- Departamento de Psicobiologia, Escola Paulista de Medicina, Universidade Federal de São Paulo, São Paulo, Brazil
Parental care is essential for proper development of stress response and emotion-related behaviours. Epidemiological studies show that parental loss in childhood represents a major risk factor for the development of mental disorders throughout the lifespan, including schizophrenia, depression, and anxiety. In most mammalian species, the mother is the main source of care and maternal behaviours regulate several physiological systems. Maternal deprivation (DEP) for 24 h is a paradigm widely used to disinhibit the hypothalamic–pituitary–adrenal axis response to stress during the stress hyporesponsive period. In this mini-review we will highlight the main DEP-induced neurobiological and behavioural outcomes, including alterations on stress-related hormones, neurogenesis, neurotransmitter/neuromodulatory systems and neuroinflammation. These neurobiological changes may be reflected by aberrant behaviours, which are relevant to the study of mental disorders. The evidence indicates that DEP consequences depend on the sex, the age when the DEP takes place and the age when the animals are evaluated, reflecting dynamic plasticity and individual variability. Individual variability and sex differences have a great relevance for the study of biological factors of stress resilience and vulnerability and the DEP paradigm is a suitable model for evaluation of phenotypes of stress- and emotion-related psychopathologies.
1. Introduction
Disruption of parent-infant relationship, including parental loss, abandonment or incarceration, represent risk factors for the development of depression (Berg et al., 2016), schizophrenia (Agid et al., 1999), and posttraumatic stress disorder (PTSD) in adulthood (Koenen et al., 2007; Lis-Turlejska et al., 2008). These events lead to dysregulation of the hypothalamic–pituitary–adrenal (HPA) axis stress response (Luecken, 1998; Tyrka et al., 2008a,b; Hagan et al., 2011; Kaplow et al., 2013), which may impact the trajectory of neurodevelopment and functioning of several brain systems. Maternal deprivation for 24 h (DEP) was developed in rats and mice to understand the effects of mother-infant disruptions on neurodevelopment, physiology and behaviour (Levine et al., 1991; Suchecki, 2018). It is a model of extreme neglect (Oomen et al., 2010) and transient loss of parental care (Suchecki, 2018). Maternal separation and DEP have been used as synonyms, but there are important differences between these protocols as well as in the outcomes and physiological mechanisms involved (for a comprehensive review on the subject, see Faturi et al. (2010)).
2. DEP effects on the HPA axis
In rats and mice, the importance of maternal presence goes beyond nourishment, induction of excretion and thermoregulation, as specific behaviours can stimulate physiological processes in the pups, such as heart rate, motor activity, sleep–wake cycle (Hofer, 1994; Hofer, 2006) and release of growth hormone (Evoniuk et al., 1979) or inhibit others, like the adrenocortical stress response (Levine et al., 1991). The stress hyporesponsive period (SHRP) is a hallmark of the ontogenesis of the stress response in rats and mice. It is characterised by adrenal insensitivity to its trophic hormone, adrenocorticotropin (ACTH) (Witek-Janusek, 1988) and lower responsiveness to stressful stimuli (Witek-Janusek, 1988; Walker et al., 1991), resulting in low and stable corticosterone (CORT) levels. In mice this period spans from days 1 to 12, and in rats, from days 4 to 14 (reviewed in van Bodegom et al. (2017)). Specific maternal behaviours regulate the stress response, such that nursing inhibits CORT and anogenital licking suppresses the ACTH stress response (Stanton et al., 1987; Rosenfeld et al., 1993; Suchecki et al., 1993; van Oers et al., 1998). Therefore, in both rats and mice, DEP during the SHRP augments the stress response (Levine et al., 1991; Schmidt et al., 2004; Enthoven et al., 2010; Faturi et al., 2010). The elevation of CORT basal and stress-induced levels is observed from 8 h of separation, reaching the highest levels by 24 h in both species (Levine et al., 1991; Schmidt et al., 2004). The effects of three 8 h separations on CORT response do not accumulate, since reunion with the mother resets the system to control (CTL) levels (Rosenfeld et al., 1992). After DEP, the CORT and ACTH responses to a saline injection remain elevated for at least 2 h (Suchecki et al., 1995; Girardi et al., 2014).
In rats, corticotropin releasing hormone (CRH) mRNA expression in the paraventricular nucleus of the hypothalamus (PVN) reaches adult levels by PND 4 (Baram and Lerner, 1991) and DEP on PND 9 (DEP9) reduces CRH levels in the median eminence (Pihoker et al., 1993), suggesting that DEP increases the release of this neuropeptide. In mice, CRH mRNA expression is high between PNDs 1 and 9, decreasing between PNDs 12 and 16 (Schmidt et al., 2003). In rats, DEP on day 9 (Schmidt et al., 2002) or on day 11 (DEP11) reduces CRH mRNA expression (Smith et al., 1997), whilst it enhances ACTH and CORT levels, suggesting that maternal inhibition of the HPA axis is confined to the peripheral components, e.g., pituitary and adrenals. Moreover, in 15 day-old mice, gene expression for CRH, vasopressin, glucocorticoid and mineralocorticoid receptors was similar between DEP9 and CTL animals (Reshetnikov et al., 2020).
Besides its immediate effects, DEP increases stress-induced CORT levels and adrenal relative weight in DEP3 male and female adolescent rats (Miragaia et al., 2018; Ceschim et al., 2021). CORT stress response is also higher in adult, but not aged, DEP4, DEP9 and DEP18 rats (Lehmann et al., 2002). DEP11 blunts the ACTH (van Oers et al., 1998) and CORT responses to a saline injection (Suchecki and Tufik, 1997) in juvenile rats (20–30 day olds), as well as the ACTH response to a hypertonic saline injection in adult DEP11 male and female rats (Faturi et al., 2010). However, in response to a session in the elevated plus maze, which represents a psychological stress, DEP11 adults of both sexes display a CORT response similar to their CTL counterparts (Cabbia et al., 2018). These results indicate that DEP induces plastic changes in the peripheral parts of the HPA axis that are not permanent and may depend on the nature of stressful stimulus (physical vs. psychological).
The immediate disinhibition of the HPA axis by DEP is a consistent finding, but very little is known about how other systems are altered right after this adversity. The long-term outcomes on brain systems and behaviour are far more complex and seem to be dependent on the sex, age of DEP and age at evaluation. In this mini review we will briefly describe the main outcomes of DEP on PND 3 (DEP3), 9 (DEP9) or 11 (DEP11), on several neurobiological systems and, when possible, we will highlight the relationship between changes in neurobiology and behaviours relevant to psychiatric disorders. These ages were chosen because they result in behavioural changes relevant for the study of co-morbid anxiety and depression (DEP3), depression (DEP9 and DEP11) and schizophrenia, and depression (DEP9).
