Corrigendum: Potential therapeutic role of SIRT1 in age- related hearing loss
- 1Department of Otorhinolaryngology-Head and Neck Surgery, The Third Affiliated Hospital of Southern Medical University, Guangzhou, China
- 2Guangdong Provincial Key Laboratory of Bone and Joint Degeneration Diseases, Guangzhou, China
Age-related hearing loss (ARHL) is a major public health burden worldwide that profoundly affects the daily life of elderly people. Silent information regulator 1 (SIRT1 or Sirtuin1), known as a regulator of the cell cycle, the balance of oxidation/antioxidant and mitochondrial function, has been proven to have anti-aging and life-extending effects, and its possible connection with ARHL has received increasing attention in recent years. This paper provides an overview of research on the connection between SIRT1 and ARHL. Topics cover both the functions of SIRT1 and its important role in ARHL. This review concludes with a look at possible research directions for ARHL in the future.
Introduction
Age-related hearing loss
Hearing loss, in its variants conductive, sensorineural or mixed, may be classified according to its etiology as hereditary, traumatic, environmental, and induced by drugs (Cunningham and Tucci, 2017). According to the WHO 2021 World Report on Hearing, hearing loss currently affects approximately 1.6 billion people worldwide, or 20.3% of the global population, and more than 5.5% (430 million) of the world’s population have moderate or higher hearing loss. Globally, hearing loss is the third leading cause of disability (GBD 2019 Diseases and Injuries Collaborators, 2020; Tordrup et al., 2021). The number of people with hearing loss is expected to reach nearly 250 million by 2050. Unresolved hearing loss costs more than $980 billion annually globally (World Health Organization [WHO], 2021). WHO is scaling up health interventions for the ear and hearing, and expects to avert more than 130 million disability-adjusted life years over the next decade. These gains translate into a monetary value of more than $1.3 trillion. In addition, investments in hearing care will result in more than $2 trillion in productivity gains worldwide by 2030 (Tordrup et al., 2021). Therefore, it is significant to intervene and research on hearing loss.
Age-related hearing loss (ARHL), also known as presbycusis, is a progressive decline in hearing sensitivity and language comprehension that is associated with aging. Therefore, this can seriously affect the social function and mental health of the elderly individuals (Bowl and Dawson, 2019; Noble et al., 2022). Current research suggests that ARHL can promote the development of cognitive dysfunction (Loughrey et al., 2018; Golub et al., 2020). As the population ages, the prevalence of ARHL will continue to increase, which will greatly increase the socioeconomic burden (Jafari et al., 2019). Therefore, research on ARHL is urgently needed.
Hearing, as people’s internal sense, is important for daily communication, and hearing impairment can greatly affect social function and mental health. Hearing impairment can seriously affect people’s social functioning and social health. It is now generally accepted that hearing loss is an independent risk factor for cognitive dysfunction (Golub et al., 2020; Lozupone et al., 2020). There are several hypotheses to explain the potential relationship between auditory and cognitive impairment; one hypothesis is that is that the relationship is underpinned by general neurodegeneration in aging, while the other hypothesis suggests that auditory impairment and sensory deprivation are causally linked to cognitive impairment (Slade et al., 2020). At present, whether the two are causal or mutually reinforcing needs to be further verified. With the progress of population aging, the prevalence of ARHL will continue to increase in the future, which will greatly increase the socioeconomic burden.
The inner ear pathology of ARHL is characterized by loss of inner and outer hair cells, vascular stripe atrophy, loss of spiral ganglion cells, and degeneration of spiral ligaments (Bowl and Dawson, 2019). It is currently believed that ARHL can be caused by deafness-related genes, noise pollution, and other single or interactive effects, while the accumulation of chronic oxidative stress damage with aging is likely to be a major cause of ARHL (Fujimoto and Yamasoba, 2014). The C57BL/6J mouse is an excellent animal model for studying ARHL. C57BL/6J mice show hearing loss with age, with elevated hearing thresholds starting at 6 months or even as early as approximately 3 months of age (Hequembourg and Liberman, 2001) and a linear trend of increasing hearing thresholds as time progresses (Ison et al., 2007).
Comparing human glucose-6-phosphate dehydrogenase (G6PD) gene-transferred C57BL/6 mice with wild-type mice, G6PD overexpression was found to enhance antioxidant capacity, attenuate oxidative damage and delay the onset of hearing loss (Bermúdez-Muñoz et al., 2020). p43 knockout mice exhibited more severe and earlier onset of hearing loss with age progression than wild-type C57BL/6 mice, precisely because of mitochondrial dysfunction and increased oxidative damage due to p43 gene deletion (Affortit et al., 2021). Mitochondrial dysfunction and oxidative stress are common features in neurodegeneration and aging (Giordano et al., 2013; Fang et al., 2014), now evidence indicates that the dysregulation of glial cells (e.g., satellite cells) and degeneration of the ganglion node structure are important mechanisms of ARHL (Panganiban et al., 2022). These studies suggest that a strong antioxidant function plays an important role in delaying ARHL. Mitigating oxidative damage and restoring the balance of the oxidative/antioxidant system are major hot spots in current research on the prevention and treatment of ARHL. Therefore, SIRT1, which has good antioxidant, anti-aging, and neuroprotective effects (Giordano et al., 2013), is receiving increasing attention in the study of ARHL.
Silent information regulator 1
SIRT1, a nicotinamide adenine dinucleotide (NAD+)-dependent class III histone deacetylase, is associated with lifespan extension. It is known for its ability to balance oxidation/antioxidation and reduce mitochondrial damage, DNA damage and apoptosis (Chen C. et al., 2020). It has been found to play a non-negligible role in ARHL (Salam et al., 2021).
