- 1College of Kinesiology, Shenyang Sport University, Shenyang, China
- 2Department of Sport Rehabilitation, Shanghai University of Sport, Shanghai, China
- 3Department of Rehabilitation Medicine, Shanghai Shangti Orthopaedic Hospital, Shanghai, China
Post-stroke pain (PSP) is a common complication after stroke and affects patients' quality of life. Currently, drug therapy and non-invasive brain stimulation are common treatments for PSP. Given the poor efficacy of drug therapy and various side effects, non-invasive brain stimulation, such as repetitive transcranial magnetic stimulation (rTMS), has been accepted by many patients and attracted the attention of many researchers because of its non-invasive and painless nature. This article reviews the therapeutic effect of rTMS on PSP and discusses the possible mechanisms. In general, rTMS has a good therapeutic effect on PSP. Possible mechanisms of its analgesia include altering cortical excitability and synaptic plasticity, modulating the release of related neurotransmitters, and affecting the structural and functional connectivity of brain regions involved in pain processing and modulation. At present, studies on the mechanism of rTMS in the treatment of PSP are lacking, so we hope this review can provide a theoretical basis for future mechanism studies.
1. Introduction
Stroke is a disease with high morbidity, disability, and mortality worldwide (Lou et al., 2020). The many sequelae after stroke include motor dysfunction, pain, cognitive dysfunction, and paresthesia. Pain is one of the common sequelae after stroke and affects the quality of life. The incidence of post-stroke pain (PSP) is reported to be 10–45.8% (Yang and Chang, 2021; Zhang et al., 2021). PSP can manifest in many forms, including central PSP (CPSP), painful spasms, hemiplegia, tension headaches, and musculoskeletal pain (Delpont et al., 2018; Torres-Parada et al., 2020; Yang and Chang, 2021). CPSP is a neuropathic pain syndrome with challenging treatment due to vascular lesions of the somatosensory pathways in the brain (Boivie et al., 1989; Jang et al., 2019). The reported incidence of CPSP is 1–12% (Hansen et al., 2012). In most CPSP patients with dysesthesia, hyperalgesia, and paresthesia (Klit et al., 2011), pain can be characterized by spontaneous continuous pain (usually burning pain, squeezing, penetrating, and numbness) or spontaneous intermittent pain (Kumar et al., 2009). Pain severity is variable and involves temperature changes and emotional stress; movement aggravating pain, rest, and distraction can reduce pain (de Oliveira et al., 2012). CPSP has negative effects on mood, sleep, rehabilitation, and quality of life in stroke patients. PSP is often managed with a combination of medications, cognitive behavioral therapy, psychotherapy, and/or other non-pharmacological treatments (repetitive transcranial magnetic stimulation [rTMS] (Chen et al., 2022), electromotor core cortical stimulation [EMCS], and deep brain stimulation [DBS] (Cruccu et al., 2007; Elias et al., 2018)).
The management of CPSP remains challenging, and some evidence-based studies suggest limited pain relief even with high doses of different medications (Scuteri et al., 2020; Choi et al., 2021). Invasive and non-invasive neurostimulation can provide at least moderate chronic pain relief. However, invasive treatment entails risks, and non-invasive transcranial magnetic stimulation is the current treatment option for many patients (Hosomi et al., 2015; Yang et al., 2022).
rTMS provides a non-invasive and non-painful means of central nervous modulation for studying and treating neuropathic pain states. The physical principle of rTMS is electromagnetic induction. Current pulses pass through induction coils on the scalp to generate magnetic pulses and transmit them to the brain. Magnetic pulses delivered by the coil induce an electric field in the cortex, which activates neurons in the cerebral cortex (Ridding and Rothwell, 2007; Afuwape et al., 2021). Transcranial magnetic stimulation activates remote, interconnected parts of the brain in addition to targeted areas (Hallett et al., 2017).
Few reviews have summarized the effect and mechanism of rTMS on PSP. This work reviewed recent studies on rTMS improving PSP and analyzed the analgesic effect and potential mechanism of rTMS on PSP. This review discusses the therapeutic effects of different treatment parameters, hoping to provide help for the formulation of standard rTMS treatment of PSP. It also discusses the possible mechanism of rTMS in the treatment of PSP. We hope to provide more references for future research on the mechanism of rTMS.
2. Effect of rTMS on PSP
Pain is one of the common sequelae after stroke, and pain usually occurs on the opposite side of the central lesion, mainly in the upper extremities. Burning, hurting, tingling, freezing, crushing, shooting, or stabbing sensations are common descriptors. It is often affected by factors such as temperature, psychological stress, fatigue, and physical exercise. In clinical practice, CPSP can be difficult to distinguish from other types of PSP, such as hemiplegic shoulder pain, painful cramps, tension headaches, and other musculoskeletal pain (Klit et al., 2009). CPSP can impair quality of life, disrupt recovery, interfere with sleep, affect mood (produce depression or anxiety), and occasionally lead to suicide. The treatment of refractory CPSP usually adopts comprehensive treatment, but the treatment effect is often not ideal. Non-invasive treatment has received extensive attention in recent years in improving refractory CPSP. rTMS is a safe, non-invasive, tolerable, and effective mode of therapeutic intervention. It delivers many pulses continuously at a constant rate and is widely used clinically (Hosomi et al., 2015; Gu and Chang, 2017). More studies on the effect of rTMS on CPSP are detailed in Table 1.
