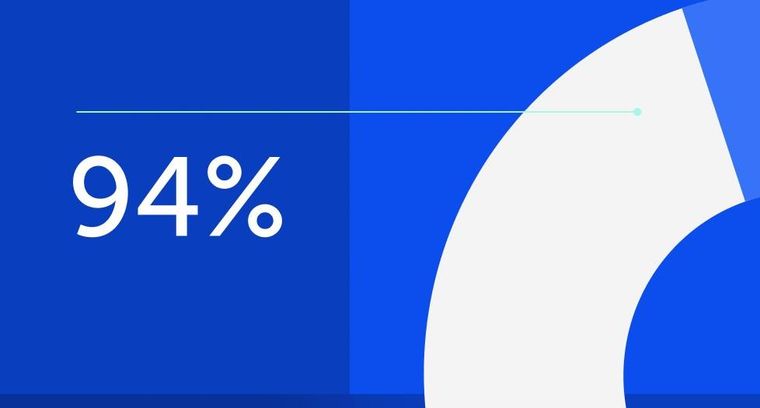
94% of researchers rate our articles as excellent or good
Learn more about the work of our research integrity team to safeguard the quality of each article we publish.
Find out more
OPINION article
Front. Mol. Neurosci., 31 May 2023
Sec. Molecular Signalling and Pathways
Volume 16 - 2023 | https://doi.org/10.3389/fnmol.2023.1095455
Obsessive-compulsive disorder (OCD) is a psychiatric disorder characterized by recurrent unwanted thoughts (obsessions) and associated repetitive behaviors (compulsions), affecting around 1.1–1.3% of the global population (Stein et al., 2016a; Fawcett et al., 2020). Over the past two decades, researchers have identified several distinct domains or dimensions of OCD symptomatology, with apparently distinctive neural correlates and differential responses to specific treatments (Mataix-Cols et al., 1999, 2004, 2005; van den Heuvel et al., 2009; Kichuk et al., 2013; Williams et al., 2014). These dimensions have also been identified at a “sub-syndromal” level in up to 13% of a large sample of adults from six countries, and include Contamination/Cleaning, Harm/Checking, Symmetry/Ordering, Hoarding, Sexual/Religious, Somatic and Moral obsessions and compulsions (Fullana et al., 2010). These findings suggest that OCD is best understood not as a unitary disorder, but as a group of related disorders.
Most contemporary models of OCD place a high degree of emphasis on the role of higher-level processes, such as cognitive flexibility or the sense of responsibility, in the genesis and maintenance of OCD symptoms. Such models can be considered as taking a “top-down” perspective (Poletti et al., 2022a). However, OCD-like phenomena have been documented in animals, such as dogs, cats and primates (Luescher et al., 1991; Overall and Dunham, 2002; Lutz, 2014). OCD is also frequently encountered in children, where higher-level cognitive processes are not yet fully developed (Geller, 2006). Such findings suggest a need for a “bottom-up” perspective based on evolutionary and neurodevelopmental models. Sigmund Freud was among the first to suggest a similarity between the rituals seen in OCD and behaviors that maintain social stability in the face of conflicting human drives. Though his model of OCD is no longer widely accepted, it does show some points of correspondence with contemporary biochemical or cognitive models (Katz, 1991; Moritz et al., 2011). More generally, it is now understood that many of the symptoms of OCD may represent exaggerations or distortions of phylogenetically ancient adaptive behaviors or defense mechanisms, whose purpose is to ensure individual or group wellbeing and safety (Boyer and Lienard, 2006; Feygin et al., 2006; Stein et al., 2016b). Such an evolutionary perspective regarding OCD entails a neurodevelopmental perspective, in which alterations in normal brain development could perturb basic, evolutionarily conserved neural processing systems and predispose to the development of OCD at specific stages of the life cycle in a “bottom-up” manner (Leckman and Bloch, 2008; Poletti et al., 2022a). Such mechanisms could potentially be identified in animals as well as humans.
In this connection, it is relevant to examine two particular rodent models of obsessive-compulsive disorder which share certain unexpected similarities. In 2009, it was observed that mice in whom the SAPAP3 gene had been deleted exhibited compulsive behaviors and increased anxiety reminiscent of OCD (Welch et al., 2007). This gene codes for a protein that is highly expressed in the corpus striatum and involved in post-synaptic scaffolding, and its disruption was associated with altered glutamatergic, gamma-amino butyric-acid (GABA)-ergic and dopaminergic transmission in the orbitofrontal cortex, corpus striatum and nucleus accumbens. These changes were associated not just with OCD-like behavior, but with impairments in lower-level (sensory processing) and higher-level (reversal learning, a measure of cognitive flexibility) processes (Wan et al., 2011; Manning et al., 2021; Yang et al., 2021). Observation of neonatal mice deficient in SAPAP3 has identified increases in ultrasonic vocalizations, a marker of altered communication and social development (Tesdahl et al., 2017).