3. DEP consequences on neurobiology and affective behaviour
Table 1 presents the main results of the studies included in this mini review. Figure 1 shows a graphical abstract of the neurobiological and behavioural parameters assessed in the studies that employed DEP on postnatal days 3, 9, and 11.
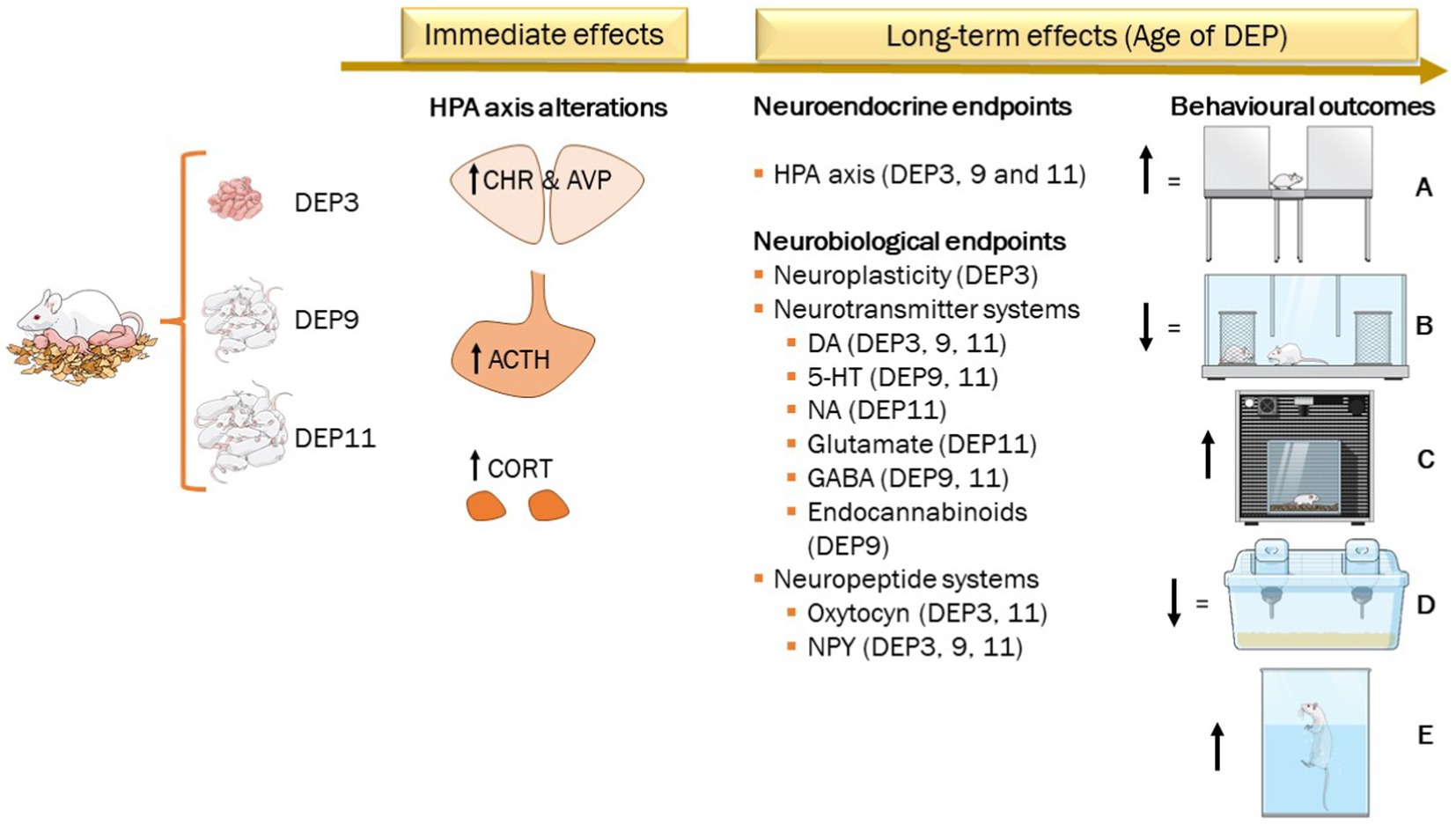
Figure 1. Immediate effects of maternal deprivation (DEP) on postnatal days (PND) 3 (DEP3), 9 (DEP9) or 11 (DEP11) on the hypothalamic–pituitary–adrenal (HPA) axis and the long-term effects neurobiological and behavioural endpoints. Although DEP increases corticosterone (CORT) levels, the magnitude of this effect depends on the age. (A) Anxiety-like behaviour measured in the elevated plus maze, (B) Social investigation test, (C) Prepulse inhibition, (D) Sucrose preference tests, and (E) Immobility in the forced swim test. Images were obtained from Mind the Graph (www.mindthegraph.com).
3.1. Neurogenesis and neuroplasticity
Neurogenesis depends on several factors, including brain derived neurotrophic factor (BDNF). Neurogenesis rate peaks between the last prenatal week and the first two weeks after birth (Morgane et al., 2002). Most granule hippocampal neurons are produced during the SHRP (Schlessinger et al., 1975). CORT, on the other hand, impairs BDNF production and neurogenesis (Tanapat et al., 1998; Schoenfeld and Gould, 2012). Therefore, DEP-induced persistent elevations in CORT levels during this period could be harmful for adequate hippocampal development. One study confirmed this prediction as 12 day old rats submitted to DEP11 exhibited more cell death, not only in the dentate gyrus (DG), but also in the parietal and cerebellar cortices. Moreover, after DEP11 there was more cell death in white matter tracts and this effect was proportional to the increase in nerve growth factor mRNA (Zhang et al., 2002). The short-term effects on hippocampal neurogenesis were investigated in 15 day old DEP9 male mice and the results show greater cell proliferation in the DG compared to CTL mice (Reshetnikov et al., 2020). These apparently contradictory findings may result from different ages of DEP and/or from a recovery process that might have taken place in Reshetnikov and colleague’s study (Reshetnikov et al., 2020).
