- 1The Graduate School, Tianjin Medical University, Tianjin, China
- 2Department of Anesthesiology, Tianjin Medical University General Hospital, Tianjin, China
Menthol is an important flavoring additive that triggers a cooling sensation. Under physiological condition, low to moderate concentrations of menthol activate transient receptor potential cation channel subfamily M member 8 (TRPM8) in the primary nociceptors, such as dorsal root ganglion (DRG) and trigeminal ganglion, generating a cooling sensation, whereas menthol at higher concentration could induce cold allodynia, and cold hyperalgesia mediated by TRPM8 sensitization. In addition, the paradoxical irritating properties of high concentrations of menthol is associated with its activation of transient receptor potential cation channel subfamily A member 1 (TRPA1). Under pathological situation, menthol activates TRPM8 to attenuate mechanical allodynia and thermal hyperalgesia following nerve injury or chemical stimuli. Recent reports have recapitulated the requirement of central group II/III metabotropic glutamate receptors (mGluR) with endogenous κ-opioid signaling pathways for menthol analgesia. Additionally, blockage of sodium channels and calcium influx is a determinant step after menthol exposure, suggesting the possibility of menthol for pain management. In this review, we will also discuss and summarize the advances in menthol-related drugs for pathological pain treatment in clinical trials, especially in neuropathic pain, musculoskeletal pain, cancer pain and postoperative pain, with the aim to find the promising therapeutic candidates for the resolution of pain to better manage patients with pain in clinics.
Introduction
Chronic pain affecting more than 30% of people worldwide (Cohen et al., 2021) with enormous personal and financial burdens. Concerns about the adverse effects, interactions, and potential for abuse of analgesic drugs have forced the search for alternative methods to manage pain effectively and safely. Although several therapies have been proposed, inadequate knowledge of the underlying pathophysiological mechanisms is one of the reasons for the current lack of pain relief.
Menthol have been used for pain relief since ancient times, first described by Hippocrates (Sprengell, 1708) and Galen (Siegel, 1970). Hippocrates considered mint as a cooling agent for peripheral pain. Galen expanded the use of menthol even further, so that existing topical analgesic containing menthol were named “Menthol Galen-Pharma.” Menthol is a cyclic monoterpene alcohol, which is derived primarily from aromatic plants. It is a natural compound with three asymmetric carbon atoms and, thus occurs as four pairs of optical isomers namely (+)- and (-)-isomenthol, (+)- and (-)- menthol, (+)- and (-)-neumenthol, (+)- and (-)-neoisomenthol. The principal form of menthol found in nature is (-)-menthol (L-menthol), and the main supply is obtained naturally. The Nobel Prize for chemistry was awarded to Ryoji Noyori in 2001 for the work on asymmetric catalysis for menthol chemical synthesis (Kamatou et al., 2013).
Menthol is one of the most important flavorings additives besides vanilla and citrus (Kamatou et al., 2013) and is used as a cooling and/or flavors enhancing ingredient in medicines, cosmetics and insecticides, confectionery, chewing gum, liqueurs, toothpaste, shampoos, and soaps (Patel et al., 2007; Kolassa, 2013). Menthol exhibits unique, multiple, and paradoxical sensory effects when applied externally to the skin or mucous membranes. menthol application at low doses produces a cooling sensation, whereas at higher doses, it evokes burning, irritation, and pain (Wei and Seid, 1983; Wasner et al., 2004; Namer et al., 2005; Proudfoot et al., 2006). In practice, various topical over-the-counter products containing menthol for pain relief have concentrations ranging 5−16% (320−1024 mM) (Oz et al., 2017).
Several in vitro and in vivo studies have demonstrated menthol’s biological properties, such as its analgesic, antibacterial, antifungal, anesthetic, and osmotic enhancement, as well as chemoprophylaxis and immunomodulatory effects (Kamatou et al., 2013). The multiple physiological effects of menthol appear to depend on its interaction with a variety of receptors throughout the body. It has been proposed to sensitize transient receptor potential cation channel subfamily M member 8 (TPRM8) and transient receptor potential cation channel subfamily V member 3 (TRPV3), desensitize transient receptor potential cation channel subfamily A member 1 (TRPA1) and transient receptor potential cation channel subfamily V member 1 (TRPV1), stimulate κ-opioid systems, and enhance central glutamate dependent gate control mechanisms (Green and McAuliffe, 2000; Macpherson et al., 2006; Proudfoot et al., 2006; Zanotto et al., 2008). This complex and unique effect of menthol has contributed to its potential as a promising area of pain research. This review summarizes the current pre-clinical results and clinical practice evidence on menthol in analgesic research.
Analgesic mechanism of menthol
Menthol and transient receptor potential family
Transient receptor potential (TRP) channels are a family of proteins which are present in nociceptors and allow organisms to transduce environmental signals that produce pain (Rosenbaum et al., 2022), of which, the discovery is a Nobel physiology and medicine-awarded subject in 2021. Currently, menthol is considered to be the activity regulator of TRPM8, TRPA1 and TRPV1, etc., in the TRP family, and thus menthol and its chemically modified derivatives are currently highlighted again.
Transient receptor potential cation channel subfamily M member 8
In general, menthol selectively acts on TRPM8, a non-selective calcium-permeable channel expressed in a subset of sensory neurons in the dorsal root ganglion (DRG) and trigeminal ganglion (Galeotti et al., 2002; McKemy et al., 2002), which are thought to be the main molecular sensor of cold somatosensation in human body (activation temperature is 8−28°C) (Andersen et al., 2014; Olsen et al., 2014). In addition to menthol and low temperature, TRPM8 channels can be activated by a variety of stimuli (Izquierdo et al., 2021), including the menthol analog icilin (Colburn et al., 2007; Izquierdo et al., 2021), changes in voltage (Fernández et al., 2011; Raddatz et al., 2014) and extracellular osmotic pressure (Parra et al., 2014; Quallo et al., 2015).
Transient receptor potential cation channel subfamily M member 8 participates in cold signal transmission in two general ways: one that transduces innocuous cool temperatures to trigger cool sensation, behavioral thermoregulation, and analgesia; and another that transmits nociceptive signals to trigger more overtly nocifensive behaviors (Chung and Caterina, 2007). For the body in physiological state, TRPM8 predominantly activate the first, but in pathological conditions such as nerve injury or inflammation, TRPM8 mainly plays the second role (Marwaha et al., 2016; De Caro et al., 2019). To understand how interactions among different concentrations of menthol, different chemical or physical stimuli, and the structure of the TRPM8 channel determines pain states, it is necessary to understand that there are three important properties of their function that may be affected to modulate their gating (closing and opening of the ion conduction pathway): activation, sensitization and desensitization (Rosenbaum et al., 2022). To be more specific, in physiological states, low to moderate concentrations of menthol activate TRPM8 receptors to produce a feeling of coolness; at higher concentration, menthol could induce cold allodynia, and cold hyperalgesia mediated by TRPM8 sensitization; following nerve injury or chemical stimuli, menthol activates TRPM8 to mediate relief of mechanical allodynia and thermal hyperalgesia; long-term local exposure to menthol also desensitizes TRPM8-sensitive fibers. Based on the results of experiments with topical menthol solutions applied to rodents, the dividing line between “low” and “high” concentrations we refer to here is 10% wt/vol or 640 mM.
TRPM8 activation
Almost all the analgesic potency of menthol comes from its activation of TRPM8. It is worth noting that the term “analgesia” specifically refers to the relief of noxious heat, chemical stimuli, and mechanical allodynia. In patch-clamp recordings, menthol activated wild-type TRPM8 with the half maximal effective concentration (EC50) of 185.4 ± 69.4 μM (Xu et al., 2020). TRPM8 activation evokes an influx of Ca2+ into the primary sensory neuron, leading to its activation and the propagation of action potentials. The activation of TRPM8 by menthol has been extensively studied in a variety of in vivo rodent models. Proudfoot et al. (2006) demonstrated that peripherally (4 mM) or centrally (200 nM) applied menthol, in a model of neuropathic pain (chronic compressive nerve injury, CCI) marked reverse behavioral reflex sensitization to noxious heat and mechanical stimulation. In addition, sensitization specific thermal and mechanical analgesia induced by TRPM8 activation has also been observed in focal demyelination of the sciatic nerve (Proudfoot et al., 2006), Complete Freund’s adjuvant (CFA) intra plantar injection (Proudfoot et al., 2006; Pan et al., 2012), and chemical stimulus (such as capsaicin, acrolein or cinnamaldehyde) (Proudfoot et al., 2006; Liu et al., 2013). Systemic or topical application of menthol can dose-dependently increase pain threshold in rodents in hot plate tests (Galeotti et al., 2002; Klein et al., 2010; Liu et al., 2013). In addition, direct evidence that TRPM8 may explain all the analgesic activity of menthol was provided by Liu et al. (2013) using genetic and pharmacological approaches in mice: the gene deletion and selective inhibitor (AMG2850) of TRPM8 completely eliminated the TRPM8-dependent analgesia on chemical stimuli, noxious heat, and inflammation. It is worth noting that the activation of TRPM8 can be regulated by neurotrophic factors (Lippoldt et al., 2013, 2016), phosphatidylinositol bisphosphate (PIP2) (Premkumar et al., 2005; Rohács et al., 2005), Ca2+-independent phospholipase A2 (iPLA2) and polyunsaturated fatty acids (Andersson et al., 2007), forming a complex network.
It is important to note that in the above study, menthol produced TRPM8-dependent analgesia of acute and inflammatory pain over a wide range of concentrations and administration routes (oral, intraperitoneal, intraplantar or topical), from estimated systemic levels of 60−120 μM, to a topical concentration in the molar range (30% wt/vol) (Liu et al., 2013). This means, that this concentration range may be lower than the reported TRPM8 activation concentration; means that cause analgesia in the sensitized pain state with lower doses of TRPM8 activation.
TRPM8 sensitization
In the physiological state, high concentrations of menthol can induce TRPM8 sensitization and promote the development of cold allodynia and cold hyperalgesia by excessively lowering the cold pain threshold. Menthol-induced cold hypersensitivity is believed to primarily rely on direct sensitization of TRPM8 on Aδ and C-fibers (Andersen et al., 2014). Numerous studies indicate that high concentration of menthol can cause hyperalgesia in cold perception in rodents (>10% wt/vol or >640 mM for mice topically, Tajino et al., 2007), which may make menthol valuable in pre-clinical screening of analgesic agents for cold hyperalgesia (Rossi et al., 2006; Tajino et al., 2007), and it provides a theoretical basis for the establishment of experimental cold hyperalgesia pain model in human with topically high concentration of menthol (>30% wt/vol for healthy subjects, Hatem et al., 2006).
In contrast to the peripheral sensitization of TRPM8 neurons by menthol under physiological conditions, the actual conditions leading to hypersensitivity under pathological conditions are complex (Andersen et al., 2014). In the periphery, nociceptive cold fibers can become sensitized upon axonal degeneration leading to dysregulation of TRP channel expression, resulting in a more excitable phenotype (Gold et al., 2003). Preclinical studies have shown that TRPM8 expression in sensory neurons (DRG and superficial dorsal horn) is increased with the development of hypersensitivity to mechanical, thermal, and cold stimuli in rats with CCI (Proudfoot et al., 2006; Colburn et al., 2007; Frederick et al., 2007; Xing et al., 2007; Su et al., 2011, 2017) and L5 and L6 spinal nerve ligation (SNL) (Katsura et al., 2006; Patel et al., 2014; Koh et al., 2016). Overexpression of TRPM8 has been linked to development of cold allodynia. Evaporative cooling of acetone may represent an inherently less toxic cold stimulus, so it is common to assess cold sensitivity by measuring the response of laboratory animals to acetone application. Colburn et al. (2007) used CCI rodent model to observe significant sensitivity to acetone application. In contrast, TRPM8 gene deletion mice showed no significant increase in acetone sensitivity at any time after ligation (Rimola et al., 2021). This sensory disturbance was also successfully reversed by TRPM8 mRNA antisense (Su et al., 2011). Administration of oxaliplatin and other chemotherapeutic agents can lead to chemotherapy-induced peripheral neuropathy (CIPN), which is characterized by cold abnormal pain and mechanical hyperalgesia. Oxaliplatin treatment has been shown to induce an increase in TRPM8 expression in DRG (Kawashiri et al., 2012; Mizuno et al., 2014; Yamamoto et al., 2018), and that oxaliplatin-induced cold hypersensitivity is diminished in TRPM8 knockout mice (Descoeur et al., 2011). Recently updated studies have shown that the activity of the TRPM8 but not the activity of any other member of the TRP channel family is transiently increased after oxaliplatin treatment (Rimola et al., 2021). These changes will increase the body’s sensitivity to cold and thus reduce the perception of pathological pain, which may represent a protective effect of self-pain reduction in biological evolution.
TRPM8 desensitization
Desensitization occurs when the channel becomes refractory to an activating stimulus by adopting a conformational state where the passage of ions is not allowed. In the context of the nociceptive system, desensitization may function as a protective mechanism in which hyperexcitation or cell death by ion-dependent signaling pathways is avoided (Rosenbaum et al., 2022). To be specific, the activity of the cold- and menthol-activated TRPM8 diminishes over time in the presence of extracellular Ca2+ (Yudin et al., 2011; Diver et al., 2019), which is manifested by a decrease in the cooling effect over time when exposed to menthol, along with a decrease in TRPM8 activity (Cliff and Green, 1994; Reid and Flonta, 2001; Matsu-ura et al., 2006; Daniels et al., 2009). In addition, menthol can cause a short-lasting cross-desensitization of other irritant chemicals-induced stimulus including capsaicin (Cliff and Green, 1996; Green and McAuliffe, 2000; Naganawa et al., 2015), citric acid (Morice et al., 1994; Plevkova et al., 2013), cinnamaldehyde (Zanotto et al., 2008; Klein et al., 2011), and nicotine (Dessirier et al., 2001; Fan et al., 2016; Huynh et al., 2020, etc.), that is, a reduction in irritation evoked by compounds other than itself (Dessirier et al., 2001).
Transient receptor potential cation channel subfamily A member 1
However, abnormal pain induced by high menthol concentration still exists experimental fact that is difficult to be explained by sensitization of TRPM8. Fan et al. (2016) demonstrated that mice showed a concentration-dependent oral aversion of menthol (>100 μg/mL or >640 mM), and that gene deletion of TRPM8 did not reduce this aversion. Lemon et al. (2019) conducted a brief-access exposure tests in mice to measure reduction in orosensory avoidance behaviors to aqueous menthol solutions and showed that oral aversion to menthol was reduced in mice deficient for TRPA1 but not TRPM8.