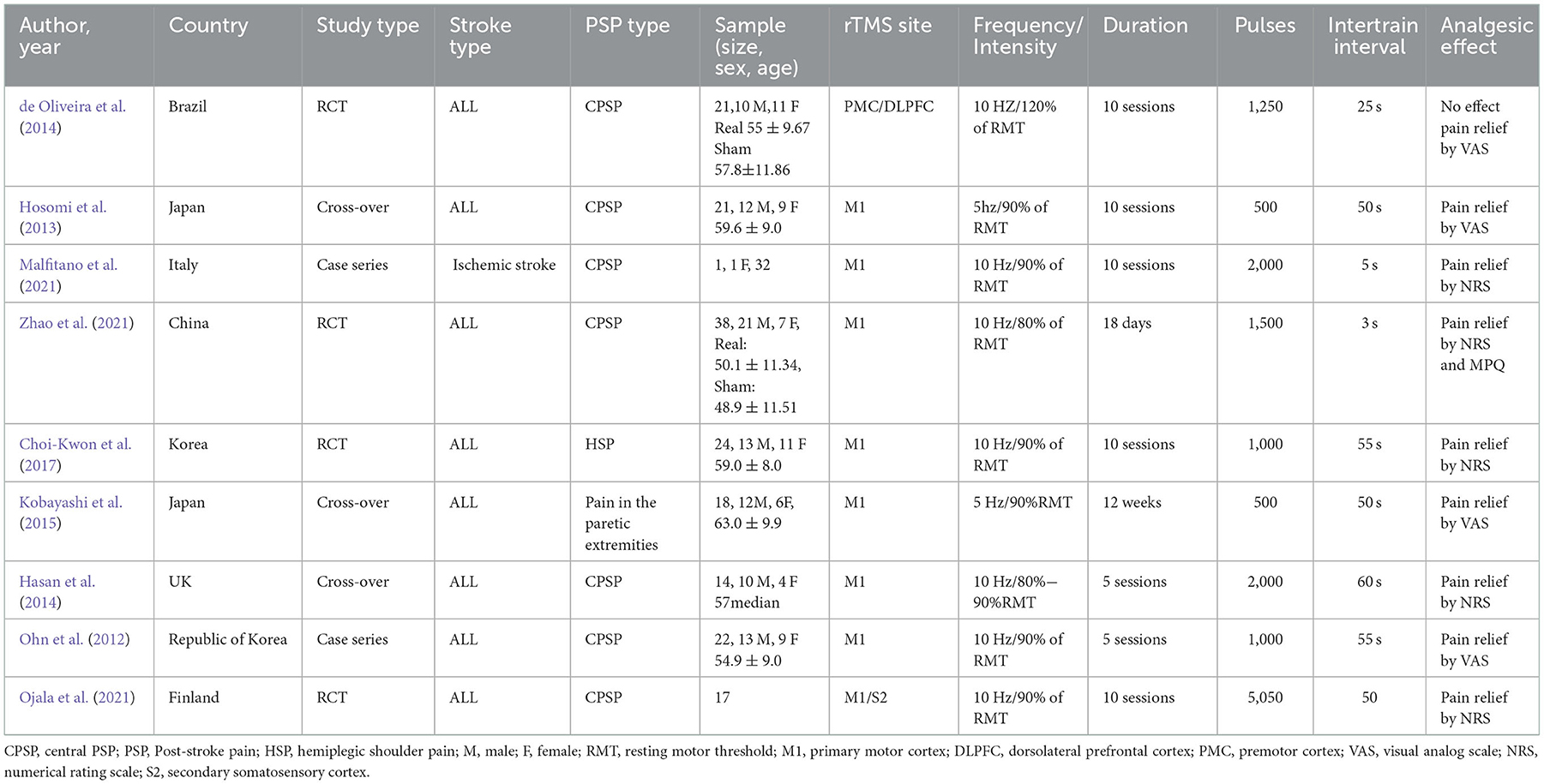
Table 1. Major findings of repetitive transcranial magnetic stimulation in post-stroke pain studies.
2.1. Analgesic effect of rTMS
2.1.1. HF-rTMS has good analgesic effect
At present, there is no unified standard for the treatment parameters of rTMS in the treatment of PSP. Different treatment parameters (stimulation frequency, stimulation site, and treatment duration) have varying analgesic effects. Traditionally, low-frequency (LF) rTMS (defined as stimulation at frequencies below 1 Hz) has been shown to reduce cortical excitability, whereas high-frequency (HF) rTMS (stimulation at frequencies over 1 Hz) has the opposite effect (Wassermann, 1998; Wagner et al., 2007; Bai et al., 2022). Multiple studies have shown that HF-rTMS provides better pain relief than LF stimulation (Cruccu et al., 2007; Borckardt et al., 2011; Pazzaglia et al., 2018). In previous studies on the analgesic effect of rTMS on PSP, HF-rTMS (5–20 Hz) was found to be effective in relieving pain in PSP. Multi-session and longer interventions can produce better analgesic effects than single sessions and short interventions (Ohn et al., 2012; Hosomi et al., 2013; Ramger et al., 2019).
2.1.2. Significant analgesic effect by targeting the M1 site
There are also significant differences in the analgesic effects of different stimulation targets. At present, the stimulation target selected in most studies is the M1 site, and the analgesic effect in this site is more intuitive and significant than that in other sites. Hirayama et al. (2006) applied 90% of RMT rTMS to the premotor cortex (M1), the primary somatosensory cortex (S1), the premotor area (preM), and supplementary motor area. Ten trains of 10 s 5 Hz TMS pulses were applied to each site, with 50's intervals between each train. Visual analog scale and the McGill Pain Questionnaire (SF-MPQ) scores were employed to determine the effect of RMT rTMS on pain, the results showed that stimulation at the M1 site has a more significant analgesic effect than that at other sites. In addition, de Oliveira et al. (2014) selected the premotor cortex/dorsolateral prefrontal cortex (PMC/DLPFC) for stimulation, each stimulation intensity was 120% of RMT, 1,250 pulses per session, stimulation interval 25 s, a total of 10 sessions, VAS score was measured after each session, and the results showed no significant pain relief effect in CPSP. Thus, this result may be related to its small sample size, but the negligible analgesic effect shows that PMC/DLPFC is not a good choice for the treatment of CPSP. However, in a recent randomized controlled trial, some scholars compared the analgesic effect of S2 and M1 on CPSP, after 10 times of stimulation at 10 HZ, each with an intensity of 5,050 pulses, the numerical rating scale (NRS) was used to measure the degree of pain of the patient, the results showed that both targeted S1 and M1 stimulation had short-term analgesic effects, but there was no difference compared to the sham group, suggesting a strong placebo effect (Ojala et al., 2021).