Studies using DEP3 show a sex-dependent outcome, with increased cell proliferation and DCX-immunoreactivity (IR) in 21-day old male rats and reduced neurogenesis in female rats (Oomen et al., 2009, 2010, 2011). In line with these results, DEP3 females display more freezing in a cued fear conditioning task, indicating greater efficiency in amygdala-dependent fear memory (Oomen et al., 2011). In males, the neurogenesis inducing effect of DEP was transient: at 10 weeks of age, DEP3 impaired proliferation, survival and differentiation in the caudal part of the hippocampus (HPC), along with impaired spatial learning and improved emotional learning (Oomen et al., 2010). In adult male mice, DEP9 only produces a small decrease in the number of DG neurons (about 20%) (Fabricius et al., 2008). These findings reinforce the need for longitudinal evaluations to better understand the plastic changes induced by DEP.
In adolescent rats, DEP9 decreases the production of BDNF and PSD95, a marker of postsynaptic density, in the prefrontal cortex (PFC) and HPC of male and female rats (Marco et al., 2013). In addition, DEP9 reduces the levels of synaptophysin in PFC and neural cell adhesion molecule in HPC of males and females. Despite the similar neurobiological effects on both sexes, only DEP9 females display impairment in the novel object test, a hippocampal-dependent task (Marco et al., 2013).
3.2. Neurotransmitter systems
Several studies explored the effects of DEP on the activity of neurotransmitter systems. DEP3 induces hyperactivity of the dopaminergic system, with higher tyrosine hydroxylase mRNA levels in the substantia nigra of adolescent rats and greater sensitivity of DA-type 2 (D2) receptor to apomorphine than CTL rats (Rots et al., 1996). DEP9 increases the volume and number of dopaminergic neurons in the substantia nigra, pars compacta and reticulata, and in the ventral tegmental area of adolescent and adult rats (Kapor et al., 2020). Despite that, another study (Rentesi et al., 2013) showed that DA levels and turnover in the striatum were lower in DEP9 and that D2 receptor protein levels were higher in the striatum and lower in the PFC (Rentesi et al., 2013). DEP11 rats also exhibit high DA levels in the frontal cortex of adult males and in the amygdala of females (Cabbia et al., 2018).
DEP9 is a translational model of schizophrenia in adult male and female rats, who show impaired prepulse inhibition (PPI), a behaviour related to sensory-motor gating, an outcome that was not observed in DEP3 male rats (Ellenbroek et al., 1998). Moreover, treatment with haloperidol or quetiapine reversed the effects of DEP9 on PPI, fulfilling predictive validation of animal models (Ellenbroek et al., 1998). Collectively, these data indicate that DEP at different ages results in hyperactivity of the dopaminergic pathways and, depending on the age, the behavioural outcomes are relevant to the study of schizophrenia.
Serotonin (5-HT) and noradrenaline (NA) systems are altered by DEP in an anatomical-and sex-dependent manner. DEP9 adolescent male rats show higher 5-HT levels in the HPC and striatum than CTL counterparts, whilst DEP9 females exhibit higher 5-HT hippocampal levels than CTL ones. Moreover, 5-HT turnover in the hypothalamus (HPT) of DEP9 adolescent males is higher than that of CTL rats (Llorente et al., 2010). In line with the increased 5-HT hippocampal levels, lower 5-HT1A receptor protein levels were found in DEP9 adolescent male rats (Henn et al., 2021). In adult DEP9 male rats, 5-HT2A receptors are reduced in the striatum and PFC and increased in the amygdala (AMY) (Rentesi et al., 2013). Compared to CTL rats, DEP11 adult males have lower 5-HT levels in the FC and higher in the hypothalamus (HPT) (Cabbia et al., 2018). Furthermore, DEP11 males display more hypoxia-induced panic-like behaviour than CTL rats and depletion of 5-HT levels by parachlorophenylalanine treatment increases panic-like behaviour in CTL, but has no effect in DEP11 rats, suggesting that 5-HT activity is already impaired in these animals (Rosa et al., 2022). DEP11 female rats display higher NA levels in the FC (Cabbia et al., 2018) and lower levels in the HPC (Barbosa Neto et al., 2012) than their CTL counterparts. Thus, DEP alters the activity of 5-HT system in an age-dependent manner: DEP9 increases and DEP11 decreases this neurotransmitter levels. DEP11 (the only age evaluated) causes small and sex-specific changes in the NA system, with no impact on emotional behaviour.
Few studies explored the effects of DEP on amino acid neurotransmitter systems. DEP9 reduces the expression of NR-2A and NR-2B subunits of the NMDA glutamatergic receptor in the HPC and PFC of adult male rats (Roceri et al., 2002) and increases binding of this receptor in the caudate-putamen of adult female rats (Zamberletti et al., 2012). Protein levels or glutamic acid decarboxylase (GAD 67)-IR, one of the GABA synthesizing enzymes, are lower in many cortical areas of DEP9 compared to CTL male rats (Janetsian-Fritz et al., 2018; Poleksic et al., 2021). DEP11 alters hippocampal levels of amino acid neurotransmitters in a sex-dependent manner. Adult male rats exhibit higher levels of the excitatory amino acids glutamate and aspartate and lower levels of the inhibitory amino acid taurine, whilst females show a slight reduction of taurine and a major reduction in GABA levels. These alterations lead to an imbalance of excitatory/inhibitory activity in the HPC of DEP11 compared to CTL rats (Barbosa Neto et al., 2012), which may compromise the adequate functioning of this brain structure.
The endocannabinoid (EC) system is a buffering regulator of the stress response (Viveros et al., 2007) and only a few studies explored the effects of DEP9 on this system. The findings show that DEP9 reduces cannabinoid receptor type 1 IR (CB1-IR) in male pups and increases CB2 receptor-IR in both sexes in several hippocampal fields of 13-day old pups (Suarez et al., 2009). In a subsequent study, the same group performed a thorough investigation of DEP9 effects on gene expression of CB1, CB2, transient receptor potential vaniloid-and G-coupled receptors and all enzymes involved in the biosynthesis and degradation of EC in several brain regions. All parameters were increased in the PFC of DEP9 males and HPC of DEP9 females (Suarez et al., 2010; Marco et al., 2014). Acute treatment of CTL males with WIN55,212-2 – a CB1 receptor agonist—reduced exploration of the open arms in the elevated plus maze. However, this effect was absent in DEP9 males. This drug treatment also increased CORT levels irrespective of sex and group, but this effect was more intense in DEP9 adolescent males (Llorente et al., 2007). In the forced swim test (FST) DEP9 adolescent males initiated floating behaviour faster and both males and females floated longer than CTLs (Llorente et al., 2007). These findings indicate that DEP9 leads to long-term region-specific changes in the EC system; however, the direction of the effect seems to depend on the technique used.