Due to the low selectivity of menthol (Macpherson et al., 2006; Vogt-Eisele et al., 2007), it can also activate TRPA1 (Karashima et al., 2007; Xiao et al., 2008), which is a member of the TRP family with TRPM8. Expression of TRPA1 is limited in a subset of nociceptive neurons of the trigeminal ganglion and DRG (Story et al., 2003; Kobayashi et al., 2005) and is a sensor for a wide variety of environmental stimuli, such as intense cold activation temperature is <18°C (Story et al., 2003), close to 8°C, the reported threshold of noxious cold, Wahren et al. (1989), Tillman et al. (1995), and Harrison and Davis (1999) irritating compounds, reactive chemicals, and endogenous signals associated with cell injury (Talavera et al., 2020) to elicit protective responses. Although both respond to cold stimuli, TRPM8 and TRPA1 are activated by harmless cooling and harmful cold (Caterina et al., 1997; Bandell et al., 2004), respectively. Irritating compounds that directly activate TRPA1 and may cause pain in humans include cinnamaldehyde, allyl isothiocyanate, allicin, formalin (Bandell et al., 2004; Bautista et al., 2005; Moran et al., 2011), and bradykinin as inflammatory mediators (Bandell et al., 2004; Obata et al., 2005). TRPA1 has been implicated in several painful and inflammatory conditions and is considered a promising potential target for the development of, amongst others, analgesic, antipruritic and anti-inflammatory pharmacotherapeutics (Andrade et al., 2012; Koivisto et al., 2012; Preti et al., 2015). The irritating properties of menthol have been shown to be associated with this activation of TRPA1 (Karashima et al., 2007; Fan et al., 2016). In concert with the sensitivity of TRPM8 for menthol, Karashima et al. (2007) showed bell-shaped dose-response curve of menthol to channels by whole-cell and single-channel recordings of heterologously expressed TRPA1, demonstrate a bimodal sensitivity of TRPA1 to menthol, further proved that menthol acts as an effective agonist of TRPA1 at relative low concentrations (100−300 μM), but acts as an antagonist at higher concentrations [≥300 mM (Karashima et al., 2007)] by causing reversible channel blocking, although this high-concentration blocking effect was only found in rodent models, not human models (Macpherson et al., 2006; Karashima et al., 2007; Xiao et al., 2008; Lemon et al., 2019).
Transient receptor potential cation channel subfamily V member 1
Menthol also causes analgesic effects through TRPM8-independent mechanisms, such as the TRPV1, which is involved in the transmission and modulation of nociception, as well as the molecular integration of diverse painful stimuli (Huang et al., 2002; Cui et al., 2006). The activators of TRPV1 are noxious heat [activation temperature is >42°C, in the range of perception that shifts from innocuous warmth to noxious heat; the reported threshold of noxious heat is 55°C (Patapoutian et al., 2003)], acidic pH, capsaicin (the irritating compound in hot chili peppers), and allyl isothiocyanate (Everaerts et al., 2011). TRPV1 is a non-selective cation channel; when it is activated, Na+ and Ca2+ flowing into the cell to depolarize nociceptive neurons, leading to action potential firing and finally leads to a painful, burning sensation (Caterina et al., 1997). In particular, the functions of TRPV1 and TRPA1 interlink with each other to a considerable extent. This is especially clear in relation to pain and neurogenic inflammation where TRPV1 is co-expressed on the vast majority of TRPA1-expressing sensory nerves and both integrate a variety of noxious stimuli (Fernandes et al., 2012). At the same time, TRPV1 also has a bimodal characteristic similar to TRPA1, which is activated by low menthol concentration and inhibited by high menthol concentration. Nguyen et al. (2021) observed that TRPV1 was activated by 3 mM menthol (HEK293T cells expressing rat TRPV1) and Takaishi et al. (2016) found in vitro that the TRPV1 current was completely inhibited at higher concentrations (>10 mM, HEK293T cells expressing human TRPV1), and this concentration is sufficient to activate TRPM8 and induce anti-nociceptive effects in response to thermal and mechanical stimuli in practice. This may be related to an important feature of TRPV1, which is rapid desensitization after activation, making the channel refractory to further stimulation (Szallasi and Blumberg, 1999). Thus, TRPV1 agonist that can cause desensitization and TRPV1 antagonists can both be considered as promising novel types of analgesics in therapeutic regimen (Gavva et al., 2008; Gunthorpe and Chizh, 2009). This suggests that the desensitization/inhibition of menthol on TRPV1 may be one of the potential mechanisms to explain the analgesic effect of menthol, and menthol and its derivatives could be promising molecules to develop TRPV1 antagonists (Takaishi et al., 2016).
In addition, menthol activates TRPV3, which specifically expressed in keratinocytes (skin cells) to produce a “warm” sensation at innocuous temperatures (activation temperature is about 34−38°C) (Peier et al., 2002; Xu et al., 2002; Macpherson et al., 2006), which would seem to explain one of the curious effects of menthol administration is that it can make human subjects report spontaneous sensations of warmth (Green, 1985; Hatem et al., 2006). In vitro heterologous TRPV3 expressing CHO cells, 0.5−2 mM menthol induced TRPV3 currents in a concentration dependent manner, while TRPA1 and TRPV1 currents were inhibited. Also, the sensitization to TRPV3 currents was observed with repeated application of 0.5 mM menthol (Macpherson et al., 2006). Although TRPV3 and TRPV1 share over 40% identity (Peier et al., 2002; Smith et al., 2002; Xu et al., 2002), little research has been done on the structural features that make them functionally so different.
As mentioned above, menthol induces analgesia through several different TRP mechanisms. For example, the simultaneous administration of menthol in response to capsaicin-induced hyperalgesia may also be involved: activation and desensitization of TRPM8 by menthol, activation of TRPA1 by menthol at higher concentrations, activation of TRPV1 and TRPA1 by capsaicin, inhibition of TRPV1 by menthol, and other mechanisms. The resulting analgesic effect of menthol should be the net effect of this complex network of mechanisms. Rodent models of menthol and its analogs are shown in Table 1.
Menthol and glutamate receptor
Activation of TRPM8 by menthol analog icilin is reported to produce analgesia by activating central inhibitory pathways (Chung and Caterina, 2007; Dhaka et al., 2007), which make use of inhibitory group II/III metabotropic glutamate receptors in the spinal cord (mGluRs). Numerous studies revealed that the mGluRs play a major role in modulatory central nervous system pathways and have been suggested to have pharmacological antinociceptive implications in inflammatory, neuropathic, and acute pain (Fisher et al., 2002; Simmons et al., 2002; Chen and Pan, 2005).
Proudfoot et al. (2006) demonstrated that intrathecal administration of group II and group III mGluRs antagonists prevented the reversal of thermal and mechanical sensitization of intrathecal menthol (200 nM) and topical icilin (200 μM) after CCI in rats, from which they hypothesized that: the activation of TRPM8 by menthol or icilin in a subpopulation of afferents results in the release of glutamate from central synapses, which then acts through inhibitory group II/III mGluRs located either pre-synaptically on injury-activated nociceptive afferents or perhaps also post-synaptically on dorsal-horn neurons, thereby attenuating neuropathic sensitization. These findings highlight the possible value of menthol and its analogs as TRPM8 activators and downstream central targets of TRPM8 action as novel analgesics for development.
Menthol and opioid
Transient receptor potential cation channel subfamily M member 8-mediated menthol antinociception was found to be dependent on activation of the endogenous opioid pathway (Galeotti et al., 2002; Liu et al., 2013; Patel et al., 2014), and intensive opioid receptors stimulation can also suppresses TRPM8 activity in DRG neurons, lead to the internalization of TRPM8 to pain relief (Shapovalov et al., 2013), suggesting that the two share a common pathway for modulating pain sensation in general. The non-selective opioid receptor antagonist naloxone (Liu et al., 2013; Shapovalov et al., 2013), and the selective κ-antagonist nor-NBI significantly reduced the TRPM8-dependent analgesia of acute and inflammatory pain (Galeotti et al., 2002), whereas the selective μ-antagonist CTOP and δ1-antagonist 7-benzylidenenal-trexone (BNTX) and δ2-antagonist naltriben did not prevent menthol-induced antinociception, therefore, the menthol-induced TRPM8-dependent analgesia is more likely mediated by the activation of the central κ-opioid system, excluding the involvement of μ and δ opioid systems (Galeotti et al., 2002).
Opioid tolerance and opioid-induced hyperalgesia is common in patients who have for chronically treated with opioids (Colvin et al., 2019). Cold allodynia is also a common complaint of patients chronically treated with opioids. In contrast with the findings that acute morphine triggers TRPM8 internalization (Shapovalov et al., 2013) and Gong and Jasmin (2017) demonstrated in rodent models that chronic morphine administration induced cold hyperalgesia and up-regulation of TRPM8. However, knockdown, or selective blocking of TRPM8 can reduce cold hyperalgesia caused by morphine. Iftinca et al. (2020) further confirmed that chronic morphine enhances cold hypersensitivity by increasing excitability and reduction desensitization in TRPM8-expressing neurons through activation of μ-opioid-receptor-protein kinase C beta (MOR-PKCβ) signaling pathway, which can be reversed by a follow-up treatment with the inverse non-selective opioid receptor antagonist naloxone (Shapovalov et al., 2013).
In addition to TRPM8, a strong connection has been suggested between the TRPV1 and the opioid pathway. Chronic administration of morphine up-regulates TRPV1 expression in spinal cord, DRG and sciatic nerve (Niiyama et al., 2007; Chen et al., 2008; Vardanyan et al., 2009); blocking TRPV1 by SB366971 (Chen et al., 2008) and capsazepine (Nguyen et al., 2010) or destructing TRPV1-expressing sensory neurons by resiniferatoxin (Chen and Pan, 2006) significantly inhibited morphine tolerance in rodents. TRPV1 has also been reported to be a physiological regulator of opioid receptors through a β-arrestin2 and PKA-dependent manner (Bao et al., 2015; Scherer et al., 2017; Basso et al., 2019). Thus, it is conceivable that menthol-mediated modulation of TRPV1, and thus regulation of opioid pathways affording a promising avenue to improve the therapeutic profile of opioid medications.
In addition, studies have shown that TRPA1, which is involved in the formation of harmful signals, significantly attenuates its association with μ-opioid receptors in the DRG, spinal cord and brain areas under the mediation by calcium-activated calmodulin in formalin-induced inflammatory pain and CCI-induced neuropathic pain in mice (Cortés-Montero et al., 2020).
In conclusion, existing studies demonstrate cross-talk interactions between opioid pathways and TRP, these mechanisms could provide a basis for better understanding general analgesia associated with opiate administration and hyperalgesia associated with abstinence. We illustrate the possible mechanisms of menthol action on the DRG or trigeminal ganglion (Figure 1).
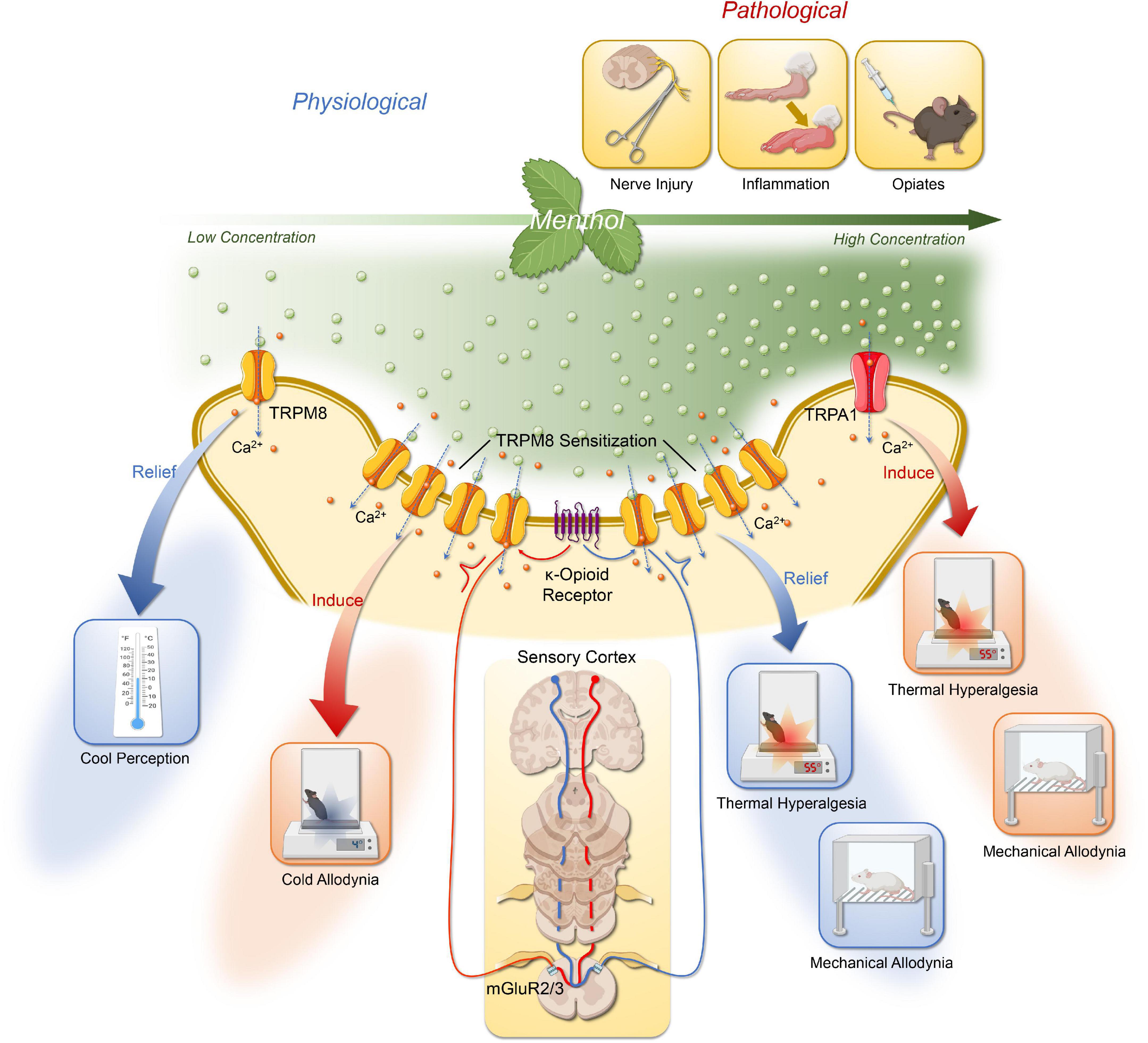
Figure 1. The possible mechanisms of menthol action on the DRG or trigeminal ganglion via TRPM8 and TRPA1. The effects of menthol are concentration dependent. Low to moderate concentrations of menthol activate TRPM8 in the DRG and trigeminal ganglion to produce a cooling sensation; at higher concentration, menthol could induce cold allodynia, and cold hyperalgesia mediated by TRPM8 sensitization; following nerve injury or chemical stimuli, menthol activates TRPM8 to mediate relief of mechanical allodynia and thermal hyperalgesia. Its analgesic effect is also dependent on the activation of central group II/III mGluR with endogenous κ-opioid signaling pathways. In addition, the thermal and mechanical allodynia of high concentrations of menthol is associated with its activation of TRPA1. Created with BioRender.com.
