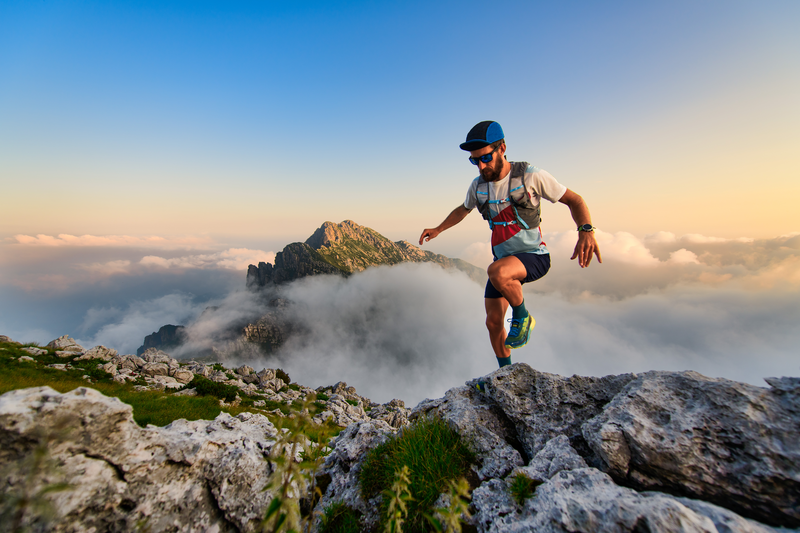
94% of researchers rate our articles as excellent or good
Learn more about the work of our research integrity team to safeguard the quality of each article we publish.
Find out more
EDITORIAL article
Front. Mol. Neurosci. , 31 July 2023
Sec. Molecular Signalling and Pathways
Volume 16 - 2023 | https://doi.org/10.3389/fnmol.2023.1240959
This article is part of the Research Topic Synergistic interactions between exosomes and tunneling nanotubes in long-range intercellular transfer View all 5 articles
Editorial on the Research Topic
Synergistic interactions between exosomes and tunneling nanotubes in long-range intercellular transfer
Cell-to-cell communication is a critical process that ensures interaction between adjacent and distant cells to maintain cellular homeostasis in normal physiology and pathophysiological conditions. Two adjacent cells communicate to transfer ions and small molecules via Gap junctions, small selective ion channels. Distant cells establish long-range communication via endocrine/paracrine secretions, extracellular vesicles (EVs) and tunneling nanotubes (TNTs) (Aguzzi and Lakkaraju, 2016). Recent studies have implicated crucial roles of exosomes and TNTs in long-range intercellular transfer of cellular materials. The exosome is the smallest of the EVs, moving between physically distant cells to exchange cellular materials, including mRNA and biomolecules. Emerging studies have shown transfer of mitochondria via exosomes between distant cells (Hough et al., 2018). In parallel, TNTs make direct actin-membrane open-ended channels to transfer cytoplasmic materials such as mRNA, proteins, and various organelles between distal cells. Recent advancements in the understanding of long-range intercellular transfer by exosomes and TNTs have demonstrated profound implications in various (patho)physiological conditions, including cancer progression, immune responses, neurodegeneration and tissue repair (Raghavan et al., 2021).
Emerging evidence suggests that cellular stress can trigger the biogenesis of these two intercellular transfer modes, i.e., both exosomes and TNTs. Studies have demonstrated that neurodegenerative protein aggregates facilitate exosome release and biogenesis of TNTs to enhance transfer of toxic materials (Victoria and Zurzolo, 2017; Rastogi et al., 2021). Other recent studies have indicated the therapeutic potential of exosomes and TNTs to counteract the detrimental effects of toxicities. Exosomes derived from neuronal cells may contain neuroprotective factors and transfer between cells to promote neuronal survival (Natale et al., 2022). Furthermore, exosomes derived from mesenchymal stem cells (MSCs) show great potential to promote tissue regeneration and repair by stimulating cell proliferation and angiogenesis (Raghavan et al., 2021). Similarly, transfer of healthy mitochondria from MSCs via TNTs demonstrated their crucial role in cell survival (Spees et al., 2006). TNT-mediated intercellular transfer of mitochondria from MSCs can rescue retinal ganglion cells and corneal epithelial in dry eye disease (Jiang et al., 2023). Similarly, exosomes released from Muller glia are potentially neurotrophic and may have therapeutic applications, Kalargyrou et al..