2.1.3. rTMS has analgesic effects on various types of pain
In addition to common neuropathic pain after stroke, HF-rTMS (10 Hz) also has a significant relieving effect on post-stroke shoulder pain, some scholars have applied rTMS with 90% of RMT and pulse 1000 to M1 for 10 consecutive sessions of treatment, the NRS score was measured on the first day, the first week, the second week, and the fourth week. The results showed that rTMS can significantly relieve HSP and maintained it for about 4 weeks, indicating that rTMS also has a certain relieving effect on peripheral pain (Choi and Chang, 2018). Studies on rTMS are mainly aimed at chronic CPSP, which may be caused by cognitive impairment in early-stage patients, leading to difficulty in diagnosis or first-line treatment of CPSP. According to expert consensus, HF-rTMS (>5 Hz) can provides moderate pain relief in chronic CPSP (Leung et al., 2020). But rTMS studies on acute or subacute CPSP are few. In a case study, 10 Hz rTMS was applied to the M1 site of a subacute CPSP patient for 2,000 pulses each time, with an intensity of 90% of RMT, for a total of 10 sessions, and the pain levels were measured before, after stimulation, and 1 month after stimulation. The results showed that the level of post-stimulation was significantly lower than the baseline level, suggesting that rTMS had the same analgesic effect on acute CPSP (Malfitano et al., 2021).
The analgesic effect of rTMS on PSP is positive correlates with frequency and treatment duration, and the M1 site as a stimulation target has a better therapeutic effect than other sites. rTMS has a good analgesic effect on pain, regardless of whether the pain is neuropathic, peripheral, acute or chronic.
2.2. rTMS has antidepressant effects
PSP is often accompanied by depression and anxiety, which aggravates the pain and hinders the recovery process. In many studies, pain relief was accompanied by depression improvement, suggesting that depression, and anxiety may influence the analgesic effect of rTMS. Ohn et al. (2012) applied 10 Hz rTMS (M1) to 22 patients with CPSP, and gave rTMS with an intensity of 90% of RMT and 1000 pulses. After 5 days of continuous treatment, the responders' VAS score and HDRS score decreased significantly. Results showed the lower the HDRS score at baseline, the more significant the analgesic effect, indicating a relationship between the improvement of depressive mood and pain relief. Galhardoni et al. (2019) applied 10 Hz rTMS to the anterior cingulate cortex (Hasan et al., 2014) and posterior superior insula (PSI), a total of 16 sessions lasted for 12 weeks, the stimulation intensity was 90 of RMT, 1,500 pulses, and the results showed that both ACC stimulation and PSI stimulation produced pain relief but no significant difference. In contrast, ACC stimulation had a more significant anxiolytic effect than PSI simulation. However, little research has been conducted in this area, and more studies are needed in the future to demonstrate the relationship between mood improvement and pain relief.
3. Mechanism of rTMS for PSP
3.1. rTMS changes cerebral cortical excitability
Although rTMS has been reported to have a significant analgesic effect on PSP, the underlying mechanism of its therapeutic effect has not been fully defined. The rTMS-promoting recovery of cortical excitability has been reported by many studies. Spontaneous pain in CPSP may be related to hyperexcitability or spontaneous firing of missing neurons in the thalamus or cortex (Vestergaard et al., 1995; Walton and Llinás, 2010), and pain relief after rTMS treatment of stroke is often accompanied by recovery of abnormal cortical excitability. This is considered one of the possible mechanisms for rTMS to treat PSP (Hosomi et al., 2013). It may involve the functional reorganization of various parts of the cerebral cortex, the recovery of abnormal inhibition between the cerebral hemispheres, and the transmission of some neurotransmitters. The mechanism of rTMS in the treatment of PSP is shown in Figure 1.
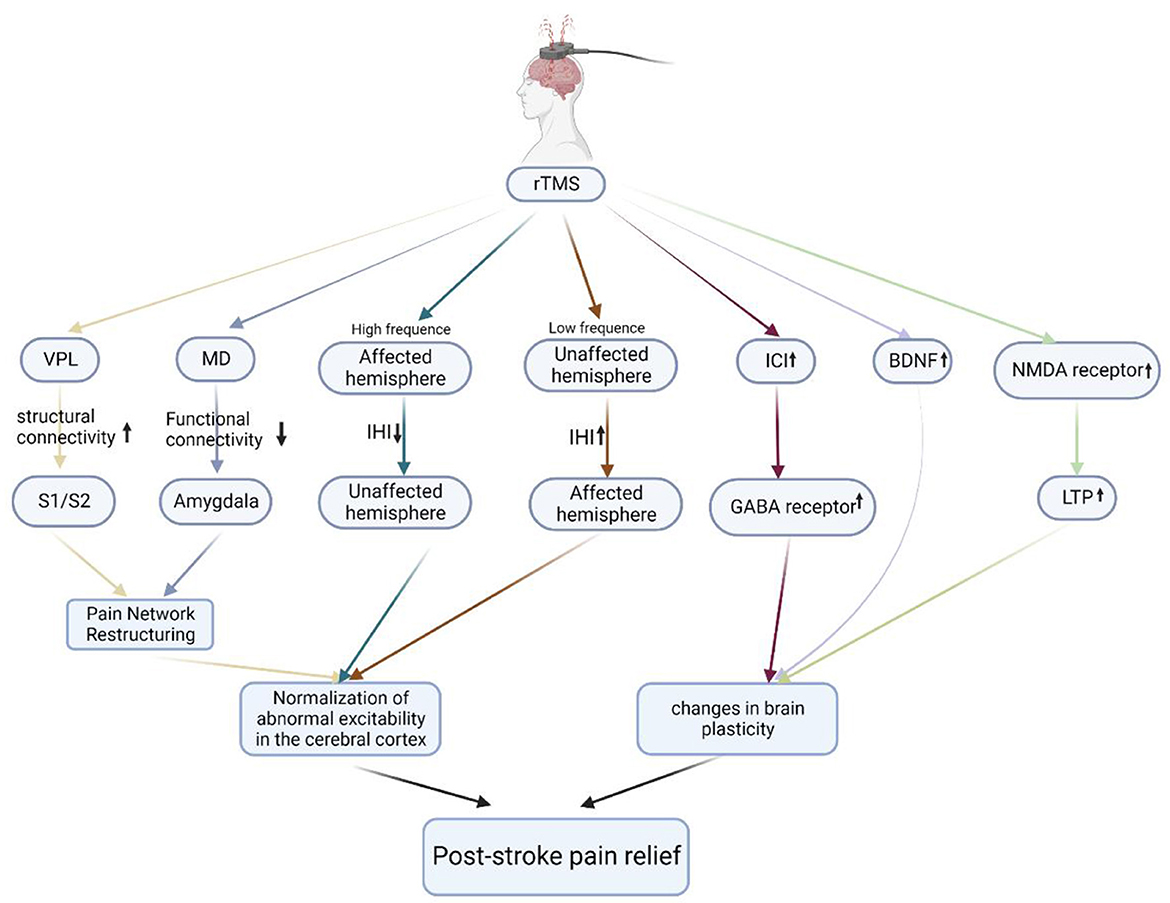
Figure 1. The mechanisms of rTMS in the treatment of PSP. Schematic illustration of the underlying mechanism of rTMS in the treatment of PSP. rTMS modulates the abnormal excitability of the cerebral cortex by modulating the pain network, improving interhemispheric inhibition, and increasing the number of GABA receptors, BDNF expression, and the number of NMDA receptors to alter brain plasticity, ultimately relieving PSP. VPL, ventral posterolateral nucleus; S1, primary somatosensory cortex; S2, secondary somatosensory cortex; MD, mediodorsal nucleus; IHI, interhemispheric inhibition; ICI, intracortical inhibition; GABA receptor, gamma-aminobutyric acid receptor; BDNF, brain-derived neurotrophic factor; NMDA receptor, N-methyl-D-aspartate receptor; LTP, long-term potentiation.