3.3. Neuropeptides
In addition to classical neurotransmitters, neuromodulatory systems have also been investigated in the context of DEP. Oxytocin (OT) is mostly synthesized in the supraoptic (SON) and paraventricular PVN nuclei in the HPT and has a role in reproduction (e.g., birth, lactation), regulates social and emotional behaviour and stress-related responses (for review, see Onaka and Takayanagi (2021)). The only study that addressed the influence of DEP on the OT system and social behaviour showed a slight increase of OT-IR in the magnocellular part of the SON and PVN of DEP3 adolescent females in comparison with DEP11 counterparts and an increased investigation of the empty container in the social investigation test in DEP3 adolescent males (Ceschim et al., 2021).
The best studied neuropeptide in the context of DEP is Neuropeptide Y (NPY), the most abundant in the brain which is mainly produced in the arcuate nucleus of the HPT (ARC) (Heilig, 2004), but also in the lateral septum, amygdala, HPC and locus coeruleus. Anatomical, pharmacological, and behavioural evidence indicates that NPY is associated with stress resilience and anxiolysis (Heilig et al., 1994; Thorsell et al., 2000; Serova et al., 2014; Thorsell and Mathé, 2017).
Adult DEP9 males display lower NPY levels in the HPC than CTL rats, accompanied by impairment of PPI (Husum et al., 2002), suggesting a possible involvement of low NPY levels with schizophrenia-like behaviour. DEP3 and DEP11 male and female adolescent rats have lower NPY-IR in the ARC, which explains their reduced food intake and lower body weight than CTL rats (Wertheimer et al., 2016). They also have less NPY-IR in the basolateral amygdala and dorsal HPC, although in males, the effect on DEP3 males was more intense than in DEP11 (Miragaia et al., 2018). In both studies, NPY-IR was smaller in females than in males, regardless of the group (Wertheimer et al., 2016; Miragaia et al., 2018). In the latter study, emotional behaviours were assessed and DEP3 male and female young adult rats displayed more anxiety-like behaviour in two tests and more immobility in the FST than DEP11 and CTL counterparts (Miragaia et al., 2018), suggesting that DEP3 results in co-occurrent anxiety and depressive-like behaviours. DEP11 male and female rats did not show changes in anxiety-like behaviour, although they also spent more time floating in the FST, but this effect was subtler than that seen in DEP3 adolescents. Interestingly, in the negative sucrose contrast test, a test that measures the loss of rewarding salience of from a high to low palatable sucrose solution, only DEP3 and DEP11 males were affected, still preferring the low sucrose solution to water. This result can be interpreted as a blunted hedonic response, characteristic of depressive patients. These findings indicate that DEP-induced impairment of NPY function may underlie the expression of behaviours relevant to stress-related psychiatric disorders. Indeed, low NPY levels in the cerebrospinal fluid were observed in depressive (Heilig et al., 2004) and PTSD patients (Yehuda et al., 2006; Sah et al., 2009).
3.4. Glial cells and neuroinflammation
Neuroinflammation plays an important role in the pathophysiology of stress-related psychiatric disorders (Dantzer et al., 2008) and many studies show that early life stress can alter microglial development and function (for a recent review, see Catale et al. (2020)), but data on the effects of DEP are scarce.
In 10 -or 60-day old rats, levels of Iba-1, a marker of microglia, in the PFC were unchanged by DEP9. However, immunohistochemistry revealed that DEP9 male pups had more Iba-1 labelling than controls in the medial orbital and infralimbic cortices and this effect vanished at day 60 (Poleksic et al., 2021). DEP9 increased the number of glial fibrillar acidic protein positive cells (GFAP+), a marker of astrocytes, in the CA1, CA3 of 13 day-old male pups, and in the molecular layer of the DG in both sexes (Llorente et al., 2009); GFAP levels were still higher in the HPC of DEP9 than in CTL male rats at 40 days of age (Marco et al., 2013). In mice, the effects of DEP9 were compared with those of maternal separation (MS) from days 2 to 14 (3 h/day) on the morphology of glial cells in the PFC and HPC at day 15 of life. DEP9 increased the number of intermediate glia, whilst MS increased ameboid glia (Reshetnikov et al., 2020), suggesting that these early life adversities retard the maturation of glial cells from ameboid to ramified morphology (Kaur et al., 2017).
One study examined the effects of DEP9 on nociceptive response of rats submitted to peripheral nerve injury and on mRNA expression of interleukin 1β (IL-1β), IL-6, and tumour necrosis factor alpha (TNF-α). DEP9 females displayed greater pain response to a non-painful stimulus, and higher TNF-α expression in the HPC than CTL ones. DEP9 reduced IL-6 expression in the PFC of males. These data show sexually dimorphic effects of DEP9 on nociception and suggest that neuroinflammatory alterations underlie these changes (Burke et al., 2013).
4. Final considerations
In rats and mice, DEP leads to immediate changes in the physiological response to stress and long-term consequences on stress response and behaviours relevant to psychiatric disorders. DEP is a useful tool to assess the effects of elevated CORT levels in specific hallmarks of neurodevelopment and how this phenomenon can alter the phenotypic trajectory, leading to vulnerability or resilience. DEP at all ages during the first two weeks of life disinhibits the stress response, but investigation of the immediate effects is restricted to DEP9 and the EC system and neuroinflammation. Despite the immediate effects of DEP on CORT levels, DEP3 and DEP9 lead to greater vulnerability to stress-related psychiatric disorders, whilst the behavioural changes of DEP11 seem to be less intense than the earlier ages. Therefore, longitudinal studies on the impact of DEP3 and DEP11 on neurobiology and behaviour in a sex-dimorphic context could help to understand how this adversity influences molecular and cellular mechanisms involved in resilience and vulnerability throughout the individual’s developmental trajectory. Disclosure of these mechanisms can provide the seed to develop new and more effective treatments and even preventive interventions for maternally/paternally deprived children and improve their mental health.
Author contributions
NZ and NA wrote the first draft of the manuscript and prepared the table. DS edited the manuscript, table, and prepared the figure. All authors contributed to the article and approved the submitted version.
Funding
The original studies cited in this review were supported by grants from AFIP, CAPES (Financial code 001), FAPESP (grants # 94/0262-2; 2006/06415-4; 2015/26364-4; 2019/21980-0) and CNPq (grants # 400039/1994-0; 470449/2008-0; 302294/2012). NZ is the recipient of a Ph.D. fellowship from CAPES and NA is the recipient of a Ph.D. fellowship from CNPq. DS is the recipient of a Research fellowship from CNPq (grant # 302608/2019-2). Payment for this publication was made possible by CAPES (Financial code 001).
