor: A comprehensive molecular modeling study. Protein Sci. 8, 2223–2233. doi: 10.1110/ps.8.11.2223
Shao, Z., Browning, J. L., Lee, X., Scott, M. L., Shulga-Morskaya, S., Allaire, N., et al. (2005). TAJ/TROY, an orphan TNF receptor family member, binds Nogo-66 receptor 1 and regulates axonal regeneration. Neuron 45, 353–359. doi: 10.1016/j.neuron.2004.12.050
Shi, J., Longo, F. M., and Massa, S. M. (2013). A small molecule p75(NTR) ligand protects neurogenesis after traumatic brain injury. Stem Cells 31, 2561–2574. doi: 10.1002/stem.1516
Shirai, T., Yamaguchi, H., Ito, H., Todd, C. W., and Wallace, R. B. (1985). Cloning and expression in Escherichia coli of the gene for human tumour necrosis factor. Nature 313, 803–806. doi: 10.1038/313803a0
Shrestha, R., Garrett-Thomson, S. C., Liu, W., Almo, S. C., and Fiser, A. (2020). Redesigning HVEM Interface for Selective Binding to LIGHT, BTLA, and CD160. Structure 28, 1197.e2–1205.e2. doi: 10.1016/j.str.2020.07.013
Smith, C. A., Davis, T., Anderson, D., Solam, L., Beckmann, M. P., Jerzy, R., et al. (1990). A receptor for tumor necrosis factor defines an unusual family of cellular and viral proteins. Science 248, 1019–1023. doi: 10.1126/science.2160731
Stevens, B., Allen, N. J., Vazquez, L. E., Howell, G. R., Christopherson, K. S., Nouri, N., et al. (2007). The classical complement cascade mediates CNS synapse elimination. Cell 131, 1164–1178.
Sugiura, D., Maruhashi, T. I, Okazaki, M., Shimizu, K., Maeda, T. K., Takemoto, T., et al. (2019). Restriction of PD-1 function by cis-PD-L1/CD80 interactions is required for optimal T cell responses. Science 364, 558–566. doi: 10.1126/science.aav7062
Sun, P. D., and Davies, D. R. (1995). The cystine-knot growth-factor superfamily. Annu. Rev. Biophys. Biomol. Struct. 24, 269–291.
Svenningsson, A., Dotevall, L., Stemme, S., and Andersen, O. (1997). Increased expression of B7-1 costimulatory molecule on cerebrospinal fluid cells of patients with multiple sclerosis and infectious central nervous system disease. J. Neuroimmunol. 75, 59–68. doi: 10.1016/s0165-5728(96)00234-2
Tep, C., Lim, T. H., Ko, P. O., Getahun, S., Ryu, J. C., Goettl, V. M., et al. (2013). Oral administration of a small molecule targeted to block proNGF binding to p75 promotes myelin sparing and functional recovery after spinal cord injury. J. Neurosci. 33, 397–410. doi: 10.1523/JNEUROSCI.0399-12.2013
Tremblay, M., Lowery, R. L., and Majewska, A. K. (2010). Microglial interactions with synapses are modulated by visual experience. PLoS Biol. 8:e1000527. doi: 10.1371/journal.pbio.1000527
Tuffereau, C., Schmidt, K., Langevin, C., Lafay, F., Dechant, G., and Koltzenburg, M. (2007). The rabies virus glycoprotein receptor p75NTR is not essential for rabies virus infection. J. Virol. 81, 13622–13630.
Vanamee, ÉS., and Faustman, D. L. (2020). On the TRAIL of better therapies: Understanding TNFRSF structure-function. Cells 9:764. doi: 10.3390/cells9030764
Vilar, M., Charalampopoulos, I., Kenchappa, R. S., Simi, A., Karaca, E., Reversi, A., et al. (2009). Activation of the p75 neurotrophin receptor through conformational rearrangement of disulphide-linked receptor dimers. Neuron 62, 72–83. doi: 10.1016/j.neuron.2009.02.020
Wajant, H., and Siegmund, D. (2019). TNFR1 and TNFR2 in the control of the life and death balance of macrophages. Front. Cell Dev. Biol. 7:91. doi: 10.3389/fcell.2019.00091
Wake, H., Moorhouse, A. J., Jinno, S., Kohsaka, S., and Nabekura, J. (2009). Resting microglia directly monitor the functional state of synapses in vivo and determine the fate of ischemic terminals. J. Neurosci. 29, 3974–3980. doi: 10.1523/JNEUROSCI.4363-08.2009
Walker, D. G., Dalsing-Hernandez, J. E., Campbell, N. A., and Lue, L. F. (2009). Decreased expression of CD200 and CD200 receptor in Alzheimer’s disease: A potential mechanism leading to chronic inflammation. Exp. Neurol. 215, 5–19. doi: 10.1016/j.expneurol.2008.09.003
Wehrman, T., He, X., Raab, B., Dukipatti, A., Blau, H., and Garcia, K. C. (2007). Structural and mechanistic insights into nerve growth factor interactions with the TrkA and p75 receptors. Neuron 53, 25–38. doi: 10.1016/j.neuron.2006.09.034
Westergaard, U. B., Kirkegaard, K., Sorensen, E. S., Jacobsen, C., Nielsen, M. S., Petersen, C. M., et al. (2005). SorCS3 does not require propeptide cleavage to bind nerve growth factor. FEBS Lett. 579, 1172–1176.
Williams, K., Ulvestad, E., and Antel, J. (1994). Immune regulatory and effector properties of human adult microglia studies in vitro and in situ. Adv. Neuroimmunol. 4, 273–281.
Wilton, D. K., Dissing-Olesen, L., and Stevens, B. (2019). Neuron-glia signaling in synapse elimination. Annu. Rev. Neurosci. 42, 107–127.