3.1.1. rTMS affects functional reorganization of the brain
CPSP typically occurs weeks or months after stroke. This late-onset feature suggests that the mechanism of CPSP development is gradual, possibly through maladaptive pain network reorganization or plastic changes (Hosomi et al., 2015). CPSP is often associated with strokes that occur around somatosensory pathways, including the ventral posterolateral nucleus (VPL) of the thalamus, anterior occipital nucleus, and lateral medulla. CPSP may also involve changes in medial emotional pathways, including the amygdala, anterior cingulate cortex, and insular cortex (Sprenger et al., 2012; Vartiainen et al., 2016). CPSP is thought to be caused by maladaptive reorganization between different regions of the brain, which neuromodulation techniques can normalize and treat (Chen et al., 2022). Evidence from animal studies suggests that CPSP reduces functional connectivity between the VPL and S1 (primary somatosensory cortex)/S2 (secondary somatosensory cortex) (responsible for perceiving pain location, intensity, and duration) and increases functional connectivity (responsible for the attentional, cognitive, and emotional aspects of pain evaluation) between the mediodorsal nucleus (Tarragó et al., 2016) and the amygdala. rTMS therapy relieves this abnormal connection (Kadono et al., 2021), so we speculate that this may be one of the mechanisms of rTMS pain relief.
3.1.2. rTMS affects interhemispheric inhibition
The mechanism of CPSP is unclear, but theories of central disinhibition have been proposed (Head and Holmes, 1911). Damage to the lateral thalamus is hypothesized to free the medial thalamus from control, inducing spontaneous or atopic pain. This is similar to the later proposed model of interhemispheric inhibition (IHI) (Duque et al., 2005), which assumes balanced inhibition between the hemispheres of a healthy brain. Although IHI theory is more commonly used to explain the recovery of dyskinesia after stroke, some scholars believe that IHI may also be involved in the CPSP mechanism (Morishita and Inoue, 2016). On the basis of IHI theory and the fact that M1 transcranial magnetic stimulation inhibits CPSP, we speculate that inhibitory signals from the contralateral hemisphere may suppress the activity of M1 in the ipsilateral hemisphere, and the mechanism of pain suppression in patients with CPSP may malfunction. The presence of post-stroke lesions results in reduced M1 excitability in the affected hemisphere, thereby reducing its neural output, including IHI to M1 in the unaffected hemisphere. This resulted in a relative increase in the excitability of M1 in the contralateral hemisphere and increased neural output, thereby increasing the IHI from M1 in the contralateral hemisphere to M1 in the affected hemisphere and inhibiting the excitability of M1 in the affected hemisphere (Gerges et al., 2022). A recent study found that rTMS induces an Increase in IHI in the affected hemisphere to the contralateral hemisphere, thereby relieving pain (Alhassani et al., 2019). Therefore, LF-rTMS of the unaffected hemisphere may reduce inhibition to the affected hemisphere. In contrast, HF-rTMS of the affected hemisphere increases inhibition to the unaffected hemisphere, normalizing the excitability of the cerebral cortex and finally achieving the effect of pain relief. Discussions of our theory focus more on movement disorders after stroke, and further research and discussion are needed on PSP.
3.1.3. RTMS affects GABAergic neuron transmission
Various experimental studies have highlighted the reduction of GABAergic neurotransmission in the central nervous system as the main reason for chronic neuropathic pain (Neto et al., 2006; Yang et al., 2019). In animal models of neuropathic pain, decreased GABAergic tone was found at the level of the dorsal spinal cord, thalamic sensory nuclei, and somatosensory cortex, which resulted in neuronal hyperactivity in the sensorimotor cortex (Guilbaud et al., 1992; Paz et al., 2010). Intracortical inhibition (ICI) is thought to reflect the function of interneurons within M1; ICI and intracortical facilitation (ICF) may reflect GABAergic inhibitory interneurons, especially GABA function (Ziemann et al., 1996; Reis et al., 2008; Lanza et al., 2020). Previous studies have shown that HF-rTMS can increase ICI and ICF, and this change is associated with PSP relief (Lefaucheur et al., 2006; Hosomi et al., 2013). HF-rTMS has been observed to enhance GABAergic synaptic connections (Lefaucheur et al., 2006); therefore, rTMS can relieve PSP through this mechanism of enhancing GABAergic neuron transmission.