Conflict of interest
The authors declare that the research was conducted in the absence of any commercial or financial relationships that could be construed as a potential conflict of interest.
Publisher’s note
All claims expressed in this article are solely those of the authors and do not necessarily represent those of their affiliated organizations, or those of the publisher, the editors and the reviewers. Any product that may be evaluated in this article, or claim that may be made by its manufacturer, is not guaranteed or endorsed by the publisher.
References
Agid, O., Shapira, B., Zislin, J., Ritsner, M., Hanin, B., Murad, H., et al. (1999). Environment and vulnerability to major psychiatric illness: a case control study of early parental loss in major depression, bipolar disorder and schizophrenia. Mol. Psychiatry 4, 163–172. doi: 10.1038/sj.mp.4000473
Baram, T. Z., and Lerner, S. P. (1991). Ontogeny of corticotropin releasing hormone gene expression in rat hypothalamus--comparison with somatostatin. Int. J. Dev. Neurosci. 9, 473–478. doi: 10.1016/0736-5748(91)90033-i
Barbosa Neto, J. B., Tiba, P. A., Faturi, C. B., de Castro-Neto, E. F., da Graça, N.-M. M., de Jesus, M. J., et al. (2012). Stress during development alters anxiety-like behavior and hippocampal neurotransmission in male and female rats. Neuropharmacology 62, 518–526. doi: 10.1016/j.neuropharm.2011.09.011
Berg, L., Rostila, M., and Hjern, A. (2016). Parental death during childhood and depression in young adults – a national cohort study. J. Child Psychol. Psychiatry 57, 1092–1098. doi: 10.1111/jcpp.12560
Burke, N. N., Llorente, R., Marco, E. M., Tong, K., Finn, D. P., Viveros, M. P., et al. (2013). Maternal deprivation is associated with sex-dependent alterations in nociceptive behavior and neuroinflammatory mediators in the rat following peripheral nerve injury. J. Pain 14, 1173–1184. doi: 10.1016/j.jpain.2013.05.003
Cabbia, R., Consoli, A., and Suchecki, D. (2018). Association of 24 h maternal deprivation with a saline injection in the neonatal period alters adult stress response and brain monoamines in a sex-dependent fashion. Stress 21, 333–346. doi: 10.1080/10253890.2018.1456525
Catale, C., Gironda, S., Lo Iacono, L., and Carola, V. (2020). Microglial function in the effects of early-life stress on brain and behavioral development. J. Clin. Med. 9:468. doi: 10.3390/jcm9020468
Ceschim, V. C., Sumarán, P., Borges, A. A., Girardi, C. E. N., and Suchecki, D. (2021). Maternal deprivation during early infancy in rats increases oxytocin immunoreactivity in females and corticosterone reactivity to a social test in both sexes without changing emotional behaviour. Horm. Behav. 129:104928. doi: 10.1016/j.yhbeh.2021.104928
Dantzer, R., O’Connor, J. C., Freund, G. G., Johnson, R. W., and Kelley, K. W. (2008). From inflammation to sickness and depression: when the immune system subjugates the brain. Nat. Rev. Neurosci. 9, 46–56. doi: 10.1038/nrn2297
Ellenbroek, B. A., van den Kroonenberg, P. T., and Cools, A. R. (1998). The effects of an early stressful life event on sensorimotor gating in adult rats. Schizophr. Res. 30, 251–260. doi: 10.1016/S0920-9964(97)00149-7
Enthoven, L., Schmidt, M. V., Cheung, Y. H., van der Mark, M. H., de Kloet, E. R., and Oitzl, M. S. (2010). Ontogeny of the HPA axis of the CD1 mouse following 24 h maternal deprivation at PND 3. Int. J. Dev. Neurosci. 28, 217–224. doi: 10.1016/j.ijdevneu.2009.10.006
Evoniuk, G. E., Kuhn, C. M., and Schanberg, S. M. (1979). The effect of tactile stimulation on serum growth hormone and tissue ornithine decarboxylase activity during maternal deprivation in rat pups. Commun. Psychopharmacol. 3, 363–370.
Fabricius, K., Wortwein, G., and Pakkenberg, B. (2008). The impact of maternal separation on adult mouse behaviour and on the total neuron number in the mouse hippocampus. Brain Struct. Funct. 212, 403–416. doi: 10.1007/s00429-007-0169-6
Faturi, C. B., Tiba, P. A., Kawakami, S. E., Catallani, B., Kerstens, M., and Suchecki, D. (2010). Disruptions of the mother-infant relationship and stress-related behaviours: altered corticosterone secretion does not explain everything. Neurosci. Biobehav. Rev. 34, 821–834. doi: 10.1016/j.neubiorev.2009.09.002
Girardi, C. E., Zanta, N. C., and Suchecki, D. (2014). Neonatal stress-induced affective changes in adolescent Wistar rats: early signs of schizophrenia-like behavior. Front. Behav. Neurosci. 8:319. doi: 10.3389/fnbeh.2014.00319
Hagan, M. J., Roubinov, D. S., Gress-Smith, J., Luecken, L. J., Sandler, I. N., and Wolchik, S. (2011). Positive parenting during childhood moderates the impact of recent negative events on cortisol activity in parentally bereaved youth. Psychopharmacology 214, 231–238. doi: 10.1007/s00213-010-1889-5
Heilig, M. (2004). The NPY system in stress, anxiety and depression. Neuropeptides 38, 213–224. doi: 10.1016/j.npep.2004.05.002
Heilig, M., Koob, G. F., Ekman, R., and Britton, K. T. (1994). Corticotropin-releasing factor and neuropeptide Y: role in emotional integration. Trends Neurosci. 17, 80–85. doi: 10.1016/0166-2236(94)90079-5
Heilig, M., Zachrisson, O., Thorsell, A., Ehnvall, A., Mottagui-Tabar, S., Sjögren, M., et al. (2004). Decreased cerebrospinal fluid neuropeptide Y (NPY) in patients with treatment refractory unipolar major depression: preliminary evidence for association with preproNPY gene polymorphism. J. Psychiatr. Res. 38, 113–121. doi: 10.1016/S0022-3956(03)00101-8
