- 1Department of Neurology, Guangdong Second Provincial General Hospital, Guangzhou, Guangdong, China
- 2Center for Precision Medicine and Division of Cardiovascular Medicine, Department of Medicine, University of Missouri School of Medicine, Columbia, MO, United States
Biomarkers are measurable substances that could be used as objective indicators for disease diagnosis, responses to treatments, and outcomes predictions. In this review, we summarized the data on a number of important biomarkers including glutamate, S100B, glial fibrillary acidic protein, receptor for advanced glycation end-products, intercellular adhesion molecule-1, von willebrand factor, matrix metalloproteinase-9, interleukin-6, tumor necrosis factor-a, activated protein C, copeptin, neuron-specific enolase, tau protein, gamma aminobutyric acid, blood glucose, endothelial progenitor cells, and circulating CD34-positive cells that could be potentially used to indicate the disease burden and/or predict clinical outcome of ischemic stroke. We examined the relationship between specific biomarkers and disease burden and outcomes and discussed the potential mechanisms underlying the relationship. The clinical significance and implications of these biomarkers were also discussed.
1. Introduction
Stroke is the second leading cause of death and long-term disability globally and 87% of the stroke patients are classified as ischemic stroke (IS; Saini et al., 2021). The Global Burden of Diseases, Injuries, and Risk Factors Study (GBD) in 2016 showed that IS patients had to bear substantial economic and social burdens including the significant costs of treatment and post-stroke care, and lost productivities (Johnson et al., 2019). Therefore, there is an urgent need to make early diagnosis of IS, identify the etiology, control the risk factors, and improve the outcomes. Currently, the main factors used for risk stratification and prognosis evaluation in patients with IS include advanced age (Chen et al., 2010), gender (Phan et al., 2017), overweight, hypertension, hyperlipidemia, diabetes mellitus, smoking, atrial fibrillation, stroke, and coronary heart disease (Khan et al., 2007), the Essen Stroke Risk Score (ESRS), and the Stroke Prognostic Instrument-II (SPI-II; Weimar et al., 2010; Meng et al., 2011). However, their predictive values are considered modest.
Biomarkers are measurable substances that could be objectively used to aid the disease diagnosis, evaluate the responses to treatments, and predict the outcomes. In this study, we intended to review a few important blood-based biomarkers that are more economical and feasible. Based on their relationship to stroke, these biomarkers can be associated with the disease burden and outcomes of stroke through one or more mechanisms including astrocyte activation, excitotoxicity, endothelial dysfunction, neuroplasticity or neurotrophicity, inflammation, fibrinolysis, metabolic abnormality, and oxidative stress as discussed below and summarized in Table 1.
2. Pathophysiology of ischemic stroke
Prior to the discussion of specific biomarkers for stroke, it is important to understand the stroke pathophysiology. The “ischemic core” of the ischemic brain tissues is completely cut off from blood supply when vascular occlusion occurs. In the ischemic core, excessive depletion of ATP and failure of aerobic glycolysis often lead to irreversible tissue damage. The region surrounding the core, called the “ischemic penumbra,” is characterized by a significant decrease in cerebral blood flow to the level below functional thresholds. Thus, a metabolic switch to anaerobic metabolism enables neurons and other brain cells in the ischemic penumbra to survive longer despite their functional impairment (Fifield and Vanderluit, 2020). However, cells in the ischemic penumbra could die if cerebral blood flow is not restored in time.
Many time-dependent events occur in the brain during an ischemic stroke, including changes in ion channel function, metabolisms, and cellular activities (Maestrini et al., 2016). Neuronal excitotoxicity and calcium overload occur within minutes of stroke onset as the first early effects of cerebral ischemia. ATP depletion induces the dysfunction of ion channels, leading to the release of glutamate into extracellular compartment (Szydlowska and Tymianski, 2010). Over-activated glutamate receptors increase intracellular calcium, sodium, and water influx, and activate catabolic processes in cells (Belov Kirdajova et al., 2020). Following calcium overload, calcium-dependent enzymes are activated, which results in the formation of superoxide, nitric oxide, and arachidonic acid metabolites (Ludhiadch et al., 2022). The next phase of response or delayed response to ischemia is characterized by inflammation in the ischemic brain tissue within hours of stroke. Cerebral vasculature is the first to be affected by the ischemic insult and develops an inflammatory response to the insult. Thus, the perivascular microglia macrophages release a variety of cytokines, including tumor necrosis factor (TNF)-a and interleukin (IL)-1b, and initiate the inflammatory response with releases of IL-8 and IL-6, resulting in sustained inflammation with increased levels of fibrinogen and C-reactive protein (CRP), and upregulation of cell adhesion molecules including immunoglobulin superfamily [such as intercellular adhesion molecule type 1 (ICAM-1) and vascular adhesion molecule type 1 (VCAM-1)], selectins (such as P- and E-selectin), and integrins (Anrather and Iadecola, 2016; Khoshnam et al., 2017; Sakai and Shichita, 2019). Both microglia and astrocytes play a dual role in IS, with two polarization states: the pro-inflammatory phenotype (M1 and A1) and the anti-inflammatory phenotype (M2 and A2; Qin et al., 2019). Microglia are activated immediately to become M1 phenotype in response to IS, simultaneously promoting astrocyte activation to A1 phenotype. As the disease conditions progress, the microenvironment changes and the reactive astrocytes transform to M2 phenotype that crosstalk with A2 (Liu et al., 2020).
Immediately after stroke, neutrophils are recruited to the brain, followed by monocytes and lymphocytes within 48 h (Fifield and Vanderluit, 2020; Kollikowski et al., 2020; Kömürcü et al., 2020). Proinflammatory cytokines, such as IL-6 and TNF-a, induce the production of matrix metalloproteinase (MMP), especially MMP-9. MMPs then disrupt blood–brain barrier (BBB), and promote the migration of leukocytes, which then interact with endothelium and adhere to vascular walls (Kurzepa et al., 2005; Polavarapu et al., 2005). Edema and hemorrhagic transformation (HT) may arise because of BBB disruption (Wang et al., 2020). Macrophages derived from blood and microglia accumulate in the border zone to clear dead cells and debris, generating proinflammatory mediators and cytotoxic molecules (Sawada, 2009). Cell apoptosis and necrosis are caused by calcium overload, which can persist for hours or days after stroke onset (Ludhiadch et al., 2022; Figure 1).
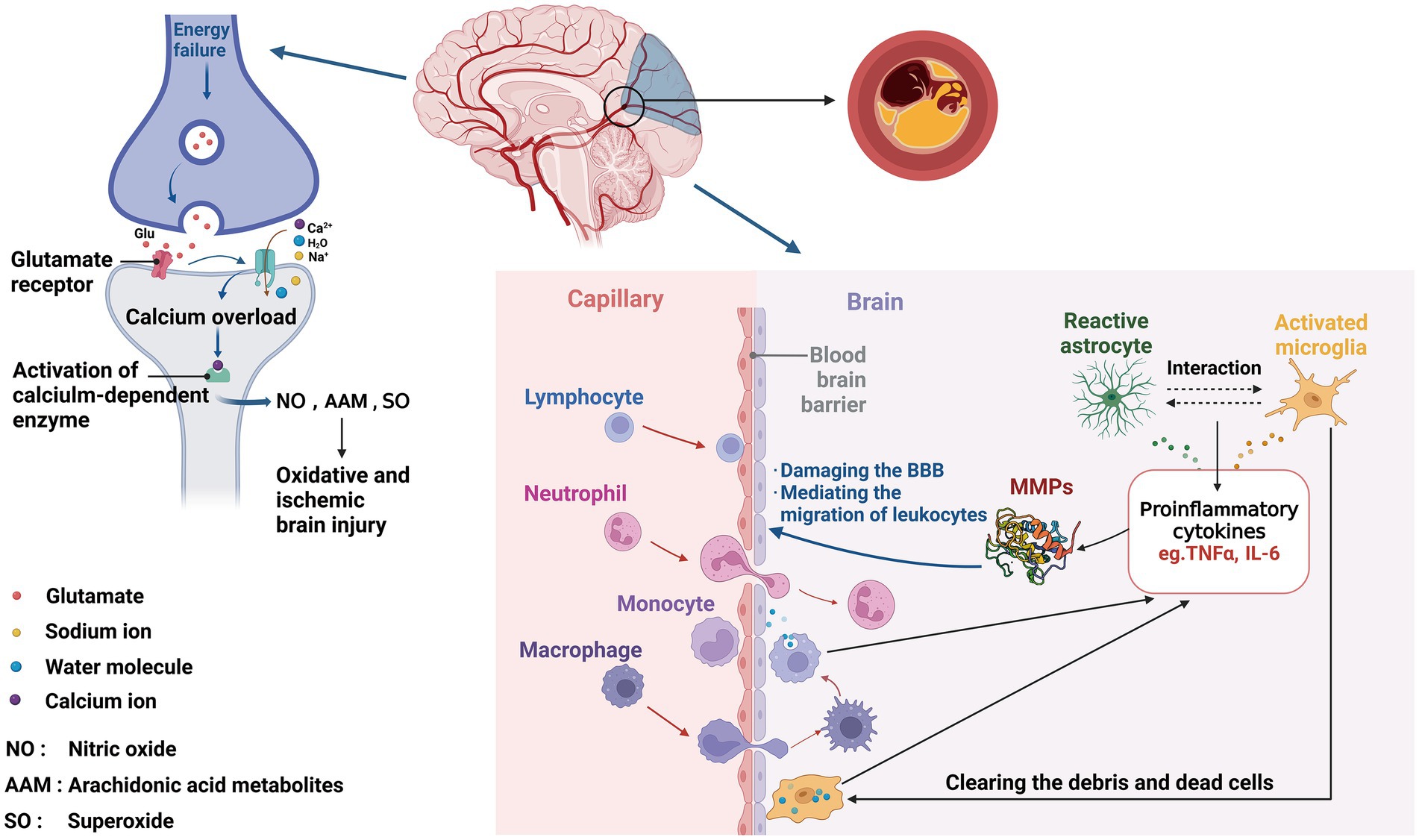
Figure 1. Involvement of biomarkers in ischemic stroke pathophysiology. After ischemic events, neurons suffer from energy failure, inducing the dysfunction of ion channels, leading to the release of glutamate, then increasing intracellular calcium, sodium, and water influx. Following calcium overload, calcium-dependent enzymes are activated, which results in the formation of NO, AAM, and SO, ultimately leading to oxidative and ischemic brain injury. Within the neurovascular unit, microglia get the activated response immediately after IS, with releasing proinflammatory cytokines (TNFɑ, IL-6, and MMP), simultaneously promoting astrocyte activation. Reversely, reactive astrocytes activate microglia, likewise secreting various factors, which damage the BBB, then inducing the migration of peripheral immune cells, leading to secondary BBB damage.