Windhagen, A., Newcombe, J., Dangond, F., Strand, C., Woodroofe, M. N., Cuzner, M. L., et al. (1995). Expression of costimulatory molecules B7-1 (CD80), B7-2 (CD86), and interleukin 12 cytokine in multiple sclerosis lesions. J. Exp. Med. 182, 1985–1996. doi: 10.1084/jem.182.6.1985
Wislet, S., Vandervelden, G., and Rogister, B. (2018). From Neural crest development to cancer and vice versa: How p75(NTR) and (Pro)neurotrophins could act on cell migration and invasion? Front. Mol. Neurosci. 11:244. doi: 10.3389/fnmol.2018.00244
Wong, L. W., Chong, Y. S., Lin, W., Kisiswa, L., Sim, E., Ibanez, C. F., et al. (2021). Age-related changes in hippocampal-dependent synaptic plasticity and memory mediated by p75 neurotrophin receptor. Aging Cell 20:e13305. doi: 10.1111/acel.13305
Wong, S. T., Henley, J. R., Kanning, K. C., Huang, K. H., Bothwell, M., and Poo, M. M. (2002). A p75(NTR) and Nogo receptor complex mediates repulsive signaling by myelin-associated glycoprotein. Nat. Neurosci. 5, 1302–1308. doi: 10.1038/nn975
Woo, N. H., Teng, H. K., Siao, C. J., Chiaruttini, C., Pang, P. T., Milner, T. A., et al. (2005). Activation of p75NTR by proBDNF facilitates hippocampal long-term depression. Nat. Neurosci. 8, 1069–1077.
Wright, G. J., Jones, M., Puklavec, M. J., Brown, M. H., and Barclay, A. N. (2001). The unusual distribution of the neuronal/lymphoid cell surface CD200 (OX2) glycoprotein is conserved in humans. Immunology 102, 173–179. doi: 10.1046/j.1365-2567.2001.01163.x
Yaar, M., Zhai, S., Pilch, P. F., Doyle, S. M., Eisenhauer, P. B., Fine, R. E., et al. (1997). Binding of beta-amyloid to the p75 neurotrophin receptor induces apoptosis. A possible mechanism for Alzheimer’s disease. J. Clin. Invest. 100, 2333–2340. doi: 10.1172/JCI119772
Yamashita, T., Tucker, K. L., and Barde, Y. A. (1999). Neurotrophin binding to the p75 receptor modulates Rho activity and axonal outgrowth. Neuron 24, 585–593. doi: 10.1016/s0896-6273(00)81114-9
Yan, H., and Chao, M. V. (1991). Disruption of cysteine-rich repeats of the p75 nerve growth factor receptor leads to loss of ligand binding. J. Biol. Chem. 266, 12099–12104.
Yang, J., Harte-Hargrove, L. C., Siao, C. J., Marinic, T., Clarke, R., Ma, Q., et al. (2014). proBDNF negatively regulates neuronal remodeling, synaptic transmission, and synaptic plasticity in hippocampus. Cell Rep. 7, 796–806.
Ye, Q., Liu, J., and Xie, K. (2019). B7 family proteins in cancer progression: Immunological and non-immunological functions. J. Cancer Treat. Diagnosis 3, 1–6.
Ye, X., Mehlen, P., Rabizadeh, S., VanArsdale, T., Zhang, H., Shin, H., et al. (1999). TRAF family proteins interact with the common neurotrophin receptor and modulate apoptosis induction. J. Biol. Chem. 274, 30202–30208. doi: 10.1074/jbc.274.42.30202
Zanin, J. P., and Friedman, W. J. (2022). p75NTR prevents the onset of cerebellar granule cell migration via RhoA activation. eLife 11:e79934. doi: 10.7554/eLife.79934
Zanin, J. P., Verpeut, J. L., Li, Y., Shiflett, M. W., Wang, S. S., Santhakumar, V., et al. (2019). The p75NTR influences cerebellar circuit development and adult behavior via regulation of cell cycle duration of granule cell progenitors. J. Neurosci. 39, 9119–9129. doi: 10.1523/JNEUROSCI.0990-19.2019
Zhong, L., Sheng, X., Wang, W., Li, Y., Zhuo, R., Wang, K., et al. (2023). TREM2 receptor protects against complement-mediated synaptic loss by binding to complement C1q during neurodegeneration. Immunity 56, 1794.e8–1808.e8. doi: 10.1016/j.immuni.2023.06.016
Keywords: p75NTR, neurotrophin, B7-1, CD80, TNF receptor
Citation: Danelon V, Garret-Thomson SC, Almo SC, Lee FS and Hempstead BL (2023) Immune activation of the p75 neurotrophin receptor: implications in neuroinflammation. Front. Mol. Neurosci. 16:1305574. doi: 10.3389/fnmol.2023.1305574
Received: 02 October 2023; Accepted: 10 November 2023;
Published: 01 December 2023.
Edited by:
Juan Carlos Arévalo, University of Salamanca, SpainReviewed by:
Mark Bothwell, University of Washington, United StatesBruce Douglas Carter, Vanderbilt University, United States
Copyright © 2023 Danelon, Garret-Thomson, Almo, Lee and Hempstead. This is an open-access article distributed under the terms of the Creative Commons Attribution License (CC BY). The use, distribution or reproduction in other forums is permitted, provided the original author(s) and the copyright owner(s) are credited and that the original publication in this journal is cited, in accordance with accepted academic practice. No use, distribution or reproduction is permitted which does not comply with these terms.
*Correspondence: Barbara L. Hempstead, blhempst@med.cornell.edu; Francis S. Lee, fslee@med.cornell.edu; Steven C. Almo, steve.almo@einsteinmed.edu
†These authors have contributed equally to this work and share first authorship