Silent information regulator 1 in the inner ear
SIRT1 is widely distributed in various organs and tissues throughout the body and is involved in anti-aging, anti-inflammatory, antioxidant, and cell cycle regulation in the brain, liver and other important organs (El Hayek et al., 2019; de Gregorio et al., 2020; Zhang X. S. et al., 2021; Kadono et al., 2022). It has been confirmed that SIRT1 is also present in the cochlea (Xiong et al., 2014). In the mouse cochlea, SIRT1 was expressed in the inner and outer hair cells, marginal, and intermediate cells of the vascular striae, spiral ligament, and spiral ganglion cells. Immunofluorescence and histochemical results showed that SIRT1 was expressed in the nuclei of the above cells, and the expression in aged mice was significantly lower than that in young mice (Chang et al., 2018; Pang et al., 2019). The results confirmed that SIRT1 is strongly associated with ARHL and deserves in-depth study by audiologists.
SIRT1 plays a crucial role in hearing loss, especially ARHL. This review elucidates the structure and function of SIRT1 and its localization in the cochlea. Moreover, it mainly explains the relationship between SIRT1 and ARHL, as well as the current research progress related to SIRT1 and the prevention or treatment of ARHL, and provides our own views on future treatment and research strategies for ARHL.
Structure and function of silent information regulator 1
Sirtuins are (NAD +)-dependent class III histone deacetylases. The family consists of seven members in mammals (SIRT1, SIRT2, SIRT3, SIRT4, SIRT5, SIRT6, and SIRT7). These family members are characterized by the possession of the sirtuin core structural domain (Frye, 1999). Sirtuins are associated with many pathophysiological activities, such as genome stabilization, cancer, oxidative stress response, apoptosis, metabolism, aging, proliferation, and inflammation (Bonkowski and Sinclair, 2016). SIRT1 is the first to be identified and is more recognized in the sirtuins family (Haigis and Sinclair, 2010). In mammals, SIRT1 is composed of 747 amino acids and includes three regions: the central core (273–517 amino acids), which possesses the structural domain of the deacetylase; the N-and C-terminal regions, which are located on either side of the enzyme core; and the catalytic domain, which consists of 250 amino acids and is highly conserved among species (Sauve et al., 2006). SIRT1 controls DNA transcription by transferring acetyl groups from ε-N-acetyllysine amino acids to histones of DNA (Maiese, 2021). It has been suggested that SIRT1 is a possible substrate for autophagy. SIRT1 is mainly located in the nucleus, but also in the cytoplasm and mitochondria, and is closely associated with senescence when it is present in the cytoplasm (Xu et al., 2020). Silent information regulator 2 (Sir2) is a mammalian homolog highly similar to SIRT1 (Smith, 2002). In 1999, it was found that the lifespan of Sir2 mutant yeast cells was significantly shorter, while the lifespan of yeast overexpressing Sir2 was longer than that of wild type yeast (Kaeberlein et al., 1999). SIRT1 was also found to extend the lifespan of Cryptobacterium hidradenum (Tissenbaum and Guarente, 2001), mice (Satoh et al., 2013), and flies (Rogina and Helfand, 2004); this effect was associated with caloric restriction, which means that caloric restriction activates SIRT1 to exert lifespan-extending effects.
The deacetylation of SIRT1 reverses the acetylation of lysine residues on its target proteins by hydrolyzing an NAD+ and generating nicotinamide and a unique metabolite called 2′-O-acetyl-ADP-ribose (Hwang et al., 2013). SIRT1 participates not only in the regulation of the cell cycle by deacetylating histones, but also in positively or negatively regulating downstream targets that play key roles in various physiological activities, such as oxidative/antioxidant homeostasis, inflammation, and energy metabolism (Figure 1). For example, adenosine 5′-monophosphate (AMP)-activated protein kinase (AMPK) is an AMP-dependent, essential kinase that regulates energy homeostasis. The AMPK and SIRT1 signaling pathways are evolutionarily conserved energy sensors; AMPK senses changes in the cellular AMP/ATP ratio, and SIRT1 senses changes in the NAD+/NADH ratio (Salminen and Kaarniranta, 2012). AMPK enhances SIRT1 activity by increasing the NAD + concentration; in turn, SIRT1 activation can promote AMPK activity (Cantó et al., 2009). The important function of the AMPK/SIRT1 signaling pathway in regulating autophagy, anti-inflammatory, and antioxidant activities has been studied extensively. The activation of the AMPK/SIRT1 signaling pathway by drugs to treat metabolic and aging-related diseases is also a current research topic (Maharajan and Cho, 2021; Wang et al., 2021). Peroxisome proliferator-activated receptor gamma coactivator 1-alpha (PGC-1α), the downstream factor of SIRT1, is involved in the regulation of many cellular physiological functions, including the control of mitochondrial homeostasis and reactive oxygen species (ROS) levels (Johri et al., 2013), and activation of PGC-1α requires deacetylation of SIRT1 and dephosphorylation of AMPK (Kaarniranta et al., 2018; Xu et al., 2021). Currently, aging is accompanied by a decrease in autophagic efficiency (Salminen and Kaarniranta, 2009; Barnes et al., 2019). SIRT1 can regulate the level of autophagy by deacetylating p53, which has been shown to inhibit autophagy when located in the cytoplasm but promote autophagy when located in the nucleus (Kroemer et al., 2010). This observation is consistent with the idea that SIRT1 has different cellular localizations at different stages of the cell cycle. SIRT1 is transferred from the nucleus to the cytoplasm in senescent cells, thus decreasing autophagy. SIRT1 also deacetylates forkhead box Os (FoXOs), enhancing cellular resistance to oxidative stress (Singh and Ubaid, 2020). Moreover, nuclear factor kappa B (NF-κB), a key regulator of inflammation, can be inhibited by SIRT1, reducing the expression of inflammatory factors and thus acting as an anti-inflammatory agent (de Gregorio et al., 2020). There is growing evidence that microRNAs, small non-coding single-stranded RNAs, are involved in SIRT1 regulation, and regulating the expression levels of specific miRNAs may be a new therapeutic strategy (Munk et al., 2017; Barnes et al., 2019).