Interestingly, studies have implicated two disparate TNT-based fates due to cell-to-cell transfer of aggregation-prone proteins, the spreading of pathology and rescuing of toxic burdens of aggregates. The review by Lagalwar, 2022 discusses these two disparate TNT-based fates in neurodegenerative pathologies, such as Alzheimer's, Parkinson's, and Huntington's disease, and ALS. After the discovery of the TNT-structure (Rustom et al., 2004), several studies have shown TNT-based spreading of aggregation-prone proteins and pathology progression in neuronal models (Victoria and Zurzolo, 2017). On the other hand, several studies have shown that astrocytes and microglia rescue toxicities of neurodegenerative aggregates by sharing their burden with neighboring cells by means of TNTs (Rostami et al., 2017; Scheiblich et al., 2021). Moreover, one study demonstrated that neuronal cells transfer toxic materials to microglia, whereas microglia can transfer healthy mitochondria to rescue neurons and to ameliorate neurodegeneration (Chakraborty et al., 2023). This implicates the intricate interplay between intercellular transfer and cellular stress responses.
Cellular stress induced triggering of molecular events, which are associated with endolysosomal toxicities, mitochondrial toxicities, oxidative stress and intracellular trafficking interface to the biogenesis of both exosomes and TNTs (Nawaz and Fatima, 2017). This raises the question whether cells use these modes of intercellular transfer to rescue cells by facilitating clearance of toxic burdens. Moreover, cells possess other intriguing mechanisms to clear or degrade unwanted material. In addition to the conventional lysosomal and proteasomal degradation machineries, cells can activate autophagosome dependent cellular clearance in response to pathological toxic conditions. Enhanced upregulation of autophagy is evident in all neurodegenerative diseases. The perspective of intercellular transfer on leaderless neurodegenerative proteins in relation to autophagosome dependent cellular clearance has been discussed in Padmanabhan and Manjithaya. Further, studies are needed to understand the fate of conventional and autophagosome-mediated cellular clearance involving exosomes and TNT-mediated rescue of toxicities. Nevertheless, several questions remain unanswered. What factors trigger these intercellular transfer vehicles? Do dysfunction or inefficiency of the cellular clearance processes promote intercellular transfer as an alternative pathway to remove toxic components?
Another issue is the interplay between exosomes and TNTs to harness synergistic effect on intercellular communication. In this context, the work of Mentor and Fisher is timely and highly relevant. The study illustrates, using high-resolution scanning electron microscopy (HR-SEM), that membrane-bound exosomes attached to the plasma membrane of brain endothelial cells (BEC), participate in TNT formation between the cells, and facilitate in vitro blood brain barrier (BBB) genesis. Research has also shown that exosomes released by BECs transfer specific cargo to recipient brain cells (Banks et al., 2020). BECs play pivotal roles in the permeability of the BBB, which is implicated in the progression of neurodegenerative pathologies. The study of Mentor and Fisher suggested TNT-mediated trans-permeability between BEC cells. Recently another study has shown that thrombospondin-1-containing exosomes released from breast cancer cells promote biogenesis of TNTs (Mahadik and Patwardhan, 2023). Furthermore, exosomes utilize TNTs as vehicles for efficient long-distance transfer of selective cargo to the recipient cells (Thayanithy et al., 2014). This remarkable synergy and the convergence between exosomes and TNTs presents a novel mechanism in the field of intercellular transfer (Figure 1).