Other potential mechanisms
In addition to the above mentioned, a number of different kinds of ligand and voltage-gated ion channels for menthol mediated analgesia have been proposed, and these mechanisms may partially explain the TRP-independent mechanisms are responsible for menthol analgesia.
Voltage-gated Na+ channels
Menthol has local anesthetic activity. Galeotti et al. (2001) found that menthol significantly reduced electrically induced contractions of rat phrenic hemidiaphragm in a dose-dependent manner; the number of stimuli necessary to provoke the rabbit conjunctival reflex increased in a dose-dependent manner. By recording heterologously expressed whole-cell sodium inward currents in rat neuronal and human skeletal muscle, Haeseler et al. (2002) used patch-clamp to demonstrate that menthol acts like the local anesthetic lidocaine to suppress whole cell Na+ currents with an IC50 of 571 and 376 μM for neuronal cells and skeletal muscle fibers, respectively. In another electrophysiological study, Gaudioso et al. (2012) conclude that menthol inhibits Nav1.8, Nav1.9, and TTX-sensitive Na+ channels in a concentration-, voltage-, and frequency-dependent manner. It is worth mentioning that eucalyptol (an agonist of TRPM8, 1 mM), and BCTC (a TRPM8 antagonist, IC50 = 0.8 μM) had no effect on the above Na+ currents, indicating that the blocking effect of menthol on Na+ channels is independent of TRPM8. Another study on cortical neurons reported that menthol (250 μM) dampens the generation of action potentials in TRPM8 knock-out mice and in the presence of a TRPM8 blocker, suggest that menthol can modulate voltage-gated sodium channels in cortical neurons through a TRP-independent pathway (Pezzoli et al., 2014).
Voltage-gated Ca2+ channels
Several reports have demonstrated that menthol modulates the functional properties of voltage-gated Ca2+ channels. In vitro studies have shown that menthol blocks Ca2+ influx in Helix neurons (Swandulla et al., 1986), cultured DRG cells (Swandulla et al., 1987), LA-N-5 human neuroblastoma cells (Sidell et al., 1990), leech neurons (Dierkes et al., 1997), guinea-pig ileal smooth muscle, rat and guinea-pig atrial and papillary muscle, rat brain synaptosomes and chick retinal neurons (Hawthorn et al., 1988). In addition, menthol decreased the muscle contractures of tracheal (Ito et al., 2008; Wang et al., 2016), bronchial (Wright et al., 1997), vas deference (Filippov et al., 2009; Vladymyrova et al., 2011), colon (Amato et al., 2014), and aortae, mesenteric, coronary arteries (Cheang et al., 2013) smooth muscle by inhibiting of L-type voltage-gated calcium channels. Ramos-Filho et al. (2014) proved that menthol (300 μM) or nifedipine (1 μM) inhibited carbachol and electrical field stimulation induced contractions in both wild type and TRPM8 knockout bladder strips, conclude that menthol inhibits smooth muscle contraction by blocking L-type Ca2+ channels in a manner independent of TRPM8 activation. Menthol not only limits Ca2+ influx through voltage-gated Ca2+ channels, but also induces Ca2+ release from intracellular storage pools (endoplasmic reticulum and Golgi) in a TRP-independent manner (Palade, 1987; Tsuzuki et al., 2004; Lu et al., 2006; Mahieu et al., 2007; Wondergem and Bartley, 2009; Neumann and Copello, 2011; Melanaphy et al., 2016). Based on these findings, Melanaphy et al. (2016) revealed that the vasorelaxation effect of menthol and other TRPM8 agonists on isolated rat tail artery myocytes is the net effect of non-specific inhibition of L-type Ca2+ channels and activation of Ca2+ release in the sarcoplasmic reticulum. These findings will promote the understanding of the vascular effects of TRPM8, particularly the well-known hypotensive effects of menthol (Sun et al., 2014), and may also have translational implications (Melanaphy et al., 2016).
In addition, menthol can potently inhibit Kv7.2/3 channels (a voltage-gated K+ channel) isoforms (IC50 = 289 μM) and their mediated M currents. These mechanisms may be helpful in explaining TRPM8-mediated cooling and analgesic effects (Vetter et al., 2013).
GABAA receptors
In the central nervous system, γ-amino butyric acid (GABA) is the major inhibitory neurotransmitter. In responding to an action potential, it is released from the pre-synaptic button into the synaptic cleft and acts on either ionotropic GABAA receptors or metabotropic G-protein coupled GABAB receptors. Among them, the activation of GABAA receptors causes hyperpolarization and eventually the suppression of neuronal activity (Fritschy et al., 2012; Sigel and Steinmann, 2012). In practice, GABAA are the main targets of many sedatives and general anesthetics (Millan, 2003; Hemmings et al., 2005). Menthol acts as a potent positive allosteric modulator at GABAA (Hall et al., 2004; Corvalán et al., 2009). Menthol can enhance the GABAA-mediated currents in the spinal dorsal horn (Pan et al., 2012), CA1 pyramidal neurons of the hippocampus (Zhang X. B. et al., 2008), periaqueductal grey (PAG) neurons in midbrain slices (Lau et al., 2014) and Xenopus oocytes (Watt et al., 2008) in vitro. In particular, the TRPM8 agonist icilin, the non-selective TRPM8/TRPV1 antagonist Capsazepine or the TRPA1 antagonist HC-030031 did not affect menthol potentiation of GABAA receptor-mediated currents in PAG neurons in midbrain slices from rats, indicating that the menthol actions occur independently of TRP channel activation (Lau et al., 2014). Just like propofol, which acts on a similar site in the GABAA receptor and has a general anesthetic effect, menthol has been shown to be a moderate anesthetic with an EC50 of 23 μM, about 10-fold less potent anesthesia than propofol (Thorup et al., 1983; Krasowski et al., 2001). While menthol was far less potent than propofol in producing anesthesia its toxicity is minimal (Thorup et al., 1983) and therefore point to menthol as a lead compound for the development of novel GABAergic modulatory drugs (Watt et al., 2008).
Nicotinic acetylcholine receptors
Menthol is also often added to tobacco products because of the “coolness” of smoke, which is believed to bring pleasure to smokers (Werley et al., 2007); at the same time, menthol may reduce respiratory irritation response found in tobacco smoke (Hans et al., 2012). These applications have been proved to be related to possible functional interaction between menthol and nicotinic acetylcholine receptors (nAChRs) (Dessirier et al., 2001; Ruskin et al., 2008; Willis et al., 2011; Hans et al., 2012; Ashoor et al., 2013a). The nAChRs are cation-permeable ion channel-receptor complex activated by the neurotransmitter acetylcholine, which play important roles in several physiological functions and pathological conditions (Dani and Bertrand, 2007; Dineley et al., 2015). In vitro electrophysiologic studies showed that menthol was a negative allosteric regulator of α4β2 subtypes of nAChRs (Hans et al., 2012); menthol can even desensitize α3β4 subtype of nAChRs through allosteric mechanism (Ton et al., 2015); and menthol can also inhibit the α7 subtype of nAChRs non-competitively (Ashoor et al., 2013a). In addition, Henderson et al. (2016) demonstrated that chronic menthol induces cell-type-selective up-regulation of α4* subtype of nAChRs and also alters midbrain dopamine neuron function; more importantly, since menthol plus nicotine can produce greater reward-related behavior than nicotine alone (Henderson et al., 2017), this may explain the difficulty in quitting smoking (Foulds et al., 2010), whereas menthol can enhance smoking behavior and stimulate adverse effect of smoking on health as an additive to tobacco products (Kabbani, 2013; Wickham, 2015).
Menthol is also an allosteric non-competitive inhibitor of the 5-hydroxytryptamine type 3 (5-HT3) receptor (Heimes et al., 2011; Ashoor et al., 2013b; Walstab et al., 2014; Ziemba et al., 2015), which may explain its effective antiemetic properties.
It is worth noting that Liu et al. (2013) pointed out in their study on the role of TRPM8 in menthol induced analgesia that, in addition to activating TRPM8 channels, the concentration in the experiment (60−120 μM for systemic use and 30% wt/vol for external use) was sufficient or far more than the concentration required for pharmacological effects on other potential targets involved in menthol induced analgesia mediator mentioned above. However, any residual analgesic effect of menthol was not observed in TRPM8 knockout mice, which may imply these alternative targets: 1. may have little or no interaction with menthol to affect pain perception; 2. may only play a role in menthol analgesia under specific situations; 3. may have specific analgesic effect for other menthol isoforms (Liu et al., 2013). Therefore, further studies are needed to explore the interaction between menthol and these other potential alternative targets in analgesic effects.
Menthol in clinical practice
Over the past 15 years, a large number of menthol and its derivatives have been described by large pharmaceutical companies, small biotech companies and the academic community, but only a few have made it into clinical trials and even fewer have completed clinical trials.
Menthol-induced human cold hyperalgesia pain model
Under pathophysiological conditions, normally innocuous skin cooling can induce pain. This cold hyperalgesia (allodynia) is a striking symptom in patients with neuropathic pain (Lindblom and Verrillo, 1979; Wahren and Torebjörk, 1992; Woolf and Mannion, 1999; Baron, 2000). Translational surrogate models of pain, allodynia and hyperalgesia make it possible to evaluate the efficacy of drugs in pre-clinical settings and contribute to improved screening, diagnosis and treatment strategies for patients by applying these models in healthy volunteers to narrow the gap between animals and patients (Arendt-Nielsen and Yarnitsky, 2009). However, pathological animal models such as CCI, SNL and CIPN that induce neuropathic pain or hyperalgesia are often irreversible and invasive and cannot be used as human replacement models per se. As above, there have been numerous pre-clinical studies using menthol to establish cold hypersensitivity. In view of this, topical high concentration of L-menthol is the only human experimental pain model established to investigate the mechanism of cold hyperalgesia (Andersen et al., 2015). The work of Andersen et al. (2014) summarized the topical application of menthol in humans as a transformational model for cold abnormal pain and hyperalgesia. According to their findings, most of the menthol was applied to the volar forearm (Wasner et al., 2004; Namer et al., 2005; Hatem et al., 2006; Flühr et al., 2009); the highest menthol concentration that can actually be triggered effectively is about 40% (Wasner et al., 2004, 2008; Namer et al., 2005, 2008; Seifert and Maihöfner, 2007; Altis et al., 2009; Flühr et al., 2009; Binder et al., 2011; Mahn et al., 2014), but a 30% menthol ethanol solution proved to be the ideal formulation because of its effectiveness and safety (Hatem et al., 2006); the most common spontaneous sensation reported by subjects during application was coldness or mild pain; all studies reviewed consistently found a significant increase in cold pain threshold in healthy volunteers (Andersen et al., 2015). Human studies on topical menthol induced cold pain are summarized in Table 2.
Musculoskeletal pain
Knowledge about the feasibility of topical menthol for musculoskeletal pain dates back centuries, as topical menthol has been widely used to treat muscle soreness (Silva, 2020). The most common musculoskeletal pain is low back pain, which affects 30% to 40% of the general population and is the leading cause of disability (Hartvigsen et al., 2018). Using a randomized controlled design, Zhang J. et al. (2008) determined to add Biofreeze (Performance Health Inc., Export, PA, United States), a locally applied pain-killing agent containing menthol, to the chiropractic treatment plan for patients with low back pain, with significant pain relief effect. However, there were no significant changes in Roland-Morris Disability Questionnaire (RMDQ), heart rate variability, or LBP electromyography (EMG) compared with the control group, and further studies with a larger sample size are needed to confirm the findings (Zhang J. et al., 2008). In addition, NCT03012503 has compared the effects of Biofreeze with placebo on neck pain after cervical massage with chiropractic, but no results have been published to date.
Osteoarthritis (OA) is the main cause of disability in the elderly (Glyn-Jones et al., 2015). Topp et al. compare the ability to complete functional tasks and knee pain while completing functional tasks among 20 patients with knee OA after topical application of either 3.5% menthol gel or an inert placebo gel, but no differences were found in functional tasks or pain (Topp et al., 2013). NCT03888807 attempted to determine the effects of Biofreeze vs. placebo on walking gait characteristics and walking pain in patients with bilateral knee OA but announced early termination due to difficulty in enrolling subjects. In addition, NCT03888807, NCT04351594, and NCT01565070 have been evaluating the effect of Biofreeze on knee OA, but no results have been obtained.
Post-exercise myalgia and delayed onset muscle soreness (DOMS) are common symptoms after exercise and physical activity. Stefanelli et al. (2019) found that DOMS reduced corticospinal excitability and after the administration of menthol-based topical analgesic, there was a reduction in pain, which was accompanied by increased corticospinal inhibition. Although ice application is considered the traditional method for post-exercise pain relief, Johar et al. (2012) demonstrated that compared to ice, the topical menthol-based analgesic decreased perceived discomfort to a greater extent and permitted greater tetanic forces to be produced, and the effectiveness of ice for acute injury has not been proven in any clinical trials and may even be harmful (Bleakley et al., 2004). Airaksinen et al. (2003) showed that topical menthol gels not only provide excellent pain relief, but also promote recovery in patients with exercise-related soft tissue injuries. Higashi et al. (2010) reported in a double-blind randomized controlled trial that a single 8-h patch containing methyl salicylate and menthol significantly alleviated pain associated with mild to moderate muscle strain in these adult patients compared to patients receiving a placebo patch. NCT02100670 is a multicenter, randomized, double-blind, placebo-controlled, parallel-group trial performed to evaluate topical 1% diclofenac/3% menthol gel in treating ankle sprain, but no significant improvement was observed with topical 1% diclofenac/3% menthol gel compared with placebo, 1% diclofenac, or 3% menthol gel in treating pain from ankle sprain (Lai et al., 2017).
Carpal tunnel syndrome is a neuromuscular disease caused by increased pressure on the median nerve of the wrist, with common symptoms include wrist and hand pain, paresthesia, thenar muscle weakness and loss of flexibility (Padua et al., 2016). A three-blind randomized placebo-controlled crossover trial demonstrated that topical menthol significantly reduced pain intensity compared with placebo in abattoir workers with chronic pain and carpal tunnel syndrome symptoms (Sundstrup et al., 2014).
Neuropathic pain
Neuropathic pain is defined as pain caused by a lesion or disease of the somatosensory nervous system and is a major therapeutic challenge. Common causes of neuropathic pain include cerebrovascular accident, multiple sclerosis or spinal cord injury, or peripheral nervous system, for example, painful diabetic neuropathy, postherpetic neuralgia, or surgery (Boyd et al., 2019). The first reported use of menthol in neuropathic pain dates to 1870, when menthol oil was successfully used to treat neuropathic facial pain.