Therefore, a significant number of cell populations and/or bioactive substances are closely associated with various phases of disease process after stroke. Thus, these cell populations and/or bioactive substances could serve as biomarkers for disease burden and/or clinical outcomes of stroke. According to the pathophysiology of ischemic stroke, these potential biomarkers can be classified as markers or indicators relating to excitotoxicity, endothelial dysfunction, astrocyte activation, inflammation, fibrinolysis, neuroplasticity or neurotrophy, metabolic abnormality, and oxidative stress as discussed and summarized in Table 1 below.
3. Specific biomarkers for disease burden and outcomes for patients with IS
3.1. Biomarkers associated with excitotoxicity
3.1.1. Glutamate
Glutamate is one of the most common excitatory neurotransmitters in the central nervous system (CNS) in a healthy subject. It is well known that, during ischemia, glutamate is released in massive amounts from neurons and astrocytes, and acts as an important mediator of neuronal degeneration due to cellular overload of calcium primarily caused by calcium-permeable NMDA receptor (Chao and Li, 2014; Kaplan-Arabaci et al., 2022). As a result of calcium overload, cells necrotize and phospholipids, proteins, and DNA are broken down (Zhivotovsky and Orrenius, 2011). Disruption of energy metabolism and failure of ion channel functions as well as dysfunction of reuptake mechanisms in stroke largely contribute to glutamate accumulation in the extracellular space, making the brain vulnerable to ischemic insult. Glutamate overload in turn leads to prolonged stimulation of NMDA and AMPA ionotropic receptor subtypes, and dramatically enhances calcium, water, and sodium influx into neurons, thus activating intracellular enzymes and triggering neuronal death (Saha et al., 2021). In addition, cortical spreading depolarization (CSD), a high energy consuming electrical activity with a wave of neuronal and glial depolarization across the brain’s gray matter, is triggered by high glutamate and K+ levels extracellularly, resulting in slowly propagating massive depolarization of astrocytes and neurons accompanied by drastic disruptions of ionic gradients.
Studies have revealed that neurologic deterioration of patients with ischemic stroke is positively associated with higher glutamate levels in blood and cerebrospinal fluid (Castellanos et al., 2008). Human plasma glutamate levels correlate with ischemic lesions on CT scans or MRI and neurological outcomes during the first 24 h after stroke (Meng et al., 2015). It has been reported that an increased blood glutamate level is closely related to the development of acute lung injury (ALI), a serious complication of stroke, and an indicator of poor prognosis after stroke (Bai et al., 2017).
3.1.2. Glutamate oxaloacetate transaminase
Glutamate oxaloacetate transaminase (GOT) is a transaminase enzyme that is normally expressed abundantly in the liver and cardiomyocytes (Campos et al., 2011a; Rink et al., 2011). Using oxaloacetate as co-substrate, GOT converts glutamate to 2-ketoglutarate and aspartate. This enzyme can metabolize glutamate in the peripheral blood as well. Under physiological conditions, glutamate is released in synaptic cleft and then quickly removed by the excitatory amino acid transporters (EAATs) in astrocytes and neurons (Divito and Underhill, 2014; Malik and Willnow, 2019). Besides astrocytes and neurons, EAATs have been identified in the endothelial cells of brain vasculature (Hawkins et al., 2006; Hawkins, 2009), indicating a potential important role of vascular endothelial cells in the regulation of glutamate dynamics. In fact, it has been described that glutamate carriers are present in the luminal membrane of endothelial cells and EAATs in the abluminal membrane (Cohen-Kashi-Malina et al., 2012; Hinca et al., 2021). The distributions of glutamate carriers and EAATs in endothelial cells could allow glutamate diffusion from brain tissue to blood following its concentration gradient. Therefore, a decrease in blood glutamate level could reduce the extracellular levels of glutamate in brain (Teichberg et al., 2009). In an animal model of cerebral ischemia, oxaloacetate-mediated GOT activation induced a decrease in blood and cerebral glutamate levels that reduced edema volume, infarct size, and sensorimotor deficits (Campos et al., 2011b). Similarly, clinical studies have revealed a positive association between high levels of blood GOT and favorable outcomes in ischemic stroke patients (Campos et al., 2011c).
3.2. Biomarkers associated with astrocyte activation
3.2.1. S100B
The 21-kDa Ca2+-binding protein S100B is expressed mainly in astrocytes and Schwann cells of the central nervous system (Michetti et al., 2019). S100B levels in peripheral tissues after ischemic stroke have been significantly elevated in patients and animals (Choi et al., 2020; Onatsu et al., 2020; Kadirhan et al., 2022). Mechanistically, previous studies suggested that S100B could be released from activated and/or disrupted glial cells (including cells within ischemic brain tissue) into cerebrospinal fluid (CSF), and with subsequent leaking into the bloodstream via a ruptured blood–brain barrier (BBB; Herrmann et al., 2000; Ahmad et al., 2012).
Data from six longitudinal studies has demonstrated that, although serum levels of S100B are not raised immediately following acute ischemic stroke, a spike of serum S100B levels occurs 3 days after symptom onset of stroke. The data has also shown that serum S100B levels are closely associated with infarct volume and are positively correlated with the risk of malignant infarction or hemorrhagic transformation after thrombolysis as well as with functional outcome in stroke patients (Dassan et al., 2009). Thus, serum S100B levels may be used as a promising marker to identify patients at increased risk of specific early neurological complications like hemorrhagic transformation after stroke and as an indicator of cerebral damage and functional outcome (Foerch et al., 2007). However, further studies are needed to establish the predictive values of serum S100B levels for disease burden and clinical outcomes in stroke patients.
3.2.2. Receptor for advanced glycation end-products
The receptors for advanced glycation end-products (RAGE) are cell membrane bound molecules, and expressed in glia, neurons, and microglia in human cortex and hippocampus (Gasparotto et al., 2019). In addition, RAGE mediates amyloid beta transport across the blood–brain barrier into the CNS, inducing the productions of pro-inflammatory cytokines in microglia and oxidative stress in neurons (Dong et al., 2022). The soluble isoforms of RAGE (sRAGE) represent the extracellular domain of RAGE without cytosolic and trans-membrane domains. Two isoforms of sRAGE exist: cleaved RAGE (cRAGE) and endogenous secretory RAGE (esRAGE). Recently, there is evidence that cRAGE may be the main form of circulating sRAGE after ischemic stroke (IS), and it may independently predict a poor prognosis. It has been shown that plasma sRAGE level is significantly increased in IS patients shortly after the event (<48 h after onset) and then decreased in 5–7 days (Yang et al., 2018; Aida et al., 2021; Zhang et al., 2022).
3.2.3. Glial fibrillary acidic protein
Glial fibrillary acidic protein (GFAP) is a monomeric intermediate filament protein of astroglial skeleton with a molecular weight of between 40 and 53 kDa and is considered brain specific (van Bodegraven et al., 2019, 2021). GFAP is found in the white and gray matters in CNS and is released rapidly from damaged brain tissues (Brenner, 2014; Griffin et al., 2019). Serum GFAP concentrations have been reported to be increased with the highest values at 48 h after stroke onset and remains elevated for at least 5 days after stroke (Wunderlich et al., 2006). In addition, the kinetics of GFAP release are associated with the development and progression of neurological deficits and infarction, and thus may be potentially used as an additional indicator of the early and functional outcomes (Puspitasari et al., 2019). Since the serum levels of GFAP are normally low, structural damage to brain causes a leakage of GFAP from brain tissue to CSF regardless of BBB functional status. Data have shown that serum GFAP levels are associated with poor outcomes after adjustment for stroke severity and infarct volume (Chen et al., 2020; Pujol-Calderón et al., 2022). However, other mechanisms should be considered for increased levels of serum GFAP, including (1) as GFAP is an intermediate filament of the cytoskeleton, it plays an important role in reactive astrogliosis of the CNS (Eng, 1985; Sims and Yew, 2017); (2) functional changes of astrocytes in response to damages to nerve tissues, metabolic abnormalities, and status of neurodegeneration (Siracusa et al., 2019; Li et al., 2022); and (3) response to proinflammatory cytokines (Giovannoni and Quintana, 2020).
3.3. Biomarkers associated with endothelial dysfunction
3.3.1. Intercellular adhesion molecule-1
Intercellular adhesion molecule-1 (ICAM-1, also known as CD54) is a cell surface glycoprotein that is overexpressed in vascular endothelial cells and epithelial cells in response to a variety of inflammatory mediators and activation of the immune system (van de Stolpe and van der Saag, 1996; Bui et al., 2020). Various cytokines produced during the inflammatory process significantly upregulate the expression of ICAM-1, thereby strengthening the adhesion between vascular endothelial cells and activated immune/epithelial cells (Haraldsen et al., 1996; Stanimirovic et al., 1997). ICAM-1 has been shown to be involved in acute inflammation during reperfusion injury, resulting in tissue destruction and brain damage after acute ischemia and subarachnoid hemorrhage. A strong correlation has been reported between prognosis and the soluble adhesion molecules concentrations in the patients with acute spontaneous intracerebral hemorrhage (ICH; Wang et al., 2011). A multivariate analysis has shown that sICAM-1 (soluble intercellular adhesion molecule 1) serum levels on admission are associated with neurological deterioration in stroke patients (Wang et al., 2006).
