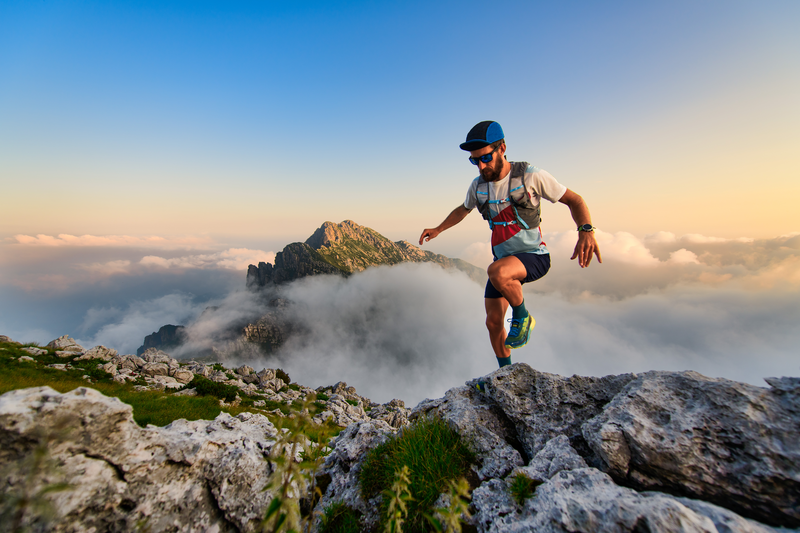
94% of researchers rate our articles as excellent or good
Learn more about the work of our research integrity team to safeguard the quality of each article we publish.
Find out more
ORIGINAL RESEARCH article
Front. Mol. Neurosci. , 02 June 2023
Sec. Brain Disease Mechanisms
Volume 16 - 2023 | https://doi.org/10.3389/fnmol.2023.1194769
This article is part of the Research Topic Neuropsychiatric and Neurodegenerative aspects of acute and Long COVID View all 5 articles
Critical COVID-19 disease is accompanied by depletion of plasma tryptophan (TRY) and increases in indoleamine-dioxygenase (IDO)-stimulated production of neuroactive tryptophan catabolites (TRYCATs), including kynurenine (KYN). The TRYCAT pathway has not been studied extensively in association with the physiosomatic and affective symptoms of Long COVID. In the present study, we measured serum TRY, TRYCATs, insulin resistance (using the Homeostatic Model Assessment Index 2-insulin resistance, HOMA2-IR), C-reactive protein (CRP), physiosomatic, depression, and anxiety symptoms in 90 Long COVID patients, 3–10 months after remission of acute infection. We were able to construct an endophenotypic class of severe Long COVID (22% of the patients) with very low TRY and oxygen saturation (SpO2, during acute infection), increased kynurenine, KYN/TRY ratio, CRP, and very high ratings on all symptom domains. One factor could be extracted from physiosomatic symptoms (including chronic fatigue-fibromyalgia), depression, and anxiety symptoms, indicating that all domains are manifestations of the common physio-affective phenome. Three Long COVID biomarkers (CRP, KYN/TRY, and IR) explained around 40% of the variance in the physio-affective phenome. The latter and the KYN/TRY ratio were significantly predicted by peak body temperature (PBT) and lowered SpO2 during acute infection. One validated latent vector could be extracted from the three symptom domains and a composite based on CRP, KYN/TRY, and IR (Long COVID), and PBT and SpO2 (acute COVID-19). In conclusion, the physio-affective phenome of Long COVID is a manifestation of inflammatory responses during acute and Long COVID, and lowered plasma tryptophan and increased kynurenine may contribute to these effects.
After the COVID-19 pandemic, which caused millions of acute cases and millions of deaths, healthcare professionals are facing another crisis due to the development and/or persistence of symptoms following the acute phase of SARS-CoV-2 infection (typically after 3 months), a condition known as Long COVID (Fernández-de-Las-Peñas, 2022). Long COVID is most often defined as symptoms lasting more than 3 months after the onset of acute COVID-19 (Shah et al., 2021; Yong, 2021). The most frequently reported symptoms associated with long-term COVID are chronic fatigue, dyspnea, affective symptoms (anxiety and depression), and cognitive impairments (Arnold et al., 2021; Bliddal et al., 2021; Davis et al., 2021; Huang et al., 2021; Shah et al., 2021; Van den Borst et al., 2021; Yong, 2021; Guo et al., 2022). Within 6 months following the onset of the first COVID-19 symptom, about one-third of COVID-19 survivors experience neuropsychiatric symptoms, such as sleeplessness, anxiety, or depression (Taquet et al., 2021).
Several pathways are believed to underlie the physio-affective phenome of Long COVID, which includes physiosomatic and affective (depression and anxiety) symptoms (Al-Hakeim et al., 2022). Initially, the physio-affective phenome of chronic COVID was substantially predicted by reduced oxygen saturation (SpO2) and elevated peak body temperature (PBT) during the acute phase of SARS-CoV-2 infection (Al-Hadrawi et al., 2022). This suggests that the Long COVID physio-affective phenome is the outcome of immune-inflammatory processes during the acute infection, at least in part (Al-Hadrawi et al., 2022; Maes et al., 2022a; Al-Hakeim et al., 2023a,b). Second, the physio-affective phenome of Long COVID is associated with neuro-oxidative stress toxicity (OSTOX) and decreased antioxidant defenses (ANTIOX; Maes, 2011b; Maes et al., 2022b; Al-Hakeim et al., 2023a), activation of the NLRP3 inflammasome, inflammation as indicated by increased levels of C-reactive protein (CRP), and increased insulin resistance as assessed with the Homeostatic Model Assessment Index 2-insulin resistance (HOMA2-IR; Al-Hakeim et al., 2023b).
According to a recent meta-analysis, the acute infectious phase is characterized by a considerable activation of indoleamine-2,3-dioxygenase (IDO), which leads to tryptophan depletion and an increase in the synthesis of tryptophan catabolites (TRYCATs; Almulla et al., 2022c). Moreover, activation of the TRYCAT pathway is related to critical illness and higher mortality (Almulla and Maes, 2022). IDO activation is triggered by reactive oxygen species and pro-inflammatory cytokines, which are elevated during the acute infection phase (Maes et al., 2011a). These cytokines include interferon (IFN)-α, IFN-γ, interleukin (IL)-1β, tumor necrosis factor (TNF)-α-, and IL-18 (Liebau et al., 2002; Oxenkrug, 2007; Maes et al., 2011b). Tryptophan depletion and increased synthesis of neuroactive TRYCATs may result from IDO activation. Several neurotoxic TRYCATs, including kynurenine (KYN), 3-hydroxykynurenine (3HK), anthranilic acid (AA), 3-hydroxyanthranilic acid (3-HA), and quinolinic acid (QA) are formed, but also TRYCATs with more protective properties are formed, such as kynurenic acid (KA; Maes et al., 2011b; Almulla and Maes, 2022). TRYCATs such as 3HK and QA, for instance, generate neuro-oxidative toxicity, resulting in oxidative cell damage and lipid peroxidation (Goldstein et al., 2000; Smith et al., 2009; Reyes Ocampo et al., 2014; Almulla and Maes, 2022). Tryptophan deficiency is also characteristic of major depression, somatization disorder, schizophrenia, cognitive deficits, and physiosomatic symptoms (Maes et al., 1991; Maes and Rief, 2012; Almulla et al., 2022c,d). It is thought that increased production of neurotoxic TRYCATs may contribute to the neuropsychiatric symptoms of major depression, bipolar disorder, schizophrenia, anxiety, somatization disorder, and chronic fatigue syndrome, as well as neurocognitive impairments (Maes et al., 2011a; Cordeiro et al., 2014; Morris et al., 2016; Ormstad et al., 2020; Milaneschi et al., 2021). Nevertheless, no research has investigated whether the physio-affective phenome of Long COVID is associated with IDO activation above and beyond the effects of inflammation and IR.
