- 1Brain, Behavior and the Environment Laboratory, Department of Environmental Health Sciences, Robert Stempel College of Public Health and Social Work, Florida International University, Miami, FL, United States
- 2InVitro Cell Research, LLC, New York, NY, United States
- 3Department of Biostatistics, Robert Stempel College of Public Health and Social Work, Florida International University, Miami, FL, United States
Exposure to heavy metals has been associated with psychiatric disorders and recent studies suggest an association between childhood lead (Pb2+) intoxication and schizophrenia (SZ). In animal models, Pb2+ exposure recapitulates key neuropathological and dopaminergic system alterations present in SZ. Given the high comorbidity of mental disorders such as SZ and substance abuse, coupled with evidence showing that Pb2+ exposure affects addiction circuits, we hypothesized that early life Pb2+ exposure could sensitize neuronal systems relevant to SZ and substance abuse. To this goal, we examined the effects of chronic developmental Pb2+ exposure on the acute locomotor response to cocaine (0, 5, and 15 mg kg–1) and behavioral sensitization. We also examined the role of the dopaminergic system in the psychostimulant effects of cocaine, and measured D1-dopamine receptor (D1R) levels in the rat brain using [3H]-SCH23390 quantitative receptor autoradiography, as well as the ability of the D1R antagonist SCH23390 to block the cocaine effects on locomotor activation. These studies were performed in male and female rats at different developmental ages consisting of juveniles (postnatal, PN14), early-adolescent (PN28), late adolescent (PN50), and adults (PN120). Our results show that chronic developmental Pb2+ exposure increases the acute locomotor response to the higher dose of cocaine in Pb2+-exposed male adolescent (PN28 and PN50) rats, and to the lower dose of cocaine in adolescent female rats. No changes in the locomotor activity were detected in adult rats. Behavioral sensitization experiments showed a sustained sensitization in early adolescent Pb2+-exposed male but not female rats. The cocaine-induced effects on locomotor activity were abrogated by injection of a D1R antagonist suggesting the involvement of this dopamine receptor subtype. Furthermore, Pb2+-induced increases D1R levels in several brain regions were prominent in juveniles and early adolescence but not in late adolescence or in adults. In summary, early chronic developmental Pb2+ exposure results in age and sex-dependent effect on the locomotor response to cocaine, suggesting differential susceptibilities to the neurotoxic effects of Pb2+ exposure. Our data provides further support to the notion that Pb2+ exposure is an environmental risk factor for psychiatric disorders and substance abuse.
Introduction
Lead (Pb2+) exposure continues to be a global public health problem (Tong et al., 2000; Rees and Fuller, 2020). Despite the efforts to reduce Pb2+ exposure in the United States, the levels of exposure remain significant in many major urban centers, with blood lead levels (BLL) in children under 5 years of age, above the current CDC level of 5 μg/dL (Raymond and Brown, 2017; Shah et al., 2017). In many other countries of Latin America, the Caribbean, the Middle East, Asia, and Africa there are reports of children with BLL ranging 3.3–43 μg/dL (Tuakuila et al., 2013; Olympio et al., 2017; Carpenter et al., 2019). This is best described in a recent UNICEF report of Pb2+-exposed children around the world (Rees and Fuller, 2020). This report estimates that there are approximately 123,000–269,000 infants in the United States with BLL > 10 μg/dL, and a worldwide estimate of children with BLL > 10 μg/dL in the 157–574 million range.
Children and their developing central nervous system are the most sensitive group to the neurotoxic effects of Pb2+ exposure (Delgado et al., 2018). Recent studies reported that Pb2+ exposure (BLL < 10 μg/dL) contributes to an increased risk of mental and behavioral disorders due to psychoactive substance use (Yoon and Ahn, 2016). The neurotoxic effects of childhood Pb2+ exposure also results in behavioral and cognitive disorders, and it has been recently suggested to be a risk factor for mental disorders, including schizophrenia (SZ) (Guilarte, 2004, 2009; Opler et al., 2008; Guilarte et al., 2012; Abazyan et al., 2014; Stansfield et al., 2015), a mental disease that affects 1.1% of the world’s population and approximately 3.5 million people in the United States.
Experimental animal studies have shown that Pb2+ exposure results in developmental, molecular, and cellular changes that resemble several key features of SZ pathophysiology (Stansfield et al., 2015). Thus, we used this exposure model as an approach to evaluate if Pb2+ exposure contributes to increased sensitivity to drugs of abuse. This is of special interest given the high co-morbidity of SZ and substance abuse (Volkow, 2009; Khokhar et al., 2018). Drug abuse is threefold higher in schizophrenic patients than in healthy controls (Volkow, 2009), and approximately 50% of schizophrenic patients have a substance abuse problem, principally with alcohol, marijuana, or cocaine (Khokhar et al., 2018). This high co-morbidity affects their life quality and contributes to a higher mortality rate (Khokhar et al., 2018).
In the United States, the use of psychostimulant drugs is still a significant public health concern, as it is estimated that around 1.6 million Americans have drug abuse problems (Center for Behavioral Health Statistics and Quality, 2016). Recent studies from our laboratory show that chronic developmental Pb2+ exposure increases μ-opiate receptor levels in brain regions implicated with addiction circuits (Albores-Garcia et al., 2021) which could sensitize the animals to the effects of opioids, and other drugs. However, the consequences of these changes in the animal behavior and the underlying mechanisms behind SZ and substance abuse comorbidity, as well as the potential role of Pb2+ exposure as a risk factor for both pathologies have not been completely elucidated or investigated. The present study aimed to determine if Pb2+-exposed animals have an altered sensitivity to the psychostimulant effects of cocaine and its role on the dopaminergic system.
Materials and methods
Animal husbandry and Pb2+ exposure paradigm
Adult female Long-Evans rats (225–250 g; Charles River Inc., Wilmington, MA, United States; RRID:RGD_2308852) were fed a diet containing either 0 or 1,500 parts per million (ppm) Pb2+-acetate (RMH 1000; Dyets, Bethlehem, PA, United States) for 10 days prior to breeding and throughout gestation and lactation. Litters were culled to 10 pups, weaned at twenty-one days of age (PN21) and maintained on the same diet as their respective dam to maintain a continuous Pb2+ exposure. At twenty-eight days (PN28), fifty days (PN50), or one hundred and twenty days after birth (PN120), male and female offspring were used for behavioral testing. For statistical purposes in each experiment, a single data point consisted of only one male or female pup per litter. Thus, the litter was the statistical unit. All animal studies were approved by the Florida International University Animal Care and Use Committee and have been carried out in accordance with the Guide for Care and Use of Laboratory Animals as stated by the U.S. National Institutes of Health.
Blood Pb2+ levels
Rats were euthanized using CO2 and blood was collected transcardially and placed into 1 cc vacutainer EDTA tubes. Fifty μL of the blood sample was added to the LeadCare plus tubes and blood Pb2+ levels were measured 24–72 h after, using a Meridian Bioscience LeadCare plus analyzer following manufacturer’s instructions (Meridian Bioscience, Inc., Cincinnati, OH, United States).
Drugs
Cocaine hydrochloride (Cocaine-HCl; Millipore Sigma, St. Louis, MO) was dissolved in sterile pharmaceutical-grade 0.9% saline to 5 or 15 mg mL–1 concentrations. Cocaine-HCl doses were delivered via intraperitoneal (IP) injections in a volume of 1 mL kg–1 body weight (bw) to yield doses of 5 or 15 mg kg–1. SCH23390 (Millipore Sigma, St. Louis, MO) was dissolved in sterile pharmaceutical-grade 0.9% saline solution to 0.05 and 0.1 mg mL–1 concentrations, administered via IP injections in a volume of 1 mL kg–1 bw to yield doses of 0.05 and 0.1 mg kg–1 bw. The rats were weighed the day the experimental procedures were performed.
Locomotor activity
To determine if Pb2+ exposure increases the psychostimulant effects of an acute dose of cocaine at PN28, PN50, and PN120, naïve control animals or Pb2+-exposed male and female rats were placed into one of three experimental groups for the administration of vehicle-saline, 5 or 15 mg kg–1 cocaine-HCl. Prior to injection, all rats were placed in an automated activity chamber (Digiscan Animal Activity Monitor, Omnitech Electronics, Inc., Nova Scotia, Canada) and locomotor activity was recorded for 60 min to allow for behavioral habituation. After 60 min elapsed, rats were administered a single IP injection of vehicle-saline, 5 or 15 mg kg–1 cocaine-HCl before being placed back in the activity chamber for an additional 60 min to monitor locomotor activity. Total distance traveled (cm) was measured. Data are expressed as the percentage of the total distance traveled of the control-saline group within an age group. For subsequent experiments only the dose of cocaine that showed a significant locomotor activation in both experimental groups was used.
Behavioral sensitization
Behavioral sensitization is defined as the augmented motor-stimulant response that occurs with repeated, intermittent exposure to a specific drug. Control and Pb2+ (1,500 ppm) PN28 and PN50 male or female rats were placed in the activity chamber for 60 min to record baseline activity. They were then given an i.p. injection of cocaine-HCl (15 mg kg–1), and allowed to explore an additional 60 min. This procedure took place for 5 consecutive days followed by 2 days of abstinence. On day 8 (reinstatement), animals were injected with 15 mg kg–1 of cocaine-HCl to determine behavioral sensitization. Total distance traveled was recorded and data are presented as the percentage of the total distance traveled on Day 1 for each treatment group.
D1-dopamine receptor antagonist treatment
To determine the role of D1-dopamine receptor (D1R) on the effects of cocaine-induced locomotor sensitization, control, or Pb2+-exposed male and female rats were exposed to either vehicle or the selective D1R-antagonist, SCH-23390 (0.05 or 0.1 mg kg–1, IP) 15 min prior to the cocaine-HCl injection. At PN28, PN50, and PN120, animals were placed in an activity chamber and baseline locomotor activity was recorded for 60 min. Rats were then injected with either saline or SCH23390, and 15 min later injected with 15 mg kg–1 cocaine-HCl before being placed into the activity chamber for an additional 60 min. Total distance traveled (cm) was measured. Data are expressed as the percentage of inhibition when compared to the total distance traveled by the control group after receiving saline + 15 mg kg–1 cocaine-HCl.