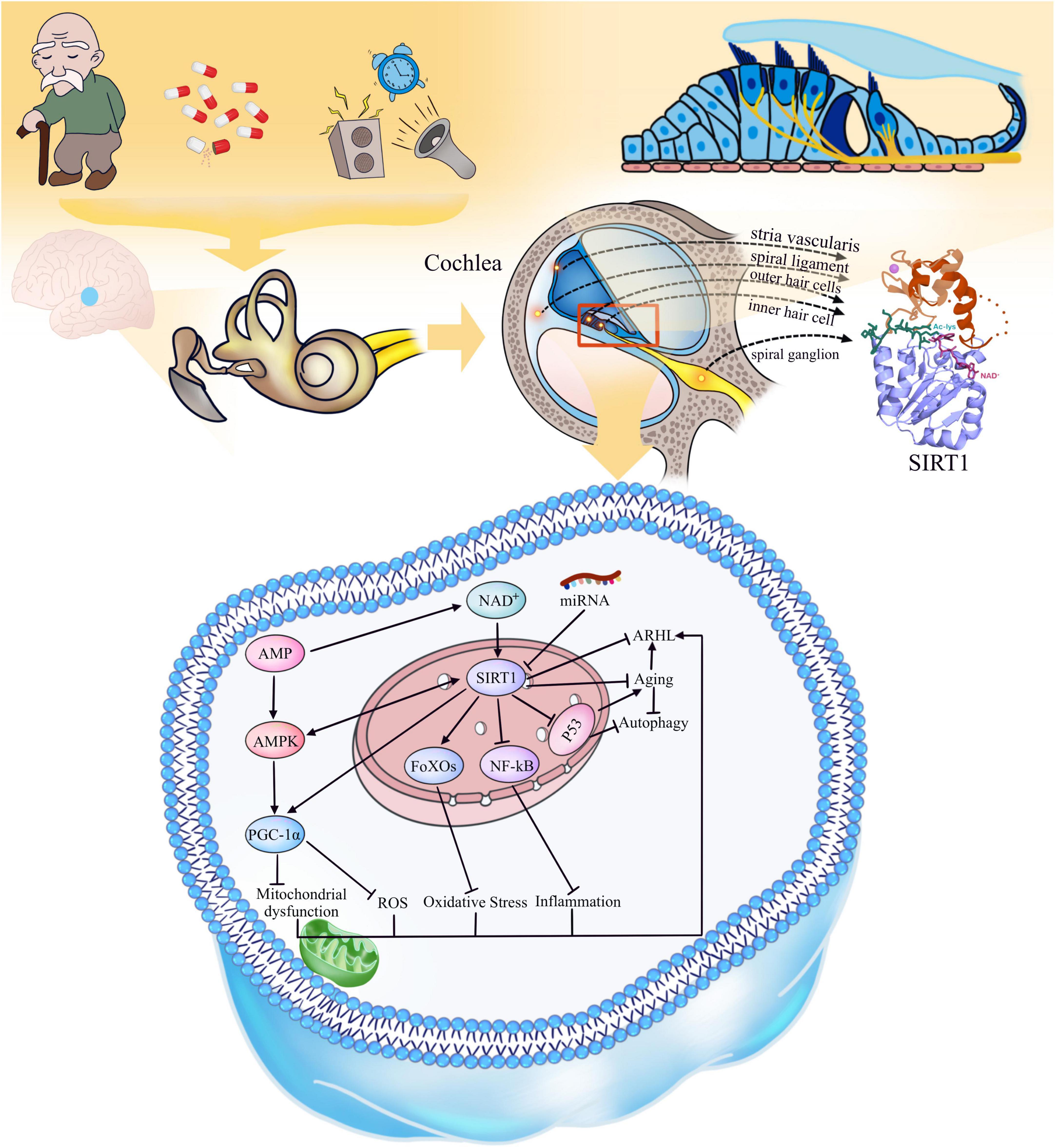
Figure 1. Aging and environmental factors lead to the decline of auditory center and inner ear functions, resulting in ARHL. Distribution of SIRT1 in cochlea: SIRT1 is expressed in inner and outer hair cells, spiral ligament, margin cells and intermediate cells of stria vascularis, and spiral ganglion cells of cochlea. The function of SIRT1: SIRT1 is regulated by NAD +, AMPK is regulated by AMP, and AMPK and SIRT1 can promote each other, while some specific miRNAs can inhibit the activity of SIRT1. SIRT1 can activate PGC-1α and FoXOs, enhance the resistance to oxidative stress damage, keep the normal function of mitochondria; SIRT1 can also deacetylate to reduce the activity of NF-κB, p53 and other inflammatory and aging-related factors to reduce their activity and promote the increase of autophagy. As a consequence, SIRT1 can delay the aging and age-related hearing loss.
Current studies on silent information regulator 1 and age-related hearing loss
A search of PUBMED for the keywords “SIRT1,” “hearing loss,” “cochlea,” “presbycusis” and “age-related hearing loss,” showed that there were less than 50 studies related to SIRT1 and hearing until now, of which 14 studies (Table 1) were related to ARHL.
In 2013, this is the first study to demonstrate the presence of SIRT1 in the cochlea and auditory cortex, which was significantly expressed in the nuclei of inner and outer hair cells, vascular striated marginal and basal cells, spiral ligament fibroblasts, and spiral ganglion cells and decreased with age (Xiong et al., 2014). Overexpression of miR-34a inhibited SIRT1 with increased apoptosis. After resveratrol administration, miR-34a could be inhibited and the apoptosis of hair cells was reduced, as well as the threshold drift in mice (Xiong et al., 2015). Later, SIRT1 overexpression in mice by the transgenic technique confirmed that overexpression of SIRT1 could protect cochlear hair cells and improve ARHL (Xiong et al., 2019). In contrast, one study in 2016 suggested that SIRT1 deficiency activated Foxo3a, enhanced the resistance of cochlear hair cells to oxidative stress damage, and delayed the occurrence of ARHL (Han et al., 2016). However, the results of several relevant studies in recent years suggest the opposite of this conclusion.