3.2. rTMS induces changes in brain plasticity
Chronic pain states are known to be associated with neuroplastic changes, and the development of neuropathic pain states may involve changes in supraspinal canal function associated with pain perception (Lorenz and Casey, 2005; Maihöfner et al., 2006; Thompson and Neugebauer, 2019). The neurotrophic factor BDNF is closely related to neuronal plasticity, which is important for neuropathic pain recovery. Increased expression of BDNF has been observed in subacute and chronic stroke patients with neuropathic pain, suggesting that BDNF is involved in pain recovery after stroke (Siotto et al., 2017). The effect of rTMS on brain plasticity is mainly through two forms of long-term potentiation (LTP) and long-term depression (LTD) (Hoogendam et al., 2010). LTP can durably enhance synaptic strength from days to months, whereas LTD causes a secular decrease in synaptic strength (Duffau, 2006). The induction of LTP and LTD may be related to NMDA receptors, NMDA receptors contain ion channels that are blocked by resting magnesium ions, but membrane depolarization unblocks this channel, allowing calcium ions to enter the postsynaptic neuron and finally induce LTP (Cooke and Bliss, 2006). NMDA receptor activation is also involved in LTD, but in a different way. Rapid increases in postsynaptic calcium content induce LTP, whereas small and slow flows of calcium induce LTD. Previous studies have also demonstrated that rTMS can increase the number of NMDA receptors in the ventromedial thalamus, amygdala, and parietal cortex (Lisanby and Belmaker, 2000). Accordingly, we speculated that rTMS modifies the plasticity changes of the nervous system by increasing the expression of NMDA receptors. BDNF is a “classical” neurotrophic factor that has been shown to be closely related to neuronal plasticity and neuropathic pain. Many studies have shown that serum BDNF is inversely correlated with pain levels (Zhao et al., 2021). In a previous study (Dall'agnol et al., 2014), patients with CPSP experienced significant increases in serum BDNF levels and less pain after 3 weeks of rTMS treatment. In another experiment (Zhao et al., 2021), 10 HZ rTMS was applied to 40 patients with acute CPSP. After 3 weeks of treatment, the pain was significantly relieved and accompanied by an increase in serum BDNF. This result was consistent with previous findings, suggesting that the pain relief effect of rTMS may be related to an increase in BDNF.
4. Conclusion
rTMS is the preferred non-invasive, non-drug therapy for PSP, which is safer than invasive therapy and more easily accepted by patients. rTMS has good analgesic effects on both neuropathic and peripheral pain after stroke, which may be related to the recovery of cortical excitability, changes in brain plasticity, and pain-related mood and sensory improvement. Most of the current research results show that HF, M1, and multiple courses of rTMS have better therapeutic effects than LF and a single session of rTMS. However, there is currently no standard treatment plan for rTMS treatment of PSP. The mechanism by which rTMS relieves pain has yet to be determined. This review discusses the possible mechanism of rTMS to improve pain, and it is hoped to provide a theoretical basis for future research.
Author contributions
X-QW and X-AZ: draft conception, project administration, and funding acquisition. L-JP, H-QZ, X-QW, and X-AZ: writing, reviewing, and editing. All authors contributed to the article and approved the submitted version.
Funding
This work was supported by the Talent Development Fund of Shanghai Municipal (2021081); Shanghai Key Lab of Human Performance (Shanghai University of Sport) (11DZ2261100) and Innovative Talents Support Program for Universities of Liaoning Province, No. WR2019024.
Acknowledgments
Figure was created with BioRender software, Biorender.com.
Conflict of interest
The authors declare that the research was conducted in the absence of any commercial or financial relationships that could be construed as a potential conflict of interest.
Publisher's note
All claims expressed in this article are solely those of the authors and do not necessarily represent those of their affiliated organizations, or those of the publisher, the editors and the reviewers. Any product that may be evaluated in this article, or claim that may be made by its manufacturer, is not guaranteed or endorsed by the publisher.
References
Afuwape, O. F., Oya, H., Boes, A. D., and Jiles, D. C. (2021). Measurement and modeling of the effects of transcranial magnetic stimulation on the brain. IEEE Trans. Magn. 57, 8554. doi: 10.1109/TMAG.2020.3008554
Alhassani, G., Liston, M. B., and Schabrun, S. M. (2019). Interhemispheric inhibition is reduced in response to acute muscle pain: a cross-sectional study using transcranial magnetic stimulation. J. Pain 20, 1091–1099. doi: 10.1016/j.jpain.2019.03.007
Bai, Z. F., Zhang, J. Q., and Fong, K. N. K. (2022). Effects of transcranial magnetic stimulation in modulating cortical excitability in patients with stroke: a systematic review and meta-analysis. J. Neuroeng. Rehabil. 19, doi: 10.1186/s12984-022-00999-4
Boivie, J., Leijon, G., and Johansson, I. (1989). Central post-stroke pain–a study of the mechanisms through analyses of the sensory abnormalities. Pain 37, 173–185. doi: 10.1016/0304-3959(89)90128-0
Borckardt, J. J., Reeves, S. T., Beam, W., Jensen, M. P., Gracely, R. H., Katz, S., et al. (2011). A randomized, controlled investigation of motor cortex transcranial magnetic stimulation (TMS) effects on quantitative sensory measures in healthy adults: evaluation of TMS device parameters. Clin. J. Pain 27, 486–94. doi: 10.1097/AJP.0b013e31820d2733