Chemotherapy-induced peripheral neuropathy is a severe and painful adverse reaction of cancer treatment in patients. CIPN that occurs during chemotherapy, sometimes requiring dose reduction or cessation, impacting on survival (Colvin, 2019). Menthol activated TRPM8 channels are a promising therapeutic target in CIPN (Colvin, 2019). In Fallon et al. (2015), in a proof-of-concept study, determined that 82% of evaluable patients had an improvement in their total pain scores after 4−6 weeks of treatment with topical 1% menthol cream, and 50% had a clinically relevant reduction in pain scores of at least 30%. Cortellini et al. (2017) reported the remarkable treatment with menthol cream of a male patient with a history of metastatic colon cancer and previous chemotherapies who had neuropathy that impaired his quality of life and limited further chemotherapy. Another clinical study (NCT01855607, as shown in Table 2) assessed whether 6 weeks of treatment with topical menthol twice daily would reduce CIPN in patients have completed adjuvant or neo-adjuvant Taxane based breast cancer therapy or Oxaliplatin based colon cancer chemotherapy. Recently, a phase II study (NCT04276727, as shown in Table 2) used a special brain scan called functional magnetic resonance imaging (fMRI) to help determine whether topical menthol therapy has potential for CIPN patients. In a word, CIPN patients may benefit from the use of menthol, either during the treatment of patients complain of subjective improvement, lead to a better quality of life, and can be implemented without interruption of chemotherapy and effective chemotherapy dose delivery, which in turn lead to longer survival, is likely to be an effective potential palliative treatment option.
Neuropathy is one of the most common long-term complications of diabetes, characterized by altered thermal, mechanical, and chemical sensation. Of three large, clinic-based studies from Europe, the prevalence of diabetic neuropathy varied from 23–29% (Young et al., 1993; Tesfaye et al., 1996; Cabezas-Cerrato, 1998). Preclinical studies have shown that there was an increase in TRPV1-mediated currents but a decrease in TRPM8-mediated currents in DRG isolated from diabetic hyperalgesia mice. Therefore, menthol, which has the effect of targeting TRPV1 or TRPM8, may be a useful approach to treat pain associated with diabetic neuropathy (Pabbidi and Premkumar, 2017). There has been a randomized, double-blind, placebo-controlled crossover trial of the efficacy and safety of menthol topically alone or in combination with mannitol in the relief of diabetic neuropathy (NCT02728687), with no results have been reported.
In addition, Davies et al. (2002) reported successful relief of postherpetic neuralgia using topical peppermint oil in combination with other interventions.
Others
Management of postoperative pain relieve suffering and leads to earlier mobilization, shortened hospital stay, reduced hospital costs, and increased patient satisfaction (Gupta et al., 2010). Engelhard et al. (2019) observed a beneficial effect of cooling by a menthol-containing bandage during the rehabilitation phase after anterior cruciate ligament reconstruction. The reduction of muscle cross section within 30 days after surgery was prevented by menthol dressings, which highly contributed to rehabilitation success after 90 days of therapy and can reduce painkiller consumption (Engelhard et al., 2019).
Genome-wide association analysis have revealed that TRPM8 is associated with susceptibility to migraine without aura (Freilinger et al., 2012), and reduced TRPM8 expression (rs10166942 carriers) helps reduce migraine risk in humans (Gavva et al., 2019), which may provide the rationale for the topical menthol as an alternative treatment option for migraine patients. A randomized, triple-blind, placebo-controlled, crossed-over study demonstrated the efficacy, safety, and relative tolerability of cutaneous application of 10% menthol solution in the treatment of migraine without aura (Borhani Haghighi et al., 2010). An open-label pilot study showed that 52% of subjects experienced at least one statistically significant improvement in migraine severity 2 h after using topical menthol (St Cyr et al., 2015). Due to the limitations of these studies, larger placebo-controlled clinical trials are needed.
Pruritus and pain are closely related but distinct sensations. There is a broad overlap between pain- and itch-related peripheral mediators and/or receptors, and there are astonishingly similar mechanisms of neuronal sensitization in the peripheral and central (Ikoma et al., 2006). Analgesia and antipruritic therapy should have common targets. In 1995, Bromm et al. found that topical menthol had a central inhibitory effect on histamine-induced pruritus of the lower left arm in 15 healthy male volunteers (Bromm et al., 1995). Andersen et al. (2017) conducted a similar study and got the same conclusion, further verified the antipruritic effect of menthol like doxepin. Using a combination of pharmacologic, genetic, and mouse behavioral assays, Palkar et al. (2018) found that the inhibition of pruritus by menthol requires TRPM8 channels or intact and functional TRPM8-expressing afferent neurons. Menthol and its analogs that rely on TRPM8 channels are a promising target for the development of pruritus therapies, and further clinical trials could significantly improve the management of pruritus.
Conclusion
We summarize the progress of menthol in pain and analgesia research based on existing evidence and demonstrate the important role of menthol in clinical analgesia. Although TRPM8 may explain the analgesic effect of menthol, the molecular mechanism between upstream and downstream has not been fully elucidated, and the exact association between menthol, TRP family, mGluRs and endogenous κ-opioid signaling pathway has not been established. Furthermore, menthol interactions with TRPA1 and other targets may have pro-nociceptive and inflammatory effects. In fact, topical menthol treatment is often accompanied by skin irritation, and inhalation of menthol can aggravate asthma in some patients, which may be the role of TRPA1 (Bautista et al., 2006; Zhang X. B. et al., 2008; Caceres et al., 2009). This is very similar to the action of beta-adrenergic receptors in the circulatory system. Therefore, it is urgent to develop TRPM8-specific drugs to replace menthol in analgesic and anti-irritant therapy, to prevent these adverse effects and allow more effective analgesic treatment.
Author contributions
LZ, ZL, and HZ designed and collected the literatures, and wrote the manuscript. YW and YL collected some literatures and created the figure and tables. LZ and QL revised the manuscript. All authors contributed to the article and approved the submitted version.
Funding
This work was supported by research grants of National Natural Science Foundation of China to LZ (Grant nos. 82171205 and 81801107) and National Natural Science Foundation of China to YL (Grant no. 82071243).
Conflict of interest
The authors declare that the research was conducted in the absence of any commercial or financial relationships that could be construed as a potential conflict of interest.
Publisher’s note
All claims expressed in this article are solely those of the authors and do not necessarily represent those of their affiliated organizations, or those of the publisher, the editors and the reviewers. Any product that may be evaluated in this article, or claim that may be made by its manufacturer, is not guaranteed or endorsed by the publisher.
References
Airaksinen, O. V., Kyrklund, N., Latvala, K., Kouri, J. P., Grönblad, M., and Kolari, P. (2003). Efficacy of cold gel for soft tissue injuries: A prospective randomized double-blinded trial. Am. J. Sports Med. 31, 680–684. doi: 10.1177/03635465030310050801
Altis, K., Schmidtko, A., Angioni, C., Kuczka, K., Schmidt, H., Geisslinger, G., et al. (2009). Analgesic efficacy of tramadol, pregabalin and ibuprofen in menthol-evoked cold hyperalgesia. Pain 147, 116–121. doi: 10.1016/j.pain.2009.08.018
Amato, A., Liotta, R., and Mulè, F. (2014). Effects of menthol on circular smooth muscle of human colon: Analysis of the mechanism of action. Eur. J. Pharmacol. 740, 295–301. doi: 10.1016/j.ejphar.2014.07.018
Andersen, H. H., Gazerani, P., and Arendt-Nielsen, L. (2016). High-concentration L-menthol exhibits counter-irritancy to neurogenic inflammation, thermal and mechanical hyperalgesia caused by trans-cinnamaldehyde. J. Pain 17, 919–929. doi: 10.1016/j.jpain.2016.05.004
Andersen, H. H., Melholt, C., Hilborg, S. D., Jerwiarz, A., Randers, A., Simoni, A., et al. (2017). Antipruritic effect of cold-induced and transient receptor potential-agonist-induced counter-irritation on histaminergic itch in humans. Acta Derm. Venereol. 97, 63–67. doi: 10.2340/00015555-2447
Andersen, H. H., Olsen, R. V., Møller, H. G., Eskelund, P. W., Gazerani, P., and Arendt-Nielsen, L. (2014). A review of topical high-concentration L-menthol as a translational model of cold allodynia and hyperalgesia. Eur. J. Pain 18, 315–325. doi: 10.1002/j.1532-2149.2013.00380.x
Andersen, H. H., Poulsen, J. N., Uchida, Y., Nikbakht, A., Arendt-Nielsen, L., and Gazerani, P. (2015). Cold and L-menthol-induced sensitization in healthy volunteers–a cold hypersensitivity analogue to the heat/capsaicin model. Pain 156, 880–889. doi: 10.1097/j.pain.0000000000000123
Andersson, D. A., Nash, M., and Bevan, S. (2007). Modulation of the cold-activated channel TRPM8 by lysophospholipids and polyunsaturated fatty acids. J. Neurosci. 27, 3347–3355. doi: 10.1523/jneurosci.4846-06.2007
Andrade, E. L., Meotti, F. C., and Calixto, J. B. (2012). TRPA1 antagonists as potential analgesic drugs. Pharmacol. Ther. 133, 189–204. doi: 10.1016/j.pharmthera.2011.10.008
Arendt-Nielsen, L., and Yarnitsky, D. (2009). Experimental and clinical applications of quantitative sensory testing applied to skin, muscles and viscera. J. Pain 10, 556–572. doi: 10.1016/j.jpain.2009.02.002
Ashoor, A., Nordman, J. C., Veltri, D., Yang, K. H., Al Kury, L., Shuba, Y., et al. (2013a). Menthol binding and inhibition of α7-nicotinic acetylcholine receptors. PLoS One 8:e67674. doi: 10.1371/journal.pone.0067674
Ashoor, A., Nordman, J. C., Veltri, D., Yang, K. H., Shuba, Y., Al Kury, L., et al. (2013b). Menthol inhibits 5-HT3 receptor-mediated currents. J. Pharmacol. Exp. Ther. 347, 398–409. doi: 10.1124/jpet.113.203976
Bandell, M., Story, G. M., Hwang, S. W., Viswanath, V., Eid, S. R., Petrus, M. J., et al. (2004). Noxious cold ion channel TRPA1 is activated by pungent compounds and bradykinin. Neuron 41, 849–857. doi: 10.1016/s0896-6273(04)00150-3
Bao, Y., Gao, Y., Yang, L., Kong, X., Yu, J., Hou, W., et al. (2015). The mechanism of μ-opioid receptor (MOR)-TRPV1 crosstalk in TRPV1 activation involves morphine anti-nociception, tolerance and dependence. Channels (Austin) 9, 235–243. doi: 10.1080/19336950.2015.1069450
Baron, R. (2000). Peripheral neuropathic pain: From mechanisms to symptoms. Clin. J. Pain 16, S12–S20. doi: 10.1097/00002508-200006001-00004
Basso, L., Aboushousha, R., Fan, C. Y., Iftinca, M., Melo, H., Flynn, R., et al. (2019). TRPV1 promotes opioid analgesia during inflammation. Sci. Signal. 12:eaav0711. doi: 10.1126/scisignal.aav0711
Bautista, D. M., Jordt, S. E., Nikai, T., Tsuruda, P. R., Read, A. J., Poblete, J., et al. (2006). TRPA1 mediates the inflammatory actions of environmental irritants and proalgesic agents. Cell 124, 1269–1282. doi: 10.1016/j.cell.2006.02.023
Bautista, D. M., Movahed, P., Hinman, A., Axelsson, H. E., Sterner, O., Högestätt, E. D., et al. (2005). Pungent products from garlic activate the sensory ion channel TRPA1. Proc. Natl. Acad. Sci. U.S.A. 102, 12248–12252. doi: 10.1073/pnas.0505356102
Binder, A., Stengel, M., Klebe, O., Wasner, G., and Baron, R. (2011). Topical high-concentration (40%) menthol-somatosensory profile of a human surrogate pain model. J. Pain 12, 764–773. doi: 10.1016/j.jpain.2010.12.013
Bleakley, C., McDonough, S., and MacAuley, D. (2004). The use of ice in the treatment of acute soft-tissue injury: A systematic review of randomized controlled trials. Am. J. Sports Med. 32, 251–261. doi: 10.1177/0363546503260757
Borhani Haghighi, A., Motazedian, S., Rezaii, R., Mohammadi, F., Salarian, L., Pourmokhtari, M., et al. (2010). Cutaneous application of menthol 10% solution as an abortive treatment of migraine without aura: A randomised, double-blind, placebo-controlled, crossed-over study. Int. J. Clin. Pract. 64, 451–456. doi: 10.1111/j.1742-1241.2009.02215.x
Boyd, A., Bleakley, C., Hurley, D. A., Gill, C., Hannon-Fletcher, M., Bell, P., et al. (2019). Herbal medicinal products or preparations for neuropathic pain. Cochrane Database Syst. Rev. 4:Cd010528. doi: 10.1002/14651858.CD010528.pub4
Brid, T., Miranda, G., Lozano, B., and Baamonde, A. (2015). Topical L-menthol for postradiotherapy neuropathic pain: A case report. J. Pain Symptom Manage. 50, e2–e4. doi: 10.1016/j.jpainsymman.2015.04.024
Bromm, B., Scharein, E., Darsow, U., and Ring, J. (1995). Effects of menthol and cold on histamine-induced itch and skin reactions in man. Neurosci Lett. 187, 157–160. doi: 10.1016/0304-3940(95)11362-z