Consequently, the objectives of the current study are to: (a) examine the association between the Long COVID physio-affective phenome and TRYCAT pathway activity; (b) determine whether the TRYCAT pathway during Long COVID is associated with inflammation during the acute infectious phase; and (c) determine whether TRYCATs have an effect beyond that of CRP and IR.
We employed both a case–control research design (to explore differences between COVID-19 participants who developed mild versus severe Long COVID and those without Long COVID) and a retrospective cohort study design (to examine the effects of acute-phase biomarkers on Long COVID symptoms). We recruited 60 Long COVID patients and 30 control subjects who did not develop Long COVID in the last 3 months of 2022. The mild and severe Long COVID patients were diagnosed using the officially published WHO criteria of post-COVID (Long COVID; WHO, 2021), namely: (a) they suffer from at least two symptoms that impair daily functioning, including fatigue, memory or concentration problems, shortness of breath, chest pain, persistent cough, difficulty speaking, muscle aches, loss of smell or taste, affective symptoms, cognitive impairment, or fever; (b) they have a history of proven SARS-CoV-2 infection, and (c) the symptoms persisted beyond the acute phase of illness or are manifested during recovery from acute COVID-19 infection, lasted at least 2 months and are present 3–4 months after the onset of COVID-19 (WHO, 2021). The 30 controls did not comply with any of these criteria. During the acute phase of the illness, patients and controls were admitted to one of the official quarantine facilities, Al-Muthanna General Hospital and Al-Khidr General Hospital, in Al-Muthanna Governorate, Iraq. Senior doctors and virologists made the diagnosis of SARS-CoV-2 infection and acute COVID-19 based on (a) presence of an acute respiratory syndrome and the disease’s standard symptoms of fever, breathing difficulties, coughing, and loss of smell and taste; (b) positive reverse transcription real-time polymerase chain reaction findings (rRT-PCR); and (c) positive IgM directed to SARS-CoV-2. All patients showed, upon recovery from the acute phase, a negative rRT-PCR result. We selected 30 controls from the same catchment area, who were either staff members or their family or friends. Additionally, we included controls who tested negative for rRT-PCR and exhibited no clinical indications of acute infection, such as dry cough, sore throat, shortness of breath, lack of appetite, flu-like symptoms, fever, night sweats, or chills. Nevertheless, we selected the controls so that about one-third of them had distress or adjustment symptoms as a result of lockdowns and social isolation to account for their confounding effects, which are also seen in Long COVID patients. Thus, one-third of the controls showed Hamilton Depression Rating Scale (HAMD; Hamilton, 1960) values between 7 and 10. COVID patients and controls were excluded if they had a lifetime history of psychiatric axis-1 disorders, including major affective disorders such as major depression and bipolar disorder, dysthymia, generalized anxiety disorder and panic disorder, schizo-affective disorder, schizophrenia, psycho-organic syndrome, and substance use disorders except tobacco use disorder (TUD). Moreover, we excluded patients and controls who suffered from neurodegenerative and neuroinflammatory disorders (based on patients records and lifetime history), such as chronic fatigue syndrome, Parkinson’s or Alzheimer’s disease, multiple sclerosis, stroke, or systemic (auto)immune diseases such as diabetes mellitus, psoriasis, rheumatoid arthritis, inflammatory bowel disease and scleroderma, and liver and renal disease. Additionally, pregnant and breastfeeding women were omitted from this study.
All controls and patients, or their parents/legal guardians, gave written informed permission before participating in the research. The research was approved by the University of Kufa’s institutional ethics board (8298/2022). The study was conducted under Iraqi and international ethical and privacy laws, including the World Medical Association’s Declaration of Helsinki, the Belmont Report, the CIOMS Guideline, and the International Conference on Harmonization of Good Clinical Practice; our institutional review board adheres to the International Guideline for Human Research Safety (ICH-GCP).
A senior psychiatrist used a semi-structured interview to collect socio-demographic and clinical data from controls and Long COVID patients 3–4 months after the acute infectious phase of COVID-19. Three to four months after the onset of acute COVID-19, a senior psychiatrist assessed the following rating scales: (a) the 12-item Fibro-Fatigue (FF) scale to assess chronic fatigue and fibromyalgia symptoms (Zachrisson et al., 2002); (b) the HAMD to assess the severity of depression (Hamilton, 1960); and (c) the Hamilton Anxiety Rating Scale (HAMA; Hamilton, 1959) to assess the severity of anxiety. Two HAMD subdomain scores were calculated: (a) pure depressive symptoms (pure HAMD) as the sum of sad mood + feelings of guilt + suicidal thoughts + loss of interest; and (b) physiosomatic HAMD symptoms (Physiosom HAMD) as the sum of somatic anxiety + gastrointestinal (GIS) anxiety + genitourinary anxiety + hypochondriasis. Two HAMA subdomain scores were calculated: (a) key anxiety symptoms (Key HAMA), which were defined as anxious mood + tension + fears + anxiety behavior during the interview; and (b) physiosomatic HAMA symptoms (Physiosom HAMA), defined as somatic sensory + cardiovascular + GIS + genitourinary + autonomic symptoms (respiratory symptoms were not included in the sum). We calculated a single pure physiosom FF subdomain score as: muscular pain + muscle tension + fatigue + autonomous symptoms + gastrointestinal symptoms + headache + a flu-like malaise (thus excluding the cognitive and affective symptoms). It is of paramount importance to consider both physiosomatic and affective symptom domains because the rating scales used here encompass both type of symptoms. Not considering both symptom domains would not allow us to draw any conclusions on depression and anxiety in relation to the TRYCAT pathway. To construct a score reflecting the severity of the physio-affective phenome, we extracted the first factor from the pure FF and pure and physiosom HAMA and HAMD scores (labeled: the physio-affective phenome; Al-Jassas et al., 2022; Al-Hadrawi et al., 2022). Additionally, we constructed z unit-based composite scores indicating autonomic symptoms, sleep problems, fatigue, gastrointestinal symptoms, and cognitive symptoms using all relevant HAMD, HAMA, and FF items (z transformed). We recorded the immunizations received by each participant, namely AstraZeneca, Pfizer, or Sinopharm. TUD was diagnosed using DSM-5 criteria. We determined the body mass index (BMI) by dividing the body weight in kilograms by the squared height in meters.
Fasting blood samples were taken in the early morning between 7.30 and 9.00 a.m. after awakening and before having breakfast. Five milliliters of venous blood were drawn and transferred into clean plain tubes. Hemolyzed samples were rejected. After 10 min, the clotted blood samples were centrifuged for 5 min at 3,000 rpm, and then the serum was separated and transported into three new Eppendorf tubes until assay. Serum tryptophan, kynurenine, and insulin were measured using ELISA kits supplied by Nanjing Pars Biochem Co., Ltd. (Nanjing, China). Serum 3HK was estimated by ELISA kits supplied by Melsin Medical Co. (Jilin, China). ELISA kits for the estimation of KA and QA were supplied by ELK Biotech. Co., Ltd. (Wuhan, China). All kits have an inter-assay CV of less than 10%. CRP latex slide tests (Spinreact®, Barcelona, Spain) were utilized for CRP measurements in human serum. The serum titer is the reciprocal of the maximum dilution with a positive response multiplied by the positive control concentration. Calculating the patient’s sample’s estimated CRP concentration: six times CRP Titer = mg/L. Serum fasting blood glucose (FBG) was measured spectrophotometrically using a ready-for-use kit supplied by Biolabo® (Maizy, France).