More recently, it has been observed that mice in whom the SPRED2 gene was knocked out exhibit OCD-like behavior and anxiety, both of which are highly similar to those observed in SAPAP3-deficient mice (Ullrich et al., 2018). SPRED2 codes for a protein that is a key regulator of the Ras/ERK-MAPK pathway, an intracellular cascade that can be activated by brain-derived neurotrophic factor (BDNF); it has been shown to play a key role in neurogenesis and neural development, and possibly in synaptic vesicle transport. In SPRED2-deficient mice, alterations in neural transmission were observed in thalamo-amygdala circuits. Subsequently, it was found that these mice, like those in which SAPAP3 had been deleted, also showed altered ultrasonic vocalizations. These changes were observed in both young and older mice, and appeared to increase with age (Hepbasli et al., 2021). That these changes reflect a developmental anomaly is supported by evidence that SPRED2 is involved in central nervous system development in mice (Tuduce et al., 2010).
A relevant question in this context is whether alterations in either SPRED2 or SAPAP3 are associated with OCD in humans. While no studies of SPRED2 in patients with OCD have been published to date, a cautious affirmative answer can be offered in the case of SAPAP3. A specific four-locus haplotype of SAPAP3 has been associated with an earlier age of onset in OCD, again pointing to a possible effect on neurodevelopment (Boardman et al., 2011); an allelic variant in a specific single nucleotide polymorphism (rs6662980) of SAPAP3 has been specifically associated with the Contamination/Washing dimension of OCD, as well as with a poor response to serotonin reuptake inhibitors (Naaz et al., 2020); and two single-nucleotide polymorphisms in SAPAP3 have been associated with symptom severity in early-onset OCD (Mas et al., 2016). In addition, a genome-wide association study has found that variations in SAPAP1 (also known as DLGAP1), coding for a protein related to SAPAP3 which is also involved in synaptic connectivity, were significantly associated with clinical OCD (Stewart et al., 2013).
Copy number variants in SAPAP1 and the related gene SAPAP2 (DLGAP2) have also been associated with childhood OCD (Gazzellone et al., 2016). Mice in which SAPAP1 has been knocked out exhibit impaired scaffolding at glutamatergic synapses and altered social behavior (Coba et al., 2018). There is also evidence that variations in the BDNF and NTRK2 genes, which are proximal components of the same cellular cascade as SPRED2, may exert a protective effect against OCD; these effects may be mediated by beneficial effects on this particular signaling pathway (Alonso et al., 2008).
Taken together, these findings suggest that genes involved in neurodevelopment and synaptic connectivity, when disrupted, induce not just OCD-like behavior but alterations in brain development, sensory processing, cognitive functioning and social behavior in animals. At least one of these genes is also associated with certain facets of OCD in humans. There is evidence from animal research that alterations in these genes are associated with functional changes involving prefrontal, striatal and limbic brain regions. The consistency of these findings across rodents and humans suggests that at least some of the genetic mechanisms underlying OCD could be evolutionarily conserved. The fundamental phenotype involved in this process may represent lower-order deficits arising from alterations in neural development, which could influence higher-order cognitive processes in a “bottom-up” manner (Benzina et al., 2021; Poletti et al., 2022a,b). These findings are also consistent with research suggesting that deficits in lower-order sensory and affective processing may underline the cognitive and behavioral changes seen in patients with OCD (Cavedini et al., 2012; Martoni et al., 2015).
The argument presented above would gain support if it were possible to demonstrate neurodevelopmental antecedents of OCD in humans. In this case, too, the available evidence suggests that at least some types of OCD have developmental antecedents. The evidence for these developmental alterations has been summarized in recent reviews (Poletti et al., 2022a) and includes altered cortical and white matter development in early-onset OCD (Li et al., 2021; Park et al., 2022), functional alterations in cortico-striato-thalamic circuits in childhood OCD (Huyser et al., 2009; Liu et al., 2016), a higher frequency of neurological soft signs in OCD patients with poor insight or comorbid tics (Karadag et al., 2011; Ekinci and Ekinci, 2020), subtle alterations in facial morphology in early-onset OCD (Wang et al., 2021), and associations between OCD and events that could alter brain development either pre- or perinatally (Vasconcelos et al., 2007) or in early childhood (Barzilay et al., 2019; Wislocki et al., 2022).
Specific phenotypes have also been linked to these developmental alterations. For example, patients with OCD show evidence of impaired olfaction, which is a marker of brain dysfunction of developmental origin (Crow et al., 2020). However, the most frequently replicated phenotype of possible developmental origin in OCD involves alterations in sensory processing, most specifically sensory over-sensitivity. This phenomenon, which is characterized by increased sensitivity and reactivity to sensory stimuli in various modalities, has been documented both in children and adolescents (Houghton et al., 2020) and adults (Dar et al., 2012; Isaacs et al., 2022) with OCD. Sensory over-sensitivity has also been associated with childhood ritualistic behavior (Dar et al., 2012). While early childhood rituals are common and are usually “outgrown” in later childhood and adolescence, they may persist and evolve into OCD in some cases; in these cases, they may represent a developmental precursor of the full syndrome of OCD (Leckman and Bloch, 2008; Evans et al., 2011). Like clinical OCD, these childhood forerunners are associated with alterations in functional connectivity between limbic, sensorimotor, striatal and thalamic brain regions (Sunol et al., 2021). These changes have been linked to alterations in genes linked to glutamatergic neurotransmission (Sunol et al., 2022), which is one of the key pathways disrupted in SAPAP3 or SPRED2 knock-out mice.