Henn, L., Zanta, N. C., Girardi, C. E. N., and Suchecki, D. (2021). Chronic Escitalopram treatment does not alter the effects of neonatal stress on hippocampal BDNF levels, 5-HT1A expression and emotional behaviour of male and female adolescent rats. Mol. Neurobiol. 58, 926–943. doi: 10.1007/s12035-020-02164-1
Hofer, M. A. (1994). Hidden regulators in attachment, separation, and loss. Monogr. Soc. Res. Child Dev. 59, 192–207. doi: 10.1111/j.1540-5834.1994.tb01285.x
Hofer, M. A. (2006). Psychobiological roots of early attachment. Curr. Dir. Psychol. Sci. 15, 84–88. doi: 10.1111/j.0963-7214.2006.00412.x
Husum, H. E. T., Mathé, A. A., Bolwig, T. G., and Ellenbroek, B. A. (2002). Early maternal deprivation alters hippocampal levels of neuropeptide Y and calcitonin-gene related peptide in adult rats. Neuropharmacology 42, 798–806. doi: 10.1016/S0028-3908(02)00038-2
Janetsian-Fritz, S. S., Timme, N. M., Timm, M. M., McCane, A. M., Baucum, A. J., O’Donnell, B. F., et al. (2018). Correction: maternal deprivation induces alterations in cognitive and cortical function in adulthood. Transl. Psychiatry 8:137. doi: 10.1038/s41398-018-0207-6
Kaplow, J. B., Shapiro, D. N., Wardecker, B. M., Howell, K. H., Abelson, J. L., Worthman, C. M., et al. (2013). Psychological and environmental correlates of HPA axis functioning in parentally bereaved children: preliminary findings. J. Trauma. Stress. 26, 233–240. doi: 10.1002/jts.21788
Kapor, S., Aksic, M., Puskas, L., Jukic, M., Poleksic, J., Milosavljevic, F., et al. (2020). Long-term effects of maternal deprivation on the volume of dopaminergic nuclei and number of dopaminergic neurons in Substantia Nigra and ventral tegmental area in rats. Front. Neuroanat. 14:578900. doi: 10.3389/fnana.2020.578900
Kaur, C., Rathnasamy, G., and Ling, E. A. (2017). Biology of microglia in the developing brain. J. Neuropathol. Exp. Neurol. 76, 736–753. doi: 10.1093/jnen/nlx056
Koenen, K. C., Moffitt, T. E., Poulton, R., Martin, J., and Caspi, A. (2007). Early childhood factors associated with the development of post-traumatic stress disorder: results from a longitudinal birth cohort. Psychol. Med. 37, 181–192. doi: 10.1017/S0033291706009019
Lehmann, J., Russig, H., Feldon, J., and Pryce, C. R. (2002). Effect of a single maternal separation at different pup ages on the corticosterone stress response in adult and aged rats. Pharmacol. Biochem. Behav. 73, 141–145. doi: 10.1016/S0091-3057(02)00788-8
Levine, S., Huchton, D. M., Wiener, S. G., and Rosenfeld, P. (1991). Time course of the effect of maternal deprivation on the hypothalamic-pituitary-adrenal axis in the infant rat. Dev. Psychobiol. 24, 547–558. doi: 10.1002/dev.420240803
Lis-Turlejska, M., Luszczynska, A., Plichta, A., and Benight, C. C. (2008). Jewish and non-Jewish world war II child and adolescent survivors at 60 years after war: effects of parental loss and age at exposure on well-being. Am. J. Orthopsychiatry 78, 369–377. doi: 10.1037/a0014166
Llorente, R., Arranz, L., Marco, E. M., Moreno, E., Puerto, M., Guaza, C., et al. (2007). Early maternal deprivation and neonatal single administration with a cannabinoid agonist induce long-term sex-dependent psychoimmunoendocrine effects in adolescent rats. Psychoneuroendocrinology 32, 636–650. doi: 10.1016/j.psyneuen.2007.04.002
Llorente, R., Gallardo, M. L., Berzal, A. L., Prada, C., Garcia-Segura, L. M., and Viveros, M. P. (2009). Early maternal deprivation in rats induces gender-dependent effects on developing hippocampal and cerebellar cells. Int. J. Dev. Neurosci. 27, 233–241. doi: 10.1016/j.ijdevneu.2009.01.002
Llorente, R., O’Shea, E., Gutierrez-Lopez, M. D., Llorente-Berzal, A., Colado, M. I., and Viveros, M. P. (2010). Sex-dependent maternal deprivation effects on brain monoamine content in adolescent rats. Neurosci. Lett. 479, 112–117. doi: 10.1016/j.neulet.2010.05.039
Luecken, L. J. (1998). Childhood attachment and loss experiences affect adult cardiovascular and cortisol function. Psychosom. Med. 60, 765–772. doi: 10.1097/00006842-199811000-00021
Marco, E. M., Echeverry-Alzate, V., Lopez-Moreno, J. A., Gine, E., Penasco, S., and Viveros, M. P. (2014). Consequences of early life stress on the expression of endocannabinoid-related genes in the rat brain. Behav. Pharmacol. 25, 547–556. doi: 10.1097/FBP.0000000000000068
Marco, E. M., Valero, M., de la Serna, O., Aisa, B., Borcel, E., Ramirez, M. J., et al. (2013). Maternal deprivation effects on brain plasticity and recognition memory in adolescent male and female rats. Neuropharmacology 68, 223–231. doi: 10.1016/j.neuropharm.2012.08.014
Miragaia, A. S., de Oliveira Wertheimer, G. S., Consoli, A. C., Cabbia, R., Longo, B. M., Girardi, C. E. N., et al. (2018). Maternal deprivation increases anxiety-and depressive-like behaviors in an age-dependent fashion and reduces neuropeptide Y expression in the amygdala and hippocampus of male and female young adult rats. Front. Behav. Neurosci. 12:159. doi: 10.3389/fnbeh.2018.00159
Morgane, P. J., Mokler, D. J., and Galler, J. R. (2002). Effects of prenatal protein malnutrition on the hippocampal formation. Neurosci. Biobehav. Rev. 26, 471–483. doi: 10.1016/S0149-7634(02)00012-X
Onaka, T., and Takayanagi, Y. (2021). The oxytocin system and early-life experience-dependent plastic changes. J. Neuroendocrinol. 33:e13049. doi: 10.1111/jne.13049
Oomen, C. A., Girardi, C. E., Cahyadi, R., Verbeek, E. C., Krugers, H., Joëls, M., et al. (2009). Opposite effects of early maternal deprivation on neurogenesis in male versus female rats. PLoS One 4:e3675. doi: 10.1371/journal.pone.0003675