Tissue collection
Rat brains were harvested immediately after decapitation, snap-frozen, and then stored at −80°C until used. Fresh-frozen brains of control and Pb2+-exposed rats at PN14, PN28, PN50, and PN120 were sectioned at a 20-micron thickness in the coronal plane on a freezing cryostat (NX70, Epredia, Kalamazoo, MI) and thaw-mounted on poly-L-lysine-coated slides (Millipore Sigma, St. Louis, MO). Slides were stored at −80°C until used.
Quantitative autoradiography
For D1R autoradiography, slides were pre-incubated in 50 mM Tris buffer (pH 7.4) at room temperature for 20 min. For total binding, slides were incubated in Tris buffer with [3H]-SCH23390 (1 nM; Perkin Elmer, Akron, OH) in Tris buffer (pH 7.4) for 30 min at room temperature. Non-specific binding was determined in adjacent slides by adding 5 μM butaclamol to the buffer. Slides were rinsed twice in buffer at 4°C and then dipped once in dH2O at 4°C. The slides were then dried at room temperature overnight.
After drying overnight, slides were apposed to Kodak Biomax MR film (MR-1, Millipore Sigma, St. Louis, MO), for 6 weeks. [3H]-Microscales (Amersham, Arlington Heights, IL) were included with each film to allow for quantitative analysis of images. Images were captured and analyzed using MCID Imaging software (InterFocus Imaging, Cambridgeshire, United Kingdom; MCID Core RRID:SCR_014419). A rat brain atlas (Paxinos and Watson, 1998) was used to define regions in the Olfactory Tubercle (OT), Nucleus Accumbens (NAC), Cerebral cortex, and Striatum (STR) to be analyzed. D1R levels in OT were evaluated by analyzing binding intensity measurements for anterior OT (aOT) (at Bregma 1.60 mm) and posterior OT (pOT) (at Bregma 0.70 mm). D1R levels in NAC were determined by analyzing binding intensity measurements for the NAC core (NACc) and NAC shell (NACs) (both at Bregma 1.60 mm). D1R in STR was determined by binding intensity measurements for rostral STR (rSTR) (at Bregma 1.60 mm), medial STR (mSTR) (at Bregma −0.26 mm), and caudal STR (cSTR) (at Bregma −0.92 mm). D1R binding in the anterior cingulate cortex (Acg), agranular region frontoparietal cortex (Agran), and the frontoparietal cortex motor area (FrPaM) were determined by binding measurement at bregma 1.60 mm.
Statistical analysis
Statistical analyses were performed with SAS/STATv14.2 (SAS Institute Inc., Cary, NC; Statistical Analysis System RRID:SCR_008567) and GraphPad Prism 9 (GraphPad Software, San Diego, CA; GraphPad Prism RRID:SCR_002798). The outcome variable was examined for outliers and normality using a Kolmogorov-Smirnov test. Summary statistics including means, standard errors, frequencies, and proportions were generated for main outcome cocaine-induced locomotor activity, antagonist-dependent locomotor activity, and D1R levels and independent experimental conditions, treatment, sex, age, and brain region, respectively. Behavioral sensitization studies were analyzed using 2-way ANOVA with Repeated Measures, comparing Pb2+-treatment across days at each individual age and/or sex. Summary statistics for the D1R were further generated for all levels of independent experimental conditions. Univariate comparisons for D1R across experimental conditions consisted of t-test and ANOVA, depending on the number of categories in each condition, respectively. Sensitivity analysis was performed using the non-parametric equivalent tests, Wilcoxon Mann-Whitney U and Kruskal-Wallis test, respectively. To test all experimental conditions in one general linear model we applied linear regression starting with all four conditions. We tested two-way treatment interactions with age, sex, and brain region. Instances where interactions were significant warranted further stratified analysis of simplified models. All associations were considered significant at the alpha level of 0.05. All values are expressed as mean ± S.E.M.
Results
Animal weight and blood Pb2+ Levels
The Pb2+ exposure paradigm used in this study did not change the body weight gain of Pb2+-exposed rats at PN28, PN50, or PN120 (Albores-Garcia et al., 2021). BLL were significantly higher in both exposed groups in male and female rats, at all evaluated ages (Albores-Garcia et al., 2021).
Locomotor activity
We have previously reported that Pb2+ exposure increases the cocaine-induced locomotor activity in PN50 male rats with BLL averaging 22 ± 0.7 μg/dL at PN50 (Stansfield et al., 2015). However, the possible role of Pb2+ exposure in regulating the sensitivity to cocaine-induced locomotor activity has not been assessed in younger or older animals nor in female rats. Here we show that chronic developmental Pb2+ exposure resulted in a statistically significant increase in the locomotor activity at PN28 and PN50 regardless of sex (Table 1). Table 1 shows the effect of Pb2+ exposure on cocaine-induced locomotor activation at different ages and the corrected pairwise comparisons by age, sex, and cocaine dose.
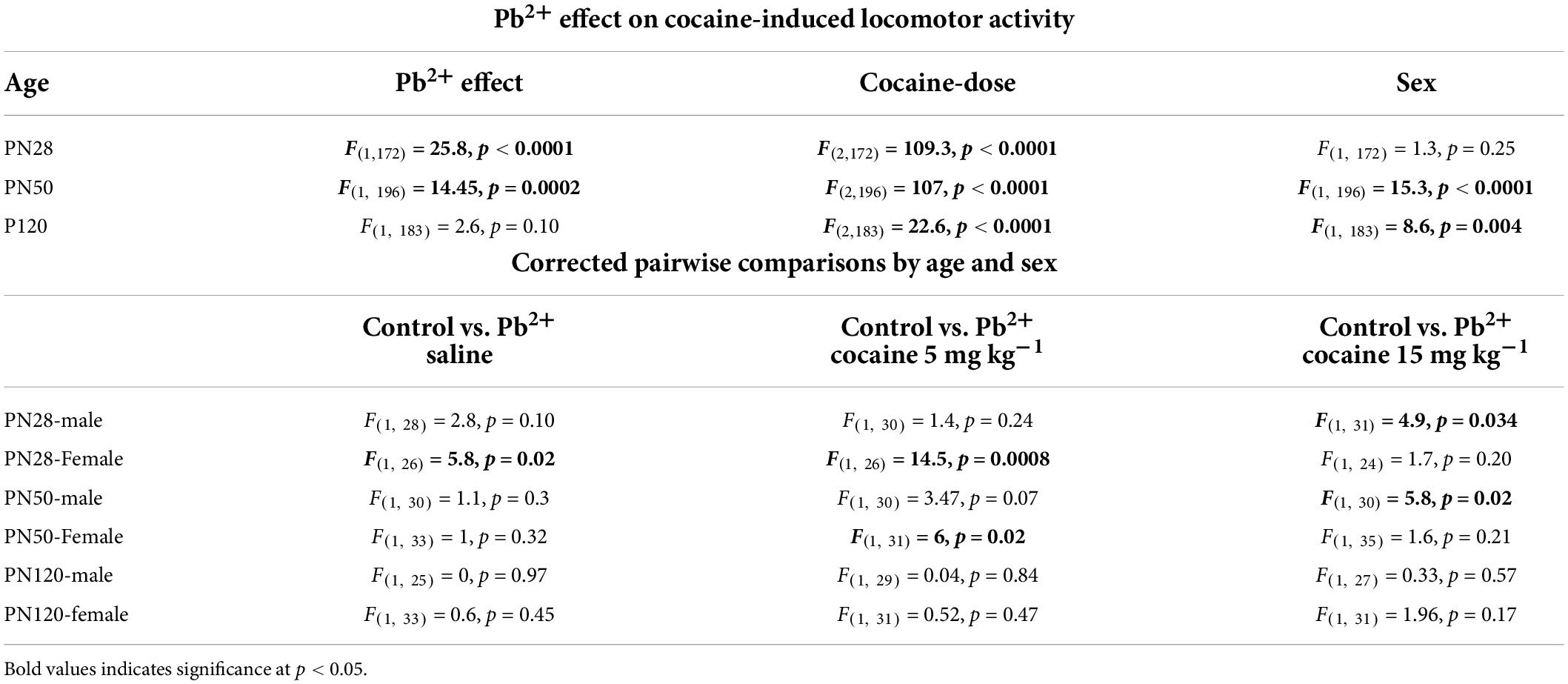
Table 1. Effect of Pb2+ exposure on the cocaine-induced locomotor activity in early adolescence (PN28), late adolescence (PN50), and adult rats (PN120).
Figure 1 shows the effects of Pb2+ exposure in the cocaine-induced locomotor activity in male and female rats at different ages. Early-adolescent (PN28) Pb2+-exposed rats had an overall higher response to cocaine-induced locomotor activity (Table 1). In early adolescent rats (PN28), we found a Pb2+ exposure effect on the locomotor activation (Table 1). In male rats, Pb2+ exposure did not affect the locomotor activity after saline or cocaine (5 mg kg–1) administration (Table 1 and Figure 1A), however a higher dose of cocaine (15 mg kg–1) induced an increase in the locomotor activity (Table 1 and Figure 1A). PN28 females showed a heightened sensitivity to Pb2+ exposure, showing an increase in locomotor activity in the absence of cocaine (a Pb2+ effect) and cocaine at 5 mg kg–1, but no changes after administering a higher dose of cocaine (15 mg kg–1) when compared to their control counterparts (Table 1 and Figure 1D).