A well-trained paramedical specialist measured SpO2 using an electronic oximeter supplied by Shenzhen Jumper Medical Equipment Co. Ltd., and a digital thermometer was used to assess peak body temperature (PBT; sublingual). We collected these indicators from patient records and analyzed the lowest SpO2 and peak body temperature values recorded during the acute phase of the illness. We created a new indicator based on these two evaluations that represents the severity of inflammation during the acute phase as z PBT – z SpO2. Consequently, we computed some composite scores: (a) the acute phase of infection: z PBT + z duration of acute infection – z SpO2, (b) insulin resistance: z FBG + z insulin (z IR), (c) IDO activity: z KYN – z TRY (labeled KYN/TRY ratio) and the sum of all z transformed TRYCATs, (d) a composite based on z CRP + z KYN/TRY + z IR; and (e) a composite based on acute and Long COVID biomarkers: z CRP + z KYN/TRY + z IR + z PBT – z SpO2. The latter thus reflects the severity of inflammation during acute infection (PBT and SpO2) and IR and inflammation (IDO, CRP) during Long COVID.
ANOVA was performed to determine if there were variations in scale variables across groups, and analysis of contingency tables was employed to determine connections between nominal variables. We used Pearson’s product–moment correlation coefficients to examine relationships between biomarkers and SpO2, PBT, and clinical physio-affective assessments. We employed a univariate general linear model (GLM) analysis to characterize the associations between classifications and biomarkers while accounting for confounding factors such as TUD, sex, age, BMI, and education. Additionally, we obtained model-generated estimated marginal means (SE) values and used protected pairwise comparisons between group means using Fisher’s least significant difference. Multiple comparisons were examined using a p-correction for false discovery rate (FDR; Benjamini and Hochberg, 1995). Multiple regression analysis was used to determine the important TRYCATs, other biomarkers, or cofounders that predict physio-affective measures. We used an automated stepwise technique with a 0.05 value of p to enter and p = 0.06 to remove. We calculated the standardized beta coefficients for each significant explanatory variable using t statistics with exact value of p, as well as the model F statistics and total variance explained (R2) as effect size. Additionally, we examined multicollinearity using the variance inflation factor (VIF) and tolerance. We checked for heteroskedasticity using the White and modified Breusch-Pagan tests, and where necessary, we generated parameter estimates with robust errors. The tests were two-tailed, and statistical significance was defined as a value of p of 0.05. Based on clinical and biomarker analyses, a two-step cluster analysis was done to generate patient clusters. Using a silhouette measure of cohesiveness and separation of more than 0.5, the cluster solution was accepted. Using principal component analysis (PCA), we extracted a first PC that could be validated as a construct if the variance explained is greater than 50.0%, all loadings on the first PC are greater than 0.70, and the anti-image correlation matrix, Kaiser-Meyer-Olkin (KMO), and Bartlett’s sphericity test are satisfactory. We used IBM SPSS 28 for Windows to examine the data. With an effect size of 0.2, an alpha of 0.05, power of 0.8, and five predictors, an a priori power analysis (G*Power 3.1.9.4) determined that the minimum sample size for multiple regression analysis should be at least 70.
We were able to extract one PC from the pure depression and anxiety scores and physiosomatic scores (KMO = 0.820, Bartlett’s test of sphericity: χ2 = 136.85, df = 3, p < 0.001, variance explained = 75.55%, all loadings on the first PC are >0.810). As such, this first PC reflects the severity of the physio-affective phenome of Long COVID. Using two-step cluster analysis performed on the pure depression and anxiety scores and physiosomatic scores, CRP, z IR, z KYN/TRY, SpO2, PBT, and the diagnosis controls versus patients, as a categorial variable, we constructed three clusters (the silhouette measure of cohesion and separation was 0.61 indicating an adequate cluster solution). Table 1 shows the demographic and clinical data in subjects who had suffered from acute COVID-19, and who were divided into those without any symptoms (No Long COVID) and patients with Long COVID divided into two groups: severe and less severe Long COVID.
Table 1. Demographic and clinical data in subjects, 3–12 months after suffering from acute COVID-19, divided into those without any symptoms of Long COVID (No Long COVID), and Long-COVID patients divided into two clusters, namely those with severe (severe Long COVID) and less severe (mild Long COVID) illness.
Table 1 shows that there were no significant differences among groups in age, sex ratio, marital status, residency, employment, BMI, TUD, vaccination type, and time of blood sampling after COVID-19. The duration of the acute infection was greater in severe Long-COVID than in mild Long-COVID and control groups with the lowest duration in the last group. The severe Long-COVID group showed a significantly higher PBT than mild Long-COVID and controls. During the acute phase of COVID infection, SpO2 was lower in the severe Long COVID group compared with the mild Long COVID followed by the control group. Pure depression, pure anxiety, physiosomatic symptoms, fibro-fatigue symptoms, insomnia, physio-affective phenome scores, SpO2, z PBT – z SpO2, and z PBT + z duration – z SpO2 were significantly different between the three groups, and increased from controls ➔ mild Long COVID ➔ severe Long COVID. PBT and cognitive symptoms were higher in patients than in controls.
The biomarker assessments in the three study groups are presented in Table 2. There was no significant difference among the three study groups in serum KYN, KA, QA, 3-HK, and the composite of all four TRYCATs. Severe Long COVID showed significantly higher CRP, z CRP + z KYN/TRY + z IR, and z CRP + z KYN/TRY + z IR + z PBT – z SpO2 scores as compared with the other groups with the lowest values in controls. FBG is significantly higher in the severe Long COVID group compared with controls. Tryptophan is significantly lower in the severe Long COVID group compared with the mild Long COVID group. Serum insulin is higher in patients than in controls. The z KYN/TRY composite is higher in severe Long COVID than in both other groups.
Table 2. Biomarker measurements in subjects 3–12 months after suffering from acute COVID-19, divided into those without any symptoms of Long COVID (No Long COVID), and Long-COVID patients divided into two clusters, namely those with severe (severe Long COVID) and less severe (mild Long COVID) illness.
The intercorrelation matrix of selected neuro-psychiatric symptoms (Fibro-Fatigue, pure anxiety, pure depression, and physiosomatic) and biomarkers is presented in Table 3. The four clinical scores were significantly correlated with indicants of acute COVID-19 infection, CRP, and IR. There were significant associations between TRY (inversely) and the z KYN/TRY composite (positively) and Fibro-Fatigue, pure anxiety, and physiosomatic (but not pure depression) symptoms.
Table 3. Intercorrelation matrix between physio-affective symptom domains and biomarkers and acute COVID-19 and Long COVID.
This table shows that the severity of the acute infectious phase (z PBT – SpO2) is significantly correlated with all four symptom domains, z KYN/TRY, and z CRP + z KYN/TRY + z IR. The latter composite and z CRP + z KYN/TRY + z IR + z PBT – SpO2 were strongly associated with the four symptom domains as shown in Table 3. Moreover, the duration of the acute infectious phase was significantly correlated with the Fibro-Fatigue (r = 0.339, p = 0.001, n = 90), pure anxiety (r = 0.281, p = 0.007), physiosomatic symptoms (r = 0.390, p < 0.001), and z PBT – z SpO2 (r = 0.335, p = 0.001).