In 2019, researchers compared 2- and 12-month-old C57BL/6 mice, showing that SIRT1 expression was significantly decreased in 12-month-old mice. However, resveratrol, a natural SIRT1 activator, was added to the diet, and the mice were fed for a total of 10 months, beginning at ∼2 months of age. Compared with the conventionally fed mice of the same age, the supplementation of resveratrol increased the SIRT1 level in the outer hair cells of the cochlea of mice and induced autophagy and significantly improved the ARHL (Pang et al., 2019).
The normal and orderly physiological activities cannot be separated from the normal functioning of mitochondria (Rossmann et al., 2021). P43, as the mitochondrial receptor of T3 (Wrutniak et al., 1995), is closely related to mitochondrial function (Grandemange et al., 2005). It was found that mice with selective knockout of the P43 gene did not exhibit hearing loss at a young age, but compared with wild-type C57BL/6J mice, p43 knockout mice (p43-/-) showed an earlier increase in hearing threshold and more severe hearing loss with age and exhibited greater sensitivity to noise damage. The authors suggested that this may be related to the altered mitochondrial morphology and function in p43-/- mice, the dramatic decrease in SIRT1 activity and Bcl-2 expression, and the subsequent increase in oxidative stress, inflammation and apoptosis (Affortit et al., 2021). There may be a direct causal relationship between decreased SIRT1 activity and earlier onset and more severe ARHL, which needs to be further verified using subsequent treatments such as SIRT1 activators, inhibitors or transgenics.
In 2021, another study exposed SD rats to environmental enrichment (EE) for 12 weeks, where the animals were placed in cages full of toys and encouraged to forage and explore by moving the toys, feeding boxes and water tanks at least once a week. After 12 weeks, they found that EE increased SIRT1 activity in the auditory cortex and improved ARHL (Song et al., 2021). A high-fat diet reduced cochlear SIRT1 and aggravated ARHL, while the addition of MNAM (N1-methylnicotinamide) elevated SIRT1 levels and inhibited ARHL (Miwa, 2021). These results show that adequate SIRT1 expression plays a key role in ARHL. The study of SIRT1 as a target for ARHL and the use of drug activation of SIRT1 to prevent or medicate ARHL are hot spots for future research.
Since the acquisition of ARHL in animal models is time-consuming, it increases the difficulty of the experiment, while the modeling time of noise-induced and drug-induced deafness is fast, and the modeling effect is stable. At present, there are many studies on cisplatin-induced hearing loss and noise-induced deafness. The protective effect of SIRT1 in cisplatin-induced hearing loss and noise-induced deafness confirms the potential therapeutic role of SIRT1 in the cochlea (Pang et al., 2018; Chen X. M. et al., 2020; Zhan et al., 2021; Liu et al., 2022). To obtain the cytotoxic or cytoprotective effect of a certain treatment on hearing in a short time, experiments in vitro are preferred. The HEI-OC1 cell line, one of the auditory cell lines, is now commonly used in hearing research (Kang et al., 2020; Zheng et al., 2020; Zhang X. S. et al., 2021). Cochlear explants or basilar membranes cultured in vitro have also been used to study the effect of drugs on hearing (He et al., 2020). However, experiments in vitro do not reflect the systemic effects of a treatment as in vivo do, and administration in vivo may not work well in the inner ear because of the blood-vagus barrier of the inner ear (Nyberg et al., 2019), leading to different results after in vivo and in vitro administration, which is a challenge that needs to be addressed. Hearing loss is not only determined by hair cells but is also closely related to spiral ganglia, spiral ligaments, vascular striae, and endolymph (Cunningham and Tucci, 2017; Korver et al., 2017). The in vitro cell assays provide information about the cellular response to the treatment and can be used to understand drug effects on hair cells, but they are not representative of drug effects on hearing.
Prospects
Age-related hearing loss is a disease associated with aging, the exact cause of which is unknown and for which there is no clear and effective treatment. Caloric restriction, currently recognized as an effective measure to delay aging, can alter NAD+/NADH and then activate SIRT1 (Madeo et al., 2019). Perhaps the prevention and treatment of ARHL needs to be carried out on many fronts, including life, diet and medication. Studies have confirmed that SIRT1 plays a highly important role in ARHL as well as aging, tumors, and neurodegenerative diseases by acting on downstream signaling molecules such as p53 and NF-κB to mitigate DNA damage, maintain the oxidative/antioxidant balance, and reduce apoptosis. Moreover, a number of drugs have been shown to activate SIRT1, which has positive anti-aging, anti-inflammatory and antioxidant effects. However, these studies are currently limited to animal or cellular experiments. A large number of clinical studies are needed to clarify whether SIRT1 plays the same positive role in the human cochlea, whether these drugs can be safely used in humans and the timing of interventions to prevent ARHL.
Author contributions
TZ wrote the manuscript and prepared all the figure and table. GT supervised the manuscript writing and editing. Both authors contributed to the article and approved the submitted version.
Funding
This work was supported by the University Characteristic Innovation Project of the Department of Education of Guangdong Province (2021KTSCX019).
Acknowledgments
We thank to Dafei Li for his valuable comments in writing this article.
Conflict of interest
The authors declare that the research was conducted in the absence of any commercial or financial relationships that could be construed as a potential conflict of interest.
Publisher’s note
All claims expressed in this article are solely those of the authors and do not necessarily represent those of their affiliated organizations, or those of the publisher, the editors and the reviewers. Any product that may be evaluated in this article, or claim that may be made by its manufacturer, is not guaranteed or endorsed by the publisher.