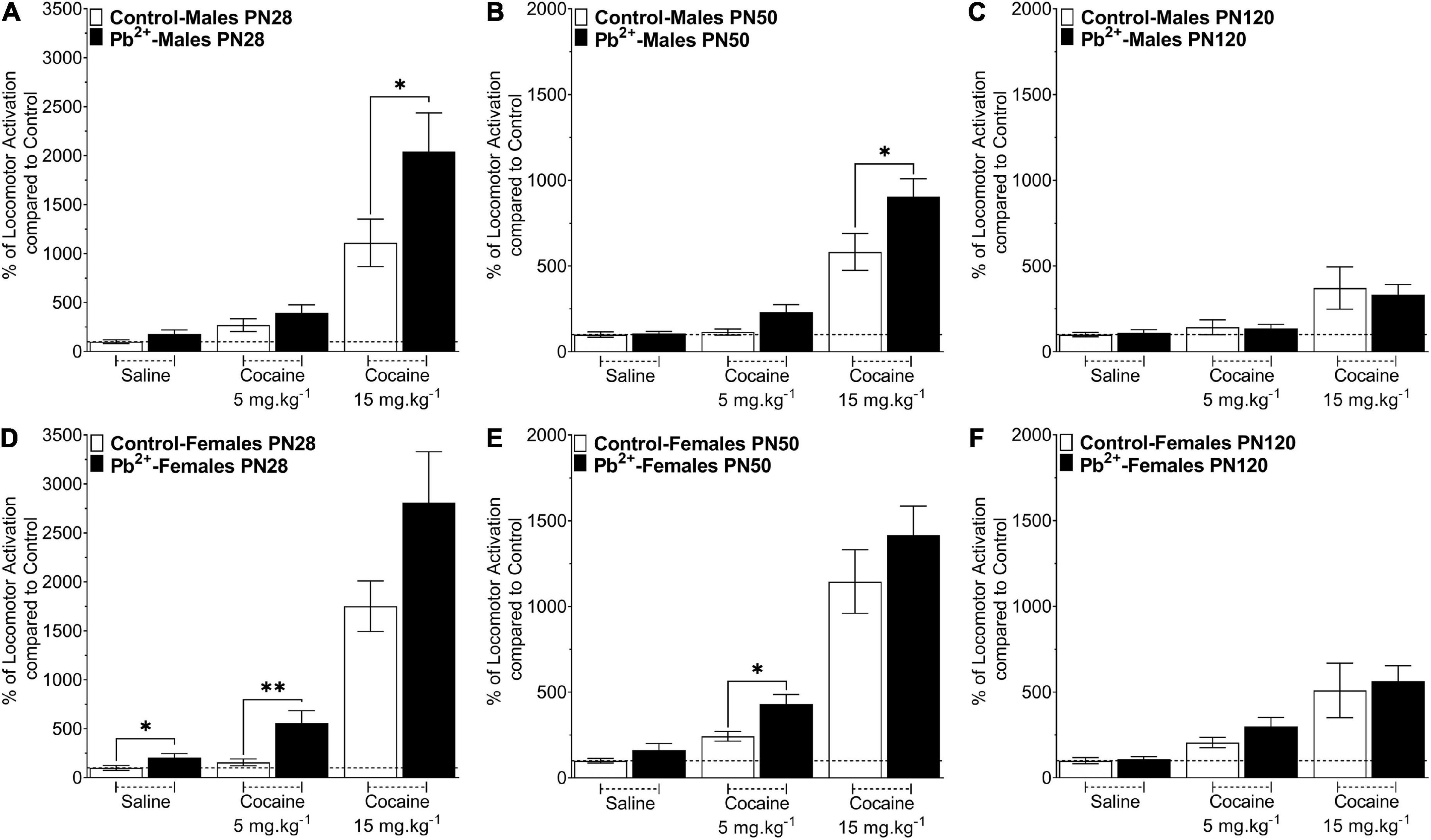
Figure 1. Effect of cocaine administration on the locomotor activity of control or Pb2+-exposed male (A–C) and female rats (D–F) at early-adolescence, postnatal day (PN) PN28 (A,D) late-adolescence PN50 (B,E) and adulthood (C,F). Data are expressed as the percentage of locomotor activation compared to control-saline of the total distance traveled (cm) ± S.E.M for 60 min, immediately after the IP injection of Cocaine-HCl (0, 5 or 15 mg kg– 1). n = 11–21 animals/experimental group. *p < 0.05 relative to saline; **p < 0.001 relative to saline.
In late adolescent rats (PN50) (Figures 1B,E), we observed cocaine-dose dependent effects in Pb2+ exposed rats compared to the control group (Table 1). More specifically, PN50 Pb2+-exposed male rats showed an increase in the cocaine-induced locomotor activity at the higher dose of cocaine (Table 1 and Figure 1B). PN50 female rats only showed an increase in the cocaine-induced locomotor activity at the lower cocaine dose (Table 1 and Figure 1E).
In adult (PN120) rats (Figures 1C,F), there was no effect of Pb2+ exposure (Table 1), as Pb2+ exposure did not cause an increase in locomotor activation in male or female rats at any of the cocaine doses administered (Table 1 and Figures 1C,F).
Behavioral sensitization
Chronic developmental Pb2+ exposure had a differential effect on male and female sensitization to cocaine. In early adolescence, Pb2+-exposed male rats showed a sustained exacerbation of the cocaine-induced locomotor activation during the sensitization phase of the protocol (days 1–5) suggesting a sustained disturbance of dopaminergic neurotransmission due to Pb2+ exposure. At reinstatement, PN28 Pb2+-exposed male rats exhibited a 300% increase in the cocaine-induced locomotor activation compared to their control counterparts (Figure 2 and Table 2). PN28 Pb2+-exposed females did not show any differences in behavioral sensitization (Figure 2 and Table 2). At PN50, male and female Pb2+-exposed rats showed an increase in the cocaine-induced locomotor activation on day 5 of the protocol, with no differences during reinstatement (Figure 2 and Table 2).
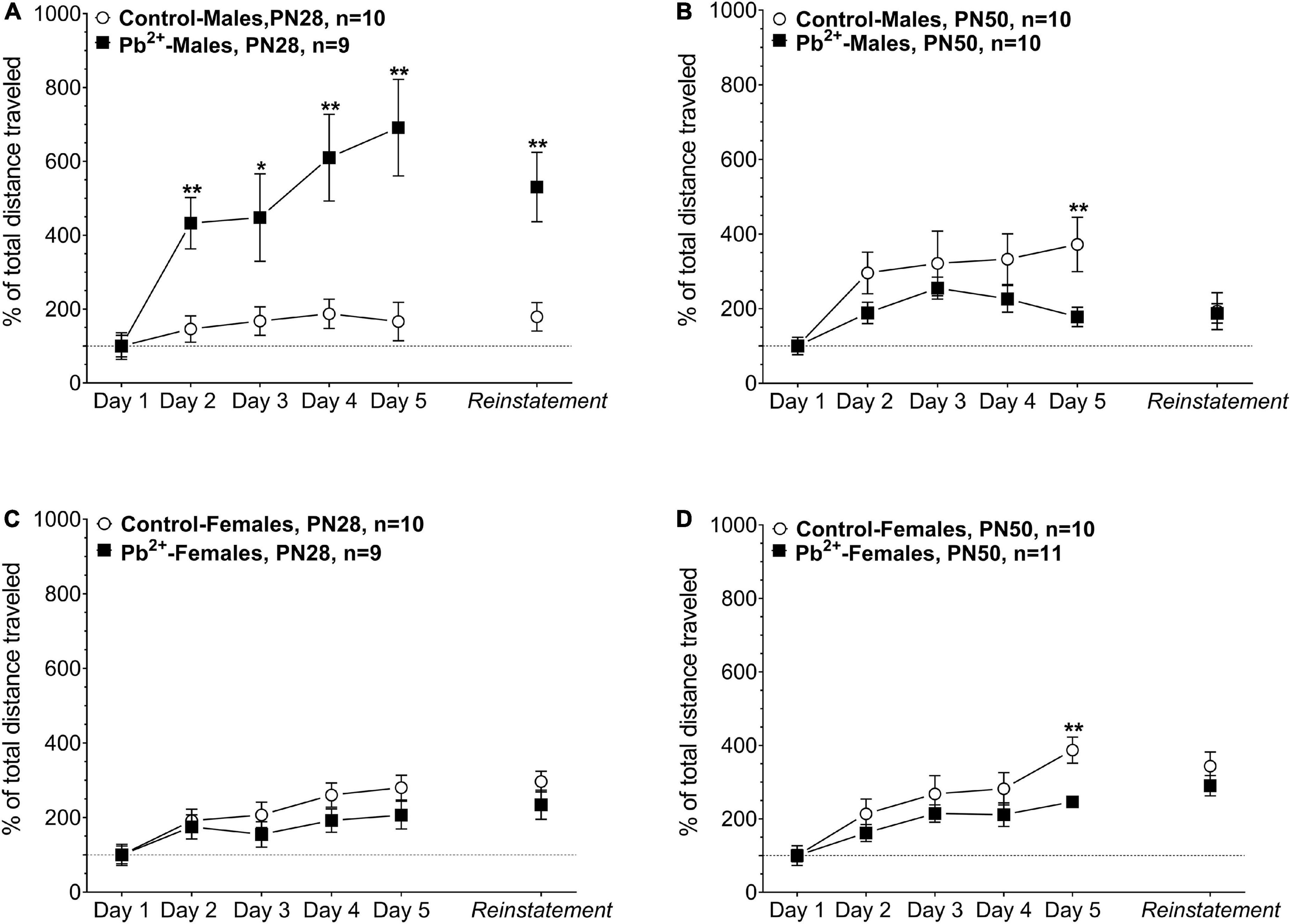
Figure 2. Effect of cocaine administration on the behavioral sensitization of control or Pb2+-exposed male (A,B) and female rats (C,D) at early-adolescence, postnatal day (PN) PN28 (A,C) late-adolescence PN50 (B,D). Data are expressed as the percentage of locomotor activation compared to control-saline of the total distance traveled (cm) ± S.E.M. for 60 min, immediately after the IP injection of Cocaine-HCl (15 mg kg– 1). n = 9–11 animals/experimental group. *p < 0.05 relative to control; **p < 0.001 relative to control.