An endophenotype is a heritable trait that can be measured in an objective manner, and which is present in individuals with a given psychiatric disorder, as well as their unaffected first-degree relatives, at rates significantly higher than in healthy controls or in the general population (Gottesman and Gould, 2003). It represents an “intermediate phenotype” that is genetically linked to the disorder in question and more amenable to study using biological methods. A number of candidate endophenotypes have been proposed for OCD (Vaghi, 2021). These include alterations in specific domains of cognition (Zartaloudi et al., 2019; Bora, 2020), structural abnormalities in specific brain regions such as the insula (Besiroglu et al., 2022), and altered patterns of functional activity within and between specific neural circuits involved in sensorimotor function, cognition and resting-state activity (Peng et al., 2021). Among these, cognitive endophenotypes have been the most frequently documented (Vaghi, 2021) and have been observed even in pediatric OCD (Abramovitch et al., 2021). Recent evidence suggests that the polygenic risk score, a measure of genetic vulnerability toward OCD, is significantly correlated with alterations in brain activity during the performance of cognitive tasks not just in patients with OCD and their unaffected relatives, but in healthy controls (Heinzel et al., 2021). This result suggests the possibility of a “continuum” of genetic vulnerability to OCD that could cause subtle deficits in higher-order cognitive functions, most probably through alterations in brain development and functional connectivity. Such a continuum has also been demonstrated in a genome-wide analysis of pediatric obsessive-compulsive disorder and traits (Burton et al., 2021). In the latter study, suggestive associations were identified for the genes GRID2 and PTPRD, which may be functionally linked to SAPAP3 (Pauls et al., 2014). As of now, there is no direct evidence linking this putative genetic continuum to abnormalities of sensory processing. However, a recent study of over 1,400 adolescents and adults, examining the entire spectrum of obsessive-compulsive phenomena, reported that sensory over-responsiveness was associated with this spectrum in a transdiagnostic manner (Moreno-Amador et al., 2023). Though requiring replication, this result suggests that the possibility of a genetically influenced neurodevelopmental vulnerability to symptoms across the OC spectrum may be linked to sensory over-sensitivity. This implies that the latter may be a useful endophenotype for OCD.
A tentative integration of the findings described above is presented in Figure 1, with the left-hand side of the figure indicating “normal” development and the right-hand side indicating the processes implicated in the pathogenesis of OCD. In this model, genetic factors (particularly those involved in neural development and synaptic scaffolding) interact with pre, peri- and post-natal exposures to cause structural and functional alterations in brain circuits involved in “lower-level” processes that are operational from early childhood, such as sensory processing and early social behavior (de Oliveira et al., 2021; Schiele et al., 2022). The available evidence suggests that neurotransmitters such as glutamate (Karthik et al., 2020; Auerbach et al., 2021) and oxytocin (Crucianelli et al., 2019; Bey et al., 2022) may be particularly involved in these processes as well as in OCD.
Figure 1. The relationship between genes involved in brain development and synaptic connectivity, “lower-” and “higher-order” information processing, and the development of obsessive-compulsive symptoms.
During the course of cognitive development in early life, alterations in these processes affects “higher-order” cognitive processes such as flexibility and decision-making capacities (Abramovitch et al., 2021). In children with no or minimal alterations to these circuits and processes, the result is transient childhood rituals and the subsequent development of appropriate rule- or ritual-based social and risk-avoidance behavior in later life. In those with more marked alterations in these processes, a transition to clinical OCD occurs (Poletti et al., 2022b). This may be more likely to happen at “critical” stages of the life cycle, or at any other period characterized by increased stress and a higher cognitive, affective or sensory load (Sousa-Lima et al., 2019; Imthon et al., 2020; Raposo-Lima and Morgado, 2020). Such periods include the transition from early to later childhood (Geller et al., 2001), the transition from adolescence to adulthood (Horwath and Weissman, 2000; Solmi et al., 2022), and pregnancy or childbirth in women (Starcevic et al., 2020). In other cases, infections or immune-inflammatory alterations may act as triggering factors (Gerentes et al., 2019). Finally, in those with intermediate alterations and/or lower levels of stress, subsyndromal OCD symptoms may occur and persist for a variable period (Fullana et al., 2009; Ramakrishnan et al., 2022).
The model outlined above represents a tentative yet coherent approach to understanding the mechanisms through which evolutionarily conserved cellular and neurobiological processes could contribute to the development of OCD in humans. Much remains to be learned about the specific association of each process with OCD, their relationship to the different dimensions of OCD, and the opportunities they offer for early intervention, improved treatment, and the identification of specific endophenotypes such as sensory over-sensitivity (Fontenelle et al., 2022). While many of the conclusions presented here require verification, they could potentially deepen our understanding of OCD and its evolutionary and developmental roots.
The sole author of this work was responsible for the conceptual framework, literature review, writing, editing, and proofreading of this paper.
The author declares that the research was conducted in the absence of any commercial or financial relationships that could be construed as a potential conflict of interest.
All claims expressed in this article are solely those of the authors and do not necessarily represent those of their affiliated organizations, or those of the publisher, the editors and the reviewers. Any product that may be evaluated in this article, or claim that may be made by its manufacturer, is not guaranteed or endorsed by the publisher.