There is still no explanation for the association between ICAM-1 and neurological deterioration following an ischemic stroke. There are currently three hypotheses: (1) as a vital adhesion molecule, ICAM-1 mediates the neutrophil recruitment and migration effectively, and thus significantly contributing to the edema and inflammatory responses in ischemic brain (Clark et al., 1993; Lindsberg et al., 1996; Simundic et al., 2004). Thus, ICAM-1 level would be expected to be associated with the degree of inflammatory responses and cerebral edema in the lesions. However, a relationship of periphery ICAM-1 levels with the levels of inflammation in CNS in ischemic stroke remains to be established. (2) In early stages of ischemic stroke, ICAM-1 expression is partly regulated by other proinflammatory cytokines (Lee et al., 2000; Frank and Lisanti, 2008; Siniscalchi et al., 2014). Proinflammatory cytokines are directly responsible for increasing ICAM-1 expression in endothelial cells, promoting leukocyte-endothelium interactions, and activating local endothelium to be prothrombotic (Stanimirovic et al., 1997; Zhang, 2008; Maloney and Gao, 2015). And (3) ICAM-1 might be related to post ischemic stroke thrombosis since a recent study has suggested the involvement of ICAM-1 in coagulation (Wang et al., 2006).
3.3.2. Von Willebrand factor
Von Willebrand factor (VWF) is predominantly produced and released from endothelial cells and plays an important role in adhesion and aggregation of platelets as well as in thrombus formation. An increased level of VWF indicates a significant endothelial injury or dysfunction. VWF is a major factor for accumulation and activation of platelets in stenotic arteries, leading to acute thrombotic occlusion (Menih et al., 2017). It has been suggested that high levels of VWF could be associated with increased severity of stroke as well as with poor clinical outcome. Elevated VWF levels immediately after lysis and at 24 h post-therapy have been shown to be an independent prognostic predictor for poor functional outcomes at 90 days for patients with ischemic stroke (Toth et al., 2017). By breaking down VWF, a disintegrin and metalloprotease with thrombospondintype 1 motif, member 13 (ADAMTS13) has been shown to reduce inflammatory reaction and promote ischemic vascular remodeling (Xu et al., 2017). The recombinant ADAMTS13 may potentially become a novel and effective pharmacological agent (Wang L. et al., 2013; Nakano et al., 2015; Chen X. et al., 2019).
3.3.3. CD-34 cells and endothelial progenitor cells
There are three main causes of cerebral small vessel disease (CSVD): lacunar infarction, cerebral microbleeds, or white matter hyperintensities (Wardlaw et al., 2013b; Hilal et al., 2017). Uncertainty exists regarding how CSVD develops and progresses. Brain function declines as a result of abnormalities in cerebral microcirculation related to cerebral microvascular disease (Hilal et al., 2017; Wardlaw et al., 2019). Microcirculation, as well as the vasculature, relies heavily on endothelial cells (Diaz-Otero et al., 2017). Vascular structural and functional integrity is influenced by circulating endothelial progenitor cells (EPCs), which promote endothelial regeneration/repair and contribute to new vascular formation (Tesfamariam, 2016). Moreover, EPCs play a critical role in the integrity of the brain’s blood–brain barrier (BBB) and neurovascular integrity (Rouhl et al., 2008; Huang et al., 2013). Pathogenesis of CSVD is believed to be influenced by BBB dysfunction (Wardlaw, 2010; Wardlaw et al., 2013a). A recent study of 364 patients with CSVD showed that the odds ratio (OR) for subjects with a high CSVD burden was 0.42, and circulating CD34+CD133+CD309+ and CD34+CD133+ cells were significantly higher for patients with a low CSVD burden (Huang et al., 2021). According to these findings, circulating CD34+ cells, CD34+CD133+ cells, and CD34+CD133+CD309+ cells may serve as biomarkers to track CSVD disease progression.
3.4. Biomarkers associated with inflammation
3.4.1. Matrix metalloproteinase-9
The matrix metalloproteinase-9 (MMP-9) belongs to the zinc-dependent MMP family and is essential for extracellular matrix remodeling (Shih et al., 2021; Gross et al., 2022). During cerebral ischemia, MMP-9 expression increases significantly, which is associated with BBB disruption, edema formation, or hemorrhage. As a result of neuronal cell death during an ischemic stroke, inflammation cells infiltrate and activate. The activated inflammatory cells in turn release cytotoxic substances including MMPs, inducing further cell damage and BBB disruption (Abdelnaseer et al., 2017). It has been reported that, although elevated serum levels of MMP-9 in patients with acute ischemic stroke are positively correlated with poor stroke outcomes in the first 24 h after onset of symptoms (Abdelnaseer et al., 2017), the serum MMP-9 level after 1 month of stroke could serve as a positive predictor of stroke recovery beyond the acute stage (Abdelnaseer et al., 2017). Another study also showed that an increase in serum MMP-9 levels during the acute phase of ischemic stroke contributed to an increased mortality and major disability rate (Kots and Weinstein, 2021), suggesting that serum MMP-9 could be a significant predictor of ischemic stroke prognosis.
3.4.2. Tumor necrosis factor-a
Proinflammatory cytokines can activate inflammatory pathways, leading to increased productions (either locally or systematically or both) of nitric oxide, adhesion molecules, TNF-a, and interleukin (IL)-6, thus, contributing to neurotoxicity/neuroprotection (Maestrini et al., 2016). In addition, it is shown that TNF-α from M1 type microglia and TNF receptor 1 (TNFR1) in endothelial cells are critically involved in mediating perivascular M1-like microglia-induced endothelial necroptosis and subsequent BBB disruption (Chen A.Q. et al., 2019). The levels of TNF-a in CSF have been found to be increased in patients at 24 h, 1 week, and 2 weeks after stroke, and correlate with infarct volume and severity of neurological impairment (Shang et al., 2020; Deng et al., 2021). Real-time PCR analysis in rats after t-MCAO has revealed that the mRNA levels for TNF-a, IL-1b, IL-6, E-selectin, and ICAM-1 are increased 3 h after stroke that persist for 24 h in the hemisphere ipsilateral to occlusion (Elango and Devaraj, 2010; Luo et al., 2021).
3.4.3. Interleukin-6
Interleukin-6 (IL-6) is an important inflammatory cytokine that is involved in the pathogenesis of various neurological disorders including strokes by acting as a messenger molecule between vascular endothelium, leukocytes, and parenchymal cells (Kim et al., 1996; Huang et al., 2006; Tuttolomondo et al., 2008). It has been shown that IL-6 is a critical mediator of cerebral ischemia-induced inflammatory responses (Dziedzic et al., 2004; Zeng et al., 2013). In experimental models of brain ischemia, IL-6 can be detected in nerve tissue as early as an hour after the middle cerebral artery (MCA) is occluded (Choi et al., 2010; Maddahi and Edvinsson, 2010). In a cross-sectional descriptive study of 31 acute stroke patients, IL-6 levels correlated significantly with NIHSS and mRS scores in the patients from time of admission to the end of the follow-up period (Mosarrezaii et al., 2020). However, large studies are needed to confirm the finding and to determine if serum IL-6 levels could be used as a predictor of prognosis for patients with ischemic strokes.
3.4.4. Activated protein C
Endogenous circulating serine proteases such as activated protein C (APC) exhibit anti-apoptotic, antithrombotic, anti-inflammatory, and neuroprotective qualities (Mosnier, 2019; Sinha et al., 2019). The protease activated receptor 1 (PAR1) is an important receptor for APC-mediated cell-signaling in many types of CNS and periphery cells. Within the neuro vascular unit, APC activates neuroprotective, vasculoprotective, and anti-inflammatory pathways in brain neurons, microglia, and endothelium, thus, protecting CNS after acute or chronic injuries (Wang Y. et al., 2013), by blocking p53-mediated apoptosis in ischemic human brain endothelial cells (Mendioroz et al., 2009).
3.5. Biomarkers associated with neuroendocrine system—Copeptin
A glycopeptide of 39 amino acids, copeptin is a fragment of the prohormone pre-pro-vasopressin, which is a precursor to vasopressin. Inside the posterior pituitary gland, pre-pro-vasopressin is cleaved into copeptin and vasopressin, which are circulating in equimolar amounts (Blek et al., 2022). The serum copeptin level is a marker of vasopressin secretion due to its high stability, and reflects the degree of activation of hypothalamic–pituitary–adrenal-axis in IS patients (Jalleh and Torpy, 2021). A study has demonstrated that a 24-h plasma copeptin levels after stroke onset are related to mortality and unfavorable functional outcome at 1 year (Spagnolello et al., 2019). Recently, a new prognostic score system “the CoRisk score” was developed for patients with acute ischemic stroke with improved predictive power, based on the blood marker copeptin (de Marchis et al., 2019).
3.6. Others
3.6.1. Neuron-specific enolase
Neuron-specific enolase (NSE) is an intracellular enzyme in neurons and neuroendocrine cells (Isgrò et al., 2015). It is released into the extracellular medium when neuron death occurs, thus making it possible to assess the extent of structural impairment of the biomembranes in CNS (Missler et al., 1997; Oh et al., 2003), serving as a marker of neuron damages. In addition, upon stimulation, cell surfaces can be damaged by NSE as it acts as a plasminogen receptor and induce tissue damage and extracellular matrix degradation, production of pro-inflammatory chemokines/cytokines, and accumulation of inflammatory cells at inflamed and injured sites (Haque et al., 2016). Recently, Kurakina et al. (2021) reported that, along with the severity of neurological symptoms below the score of 15 (NIHSS), a blood NSE concentration of less than 2 ng/mL in patients with acute ischemic stroke at the acute phase predicts a good functional outcome at 12–14 days after disease onset.