Figure 1. Cellular stress induced exosomes and TNTs, and their synergistic interactions in long-range intercellular transfer.
In conclusion, the synergistic interplay between exosomes and TNTs in long-range intercellular transfer is rapidly evolving as a promising area of cellular communication. Several important questions have been raised by recent advancements in this emerging field of research. What molecular mechanisms govern their on-demand biogenesis in response to cellular toxicities to rescue pathologies? How are exosomes selectively incorporated into TNTs? Do specific pathophysiological conditions enhance or restrict biogenesis of these intercellular conduits and their functionality? Addressing these questions could provide valuable insights into intercellular communications and their complexities, and will open up new avenues for therapeutic intervention.
All authors listed have made a substantial, direct, and intellectual contribution to the work and approved it for publication.
SN was supported by the research grants from the Indian Council of Medical Research of India (#5/4-5/Ad hoc/Neuro/216/2020-NCD-I) and the Science and Engineering Research Board of India (#SRG/2021/001315). JN was supported in part by grant 21-04607X from the Czech Science Foundation.
We acknowledge Mr. Deepak KV, PhD student of Manipal Academy of Higher Education, India, for contributing the art work of the Figure 1.
The authors declare that the research was conducted in the absence of any commercial or financial relationships that could be construed as a potential conflict of interest.
All claims expressed in this article are solely those of the authors and do not necessarily represent those of their affiliated organizations, or those of the publisher, the editors and the reviewers. Any product that may be evaluated in this article, or claim that may be made by its manufacturer, is not guaranteed or endorsed by the publisher.
Aguzzi, A., and Lakkaraju, A. K. K. (2016). Cell biology of prions and prionoids: a status report. Trends Cell Biol. 26, 40–51. doi: 10.1016/j.tcb.2015.08.007
Banks, W. A., Sharma, P., Bullock, K. M., Hansen, K. M., Ludwig, N., Whiteside, T. L., et al. (2020). Transport of extracellular vesicles across the blood-brain barrier: brain pharmacokinetics and effects of inflammation. Int. J. Mol. Sci. 21, 4407. doi: 10.3390/ijms21124407
Chakraborty, R., Nonaka, T., Hasegawa, M., and Zurzolo, C. (2023). Tunnelling nanotubes between neuronal and microglial cells allow bi-directional transfer of alpha-Synuclein and mitochondria. Cell Death Dis. 14, 329. doi: 10.1038/s41419-023-05835-8
Hough, K. P., Trevor, J. L., Strenkowski, J. G., Wang, Y., Chacko, B. K., Tousif, S., et al. (2018). Exosomal transfer of mitochondria from airway myeloid-derived regulatory cells to T cells. Redox Biol. 18, 54–64. doi: 10.1016/j.redox.2018.06.009
Jiang, D., Xu, W., Peng, F., Sun, Y., Pan, C., Yu, J., et al. (2023). Tunneling nanotubes-based intercellular mitochondrial trafficking as a novel therapeutic target in dry eye. Exp. Eye Res. 232, 109497. doi: 10.1016/j.exer.2023.109497
Mahadik, P., and Patwardhan, S. E. C. M. (2023). Stiffness-regulated exosomal thrombospondin-1 promotes tunneling nanotubes-based cellular networking in breast cancer cells. Arch. Biochem. Biophys. 742, 109624. doi: 10.1016/j.abb.2023.109624
Natale, F., Fusco, S., and Grassi, C. (2022). Dual role of brain-derived extracellular vesicles in dementia-related neurodegenerative disorders: cargo of disease spreading signals and diagnostic-therapeutic molecules. Transl. Neurodegener. 11, 50. doi: 10.1186/s40035-022-00326-w