Abramovitch, A., De Nadai, A. S., and Geller, D. A. (2021). Neurocognitive endophenotypes in pediatric OCD probands, their unaffected parents and siblings. Prog. Neuropsychopharmacol. Biol. Psychiatry 110:110283. doi: 10.1016/j.pnpbp.2021.110283
Alonso, P., Gratacos, M., Menchon, J. M., Saiz-Ruiz, J., Segalas, C., Baca-Garcia, E., et al. (2008). Extensive genotyping of the BDNF and NTRK2 genes defines protective haplotypes against obsessive-compulsive disorder. Biol. Psychiatry 63, 619–628. doi: 10.1016/j.biopsych.2007.06.020
Auerbach, B. D., Manohar, S., Radziwon, K., and Salvi, R. (2021). Auditory hypersensitivity and processing deficits in a rat model of fragile X syndrome. Neurobiol. Dis. 161:105541. doi: 10.1016/j.nbd.2021.105541
Barzilay, R., Patrick, A., Calkins, M. E., Moore, T. M., Gur, R. C., and Gur, R. E. (2019). Association between early-life trauma and obsessive compulsive symptoms in community youth. Depress. Anxiety 36, 586–595. doi: 10.1002/da.22907
Benzina, N., N'Diaye, K., Pelissolo, A., Mallet, L., and Burguiere, E. (2021). A cross-species assessment of behavioral flexibility in compulsive disorders. Commun. Biol. 4, 96. doi: 10.1038/s42003-020-01611-y
Besiroglu, L., Zalesky, A., Kasal, M. I., Dikmeer, N., Bilge, A., Durmaz, E., et al. (2022). Cortical thickness and surface area in patients with obsessive compulsive disorder and their unaffected siblings. Brain Imaging Behav. 16, 1946–1953. doi: 10.1007/s11682-022-00660-7
Bey, K., Campos-Martin, R., Klawohn, J., Reuter, B., Grutzmann, R., Riesel, A., et al. (2022). Hypermethylation of the oxytocin receptor gene (OXTR) in obsessive-compulsive disorder: further evidence for a biomarker of disease and treatment response. Epigenetics 17, 642–652. doi: 10.1080/15592294.2021.1943864
Boardman, L., van der Merwe, L., Lochner, C., Kinnear, C. J., Seedat, S., Stein, D. J., et al. (2011). Investigating SAPAP3 variants in the etiology of obsessive-compulsive disorder and trichotillomania in the South African white population. Compr. Psychiatry 52, 181–187. doi: 10.1016/j.comppsych.2010.05.007
Bora, E. (2020). Meta-analysis of neurocognitive deficits in unaffected relatives of obsessive-compulsive disorder (OCD): comparison with healthy controls and patients with OCD. Psychol. Med. 50, 1257–1266. doi: 10.1017/S0033291720001634
Boyer, P., and Lienard, P. (2006). Why ritualized behavior? precaution systems and action parsing in developmental, pathological and cultural rituals. Behav. Brain. Sci. 29, 595–613. doi: 10.1017/S0140525X06009332
Burton, C. L., Lemire, M., Xiao, B., Corfield, E. C., Erdman, L., Bralten, J., et al. (2021). Genome-wide association study of pediatric obsessive-compulsive traits: shared genetic risk between traits and disorder. Transl. Psychiatry 11, 91. doi: 10.1038/s41398-020-01121-9
Cavedini, P., Zorzi, C., Baraldi, C., Patrini, S., Salomoni, G., Bellodi, L., et al. (2012). The somatic marker affecting decisional processes in obsessive-compulsive disorder. Cogn. Neuropsychiatry 17, 177–190. doi: 10.1080/13546805.2011.614152
Coba, M. P., Ramaker, M. J., Ho, E. V., Thompson, S. L., Komiyama, N. H., Grant, S. G. N., et al. (2018). Dlgap1 knockout mice exhibit alterations of the postsynaptic density and selective reductions in sociability. Sci. Rep. 8, 2281. doi: 10.1038/s41598-018-20610-y
Crow, A. J. D., Janssen, J. M., Vickers, K. L., Parish-Morris, J., Moberg, P. J., and Roalf, D. R. (2020). Olfactory dysfunction in neurodevelopmental disorders: a meta-analytic review of autism spectrum disorders, attention deficit/hyperactivity disorder and obsessive-compulsive disorder. J. Autism Dev. Disord. 50, 2685–2697. doi: 10.1007/s10803-020-04376-9
Crucianelli, L., Paloyelis, Y., Ricciardi, L., Jenkinson, P. M., and Fotopoulou, A. (2019). Embodied precision: intranasal oxytocin modulates multisensory integration. J. Cogn. Neurosci. 31, 592–606. doi: 10.1162/jocn_a_01366
Dar, R., Kahn, D. T., and Carmeli, R. (2012). The relationship between sensory processing, childhood rituals and obsessive-compulsive symptoms. J. Behav. Ther. Exp. Psychiatry 43, 679–684. doi: 10.1016/j.jbtep.2011.09.008
de Oliveira, K. C., Camilo, C., Gastaldi, V. D., Feltrin, A. S., Lisboa, B. C. G., de Paula, V., et al. (2021). Brain areas involved with obsessive-compulsive disorder present different DNA methylation modulation. BMC Genom. Data 22, 45. doi: 10.1186/s12863-021-00993-0