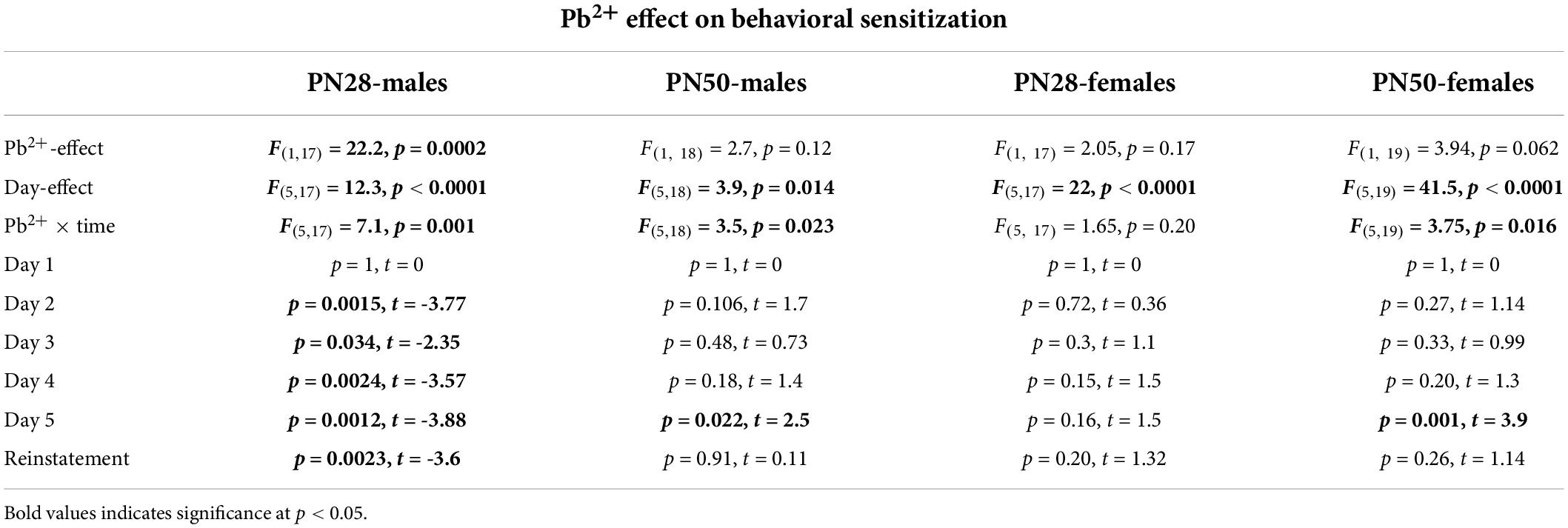
Table 2. Effect of Pb2 + exposure on the cocaine-induced behavioral sensitization in early (PN28) and late (PN50) adolescent rats.
Role of D1-dopamine receptor in cocaine-induced locomotor activity
We have previously reported that Pb2+-exposed PN50 male rats had a greater locomotor response to cocaine when compared to control animals (Stansfield et al., 2015) consistent with our present results (Figure 1). To show the role of the dopaminergic system in the cocaine-induced locomotor activation, we used different doses of the D1R antagonists SCH-23390. The data in Table 3 depicts the effect of the D1R antagonist on the percent inhibition of the cocaine-induced locomotor activation (15 mg kg–1), where saline + cocaine (15 mg kg–1) equals 0% inhibition (or 100% activation). Our data shows that the increased response in the cocaine-induced locomotor activity is completely blocked by the D1R antagonist SCH23390 in males and females at PN28 and PN120 relative to no antagonist (Table 3). At PN50, cocaine-induced locomotor activity is partially blocked by the D1R antagonist in males and completely blocked in females (Table 3).
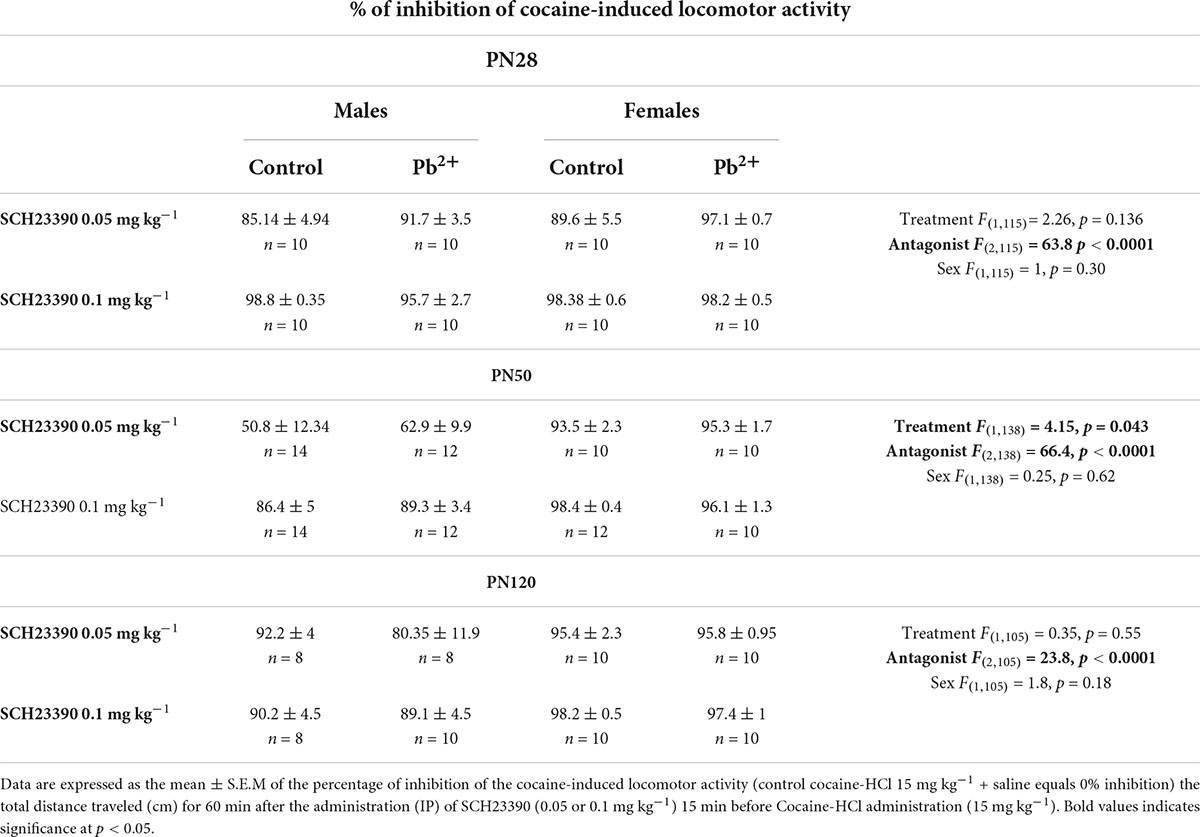
Table 3. Effect of an antagonist of the dopamine-1 receptor (SCH23390) on the cocaine-induced locomotor activity of control or Pb2+-exposed male and female rats as a function of age.
D1-dopamine receptor quantitative autoradiography
To understand the underlying neurobiology of the exacerbated locomotor response to different doses of cocaine in Pb2+-exposed rats, we examined D1R levels in several brain regions implicated in reward and addiction (Figure 3). Given the increased susceptibility to Pb2+ neurotoxic effects at younger ages, we also examined D1R levels at PN14, a highly susceptible developmental age, at which it is difficult to assess the cocaine-induced locomotor effects.
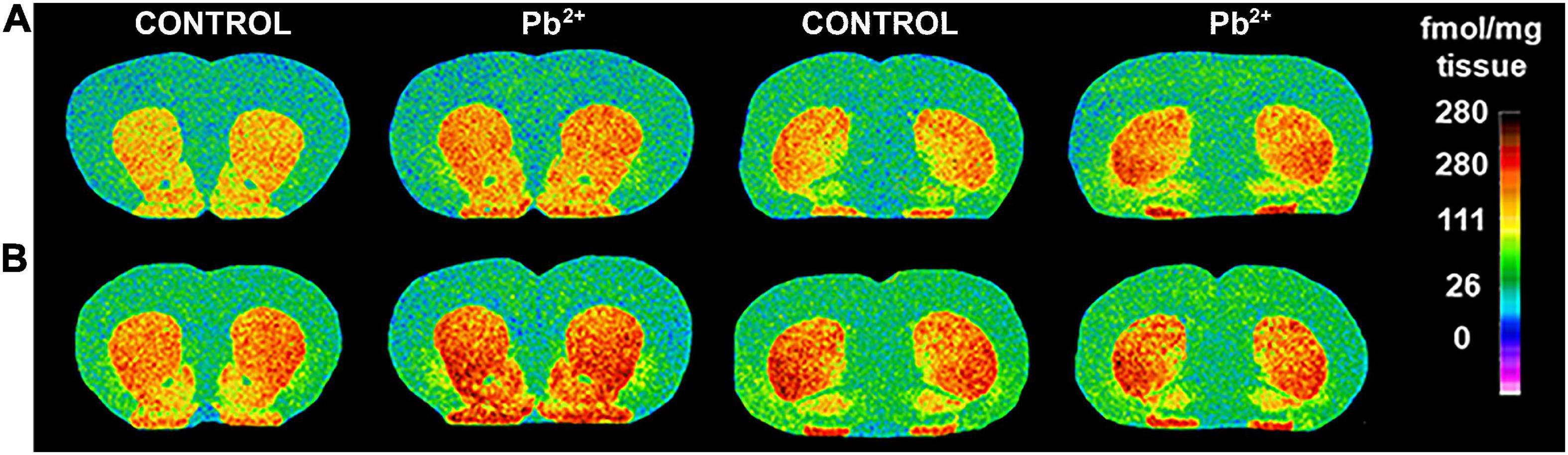
Figure 3. Representative images of [3H]-SCH23390 autoradiography binding to D1R in different brain regions of male (A) and female (B) control and Pb2+-exposed rats at postnatal day (PN) 28. The pseudocolor bar on the right side of each image indicates the level of specific receptor binding in the regions of interest (fmol/mg tissue).