Abbreviations
SIRT1, silent information regulator 1; ARHL, age-related hearing loss; NAD+, nicotinamide adenine dinucleotide; Sir2, silent information regulator 2; AMPK, AMP-activated protein kinase; PGC-1α, peroxisome proliferator-activated receptor gamma coactivator 1-alpha; FOXO, forkhead box O; NF-κB,: nuclear factor kappa B; ROS, reactive oxygen species; G6PD, glucose-6-phosphate dehydrogenase.
References
Affortit, C., Casas, F., Ladrech, S., Ceccato, J. C., Bourien, J., Coyat, C., et al. (2021). Exacerbated age-related hearing loss in mice lacking the p43 mitochondrial T3 receptor. BMC Biol. 19:18. doi: 10.1186/s12915-021-00953-1
Barnes, P. J., Baker, J., and Donnelly, L. E. (2019). Cellular Senescence as a Mechanism and Target in Chronic Lung Diseases. Am. J. Respir. Crit. Care Med. 200, 556–564. doi: 10.1164/rccm.201810-1975TR
Bermúdez-Muñoz, J. M., Celaya, A. M., Hijazo-Pechero, S., Wang, J., Serrano, M., and Varela-Nieto, I. (2020). G6PD overexpression protects from oxidative stress and age-related hearing loss. Aging Cell 19:e13275. doi: 10.1111/acel.13275
Bonkowski, M. S., and Sinclair, D. A. (2016). Slowing ageing by design: The rise of NAD+ and sirtuin-activating compounds. Nat. Rev. Mol. Cell. Biol. 17, 679–690. doi: 10.1038/nrm.2016.93
Bowl, M. R., and Dawson, S. J. (2019). Age-Related Hearing Loss. Cold Spring Harb. Perspect. Med. 9:a033217. doi: 10.1101/cshperspect.a033217
Cantó, C., Gerhart-Hines, Z., Feige, J. N., Lagouge, M., Noriega, L., Milne, J. C., et al. (2009). AMPK regulates energy expenditure by modulating NAD+ metabolism and SIRT1 activity. Nature 458, 1056–1060. doi: 10.1038/nature07813
Chang, M. Y., Rhee, J., Kim, S. H., and Kim, Y. H. (2018). The Protective Effect of Egb 761 Against 3-Nitropropionic Acid-Induced Hearing Loss: The Role of Sirtuin 1. Clin. Exp. Otorhinolaryngol. 11, 9–16. doi: 10.21053/ceo.2017.00626
Chen, C., Zhou, M., Ge, Y., and Wang, X. (2020). SIRT1 and aging related signaling pathways. Mech. Ageing Dev. 187:111215. doi: 10.1016/j.mad.2020.111215
Chen, X. M., Ji, S. F., Liu, Y. H., Xue, X. M., Xu, J., Gu, Z. H., et al. (2020). Ginsenoside Rd Ameliorates Auditory Cortex Injury Associated With Military Aviation Noise-Induced Hearing Loss by Activating SIRT1/PGC-1α Signaling Pathway. Front. Physiol. 11:788. doi: 10.3389/fphys.2020.00788
Cunningham, L. L., and Tucci, D. L. (2017). Hearing Loss in Adults. New Engl. J. Med. 377:2465. doi: 10.1056/NEJMra1616601
de Gregorio, E., Colell, A., Morales, A., and Marí, M. (2020). Relevance of SIRT1-NF-κB Axis as Therapeutic Target to Ameliorate Inflammation in Liver Disease. Int. J. Mol. Sci. 21:E3858. doi: 10.3390/ijms21113858
El Hayek, L., Khalifeh, M., Zibara, V., Abi Assaad, R., Emmanuel, N., Karnib, N., et al. (2019). Lactate Mediates the Effects of Exercise on Learning and Memory through SIRT1-Dependent Activation of Hippocampal Brain-Derived Neurotrophic Factor (BDNF). J. Neurosci. 39, 2369–2382. doi: 10.1523/JNEUROSCI.1661-18.2019
Fang, E. F., Scheibye-Knudsen, M., Brace, L. E., Kassahun, H., SenGupta, T., Nilsen, H., et al. (2014). Defective Mitophagy in XPA via PARP1 Hyperactivation and NAD+/SIRT1 Reduction. Cell 157, 882–896. doi: 10.1016/j.cell.2014.03.026
Frye, R. A. (1999). Characterization of five human cDNAs with homology to the yeast SIR2 gene: Sir2-like proteins (sirtuins) metabolize NAD and may have protein ADP-ribosyltransferase activity. Biochem. Biophys. Res. Commun. 260, 273–279. doi: 10.1006/bbrc.1999.0897
Fujimoto, C., and Yamasoba, T. (2014). Oxidative stresses and mitochondrial dysfunction in age-related hearing loss. Oxid. Med. Cell. Longev. 2014:582849. doi: 10.1155/2014/582849
GBD 2019 Diseases and Injuries Collaborators (2020). Global burden of 369 diseases and injuries in 204 countries and territories, 1990-2019: A systematic analysis for the Global Burden of Disease Study 2019. Lancet 396, 1204–1222. doi: 10.1016/S0140-6736(20)30925-9
Giordano, S., Darley-Usmar, V., and Zhang, J. (2013). Autophagy as an essential cellular antioxidant pathway in neurodegenerative disease. Redox Biol. 2, 82–90. doi: 10.1016/j.redox.2013.12.013
Golub, J. S., Brickman, A. M., Ciarleglio, A. J., Schupf, N., and Luchsinger, J. A. (2020). Association of Subclinical Hearing Loss With Cognitive Performance. JAMA Otolaryngol. Head Neck Surg. 146, 57–67. doi: 10.1001/jamaoto.2019.3375
Grandemange, S., Seyer, P., Carazo, A., Bécuwe, P., Pessemesse, L., Busson, M., et al. (2005). Stimulation of mitochondrial activity by p43 overexpression induces human dermal fibroblast transformation. Cancer Res. 65, 4282–4291. doi: 10.1158/0008-5472.CAN-04-3652