Ekinci, O., and Ekinci, A. E. (2020). Neurological soft signs and clinical features of tic-related obsessive-compulsive disorder indicate a unique subtype. J. Nerv. Ment. Dis. 208, 21–27. doi: 10.1097/NMD.0000000000001098
Evans, D. W., Hersperger, C., and Capaldi, P. A. (2011). Thought-action fusion in childhood: measurement, development, and association with anxiety, rituals and other compulsive-like behaviors. Child Psychiatry Hum. Dev. 42, 12–23. doi: 10.1007/s10578-010-0198-x
Fawcett, E. J., Power, H., and Fawcett, J. M. (2020). Women are at greater risk of OCD than men: a meta-analytic review of OCD prevalence worldwide. J. Clin. Psychiatry 81:19r13085. doi: 10.4088/JCP.19r13085
Feygin, D. L., Swain, J. E., and Leckman, J. F. (2006). The normalcy of neurosis: evolutionary origins of obsessive-compulsive disorder and related behaviors. Prog. Neuropsychopharmacol. Biol. Psychiatry 30, 854–864. doi: 10.1016/j.pnpbp.2006.01.009
Fontenelle, L. F., Nicolini, H., and Brakoulias, V. (2022). Early intervention in obsessive-compulsive disorder: from theory to practice. Compr. Psychiatry 119:152353. doi: 10.1016/j.comppsych.2022.152353
Fullana, M. A., Mataix-Cols, D., Caspi, A., Harrington, H., Grisham, J. R., Moffitt, T. E., et al. (2009). Obsessions and compulsions in the community: prevalence, interference, help-seeking, developmental stability, and co-occurring psychiatric conditions. Am. J. Psychiatry 166, 329–336. doi: 10.1176/appi.ajp.2008.08071006
Fullana, M. A., Vilagut, G., Rojas-Farreras, S., Mataix-Cols, D., de Graaf, R., Demyttenaere, K., et al. (2010). Obsessive-compulsive symptom dimensions in the general population: results from an epidemiological study in six European countries. J. Affect. Disord. 124, 291–299. doi: 10.1016/j.jad.2009.11.020
Gazzellone, M. J., Zarrei, M., Burton, C. L., Walker, S., Uddin, M., Shaheen, S. M., et al. (2016). Uncovering obsessive-compulsive disorder risk genes in a pediatric cohort by high-resolution analysis of copy number variation. J. Neurodev. Disord. 8, 36. doi: 10.1186/s11689-016-9170-9
Geller, D. A. (2006). Obsessive-compulsive and spectrum disorders in children and adolescents. Psychiatr. Clin. North. Am. 29, 353–370. doi: 10.1016/j.psc.2006.02.012
Geller, D. A., Biederman, J., Faraone, S. V., Bellordre, C. A., Kim, G. S., Hagermoser, L., et al. (2001). Disentangling chronological age from age of onset in children and adolescents with obsessive-compulsive disorder. Int. J. Neuropsychopharmacol. 4, 169–178. doi: 10.1017/S1461145701002395
Gerentes, M., Pelissolo, A., Rajagopal, K., Tamouza, R., and Hamdani, N. (2019). Obsessive-compulsive disorder: autoimmunity and neuroinflammation. Curr. Psychiatry Rep. 21, 78. doi: 10.1007/s11920-019-1062-8
Gottesman, I. I., and Gould, T. D. (2003). The endophenotype concept in psychiatry: etymology and strategic intentions. Am. J. Psychiatry 160, 636–645. doi: 10.1176/appi.ajp.160.4.636
Heinzel, S., Kaufmann, C., Grutzmann, R., Klawohn, J., Riesel, A., Bey, K., et al. (2021). Polygenic risk for obsessive-compulsive disorder (OCD) predicts brain response during working memory task in OCD, unaffected relatives, and healthy controls. Sci. Rep. 11, 18914. doi: 10.1038/s41598-021-98333-w
Hepbasli, D., Gredy, S., Ullrich, M., Reigl, A., Abeβer, M., Raabe, T., et al. (2021). Genotype- and age-dependent differences in ultrasound vocalizations of SPRED2 mutant mice revealed by machine deep learning. Brain Sci. 11:1365. doi: 10.3390/brainsci11101365
Horwath, E., and Weissman, M. M. (2000). The epidemiology and cross-national presentation of obsessive-compulsive disorder. Psychiatr. Clin. North. Am. 23, 493–507. doi: 10.1016/s0193-953x(05)70176-3
Houghton, D. C., Stein, D. J., and Cortese, B. M. (2020). Review: exteroceptive sensory abnormalities in childhood and adolescent anxiety and obsessive-compulsive disorder: a critical review. J. Am. Acad. Child Adolesc. Psychiatry 59, 78–87. doi: 10.1016/j.jaac.2019.06.007
Huyser, C., Veltman, D. J., de Haan, E., and Boer, F. (2009). Paediatric obsessive-compulsive disorder, a neurodevelopmental disorder? evidence from neuroimaging. Neurosci. Biobehav. Rev. 33, 818–830. doi: 10.1016/j.neubiorev.2009.01.003
Imthon, A. K., Caldart, C. A., do Rosario, M. C., Fontenelle, L. F., Miguel, E. C., and Ferrao, Y. A. (2020). Stressful life events and the clinical expression of obsessive-compulsive disorder: an exploratory study. J. Clin. Med. 9, 3371. doi: 10.3390/jcm9103371