In males, Pb2+ exposure increased the levels of D1R in the NACc (Table 4 and Figure 4) at PN14. No other changes in D1R levels were detected at this age. At PN28, D1R levels of male rats were increased in the mSTR, cSTR, aOT, pOT, and Acg of Pb2+-exposed animals compared to controls (Table 4 and Figure 4). No changes were detected in the other brain regions evaluated at this age in Pb2+-exposed rats compared to controls. No changes in D1R levels in Pb2+-exposed animals vs. controls were detected in males at PN50 and PN120 (Table 4 and Figure 4).
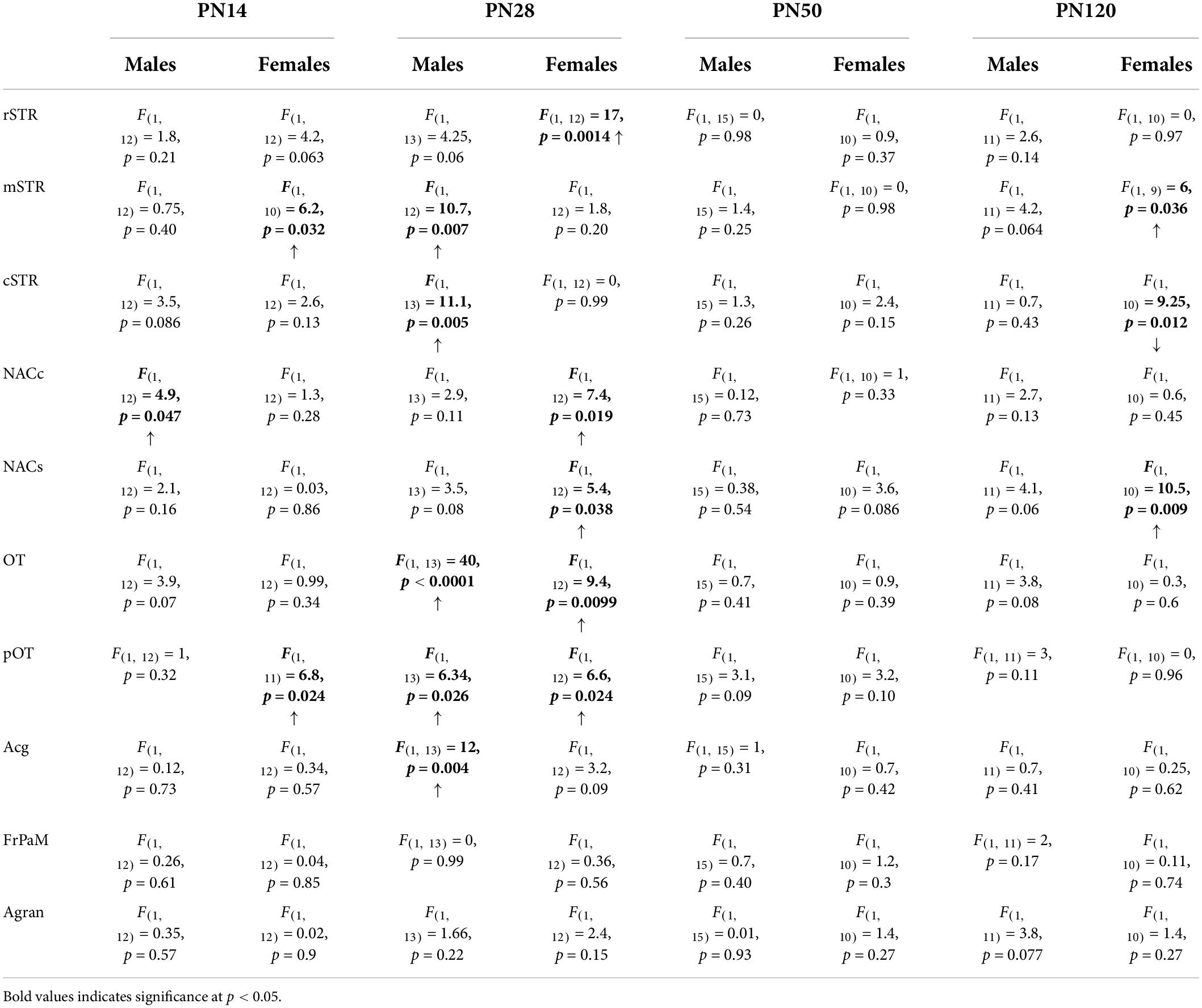
Table 4. Summary statistics of [3H]-SCH23390 autoradiography in different brain regions of male and female rats as a function of age.
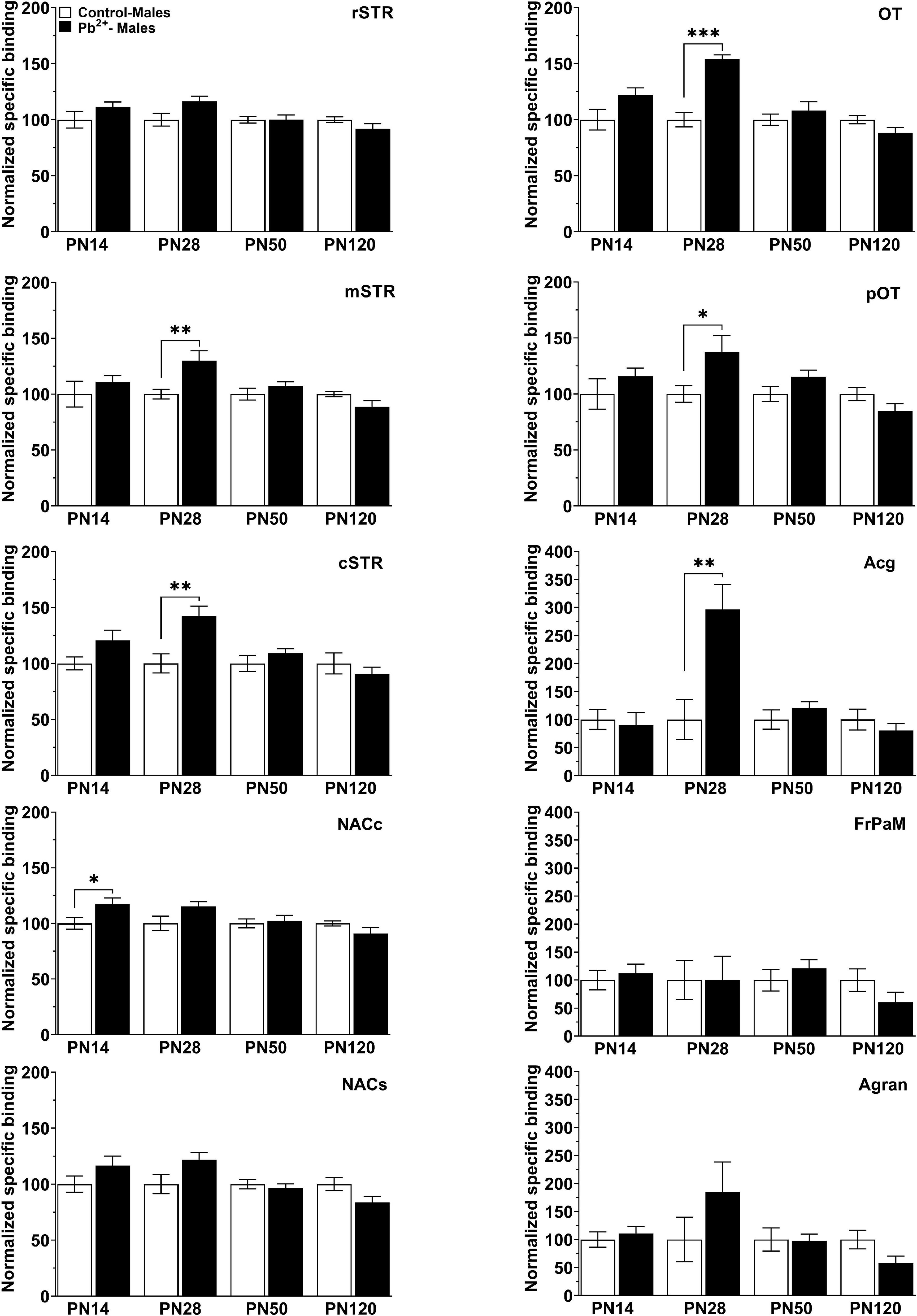
Figure 4. [3H]-SCH23390 specific binding to D1R in different brain regions in control and Pb2+-exposed male rats as a function of age. rstr, Rostral striatum; mSTR, medial striatum; cSTR, caudal striatum; NACc, nucleus accumbens core; NACs, nucleus accumbens shell; OT, olfactory tubercle; pOT, posterior olfactory tubercle; Acg, anterior cingulate cortex; Agran, agranular region frontoparietal cortex; FrPaM, frontoparietal cortex motor area. Values are mean ± S.E.M. n = 6–8 animals/experimental group. *p < 0.05 relative to control; **p < 0.01 relative to control; ***p < 0.001 relative to control.