Cabezas-Cerrato, J. (1998). The prevalence of clinical diabetic polyneuropathy in Spain: A study in primary care and hospital clinic groups. Neuropathy spanish study group of the spanish diabetes society (SDS). Diabetologia 41, 1263–1269. doi: 10.1007/s001250051063
Caceres, A. I., Brackmann, M., Elia, M. D., Bessac, B. F., del Camino, D., D’Amours, M., et al. (2009). A sensory neuronal ion channel essential for airway inflammation and hyperreactivity in asthma. Proc. Natl. Acad. Sci. U.S.A. 106, 9099–9104. doi: 10.1073/pnas.0900591106
Caspani, O., Zurborg, S., Labuz, D., and Heppenstall, P. A. (2009). The contribution of TRPM8 and TRPA1 channels to cold allodynia and neuropathic pain. PLoS One 4:e7383. doi: 10.1371/journal.pone.0007383
Caterina, M. J., Schumacher, M. A., Tominaga, M., Rosen, T. A., Levine, J. D., and Julius, D. (1997). The capsaicin receptor: A heat-activated ion channel in the pain pathway. Nature 389, 816–824. doi: 10.1038/39807
Cheang, W. S., Lam, M. Y., Wong, W. T., Tian, X. Y., Lau, C. W., Zhu, Z., et al. (2013). Menthol relaxes rat aortae, mesenteric and coronary arteries by inhibiting calcium influx. Eur. J. Pharmacol. 702, 79–84. doi: 10.1016/j.ejphar.2013.01.028
Chen, S. R., and Pan, H. L. (2005). Distinct roles of group III metabotropic glutamate receptors in control of nociception and dorsal horn neurons in normal and nerve-injured Rats. J. Pharmacol. Exp. Ther. 312, 120–126. doi: 10.1124/jpet.104.073817
Chen, S. R., and Pan, H. L. (2006). Loss of TRPV1-expressing sensory neurons reduces spinal mu opioid receptors but paradoxically potentiates opioid analgesia. J. Neurophysiol. 95, 3086–3096. doi: 10.1152/jn.01343.2005
Chen, Y., Geis, C., and Sommer, C. (2008). Activation of TRPV1 contributes to morphine tolerance: Involvement of the mitogen-activated protein kinase signaling pathway. J. Neurosci. 28, 5836–5845. doi: 10.1523/jneurosci.4170-07.2008
Chung, M. K., and Caterina, M. J. (2007). TRP channel knockout mice lose their cool. Neuron 54, 345–347. doi: 10.1016/j.neuron.2007.04.025
Cliff, M. A., and Green, B. G. (1994). Sensory irritation and coolness produced by menthol: Evidence for selective desensitization of irritation. Physiol. Behav. 56, 1021–1029. doi: 10.1016/0031-9384(94)90338-7
Cliff, M. A., and Green, B. G. (1996). Sensitization and desensitization to capsaicin and menthol in the oral cavity: Interactions and individual differences. Physiol. Behav. 59, 487–494. doi: 10.1016/0031-9384(95)02089-6
Cohen, S. P., Vase, L., and Hooten, W. M. (2021). Chronic pain: An update on burden, best practices, and new advances. Lancet 397, 2082–2097. doi: 10.1016/s0140-6736(21)00393-7
Colburn, R. W., Lubin, M. L., Stone, D. J. Jr., Wang, Y., Lawrence, D., D’Andrea, M. R., et al. (2007). Attenuated cold sensitivity in TRPM8 null mice. Neuron 54, 379–386. doi: 10.1016/j.neuron.2007.04.017
Colvin, L. A. (2019). Chemotherapy-induced peripheral neuropathy: Where are we now? Pain 160 Suppl 1, S1–S10. doi: 10.1097/j.pain.0000000000001540
Colvin, L. A., Bull, F., and Hales, T. G. (2019). Perioperative opioid analgesia-when is enough too much? A review of opioid-induced tolerance and hyperalgesia. Lancet 393, 1558–1568. doi: 10.1016/s0140-6736(19)30430-1
Cortellini, A., Verna, L., Cannita, K., Napoleoni, L., Parisi, A., Ficorella, C., et al. (2017). Topical menthol for treatment of chemotherapy-induced peripheral neuropathy. Indian J. Palliat. Care 23, 350–352. doi: 10.4103/ijpc.Ijpc_23_17
Cortés-Montero, E., Rodríguez-Muñoz, M., Ruiz-Cantero, M. D. C., Cobos, E. J., Sánchez-Blázquez, P., and Garzón-Niño, J. (2020). Calmodulin supports TRPA1 channel association with opioid receptors and glutamate NMDA receptors in the nervous tissue. Int. J. Mol. Sci. 22:229. doi: 10.3390/ijms22010229
Corvalán, N. A., Zygadlo, J. A., and García, D. A. (2009). Stereo-selective activity of menthol on GABA(A) receptor. Chirality 21, 525–530. doi: 10.1002/chir.20631
Cui, M., Honore, P., Zhong, C., Gauvin, D., Mikusa, J., Hernandez, G., et al. (2006). TRPV1 receptors in the CNS play a key role in broad-spectrum analgesia of TRPV1 antagonists. J. Neurosci. 26, 9385–9393. doi: 10.1523/jneurosci.1246-06.2006
Dani, J. A., and Bertrand, D. (2007). Nicotinic acetylcholine receptors and nicotinic cholinergic mechanisms of the central nervous system. Annu. Rev. Pharmacol. Toxicol. 47, 699–729. doi: 10.1146/annurev.pharmtox.47.120505.105214
Daniels, R. L., Takashima, Y., and McKemy, D. D. (2009). Activity of the neuronal cold sensor TRPM8 is regulated by phospholipase C via the phospholipid phosphoinositol 4,5-bisphosphate. J. Biol. Chem. 284, 1570–1582. doi: 10.1074/jbc.M807270200
Davies, S. J., Harding, L. M., and Baranowski, A. P. (2002). A novel treatment of postherpetic neuralgia using peppermint oil. Clin. J. Pain 18, 200–202. doi: 10.1097/00002508-200205000-00011
De Caro, C., Cristiano, C., Avagliano, C., Bertamino, A., Ostacolo, C., Campiglia, P., et al. (2019). Characterization of new TRPM8 modulators in pain perception. Int. J. Mol. Sci. 20:5544. doi: 10.3390/ijms20225544
Descoeur, J., Pereira, V., Pizzoccaro, A., Francois, A., Ling, B., Maffre, V., et al. (2011). Oxaliplatin-induced cold hypersensitivity is due to remodelling of ion channel expression in nociceptors. EMBO Mol. Med. 3, 266–278. doi: 10.1002/emmm.201100134
Dessirier, J. M., O’Mahony, M., and Carstens, E. (2001). Oral irritant properties of menthol: Sensitizing and desensitizing effects of repeated application and cross-desensitization to nicotine. Physiol. Behav. 73, 25–36. doi: 10.1016/s0031-9384(01)00431-0
Dhaka, A., Murray, A. N., Mathur, J., Earley, T. J., Petrus, M. J., and Patapoutian, A. (2007). TRPM8 is required for cold sensation in mice. Neuron 54, 371–378. doi: 10.1016/j.neuron.2007.02.024
Dierkes, P. W., Hochstrate, P., and Schlue, W. R. (1997). Voltage-dependent Ca2+ influx into identified leech neurones. Brain Res. 746, 285–293. doi: 10.1016/s0006-8993(96)01264-4
Dineley, K. T., Pandya, A. A., and Yakel, J. L. (2015). Nicotinic ACh receptors as therapeutic targets in CNS disorders. Trends Pharmacol. Sci. 36, 96–108. doi: 10.1016/j.tips.2014.12.002
Diver, M. M., Cheng, Y., and Julius, D. (2019). Structural insights into TRPM8 inhibition and desensitization. Science 365, 1434–1440. doi: 10.1126/science.aax6672
Engelhard, D., Hofer, P., and Annaheim, S. (2019). Evaluation of the effect of cooling strategies on recovery after surgical intervention. BMJ Open Sport Exerc. Med. 5:e000527. doi: 10.1136/bmjsem-2019-000527
Everaerts, W., Gees, M., Alpizar, Y. A., Farre, R., Leten, C., Apetrei, A., et al. (2011). The capsaicin receptor TRPV1 is a crucial mediator of the noxious effects of mustard oil. Curr. Biol. 21, 316–321. doi: 10.1016/j.cub.2011.01.031
Fallon, M. T., Storey, D. J., Krishan, A., Weir, C. J., Mitchell, R., Fleetwood-Walker, S. M., et al. (2015). Cancer treatment-related neuropathic pain: Proof of concept study with menthol–a TRPM8 agonist. Support. Care Cancer 23, 2769–2777. doi: 10.1007/s00520-015-2642-8
Fan, L., Balakrishna, S., Jabba, S. V., Bonner, P. E., Taylor, S. R., Picciotto, M. R., et al. (2016). Menthol decreases oral nicotine aversion in C57BL/6 mice through a TRPM8-dependent mechanism. Tob. Control. 25, ii50–ii54. doi: 10.1136/tobaccocontrol-2016-053209
Fernandes, E. S., Fernandes, M. A., and Keeble, J. E. (2012). The functions of TRPA1 and TRPV1: Moving away from sensory nerves. Br. J. Pharmacol. 166, 510–521. doi: 10.1111/j.1476-5381.2012.01851.x
Fernández, J. A., Skryma, R., Bidaux, G., Magleby, K. L., Scholfield, C. N., McGeown, J. G., et al. (2011). Voltage- and cold-dependent gating of single TRPM8 ion channels. J. Gen. Physiol. 137, 173–195. doi: 10.1085/jgp.201010498
Filippov, I. B., Vladymyrova, I. A., Kuliieva Ie, M., Skryma, R., Prevarskaia, N., and Shuba, et al. (2009). [Modulation of the smooth muscle contractions of the rat vas deferens by TRPM8 channel agonist menthol]. Fiziol Zh (1994) 55, 30–40.
Fisher, K., Lefebvre, C., and Coderre, T. J. (2002). Antinociceptive effects following intrathecal pretreatment with selective metabotropic glutamate receptor compounds in a rat model of neuropathic pain. Pharmacol. Biochem. Behav. 73, 411–418. doi: 10.1016/s0091-3057(02)00832-8
Flühr, K., Neddermeyer, T. J., and Lötsch, J. (2009). Capsaicin or menthol sensitization induces quantitative but no qualitative changes to thermal and mechanical pain thresholds. Clin. J. Pain 25, 128–131. doi: 10.1097/AJP.0b013e3181817aa2
Foulds, J., Hooper, M. W., Pletcher, M. J., and Okuyemi, K. S. (2010). Do smokers of menthol cigarettes find it harder to quit smoking? Nicotine Tob. Res. 12, Suppl 2 S102–S109. doi: 10.1093/ntr/ntq166
Frederick, J., Buck, M. E., Matson, D. J., and Cortright, D. N. (2007). Increased TRPA1, TRPM8, and TRPV2 expression in dorsal root ganglia by nerve injury. Biochem. Biophys. Res. Commun. 358, 1058–1064. doi: 10.1016/j.bbrc.2007.05.029
Freilinger, T., Anttila, V., de Vries, B., Malik, R., Kallela, M., Terwindt, G. M., et al. (2012). Genome-wide association analysis identifies susceptibility loci for migraine without aura. Nat. Genet. 44, 777–782. doi: 10.1038/ng.2307
Fritschy, J. M., Panzanelli, P., and Tyagarajan, S. K. (2012). Molecular and functional heterogeneity of GABAergic synapses. Cell. Mol. Life Sci. 69, 2485–2499. doi: 10.1007/s00018-012-0926-4
Galeotti, N., Di Cesare Mannelli, L., Mazzanti, G., Bartolini, A., and Ghelardini, C. (2002). Menthol: A natural analgesic compound. Neurosci. Lett. 322, 145–148. doi: 10.1016/s0304-3940(01)02527-7
Galeotti, N., Ghelardini, C., Mannelli, L., Mazzanti, G., Baghiroli, L., and Bartolini, A. (2001). Local anaesthetic activity of (+)- and (-)-menthol. Planta Med. 67, 174–176. doi: 10.1055/s-2001-11515
Gaudioso, C., Hao, J., Martin-Eauclaire, M. F., Gabriac, M., and Delmas, P. (2012). Menthol pain relief through cumulative inactivation of voltage-gated sodium channels. Pain 153, 473–484. doi: 10.1016/j.pain.2011.11.014
Gavva, N. R., Sandrock, R., Arnold, G. E., Davis, M., Lamas, E., Lindvay, C., et al. (2019). Reduced TRPM8 expression underpins reduced migraine risk and attenuated cold pain sensation in humans. Sci. Rep. 9:19655. doi: 10.1038/s41598-019-56295-0
Gavva, N. R., Treanor, J. J., Garami, A., Fang, L., Surapaneni, S., Akrami, A., et al. (2008). Pharmacological blockade of the vanilloid receptor TRPV1 elicits marked hyperthermia in humans. Pain 136, 202–210. doi: 10.1016/j.pain.2008.01.024
Gillis, D. J., Vellante, A., Gallo, J. A., and D’Amico, A. P. (2020). Influence of menthol on recovery from exercise-induced muscle damage. J. Strength Cond. Res. 34, 451–462. doi: 10.1519/jsc.0000000000002833
Glyn-Jones, S., Palmer, A. J., Agricola, R., Price, A. J., Vincent, T. L., Weinans, H., et al. (2015). Osteoarthritis. Lancet 386, 376–387. doi: 10.1016/s0140-6736(14)60802-3
Gold, M. S., Weinreich, D., Kim, C. S., Wang, R., Treanor, J., Porreca, F., et al. (2003). Redistribution of Na(V)1.8 in uninjured axons enables neuropathic pain. J. Neurosci. 23, 158–166. doi: 10.1523/jneurosci.23-01-00158.2003
Gong, K., and Jasmin, L. (2017). Sustained morphine administration induces TRPM8-dependent cold hyperalgesia. J. Pain 18, 212–221. doi: 10.1016/j.jpain.2016.10.015
Green, B. G. (1985). Menthol modulates oral sensations of warmth and cold. Physiol. Behav. 35, 427–434. doi: 10.1016/0031-9384(85)90319-1
Green, B. G., and McAuliffe, B. L. (2000). Menthol desensitization of capsaicin irritation. Evidence of a short-term anti-nociceptive effect. Physiol. Behav. 68, 631–639. doi: 10.1016/s0031-9384(99)00221-8
Gudin, J. A., Dietze, D. T., and Hurwitz, P. L. (2020). Improvement of pain and function after use of a topical pain relieving patch: Results of the RELIEF study. J. Pain Res. 13, 1557–1568. doi: 10.2147/jpr.S258883
Gunthorpe, M. J., and Chizh, B. A. (2009). Clinical development of TRPV1 antagonists: Targeting a pivotal point in the pain pathway. Drug Discov. Today 14, 56–67. doi: 10.1016/j.drudis.2008.11.005
Gupta, A., Kaur, K., Sharma, S., Goyal, S., Arora, S., and Murthy, R. S. (2010). Clinical aspects of acute post-operative pain management & its assessment. J. Adv. Pharm. Technol. Res. 1, 97–108.
Haeseler, G., Maue, D., Grosskreutz, J., Bufler, J., Nentwig, B., Piepenbrock, S., et al. (2002). Voltage-dependent block of neuronal and skeletal muscle sodium channels by thymol and menthol. Eur. J. Anaesthesiol. 19, 571–579. doi: 10.1017/s0265021502000923
Hagenacker, T., Lampe, M., and Schäfers, M. (2014). Icilin reduces voltage-gated calcium channel currents in naïve and injured DRG neurons in the rat spinal nerve ligation model. Brain Res. 1557, 171–179. doi: 10.1016/j.brainres.2014.02.022
Hall, A. C., Turcotte, C. M., Betts, B. A., Yeung, W. Y., Agyeman, A. S., and Burk, L. A. (2004). Modulation of human GABAA and glycine receptor currents by menthol and related monoterpenoids. Eur. J. Pharmacol. 506, 9–16. doi: 10.1016/j.ejphar.2004.10.026
Hanprasertpong, T., Kor-anantakul, O., Leetanaporn, R., Suwanrath, C., Suntharasaj, T., Pruksanusak, N., et al. (2015). Reducing pain and anxiety during second trimester genetic amniocentesis using aromatic therapy: A randomized trial. J. Med. Assoc. Thai. 98, 734–738.