Haigis, M. C., and Sinclair, D. A. (2010). Mammalian sirtuins: Biological insights and disease relevance. Annu. Rev. Pathol. 5, 253–295. doi: 10.1146/annurev.pathol.4.110807.092250
Han, C., Linser, P., Park, H. J., Kim, M. J., White, K., Vann, J. M., et al. (2016). Sirt1 deficiency protects cochlear cells and delays the early onset of age-related hearing loss in C57BL/6 mice. Neurobiol. Aging 43, 58–71. doi: 10.1016/j.neurobiolaging.2016.03.023
Hao, S., Wang, L., Zhao, K., Zhu, X., and Ye, F. (2019). Rs1894720 polymorphism in MIAT increased susceptibility to age-related hearing loss by modulating the activation of miR-29b/SIRT1/PGC-1α signaling. J. Cell Biochem. 120, 4975–4986. doi: 10.1002/jcb.27773
He, Y., Li, W., Zheng, Z., Zhao, L., Li, W., Wang, Y., et al. (2020). Inhibition of Protein arginine methyltransferase 6 reduces reactive oxygen species production and attenuates aminoglycoside- and cisplatin-induced hair cell death. Theranostics 10, 133–150. doi: 10.7150/thno.37362
Hequembourg, S., and Liberman, M. C. (2001). Spiral ligament pathology: A major aspect of age-related cochlear degeneration in C57BL/6 mice. J. Assoc. Res. Otolaryngol. 2, 118–129. doi: 10.1007/s101620010075
Hwang, J., Yao, H., Caito, S., Sundar, I. K., and Rahman, I. (2013). Redox regulation of SIRT1 in inflammation and cellular senescence. Free Radic. Biol. Med. 61, 95–110. doi: 10.1016/j.freeradbiomed.2013.03.015
Ison, J. R., Allen, P. D., and O’Neill, W. E. (2007). Age-related hearing loss in C57BL/6J mice has both frequency-specific and non-frequency-specific components that produce a hyperacusis-like exaggeration of the acoustic startle reflex. J. Assoc. Res. Otolaryngol. 8, 539–550. doi: 10.1007/s10162-007-0098-3
Jafari, Z., Kolb, B. E., and Mohajerani, M. H. (2019). Age-related hearing loss and tinnitus, dementia risk, and auditory amplification outcomes. Ageing Res. Rev. 56:100963. doi: 10.1016/j.arr.2019.100963
Johri, A., Chandra, A., and Flint Beal, M. (2013). PGC-1α, mitochondrial dysfunction, and Huntington’s disease. Free Radic. Biol. Med. 62, 37–46. doi: 10.1016/j.freeradbiomed.2013.04.016
Kaarniranta, K., Kajdanek, J., Morawiec, J., Pawlowska, E., and Blasiak, J. (2018). PGC-1α Protects RPE Cells of the Aging Retina against Oxidative Stress-Induced Degeneration through the Regulation of Senescence and Mitochondrial Quality Control. The Significance for AMD Pathogenesis. Int. J. Mol. Sci. 19:E2317. doi: 10.3390/ijms19082317
Kadono, K., Kageyama, S., Nakamura, K., Hirao, H., Ito, T., Kojima, H., et al. (2022). Myeloid Ikaros-SIRT1 signaling axis regulates hepatic inflammation and pyroptosis in ischemia-stressed mouse and human liver. J. Hepatol. 76, 896–909. doi: 10.1016/j.jhep.2021.11.026
Kaeberlein, M., McVey, M., and Guarente, L. (1999). The SIR2/3/4 complex and SIR2 alone promote longevity in Saccharomyces cerevisiae by two different mechanisms. Genes Dev. 13, 2570–2580. doi: 10.1101/gad.13.19.2570
Kang, H., Choi, S. J., Park, K. H., Lee, C. K., and Moon, J. S. (2020). Impaired Glycolysis Promotes Alcohol Exposure-Induced Apoptosis in HEI-OC1 Cells via Inhibition of EGFR Signaling. Int. J. Mol. Sci. 21:476. doi: 10.3390/ijms21020476
Korver, A. M. H., Smith, R. J. H., Van Camp, G., Schleiss, M. R., Bitner-Glindzicz, M. A. K., Lustig, L. R., et al. (2017). Congenital hearing loss. Nat. Rev. Dis. Primers 3:16094. doi: 10.1038/nrdp.2016.94
Kroemer, G., Mariño, G., and Levine, B. (2010). Autophagy and the integrated stress response. Mol. Cell 40, 280–293. doi: 10.1016/j.molcel.2010.09.023
Liu, Y. H., Jiang, Y. H., Li, C. C., Chen, X. M., Huang, L. G., Zhang, M., et al. (2022). Involvement of the SIRT1/PGC-1α Signaling Pathway in Noise-Induced Hidden Hearing Loss. Front. Physiol. 13:798395. doi: 10.3389/fphys.2022.798395
Loughrey, D. G., Kelly, M. E., Kelley, G. A., Brennan, S., and Lawlor, B. A. (2018). Association of Age-Related Hearing Loss With Cognitive Function, Cognitive Impairment, and Dementia: A Systematic Review and Meta-analysis. JAMA Otolaryngol. Head Neck Surg. 144, 115–126. doi: 10.1001/jamaoto.2017.2513
Lozupone, M., Sardone, R., and Panza, F. (2020). Age-related hearing loss and neuropathologic burden: A step inside the cognitive ear. Neurology 95, 511–512. doi: 10.1212/WNL.0000000000010580
Madeo, F., Carmona-Gutierrez, D., Hofer, S. J., and Kroemer, G. (2019). Caloric Restriction Mimetics against Age-Associated Disease: Targets, Mechanisms, and Therapeutic Potential. Cell Metab. 29, 592–610. doi: 10.1016/j.cmet.2019.01.018