In females, Pb2+ exposure increased the levels of D1R in the mSTR and pOT (Table 4 and Figure 3) at PN14, with no other changes detected at this age. At PN28, D1R levels of female rats were increased in rSTR, NACc, NACs, aOT, and pOT (Table 4 and Figure 5). At PN50, Pb2+ exposure did not change the D1R levels of female rats in any of the brain regions evaluated (Table 4 and Figure 5). In PN120 female rats, there was an increase in the D1R levels of the mSTR and NACs of Pb2+-exposed rats, and a decrease of the D1R levels in the cSTR of Pb2+-exposed rats compared to controls (Table 4 and Figure 5).
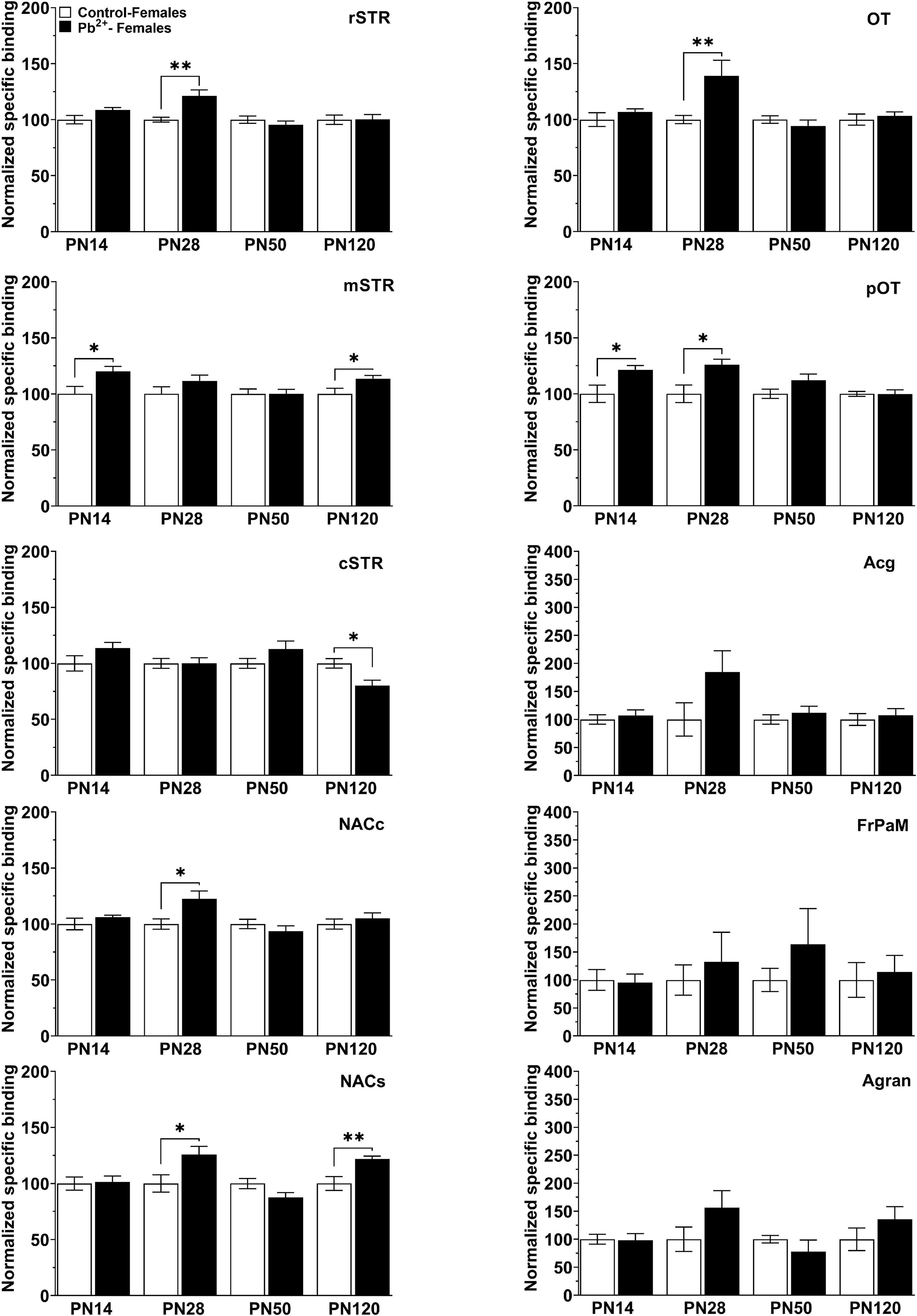
Figure 5. [3H]-SCH23390 specific binding to D1R in different brain regions in control and Pb2+-exposed female rats as a function of age. rstr, Rostral striatum; mSTR, medial striatum; cSTR, caudal striatum; NACc, nucleus accumbens core; NACs, nucleus accumbens shell; OT, olfactory tubercle; pOT, posterior olfactory tubercle; Acg, anterior cingulate cortex; Agran, agranular region frontoparietal cortex; FrPaM, frontoparietal cortex motor area. Statistical differences compared to their correspondent control group are indicated. Values are mean ± S.E.M. n = 6–8 animals/experimental group. *p < 0.05 relative to control; **p < 0.01 relative to control.
Discussion
The main finding of this study was that chronic developmental Pb2+ exposure exacerbates the acute psychostimulant effects of cocaine in adolescent male and female rats, but not in adult rats. The increased susceptibility to Pb2+ at earlier developmental stages is also supported at the receptor level, where we found that Pb2+ exposure significantly increased D1R levels in the STR, NAC core and shell, and the OT of male and female juvenile and early adolescent rats (PN14, PN28). It is of special interest that the key roles of these brain regions are associated with goal-directed behaviors, reward, and addiction.
Alterations in dopamine homeostasis have the ability to impact processes regulated by the dopaminergic system, such as motor activity (Centonze et al., 2003). Importantly, changes in dopaminergic transmission could alter dopaminergic circuits underlying reward, motivation, and executive function which could modify the motivational value of a drug and contribute to an increased risk of substance use disorder (Baler and Volkow, 2006; Volkow et al., 2011, 2012). Further studies are needed to understand how Pb2+ exposure could modify these circuits.
Dopamine regulates molecular signaling through the STR, the NAC and the OT, and the downstream effects of dopamine are dependent on the activation of D1 and D2 dopaminergic receptors subtypes. Activation of either opposing pathway regulates many central nervous system processes, which are susceptible to the neurotoxic effects of Pb2+ exposure (Cory-Slechta and Widzowski, 1991; Nation et al., 2000; Gedeon et al., 2001; Szczerbak et al., 2007). For example, previous work from our laboratory has shown that Pb2+ exposure increases dopamine turnover and dopamine-2 receptor levels in the STR of Pb2+-exposed PN50 male rats (Stansfield et al., 2015).
To the best of our knowledge, this is the first study to describe significant increases in D1R in the OT of Pb2+-exposed male and female juvenile and adolescent rats. Furthermore, our results demonstrate that D1R levels in the OT are markedly elevated by Pb2+ exposure relative to the STR and NAC. This is important and relevant because the OT has been identified as a trigger zone, acting as a synaptic junction in the mesocorticolimbic system where the circuitry underlying reward function is first activated (Ikemoto, 2010). The OT serves as a sensory integration site where the auditory, visual, gustatory, and olfactory cues that lead to a reward response first converge (Chiang and Strowbridge, 2007; Wesson and Wilson, 2010; Wesson, 2020), hence its activation by psychostimulant drugs may be a central component triggering circuitry underlying drug-related reward (Ikemoto, 2010).
Experimental evidence suggests an increased sensitivity to drugs in the OT (Ikemoto, 2003, 2007, 2010). For example, studies have shown an increased rate of self-administration in psychostimulant treated rats when injected in the OT (Ikemoto, 2003, 2010). These studies suggest that neurotransmission disturbances in the OT could play a significant role in the dopaminergic system dysregulation that underlies reward, drug use and abuse (Volkow and Li, 2005; Volkow et al., 2011, 2012; Wesson, 2020). However, the potential role of the OT as the mediator of Pb2+-induced behavioral changes needs investigation.
We found an exacerbated and sustained increase in the psychostimulant effects of cocaine in Pb2+-exposed early-adolescent male rats, reinforcing our hypothesis that Pb2+ exposure effects are more pronounced at early developmental stages and may play a role in addictive behaviors. Our results also suggest that Pb2+ exposure produces a sex-dependent susceptibility to cocaine, as Pb2+-exposed females exhibited a heightened locomotor response to a lower dose of cocaine (5 mg kg–1), when compared to Pb2+-exposed males (15 mg kg–1). These sex-dependent differences could be due to estrous cycle-dependent variations in progesterone and estrogen levels, as it has been shown that fluctuations in hormonal levels alters dopaminergic transmission (Becker and Beer, 1986; Bobzean et al., 2014).
Behavioral sensitization has a strong dopaminergic component, shown by a prevention of sensitization after the administration of the D1R antagonist SCH23390 on the reinstatement day (McDougall et al., 2017). It has been suggested that the behavioral sensitization protocol could assess the relapse rate based on the strength of withdrawal, aiming that those individuals that experienced more physical and emotional adverse effects related to drug withdrawal are more susceptible to relapse. Future studies are necessary to elucidate if the changes in D1R levels induced by Pb2+ exposure are further manifested in other stages of addiction and to assess if other neurotransmitter systems are affected, as well as to evaluate the consequences of Pb2+ exposure on acquisition, maintenance, escalation, withdrawal, and relapse. Our work shows that Pb2+ exposure increases the locomotor response to different doses of cocaine, however, it does not explore the possible role of Pb2+ exposure on self-administration and drug relapse, aspects of drug addiction that should be further explored.