Nawaz, M., and Fatima, F. (2017). Extracellular vesicles, tunneling nanotubes, and cellular interplay: synergies and missing links. Front Mol Biosci. 4, 50. doi: 10.3389/fmolb.2017.00050
Raghavan, A., Rao, P., Neuzil, J., Pountney, D. L., and Nath, S. (2021). Oxidative stress and Rho GTPases in the biogenesis of tunnelling nanotubes: implications in disease and therapy. Cell. Mol. Life Sci. 79, 36. doi: 10.1007/s00018-021-04040-0
Rastogi, S., Sharma, V., Bharti, P. S., Rani, K., Modi, G. P., Nikolajeff, F., et al. (2021). The evolving landscape of exosomes in neurodegenerative diseases: exosomes characteristics and a promising role in early diagnosis. Int. J. Mol. Sci. 22, 440. doi: 10.3390/ijms22010440
Rostami, J., Holmqvist, S., Lindström, V., Sigvardson, J., Westermark, G. T., Ingelsson, M., et al. (2017). Human astrocytes transfer aggregated alpha-synuclein via tunneling nanotubes. J. Neurosci. 37, 11835–11853. doi: 10.1523/JNEUROSCI.0983-17.2017
Rustom, A., Saffrich, R., Markovic, I., Walther, P., and Gerdes, H.-.H. (2004). Nanotubular highways for intercellular organelle transport. Science 303, 1007–10. doi: 10.1126/science.1093133
Scheiblich, H., Dansokho, C., Mercan, D., Schmidt, S. V., Bousset, L., Wischhof, L., et al. (2021). Microglia jointly degrade fibrillar alpha-synuclein cargo by distribution through tunneling nanotubes. Cell. 184, 5089–5106 e21. doi: 10.1016/j.cell.2021.09.007
Spees, J. L., Olson, S. D., Whitney, M. J., and Prockop, D. J. (2006). Mitochondrial transfer between cells can rescue aerobic respiration. Proc. Natl. Acad. Sci. U. S. A. 103, 1283–1288. doi: 10.1073/pnas.0510511103
Thayanithy, V., Babatunde, V., Dickson, E. L., Wong, P., Oh, S., Ke, X., et al. (2014). Tumor exosomes induce tunneling nanotubes in lipid raft-enriched regions of human mesothelioma cells. Exp Cell Res. 323, 178–188. doi: 10.1016/j.yexcr.2014.01.014
Keywords: exosomes, tunneling nanotubes, intercellular transfer, neurodegenerative aggregates, cell-to-cell communication
Citation: Nath S, Pountney DL and Neuzil J (2023) Editorial: Synergistic interactions between exosomes and tunneling nanotubes in long-range intercellular transfer. Front. Mol. Neurosci. 16:1240959. doi: 10.3389/fnmol.2023.1240959
Received: 15 June 2023; Accepted: 05 July 2023;
Published: 31 July 2023.
Edited by:
Jean-Marc Taymans, Institut National de la Santé et de la Recherche Médicale (INSERM), FranceReviewed by:
Jochen C. Meier, Technical University of Braunschweig, GermanyCopyright © 2023 Nath, Pountney and Neuzil. This is an open-access article distributed under the terms of the Creative Commons Attribution License (CC BY). The use, distribution or reproduction in other forums is permitted, provided the original author(s) and the copyright owner(s) are credited and that the original publication in this journal is cited, in accordance with accepted academic practice. No use, distribution or reproduction is permitted which does not comply with these terms.
*Correspondence: Sangeeta Nath, c2FuZ2VldGEubmF0aEBtYW5pcGFsLmVkdQ==
Disclaimer: All claims expressed in this article are solely those of the authors and do not necessarily represent those of their affiliated organizations, or those of the publisher, the editors and the reviewers. Any product that may be evaluated in this article or claim that may be made by its manufacturer is not guaranteed or endorsed by the publisher.
Research integrity at Frontiers
Learn more about the work of our research integrity team to safeguard the quality of each article we publish.