Maharajan, N., and Cho, G. W. (2021). Camphorquinone Promotes the Antisenescence Effect via Activating AMPK/SIRT1 in Stem Cells and D-Galactose-Induced Aging Mice. Antioxidants 10:1916. doi: 10.3390/antiox10121916
Maiese, K. (2021). Targeting the core of neurodegeneration: FoxO, mTOR, and SIRT1. Neural Regener. Res. 16, 448–455. doi: 10.4103/1673-5374.291382
Miwa, T. (2021). Protective Effects of N1-Methylnicotinamide Against High-Fat Diet- and Age-Induced Hearing Loss via Moderate Overexpression of Sirtuin 1 Protein. Front. Cell. Neurosci. 15:634868. doi: 10.3389/fncel.2021.634868
Munk, R., Panda, A. C., Grammatikakis, I., Gorospe, M., and Abdelmohsen, K. (2017). Senescence-Associated MicroRNAs. Int. Rev. Cell. Mol. Biol. 334, 177–205. doi: 10.1016/bs.ircmb.2017.03.008
Noble, K., Brown, L., Elvis, P., and Lang, H. (2022). Cochlear Immune Response in Presbyacusis: A Focus on Dysregulation of Macrophage Activity. J. Assoc. Res. Otolaryngol. 23, 1–16. doi: 10.1007/s10162-021-00819-x
Nyberg, S., Abbott, N. J., Shi, X., Steyger, P. S., and Dabdoub, A. (2019). Delivery of therapeutics to the inner ear: The challenge of the blood-labyrinth barrier. Sci. Transl. Med. 11:eaao0935. doi: 10.1126/scitranslmed.aao0935
Pang, J., Xiong, H., Ou, Y., Yang, H., Xu, Y., Chen, S., et al. (2019). SIRT1 protects cochlear hair cell and delays age-related hearing loss via autophagy. Neurobiol. Aging 80, 127–137. doi: 10.1016/j.neurobiolaging.2019.04.003
Pang, J., Xiong, H., Yang, H., Ou, Y., Xu, Y., Huang, Q., et al. (2016). Circulating miR-34a levels correlate with age-related hearing loss in mice and humans. Exp. Gerontol. 76, 58–67. doi: 10.1016/j.exger.2016.01.009
Pang, J., Xiong, H., Zhan, T., Cheng, G., Jia, H., Ye, Y., et al. (2018). Sirtuin 1 and Autophagy Attenuate Cisplatin-Induced Hair Cell Death in the Mouse Cochlea and Zebrafish Lateral Line. Front. Cell Neurosci. 12:515. doi: 10.3389/fncel.2018.00515
Panganiban, C. H., Barth, J. L., Tan, J., Noble, K. V., McClaskey, C. M., Howard, B. A., et al. (2022). Two distinct types of nodes of Ranvier support auditory nerve function in the mouse cochlea. Glia 70, 768–791. doi: 10.1002/glia.24138
Rogina, B., and Helfand, S. L. (2004). Sir2 mediates longevity in the fly through a pathway related to calorie restriction. Proc. Natl. Acad. Sci. U. S. A. 101, 15998–16003. doi: 10.1073/pnas.0404184101
Rossmann, M. P., Dubois, S. M., Agarwal, S., and Zon, L. I. (2021). Mitochondrial function in development and disease. Dis. Model. Mech. 14:dmm048912. doi: 10.1242/dmm.048912
Salam, S. A., Mostafa, F., Alnamshan, M. M., Elshewemi, S. S., and Sorour, J. M. (2021). Thymoquinone ameliorates age-related hearing loss in C57BL/6J mice by modulating Sirt1 activity and Bak1 expression. Biomed. Pharmacother. 143:112149. doi: 10.1016/j.biopha.2021.112149
Salminen, A., and Kaarniranta, K. (2009). Regulation of the aging process by autophagy. Trends Mol. Med. 15, 217–224. doi: 10.1016/j.molmed.2009.03.004
Salminen, A., and Kaarniranta, K. (2012). AMP-activated protein kinase (AMPK) controls the aging process via an integrated signaling network. Ageing Res. Rev. 11, 230–241. doi: 10.1016/j.arr.2011.12.005
Satoh, A., Brace, C. S., Rensing, N., Cliften, P., Wozniak, D. F., Herzog, E. D., et al. (2013). Sirt1 extends life span and delays aging in mice through the regulation of Nk2 homeobox 1 in the DMH and LH. Cell Metab. 18, 416–430. doi: 10.1016/j.cmet.2013.07.013
Sauve, A. A., Wolberger, C., Schramm, V. L., and Boeke, J. D. (2006). The biochemistry of sirtuins. Annu. Rev. Biochem. 75, 435–465. doi: 10.1146/annurev.biochem.74.082803.133500
Singh, V., and Ubaid, S. (2020). Role of Silent Information Regulator 1 (SIRT1) in Regulating Oxidative Stress and Inflammation. Inflammation 43, 1589–1598. doi: 10.1007/s10753-020-01242-9
Slade, K., Plack, C. J., and Nuttall, H. E. (2020). The Effects of Age-Related Hearing Loss on the Brain and Cognitive Function. Trends Neurosci. 43, 810–821. doi: 10.1016/j.tins.2020.07.005
Smith, J. (2002). Human Sir2 and the “silencing” of p53 activity. Trends Cell Biol. 12, 404–406. doi: 10.1016/s0962-8924(02)02342-5
Song, M. K., Kim, Y. J., Kim, S. H., Yeo, S. G., and Kim, Y. J. (2021). Environmental enrichment modulates silent information regulator 1 (SIRT1) activity to attenuate central presbycusis in a rat model of normal aging. Exp. Gerontol. 155:111552. doi: 10.1016/j.exger.2021.111552
Takumida, M., Takumida, H., Katagiri, Y., and Anniko, M. (2016). Localization of sirtuins (SIRT1-7) in the aged mouse inner ear. Acta Otolaryngol. 136, 120–131. doi: 10.3109/00016489.2015.1093172