Chen, Q., Shen, W., Sun, H., Zhang, H., Liu, C., Chen, Z., et al. (2022). The effect of coupled inhibitory-facilitatory repetitive transcranial magnetic stimulation on shaping early reorganization of the motor network after stroke. Brain Res. 1790, 147959. doi: 10.1016/j.brainres.2022.147959
Choi, G. S., and Chang, M. C. (2018). Effects of high-frequency repetitive transcranial magnetic stimulation on reducing hemiplegic shoulder pain in patients with chronic stoke: a randomized controlled trial. Int. J. Neurosci. 128, 110–116. doi: 10.1080/00207454.2017.1367682
Choi, H. R., Aktas, A., and Bottros, M. M. (2021). Pharmacotherapy to manage central post-stroke pain. CNS Drugs 35, 151–160. doi: 10.1007/s40263-021-00791-3
Choi-Kwon, S., Choi, S. H., Suh, M., Choi, S., Cho, K. H., Nah, H. W., et al. (2017). Musculoskeletal and central pain at 1 year post-stroke: associated factors and impact on quality of life. Acta Neurol. Scand. 135, 419–425. doi: 10.1111/ane.12617
Cooke S. F,. and Bliss, T. V.. (2006). Plasticity in the human central nervous system. Brain 129, 1659–73. doi: 10.1093/brain/awl082
Cruccu, G., Aziz, T. Z., Garcia-Larrea, L., Hansson, P., Jensen, T. S., Lefaucheur, J. P., et al. (2007). EFNS guidelines on neurostimulation therapy for neuropathic pain. Eur. J. Neurol. 14, 952–970. doi: 10.1111/j.1468-1331.2007.01916.x
Dall'agnol, L., Medeiros, L. F., Torres, I. L., Deitos, A., Brietzke, A., Laste, G., et al. (2014). Repetitive transcranial magnetic stimulation increases the corticospinal inhibition and the brain-derived neurotrophic factor in chronic myofascial pain syndrome: an explanatory double-blinded, randomized, sham-controlled trial. J. Pain 15, 845–855. doi: 10.1016/j.jpain.2014.05.001
de Oliveira De Andrade, R. A., Mendonça, D. C., Barros, M., Luvisoto, R., Myczkowski, T., et al. (2014). Repetitive transcranial magnetic stimulation of the left premotor/dorsolateral prefrontal cortex does not have analgesic effect on central poststroke pain. J. Pain 15, 1271–1281. doi: 10.1016/j.jpain.2014.09.009
de Oliveira, De Andrade, R. A., Machado, D. C. A. G., and Teixeira, M. J. (2012). Central poststroke pain: somatosensory abnormalities and the presence of associated myofascial pain syndrome. BMC Neurol. 12, 89. doi: 10.1186/1471-2377-12-89
Delpont, B., Blanc, C., Osseby, G. V., Hervieu-Bègue, M., Giroud, M., and Béjot, Y. (2018). Pain after stroke: a review. Rev Neurol 174, 671–674. doi: 10.1016/j.neurol.2017.11.011
Duffau, H. (2006). Brain plasticity: from pathophysiological mechanisms to therapeutic applications. J. Clin. Neurosci. 13, 885–897. doi: 10.1016/j.jocn.2005.11.045
Duque, J., Hummel, F., Celnik, P., Murase, N., and Mazzocchio, R. (2005). and Cohen, L. G. (2005). Transcallosal inhibition in chronic subcortical stroke. Neuroimage 28, 940–946. doi: 10.1016/j.neuroimage.2005.06.033
Elias, G. J. B., Namasivayam, A. A., and Lozano, A. M. (2018). Deep brain stimulation for stroke: Current uses and future directions. Brain Stimul. 11, 3–28. doi: 10.1016/j.brs.2017.10.005
Galhardoni, R., Aparecida Da Silva, V., García-Larrea, L., Dale, C., Baptista, A. F., Barbosa, L. M., et al. (2019). Insular and anterior cingulate cortex deep stimulation for central neuropathic pain: Disassembling the percept of pain. Neurology 92, e2165–e2175. doi: 10.1212/wnl.0000000000007396
Gerges, A. N. H., Hordacre, B., Pietro, F. D., Moseley, G. L., and Berryman, C. (2022). Do adults with stroke have altered interhemispheric inhibition? A systematic review with meta-analysis. J. Stroke Cerebrovasc. Dis. 31, 106494. doi: 10.1016/j.jstrokecerebrovasdis.2022.106494
Gu, S. Y., and Chang, M. C. (2017). The effects of 10-Hz repetitive transcranial magnetic stimulation on depression in chronic stroke patients. Brain Stimul. 10, 270–274. doi: 10.1016/j.brs.2016.10.010
Guilbaud, G., Benoist, J. M., Levante, A., Gautron, M., and Willer, J. C. (1992). Primary somatosensory cortex in rats with pain-related behaviours due to a peripheral mononeuropathy after moderate ligation of one sciatic nerve: neuronal responsivity to somatic stimulation. Exp. Brain Res. 92, 227–45. doi: 10.1007/BF00227967
Hallett, M., Iorio, D. I., Rossini, R., Park, P. M., Chen, J. E., Celnik, R., et al. (2017). Contribution of transcranial magnetic stimulation to assessment of brain connectivity and networks. Clin. Neurophysiol. 128, 2125–2139. doi: 10.1016/j.clinph.2017.08.007
Hansen, A. P., Marcussen, N. S., Klit, H., Andersen, G., Finnerup, N. B., and Jensen, T. S. (2012). Pain following stroke: a prospective study. Eur. J. Pain 16, 1128–1136. doi: 10.1002/j.1532-2149.2012.00123.x
Hasan, M., Whiteley, J., Bresnahan, R., Maciver, K., Sacco, P., Das, K., et al. (2014). Somatosensory change and pain relief induced by repetitive transcranial magnetic stimulation in patients with central poststroke pain. Neuromodulation, 17, 731–6. discussion 736. doi: 10.1111/ner.12198
Head, H., and Holmes, G. (1911). Sensory disturbances from cerebral lesions. Brain 34, 102–254. doi: 10.1093/brain/34.2-3.102
Hirayama, A., Saitoh, Y., Kishima, H., Shimokawa, T., Oshino, S., Hirata, M., et al. (2006). Reduction of intractable deafferentation pain by navigation-guided repetitive transcranial magnetic stimulation of the primary motor cortex. Pain 122, 22–27. doi: 10.1016/j.pain.2005.12.001
Hoogendam, J. M., Ramakers, G. M., and Di Lazzaro, V. (2010). Physiology of repetitive transcranial magnetic stimulation of the human brain. Brain Stimul. 3, 95–118. doi: 10.1016/j.brs.2009.10.005