Hans, M., Wilhelm, M., and Swandulla, D. (2012). Menthol suppresses nicotinic acetylcholine receptor functioning in sensory neurons via allosteric modulation. Chem. Senses 37, 463–469. doi: 10.1093/chemse/bjr128
Harrison, J. L., and Davis, K. D. (1999). Cold-evoked pain varies with skin type and cooling rate: A psychophysical study in humans. Pain 83, 123–135. doi: 10.1016/s0304-3959(99)00099-8
Hartvigsen, J., Hancock, M. J., Kongsted, A., Louw, Q., Ferreira, M. L., Genevay, S., et al. (2018). What low back pain is and why we need to pay attention. Lancet 391, 2356–2367. doi: 10.1016/s0140-6736(18)30480-x
Hatem, S., Attal, N., Willer, J. C., and Bouhassira, D. (2006). Psychophysical study of the effects of topical application of menthol in healthy volunteers. Pain 122, 190–196. doi: 10.1016/j.pain.2006.01.026
Hawthorn, M., Ferrante, J., Luchowski, E., Rutledge, A., Wei, X. Y., and Triggle, D. J. (1988). The actions of peppermint oil and menthol on calcium channel dependent processes in intestinal, neuronal and cardiac preparations. Aliment. Pharmacol. Ther. 2, 101–118. doi: 10.1111/j.1365-2036.1988.tb00677.x
Heimes, K., Hauk, F., and Verspohl, E. J. (2011). Mode of action of peppermint oil and (-)-menthol with respect to 5-HT3 receptor subtypes: Binding studies, cation uptake by receptor channels and contraction of isolated rat ileum. Phytother. Res. 25, 702–708. doi: 10.1002/ptr.3316
Hemmings, H. C. Jr., Akabas, M. H., Goldstein, P. A., Trudell, J. R., Orser, B. A., and Harrison, N. L. (2005). Emerging molecular mechanisms of general anesthetic action. Trends Pharmacol. Sci. 26, 503–510. doi: 10.1016/j.tips.2005.08.006
Henderson, B. J., Wall, T. R., Henley, B. M., Kim, C. H., McKinney, S., and Lester, H. A. (2017). Menthol enhances nicotine reward-related behavior by potentiating nicotine-induced changes in nAChR function, nAChR upregulation, and DA neuron excitability. Neuropsychopharmacology 42, 2285–2291. doi: 10.1038/npp.2017.72
Henderson, B. J., Wall, T. R., Henley, B. M., Kim, C. H., Nichols, W. A., Moaddel, R., et al. (2016). Menthol alone upregulates midbrain nachrs, alters nachr subtype stoichiometry, alters dopamine neuron firing frequency, and prevents nicotine reward. J. Neurosci. 36, 2957–2974. doi: 10.1523/jneurosci.4194-15.2016
Higashi, Y., Kiuchi, T., and Furuta, K. (2010). Efficacy and safety profile of a topical methyl salicylate and menthol patch in adult patients with mild to moderate muscle strain: A randomized, double-blind, parallel-group, placebo-controlled, multicenter study. Clin. Ther. 32, 34–43. doi: 10.1016/j.clinthera.2010.01.016
Hilfiger, L., Triaux, Z., Marcic, C., Héberlé, E., Emhemmed, F., Darbon, P., et al. (2021). Anti-hyperalgesic properties of menthol and pulegone. Front. Pharmacol. 12:753873. doi: 10.3389/fphar.2021.753873
Huang, S. M., Bisogno, T., Trevisani, M., Al-Hayani, A., De Petrocellis, L., Fezza, F., et al. (2002). An endogenous capsaicin-like substance with high potency at recombinant and native vanilloid VR1 receptors. Proc. Natl. Acad. Sci. U.S.A. 99, 8400–8405. doi: 10.1073/pnas.122196999
Huynh, Y. W., Raimondi, A., Finkner, A., Kuck, J. D., Selleck, C., and Bevins, R. A. (2020). Menthol blunts the interoceptive discriminative stimulus effects of nicotine in female but not male rats. Psychopharmacology (Berl) 237, 2395–2404. doi: 10.1007/s00213-020-05542-8
Iftinca, M., Basso, L., Flynn, R., Kwok, C., Roland, C., Hassan, A., et al. (2020). Chronic morphine regulates TRPM8 channels via MOR-PKCβ signaling. Mol Brain 13:61. doi: 10.1186/s13041-020-00599-0
Ikoma, A., Steinhoff, M., Ständer, S., Yosipovitch, G., and Schmelz, M. (2006). The neurobiology of itch. Nat. Rev. Neurosci. 7, 535–547. doi: 10.1038/nrn1950
Ito, S., Kume, H., Shiraki, A., Kondo, M., Makino, Y., Kamiya, K., et al. (2008). Inhibition by the cold receptor agonists menthol and icilin of airway smooth muscle contraction. Pulm. Pharmacol. Ther. 21, 812–817. doi: 10.1016/j.pupt.2008.07.001
Izquierdo, C., Martín-Martínez, M., Gómez-Monterrey, I., and González-Muñiz, R. (2021). TRPM8 channels: Advances in structural studies and pharmacological modulation. Int. J. Mol. Sci. 22:8502. doi: 10.3390/ijms22168502
Ji, G., Zhou, S., Kochukov, M. Y., Westlund, K. N., and Carlton, S. M. (2007). Plasticity in intact A delta- and C-fibers contributes to cold hypersensitivity in neuropathic rats. Neuroscience 150, 182–193. doi: 10.1016/j.neuroscience.2007.09.002
Johar, P., Grover, V., Topp, R., and Behm, D. G. (2012). A comparison of topical menthol to ice on pain, evoked tetanic and voluntary force during delayed onset muscle soreness. Int. J. Sports. Phys. Ther. 7, 314–322.
Kabbani, N. (2013). Not so Cool? Menthol’s discovered actions on the nicotinic receptor and its implications for nicotine addiction. Front. Pharmacol. 4:95. doi: 10.3389/fphar.2013.00095
Kamatou, G. P., Vermaak, I., Viljoen, A. M., and Lawrence, B. M. (2013). Menthol: A simple monoterpene with remarkable biological properties. Phytochemistry 96, 15–25. doi: 10.1016/j.phytochem.2013.08.005
Karashima, Y., Damann, N., Prenen, J., Talavera, K., Segal, A., Voets, T., et al. (2007). Bimodal action of menthol on the transient receptor potential channel TRPA1. J. Neurosci. 27, 9874–9884. doi: 10.1523/jneurosci.2221-07.2007
Katsura, H., Obata, K., Mizushima, T., Yamanaka, H., Kobayashi, K., Dai, Y., et al. (2006). Antisense knock down of TRPA1, but not TRPM8, alleviates cold hyperalgesia after spinal nerve ligation in rats. Exp. Neurol. 200, 112–123. doi: 10.1016/j.expneurol.2006.01.031
Kawashiri, T., Egashira, N., Kurobe, K., Tsutsumi, K., Yamashita, Y., Ushio, S., et al. (2012). L type Ca2+ channel blockers prevent oxaliplatin-induced cold hyperalgesia and TRPM8 overexpression in rats. Mol. Pain 8:7. doi: 10.1186/1744-8069-8-7
Kayama, Y., Shibata, M., Takizawa, T., Ibata, K., Shimizu, T., Ebine, T., et al. (2018). Functional interactions between transient receptor potential M8 and transient receptor potential V1 in the trigeminal system: Relevance to migraine pathophysiology. Cephalalgia 38, 833–845. doi: 10.1177/0333102417712719
Klein, A. H., Carstens, M. I., Zanotto, K. L., Sawyer, C. M., Ivanov, M., Cheung, S., et al. (2011). Self- and cross-desensitization of oral irritation by menthol and cinnamaldehyde (CA) via peripheral interactions at trigeminal sensory neurons. Chem. Sense 36, 199–208. doi: 10.1093/chemse/bjq115
Klein, A. H., Sawyer, C. M., Carstens, M. I., Tsagareli, M. G., Tsiklauri, N., and Carstens, E. (2010). Topical application of L-menthol induces heat analgesia, mechanical allodynia, and a biphasic effect on cold sensitivity in rats. Behav. Brain. Res. 212, 179–186. doi: 10.1016/j.bbr.2010.04.015
Kobayashi, K., Fukuoka, T., Obata, K., Yamanaka, H., Dai, Y., Tokunaga, A., et al. (2005). Distinct expression of TRPM8, TRPA1, and TRPV1 mRNAs in rat primary afferent neurons with adelta/c-fibers and colocalization with trk receptors. J. Comp. Neurol. 493, 596–606. doi: 10.1002/cne.20794
Koh, W. U., Choi, S. S., Kim, J. H., Yoon, H. J., Ahn, H. S., Lee, S. K., et al. (2016). The preventive effect of resiniferatoxin on the development of cold hypersensitivity induced by spinal nerve ligation: Involvement of TRPM8. BMC Neurosci. 17:38. doi: 10.1186/s12868-016-0273-8
Koivisto, A., Hukkanen, M., Saarnilehto, M., Chapman, H., Kuokkanen, K., Wei, H., et al. (2012). Inhibiting TRPA1 ion channel reduces loss of cutaneous nerve fiber function in diabetic animals: Sustained activation of the TRPA1 channel contributes to the pathogenesis of peripheral diabetic neuropathy. Pharmacol. Res. 65, 149–158. doi: 10.1016/j.phrs.2011.10.006
Kolassa, N. (2013). Menthol differs from other terpenic essential oil constituents. Regul. Toxicol. Pharmacol. 65, 115–118. doi: 10.1016/j.yrtph.2012.11.009
Kosiba, J. D., Hughes, M. T., LaRowe, L. R., Zvolensky, M. J., Norton, P. J., Smits, J. A. J., et al. (2019). Menthol cigarette use and pain reporting among African American adults seeking treatment for smoking cessation. Exp. Clin. Psychopharmacol. 27, 276–282. doi: 10.1037/pha0000254
Krasowski, M. D., Jenkins, A., Flood, P., Kung, A. Y., Hopfinger, A. J., and Harrison, N. L. (2001). General anesthetic potencies of a series of propofol analogs correlate with potency for potentiation of gamma-aminobutyric acid (GABA) current at the GABA(A) receptor but not with lipid solubility. J. Pharmacol. Exp. Ther. 297, 338–351.
Lai, P. M., Collaku, A., and Reed, K. (2017). Efficacy and safety of topical diclofenac/menthol gel for ankle sprain: A randomized, double-blind, placebo- and active-controlled trial. J. Int. Med. Res. 45, 647–661. doi: 10.1177/0300060517700322
Lau, B. K., Karim, S., Goodchild, A. K., Vaughan, C. W., and Drew, G. M. (2014). Menthol enhances phasic and tonic GABAA receptor-mediated currents in midbrain periaqueductal grey neurons. Br. J. Pharmacol. 171, 2803–2813. doi: 10.1111/bph.12602
Lemon, C. H., Norris, J. E., and Heldmann, B. A. (2019). The TRPA1 ion channel contributes to sensory-guided avoidance of menthol in mice. eNeuro 6, 304–319. doi: 10.1523/eneuro.0304-19.2019
Lindblom, U., and Verrillo, R. T. (1979). Sensory functions in chronic neuralgia. J. Neurol. Neurosurg. Psychiatry 42, 422–435. doi: 10.1136/jnnp.42.5.422
Lippoldt, E. K., Elmes, R. R., McCoy, D. D., Knowlton, W. M., and McKemy, D. D. (2013). Artemin, a glial cell line-derived neurotrophic factor family member, induces TRPM8-dependent cold pain. J. Neurosci. 33, 12543–12552. doi: 10.1523/jneurosci.5765-12.2013
Lippoldt, E. K., Ongun, S., Kusaka, G. K., and McKemy, D. D. (2016). Inflammatory and neuropathic cold allodynia are selectively mediated by the neurotrophic factor receptor GFRα3. Proc. Natl. Acad. Sci. U.S.A. 113, 4506–4511. doi: 10.1073/pnas.1603294113
Liu, B., Fan, L., Balakrishna, S., Sui, A., Morris, J. B., and Jordt, S. E. (2013). TRPM8 is the principal mediator of menthol-induced analgesia of acute and inflammatory pain. Pain 154, 2169–2177. doi: 10.1016/j.pain.2013.06.043
Liu, Y., Ye, X., Feng, X., Zhou, G., Rong, Z., Fang, C., et al. (2005). Menthol facilitates the skin analgesic effect of tetracaine gel. Int. J. Pharm. 305, 31–36. doi: 10.1016/j.ijpharm.2005.08.005
Lu, H. F., Hsueh, S. C., Yu, F. S., Yang, J. S., Tang, N. Y., Chen, S. C., et al. (2006). The role of Ca2+ in (-)-menthol-induced human promyelocytic leukemia HL-60 cell death. In. Vivo 20, 69–75.