Table 4 presents the results of multiple regression analyses with physio-affective symptom domains as dependent variables and biomarkers as explanatory variables. Regression #1 shows that 25.0% of the variance in the fibro-fatigue symptom score was explained by the regression on z IR, CRP, and z KYN/TRY. Figure 1 shows the partial regression of the Fibo-Fatigue score on the z CRP + z KYN/TRY + z IR composite. Regression #2 shows that 20.6% of the variance in the cognitive deficit score was explained by the regression on CRP and z IR. Regression #3 shows that 23.9% of the variance in insomnia score was explained by the regression on CRP, z KYN/TRY, and z IR. Regression #4 shows that a considerable part of the variance in pure anxiety (23.9%) was explained by the regression on z KYN/TRY and CRP. Figure 2 shows the partial regression of the pure anxiety score on the z KYN/TRY ratio. Regression #5 shows that 41.2% of the variance in the pure depression score was explained by CRP, z IR, and z KYN/TRY (all positively associated) and sex. A significant part of the variance of the physiosomatic symptoms score (33.6%) can be explained by the regression on CRP, z KYN/TRY, z IR, and age as seen in Regression #6. Regression #7 shows that 41.0% of the variance in the physio-affective phenome was explained by the regression on age and the composite z CRP + zKYN/TRY + z IR. Figure 3 shows the partial regression of the physio-affective phenome in Long COVID patients on z CRP + z KYN/TRY + z IR.
Table 4. Results of multiple regression analysis with physio-affective symptom domains as dependent variables and biomarkers as explanatory variables.
Figure 1. Partial regression of the Fibro-Fatigue score on the biomarkers of Long COVID (a composite based on C-reactive protein, the kynurenine/tryptophan ratio, and insulin resistance).
Figure 3. Partial regression of the physio-affective phenome of Long COVID on the biomarkers of that condition (a composite based on C-reactive protein, the kynurenine/tryptophan ratio, and insulin resistance).
Table 5 shows the results of the multiple regression analyses with physio-affective symptom domains as dependent variables and the biomarkers of Long COVID (z IR, z KYN/TRY, and CRP) and acute COVID-19 infection (SpO2, PBT, and duration of infection) as explanatory variables. Regression 1 revealed that 32.1% of the variance in the Fibro-Fatigue score can be explained by the regression on z PBT + z duration − z SpO2, CRP, and z IR. Figure 4 shows the partial regression of the Fibro-Fatigue score on a composite based on all acute and Long COVID biomarkers. In regression #2, 27.0% of the variance in the cognition score was explained by the regression on CRP, PBT, and z IR. Regression #3 indicates that the regression on CRP and z PBT – z SpO2 can explain 23.9% of the variance in the insomnia score. According to regression #4, z KYN/TRY, CRP, z duration, and BMI may account for 36.5% of the variation in the pure anxiety score. Regression #5 found that z PBT – z SpO2 and CRP explain together 37.0% of the variation in the pure depression score. The regression on CRP, z PBT + z duration – z SpO2, and z KYN/TRY explained 41.1% of the variance in the physiosomatic symptoms score. Regression #7 shows that the regression on CRP, z PBT + z duration – z SpO2, and z KYN/TRY can explain 42.8% of the variance in the physio-affective phenome score. Figure 4 shows that a large part of the variance in the physio-affective phenome is predicted by the cumulative effects of biomarkers of acute and Long COVID.
Table 5. Results of multiple regression analysis with physio-affective symptom domains as dependent variables and biomarkers, peak body temperature (PBT), and peripheral blood oxygen saturation (SpO2) as explanatory variables.
Figure 4. Partial regression of the Fibro-Fatigue symptoms of Long COVID on the biomarkers of acute COVID-19 (peak body temperature and oxygen saturation) and Long COVID (C-reactive protein, the kynurenine/tryptophan ratio, and insulin resistance).
The first major finding of this study is that a common core (latent vector) was identified from physiosomatic, depressive, and anxiety symptoms, demonstrating that these symptom domains are highly interrelated expressions of the physio-affective phenome of Long COVID. These findings are consistent with our prior findings that a single component drives both physiosomatic and affective symptoms in individuals with Long COVID (Al-Hadrawi et al., 2022; Almulla et al., 2023; Al-Hakeim et al., 2023a). In the present investigation, however, the relationships were calculated in patients who had all suffered from acute COVID-19 infection, while in our prior studies, the connections were calculated in healthy controls and participants with long-term COVID. Prior research, including meta-analyses, has generally confirmed that Long COVID is characterized by physiosomatic and affective symptoms (Huang et al., 2021; Taquet et al., 2021; Badenoch et al., 2022; Premraj et al., 2022; Titze-de-Almeida et al., 2022). In numerous other illnesses, such as rheumatoid arthritis, major depressive disorder, and schizophrenia, we have previously established intertwined associations between high physiosomatic and affective symptom levels (Kanchanatawan et al., 2019; Almulla et al., 2020, 2021; Smesam et al., 2022).
Previously, we demonstrated that the acute infectious phase of COVID-19 was characterized by increases in physiosomatic and affective symptoms and that a common core could be identified from these domains (Al-Jassas et al., 2022). Hence, both acute COVID-19 and Long COVID are linked with significant increases in the physio-affective phenome, indicating that comparable pathways may be responsible for the physio-affective phenome of both acute COVID-19 and Long COVID.
We found that 31.1% of COVID-19 patients experienced moderate to severe symptoms of depression consistent with major depression when a cutoff value of 23 on the BDI-II was used (Park et al., 2020). The mean HAMD, HAMA, and FF scores (23.6 ± 5.6; 25.4 ± 8.6; and 30.9 ± 10.1, respectively) for the group of patients with severe COVID indicate that they also suffer from moderate to severe anxiety and mild chronic fatigue syndrome (CFS). It is important to note that blood samples were collected between 3 and 12 months (mean: 7.3 ± 2.6 months) after remission from the acute phase and that all participants exhibited symptoms that persisted beyond the acute phase of illness, indicating that some long-term COVID patients developed chronic depressive symptoms, chronic major depression, chronic anxiety disorders, and CFS. These results indicate that CFS and affective symptoms belong to the same neuropsychiatric symptom spectrum. In addition, the exclusion of patients with primary depression, anxiety disorders, and CFS from our study suggests that some individuals with COVID-19 develop de novo physiologic and affective symptoms, as well as major depression and CFS, during the chronic phase of the disease. These findings indicate that both major depression and CFS, as well as associated comorbidities, may be induced by viral infections. There is now evidence that viral infections are related to the development of CFS, severe depression, and anxiety (Maes and Twisk, 2010; Bornand et al., 2016; Hampton, 2016; Karimi et al., 2022).
Similar to earlier studies, the severity of the acute phase inflammatory response (as measured by PBT and SpO2) was substantially linked with the physiosomatic and affective symptoms of acute COVID-19 and long-term COVID, showing that common pathways underlie the acute and long-term COVID phenomes. Specifically, the findings of Al-Hadrawi et al. (2022) and the present results indicate that inflammatory pathways modulate the effects of SARS-CoV-2 infection on the acute and long-term COVID physio-affective phenome (Al-Hadrawi et al., 2022).
The second main conclusion of this research is that severe long-term COVID is related to considerably decreased blood levels of tryptophan and a higher KYN/TRY ratio, and that both are associated with anxiety, CFS, and physiosomatic symptoms. Lower levels of tryptophan and a higher ratio of kynurenine to tryptophan were previously related to somatizing diseases and somatizing symptoms rather than depression per se, and the increased ratio in depression is mostly owing to the presence of somatizing symptoms (Maes and Rief, 2012). Hence, one could conclude that the tryptophan-IDO pathway relates to physiosomatic symptoms rather than depressive symptoms. Yet, as previously mentioned, physiosomatic and affective symptoms are expressions of a shared core (i.e., the phenome), and the KYN/TRY ratio adds to the severity of the physio-affective phenome and is elevated in severe Long COVID.