Isaacs, D., Key, A. P., Cascio, C. J., Conley, A. C., Riordan, H., Walker, H. C., et al. (2022). Cross-disorder comparison of sensory over-responsivity in chronic tic disorders and obsessive-compulsive disorder. Compr. Psychiatry 113, 152291. doi: 10.1016/j.comppsych.2021.152291
Karadag, F., Tumkaya, S., Kirtas, D., Efe, M., Alacam, H., and Oguzhanoglu, N. K. (2011). Neurological soft signs in obsessive compulsive disorder with good and poor insight. Prog. Neuropsychopharmacol. Biol. Psychiatry 35, 1074–1079. doi: 10.1016/j.pnpbp.2011.03.003
Karthik, S., Sharma, L. P., and Narayanaswamy, J. C. (2020). Investigating the role of glutamate in obsessive-compulsive disorder: current perspectives. Neuropsychiatr. Dis. Treat. 16, 1003–1013. doi: 10.2147/NDT.S211703
Katz, R. J. (1991). Neurobiology of obsessive compulsive disorder – a serotonergic basis of Freudian repression. Neurosci. Biobehav. Rev. 15, 375–381. doi: 10.1016/S0149-7634(05)80030-2
Kichuk, S. A., Torres, A. R., Fontenelle, L. F., Rosario, M. C., Shavitt, R. G., Miguel, E. C., et al. (2013). Symptom dimensions are associated with age of onset and clinical course of obsessive-compulsive disorder. Prog. Neuropsychopharmacol. Biol. Psychiatry 44, 233–239. doi: 10.1016/j.pnpbp.2013.02.003
Leckman, J. F., and Bloch, M. H. (2008). A developmental and evolutionary perspective on obsessive-compulsive disorder: whence and whither compulsive hoarding? Am. J. Psychiatry 165, 1229–1233. doi: 10.1176/appi.ajp.2008.08060891
Li, Q., Zhao, Y., Huang, Z., Guo, Y., Long, J., Luo, L., et al. (2021). Microstructural white matter abnormalities in pediatric and adult obsessive-compulsive disorder: a systematic review and meta-analysis. Brain Behav. 11:e1975. doi: 10.1002/brb3.1975
Liu, Y., Bilek, E. L., and Fitzgerald, K. D. (2016). Developmental neuroimaging in pediatric obsessive-compulsive disorder. Curr. Behav. Neurosci. Rep. 3, 193–203. doi: 10.1007/s40473-016-0086-1
Luescher, U. A., McKeown, D. B., and Halip, J. (1991). Sterotypic or obsessive-compulsive disorders in dogs and cats. Vet. Clin. North Am. Small Anim. Pract. 21, 401–413. doi: 10.1016/S0195-5616(91)50041-3
Lutz, C. K. (2014). Sterotypic behavior in nonhuman primates as a model for the human condition. ILAR J. 55, 284–296. doi: 10.1093/ilar/ilu016
Manning, E. E., Wang, A. Y., Saikali, L. M., Winner, A. S., and Ahmari, S. E. (2021). Disruption of prepulse inhibition is associated with compulsive behavior severity and nucleus accumbens dopamine receptor changes in Sapap3 knockout mice. Sci. Rep. 11, 9442. doi: 10.1038/s41598-021-88769-5
Martoni, R. M., Brombin, C., Nonis, A., Salgari, G. C., Buongiorno, A., Cavallini, M. C., et al. (2015). Evaluating effect of symptoms heterogeneity on decision-making ability in obsessive-compulsive disorder. Psychiatry Clin. Neurosci. 69, 402–410. doi: 10.1111/pcn.12264
Mas, S., Gasso, P., Morer, A., Calvo, A., Bargallo, N., Lafuente, A., et al. (2016). Integrating genetic, neuropsychological and neuroimaging data to model early-onset obsessive compulsive disorder severity. PLoS ONE 11:e0153846. doi: 10.1371/journal.pone.0153846
Mataix-Cols, D., Rauch, S. L., Manzo, P. A., Jenike, M. A., and Baer, L. (1999). Use of factor-analyzed symptom dimensions to predict outcome with serotonin reuptake inhibitors and placebo in the treatment of obsessive-compulsive disorder. Am. J. Psychiatry 156, 1409–1416. doi: 10.1176/ajp.156.9.1409
Mataix-Cols, D., Rosario-Campos, M. C., and Leckman, J. F. (2005). A multidimensional model of obsessive-compulsive disorder. Am. J. Psychiatry 162, 228–238. doi: 10.1176/appi.ajp.162.2.228
Mataix-Cols, D., Wooderson, S., Lawrence, N., Brammer, M. J., Speckens, A., and Phillips, M. L. (2004). Distinct neural correlates of washing, checking, and hoarding symptom dimensions in obsessive-compulsive disorder. Arch. Gen. Psychiatry 61, 564–576. doi: 10.1001/archpsyc.61.6.564
Moreno-Amador, B., Cervin, M., Martinez-Gonzalez, A. E., Piqueras, J. A., and O. C. D.-Spectrum Spain Research Group (2023). Sensory overresponsivity and symptoms across the obsessive-compulsive spectrum: web-based longitudinal observational study. J. Med. Internet Res. 25:e37847. doi: 10.2196/37847
Moritz, S., Kempke, S., Luyten, P., Randjbar, S., and Jelinek, L. (2011). Was Freud partly right on obsessive-compulsive disorder? investigation of latent aggression in OCD. Psychiatry Res. 187, 180–184. doi: 10.1016/j.psychres.2010.09.007