Hosomi, K., Kishima, H., Oshino, S., Hirata, M., Tani, N., Maruo, T., et al. (2013). (2013). Cortical excitability changes after high-frequency repetitive transcranial magnetic stimulation for central poststroke pain. Pain 154, 1352–1357. doi: 10.1016/j.pain.2013.04.017
Hosomi, K., Seymour, B., and Saitoh, Y. (2015). Modulating the pain network–neurostimulation for central poststroke pain. Nat. Rev. Neurol. 11, 290–9. doi: 10.1038/nrneurol.2015.58
Jang, S. H., Seo, J. P., and Lee, S. J. (2019). Diffusion tensor tractography studies of central post-stroke pain due to the spinothalamic tract injury: a mini-review. Front. Neurol. 10, 787. doi: 10.3389/fneur.2019.00787
Kadono, Y., Koguchi, K., Okada, K. I., Hosomi, K., Hiraishi, M., Ueguchi, T., et al. (2021). Repetitive transcranial magnetic stimulation restores altered functional connectivity of central poststroke pain model monkeys. Sci. Rep. 11, 6126. doi: 10.1038/s41598-021-85409-w
Klit, H., Finnerup, N. B., Andersen, G., and Jensen, T. S. (2011). Central poststroke pain: a population-based study. Pain 152, 818–824. doi: 10.1016/j.pain.2010.12.030
Klit, H., Finnerup, N. B., and Jensen, T. S. (2009). Central post-stroke pain: clinical characteristics, pathophysiology, and management. Lancet Neurol. 8, 857–68. doi: 10.1016/S1474-4422(09)70176-0
Kobayashi, M., Fujimaki, T., Mihara, B., and Ohira, T. (2015). Repetitive transcranial magnetic stimulation once a week induces sustainable long-term relief of central poststroke pain. Neuromodulation 18, 249–54. doi: 10.1111/ner.12301
Kumar, B., Kalita, J., Kumar, G., and Misra, U. K. (2009). Central poststroke pain: a review of pathophysiology and treatment. Anesth. Analg. 108, 1645–57. doi: 10.1213/ane.0b013e31819d644c
Lanza, G., Arico, D., Lanuzza, B., Cosentino, F. I. I., Tripodi, M., Giardina, F., et al. (2020). Facilitatory/inhibitory intracortical imbalance in REM sleep behavior disorder: early electrophysiological marker of neurodegeneration? Sleep 43, 21–37. doi: 10.1093/sleep/zsz242
Lefaucheur, J. P., Drouot, X., Menard-Lefaucheur, I., Keravel, Y., and Nguyen, J. P. (2006). Motor cortex rTMS restores defective intracortical inhibition in chronic neuropathic pain. Neurology 67, 1568–1574. doi: 10.1212/01.wnl.0000242731.10074.3c
Leung, A., Shirvalkar, P., Chen, R., Kuluva, J., Vaninetti, M., Bermudes, R., et al. (2020). Transcranial magnetic stimulation for pain, headache, and comorbid depression: INS-NANS expert consensus panel review and recommendation. Neuromodulation 23, 267–290. doi: 10.1111/ner.13094
Lisanby, S. H., and Belmaker, R. H. (2000). Animal models of the mechanisms of action of repetitive transcranial magnetic stimulation (RTMS): comparisons with electroconvulsive shock (ECS). Depress. Anxiety 12, 178–87. doi: 10.1002/1520-6394(2000)12:3<178::AID-DA10>3.0.CO;2-N
Lorenz, J., and Casey, K. L. (2005). Imaging of acute versus pathological pain in humans. Eur. J. Pain 9, 163–165. doi: 10.1016/j.ejpain.2004.07.009
Lou, M., Ding, J., Hu, B., Zhang, Y. S., Li, H., Tan, Z. F., et al. (2020). Chinese Stroke Association guidelines for clinical management of cerebrovascular disorders: executive summary and 2019 update on organizational stroke management. Stroke Vasc. Neurol. 5, 260–269. doi: 10.1136/svn-2020-000355
Maihöfner, C., Handwerker, H. O., and Birklein, F. (2006). Functional imaging of allodynia in complex regional pain syndrome. Neurology 66, 711–7. doi: 10.1212/01.wnl.0000200961.49114.39
Malfitano, C., Rossetti, A., Scarano, S., Malloggi, C., and Tesio, L. (2021). Efficacy of repetitive transcranial magnetic stimulation for acute central post-stroke pain: a case study. Front. Neurol. 12, 742567. doi: 10.3389/fneur.2021.742567
Morishita, T., and Inoue, T. (2016). Brain stimulation therapy for central post-stroke pain from a perspective of interhemispheric neural network remodeling. Front. Hum. Neurosci. 10. doi: 10.3389/fnhum.2016.00166
Neto, F. L., Ferreira-Gomes, J., and Castro-Lopes, J. M. (2006). Distribution of GABA Receptors in the Thalamus and Their Involvement in Nociception. In: Enna, S. J. (ed.) Gaba.
Ohn, S. H., Chang, W. H., Park, C. H., Kim, S. T., Lee, J. I., Pascual-Leone, A., et al. (2012). Neural correlates of the antinociceptive effects of repetitive transcranial magnetic stimulation on central pain after stroke. Neurorehabil. Neural Repair 26, 344–52. doi: 10.1177/1545968311423110
Ojala, J., Vanhanen, J., Harno, H., Lioumis, P., Vaalto, S., Kaunisto, M. A., et al. (2021). A randomized, sham-controlled trial of repetitive transcranial magnetic stimulation targeting M1 and S2 in central poststroke pain: a pilot trial. Neuromodulation 25, 538–548. doi: 10.1111/ner.13496
Paz, J. T., Christian, C. A., Parada, I., Prince, D. A., and Huguenard, J. R. (2010). Focal cortical infarcts alter intrinsic excitability and synaptic excitation in the reticular thalamic nucleus. J. Neurosci. 30, 5465–79. doi: 10.1523/JNEUROSCI.5083-09.2010
Pazzaglia, C., Vollono, C., Testani, E., Coraci, D., Granata, G., Padua, L., et al. (2018). Low-frequency rTMS of the primary motor area does not modify the response of the cerebral cortex to phasic nociceptive stimuli. Front. Neurosci. 12, 878. doi: 10.3389/fnins.2018.00878
Ramger, B. C., Bader, K. A., Davies, S. P., Stewart, D. A., Ledbetter, L. S., Simon, C. B., et al. (2019). Effects of non-invasive brain stimulation on clinical pain intensity and experimental pain sensitivity among individuals with central post-stroke pain: a systematic review. J. Pain Res. 12, 3319–3329. doi: 10.2147/JPR.S216081