Our findings that tryptophan is decreased and kynurenine is marginally increased in a cluster of severe Long COVID patients and that the KYN/TRY ratio is associated with the physio-affective phenome of Long COVID (after allowing for the effects of the other biomarkers) suggest that IDO activity may be increased in this patient cluster, albeit to a small degree. Nonetheless, the correct interpretation of our findings is that tryptophan is probably reduced due to non-IDO-related mechanisms and that, if IDO activity were to increase in any way, IDO self-inhibition has prevented further activation of the TRYCAT pathway in Long COVID despite the mild chronic inflammatory process.
As a substrate, tryptophan (plasma/serum concentration approximately 12 ng/mL) is readily accessible for kynurenine production (plasma/serum concentration around 3.3 ng/mL). As a result of the decline in tryptophan in long-term COVID patients, the IDO enzyme may self-regulate and become inactive (Nelp et al., 2018), and catalytically inactive ferric IDO1 may accumulate and autooxidize (Booth et al., 2015). In moderately chronic inflammatory circumstances, the latter is likely a self-regulating negative feedback loop that precludes the creation of neurotoxic TRYCATs. In acute inflammatory and infectious circumstances, tryptophan starvation as a result of cytokine-induced tryptophan depletion is an essential component of the innate immune response that protects against invasive infections and hyperinflammation (Maes et al., 2011b; Almulla and Maes, 2022). Nevertheless, increased kynurenine synthesis produces depressogenic, anxious, excitotoxic, and neurotoxic consequences (Wichers et al., 2005; Maes, 2011a). Hence, elevated levels may contribute to the physio-affective phenome, which explains why the KYN/TRY ratio is a more accurate index than tryptophan alone.
In recent meta-analyses, no notable changes in TRYCAT production have been observed in depression and schizophrenia, even though low tryptophan levels are characteristic of both diseases (Almulla et al., 2022c,d). Increased production of TRYCATs appears to be a characteristic of acute inflammatory conditions, such as critical acute COVID-19 infection (Almulla et al., 2023) and IFN-induced depression (Bonaccorso et al., 2002), as opposed to mild chronic inflammatory conditions, such as depression, schizophrenia, and Alzheimer’s disease (Almulla et al., 2022a,c,d). Reduced blood albumin, which binds tryptophan, is likely the most significant mechanism leading to decreased tryptophan in moderately chronic inflammatory diseases (such as depression and schizophrenia; McMenamy and Oncley, 1958; Yuwiler et al., 1977; Maes et al., 1991, 1996, 1997). As the availability of plasma tryptophan controls tryptophan levels in the brain (Fernstrom and Wurtman, 1972; Partridge, 1979), low levels of peripheral tryptophan may contribute to physio-affective symptoms by decreasing central serotonin signaling and decreasing neuroprotective mechanisms (Maes et al., 2011a; Almulla et al., 2022c). In any event, our data indicate that elevated levels of neurotoxic TRYCATs other than kynurenine do not play a significant role in the establishment of Long COVID, thereby contradicting findings in severe COVID-19 infection (Almulla et al., 2022b).
Our findings further indicate that the intensity of the inflammatory response during acute COVID-19 infection (as measured by a rise in PBT and a decrease in SpO2) is predictive of increases in the KYN/TRY ratio during chronic COVID. Hypoxia may reduce the intracellular concentration of serotonin in lung neuroendocrine cells, and the severity of hypoxia influences the extent of serotonin release (Cutz et al., 1993). Consequently, hypoxia during the acute phase of infection may influence the processes of tryptophan depletion and conversion to serotonin. Interestingly, hypoxia increases IDO activity in dendritic cells and hence plays a role in immunological tolerance (Song et al., 2018).
The third major finding of this study is that the three biomarkers of Long COVID assessed in our study (CRP, KYN/TRY, and IR) or acute COVID-19 + Long COVID (CRP, KYN/TRY, IR, SpO2, and PBT) together explain a large part of the variance in the physio-affective phenome of Long COVID (around 40–41%). As such, our findings indicate that the inflammatory processes during acute COVID-19 and the combination of later immune-inflammatory processes (CRP and KYN/TRY) and IR determine to a large extent the physio-affective phenome of Long COVID. Previously, we reported that immune-inflammatory processes (CRP, activation of the NLRP-3 inflammasome, neuro-oxidative pathways), and IR predict the physio-affective phenome of Long COVID (Al-Hadrawi et al., 2022; Al-Hakeim et al., 2023a,b), and that inflammatory processes predict the physio-affective phenome in COVID-19 infection (Al-Jassas et al., 2022). Previously, we have discussed the mechanistic processes that may explain the effects of these different pathways on the onset of physio-affective symptoms, and that the major culprits are: increased neurotoxicity of immune products, neuro-oxidative stress, and increased IR, and lowered neuroprotection by lowered levels of protective antioxidants and tryptophan (Al-Hadrawi et al., 2022; Al-Jassas et al., 2022; Al-Hakeim et al., 2023a,b).
Nevertheless, even more important are our findings that one validated latent construct could be extracted from the three major symptom domains and the composite constructed using the five acute and Long COVID biomarkers (CRP, KYN/TRY, IR, SpO2, and PBT). This indicates that the physio-affective phenome of Long-COVID is the clinical manifestation of immune-inflammatory processes and new onset IR. Likewise, we were able to construct a new endophenotypic cluster of Long COVID patients with extremely low SpO2, increased kynurenine and very low tryptophan (and thus increased KYN/TRY ratio), very high CRP, moderate increases in IR, and very high ratings on all symptom domains. Lowered SpO2 during the acute phase is therefore a key factor in the onset of severe Long COVID.
This article would have been more interesting if we had measured the cytokines known to affect IDO, such as interferons and IL-1β, as well as reactive oxygen species, which may also increase IDO. It would be interesting to assess these biomarkers during the acute infectious phase and Long COVID as well. It would have been more interesting if we also had assessed cortisol levels during the morning hours, as cortisol may stimulate tryptophan-2,3-dioxygenase (TDO), resulting in TRYCAT pathway activation (Maes et al., 1991, 2011b). One may argue that a sample size of 90 is quite small, decreasing the accuracy of the parameter estimations. Nevertheless, a priori computation of the effect size revealed that a sample size of at least 70 is required to perform multiple regression analyses (the primary outcome of our study) assuming an effect size of 0.2, an alpha of 0.05, and a power of 0.80. In addition, post hoc analysis performed on the results of our regression studies revealed that even for the regression with the lowest effect size (see Table 4, cognitive impairments), we operated with a power of 0.991, and that in all other regressions, we attained a power of 1.
We were able to develop an endophenotypic class of severe Long COVID with very low SpO2 during acute infection, and Long COVID biomarkers including low tryptophan, and elevated kynurenine, KYN/TRY ratio, CRP, and a very high score on physiosomatic, Fibro-Fatigue, depression and anxiety symptom domains. The latter domains are all indicators of the shared physio-affective phenome of Long COVID. Around 40% of the variation in this phenome was explained by the Long COVID biomarkers, CRP, KYN/TRY, and IR. The physio-affective phenome of Long COVID is a manifestation of inflammatory reactions during acute and Long COVID, with decreased plasma tryptophan and increased plasma kynurenine potentially contributing to these effects.
The raw data supporting the conclusions of this article will be made available by the authors, without undue reservation.
The studies involving human participants were reviewed and approved by the University of Kufa’s institutional ethics board (8298/2022). The patients/participants provided their written informed consent to participate in this study.
All authors listed have made a substantial, direct, and intellectual contribution to the work and approved it for publication.
This work was supported by an FF66 grant and a Sompoch Endowment Fund from the Faculty of Medicine, MDCU (RA66/016) to MM.