Naaz, S., Balachander, S., Murthy, N. S., Bhagyalakshmi, M. S., Sud, R., Saha, P., et al. (2020). Exp. Clin. Psychopharmacol. 30, 106–112. doi: 10.1037/pha0000422
Overall, K. L., and Dunham, A. E. (2002). Clinical features and outcome in dogs and cats with obsessive-compulsive disorder: 126 cases (1989-2000). J. Am. Vet. Med. Assoc. 221, 1445–1452. doi: 10.2460/javma.2002.221.1445
Park, I., Ha, M., Kim, T., Lho, S. K., Moon, S. -Y., Kim, M., et al. (2022). Cortical gyrification differences between early- and late-onset obsessive-compulsive disorder: neurobiological evidence for neurodevelopmentally distinct subtypes. Psychol. Med. 1–10. doi: 10.1017/S0033291722003129
Pauls, D. L., Abramovitch, A., Rauch, S. L., and Geller, D. A. (2014). Obsessive-compulsive disorder: an integrative genetic and neurobiological perspective. Nat. Rev. Neurosci. 15, 410–424. doi: 10.1038/nrn3746
Peng, Z., Guo, Y., Wu, X., Yang, Q., Wei, Z., Seger, C. A., et al. (2021). Abnormal brain functional network dynamics in obsessive-compulsive disorder patients and their unaffected first-degree relatives. Hum. Brain Mapp. 42, 4387–4398. doi: 10.1002/hbm.25555
Poletti, M., Gebhardt, E., Pelizza, L., Preti, A., and Raballo, A. (2022a). Neurodevelopmental antecedents and sensory phenomena in obsessive compulsive disorder: a systematic review supporting a phenomenological-developmental model. Psychopathology. 1–11. doi: 10.1159/000526708
Poletti, M., Gebhardt, E., and Raballo, A. (2022b). Along the fringes of agency: neurodevelopmental account of the obsessive mind. CNS Spectr. 57, 557–560. doi: 10.1017/S1092852921000560
Ramakrishnan, S., Robbins, T. W., and Zmigrod, L. (2022). Cognitive rigidity, habitual tendencies, and obsessive-compulsive symptoms: individual differences and compensatory interactions. Front. Psychiatry 13:865896. doi: 10.3389/fpsyt.2022.865896
Raposo-Lima, C., and Morgado, P. (2020). The role of stress in obsessive-compulsive disorder: a narrative review. Harv. Rev. Psychiatry 28, 356–370. doi: 10.1097/HRP.0000000000000274
Schiele, M. A., Lipovsek, J., Schlosser, P., Soutschek, M., Schratt, G., Zaudig, M., et al. (2022). Epigenome-wide DNA methylation in obsessive-compulsive disorder. Transl. Psychiatry 12, 221. doi: 10.1038/s41398-022-01996-w
Solmi, M., Radua, J., Olivola, M., Croce, E., Soardo, L., Salazar de Pablo, G., et al. (2022). Age at onset of mental disorders worldwide: large-scale meta-analysis of 192 epidemiological studies. Mol. Psychiatry 27, 281–295. doi: 10.1038/s41380-021-01161-7
Sousa-Lima, J., Moreira, P. S., Raposo-Lima, C., Sousa, N., and Morgado, P. (2019). Relationship between obsessive compulsive disorder and cortisol: systematic review and meta-analysis. Eur. Neuropsychopharmacol. 29, 1185–1198. doi: 10.1016/j.euroneuro.2019.09.001
Starcevic, V., Eslick, G. D., Viswasam, K., and Berle, D. (2020). Symptoms of obsessive-compulsive disorder during pregnancy and the postpartum period: a systematic review and meta-analysis. Psychiatr. Q. 91, 965–981. doi: 10.1007/s11126-020-09769-8
Stein, D. J., Hermesh, H., Eilam, D., Segalas, C., Zohar, J., Menchon, J., et al. (2016b). Human compulsivity: a perspective from evolutionary medicine. Eur. Neuropsychopharmacol. 26, 869–876. doi: 10.1016/j.euroneuro.2015.12.004
Stein, D. J., Kogan, C. S., Atmaca, M., Fineberg, N. A., Fontenelle, L. F., Grant, J. E., et al. (2016a). The classification of obsessive-compulsive and related disorders in the ICD-11. J. Affect. Disord. 190, 663–674. doi: 10.1016/j.jad.2015.10.061
Stewart, S. E., Yu, D., Scharf, J. M., Neale, B. M., Fagerness, J. A., Mathews, C. A., et al. (2013). Genome-wide association study of obsessive-compulsive disorder. Mol. Psychiatry 18, 788–798. doi: 10.1038/mp.2012.85
Sunol, M., Alemany, S., Bustamante, M., Diez, I., Contreras-Rodriguez, O., Laudo, B., et al. (2022). Neurogenetics of dynamic connectivity patterns associated with obsessive-compulsive symptoms in healthy children. Biol. Psychiatry Glob. Open Sci. 2, 411–420. doi: 10.1016/j.bpsgos.2021.11.009
Sunol, M., Saiz-Masvidal, C., Contreras-Rodriguez, M.acia, D., Martinez-Vilavella, G., Martinez-Zalacain, I., Menchon, J. M., et al. (2021). Brain functional connectivity correlates of subclinical obsessive-compulsive symptoms in healthy children. J. Am. Acad. Child Adolesc. Psychiatry 60, 757–767. doi: 10.1016/j.jaac.2020.08.435