3.6.2. Tau protein
Tau protein, a microtubule-associated protein, is mainly expressed in the brain, and to a lesser extent in the heart, lung, kidney and testis (Pluta et al., 2018). It helps to maintain the integrity of neuronal cellular structure. Tau protein is also involved in the regulation of apoptosis, oxidative stress, autophagy, excitotoxicity, angiogenesis, endothelium and inflammation, and mitochondrial function of the brain tissues (Chen and Jiang, 2019). It was observed that, within 6 h after ischemic symptom onset, measurable amount of tau protein was present in the serum and reached its concentration after 3–5 days, then returned to baseline value 3 months later (Bitsch et al., 2002). Test for Tau protein in the serum of patients with ischemic stroke is now considered a potential prognostic factor for more severe neurological deficits and worse functional outcomes in early and late phase of ischemic stroke (Bielewicz et al., 2011).
3.6.3. Gamma aminobutyric acid
Gamma aminobutyric acid (GABA) is a major inhibitory neurotransmitter in CNS (Liu et al., 2018). At the time when glutamate is released in the cerebral regions due to ischemic insult, GABA might be released simultaneously to counteract the neuronal excitotoxicity by hyperpolarizing neuron membrane potential and blocking glutamatergic transmission (Lyden and Lonzo, 1994; Bhattacharya et al., 2014). It has been demonstrated that GABA levels of <240 nmol/L had a positive predictive value for neurological deterioration in acute lacunar infarctions (Serena et al., 2001). There are currently active investigations on therapeutic drugs targeting GABA and its receptor (agonists), such as muscimol, diazepam, and chlormethiazole, to improve stroke outcomes (Serena et al., 2001; Liu et al., 2018).
3.6.4. Blood glucose
Those with hyperglycemia are more likely to suffer a stroke, regardless of whether they have diabetes or not (Gordon et al., 2018; Wang et al., 2018). Acute ischemic stroke (AIS) patients with early neurological deterioration (END) have a poor prognosis (He et al., 2019). It has been found that male patients with acute ischemic stroke have a significantly higher risk for END than female patients, and blood glucose levels at admission are independently associated with END. Subgroup analysis, however, showed that admission glucose levels were significantly related to increased risk of END only for females. An admission blood glucose level of 107.1 mg/dL had a significant predictive value for END prediction, with a specificity of 53% and a sensitivity of 100% in females (Huang et al., 2020). Researchers found that END development in AIS patients might be sex-dependent, with males at a higher risk, and admission glucose levels might be a significant predictor for END in female patients with AIS.
3.6.5. Arginine-vasopressin
Brain edema after stroke is closely related to arginine-vasopressin (AVP), a powerful endogenous hormone that regulates plasma osmolality and volume (Vakili et al., 2005). A significant rise in blood AVP level is associated with an increase in the size of infarct core area and post-stroke edema (Zhao et al., 2015). Although the value of AVP level as a biomarker for predicting the outcome of stroke has yet to be established, experimental therapy using conivaptan, a mixed vasopressin antagonist, has been tested in stroke patients for brain edema management safely (Hedna et al., 2014).
4. Significance and clinical implications
Biomarkers have been playing important roles in disease diagnosis and progression as well as evaluations of treatment response, side/adverse effects, and clinical outcomes for many disease conditions including cardiovascular diseases and cancers. To date, a number of biomarkers of CVDs have been identified, and their relationships with CVDs have been studied to some extent. Some of the biomarkers are specific to neurological system such as NSE, copeptin, and tau protein. However, the use of these markers has not been widely used clinically for predicting the outcomes of stroke very likely due to their limited sensitivity and/or specificity for a single biomarker. Recently, efforts have been made to use a panel of biomarkers to provide more prognostic information on clinical outcomes and function than using a single biomarker (Zhong et al., 2019). A combination of multiple biomarkers (including complement C3, high-sensitive C-reactive protein, hepatocyte growth factor, matrix metalloproteinase-9, and anti-phosphatidylserine antibodies) could substantially improve the risk stratification of adverse outcomes in ischemic stroke patients (Haque et al., 2016).
In addition to conivaptan, a few agents have been under development to target MMP9, including luteolin (Luo et al., 2019), carnosine (Kim et al., 2021), and memantine (Beladi Moghadam et al., 2021), to protect against cerebral ischemic injury.
5. Conclusion
There are many biomarkers for disease burden and clinical outcomes of CVDs. However, the value of most identified biomarkers as a predictor of clinical outcomes or in the management of stroke patients to improve patient’s outcomes remains uncertain. Systematic and well-designed large clinical studies are needed to establish the predictive values of each biomarker and/or their combinations on disease burden and clinical outcomes for patients with CVDs.
6. Limitations
Many epidemiological studies suggest that genetic susceptibility might be associated with stroke (Meschia et al., 2011; Traylor et al., 2021). In this review, no genetic biomarkers were discussed due to their complexity and needs of further validations.
Author contributions
Z-XH and ZL contributed to the conception and design of the study. YH wrote the first draft of the manuscript. ZW and Z-XH designed the figure. Multiple revisions and additions to the final manuscript are contributed by YH, ZL, and Z-XH. All authors contributed to the article and approved the submitted version.
Funding
This work was partially supported by the Science and Technology Program of Guangzhou, China (202201020359).
Acknowledgments
We thank platforms such as PubMed for searching the literature.
Conflict of interest
The authors declare that the research was conducted in the absence of any commercial or financial relationships that could be construed as a potential conflict of interest.
Publisher’s note
All claims expressed in this article are solely those of the authors and do not necessarily represent those of their affiliated organizations, or those of the publisher, the editors and the reviewers. Any product that may be evaluated in this article, or claim that may be made by its manufacturer, is not guaranteed or endorsed by the publisher.
References
Abdelnaseer, M. M., Elfauomy, N. M., Esmail, E. H., Kamal, M. M., and Elsawy, E. H. (2017). Matrix Metalloproteinase-9 and recovery of acute ischemic stroke. J. Stroke Cerebrovasc. Dis. 26, 733–740. doi: 10.1016/j.jstrokecerebrovasdis.2016.09.043
Ahmad, O., Wardlaw, J., and Whiteley, W. N. (2012). Correlation of levels of neuronal and glial markers with radiological measures of infarct volume in ischaemic stroke: a systematic review. Cerebrovasc. Dis. 33, 47–54. doi: 10.1159/000332810
Aida, Y., Kamide, T., Ishii, H., Kitao, Y., Uchiyama, N., Nakada, M., et al. (2021). Soluble receptor for advanced glycation end products as a biomarker of symptomatic vasospasm in subarachnoid hemorrhage. J. Neurosurg. 134, 122–130. doi: 10.3171/2019.8.JNS191269
Anrather, J., and Iadecola, C. (2016). Inflammation and stroke: an overview. Neurotherapeutics 13, 661–670. doi: 10.1007/s13311-016-0483-x
Bai, W., Li, W., Ning, Y. L., Li, P., Zhao, Y., Yang, N., et al. (2017). Blood glutamate levels are closely related to acute lung injury and prognosis after stroke. Front. Neurol. 8:755. doi: 10.3389/fneur.2017.00755
Beladi Moghadam, N., Pourheidar, E., Ahmadpour, F., Kafi, H., Salamzadeh, J., Nasiri, S., et al. (2021). The effects of memantine on the serum concentrations of matrix metalloproteinases and neurologic function of patients with ischemic stroke. J. Clin. Neurosci. 90, 268–272. doi: 10.1016/j.jocn.2021.06.005
Belov Kirdajova, D., Kriska, J., Tureckova, J., and Anderova, M. (2020). Ischemia-triggered glutamate excitotoxicity from the perspective of glial cells. Front. Cell. Neurosci. 14:51. doi: 10.3389/fncel.2020.00051
Bhattacharya, P., Pandey, A. K., Paul, S., and Patnaik, R. (2014). Alleviation of glutamate mediated neuronal insult by piroxicam in rodent model of focal cerebral ischemia: a possible mechanism of GABA agonism. J. Physiol. Biochem. 70, 901–913. doi: 10.1007/s13105-014-0358-8