The authors thank the staff of the Dialysis Unit at Al-Hakeem General Hospital and Al-Sader Medical City in Najaf Governorate-Iraq for their help in the collection of samples.
The authors declare that the research was conducted in the absence of any commercial or financial relationships that could be construed as a potential conflict of interest.
All claims expressed in this article are solely those of the authors and do not necessarily represent those of their affiliated organizations, or those of the publisher, the editors and the reviewers. Any product that may be evaluated in this article, or claim that may be made by its manufacturer, is not guaranteed or endorsed by the publisher.
Al-Jassas, H. K., Al-Hakeim, H. K., and Maes, M. (2022). Intersections between pneumonia, lowered oxygen saturation percentage and immune activation mediate depression, anxiety, and chronic fatigue syndrome-like symptoms due to COVID-19: a nomothetic network approach. J. Affect. Disord. 297, 233–245. doi: 10.1016/j.jad.2021.10.039
Al-Hadrawi, D. S., Al-Rubaye, H. T., Almulla, A. F., Al-Hakeim, H. K., and Maes, M. (2022). Lowered oxygen saturation and increased body temperature in acute COVID-19 largely predict chronic fatigue syndrome and affective symptoms due to LONG COVID: a precision nomothetic approach. Acta Neuropsychiat. 297, 1–12. doi: 10.1017/neu.2022.21
Al-Hakeim, H. K., Al-Rubaye, H. T., Al-Hadrawi, D. S., Almulla, A. F., and Maes, M. (2023a). Long-COVID post-viral chronic fatigue and affective symptoms are associated with oxidative damage, lowered antioxidant defenses and inflammation: a proof of concept and mechanism study. Mol. Psychiatry 28, 564–578. doi: 10.1038/s41380-022-01836-9
Al-Hakeim, H. K., Al-Rubaye, H. T., Almulla, A. F., Al-Hadrawi, D. S., and Maes, M. (2023b). Chronic fatigue, depression and anxiety symptoms in long COVID are strongly predicted by Neuroimmune and Neuro-oxidative pathways which are caused by the inflammation during acute infection. J. Clin. Med. 12:511. doi: 10.3390/jcm12020511
Al-Hakeim, H.K., Al-Rubaye, H., Jubran, A., Almulla, A., Moustafa, S., and Maes, M. (2022). Increased insulin resistance due to long COVID is associated with depressive symptoms and partly predicted by the inflammatory response during acute infection. Braz. J. Psychiatry doi: 10.47626/1516-4446-2022-3002 [Epub ahead of print].
Almulla, A. F., Al-Hakeim, H. K., Abed, M. S., Carvalho, A. F., and Maes, M. (2020). Chronic fatigue and fibromyalgia symptoms are key components of deficit schizophrenia and are strongly associated with activated immune-inflammatory pathways. Schizophr. Res. 222, 342–353. doi: 10.1016/j.schres.2020.05.003
Almulla, A. F., Al-Hakeim, H. K., and Maes, M. (2023). Chronic fatigue and affective symptoms in acute and long COVID are attributable to immune-inflammatory pathways. Psychiatry Clin. Neurosci. 77, 125–126. doi: 10.1111/pcn.13514
Almulla, A. F., Al-Rawi, K. F., Maes, M., and Al-Hakeim, H. K. (2021). In schizophrenia, immune-inflammatory pathways are strongly associated with depressive and anxiety symptoms, which are part of a latent trait which comprises neurocognitive impairments and schizophrenia symptoms. J. Affect. Disord. 287, 316–326. doi: 10.1016/j.jad.2021.03.062
Almulla, A. F., and Maes, M. (2022). The tryptophan Catabolite or Kynurenine Pathway's role in major depression. Curr. Top. Med. Chem. 22, 1731–1735. doi: 10.2174/1568026622666220428095250
Almulla, A. F., Supasitthumrong, T., Amrapala, A., Tunvirachaisakul, C., Jaleel, A.-K. K. A., Oxenkrug, G., et al. (2022a). The tryptophan catabolite or kynurenine pathway in Alzheimer’s disease: a systematic review and meta-analysis. J. Alzheimers Dis. 88, 1–15. doi: 10.3233/JAD-220295
Almulla, A. F., Supasitthumrong, T., Tunvirachaisakul, C., Algon, A. A. A., Al-Hakeim, H. K., and Maes, M. (2022b). The tryptophan catabolite or kynurenine pathway in COVID-19 and critical COVID-19: a systematic review and meta-analysis. BMC Infect. Dis. 22, 1–14. doi: 10.1186/s12879-022-07582-1
Almulla, A. F., Thipakorn, Y., Vasupanrajit, A., Tunvirachaisakul, C., Oxenkrug, G., Al-Hakeim, H. K., et al. (2022c). The tryptophan Catabolite or Kynurenine pathway in a major depressive episode with melancholia, psychotic features and suicidal behaviors: a systematic review and Meta-analysis. Cells 11:3112. doi: 10.3390/cells11193112
Almulla, A. F., Vasupanrajit, A., Tunvirachaisakul, C., Al-Hakeim, H. K., Solmi, M., Verkerk, R., et al. (2022d). The tryptophan catabolite or kynurenine pathway in schizophrenia: meta-analysis reveals dissociations between central, serum, and plasma compartments. Mol. Psychiatry 27, 3679–3691. doi: 10.1038/s41380-022-01552-4
Arnold, D. T., Hamilton, F. W., Milne, A., Morley, A. J., Viner, J., Attwood, M., et al. (2021). Patient outcomes after hospitalisation with COVID-19 and implications for follow-up: results from a prospective UK cohort. Thorax 76, 399–401. doi: 10.1136/thoraxjnl-2020-216086
Badenoch, J. B., Rengasamy, E. R., Watson, C., Jansen, K., Chakraborty, S., Sundaram, R. D., et al. (2022). Persistent neuropsychiatric symptoms after COVID-19: a systematic review and meta-analysis. Brain Commun. 4:fcab297. doi: 10.1093/braincomms/fcab297
Benjamini, Y., and Hochberg, Y. (1995). Controlling the false discovery rate: a practical and powerful approach to multiple testing. J. R. Stat. Soc. Ser. B Stat Methodol. 57, 289–300. doi: 10.1111/j.2517-6161.1995.tb02031.x
Bliddal, S., Banasik, K., Pedersen, O. B., Nissen, J., Cantwell, L., Schwinn, M., et al. (2021). Acute and persistent symptoms in non-hospitalized PCR-confirmed COVID-19 patients. Sci. Rep. 11:13153. doi: 10.1038/s41598-021-92045-x
Bonaccorso, S., Marino, V., Biondi, M., Grimaldi, F., Ippoliti, F., and Maes, M. (2002). Depression induced by treatment with interferon-alpha in patients affected by hepatitis C virus. J. Affect. Disord. 72, 237–241. doi: 10.1016/s0165-0327(02)00264-1
Booth, E. S., Basran, J., Lee, M., Handa, S., and Raven, E. L. (2015). Substrate oxidation by indoleamine 2, 3-dioxygenase. J. Biol. Chem. 290, 30924–30930. doi: 10.1074/jbc.M115.695684
Bornand, D., Toovey, S., Jick, S. S., and Meier, C. R. (2016). The risk of new onset depression in association with influenza–a population-based observational study. Brain Behav. Immun. 53, 131–137. doi: 10.1016/j.bbi.2015.12.005
Cordeiro, L., Guimaraes, J., Wanner, S., La Guardia, R., Miranda, R., Marubayashi, U., et al. (2014). Inhibition of tryptophan hydroxylase abolishes fatigue induced by central tryptophan in exercising rats. Scand. J. Med. Sci. Sports 24, 80–88. doi: 10.1111/j.1600-0838.2012.01464.x
Cutz, E., Speirs, V., Yeger, H., Newman, C., Wang, D., and Perrin, D. G. (1993). Cell biology of pulmonary neuroepithelial bodies--validation of an in vitro model. I. Effects of hypoxia and Ca2+ ionophore on serotonin content and exocytosis of dense core vesicles. Anat. Rec. 236, 41–52. doi: 10.1002/ar.1092360109
Davis, H. E., Assaf, G. S., McCorkell, L., Wei, H., Low, R. J., Re'em, Y., et al. (2021). Characterizing long COVID in an international cohort: 7 months of symptoms and their impact. EClinicalMedicine 38:101019. doi: 10.1016/j.eclinm.2021.101019
Fernández-de-Las-Peñas, C. (2022). Long COVID: current definition. Infection 50, 285–286. doi: 10.1007/s15010-021-01696-5
Fernstrom, J., and Wurtman, R. (1972). Elevation of plasma tryptophan by insulin in rat. Metabolism 21, 337–342. doi: 10.1016/0026-0495(72)90078-9