Oomen, C. A., Soeters, H., Audureau, N., Vermunt, L., van Hasselt, F. N., Manders, E. M., et al. (2010). Severe early life stress hampers spatial learning and neurogenesis, but improves hippocampal synaptic plasticity and emotional learning under high-stress conditions in adulthood. J. Neurosci. 30, 6635–6645. doi: 10.1523/JNEUROSCI.0247-10.2010
Oomen, C. A., Soeters, H., Audureau, N., Vermunt, L., van Hasselt, F. N., Manders, E. M., et al. (2011). Early maternal deprivation affects dentate gyrus structure and emotional learning in adult female rats. Psychopharmacology 214, 249–260. doi: 10.1007/s00213-010-1922-8
Pihoker, C., Owens, M. J., Kuhn, C. M., Schanberg, S. M., and Nemeroff, C. B. (1993). Maternal separation in neonatal rats elicits activation of the hypothalamic-pituitary-adrenocortical axis: a putative role for corticotropin-releasing factor. Psychoneuroendocrinology 18, 485–493. doi: 10.1016/0306-4530(93)90042-J
Poleksic, J., Aksic, M., Kapor, S., Aleksic, D., Stojkovic, T., Radovic, M., et al. (2021). Effects of maternal deprivation on the prefrontal cortex of male rats: cellular, neurochemical, and behavioral outcomes. Front. Behav. Neurosci. 15:666547. doi: 10.3389/fnbeh.2021.666547
Rentesi, G., Antoniou, K., Marselos, M., Syrrou, M., Papadopoulou-Daifoti, Z., and Konstandi, M. (2013). Early maternal deprivation-induced modifications in the neurobiological, neurochemical and behavioral profile of adult rats. Behav. Brain Res. 244, 29–37. doi: 10.1016/j.bbr.2013.01.040
Reshetnikov, V., Ryabushkina, Y., Kovner, A., Lepeshko, A., and Bondar, N. (2020). Repeated and single maternal separation specifically alter microglial morphology in the prefrontal cortex and neurogenesis in the hippocampus of 15-day-old male mice. Neuroreport 31, 1256–1264. doi: 10.1097/WNR.0000000000001544
Roceri, M., Hendriks, W., Racagni, G., Ellenbroek, B. A., and Riva, M. A. (2002). Early maternal deprivation reduces the expression of BDNF and NMDA receptor subunits in rat hippocampus. Mol. Psychiatry 7, 609–616. doi: 10.1038/sj.mp.4001036
Rosa, D. S., Frias, A. T., Vilela-Costa, H. H., Spiacci, A., Sant’Ana, A. B., Fusse, E. J., et al. (2022). Neonatal maternal deprivation facilitates the expression of a panic-like escape behavior in adult rats. Behav. Brain Res. 434:114031. doi: 10.1016/j.bbr.2022.114031
Rosenfeld, P., Ekstrand, J., Olson, E., Suchecki, D., and Levine, S. (1993). Maternal regulation of adrenocortical activity in the infant rat: effects of feeding. Dev. Psychobiol. 26, 261–277. doi: 10.1002/dev.420260504
Rosenfeld, P., Wetmore, J. B., and Levine, S. (1992). Effects of repeated maternal separations on the adrenocortical response to stress of preweanling rats. Physiol. Behav. 52, 787–791. doi: 10.1016/0031-9384(92)90415-X
Rots, N. Y., de Jong, J., Workel, J. O., Levine, S., Cools, A. R., and de Kloet, E. R. (1996). Neonatal maternally deprived rats have as adults elevated basal pituitary-adrenal activity and enhanced susceptibility to apomorphine. J. Neuroendocrinol. 8, 501–506. doi: 10.1046/j.1365-2826.1996.04843.x
Sah, R., Ekhator, N. N., Strawn, J. R., Sallee, F. R., Baker, D. G., Horn, P. S., et al. (2009). Low cerebrospinal fluid neuropeptide Y concentrations in posttraumatic stress disorder. Biol. Psychiatry 66, 705–707. doi: 10.1016/j.biopsych.2009.04.037
Schlessinger, A. R., Cowan, W. M., and Gottlieb, D. I. (1975). An autoradiographic study of the time of origin and the pattern of granule cell migration in the dentate gyrus of the rat. J. Comp. Neurol. 159, 149–175. doi: 10.1002/cne.901590202
Schmidt, M., Enthoven, L., van Woezik, J. H., Levine, S., de Kloet, E. R., and Oitzl, M. S. (2004). The dynamics of the hypothalamic-pituitary-adrenal axis during maternal deprivation. J. Neuroendocrinol. 16, 52–57. doi: 10.1111/j.1365-2826.2004.01123.x
Schmidt, M. V., Schmidt, M., Enthoven, L., van der Mark, M., Levine, S., de Kloet, E. R., et al. (2003). The postnatal development of the hypothalamic-pituitary-adrenal axis in the mouse. Int. J. Dev. Neurosci. 21, 125–132. doi: 10.1016/S0736-5748(03)00030-3
Schmidt, M. V., Schmidt, M., Oitzl, M. S., Levine, S., and de Kloet, E. R. (2002). The HPA system during the postnatal development of CD1 mice and the effects of maternal deprivation. Brain Res. Dev. Brain Res. 139, 39–49. doi: 10.1016/S0165-3806(02)00519-9
Schoenfeld, T. J., and Gould, E. (2012). Stress, stress hormones, and adult neurogenesis. Exp. Neurol. 233, 12–21. doi: 10.1016/j.expneurol.2011.01.008
Serova, L. I., Laukova, M., Alaluf, L. G., Pucillo, L., and Sabban, E. L. (2014). Intranasal neuropeptide Y reverses anxiety and depressive-like behavior impaired by single prolonged stress PTSD model. Eur. Neuropsychopharmacol. 24, 142–147. doi: 10.1016/j.euroneuro.2013.11.007
Smith, M. A., Kim, S. Y., van Oers, H. J., and Levine, S. (1997). Maternal deprivation and stress induce immediate early genes in the infant rat brain. Endocrinology 138, 4622–4628. doi: 10.1210/endo.138.11.5529
Stanton, M. E., Wallstrom, J., and Levine, S. (1987). Maternal contact inhibits pituitary-adrenal stress responses in preweanling rats. Dev. Psychobiol. 20, 131–145. doi: 10.1002/dev.420200204
Suarez, J., Llorente, R., Romero-Zerbo, S. Y., Mateos, B., Bermudez-Silva, F. J., de Fonseca, F. R., et al. (2009). Early maternal deprivation induces gender-dependent changes on the expression of hippocampal CB(1) and CB(2) cannabinoid receptors of neonatal rats. Hippocampus 19, 623–632. doi: 10.1002/hipo.20537
Suarez, J., Rivera, P., Llorente, R., Romero-Zerbo, S. Y., Bermudez-Silva, F. J., de Fonseca, F. R., et al. (2010). Early maternal deprivation induces changes on the expression of 2-AG biosynthesis and degradation enzymes in neonatal rat hippocampus. Brain Res. 1349, 162–173. doi: 10.1016/j.brainres.2010.06.042