Tian, G., Sawashita, J., Kubo, H., Nishio, S., Hashimoto, S., Suzuki, N., et al. (2014). Ubiquinol-10 supplementation activates mitochondria functions to decelerate senescence in senescence-accelerated mice. Antioxid. Redox Signal. 20, 2606–2620. doi: 10.1089/ars.2013.5406
Tissenbaum, H. A., and Guarente, L. (2001). Increased dosage of a sir-2 gene extends lifespan in Caenorhabditis elegans. Nature 410, 227–230. doi: 10.1038/35065638
Tordrup, D., Smith, R., Kamenov, K., Bertram, M. Y., Green, N., and Chadha, S. (2021). Global return on investment and cost-effectiveness of WHO’s HEAR interventions for hearing loss: A modelling study. Lancet Glob. Health 10, e52–e62. doi: 10.1016/S2214-109X(21)00447-2
Wang, B., Li, J., Bao, M., Chen, R., Li, H., Lu, B., et al. (2021). Melatonin Attenuates Diabetic Myocardial Microvascular Injury through Activating the AMPK/SIRT1 Signaling Pathway. Oxid. Med. Cell. Longev. 2021:8882130. doi: 10.1155/2021/8882130
Wrutniak, C., Cassar-Malek, I., Marchal, S., Rascle, A., Heusser, S., Keller, J. M., et al. (1995). A 43-kDa protein related to c-Erb A alpha 1 is located in the mitochondrial matrix of rat liver. J. Biol. Chem. 270, 16347–16354. doi: 10.1074/jbc.270.27.16347
Xiong, H., Chen, S., Lai, L., Yang, H., Xu, Y., Pang, J., et al. (2019). Modulation of miR-34a/SIRT1 signaling protects cochlear hair cells against oxidative stress and delays age-related hearing loss through coordinated regulation of mitophagy and mitochondrial biogenesis. Neurobiol. Aging 79, 30–42. doi: 10.1016/j.neurobiolaging.2019.03.013
Xiong, H., Dai, M., Ou, Y., Pang, J., Yang, H., Huang, Q., et al. (2014). SIRT1 expression in the cochlea and auditory cortex of a mouse model of age-related hearing loss. Exp. Gerontol. 51, 8–14. doi: 10.1016/j.exger.2013.12.006
Xiong, H., Pang, J., Yang, H., Dai, M., Liu, Y., Ou, Y., et al. (2015). Activation of miR-34a/SIRT1/p53 signaling contributes to cochlear hair cell apoptosis: Implications for age-related hearing loss. Neurobiol. Aging 36, 1692–1701. doi: 10.1016/j.neurobiolaging.2014.12.034
Xu, C., Wang, L., Fozouni, P., Evjen, G., Chandra, V., Jiang, J., et al. (2020). SIRT1 is downregulated by autophagy in senescence and ageing. Nat. Cell Biol. 22, 1170–1179. doi: 10.1038/s41556-020-00579-5
Xu, W., Yan, J., Ocak, U., Lenahan, C., Shao, A., Tang, J., et al. (2021). Melanocortin 1 receptor attenuates early brain injury following subarachnoid hemorrhage by controlling mitochondrial metabolism via AMPK/SIRT1/PGC-1α pathway in rats. Theranostics 11, 522–539. doi: 10.7150/thno.49426
Xue, T., Wei, L., Zha, D. J., Qiu, J. H., Chen, F. Q., Qiao, L., et al. (2016). miR-29b overexpression induces cochlear hair cell apoptosis through the regulation of SIRT1/PGC-1α signaling: Implications for age-related hearing loss. Int. J. Mol. Med. 38, 1387–1394. doi: 10.3892/ijmm.2016.2735
Zhan, T., Xiong, H., Pang, J., Zhang, W., Ye, Y., Liang, Z., et al. (2021). Modulation of NAD+ biosynthesis activates SIRT1 and resists cisplatin-induced ototoxicity. Toxicol. Lett. 349, 115–123. doi: 10.1016/j.toxlet.2021.05.013
Zhang, X. S., Lu, Y., Li, W., Tao, T., Peng, L., Wang, W. H., et al. (2021). Astaxanthin ameliorates oxidative stress and neuronal apoptosis via SIRT1/NRF2/Prx2/ASK1/p38 after traumatic brain injury in mice. Br. J. Pharmacol. 178, 1114–1132. doi: 10.1111/bph.15346
Zhang, Y., Lv, Z., Liu, Y., Cao, H., Yang, J., and Wang, B. (2021). PIN1 Protects Hair Cells and Auditory HEI-OC1 Cells against Senescence by Inhibiting the PI3K/Akt/mTOR Pathway. Oxid. Med. Cell. Longev. 2021:9980444. doi: 10.1155/2021/9980444
Keywords: SIRT1, age-related hearing loss, ARHL, cochlea, hearing loss, age
Citation: Zhao T and Tian G (2022) Potential therapeutic role of SIRT1 in age- related hearing loss. Front. Mol. Neurosci. 15:984292. doi: 10.3389/fnmol.2022.984292
Received: 01 July 2022; Accepted: 30 August 2022;
Published: 20 September 2022.
Edited by:
Lili Cui, Guangdong Medical University, ChinaReviewed by:
Federico Kalinec, University of California, Los Angeles, United StatesJoern R. Steinert, University of Nottingham, United Kingdom
Copyright © 2022 Zhao and Tian. This is an open-access article distributed under the terms of the Creative Commons Attribution License (CC BY). The use, distribution or reproduction in other forums is permitted, provided the original author(s) and the copyright owner(s) are credited and that the original publication in this journal is cited, in accordance with accepted academic practice. No use, distribution or reproduction is permitted which does not comply with these terms.
*Correspondence: Guangyong Tian, dGlhbjE5NzBAc211LmVkdS5jbg==