Reis, J., Swayne, O. B., Vandermeeren, Y., Camus, M., Dimyan, M. A., Harris-Love, M., et al. (2008). Contribution of transcranial magnetic stimulation to the understanding of cortical mechanisms involved in motor control. J. Physiol. 586, 325–51. doi: 10.1113/jphysiol.2007.144824
Ridding, M. C., and Rothwell, J. C. (2007). Is there a future for therapeutic use of transcranial magnetic stimulation? Nat. Rev. Neurosci. 8, 559–67. doi: 10.1038/nrn2169
Scuteri, D., Mantovani, E., Tamburin, S., Sandrini, G., Corasaniti, M. T., Bagetta, G., et al. (2020). Opioids in post-stroke pain: a systematic review and meta-analysis. Front. Pharmacol. 11. doi: 10.3389/fphar.2020.587050
Siotto, M., Aprile, I., Simonelli, I., Pazzaglia, C., Ventriglia, M., Santoro, M., et al. (2017). An exploratory study of BDNF and oxidative stress marker alterations in subacute and chronic stroke patients affected by neuropathic pain. J. Neural Transm. 124, 1557–1566. doi: 10.1007/s00702-017-1805-9
Sprenger, T., Seifert, C. L., Valet, M., Andreou, A. P., Foerschler, A., Zimmer, C., et al. (2012). Assessing the risk of central post-stroke pain of thalamic origin by lesion mapping. Brain 135, 2536–45 doi: 10.1093/brain/aws153
Tarragó, M., Deitos, A., Brietzke, A. P., Vercelino, R., Torres, I. L. S., Fregni, F., et al. (2016). Descending control of nociceptive processing in knee osteoarthritis is associated with intracortical disinhibition: an exploratory study. Medicine 95, e3353. doi: 10.1097/MD.0000000000003353
Thompson, J. M., and Neugebauer, V. (2019). Cortico-limbic pain mechanisms. Neurosci. Lett. 702, 15–23. doi: 10.1016/j.neulet.2018.11.037
Torres-Parada, M., Vivas, J., Balboa-Barreiro, V., and Marey-López, J. (2020). Post-stroke shoulder pain subtypes classifying criteria: towards a more specific assessment and improved physical therapeutic care. Braz. J. Phys. Ther. 24, 124–134. doi: 10.1016/j.bjpt.2019.02.010
Vartiainen, N., Perchet, C., Magnin, M., Creac'h, C., Convers, P., Nighoghossian, N., et al. (2016). Thalamic pain: anatomical and physiological indices of prediction. Brain 139, 708–22. doi: 10.1093/brain/awv389
Vestergaard, K., Nielsen, J., Andersen, G., Ingeman-Nielsen, M., Arendt-Nielsen, L., and Jensen, T. S. (1995). Sensory abnormalities in consecutive, unselected patients with central post-stroke pain. Pain 61, 177–186. doi: 10.1016/0304-3959(94)00140-A
Wagner, T., Valero-Cabre, A., and Pascual-Leone, A. (2007). Non-invasive human brain stimulation. Annu. Rev. Biomed. Eng. 9, 527–65. doi: 10.1146/annurev.bioeng.9.061206.133100
Walton, K. D., and Llinás, R. R. (2010). Frontiers in neuroscience. central pain as a thalamocortical dysrhythmia: a thalamic efference disconnection? In: Kruger, L. and Light, A. R. (eds.) Translational Pain Research: From Mouse to Man. Boca Raton (FL): CRC Press/Taylor and Francis Copyright © 2010 by Taylor and Francis Group, LLC.
Wassermann, E. M. (1998). Risk and safety of repetitive transcranial magnetic stimulation: report and suggested guidelines from the international workshop on the safety of repetitive transcranial magnetic stimulation, June 5–7, 1996. Electroencephalogr. Clin. Neurophysiol. 108, 1–16. doi: 10.1016/S0168-5597(97)00096-8
Yang, L. J., Liu, X. Y., Yao, K., Sun, Y., Jiang, F., Yan, H. T., et al. (2019). HCN channel antagonist ZD7288 ameliorates neuropathic pain and associated depression. Brain Res. 1717, 204–213. doi: 10.1016/j.brainres.2019.03.036
Yang, Q. H., Zhang, Y. H., Du, S. H., Wang, Y. C., Fang, Y., and Wang, X. Q. (2022). Non-invasive brain stimulation for central neuropathic pain. Front. Mol. Neurosci. 15. doi: 10.3389/fnmol.2022.879909
Yang, S., and Chang, M. C. (2021). Poststroke pain. Semin. Neurol. 41, 67–74. doi: 10.1055/s-0040-1722641
Zhang, Q., Chen, D., Shen, Y., Bian, M., Wang, P., and Li, J. (2021). Incidence and Prevalence of poststroke shoulder pain among different regions of the world: a systematic review and meta-analysis. Front. Neurol. 12, 724281. doi: 10.3389/fneur.2021.724281
Zhao, C. G., Sun, W., Ju, F., Jiang, S., Wang, H., Sun, X. L., et al. (2021). Analgesic effects of navigated repetitive transcranial magnetic stimulation in patients with acute central poststroke pain. Pain Ther 10, 1085–1100. doi: 10.1007/s40122-021-00261-0
Keywords: rTMS, stroke, pain, mechanisms, therapy, review
Citation: Pan L-J, Zhu H-Q, Zhang X-A and Wang X-Q (2023) The mechanism and effect of repetitive transcranial magnetic stimulation for post-stroke pain. Front. Mol. Neurosci. 15:1091402. doi: 10.3389/fnmol.2022.1091402
Received: 07 November 2022; Accepted: 05 December 2022;
Published: 06 January 2023.
Edited by:
Yan Lu, Fourth Military Medical University, ChinaReviewed by:
Zheng Yongjun, Fudan University, ChinaShuiqing Li, Peking University Third Hospital, China
Hong Xiao, Sichuan University, China
Copyright © 2023 Pan, Zhu, Zhang and Wang. This is an open-access article distributed under the terms of the Creative Commons Attribution License (CC BY). The use, distribution or reproduction in other forums is permitted, provided the original author(s) and the copyright owner(s) are credited and that the original publication in this journal is cited, in accordance with accepted academic practice. No use, distribution or reproduction is permitted which does not comply with these terms.
*Correspondence: Xin-An Zhang, zhangxa2725@163.com; Xue-Qiang Wang,
wangxueqiang@sus.edu.cn
†These authors have contributed equally to this work and share first authorship
‡These authors have contributed equally to this work