Suchecki, D. (2018). Maternal regulation of the infant’s hypothalamic-pituitary-adrenal axis stress response: Seymour ‘Gig’ Levine’s legacy to neuroendocrinology. J. Neuroendocrinol. 30:e12610. doi: 10.1111/jne.12610
Suchecki, D., Nelson, D. Y., van Oers, H., and Levine, S. (1995). Activation and inhibition of the hypothalamic-pituitary-adrenal axis of the neonatal rat: effects of maternal deprivation. Psychoneuroendocrinology 20, 169–182. doi: 10.1016/0306-4530(94)00051-B
Suchecki, D., Rosenfeld, P., and Levine, S. (1993). Maternal regulation of the hypothalamic-pituitary-adrenal axis in the infant rat: the roles of feeding and stroking. Brain Res. Dev. Brain Res. 75, 185–192. doi: 10.1016/0165-3806(93)90022-3
Suchecki, D., and Tufik, S. (1997). Long-term effects of maternal deprivation on the corticosterone response to stress in rats. Am. J. Phys. Regul. Integr. Comp. Phys. 273, R1332–R1338. doi: 10.1152/ajpregu.1997.273.4.R1332
Tanapat, P., Galea, L. A., and Gould, E. (1998). Stress inhibits the proliferation of granule cell precursors in the developing dentate gyrus. Int. J. Dev. Neurosci. 16, 235–239. doi: 10.1016/S0736-5748(98)00029-X
Thorsell, A., and Mathé, A. A. (2017). Neuropeptide Y in alcohol addiction and affective disorders. Front. Endocrinol. (Lausanne) 8:178. doi: 10.3389/fendo.2017.00178
Thorsell, A., Michalkiewicz, M., Dumont, Y., Quirion, R., Caberlotto, L., Rimondini, R., et al. (2000). Behavioral insensitivity to restraint stress, absent fear suppression of behavior and impaired spatial learning in transgenic rats with hippocampal neuropeptide Y overexpression. Proc. Natl Acad. Sci. U. S. A. 97, 12852–12857. doi: 10.1073/pnas.220232997
Tyrka, A. R., Wier, L., Price, L. H., Ross, N., Anderson, G. M., Wilkinson, C. W., et al. (2008a). Childhood parental loss and adult hypothalamic-pituitary-adrenal function. Biol. Psychiatry 63, 1147–1154. doi: 10.1016/j.biopsych.2008.01.011
Tyrka, A. R., Wier, L., Price, L. H., Ross, N. S., and Carpenter, L. L. (2008b). Childhood parental loss and adult psychopathology: effects of loss characteristics and contextual factors. Int. J. Psychiatry Med. 38, 329–344. doi: 10.2190/PM.38.3.h
van Bodegom, M., Homberg, J. R., and Henckens, M. (2017). Modulation of the hypothalamic-pituitary-adrenal axis by early life stress exposure. Front. Cell. Neurosci. 11:87. doi: 10.3389/fncel.2017.00087
van Oers, H. J., de Kloet, E. R., and Levine, S. (1998). Early vs. late maternal deprivation differentially alters the endocrine and hypothalamic responses to stress. Brain Res. Dev. Brain Res. 111, 245–252. doi: 10.1016/S0165-3806(98)00143-6
van Oers, H. J., de Kloet, E. R., Whelan, T., and Levine, S. (1998). Maternal deprivation effect on the infant’s neural stress markers is reversed by tactile stimulation and feeding but not by suppressing corticosterone. J. Neurosci. 18, 10171–10179. doi: 10.1523/JNEUROSCI.18-23-10171.1998
Viveros, M. P., Marco, E. M., Llorente, R., and Lopez-Gallardo, M. (2007). Endocannabinoid system and synaptic plasticity: implications for emotional responses. Neural Plast. 2007:52908. doi: 10.1155/2007/52908
Walker, C. D., Scribner, K. A., Cascio, C. S., and Dallman, M. F. (1991). The pituitary-adrenocortical system of neonatal rats is responsive to stress throughout development in a time-dependent and stressor-specific fashion. Endocrinology 128, 1385–1395. doi: 10.1210/endo-128-3-1385
Wertheimer, G. S., Girardi, C. E., de Oliveira, A. S., Monteiro Longo, B., and Suchecki, D. (2016). Maternal deprivation alters growth, food intake, and neuropeptide Y in the hypothalamus of adolescent male and female rats. Dev. Psychobiol. 58, 1066–1075. doi: 10.1002/dev.21440
Witek-Janusek, L. (1988). Pituitary-adrenal response to bacterial endotoxin in developing rats. Am. J. Phys. 255, E525–E530. doi: 10.1152/ajpendo.1988.255.4.E525
Yehuda, R., Brand, S., and Yang, R. K. (2006). Plasma neuropeptide Y concentrations in combat exposed veterans: relationship to trauma exposure, recovery from PTSD, and coping. Biol. Psychiatry 59, 660–663. doi: 10.1016/j.biopsych.2005.08.027
Zamberletti, E., Prini, P., Speziali, S., Gabaglio, M., Solinas, M., Parolaro, D., et al. (2012). Gender-dependent behavioral and biochemical effects of adolescent delta-9-tetrahydrocannabinol in adult maternally deprived rats. Neuroscience 204, 245–257. doi: 10.1016/j.neuroscience.2011.11.038
Keywords: early life stress, maternal deprivation, HPA axis, neurotransmitters, neuropeptides, affective behaviour
Citation: Zanta NC, Assad N and Suchecki D and (2023) Neurobiological mechanisms involved in maternal deprivation-induced behaviours relevant to psychiatric disorders. Front. Mol. Neurosci. 16:1099284. doi: 10.3389/fnmol.2023.1099284
Edited by:
Natividade de Sá Couto-Pereira, Federal University of Rio Grande do Sul, BrazilReviewed by:
Therese A. Kosten, University of Houston, United StatesPamela Maras, University of Michigan, United States
Copyright © 2023 Zanta, Assad and Suchecki. This is an open-access article distributed under the terms of the Creative Commons Attribution License (CC BY). The use, distribution or reproduction in other forums is permitted, provided the original author(s) and the copyright owner(s) are credited and that the original publication in this journal is cited, in accordance with accepted academic practice. No use, distribution or reproduction is permitted which does not comply with these terms.
*Correspondence: Deborah Suchecki, deborah.suchecki21@unifesp.br
†These authors have contributed equally to this work