Bielewicz, J., Kurzepa, J., Czekajska-Chehab, E., Stelmasiak, Z., and Bartosik-Psujek, H. (2011). Does serum tau protein predict the outcome of patients with ischemic stroke? J. Mol. Neurosci. 43, 241–245. doi: 10.1007/s12031-010-9403-4
Bitsch, A., Horn, C., Kemmling, Y., Seipelt, M., Hellenbrand, U., Stiefel, M., et al. (2002). Serum tau protein level as a marker of axonal damage in acute ischemic stroke. Eur. Neurol. 47, 45–51. doi: 10.1159/000047946
Blek, N., Szwed, P., Putowska, P., Nowicka, A., Drela, W. L., Gasecka, A., et al. (2022). The diagnostic and prognostic value of copeptin in patients with acute ischemic stroke and transient ischemic attack: a systematic review and meta-analysis. Cardiol. J. 29, 610–618. doi: 10.5603/CJ.a2022.0045
Brenner, M. (2014). Role of GFAP in CNS injuries. Neurosci. Lett. 565, 7–13. doi: 10.1016/j.neulet.2014.01.055
Bui, T. M., Wiesolek, H. L., and Sumagin, R. (2020). ICAM-1: a master regulator of cellular responses in inflammation, injury resolution, and tumorigenesis. J. Leukoc. Biol. 108, 787–799. doi: 10.1002/JLB.2MR0220-549R
Campos, F., Rodríguez-Yáñez, M., Castellanos, M., Arias, S., Pérez-Mato, M., Sobrino, T., et al. (2011a). Blood levels of glutamate oxaloacetate transaminase are more strongly associated with good outcome in acute ischaemic stroke than glutamate pyruvate transaminase levels. Clin. Sci. (Lond.) 121, 11–17. doi: 10.1042/CS20100427
Campos, F., Sobrino, T., Ramos-Cabrer, P., Argibay, B., Agulla, J., Pérez-Mato, M., et al. (2011b). Neuroprotection by glutamate oxaloacetate transaminase in ischemic stroke: an experimental study. J. Cereb. Blood Flow Metab. 31, 1378–1386. doi: 10.1038/jcbfm.2011.3
Campos, F., Sobrino, T., Ramos-Cabrer, P., Castellanos, M., Blanco, M., Rodríguez-Yáñez, M., et al. (2011c). High blood glutamate oxaloacetate transaminase levels are associated with good functional outcome in acute ischemic stroke. J. Cereb. Blood Flow Metab. 31, 1387–1393. doi: 10.1038/jcbfm.2011.4
Castellanos, M., Sobrino, T., Pedraza, S., Moldes, O., Pumar, J. M., Silva, Y., et al. (2008). High plasma glutamate concentrations are associated with infarct growth in acute ischemic stroke. Neurology 71, 1862–1868. doi: 10.1212/01.wnl.0000326064.42186.7e
Chao, N., and Li, S. T. (2014). Synaptic and extrasynaptic glutamate signaling in ischemic stroke. Curr. Med. Chem. 21, 2043–2064. doi: 10.2174/0929867321666131228204533
Chen, R. L., Balami, J. S., Esiri, M. M., Chen, L. K., and Buchan, A. M. (2010). Ischemic stroke in the elderly: an overview of evidence. Nat. Rev. Neurol. 6, 256–265. doi: 10.1038/nrneurol.2010.36
Chen, C.-H., Cheng, Y. W., Chen, Y. F., Tang, S. C., and Jeng, J. S. (2020). Plasma neurofilament light chain and glial fibrillary acidic protein predict stroke in CADASIL. J. Neuroinflammation 17:124. doi: 10.1186/s12974-020-01813-5
Chen, X., Cheng, X., Zhang, S., and Wu, D. (2019). ADAMTS13: an emerging target in stroke therapy. Front. Neurol. 10:772. doi: 10.3389/fneur.2019.00772
Chen, A. Q., Fang, Z., Chen, X. L., Yang, S., Zhou, Y. F., Mao, L., et al. (2019). Microglia-derived TNF-alpha mediates endothelial necroptosis aggravating blood brain-barrier disruption after ischemic stroke. Cell Death Dis. 10:487. doi: 10.1038/s41419-019-1716-9
Chen, X., and Jiang, H. (2019). Tau as a potential therapeutic target for ischemic stroke. Aging (Albany NY) 11, 12827–12843. doi: 10.18632/aging.102547
Choi, D. W., Kim, T. S., Kim, Y. S., and Kim, D. J. (2020). Elevated plasma biomarkers of inflammation in acute ischemic stroke patients with underlying dementia. BMC Neurol. 20:293. doi: 10.1186/s12883-020-01859-1
Choi, H. S., Ko, Y. G., Lee, J. S., Kwon, O. Y., Kim, S. K., Cheong, C., et al. (2010). Neuroprotective effects of consuming bovine colostrum after focal brain ischemia/reperfusion injury in rat model. Nutr. Res. Pract. 4, 196–202. doi: 10.4162/nrp.2010.4.3.196
Clark, W. M., Coull, B. M., Briley, D. P., Mainolfi, E., and Rothlein, R. (1993). Circulating intercellular adhesion molecule-1 levels and neutrophil adhesion in stroke. J. Neuroimmunol. 44, 123–125. doi: 10.1016/0165-5728(93)90275-4
Cohen-Kashi-Malina, K., Cooper, I., and Teichberg, V. I. (2012). Mechanisms of glutamate efflux at the blood-brain barrier: involvement of glial cells. J. Cereb. Blood Flow Metab. 32, 177–189. doi: 10.1038/jcbfm.2011.121
Dassan, P., Keir, G., and Brown, M. M. (2009). Criteria for a clinically informative serum biomarker in acute ischaemic stroke: a review of S100B. Cerebrovasc. Dis. 27, 295–302. doi: 10.1159/000199468
de Marchis, G. M., Dankowski, T., König, I. R., Fladt, J., Fluri, F., Gensicke, H., et al. (2019). A novel biomarker-based prognostic score in acute ischemic stroke: the CoRisk score. Neurology 92, e1517–e1525. doi: 10.1212/WNL.0000000000007177
Deng, L., Guo, Y., Liu, J., Chen, S., Wang, X., Zhao, H., et al. (2021). Long noncoding RNA ANRIL knockdown attenuates neuroinflammation following ischemic stroke via suppressing the expression of NF-kappa B in vitro and in vivo. Neurol. Res. 43, 767–777. doi: 10.1080/01616412.2021.1934317
Diaz-Otero, J. M., Fisher, C., Downs, K., Moss, M. E., Jaffe, I. Z., Jackson, W. F., et al. (2017). Endothelial mineralocorticoid receptor mediates parenchymal arteriole and posterior cerebral artery remodeling during angiotensin II-induced hypertension. Hypertension 70, 1113–1121. doi: 10.1161/HYPERTENSIONAHA.117.09598
Divito, C. B., and Underhill, S. M. (2014). Excitatory amino acid transporters: roles in glutamatergic neurotransmission. Neurochem. Int. 73, 172–180. doi: 10.1016/j.neuint.2013.12.008
Dong, H., Zhang, Y., Huang, Y., and Deng, H. (2022). Pathophysiology of RAGE in inflammatory diseases. Front. Immunol. 13:931473. doi: 10.3389/fimmu.2022.931473
Dziedzic, T., Gryz, E. A., Turaj, W., Slowik, A., and Szczudlik, A. (2004). Serum interleukin-6 soluble receptor in relation to interleukin-6 in stroke patients. J. Mol. Neurosci. 24, 293–298. doi: 10.1385/JMN:24:2:293
Elango, C., and Devaraj, S. N. (2010). Immunomodulatory effect of hawthorn extract in an experimental stroke model. J. Neuroinflammation 7:97. doi: 10.1186/1742-2094-7-97
Eng, L. F. (1985). Glial fibrillary acidic protein (GFAP): the major protein of glial intermediate filaments in differentiated astrocytes. J. Neuroimmunol. 8, 203–214. doi: 10.1016/S0165-5728(85)80063-1
Fifield, K. E., and Vanderluit, J. L. (2020). Rapid degeneration of neurons in the penumbra region following a small, focal ischemic stroke. Eur. J. Neurosci. 52, 3196–3214. doi: 10.1111/ejn.14678
Foerch, C., Wunderlich, M. T., Dvorak, F., Humpich, M., Kahles, T., Goertler, M., et al. (2007). Elevated serum S100B levels indicate a higher risk of hemorrhagic transformation after thrombolytic therapy in acute stroke. Stroke 38, 2491–2495. doi: 10.1161/STROKEAHA.106.480111
Frank, P. G., and Lisanti, M. P. (2008). ICAM-1: role in inflammation and in the regulation of vascular permeability. Am. J. Physiol. Heart Circ. Physiol. 295, H926–H927. doi: 10.1152/ajpheart.00779.2008
Gasparotto, J., Ribeiro, C. T., da Rosa-Silva, H. T., Bortolin, R. C., Rabelo, T. K., Peixoto, D. O., et al. (2019). Systemic inflammation changes the site of RAGE expression from endothelial cells to neurons in different brain areas. Mol. Neurobiol. 56, 3079–3089. doi: 10.1007/s12035-018-1291-6
Giovannoni, F., and Quintana, F. J. (2020). The role of astrocytes in CNS inflammation. Trends Immunol. 41, 805–819. doi: 10.1016/j.it.2020.07.007
Gordon, W. R., Salamo, R. M., Behera, A., Chibnall, J., Alshekhlee, A., Callison, R. C., et al. (2018). Association of Blood Glucose and Clinical Outcome after mechanical Thrombectomy for acute ischemic stroke. Interv. Neurol. 7, 182–188. doi: 10.1159/000486456
Griffin, J. M., Fackelmeier, B., Fong, D. M., Mouravlev, A., Young, D., and O’Carroll, S. J. (2019). Astrocyte-selective AAV gene therapy through the endogenous GFAP promoter results in robust transduction in the rat spinal cord following injury. Gene Ther. 26, 198–210. doi: 10.1038/s41434-019-0075-6
Gross, K. S., Lincoln, C. M., Anderson, M. K. M., Geiger, G. E., and Frick, K. M. (2022). Extracellular matrix metalloproteinase-9 (MMP-9) is required in female mice for 17β-estradiol enhancement of hippocampal memory consolidation. Psychoneuroendocrinology 141:105773. doi: 10.1016/j.psyneuen.2022.105773
Haque, A., Ray, S. K., Cox, A., and Banik, N. L. (2016). Neuron specific enolase: a promising therapeutic target in acute spinal cord injury. Metab. Brain Dis. 31, 487–495. doi: 10.1007/s11011-016-9801-6