Goldstein, L. E., Leopold, M. C., Huang, X., Atwood, C. S., Saunders, A. J., Hartshorn, M., et al. (2000). 3-Hydroxykynurenine and 3-hydroxyanthranilic acid generate hydrogen peroxide and promote α-crystallin cross-linking by metal ion reduction. Biochemistry 39, 7266–7275. doi: 10.1021/bi992997s
Guo, P., Benito Ballesteros, A., Yeung, S. P., Liu, R., Saha, A., Curtis, L., et al. (2022). COVCOG 2: cognitive and memory deficits in long COVID: a second publication from the COVID and cognition study. Front. Aging Neurosci. 14:804937. doi: 10.3389/fnagi.2022.804937
Hamilton, M. (1959). The assessment of anxiety states by rating. Br. J. Med. Psychol. 32, 50–55. doi: 10.1111/j.2044-8341.1959.tb00467.x
Hamilton, M. (1960). A rating scale for depression. J. Neurol. Neurosurg. Psychiatry 23, 56–62. doi: 10.1136/jnnp.23.1.56
Hampton, T. (2016). How depressed mood may develop after viral infection. JAMA 315:2267. doi: 10.1001/jama.2016.6852
Huang, C., Huang, L., Wang, Y., Li, X., Ren, L., Gu, X., et al. (2021). 6-month consequences of COVID-19 in patients discharged from hospital: a cohort study. Lancet 397, 220–232. doi: 10.1016/S0140-6736(20)32656-8
Kanchanatawan, B., Sriswasdi, S., and Maes, M. (2019). Supervised machine learning to decipher the complex associations between neuro-immune biomarkers and quality of life in schizophrenia. Metab. Brain Dis. 34, 267–282. doi: 10.1007/s11011-018-0339-7
Karimi, Z., Davoodi, Z., Rabiei, L., Jafari, M., Kiani Dehkordi, M., Marzo, R. R., et al. (2022). The potential association of COVID-19 and psychological distress and anxiety in Iran. Int. J. Epidemiol. Health Sci. 3, 1–8. doi: 10.51757/ijehs.3.2022.254985
Liebau, C., Baltzer, A., Schmidt, S., Roesel, C., Karreman, C., Prisack, J. B., et al. (2002). Interleukin-12 and interleukin-18 induce indoleamine 2, 3-dioxygenase (IDO) activity in human osteosarcoma cell lines independently from interferon-gamma. Anticancer Res. 22, 931–936.
Maes, M. (2011a). Depression is an inflammatory disease, but cell-mediated immune activation is the key component of depression. Prog. Neuro-Psychopharmacol. Biol. Psychiatry 35, 664–675. doi: 10.1016/j.pnpbp.2010.06.014
Maes, M. (2011b). An intriguing and hitherto unexplained co-occurrence: depression and chronic fatigue syndrome are manifestations of shared inflammatory, oxidative and nitrosative (IO&NS) pathways. Prog. Neuro-Psychopharmacol. Biol. Psychiatry 35, 784–794. doi: 10.1016/j.pnpbp.2010.06.023
Maes, M., Al-Rubaye, H. T., Almulla, A. F., Al-Hadrawi, D. S., Stoyanova, K., Kubera, M., et al. (2022a). Lowered quality of life in long COVID is predicted by affective symptoms, chronic fatigue syndrome, inflammation and Neuroimmunotoxic pathways. Int. J. Environ. Res. Public Health 19. doi: 10.3390/ijerph191610362
Maes, M., Galecki, P., Verkerk, R., and Rief, W. (2011a). Somatization, but not depression, is characterized by disorders in the tryptophan catabolite (TRYCAT) pathway, indicating increased indoleamine 2, 3-dioxygenase and lowered kynurenine aminotransferase activity. Neuroendocrinol. Lett. 32, 264–273.
Maes, M., Leonard, B., Myint, A., Kubera, M., and Verkerk, R. (2011b). The new ‘5-HT’hypothesis of depression: cell-mediated immune activation induces indoleamine 2, 3-dioxygenase, which leads to lower plasma tryptophan and an increased synthesis of detrimental tryptophan catabolites (TRYCATs), both of which contribute to the onset of depression. Prog. Neuro-Psychopharmacol. Biol. Psychiatry 35, 702–721. doi: 10.1016/j.pnpbp.2010.12.017
Maes, M., Minner, B., and Suy, E. (1991). The relationships between the availability of L-tryptophan to the brain, the spontaneous HPA-axis activity, and the HPA-axis responses to dexamethasone in depressed patients. Amino Acids 1, 57–65. doi: 10.1007/BF00808091
Maes, M., Rachayon, M., Jirakran, K., Sodsai, P., Klinchanhom, S., Gałecki, P., et al. (2022b). The immune profile of major dysmood disorder: proof of concept and mechanism using the precision nomothetic psychiatry approach. Cells 11:1183. doi: 10.3390/cells11071183
Maes, M., and Rief, W. (2012). Diagnostic classifications in depression and somatization should include biomarkers, such as disorders in the tryptophan catabolite (TRYCAT) pathway. Psychiatry Res. 196, 249–243. doi: 10.1016/j.psychres.2011.09.029
Maes, M., and Twisk, F. N. (2010). Treatment of myalgic encephalomyelitis/chronic fatigue syndrome (ME/CFS), a multisystem disease, should target the pathophysiological aberrations (inflammatory and oxidative and nitrosative stress pathways), not the psychosocial “barriers” for a new equilibrium. Patient Educ. Couns. 80, 148–9; author reply 147. doi: 10.1016/j.pec.2010.02.017
Maes, M., Verkerk, R., Vandoolaeghe, E., Van Hunsel, F., Neels, H., Wauters, A., et al. (1997). Serotonin-immune interactions in major depression: lower serum tryptophan as a marker of an immune-inflammatory response. Eur. Arch. Psychiatry Clin. Neurosci. 247, 154–161. doi: 10.1007/BF03033069
Maes, M., Wauters, A., Verkerk, R., Demedts, P., Neels, H., Van Gastel, A., et al. (1996). Lower serum L-tryptophan availability in depression as a marker of a more generalized disorder in protein metabolism. Neuropsychopharmacology 15, 243–251. doi: 10.1016/0893-133X(95)00181-C
McMenamy, R. H., and Oncley, J. (1958). The specific binding of L-tryptophan to serum albumin. J. Biol. Chem. 233, 1436–1447. doi: 10.1016/S0021-9258(18)49353-2
Milaneschi, Y., Allers, K. A., Beekman, A. T., Giltay, E. J., Keller, S., Schoevers, R. A., et al. (2021). The association between plasma tryptophan catabolites and depression: the role of symptom profiles and inflammation. Brain Behav. Immun. 97, 167–175. doi: 10.1016/j.bbi.2021.07.007
Morris, G. F., Carvalho, A., Anderson, G., Galecki, P., and Maes, M. (2016). The many neuroprogressive actions of tryptophan catabolites (TRYCATs) that may be associated with the pathophysiology of neuro-immune disorders. Curr. Pharm. Des. 22, 963–977. doi: 10.2174/1381612822666151215102420
Nelp, M. T., Kates, P. A., Hunt, J. T., Newitt, J. A., Balog, A., Maley, D., et al. (2018). Immune-modulating enzyme indoleamine 2, 3-dioxygenase is effectively inhibited by targeting its apo-form. Proc. Natl. Acad. Sci. 115, 3249–3254. doi: 10.1073/pnas.1719190115
Ormstad, H., Simonsen, C. S., Broch, L., Maes, M., Anderson, G., and Celius, E. G. (2020). Chronic fatigue and depression due to multiple sclerosis: immune-inflammatory pathways, tryptophan catabolites and the gut-brain axis as possible shared pathways. Mult. Scler. Relat. Disord. 46:102533. doi: 10.1016/j.msard.2020.102533
Oxenkrug, G. F. (2007). Genetic and hormonal regulation of tryptophan–kynurenine metabolism: implications for vascular cognitive impairment, major depressive disorder, and aging. Ann. N. Y. Acad. Sci. 1122, 35–49. doi: 10.1196/annals.1403.003
Park, H. Y., Park, W. B., Lee, S. H., Kim, J. L., Lee, J. J., Lee, H., et al. (2020). Posttraumatic stress disorder and depression of survivors 12 months after the outbreak of Middle East respiratory syndrome in South Korea. BMC Public Health 20, 1–9. doi: 10.1186/s12889-020-08726-1
Partridge, W. (1979). The role of blood-brain barrier transport of tryptophan and other amino acids in the regulation of substratelimited pathways of brain amino acid metabolism. J. Neural Transm. 15, 43–54.