Tesdahl, N. S., King, D. K., McDaniel, L. N., and Pieper, A. A. (2017). Altered ultrasonic vocalization in neonatal SAPAP3-deficient mice. Neuroreport 28, 1115–1118. doi: 10.1097/WNR.0000000000000863
Tuduce, I. L., Schuh, K., and Bundschu, K. (2010). SPRED2 expression during mouse development. Dev. Dyn. 239, 3072–3085. doi: 10.1002/dvdy.22432
Ullrich, M., Weber, M., Post, A. M., Popp, S., Grein, J., Zechner, M., et al. (2018). OCD-like behavior is caused by dysfunction of thalamo-amygdala circuits and upregulated TrkB/ERK-MAPK signaling as a result of SPRED2 deficiency. Mol. Psychiatry 23, 444–458. doi: 10.1038/mp.2016.232
Vaghi, M. M. (2021). Neurocognitive endophenotypes of OCD. Curr. Top. Behav. Neurosci. 49, 97–124. doi: 10.1007/7854_2020_197
van den Heuvel, O. A., Remijnse, P. L., Mataix-Cols, D., Vrenken, H., Groenewegen, H. J., Uylings, H. B. M., et al. (2009). The major symptom dimensions of obsessive-compulsive disorder are mediated by partially distinct neural systems. Brain 132, 853–868. doi: 10.1093/brain/awn267
Vasconcelos, M. S., Sampaio, A. S., Hounie, A. G., Akkerman, F., Curi, M., Lopes, A. C., et al. (2007). Prenatal, perinatal, and postnatal risk factors in obsessive-compulsive disorder. Biol. Psychiatry 61, 301–307. doi: 10.1016/j.biopsych.2006.07.014
Wan, Y., Feng, G., and Calakos, N. (2011). SAPAP3 deletion causes mGluR5-dependent silencing of AMPAR synapses. J. Neurosci. 31, 16685–16691. doi: 10.1523/JNEUROSCI.2533-11.2011
Wang, Y. -R., Chang, S. -H., Ma, X. -M., Li, J. -Y., Zhang, R. -X., and Fang, J. -Q. (2021). Correlational research on facial and clinical characteristics of adolescents with obsessive-compulsive disorder. BMC Psychiatry 21, 623. doi: 10.1186/s12888-021-03612-5
Welch, J. M., Lu, J., Rodriguiz, R. M., Trotta, N. C., Peca, J., Ding, J. -D., et al. (2007). Cortico-striatal synaptic defects and OCD-like behaviors in SAPAP3 mutant mice. Nature 448, 894–900. doi: 10.1038/nature06104
Williams, M. T., Farris, S. G., Turkheimer, E. N., Franklin, M. E., Simpson, H. B., Liebowitz, M., et al. (2014). The impact of symptom dimensions on outcome for exposure and ritual prevention therapy in obsessive-compulsive disorder. J. Anxiety Disord. 28, 553–558. doi: 10.1016/j.janxdis.2014.06.001
Wislocki, K., Kratz, H. E., Martin, G., and Becker-Haimes, E. M. (2022). The relationship between trauma exposure and obsessive-compulsive disorder in youth: a systematic review. Child Psychiatry Hum. Dev. doi: 10.1007/s10578-022-01352-5
Yang, Z., Wu, G., Liu, M., Sun, X., Xu, Q., Zhang, C., et al. (2021). Dysfunction of orbitofrontal GABAergic interneurons leads to impaired reversal learning in a mouse model of obsessive-compulsive disorder. Curr. Biol. 31, 381–393. doi: 10.1016/j.cub.2020.10.045
Keywords: obsessive-compulsive disorder, SAPAP3, SPRED2, animal models, endophenotypes, sensory over-sensitivity, neurodevelopmental model
Citation: Rajkumar RP (2023) SAPAP3, SPRED2, and obsessive-compulsive disorder: the search for fundamental phenotypes. Front. Mol. Neurosci. 16:1095455. doi: 10.3389/fnmol.2023.1095455
Received: 11 November 2022; Accepted: 15 May 2023;
Published: 31 May 2023.
Edited by:
Ildikó Rácz, University Hospital Bonn, GermanyReviewed by:
Ilenia Pampaloni, South West London and St. George's Mental Health NHS Trust, United KingdomCopyright © 2023 Rajkumar. This is an open-access article distributed under the terms of the Creative Commons Attribution License (CC BY). The use, distribution or reproduction in other forums is permitted, provided the original author(s) and the copyright owner(s) are credited and that the original publication in this journal is cited, in accordance with accepted academic practice. No use, distribution or reproduction is permitted which does not comply with these terms.
*Correspondence: Ravi Philip Rajkumar, cmF2aS5wc3ljaEBnbWFpbC5jb20=
Disclaimer: All claims expressed in this article are solely those of the authors and do not necessarily represent those of their affiliated organizations, or those of the publisher, the editors and the reviewers. Any product that may be evaluated in this article or claim that may be made by its manufacturer is not guaranteed or endorsed by the publisher.
Research integrity at Frontiers
Learn more about the work of our research integrity team to safeguard the quality of each article we publish.