Haraldsen, G., Kvale, D., Lien, B., Farstad, I. N., and Brandtzaeg, P. (1996). Cytokine-regulated expression of E-selectin, intercellular adhesion molecule-1 (ICAM-1), and vascular cell adhesion molecule-1 (VCAM-1) in human microvascular endothelial cells. J. Immunol. 156, 2558–2565. doi: 10.4049/jimmunol.156.7.2558
Hawkins, R. A. (2009). The blood-brain barrier and glutamate. Am. J. Clin. Nutr. 90, 867s–874s. doi: 10.3945/ajcn.2009.27462BB
Hawkins, R. A., O’Kane, R. L., Simpson, I. A., and Viña, J. R. (2006). Structure of the blood-brain barrier and its role in the transport of amino acids. J. Nutr. 136, 218s–226s. doi: 10.1093/jn/136.1.218S
He, L., Wang, J., Zhang, L., Zhang, X., Dong, W., and Yang, H. (2019). Decreased fractal dimension of heart rate variability is associated with early neurological deterioration and recurrent ischemic stroke after acute ischemic stroke. J. Neurol. Sci. 396, 42–47. doi: 10.1016/j.jns.2018.11.006
Hedna, V., Bidari, S., Gubernick, D., Ansari, S., Satriotomo, I., Khan, A. A., et al. (2014). Treatment of stroke related refractory brain edema using mixed vasopressin antagonism: a case report and review of the literature. BMC Neurol. 14:213. doi: 10.1186/s12883-014-0213-0
Herrmann, M., Vos, P., Wunderlich, M. T., de Bruijn, C. H. M. M., and Lamers, K. J. B. (2000). Release of glial tissue-specific proteins after acute stroke: a comparative analysis of serum concentrations of protein S-100B and glial fibrillary acidic protein. Stroke 31, 2670–2677. doi: 10.1161/01.STR.31.11.2670
Hilal, S., Mok, V., Youn, Y. C., Wong, A., Ikram, M. K., and Chen, C. L. H. (2017). Prevalence, risk factors and consequences of cerebral small vessel diseases: data from three Asian countries. J. Neurol. Neurosurg. Psychiatry 88, 669–674. doi: 10.1136/jnnp-2016-315324
Hinca, S. B., Salcedo, C., Wagner, A., Goldeman, C., Sadat, E., Aibar, M. M. D., et al. (2021). Brain endothelial cells metabolize glutamate via glutamate dehydrogenase to replenish TCA-intermediates and produce ATP under hypoglycemic conditions. J. Neurochem. 157, 1861–1875. doi: 10.1111/jnc.15207
Huang, Z. X., Fang, J., Zhou, C. H., Zeng, J., Yang, D., and Liu, Z. (2021). CD34(+) cells and endothelial progenitor cell subpopulations are associated with cerebral small vessel disease burden. Biomark. Med 15, 191–200. doi: 10.2217/bmm-2020-0350
Huang, Z. X., Huang, Y., Zeng, J., Hao, H., Petroski, G. F., Lu, H., et al. (2020). Admission glucose levels may increase the risk for early neurological deterioration in females with acute ischemic stroke. Front. Neurol. 11:548892. doi: 10.3389/fneur.2020.548892
Huang, J., Upadhyay, U. M., and Tamargo, R. J. (2006). Inflammation in stroke and focal cerebral ischemia. Surg. Neurol. 66, 232–245. doi: 10.1016/j.surneu.2005.12.028
Huang, X. T., Zhang, Y. Q., Li, S. J., Li, S. H., Tang, Q., Wang, Z. T., et al. (2013). Intracerebroventricular transplantation of ex vivo expanded endothelial colony-forming cells restores blood-brain barrier integrity and promotes angiogenesis of mice with traumatic brain injury. J. Neurotrauma 30, 2080–2088. doi: 10.1089/neu.2013.2996
Isgrò, M. A., Bottoni, P., and Scatena, R. (2015). Neuron-specific enolase as a biomarker: biochemical and clinical aspects. Adv. Exp. Med. Biol. 867, 125–143. doi: 10.1007/978-94-017-7215-0_9
Jalleh, R., and Torpy, D. J. (2021). The emerging role of Copeptin. Clin. Biochem. Rev. 42, 17–25. doi: 10.33176/AACB-20-00001
Johnson, C. O., Nguyen, M., Roth, G. A., Nichols, E., Alam, T., Abate, D., et al. (2019). Global, regional, and national burden of stroke, 1990–2016: a systematic analysis for the global burden of disease study 2016. Lancet Neurol. 18, 439–458. doi: 10.1016/S1474-4422(19)30034-1
Kadirhan, O. A., Kucukdagli, O. T., and Gulen, B. (2022). The effectiveness of serum S100B, TRAIL and adropin levels in predicting clinical outcome, final infarct core and stroke subtypes of acute ischemic stroke patients. Biomedica 42, 55–63. doi: 10.7705/biomedica.5943
Kaplan-Arabaci, O., Acari, A., Ciftci, P., and Gozuacik, D. (2022). Glutamate scavenging as a neuroreparative strategy in ischemic stroke. Front. Pharmacol. 13:866738. doi: 10.3389/fphar.2022.866738
Khan, U., Porteous, L., Hassan, A., and Markus, H. S. (2007). Risk factor profile of cerebral small vessel disease and its subtypes. J. Neurol. Neurosurg. Psychiatry 78, 702–706. doi: 10.1136/jnnp.2006.103549
Khoshnam, S. E., Winlow, W., Farzaneh, M., Farbood, Y., and Moghaddam, H. F. (2017). Pathogenic mechanisms following ischemic stroke. Neurol. Sci. 38, 1167–1186. doi: 10.1007/s10072-017-2938-1
Kim, E., Kim, E. S., Shin, D., Kim, D., Choi, S., Shin, Y. J., et al. (2021). Carnosine protects against cerebral ischemic injury by inhibiting matrix-Metalloproteinases. Int. J. Mol. Sci. 22:7495. doi: 10.3390/ijms22147495
Kim, J. S., Yoon, S. S., Kim, Y. H., and Ryu, J. S. (1996). Serial measurement of interleukin-6, transforming growth factor-beta, and S-100 protein in patients with acute stroke. Stroke 27, 1553–1557. doi: 10.1161/01.STR.27.9.1553
Kollikowski, A. M., Schuhmann, M. K., Nieswandt, B., Müllges, W., Stoll, G., and Pham, M. (2020). Local leukocyte invasion during Hyperacute human ischemic stroke. Ann. Neurol. 87, 466–479. doi: 10.1002/ana.25665
Kömürcü, H. F., Gözke, E., Doğan Ak, P., Kalyoncu Aslan, I., Salt, I., and Özgenç Bi¸er, Ç. İ. (2020). Changes in neutrophil, lymphocyte, platelet ratios and their relationship with NIHSS after rtPA and/or thrombectomy in ischemic stroke. J. Stroke Cerebrovasc. Dis. 29:105004. doi: 10.1016/j.jstrokecerebrovasdis.2020.105004
Kots, E., and Weinstein, H. (2021). Computational account of the first stages in the elevator motion of the human EAAT3 glutamate transporter. Biophys. J. 120, 152A–153A. doi: 10.1016/j.bpj.2020.11.1108
Kurakina, А., Semenova, T. N., Guzanova, E. V., Nesterova, V. N., Schelchkova, N. A., Mukhina, I. V., et al. (2021). Prognostic value of investigating neuron-specific enolase in patients with ischemic stroke. Sovrem Tekhnologii Med 13, 68–72. doi: 10.17691/stm2021.13.2.08
Kurzepa, J., Bartosik-Psujek, H., Suchozebrska-Jesionek, D., Rejdak, K., Stryjecka-Zimmer, M., and Stelmasiak, Z. (2005). Role of matrix metalloproteinases in the pathogenesis of multiple sclerosis. Neurol. Neurochir. Pol. 39, 63–67.
Lee, S. J., Drabik, K., van Wagoner, N. J., Lee, S., Choi, C., Dong, Y., et al. (2000). ICAM-1-induced expression of proinflammatory cytokines in astrocytes: involvement of extracellular signal-regulated kinase and p38 mitogen-activated protein kinase pathways. J. Immunol. 165, 4658–4666. doi: 10.4049/jimmunol.165.8.4658
Li, L., Zhou, J., Han, L., Wu, X., Shi, Y., Cui, W., et al. (2022). The specific role of reactive astrocytes in stroke. Front. Cell. Neurosci. 16:850866. doi: 10.3389/fncel.2022.850866
Lindsberg, P. J., Carpe’n, O., Paetau, A., Karjalainen-Lindsberg, M. L., and Kaste, M. (1996). Endothelial ICAM-1 expression associated with inflammatory cell response in human ischemic stroke. Circulation 94, 939–945. doi: 10.1161/01.CIR.94.5.939
Liu, L. R., Liu, J. C., Bao, J. S., Bai, Q. Q., and Wang, G. Q. (2020). Interaction of microglia and astrocytes in the neurovascular unit. Front. Immunol. 11:1024. doi: 10.3389/fimmu.2020.01024
Liu, J., Zhang, J., and Wang, L. N. (2018). Gamma aminobutyric acid (GABA) receptor agonists for acute stroke. Cochrane Database Syst. Rev. 2018:Cd009622. doi: 10.1002/14651858.CD009622.pub5
Ludhiadch, A., Sharma, R., Muriki, A., and Munshi, A. (2022). Role of calcium homeostasis in ischemic stroke: a review. CNS Neurol. Disord. Drug Targets 21, 52–61. doi: 10.2174/1871527320666210212141232
Luo, C., Chen, Q., Liu, B., Wang, S., Yu, H., Guan, X., et al. (2021). The extracts of Angelica sinensis and Cinnamomum cassia from oriental medicinal foods regulate inflammatory and Autophagic pathways against neural injury after ischemic stroke. Oxidative Med. Cell. Longev. 2021, 1–15. doi: 10.1155/2021/9663208
Luo, S., Li, H., Mo, Z., Lei, J., Zhu, L., Huang, Y., et al. (2019). Connectivity map identifies luteolin as a treatment option of ischemic stroke by inhibiting MMP9 and activation of the PI3K/Akt signaling pathway. Exp. Mol. Med. 51, 1–11. doi: 10.1038/s12276-019-0229-z
Lyden, P. D., and Lonzo, L. (1994). Combination therapy protects ischemic brain in rats. A glutamate antagonist plus a gamma-aminobutyric acid agonist. Stroke 25, 189–196. doi: 10.1161/01.STR.25.1.189
Maddahi, A., and Edvinsson, L. (2010). Cerebral ischemia induces microvascular pro-inflammatory cytokine expression via the MEK/ERK pathway. J. Neuroinflammation 7:14. doi: 10.1186/1742-2094-7-14