The role of dopaminergic signaling on cocaine-induced locomotor activity and the heightened response of Pb2+-exposed animals suggests a potential role of increased D1R levels in the ventral STR as a mediator of the cocaine-induced locomotor activity in Pb2+-exposed animals. There are other neurotransmitter systems involved in SZ that have been previously shown to be affected by Pb2+ exposure, and dopaminergic signaling could be modulated by these other systems. For example, opioid receptors are highly implicated in reward and therefore a key signaling system in drug abuse mechanisms (Mulder et al., 1984; Spanagel et al., 1992; Shippenberg and Rea, 1997; Karkhanis et al., 2017), as well as a potential target of Pb2+ exposure (Albores-Garcia et al., 2021), particularly in early developmental stages. Dopamine receptors can form heteromers with opioid receptors, D2 dopamine receptors, and NMDA receptors, among others (reviewed in Derouiche and Massotte, 2019), and they can be involved in substance use disorder. Our data shows that Pb2+ exposure alters D1R levels in several brain regions implicated in substance abuse and previous studies from our group show that Pb2+ exposure affects other neurotransmission systems, which could translate at the behavioral level (Guilarte and McGlothan, 2003; Neal et al., 2010, 2011; Stansfield et al., 2015; Albores-Garcia et al., 2021). Additionally, other studies have shown the role of environmental toxin exposures on drug sensitivity. For example, developmental exposure to polychlorinated biphenyls (PCBs) has been shown to increase early cocaine-drug seeking on male rats (Miller et al., 2017a) and enhances behavioral sensitization to cocaine (Miller et al., 2017b).
The SZ brain is in a state of endogenous sensitization, where a heightened behavioral response and augmented dopamine release are observed after drug administration or exposure to stress. Animal models of SZ suppose that vulnerable neuronal circuits endure gradual changes during early development leading to psychosis. Preclinical and human studies have tried to characterize sensitization mechanisms and their link with psychosis. Our data suggest an increased susceptibility to drug sensitization in Pb2+-exposed animals, particularly at younger ages. Supplementary studies need to assess the potential of Pb2+ exposure on molecular and epigenetic targets associated with dopamine signaling and neuroplasticity.
This study presents some limitations, as it can neither prove causal relationships nor draw conclusions regarding molecular mechanisms underlying the observed behavioral associations. However, our results suggest a putative role of dopaminergic disturbances in Pb2+-exposed animals that needs to be further explored in order to explain the observed behavioral outcomes. In addition, this work is part of a long-standing project with data suggesting that exposure to environmental toxins during critical stages of brain development may contribute to the etiology of mental disorders (Abazyan et al., 2014; Stansfield et al., 2015), such as SZ and an increased susceptibility to substance use disorder (Albores-Garcia et al., 2021).
Taken together, our results support the hypothesis that chronic early life Pb2+ exposure increases sensitivity to the psychostimulant effects of cocaine by inducing a hyperactive dopaminergic state reflected by increase D1R levels in the OT, NAC and STR of young male and female rats. In addition, alterations in dopaminergic neurotransmission play a fundamental role in the increased susceptibility to drug addiction onset, and the developing brain is particularly sensitive to the detrimental effects of drug-induced neurotransmission dysregulation. As a consequence, alterations in the neurocircuitry resulting from drug use in adolescence can increase the susceptibility to drug use at a later age (Caffino et al., 2021).
Conclusion
Our results suggest that early life Pb2+ exposure sensitizes adolescent rats to the psychostimulant effects of cocaine in a rat model that recapitulates SZ-like pathophysiology. These findings are relevant in the public health context because Pb2+ exposure, drug abuse, and psychiatric disorders are of major health concern in the United States. It should be noted that etiological factors in the increased prevalence of drug abuse and psychiatric disorders have overlook the role of exposure to ubiquitous environmental neurotoxicants. In summary, this study suggests Pb2+ exposure as a risk factor for psychiatric disorders and substance abuse.
Data availability statement
The raw data supporting the conclusions of this article will be made available by the authors, without undue reservation.
Ethics statement
The animal study was reviewed and approved by the Florida International University Institutional Animal Care and Use Committee.
Author contributions
TG, DA-G, KS, and JM were responsible for the conception of the study, design of experiments, and interpretation of the data. DA-G, KS, and JM acquired the data. DA-G, KS, JM, and ZB analyzed the data. TG, DA-G, and ZB wrote the manuscript. JM and KS commented on the manuscript. All authors contributed to the manuscript and approved the submitted version.
Funding
This work was funded by the National Institute of Environmental Health Sciences (NIEHS) (grant no. R01ES006189) to TG.
Acknowledgments
We would like to thank Deborah Brooks, Ana Sanchez Alfonso, and Angie Ossorio for their technical assistance.
Conflict of interest
KS was employed by InVitro Cell Research, LLC.
The remaining authors declare that the research was conducted in the absence of any commercial or financial relationships that could be construed as a potential conflict of interest.
Publisher’s note
All claims expressed in this article are solely those of the authors and do not necessarily represent those of their affiliated organizations, or those of the publisher, the editors and the reviewers. Any product that may be evaluated in this article, or claim that may be made by its manufacturer, is not guaranteed or endorsed by the publisher.
Abbreviations
Acg, anterior cingulate cortex; Agran, agranular region frontoparietal cortex; aOT, anterior olfactory tubercle; cSTR, caudal striatum; D1R, Dopamine-1 receptor; D2R, Dopamine-2 receptor; FrPaM, frontoparietal cortex motor area; OT, olfactory tubercle; Pb2+, Lead; PN, Postnatal; pOT, posterior olfactory tubercle; STR, Striatum; mSTR, medial striatum; rSTR, rostral striatum.
References
Abazyan, B., Dziedzic, J., Hua, K., Abazyan, S., Yang, C., Mori, S., et al. (2014). Chronic exposure of mutant DISC1 mice to lead produces sex-dependent abnormalities consistent with schizophrenia and related mental disorders: A gene-environment interaction study. Schizophr. Bull. 40, 575–584. doi: 10.1093/schbul/sbt071
Albores-Garcia, D., McGlothan, J. L., Bursac, Z., and Guilarte, T. R. (2021). Chronic developmental lead exposure increases mu-opiate receptor levels in the adolescent rat brain. Neurotoxicology 82, 119–129. doi: 10.1016/j.neuro.2020.11.008
Baler, R. D., and Volkow, N. D. (2006). Drug addiction: The neurobiology of disrupted self-control. Trends Mol. Med. 12, 559–566. doi: 10.1016/j.molmed.2006.10.005
Becker, J. B., and Beer, M. E. (1986). The influence of estrogen on nigrostriatal dopamine activity: Behavioral and neurochemical evidence for both pre- and postsynaptic components. Behav. Brain Res. 19, 27–33. doi: 10.1016/0166-4328(86)90044-6
Bobzean, S. A., DeNobrega, A. K., and Perrotti, L. I. (2014). Sex differences in the neurobiology of drug addiction. Exp. Neurol. 259, 64–74. doi: 10.1016/j.expneurol.2014.01.022
Caffino, L., Mottarlini, F., Zita, G., Gawliński, D., Gawlińska, K., Wydra, K., et al. (2021). The effects of cocaine exposure in adolescence: Behavioural effects and neuroplastic mechanisms in experimental models. Br. J. Pharmacol. doi: 10.1111/bph.15523 [Epub ahead of print].