Premraj, L., Kannapadi, N. V., Briggs, J., Seal, S. M., Battaglini, D., Fanning, J., et al. (2022). Mid and long-term neurological and neuropsychiatric manifestations of post-COVID-19 syndrome: a meta-analysis. J. Neurol. Sci. 434:120162. doi: 10.1016/j.jns.2022.120162
Reyes Ocampo, J., Lugo Huitrón, R., González-Esquivel, D., Ugalde-Muñiz, P., Jiménez-Anguiano, A., Pineda, B., et al. (2014). Kynurenines with neuroactive and redox properties: relevance to aging and brain diseases. Oxidative Med. Cell. Longev. 2014, 1–22. doi: 10.1155/2014/646909
Shah, W., Hillman, T., Playford, E. D., and Hishmeh, L. (2021). Managing the long term effects of covid-19: summary of NICE, SIGN, and RCGP rapid guideline. BMJ :372. doi: 10.1136/bmj.n136
Smesam, H. N., Qazmooz, H. A., Khayoon, S. Q., Almulla, A. F., Al-Hakeim, H. K., and Maes, M. (2022). Pathway phenotypes underpinning depression, anxiety, and chronic fatigue symptoms due to acute rheumatoid arthritis: a precision nomothetic psychiatry analysis. J. Personal. Med. 12:476. doi: 10.3390/jpm12030476
Smith, A. J., Smith, R. A., and Stone, T. W. (2009). 5-Hydroxyanthranilic acid, a tryptophan metabolite, generates oxidative stress and neuronal death via p38 activation in cultured cerebellar granule neurones. Neurotox. Res. 15, 303–310. doi: 10.1007/s12640-009-9034-0
Song, X., Zhang, Y., Zhang, L., Song, W., and Shi, L. (2018). Hypoxia enhances indoleamine 2,3-dioxygenase production in dendritic cells. Oncotarget 9, 11572–11580. doi: 10.18632/oncotarget.24098
Taquet, M., Geddes, J. R., Husain, M., Luciano, S., and Harrison, P. J. (2021). 6-month neurological and psychiatric outcomes in 236 379 survivors of COVID-19: a retrospective cohort study using electronic health records. Lancet Psychiatry 8, 416–427. doi: 10.1016/S2215-0366(21)00084-5
Titze-de-Almeida, R., da Cunha, T. R., dos Santos Silva, L. D., Ferreira, C. S., Silva, C. P., Ribeiro, A. P., et al. (2022). Persistent, new-onset symptoms and mental health complaints in long COVID in a Brazilian cohort of non-hospitalized patients. BMC Infect. Dis. 22, 1–11. doi: 10.1186/s12879-022-07065-3
Van den Borst, B., Peters, J. B., Brink, M., Schoon, Y., Bleeker-Rovers, C. P., Schers, H., et al. (2021). Comprehensive health assessment 3 months after recovery from acute coronavirus disease 2019 (COVID-19). Clin. Infect. Dis. 73, e1089–e1098. doi: 10.1093/cid/ciaa1750
WHO (2021). "A clinical case definition of post COVID-19 condition by a Delphi consensus, 6 October 2021". World Health Organization.
Wichers, M. C., Koek, G., Robaeys, G., Verkerk, R., Scharpe, S., and Maes, M. (2005). IDO and interferon-α-induced depressive symptoms: a shift in hypothesis from tryptophan depletion to neurotoxicity. Mol. Psychiatry 10, 538–544. doi: 10.1038/sj.mp.4001600
Yong, S. J. (2021). Long COVID or post-COVID-19 syndrome: putative pathophysiology, risk factors, and treatments. Infect. Dis. Ther. 53, 737–754. doi: 10.1080/23744235.2021.1924397
Yuwiler, A., Oldendorf, W., Geller, E., and Braun, L. (1977). Effect of albumin binding and amino acid competition on tryptophan uptake into BRAIN 1. J. Neurochem. 28, 1015–1023. doi: 10.1111/j.1471-4159.1977.tb10664.x
Keywords: neuro-immune, neurotoxicity, inflammation, indoleamine-2,3-dioxygenase, chronic fatigue syndrome, affective symptoms
Citation: Al-Hakeim HK, Khairi Abed A, Rouf Moustafa S, Almulla AF and Maes M (2023) Tryptophan catabolites, inflammation, and insulin resistance as determinants of chronic fatigue syndrome and affective symptoms in long COVID. Front. Mol. Neurosci. 16:1194769. doi: 10.3389/fnmol.2023.1194769
Received: 27 March 2023; Accepted: 16 May 2023;
Published: 02 June 2023.
Edited by:
Mahmoud Kiaei, University of Arkansas for Medical Sciences, United StatesReviewed by:
Marta Kubera, Polish Academy of Sciences, PolandCopyright © 2023 Al-Hakeim, Khairi Abed, Rouf Moustafa, Almulla and Maes. This is an open-access article distributed under the terms of the Creative Commons Attribution License (CC BY). The use, distribution or reproduction in other forums is permitted, provided the original author(s) and the copyright owner(s) are credited and that the original publication in this journal is cited, in accordance with accepted academic practice. No use, distribution or reproduction is permitted which does not comply with these terms.
*Correspondence: Michael Maes, ZHIubWljaGFlbG1hZXNAaG90bWFpbC5jb20=
Disclaimer: All claims expressed in this article are solely those of the authors and do not necessarily represent those of their affiliated organizations, or those of the publisher, the editors and the reviewers. Any product that may be evaluated in this article or claim that may be made by its manufacturer is not guaranteed or endorsed by the publisher.
Research integrity at Frontiers
Learn more about the work of our research integrity team to safeguard the quality of each article we publish.