Maestrini, I., Ducroquet, A., Moulin, S., Leys, D., Cordonnier, C., and Bordet, R. (2016). Blood biomarkers in the early stage of cerebral ischemia. Rev. Neurol. (Paris) 172, 198–219. doi: 10.1016/j.neurol.2016.02.003
Malik, A. R., and Willnow, T. E. (2019). Excitatory amino acid transporters in physiology and disorders of the central nervous system. Int. J. Mol. Sci. 20:5671. doi: 10.3390/ijms20225671
Maloney, J. P., and Gao, L. (2015). Proinflammatory cytokines increase vascular endothelial growth factor expression in alveolar epithelial cells. Mediat. Inflamm. 2015:387842. doi: 10.1155/2015/387842
Mendioroz, M., Fernández-Cadenas, I., Alvarez-Sabín, J., Rosell, A., Quiroga, D., Cuadrado, E., et al. (2009). Endogenous activated protein C predicts hemorrhagic transformation and mortality after tissue plasminogen activator treatment in stroke patients. Cerebrovasc. Dis. 28, 143–150. doi: 10.1159/000225907
Meng, X. E., Li, N., Guo, D. Z., Pan, S. Y., Li, H., and Yang, C. (2015). High plasma glutamate levels are associated with poor functional outcome in acute ischemic stroke. Cell. Mol. Neurobiol. 35, 159–165. doi: 10.1007/s10571-014-0107-0
Meng, X., Wang, Y., Zhao, X., Wang, C., Li, H., Liu, L., et al. (2011). Validation of the Essen stroke risk score and the stroke prognosis instrument II in Chinese patients. Stroke 42, 3619–3620. doi: 10.1161/STROKEAHA.111.624148
Menih, M., Krizmaric, M., and Fabjan, T. H. (2017). Clinical role of von willebrand factor in acute ischemic stroke. Wien. Klin. Wochenschr. 129, 491–496. doi: 10.1007/s00508-017-1200-4
Meschia, J. F., Worrall, B. B., and Rich, S. S. (2011). Genetic susceptibility to ischemic stroke. Nat. Rev. Neurol. 7, 369–378. doi: 10.1038/nrneurol.2011.80
Michetti, F., D'Ambrosi, N., Toesca, A., Puglisi, M. A., Serrano, A., Marchese, E., et al. (2019). The S100B story: from biomarker to active factor in neural injury. J. Neurochem. 148, 168–187. doi: 10.1111/jnc.14574
Missler, U., Wiesmann, M., Friedrich, C., and Kaps, M. (1997). S-100 protein and neuron-specific enolase concentrations in blood as indicators of infarction volume and prognosis in acute ischemic stroke. Stroke 28, 1956–1960. doi: 10.1161/01.STR.28.10.1956
Mosarrezaii, A., Amiri-Nikpour, M. R., Mehryar, H. R., Choobi Anzali, B., Nourooz-Zadeh, S., Babaei, S., et al. (2020). Investigating the relationship between interleukin-6 serum levels and outcome in acute ischemic CVA. Brain Behav. 10:e01668. doi: 10.1002/brb3.1668
Mosnier, L. O. (2019). Activated protein C in neuroprotection and malaria. Curr. Opin. Hematol. 26, 320–330. doi: 10.1097/MOH.0000000000000528
Nakano, T., Irie, K., Hayakawa, K., Sano, K., Nakamura, Y., Tanaka, M., et al. (2015). Delayed treatment with ADAMTS13 ameliorates cerebral ischemic injury without hemorrhagic complication. Brain Res. 1624, 330–335. doi: 10.1016/j.brainres.2015.07.027
Oh, S. H., Lee, J. G., Na, S. J., Park, J. H., Choi, Y. C., and Kim, W. J. (2003). Prediction of early clinical severity and extent of neuronal damage in anterior-circulation infarction using the initial serum neuron-specific enolase level. Arch. Neurol. 60, 37–41. doi: 10.1001/archneur.60.1.37
Onatsu, J., Vanninen, R., Jäkälä, P., Mustonen, P., Pulkki, K., Korhonen, M., et al. (2020). Tau, S100B and NSE as blood biomarkers in acute cerebrovascular events. In Vivo 34, 2577–2586. doi: 10.21873/invivo.12075
Phan, H. T., Blizzard, C. L., Reeves, M. J., Thrift, A. G., Cadilhac, D., Sturm, J., et al. (2017). Sex differences in long-term mortality after stroke in the INSTRUCT (INternational STRoke oUtComes sTudy): a meta-analysis of individual participant data. Circ. Cardiovasc. Qual. Outcomes 10:e003436. doi: 10.1161/CIRCOUTCOMES.116.003436
Pluta, R., Ułamek-Kozioł, M., Januszewski, S., and Czuczwar, S. J. (2018). Tau protein dysfunction after brain ischemia. J. Alzheimers Dis. 66, 429–437. doi: 10.3233/JAD-180772
Polavarapu, R., Gongora, M. C., Winkles, J. A., and Yepes, M. (2005). Tumor necrosis factor-like weak inducer of apoptosis increases the permeability of the neurovascular unit through nuclear factor-kappa B pathway activation. J. Neurosci. 25, 10094–10100. doi: 10.1523/JNEUROSCI.3382-05.2005
Pujol-Calderón, F., Zetterberg, H., Portelius, E., Löwhagen Hendén, P., Rentzos, A., Karlsson, J. E., et al. (2022). Prediction of outcome after endovascular Embolectomy in anterior circulation stroke using biomarkers. Transl. Stroke Res. 13, 65–76. doi: 10.1007/s12975-021-00905-5
Puspitasari, V., Gunawan, P. Y., Wiradarma, H. D., and Hartoyo, V. (2019). Glial fibrillary acidic protein serum level as a predictor of clinical outcome in ischemic stroke. Open Access Maced. J. Med. Sci. 7, 1471–1474. doi: 10.3889/oamjms.2019.326
Qin, C., Zhou, L. Q., Ma, X. T., Hu, Z. W., Yang, S., Chen, M., et al. (2019). Dual functions of microglia in ischemic stroke. Neurosci. Bull. 35, 921–933. doi: 10.1007/s12264-019-00388-3
Rink, C., Gnyawali, S., Peterson, L., and Khanna, S. (2011). Oxygen-inducible glutamate oxaloacetate transaminase as protective switch transforming neurotoxic glutamate to metabolic fuel during acute ischemic stroke. Antioxid. Redox Signal. 14, 1777–1785. doi: 10.1089/ars.2011.3930
Rouhl, R. P., van Oostenbrugge, R. J., Damoiseaux, J., Tervaert, J. W. C., and Lodder, J. (2008). Endothelial progenitor cell research in stroke: a potential shift in pathophysiological and therapeutical concepts. Stroke 39, 2158–2165. doi: 10.1161/STROKEAHA.107.507251
Saha, K., Yang, J. W., Hofmaier, T., Venkatesan, S. K., Steinkellner, T., Kudlacek, O., et al. (2021). Constitutive endocytosis of the neuronal glutamate transporter excitatory amino acid Transporter-3 requires ARFGAP1. Front. Physiol. 12:671034. doi: 10.3389/fphys.2021.671034
Saini, V., Guada, L., and Yavagal, D. R. (2021). Global epidemiology of stroke and access to acute ischemic stroke interventions. Neurology 97, S6–s16. doi: 10.1212/WNL.0000000000012781
Sakai, S., and Shichita, T. (2019). Inflammation and neural repair after ischemic brain injury. Neurochem. Int. 130:104316. doi: 10.1016/j.neuint.2018.10.013
Sawada, M. (2009). Neuroprotective and toxic changes in microglia in neurodegenerative disease. Parkinsonism Relat. Disord. 15, S39–S41. doi: 10.1016/S1353-8020(09)70011-2
Serena, J., Leira, R., Castillo, J.́., Pumar, J.́. M., Castellanos, M., and Dávalos, A. (2001). Neurological deterioration in acute lacunar infarctions: the role of excitatory and inhibitory neurotransmitters. Stroke 32, 1154–1161. doi: 10.1161/01.STR.32.5.1154
Shang, J., Cao, D., Chen, Z., Shangguan, L., Wang, H., Cui, X., et al. (2020). NARINGENIN protects cerebral ischemic injury by inhibiting mitochondria-mediated neuronal apoptosis in acute ischemic stroke rats. Acta Pol. Pharm. 77, 609–617. doi: 10.32383/appdr/126314
Shih, Y. C., Chen, P. Y., Ko, T. M., Huang, P. H., Ma, H., and Tarng, D. C. (2021). MMP-9 deletion attenuates arteriovenous fistula Neointima through reduced perioperative vascular inflammation. Int. J. Mol. Sci. 22:5448. doi: 10.3390/ijms22115448
Sims, N. R., and Yew, W. P. (2017). Reactive astrogliosis in stroke: contributions of astrocytes to recovery of neurological function. Neurochem. Int. 107, 88–103. doi: 10.1016/j.neuint.2016.12.016
Simundic, A. M., Basic, V., Topic, E., Demarin, V., Vrkic, N., Kunovic, B., et al. (2004). Soluble adhesion molecules in acute ischemic stroke. Clin. Invest. Med. 27, 86–92.
Sinha, R. K., Flynn, R., Zaiken, M., Paz, K., Gavin, A. L., Nemazee, D., et al. (2019). Activated protein C ameliorates chronic graft-versus-host disease by PAR1-dependent biased cell signaling on T cells. Blood 134, 776–781. doi: 10.1182/blood.2019001259
Siniscalchi, A., Gallelli, L., Malferrari, G., Pirritano, D., Serra, R., Santangelo, E., et al. (2014). Cerebral stroke injury: the role of cytokines and brain inflammation. J. Basic Clin. Physiol. Pharmacol. 25, 131–137. doi: 10.1515/jbcpp-2013-0121
Siracusa, R., Fusco, R., and Cuzzocrea, S. (2019). Astrocytes: role and functions in brain pathologies. Front. Pharmacol. 10:1114. doi: 10.3389/fphar.2019.01114
Spagnolello, O., de Michele, M., Lorenzano, S., Cerulli Irelli, E., Naitana, F., Falcou, A., et al. (2019). Copeptin kinetics in acute ischemic stroke may differ according to revascularization strategies: pilot data. Stroke 50, 3632–3635. doi: 10.1161/STROKEAHA.119.025433
Stanimirovic, D. B., Wong, J., Shapiro, A., and Durkin, J. P. (1997). Increase in surface expression of ICAM-1, VCAM-1 and E-selectin in human cerebromicrovascular endothelial cells subjected to ischemia-like insults. Acta Neurochir. Suppl. 70, 12–16.