Carpenter, C., Potts, B., von Oettingen, J., Bonnell, R., Sainvil, M., Lorgeat, V., et al. (2019). Elevated blood lead levels in infants and children in Haiti, 2015. Public Health Rep. 134, 47–56. doi: 10.1177/0033354918807975
Center for Behavioral Health Statistics and Quality (2016). Key substance use and mental health indicators in the United States: Results from the 2015 national survey on drug use and health (HHS Publication No. SMA16-4984, NSDUH Series H-51). Available online at: https://www.samhsa.gov/data/
Centonze, D., Grande, C., Saulle, E., Martin, A. B., Gubellini, P., Pavón, N., et al. (2003). Distinct roles of D1 and D5 dopamine receptors in motor activity and striatal synaptic plasticity. J. Neurosci. 23, 8506–8512. doi: 10.1523/JNEUROSCI.23-24-08506.2003
Chiang, E., and Strowbridge, B. W. (2007). Diversity of neural signals mediated by multiple, burst-firing mechanisms in rat olfactory tubercle neurons. J. Neurophysiol. 98, 2716–2728. doi: 10.1152/jn.00807.2007
Cory-Slechta, D. A., and Widzowski, D. V. (1991). Low level lead exposure increases sensitivity to the stimulus properties of dopamine D1 and D2 agonists. Brain Res. 553, 65–74. doi: 10.1016/0006-8993(91)90231-j
Delgado, C. F., Ullery, M. A., Jordan, M., Duclos, C., Rajagopalan, S., and Scott, K. (2018). Lead exposure and developmental disabilities in preschool-aged children. J. Public Health Manage. Pract. 24, e10–e17. doi: 10.1097/PHH.0000000000000556
Derouiche, L., and Massotte, D. (2019). G protein-coupled receptor heteromers are key players in substance use disorder. Neurosci. Biobehav. Rev. 106, 73–90. doi: 10.1016/j.neubiorev.2018.09.026
Gedeon, Y., Ramesh, G. T., Wellman, P. J., and Jadhav, A. L. (2001). Changes in mesocorticolimbic dopamine and D1/D2 receptor levels after low level lead exposure: A time course study. Toxicol. Lett. 123, 217–226. doi: 10.1016/s0378-4274(01)00408-8
Guilarte, T. R. (2004). Prenatal lead exposure and schizophrenia: A plausible neurobiologic connection. Environ. Health Perspect. 112, A724. doi: 10.1289/ehp.112-a724a
Guilarte, T. R. (2009). Prenatal lead exposure and schizophrenia: Further evidence and more neurobiological connections. Environ. Health Perspect. 117, A190–A191. doi: 10.1289/ehp.0800484
Guilarte, T. R., and McGlothan, J. L. (2003). Selective decrease in NR1 subunit splice variant mRNA in the hippocampus of Pb2+-exposed rats: Implications for synaptic targeting and cell surface expression of NMDAR complexes. Brain Res. Mol. Brain Res. 113, 37–43. doi: 10.1016/s0169-328x(03)00083-4
Guilarte, T. R., Opler, M., and Pletnikov, M. (2012). Is lead exposure in early life an environmental risk factor for schizophrenia? Neurobiological connections and testable hypotheses. Neurotoxicology 33, 560–574. doi: 10.1016/j.neuro.2011.11.008
Ikemoto, S. (2003). Involvement of the olfactory tubercle in cocaine reward: Intracranial self-administration studies. J. Neurosci. 23, 9305–9311. doi: 10.1523/JNEUROSCI.23-28-09305.2003
Ikemoto, S. (2007). Dopamine reward circuitry: Two projection systems from the ventral midbrain to the nucleus accumbens-olfactory tubercle complex. Brain Res. Rev. 56, 27–78. doi: 10.1016/j.brainresrev.2007.05.004
Ikemoto, S. (2010). Brain reward circuitry beyond the mesolimbic dopamine system: A neurobiological theory. Neurosci. Biobehav. Rev. 35, 129–150. doi: 10.1016/j.neubiorev.2010.02.001
Karkhanis, A., Holleran, K. M., and Jones, S. R. (2017). Dynorphin/Kappa opioid receptor signaling in preclinical models of alcohol, drug, and food addiction. Int. Rev. Neurobiol. 136, 53–88. doi: 10.1016/bs.irn.2017.08.001
Khokhar, J. Y., Dwiel, L. L., Henricks, A. M., Doucette, W. T., and Green, A. I. (2018). The link between schizophrenia and substance use disorder: A unifying hypothesis. Schizophr. Res. 194, 78–85. doi: 10.1016/j.schres.2017.04.016
McDougall, S. A., Rudberg, K. N., Veliz, A., Dhargalkar, J. M., Garcia, A. S., Romero, L. C., et al. (2017). Importance of D1 and D2 receptor stimulation for the induction and expression of cocaine-induced behavioral sensitization in preweanling rats. Behav. Brain Res. 326, 226–236. doi: 10.1016/j.bbr.2017.03.001
Miller, M. M., Meyer, A. E., Sprowles, J. L., and Sable, H. J. (2017a). Cocaine self-administration in male and female rats perinatally exposed to PCBs: Evaluating drug use in an animal model of environmental contaminant exposure. Exp. Clin. Psychopharmacol. 25, 114–124. doi: 10.1037/pha0000113
Miller, M. M., Sprowles, J. L. N., Voeller, J. N., Meyer, A. E., and Sable, H. J. K. (2017b). Cocaine sensitization in adult Long-Evans rats perinatally exposed to polychlorinated biphenyls. Neurotoxicol. Teratol. 62, 34–41. doi: 10.1016/j.ntt.2017.04.005
Mulder, A. H., Wardeh, G., Hogenboom, F., and Frankhuyzen, A. L. (1984). Kappa- and delta-opioid receptor agonists differentially inhibit striatal dopamine and acetylcholine release. Nature 308, 278–280. doi: 10.1038/308278a0
Nation, J. R., Miller, D. K., and Bratton, G. R. (2000). Developmental lead exposure alters the stimulatory properties of cocaine at PND 30 and PND 90 in the rat. Neuropsychopharmacology 23, 444–454. doi: 10.1016/S0893-133X(00)00118-4
Neal, A. P., Stansfield, K. H., Worley, P. F., Thompson, R. E., and Guilarte, T. R. (2010). Lead exposure during synaptogenesis alters vesicular proteins and impairs vesicular release: Potential role of NMDA receptor-dependent BDNF signaling. Toxicol. Sci. 116, 249–263. doi: 10.1093/toxsci/kfq111
Neal, A. P., Worley, P. F., and Guilarte, T. R. (2011). Lead exposure during synaptogenesis alters NMDA receptor targeting via NMDA receptor inhibition. Neurotoxicology 32, 281–289. doi: 10.1016/j.neuro.2010.12.013
Olympio, K. P., Goncalves, C. G., Salles, F. J., Ferreira, A. P., Soares, A. S., Buzalaf, M. A., et al. (2017). What are the blood lead levels of children living in Latin America and the Caribbean? Environ. Int. 101, 46–58. doi: 10.1016/j.envint.2016.12.022
Opler, M. G., Buka, S. L., Groeger, J., McKeague, I., Wei, C., Factor-Litvak, P., et al. (2008). Prenatal exposure to lead, delta-aminolevulinic acid, and schizophrenia: Further evidence. Environ. Health Perspect. 116, 1586–1590. doi: 10.1289/ehp.10464
Paxinos, G., and Watson, C. (1998). The rat brain in stereotaxic coordinates, 4th Edn. San Diego, CA: Academic Press.
Raymond, J., and Brown, M. J. (2017). Childhood blood lead levels in children aged <5 years – United States, 2009-2014. MMWR Surveill. Summ. 66, 1–10. doi: 10.15585/mmwr.ss6603a1
Rees, N., and Fuller, R. (2020). The toxic truth: Children’s exposure to lead pollution undermines a generation of future potential, 2nd Edn, ed. United Nations Children’s Fund. Available online at: https://www.unicef.org/reports/toxic-truth-childrens-exposure-to-lead-pollution-2020 (accessed May 10, 2022).
Shah, K. K., Oleske, J. M., Gomez, H. F., Davidow, A. L., and Bogden, J. D. (2017). Blood lead concentrations of children in the United States: A comparison of states using two very large databases. J. Pediatr. 185, 218–223. doi: 10.1016/j.jpeds.2017.01.059
Shippenberg, T. S., and Rea, W. (1997). Sensitization to the behavioral effects of cocaine: Modulation by dynorphin and kappa-opioid receptor agonists. Pharmacol. Biochem. Behav. 57, 449–455. doi: 10.1016/s0091-3057(96)00450-9
Spanagel, R., Herz, A., and Shippenberg, T. S. (1992). Opposing tonically active endogenous opioid systems modulate the mesolimbic dopaminergic pathway. Proc. Natl. Acad. Sci. U.S.A. 89, 2046–2050. doi: 10.1073/pnas.89.6.2046
Stansfield, K. H., Ruby, K. N., Soares, B. D., McGlothan, J. L., Liu, X., and Guilarte, T. R. (2015). Early-life lead exposure recapitulates the selective loss of parvalbumin-positive GABAergic interneurons and subcortical dopamine system hyperactivity present in schizophrenia. Transl. Psychiatry 5:e522. doi: 10.1038/tp.2014.147
Szczerbak, G., Nowak, P., Kostrzewa, R. M., and Brus, R. (2007). Maternal lead exposure produces long-term enhancement of dopaminergic reactivity in rat offspring. Neurochem. Res. 32, 1791–1798. doi: 10.1007/s11064-007-9306-0
Tong, S., von Schirnding, Y. E., and Prapamontol, T. (2000). Environmental lead exposure: A public health problem of global dimensions. Bull. World Health Organ. 78, 1068–1077.
Tuakuila, J., Lison, D., Mbuyi, F., Haufroid, V., and Hoet, P. (2013). Elevated blood lead levels and sources of exposure in the population of Kinshasa, the capital of the Democratic Republic of Congo. J. Expo. Sci. Environ. Epidemiol. 23, 81–87. doi: 10.1038/jes.2012.49
Volkow, N., and Li, T. K. (2005). The neuroscience of addiction. Nat. Neurosci. 8, 1429–1430. doi: 10.1038/nn1105-1429
Volkow, N. D. (2009). Substance use disorders in schizophrenia–clinical implications of comorbidity. Schizophr. Bull. 35, 469–472. doi: 10.1093/schbul/sbp016
Volkow, N. D., Wang, G. J., Fowler, J. S., and Tomasi, D. (2012). Addiction circuitry in the human brain. Ann. Rev. Pharmacol. Toxicol. 52, 321–336. doi: 10.1146/annurev-pharmtox-010611-134625
Volkow, N. D., Wang, G. J., Fowler, J. S., Tomasi, D., and Telang, F. (2011). Addiction: Beyond dopamine reward circuitry. Proc. Natl. Acad. Sci. U.S.A. 108, 15037–15042. doi: 10.1073/pnas.1010654108
Wesson, D. W. (2020). The tubular striatum. J. Neurosci. 40, 7379–7386. doi: 10.1523/JNEUROSCI.1109-20.2020
Wesson, D. W., and Wilson, D. A. (2010). Smelling sounds: Olfactory-auditory sensory convergence in the olfactory tubercle. J. Neurosci. 30, 3013–3021. doi: 10.1523/JNEUROSCI.6003-09.2010
Keywords: dopaminergic system, cocaine, D1 dopamine receptor, lead, mental disorder (disease), substance abuse
Citation: Albores-Garcia D, Stansfield KH, McGlothan JL, Bursac Z and Guilarte TR (2022) Chronic early-life lead exposure sensitizes adolescent rats to cocaine: Role of the dopaminergic system. Front. Mol. Neurosci. 15:946726. doi: 10.3389/fnmol.2022.946726
Received: 17 May 2022; Accepted: 03 August 2022;
Published: 24 August 2022.
Edited by:
Jay S. Schneider, Thomas Jefferson University, United StatesReviewed by:
Mia Ericson, University of Gothenburg, SwedenAlena Mohd-Yusof, MPOWERHealth, United States
Copyright © 2022 Albores-Garcia, Stansfield, McGlothan, Bursac and Guilarte. This is an open-access article distributed under the terms of the Creative Commons Attribution License (CC BY). The use, distribution or reproduction in other forums is permitted, provided the original author(s) and the copyright owner(s) are credited and that the original publication in this journal is cited, in accordance with accepted academic practice. No use, distribution or reproduction is permitted which does not comply with these terms.
*Correspondence: Tomás R. Guilarte, dGd1aWxhcnRAZml1LmVkdQ==