Macpherson, L. J., Hwang, S. W., Miyamoto, T., Dubin, A. E., Patapoutian, A., and Story, G. M. (2006). More than cool: Promiscuous relationships of menthol and other sensory compounds. Mol. Cell. Neurosci. 32, 335–343. doi: 10.1016/j.mcn.2006.05.005
Mahieu, F., Owsianik, G., Verbert, L., Janssens, A., De Smedt, H., Nilius, B., et al. (2007). TRPM8-independent menthol-induced Ca2+ release from endoplasmic reticulum and Golgi. J. Biol. Chem. 282, 3325–3336. doi: 10.1074/jbc.M605213200
Mahn, F., Hüllemann, P., Wasner, G., Baron, R., and Binder, A. (2014). Topical high-concentration menthol: Reproducibility of a human surrogate pain model. Eur. J. Pain 18, 1248–1258. doi: 10.1002/j.1532-2149.2014.484.x
Marwaha, L., Bansal, Y., Singh, R., Saroj, P., Bhandari, R., and Kuhad, A. (2016). TRP channels: Potential drug target for neuropathic pain. Inflammopharmacology 24, 305–317. doi: 10.1007/s10787-016-0288-x
Matsu-ura, T., Michikawa, T., Inoue, T., Miyawaki, A., Yoshida, M., and Mikoshiba, K. (2006). Cytosolic inositol 1,4,5-trisphosphate dynamics during intracellular calcium oscillations in living cells. J. Cell. Biol. 173, 755–765. doi: 10.1083/jcb.200512141
McKemy, D. D., Neuhausser, W. M., and Julius, D. (2002). Identification of a cold receptor reveals a general role for TRP channels in thermosensation. Nature 416, 52–58. doi: 10.1038/nature719
Melanaphy, D., Johnson, C. D., Kustov, M. V., Watson, C. A., Borysova, L., Burdyga, T. V., et al. (2016). Ion channel mechanisms of rat tail artery contraction-relaxation by menthol involving, respectively, TRPM8 activation and L-type Ca2+ channel inhibition. Am. J. Physiol. Heart Circ. Physiol. 311, H1416–H1430. doi: 10.1152/ajpheart.00222.2015
Millan, M. J. (2003). The neurobiology and control of anxious states. Prog. Neurobiol. 70, 83–244. doi: 10.1016/s0301-0082(03)00087-x
Mizuno, K., Kono, T., Suzuki, Y., Miyagi, C., Omiya, Y., Miyano, K., et al. (2014). Goshajinkigan, a traditional Japanese medicine, prevents oxaliplatin-induced acute peripheral neuropathy by suppressing functional alteration of TRP channels in rat. J. Pharmacol. Sci. 125, 91–98. doi: 10.1254/jphs.13244fp
Moran, M. M., McAlexander, M. A., Bíró, T., and Szallasi, A. (2011). Transient receptor potential channels as therapeutic targets. Nat. Rev. Drug Discov. 10, 601–620. doi: 10.1038/nrd3456
Morice, A. H., Marshall, A. E., Higgins, K. S., and Grattan, T. J. (1994). Effect of inhaled menthol on citric acid induced cough in normal subjects. Thorax 49, 1024–1026. doi: 10.1136/thx.49.10.1024
Naganawa, T., Baad-Hansen, L., Ando, T., and Svensson, P. (2015). Influence of topical application of capsaicin, menthol and local anesthetics on intraoral somatosensory sensitivity in healthy subjects: Temporal and spatial aspects. Exp. Brain. Res. 233, 1189–1199. doi: 10.1007/s00221-015-4200-5
Namer, B., Kleggetveit, I. P., Handwerker, H., Schmelz, M., and Jorum, E. (2008). Role of TRPM8 and TRPA1 for cold allodynia in patients with cold injury. Pain 139, 63–72. doi: 10.1016/j.pain.2008.03.007
Namer, B., Seifert, F., Handwerker, H. O., and Maihöfner, C. (2005). TRPA1 and TRPM8 activation in humans: Effects of cinnamaldehyde and menthol. Neuroreport 16, 955–959. doi: 10.1097/00001756-200506210-00015
Neumann, J. T., and Copello, J. A. (2011). Cross-reactivity of ryanodine receptors with plasma membrane ion channel modulators. Mol. Pharmacol. 80, 509–517. doi: 10.1124/mol.111.071167
Nguyen, T. H. D., Itoh, S. G., Okumura, H., and Tominaga, M. (2021). Structural basis for promiscuous action of monoterpenes on TRP channels. Commun. Biol. 4:293. doi: 10.1038/s42003-021-01776-0
Nguyen, T. L., Nam, Y. S., Lee, S. Y., Kim, H. C., and Jang, C. G. (2010). Effects of capsazepine, a transient receptor potential vanilloid type 1 antagonist, on morphine-induced antinociception, tolerance, and dependence in mice. Br. J. Anaesth. 105, 668–674. doi: 10.1093/bja/aeq212
Niiyama, Y., Kawamata, T., Yamamoto, J., Omote, K., and Namiki, A. (2007). Bone cancer increases transient receptor potential vanilloid subfamily 1 expression within distinct subpopulations of dorsal root ganglion neurons. Neuroscience 148, 560–572. doi: 10.1016/j.neuroscience.2007.05.049
Obata, K., Katsura, H., Mizushima, T., Yamanaka, H., Kobayashi, K., Dai, Y., et al. (2005). TRPA1 induced in sensory neurons contributes to cold hyperalgesia after inflammation and nerve injury. J. Clin. Invest. 115, 2393–2401. doi: 10.1172/jci25437
Olsen, R. V., Andersen, H. H., Møller, H. G., Eskelund, P. W., and Arendt-Nielsen, L. (2014). Somatosensory and vasomotor manifestations of individual and combined stimulation of TRPM8 and TRPA1 using topical L-menthol and trans-cinnamaldehyde in healthy volunteers. Eur. J. Pain 18, 1333–1342. doi: 10.1002/j.1532-2149.2014.494.x
Oz, M., El Nebrisi, E. G., Yang, K. S., Howarth, F. C., and Al Kury, L. T. (2017). Cellular and molecular targets of menthol actions. Front. Pharmacol. 8:472. doi: 10.3389/fphar.2017.00472
Pabbidi, M. R., and Premkumar, L. S. (2017). Role of transient receptor potential channels Trpv1 and trpm8 in diabetic peripheral neuropathy. J Diabetes Treat. 2017:029.
Padua, L., Coraci, D., Erra, C., Pazzaglia, C., Paolasso, I., Loreti, C., et al. (2016). Carpal tunnel syndrome: Clinical features, diagnosis, and management. Lancet Neurol. 15, 1273–1284. doi: 10.1016/s1474-4422(16)30231-9
Palade, P. (1987). Drug-induced Ca2+ release from isolated sarcoplasmic reticulum. II. Releases involving a Ca2+-induced Ca2+ release channel. J. Biol. Chem. 262, 6142–6148.
Palkar, R., Ongun, S., Catich, E., Li, N., Borad, N., Sarkisian, A., et al. (2018). Cooling relief of acute and chronic itch requires TRPM8 channels and neurons. J. Invest. Dermatol. 138, 1391–1399. doi: 10.1016/j.jid.2017.12.025
Pan, R., Tian, Y., Gao, R., Li, H., Zhao, X., Barrett, J. E., et al. (2012). Central mechanisms of menthol-induced analgesia. J. Pharmacol. Exp. Ther. 343, 661–672. doi: 10.1124/jpet.112.196717
Parra, A., Gonzalez-Gonzalez, O., Gallar, J., and Belmonte, C. (2014). Tear fluid hyperosmolality increases nerve impulse activity of cold thermoreceptor endings of the cornea. Pain 155, 1481–1491. doi: 10.1016/j.pain.2014.04.025
Patapoutian, A., Peier, A. M., Story, G. M., and Viswanath, V. (2003). ThermoTRP channels and beyond: Mechanisms of temperature sensation. Nat. Rev. Neurosci. 4, 529–539. doi: 10.1038/nrn1141
Patel, R., Gonçalves, L., Leveridge, M., Mack, S. R., Hendrick, A., Brice, N. L., et al. (2014). Anti-hyperalgesic effects of a novel TRPM8 agonist in neuropathic rats: A comparison with topical menthol. Pain 155, 2097–2107. doi: 10.1016/j.pain.2014.07.022
Patel, T., Ishiuji, Y., and Yosipovitch, G. (2007). Menthol: A refreshing look at this ancient compound. J. Am. Acad. Dermatol. 57, 873–878. doi: 10.1016/j.jaad.2007.04.008
Peier, A. M., Reeve, A. J., Andersson, D. A., Moqrich, A., Earley, T. J., Hergarden, A. C., et al. (2002). A heat-sensitive TRP channel expressed in keratinocytes. Science 296, 2046–2049. doi: 10.1126/science.1073140
Pezzoli, M., Elhamdani, A., Camacho, S., Meystre, J., González, S. M., le Coutre, J., et al. (2014). Dampened neural activity and abolition of epileptic-like activity in cortical slices by active ingredients of spices. Sci. Rep. 4:6825. doi: 10.1038/srep06825
Plevkova, J., Kollarik, M., Poliacek, I., Brozmanova, M., Surdenikova, L., Tatar, M., et al. (2013). The role of trigeminal nasal TRPM8-expressing afferent neurons in the antitussive effects of menthol. J. Appl. Physiol. (1985) 115, 268–274. doi: 10.1152/japplphysiol.01144.2012
Premkumar, L. S., Raisinghani, M., Pingle, S. C., Long, C., and Pimentel, F. (2005). Downregulation of transient receptor potential melastatin 8 by protein kinase C-mediated dephosphorylation. J. Neurosci. 25, 11322–11329. doi: 10.1523/jneurosci.3006-05.2005
Preti, D., Saponaro, G., and Szallasi, A. (2015). Transient receptor potential ankyrin 1 (TRPA1) antagonists. Pharm. Pat. Anal. 4, 75–94. doi: 10.4155/ppa.14.60
Proudfoot, C. J., Garry, E. M., Cottrell, D. F., Rosie, R., Anderson, H., Robertson, D. C., et al. (2006). Analgesia mediated by the TRPM8 cold receptor in chronic neuropathic pain. Curr. Biol. 16, 1591–1605. doi: 10.1016/j.cub.2006.07.061
Quallo, T., Vastani, N., Horridge, E., Gentry, C., Parra, A., Moss, S., et al. (2015). TRPM8 is a neuronal osmosensor that regulates eye blinking in mice. Nat. Commun. 6:7150. doi: 10.1038/ncomms8150
Raddatz, N., Castillo, J. P., Gonzalez, C., Alvarez, O., and Latorre, R. (2014). Temperature and voltage coupling to channel opening in transient receptor potential melastatin 8 (TRPM8). J. Biol. Chem. 289, 35438–35454. doi: 10.1074/jbc.M114.612713
Ramos-Filho, A. C., Shah, A., Augusto, T. M., Barbosa, G. O., Leiria, L. O., de Carvalho, H. F., et al. (2014). Menthol inhibits detrusor contractility independently of TRPM8 activation. PLoS One 9:e111616. doi: 10.1371/journal.pone.0111616
Reid, G., and Flonta, M. L. (2001). Physiology. Cold current in thermoreceptive neurons. Nature 413:480. doi: 10.1038/35097164
Rimola, V., Osthues, T., Königs, V., Geißlinger, G., and Sisignano, M. (2021). Oxaliplatin causes transient changes in TRPM8 channel activity. Int. J. Mol. Sci. 22:4962. doi: 10.3390/ijms22094962
Rohács, T., Lopes, C. M., Michailidis, I., and Logothetis, D. E. (2005). PI(4,5)P2 regulates the activation and desensitization of TRPM8 channels through the TRP domain. Nat. Neurosci. 8, 626–634. doi: 10.1038/nn1451
Rosenbaum, T., Morales-Lázaro, S. L., and Islas, L. D. (2022). TRP channels: A journey towards a molecular understanding of pain. Nat. Rev. Neurosci. doi: 10.1038/s41583-022-00611-7 [Epub ahead of print].
Rossi, H. L., Vierck, C. J. Jr., Caudle, R. M., and Neubert, J. K. (2006). Characterization of cold sensitivity and thermal preference using an operant orofacial assay. Mol. Pain 2:37. doi: 10.1186/1744-8069-2-37
Ruskin, D. N., Anand, R., and LaHoste, G. J. (2008). Chronic menthol attenuates the effect of nicotine on body temperature in adolescent rats. Nicotine Tob. Res. 10, 1753–1759. doi: 10.1080/14622200802443734
Scherer, P. C., Zaccor, N. W., Neumann, N. M., Vasavda, C., Barrow, R., Ewald, A. J., et al. (2017). TRPV1 is a physiological regulator of μ-opioid receptors. Proc. Natl. Acad. Sci. U.S.A. 114, 13561–13566. doi: 10.1073/pnas.1717005114
Seifert, F., and Maihöfner, C. (2007). Representation of cold allodynia in the human brain–a functional MRI study. Neuroimage 35, 1168–1180. doi: 10.1016/j.neuroimage.2007.01.021
Shapovalov, G., Gkika, D., Devilliers, M., Kondratskyi, A., Gordienko, D., Busserolles, J., et al. (2013). Opiates modulate thermosensation by internalizing cold receptor TRPM8. Cell Rep. 4, 504–515. doi: 10.1016/j.celrep.2013.07.002
Sidell, N., Verity, M. A., and Nord, E. P. (1990). Menthol blocks dihydropyridine-insensitive Ca2+ channels and induces neurite outgrowth in human neuroblastoma cells. J. Cell. Physiol. 142, 410–419. doi: 10.1002/jcp.1041420226
Sigel, E., and Steinmann, M. E. (2012). Structure, function, and modulation of GABA(A) receptors. J. Biol. Chem. 287, 40224–40231. doi: 10.1074/jbc.R112.386664
Silva, H. (2020). A descriptive overview of the medical uses given to mentha aromatic herbs throughout history. Biology (Basel) 9:484. doi: 10.3390/biology9120484
Simmons, R. M., Webster, A. A., Kalra, A. B., and Iyengar, S. (2002). Group II mGluR receptor agonists are effective in persistent and neuropathic pain models in rats. Pharmacol. Biochem. Behav. 73, 419–427. doi: 10.1016/s0091-3057(02)00849-3
Smith, G. D., Gunthorpe, M. J., Kelsell, R. E., Hayes, P. D., Reilly, P., Facer, P., et al. (2002). TRPV3 is a temperature-sensitive vanilloid receptor-like protein. Nature 418, 186–190. doi: 10.1038/nature00894
Sprengell, C. J. (1708). Aphorisms of hippocrates: And the sentences of celsus; with explanations and references to the most considerable writers in physick and philosophy, both ancient and modern. London: R. Bonwick.
St Cyr, A., Chen, A., Bradley, K. C., Yuan, H., Silberstein, S. D., and Young, W. B. (2015). Efficacy and tolerability of STOPAIN for a migraine attack. Front. Neurol. 6:11. doi: 10.3389/fneur.2015.00011
Stefanelli, L., Lockyer, E. J., Collins, B. W., Snow, N. J., Crocker, J., Kent, C., et al. (2019). Delayed-onset muscle soreness and topical analgesic alter corticospinal excitability of the biceps brachii. Med. Sci. Sports Exerc. 51, 2344–2356. doi: 10.1249/mss.0000000000002055
Story, G. M., Peier, A. M., Reeve, A. J., Eid, S. R., Mosbacher, J., Hricik, T. R., et al. (2003). ANKTM1, a TRP-like channel expressed in nociceptive neurons, is activated by cold temperatures. Cell 112, 819–829. doi: 10.1016/s0092-8674(03)00158-2
Su, L., Shu, R., Song, C., Yu, Y., Wang, G., Li, Y., et al. (2017). Downregulations of TRPM8 expression and membrane trafficking in dorsal root ganglion mediate the attenuation of cold hyperalgesia in CCI rats induced by GFRα3 knockdown. Brain Res. Bull. 135, 8–24. doi: 10.1016/j.brainresbull.2017.08.002
Su, L., Wang, C., Yu, Y. H., Ren, Y. Y., Xie, K. L., and Wang, G. L. (2011). Role of TRPM8 in dorsal root ganglion in nerve injury-induced chronic pain. BMC Neurosci. 12:120. doi: 10.1186/1471-2202-12-120
Sun, J., Yang, T., Wang, P., Ma, S., Zhu, Z., Pu, Y., et al. (2014). Activation of cold-sensing transient receptor potential melastatin subtype 8 antagonizes vasoconstriction and hypertension through attenuating RhoA/Rho kinase pathway. Hypertension 63, 1354–1363. doi: 10.1161/hypertensionaha.113.02573
Sundstrup, E., Jakobsen, M. D., Brandt, M., Jay, K., Colado, J. C., Wang, Y., et al. (2014). Acute effect of topical menthol on chronic pain in slaughterhouse workers with carpal tunnel syndrome: Triple-blind, randomized placebo-controlled trial. Rehabil. Res. Pract. 2014:310913. doi: 10.1155/2014/310913
Swandulla, D., Carbone, E., Schäfer, K., and Lux, H. D. (1987). Effect of menthol on two types of Ca currents in cultured sensory neurons of vertebrates. Pflugers Arch. 409, 52–59. doi: 10.1007/bf00584749
Swandulla, D., Schäfer, K., and Lux, H. D. (1986). Calcium channel current inactivation is selectively modulated by menthol. Neurosci. Lett. 68, 23–28. doi: 10.1016/0304-3940(86)90223-5
Szallasi, A., and Blumberg, P. M. (1999). Vanilloid (Capsaicin) receptors and mechanisms. Pharmacol. Rev. 51, 159–212.