Szydlowska, K., and Tymianski, M. (2010). Calcium, ischemia and excitotoxicity. Cell Calcium 47, 122–129. doi: 10.1016/j.ceca.2010.01.003
Teichberg, V. I., Cohen-Kashi-Malina, K., Cooper, I., and Zlotnik, A. (2009). Homeostasis of glutamate in brain fluids: an accelerated brain-to-blood efflux of excess glutamate is produced by blood glutamate scavenging and offers protection from neuropathologies. Neuroscience 158, 301–308. doi: 10.1016/j.neuroscience.2008.02.075
Tesfamariam, B. (2016). Endothelial repair and regeneration following intimal injury. J. Cardiovasc. Transl. Res. 9, 91–101. doi: 10.1007/s12265-016-9677-1
Toth, N. K., Székely, E. G., Czuriga-Kovács, K. R., Sarkady, F., Nagy, O., Lánczi, L. I., et al. (2017). Elevated factor VIII and von Willebrand factor levels predict Unfavorable outcome in stroke patients treated with intravenous thrombolysis. Front. Neurol. 8:721. doi: 10.3389/fneur.2017.00721
Traylor, M., Persyn, E., Tomppo, L., Klasson, S., Abedi, V., Bakker, M. K., et al. (2021). Genetic basis of lacunar stroke: a pooled analysis of individual patient data and genome-wide association studies. Lancet Neurol. 20, 351–361. doi: 10.1016/S1474-4422(21)00031-4
Tuttolomondo, A., Di Raimondo, D., di Sciacca, R., Pinto, A., and Licata, G. (2008). Inflammatory cytokines in acute ischemic stroke. Curr. Pharm. Des. 14, 3574–3589. doi: 10.2174/138161208786848739
Vakili, A., Kataoka, H., and Plesnila, N. (2005). Role of arginine vasopressin V1 and V2 receptors for brain damage after transient focal cerebral ischemia. J. Cereb. Blood Flow Metabol. 25, 1012–1019. doi: 10.1038/sj.jcbfm.9600097
van Bodegraven, E. J., Asperen, J. V., Robe, P. A. J., and Hol, E. M. (2019). Importance of GFAP isoform-specific analyses in astrocytoma. Glia 67, 1417–1433. doi: 10.1002/glia.23594
van Bodegraven, E. J., Sluijs, J. A., Tan, A. K., Robe, P. A. J. T., and Hol, E. M. (2021). New GFAP splice isoform (GFAPμ) differentially expressed in glioma translates into 21 kDa N-terminal GFAP protein. FASEB J. 35:e21389. doi: 10.1096/fj.202001767R
van de Stolpe, A., and van der Saag, P. T. (1996). Intercellular adhesion molecule-1. J. Mol. Med. 74, 13–33. doi: 10.1007/BF00202069
Wang, L., Deng, L., Yuan, R., Liu, J., Li, Y., and Liu, M. (2020). Association of matrix metalloproteinase 9 and cellular fibronectin and outcome in acute ischemic stroke: a systematic review and Meta-analysis. Front. Neurol. 11:523506. doi: 10.3389/fneur.2020.523506
Wang, L., Fan, W., Cai, P., Fan, M., Zhu, X., Dai, Y., et al. (2013). Recombinant ADAMTS13 reduces tissue plasminogen activator-induced hemorrhage after stroke in mice. Ann. Neurol. 73, 189–198. doi: 10.1002/ana.23762
Wang, F., Jiang, B., Kanesan, L., Zhao, Y., and Yan, B. (2018). Higher admission fasting plasma glucose levels are associated with a poorer short-term neurologic outcome in acute ischemic stroke patients with good collateral circulation. Acta Diabetol. 55, 703–714. doi: 10.1007/s00592-018-1139-6
Wang, H. C., Lin, W. C., Lin, Y. J., Rau, C. S., Lee, T. H., Chang, W. N., et al. (2011). The association between serum adhesion molecules and outcome in acute spontaneous intracerebral hemorrhage. Crit. Care 15:R284. doi: 10.1186/cc10568
Wang, Y., Zhao, Z., Chow, N., Ali, T., Griffin, J. H., and Zlokovic, B. V. (2013). Activated protein C analog promotes neurogenesis and improves neurological outcome after focal ischemic stroke in mice via protease activated receptor 1. Brain Res. 1507, 97–104. doi: 10.1016/j.brainres.2013.02.023
Wang, J. Y., Zhou, D. H. D., Li, J., Zhang, M., Deng, J., Gao, C., et al. (2006). Association of soluble intercellular adhesion molecule 1 with neurological deterioration of ischemic stroke: the Chongqing stroke study. Cerebrovasc. Dis. 21, 67–73. doi: 10.1159/000090005
Wardlaw, J. M. (2010). Blood-brain barrier and cerebral small vessel disease. J. Neurol. Sci. 299, 66–71. doi: 10.1016/j.jns.2010.08.042
Wardlaw, J. M., Doubal, F. N., Valdes-Hernandez, M., Wang, X., Chappell, F. M., Shuler, K., et al. (2013a). Blood-brain barrier permeability and long-term clinical and imaging outcomes in cerebral small vessel disease. Stroke 44, 525–527. doi: 10.1161/STROKEAHA.112.669994
Wardlaw, J. M., Smith, E. E., Biessels, G. J., Cordonnier, C., Fazekas, F., Frayne, R., et al. (2013b). Neuroimaging standards for research into small vessel disease and its contribution to ageing and neurodegeneration. Lancet Neurol. 12, 822–838. doi: 10.1016/S1474-4422(13)70124-8
Wardlaw, J. M., Smith, C., and Dichgans, M. (2019). Small vessel disease: mechanisms and clinical implications. Lancet Neurol. 18, 684–696. doi: 10.1016/S1474-4422(19)30079-1
Weimar, C., Benemann, J., Michalski, D., Müller, M., Luckner, K., Katsarava, Z., et al. (2010). Prediction of recurrent stroke and vascular death in patients with transient ischemic attack or nondisabling stroke: a prospective comparison of validated prognostic scores. Stroke 41, 487–493. doi: 10.1161/STROKEAHA.109.562157
Wunderlich, M. T., Wallesch, C. W., and Goertler, M. (2006). Release of glial fibrillary acidic protein is related to the neurovascular status in acute ischemic stroke. Eur. J. Neurol. 13, 1118–1123. doi: 10.1111/j.1468-1331.2006.01435.x
Xu, H., Cao, Y., Yang, X., Cai, P., Kang, L., Zhu, X., et al. (2017). ADAMTS13 controls vascular remodeling by modifying VWF reactivity during stroke recovery. Blood 130, 11–22. doi: 10.1182/blood-2016-10-747089
Yang, D. B., Dong, X. Q., du, Q., Yu, W. H., Zheng, Y. K., Hu, W., et al. (2018). Clinical relevance of cleaved RAGE plasma levels as a biomarker of disease severity and functional outcome in aneurysmal subarachnoid hemorrhage. Clin. Chim. Acta 486, 335–340. doi: 10.1016/j.cca.2018.08.036
Zeng, L., Wang, Y., Liu, J., Wang, L., Weng, S., Chen, K., et al. (2013). Pro-inflammatory cytokine network in peripheral inflammation response to cerebral ischemia. Neurosci. Lett. 548, 4–9. doi: 10.1016/j.neulet.2013.04.037
Zhang, C. (2008). The role of inflammatory cytokines in endothelial dysfunction. Basic Res. Cardiol. 103, 398–406.
Zhang, Y., Li, F., Chen, C., Li, Y., Xie, W., Huang, D., et al. (2022). RAGE-mediated T cell metabolic reprogramming shapes T cell inflammatory response after stroke. J. Cereb. Blood Flow Metab. 42, 952–965. doi: 10.1177/0271678X211067133
Zhao, X. Y., Wu, C. F., Yang, J., Gao, Y., Sun, F. J., Wang, D. X., et al. (2015). Effect of arginine vasopressin on the cortex edema in the ischemic stroke of Mongolian gerbils. Neuropeptides 51, 55–62. doi: 10.1016/j.npep.2015.01.003
Zhivotovsky, B., and Orrenius, S. (2011). Calcium and cell death mechanisms: a perspective from the cell death community. Cell Calcium 50, 211–221. doi: 10.1016/j.ceca.2011.03.003
Keywords: biomarker, ischemic stroke, prediction, stroke, prognosis
Citation: Huang Y, Wang Z, Huang Z-X and Liu Z (2023) Biomarkers and the outcomes of ischemic stroke. Front. Mol. Neurosci. 16:1171101. doi: 10.3389/fnmol.2023.1171101
Edited by:
Miguel Diaz-Hernandez, Complutense University of Madrid, SpainReviewed by:
Hyung Jin Ahn, Rutgers, The State University of New Jersey, United StatesShereen Nizari, University College London, United Kingdom
Frederik Denorme, The University of Utah, United States
Copyright © 2023 Huang, Wang, Huang and Liu. This is an open-access article distributed under the terms of the Creative Commons Attribution License (CC BY). The use, distribution or reproduction in other forums is permitted, provided the original author(s) and the copyright owner(s) are credited and that the original publication in this journal is cited, in accordance with accepted academic practice. No use, distribution or reproduction is permitted which does not comply with these terms.
*Correspondence: Zhi-Xin Huang, hzxd6@163.com
†These authors have contributed equally to this work and share first authorship