Tajino, K., Matsumura, K., Kosada, K., Shibakusa, T., Inoue, K., Fushiki, T., et al. (2007). Application of menthol to the skin of whole trunk in mice induces autonomic and behavioral heat-gain responses. Am. J. Physiol. Regul. Integr. Comp. Physiol. 293, R2128–R2135. doi: 10.1152/ajpregu.00377.2007
Takaishi, M., Uchida, K., Suzuki, Y., Matsui, H., Shimada, T., Fujita, F., et al. (2016). Reciprocal effects of capsaicin and menthol on thermosensation through regulated activities of TRPV1 and TRPM8. J. Physiol. Sci. 66, 143–155. doi: 10.1007/s12576-015-0427-y
Talavera, K., Startek, J. B., Alvarez-Collazo, J., Boonen, B., Alpizar, Y. A., Sanchez, A., et al. (2020). Mammalian transient receptor potential TRPA1 channels: From structure to disease. Physiol. Rev. 100, 725–803. doi: 10.1152/physrev.00005.2019
Taylor, R. Jr., Gan, T. J., Raffa, R. B., Gharibo, C., Pappagallo, M., Sinclair, N. R., et al. (2012). A randomized, double-blind comparison shows the addition of oxygenated glycerol triesters to topical mentholated cream for the treatment of acute musculoskeletal pain demonstrates incremental benefit over time. Pain Pract. 12, 610–619. doi: 10.1111/j.1533-2500.2012.00529.x
Tesfaye, S., Stevens, L. K., Stephenson, J. M., Fuller, J. H., Plater, M., Ionescu-Tirgoviste, C., et al. (1996). Prevalence of diabetic peripheral neuropathy and its relation to glycaemic control and potential risk factors: The EURODIAB IDDM Complications Study. Diabetologia 39, 1377–1384. doi: 10.1007/s001250050586
Thorup, I., Würtzen, G., Carstensen, J., and Olsen, P. (1983). Short term toxicity study in rats dosed with pulegone and menthol. Toxicol. Lett. 19, 207–210. doi: 10.1016/0378-4274(83)90120-0
Tillman, D. B., Treede, R. D., Meyer, R. A., and Campbell, J. N. (1995). Response of C fibre nociceptors in the anaesthetized monkey to heat stimuli: Correlation with pain threshold in humans. J. Physiol. 485 (Pt 3) 767–774. doi: 10.1113/jphysiol.1995.sp020767
Ton, H. T., Smart, A. E., Aguilar, B. L., Olson, T. T., Kellar, K. J., and Ahern, G. P. (2015). Menthol enhances the desensitization of human α3β4 nicotinic acetylcholine receptors. Mol. Pharmacol. 88, 256–264. doi: 10.1124/mol.115.098285
Topp, R., Brosky, J. A. Jr., and Pieschel, D. (2013). The effect of either topical menthol or a placebo on functioning and knee pain among patients with knee OA. J. Geriatr. Phys. Ther. 36, 92–99. doi: 10.1519/JPT.0b013e318268dde1
Tsuzuki, K., Xing, H., Ling, J., and Gu, J. G. (2004). Menthol-induced Ca2+ release from presynaptic Ca2+ stores potentiates sensory synaptic transmission. J. Neurosci. 24, 762–771. doi: 10.1523/jneurosci.4658-03.2004
Vardanyan, A., Wang, R., Vanderah, T. W., Ossipov, M. H., Lai, J., Porreca, F., et al. (2009). TRPV1 receptor in expression of opioid-induced hyperalgesia. J. Pain 10, 243–252. doi: 10.1016/j.jpain.2008.07.004
Vase, L., Svensson, P., Nikolajsen, L., Arendt-Nielsen, L., and Jensen, T. S. (2013). The effects of menthol on cold allodynia and wind-up-like pain in upper limb amputees with different levels of phantom limb pain. Neurosci. Lett. 534, 52–57. doi: 10.1016/j.neulet.2012.11.005
Vetter, I., Hein, A., Sattler, S., Hessler, S., Touska, F., Bressan, E., et al. (2013). Amplified cold transduction in native nociceptors by M-channel inhibition. J. Neurosci. 33, 16627–16641. doi: 10.1523/jneurosci.1473-13.2013
Vladymyrova, I. A., Filippov, I. B., Kuliieva Ie, M., Iurkevych, A., Skryma, R., Prevarskaia, N., et al. (2011). [Comparative effects of menthol and icilin on the induced contraction of the smooth muscles of the vas deferens of normal and castrated rats]. Fiziol Zh (1994) 57, 21–33.
Vogt-Eisele, A. K., Weber, K., Sherkheli, M. A., Vielhaber, G., Panten, J., Gisselmann, G., et al. (2007). Monoterpenoid agonists of TRPV3. Br. J. Pharmacol. 151, 530–540. doi: 10.1038/sj.bjp.0707245
Wade, A. G., Crawford, G. M., Young, D., Corson, S., and Brown, C. (2019). Comparison of diclofenac gel, ibuprofen gel, and ibuprofen gel with levomenthol for the topical treatment of pain associated with musculoskeletal injuries. J. Int. Med. Res. 47, 4454–4468. doi: 10.1177/0300060519859146
Wahren, L. K., and Torebjörk, E. (1992). Quantitative sensory tests in patients with neuralgia 11 to 25 years after injury. Pain 48, 237–244. doi: 10.1016/0304-3959(92)90063-h
Wahren, L. K., Torebjörk, E., and Jörum, E. (1989). Central suppression of cold-induced C fibre pain by myelinated fibre input. Pain 38, 313–319. doi: 10.1016/0304-3959(89)90218-2
Walstab, J., Wohlfarth, C., Hovius, R., Schmitteckert, S., Röth, R., Lasitschka, F., et al. (2014). Natural compounds boldine and menthol are antagonists of human 5-HT3 receptors: Implications for treating gastrointestinal disorders. Neurogastroenterol. Motil. 26, 810–820. doi: 10.1111/nmo.12334
Wang, H. W., Liu, S. C., Chao, P. Z., and Lee, F. P. (2016). Menthol inhibiting parasympathetic function of tracheal smooth muscle. Int. J. Med. Sci. 13, 923–928. doi: 10.7150/ijms.17042
Wasner, G., Naleschinski, D., Binder, A., Schattschneider, J., McLachlan, E. M., and Baron, R. (2008). The effect of menthol on cold allodynia in patients with neuropathic pain. Pain Med. 9, 354–358. doi: 10.1111/j.1526-4637.2007.00290.x
Wasner, G., Schattschneider, J., Binder, A., and Baron, R. (2004). Topical menthol–a human model for cold pain by activation and sensitization of C nociceptors. Brain 127, 1159–1171. doi: 10.1093/brain/awh134
Watt, E. E., Betts, B. A., Kotey, F. O., Humbert, D. J., Griffith, T. N., Kelly, E. W., et al. (2008). Menthol shares general anesthetic activity and sites of action on the GABA(A) receptor with the intravenous agent, propofol. Eur. J. Pharmacol. 590, 120–126. doi: 10.1016/j.ejphar.2008.06.003
Wei, E. T., and Seid, D. A. (1983). AG-3-5: A chemical producing sensations of cold. J. Pharm. Pharmacol. 35, 110–112. doi: 10.1111/j.2042-7158.1983.tb04279.x
Werley, M. S., Coggins, C. R., and Lee, P. N. (2007). Possible effects on smokers of cigarette mentholation: A review of the evidence relating to key research questions. Regul. Toxicol. Pharmacol. 47, 189–203. doi: 10.1016/j.yrtph.2006.09.004
Wickham, R. J. (2015). How menthol alters tobacco-smoking behavior: A biological perspective. Yale J. Biol. Med. 88, 279–287.
Willis, D. N., Liu, B., Ha, M. A., Jordt, S. E., and Morris, J. B. (2011). Menthol attenuates respiratory irritation responses to multiple cigarette smoke irritants. Faseb J. 25, 4434–4444. doi: 10.1096/fj.11-188383
Wondergem, R., and Bartley, J. W. (2009). Menthol increases human glioblastoma intracellular Ca2+, BK channel activity and cell migration. J. Biomed. Sci. 16:90. doi: 10.1186/1423-0127-16-90
Woolf, C. J., and Mannion, R. J. (1999). Neuropathic pain: Aetiology, symptoms, mechanisms, and management. Lancet 353, 1959–1964. doi: 10.1016/s0140-6736(99)01307-0
Wright, A., Benson, H. A. E., and Moss, P. (2019). Development of a topical menthol stimulus to evaluate cold hyperalgesia. Musculoskelet Sci. Pract. 41, 55–63. doi: 10.1016/j.msksp.2019.03.010
Wright, C. E., Laude, E. A., Grattan, T. J., and Morice, A. H. (1997). Capsaicin and neurokinin A-induced bronchoconstriction in the anaesthetised guinea-pig: Evidence for a direct action of menthol on isolated bronchial smooth muscle. Br. J. Pharmacol. 121, 1645–1650. doi: 10.1038/sj.bjp.0701319
Wright, L., Baptista-Hon, D., Bull, F., Dalgaty, F., Gallacher, M., Ibbotson, S. H., et al. (2018). Menthol reduces phototoxicity pain in a mouse model of photodynamic therapy. Pain 159, 284–297. doi: 10.1097/j.pain.0000000000001096
Xiao, B., Dubin, A. E., Bursulaya, B., Viswanath, V., Jegla, T. J., and Patapoutian, A. (2008). Identification of transmembrane domain 5 as a critical molecular determinant of menthol sensitivity in mammalian TRPA1 channels. J. Neurosci. 28, 9640–9651. doi: 10.1523/jneurosci.2772-08.2008
Xing, H., Chen, M., Ling, J., Tan, W., and Gu, J. G. (2007). TRPM8 mechanism of cold allodynia after chronic nerve injury. J. Neurosci. 27, 13680–13690. doi: 10.1523/jneurosci.2203-07.2007
Xu, H., Ramsey, I. S., Kotecha, S. A., Moran, M. M., Chong, J. A., Lawson, D., et al. (2002). TRPV3 is a calcium-permeable temperature-sensitive cation channel. Nature 418, 181–186. doi: 10.1038/nature00882
Xu, L., Han, Y., Chen, X., Aierken, A., Wen, H., Zheng, W., et al. (2020). Molecular mechanisms underlying menthol binding and activation of TRPM8 ion channel. Nat. Commun. 11:3790. doi: 10.1038/s41467-020-17582-x
Yamamoto, S., Egashira, N., Tsuda, M., and Masuda, S. (2018). Riluzole prevents oxaliplatin-induced cold allodynia via inhibition of overexpression of transient receptor potential melastatin 8 in rats. J. Pharmacol. Sci. 138, 214–217. doi: 10.1016/j.jphs.2018.10.006
Yaman, D., Alpaslan, C., and Kalaycioglu, O. (2021). The effects of Biofreeze and superficial heat on masticatory myofascial pain syndrome. Eur. Oral. Res. 55, 133–138. doi: 10.26650/eor.2021858837
Yosipovitch, G., Szolar, C., Hui, X. Y., and Maibach, H. (1996). Effect of topically applied menthol on thermal, pain and itch sensations and biophysical properties of the skin. Arch. Dermatol. Res. 288, 245–248. doi: 10.1007/bf02530092
Young, M. J., Boulton, A. J., MacLeod, A. F., Williams, D. R., and Sonksen, P. H. (1993). A multicentre study of the prevalence of diabetic peripheral neuropathy in the United Kingdom hospital clinic population. Diabetologia 36, 150–154. doi: 10.1007/bf00400697
Yudin, Y., Lukacs, V., Cao, C., and Rohacs, T. (2011). Decrease in phosphatidylinositol 4,5-bisphosphate levels mediates desensitization of the cold sensor TRPM8 channels. J. Physiol. 589, 6007–6027. doi: 10.1113/jphysiol.2011.220228
Zanotto, K. L., Iodi Carstens, M., and Carstens, E. (2008). Cross-desensitization of responses of rat trigeminal subnucleus caudalis neurons to cinnamaldehyde and menthol. Neurosci. Lett. 430, 29–33. doi: 10.1016/j.neulet.2007.10.008
Zhang, X. B., Jiang, P., Gong, N., Hu, X. L., Fei, D., Xiong, Z. Q., et al. (2008). A-type GABA receptor as a central target of TRPM8 agonist menthol. PLoS One 3:e3386. doi: 10.1371/journal.pone.0003386
Zhang, J., Enix, D., Snyder, B., Giggey, K., and Tepe, R. (2008). Effects of Biofreeze and chiropractic adjustments on acute low back pain: A pilot study. J. Chiropr. Med. 7, 59–65. doi: 10.1016/j.jcme.2008.02.004
Ziemba, P. M., Schreiner, B. S., Flegel, C., Herbrechter, R., Stark, T. D., Hofmann, T., et al. (2015). Activation and modulation of recombinantly expressed serotonin receptor type 3A by terpenes and pungent substances. Biochem. Biophys. Res. Commun. 467, 1090–1096. doi: 10.1016/j.bbrc.2015.09.074
Keywords: menthol, pain, transient receptor potential channel, opioid receptor, analgesia
Citation: Li Z, Zhang H, Wang Y, Li Y, Li Q and Zhang L (2022) The distinctive role of menthol in pain and analgesia: Mechanisms, practices, and advances. Front. Mol. Neurosci. 15:1006908. doi: 10.3389/fnmol.2022.1006908
Received: 29 July 2022; Accepted: 13 September 2022;
Published: 05 October 2022.
Edited by:
Sung Jun Jung, Hanyang University, South KoreaReviewed by:
Sun Wook Hwang, Korea University, South KoreaJoo Min Park, Institute for Basic Science, South Korea
Copyright © 2022 Li, Zhang, Wang, Li, Li and Zhang. This is an open-access article distributed under the terms of the Creative Commons Attribution License (CC BY). The use, distribution or reproduction in other forums is permitted, provided the original author(s) and the copyright owner(s) are credited and that the original publication in this journal is cited, in accordance with accepted academic practice. No use, distribution or reproduction is permitted which does not comply with these terms.
*Correspondence: Linlin Zhang, bGlubGluemhhbmdAdG11LmVkdS5jbg==; Qing Li, YW5lbGl2ZWFAMTI2LmNvbQ==
†These authors have contributed equally to this work