- 1Department of Anesthesiology, The Second Affiliated Hospital of Nanchang University, Nanchang, China
- 2Key Laboratory of Anesthesiology of Jiangxi Province, Nanchang, China
- 3First Clinical Medical College, Nanchang University, Nanchang, China
- 4Department of Anesthesiology, West China Hospital, Sichuan University, Chengdu, China
- 5Department of Anesthesiology, the First Affiliated Hospital of Nanchang University, Nanchang, China
Microglia and astrocytes maintain tissue homeostasis in the nervous system. Both microglia and astrocytes have pro-inflammatory phenotype and anti-inflammatory phenotype. Activated microglia and activated astrocytes can contribute to several neurological diseases. Long non-coding RNAs (lncRNAs) and circular RNAs (circRNAs), two groups of non-coding RNAs (ncRNAs), can function as competing endogenous RNAs (ceRNAs) to impair the microRNA (miRNA) inhibition on targeted messenger RNAs (mRNAs). LncRNAs and circRNAs are involved in various neurological disorders. In this review, we summarized that lncRNAs and circRNAs participate in microglia dysfunction, astrocyte dysfunction, neuron damage, and inflammation. Thereby, lncRNAs and circRNAs can positively or negatively regulate neurological diseases, including spinal cord injury (SCI), traumatic brain injury (TBI), ischemia-reperfusion injury (IRI), stroke, neuropathic pain, epilepsy, Parkinson’s disease (PD), multiple sclerosis (MS), and Alzheimer’s disease (AD). Besides, we also found a lncRNA/circRNA-miRNA-mRNA regulatory network in microglia and astrocyte mediated neurological diseases. Through this review, we hope to cast light on the regulatory mechanisms of lncRNAs and circRNAs in microglia and astrocyte mediated neurological diseases and provide new insights for neurological disease treatment.
Introduction
Both microglia and astrocytes are glial cells in the central nervous system (Kwon and Koh, 2020). Microglia are resident immune cells and the first responders to nervous system changes. Astrocytes can modulate cerebral blood flow (Greenhalgh et al., 2020). And both of them can also promote neurotransmission, synapse formation, and synapse pruning (Vainchtein and Molofsky, 2020). In response to pathological conditions, microglia and astrocytes are activated. Activated microglia and activated astrocytes lose their homeostatic functions and involve in neuroinflammation in the central nervous system (Leng and Edison, 2021). Increasing evidence investigates that the dysfunctions of microglia and astrocytes are beneficial for various neurological diseases, like central nervous system injury and neurodegenerative disorders (Gao et al., 2013; Jha et al., 2019; Pietrowski et al., 2021). Targeting microglia and astrocytes could be a promising approach for the treatment of neurological diseases (Peterson and Binder, 2020; Huang X. et al., 2017).
Long non-coding RNA (lncRNA) and circular RNA (circRNA) are two types of non-coding RNAs (ncRNAs) without the potential to encode proteins (Han T. S. et al., 2020). LncRNAs are transcripts with more than 200 nucleotides in length. LncRNAs include five different subtypes: sense lncRNA, antisense lncRNA, bidirectional lncRNA, intergenic lncRNA, and intronic lncRNA (Braga et al., 2020). LncRNAs regulate gene transcription and translation via interacting with the 3′untranslated region (3′UTR) of messenger RNA (mRNA) (Dykes and Emanueli, 2017). MicroRNAs (miRNAs) are small single-stranded RNAs. MiRNAs recognize miRNA response elements (MREs) on 3′UTR of mRNA and silence target genes through repressing mRNA translation or degrading the mRNA (Cheng et al., 2015; Salim et al., 2021). LncRNAs contain MREs and can use MREs to bind miRNAs. LncRNAs function as competing endogenous RNAs (ceRNAs) to sponge miRNAs and reduce miRNA activity, finally, impair the interaction between miRNAs and their targeted genes (Kalpachidou et al., 2020; Moreno-García et al., 2020). LncRNAs have been demonstrated to involve in epigenetic mediation and the modulation of transcription, translation, RNA metabolism, cell autophagy, cell apoptosis, etc. (Qian et al., 2019). In particular, it is suggested that lncRNAs can influence neural cell behaviors, including neurons, microglia, astrocytes, and oligodendrocytes (Shi et al., 2018). For example, lncRNA AK148321 can abrogate microglial neuroinflammation (Gao et al., 2021). LncRNAs are also related to the development of spinal cord injury (SCI), brain injury, and neurodegenerative diseases (Cuevas-Diaz Duran et al., 2019). CircRNAs have covalently closed loop structures without 5′ caps or 3′ Poly A tails. CircRNAs are stable in eukaryotic cells. CircRNAs can interact with RNA-binding proteins and modulate mRNA stability, and gene transcription. It is proved that endogenous circRNAs can be translated into proteins (Chen and Yang, 2015; Meng et al., 2017). Moreover, like lncRNAs, circRNAs also have MREs. CircRNAs also act as ceRNAs and inhibit miRNA repression on its targeted mRNA (Moreno-García et al., 2020). Many studies report that circRNAs can influence cell proliferation, autophagy, differentiation, and pyroptosis (Fu and Sun, 2021). For instance, one research indicated that circHIPK2 knockout can suppress astrocyte autophagy and astrocyte activation (Huang R. et al., 2017). CircRNAs also play pivotal roles in neurological diseases, including ischemic stroke and cerebral ischemia-reperfusion injury (IRI) (Yang et al., 2018).
In this review, we discussed the effects of both lncRNAs and circRNAs on microglia and astrocyte mediated neurological diseases. We also found a network of lncRNA/circRNA-miRNA-mRNA in neurological diseases. In addition, there are also targeted therapies for the treatment of neurological diseases.
Microglia and Astrocytes in the Nervous System
Microglia
As tissue-resident macrophages derived from progenitors in the embryonic yolk sac, microglia are parenchyma cells and constitute approximately 10% of central nervous system cells (Salter and Stevens, 2017). Under physiological conditions, microglial cells remain in a resting state. During embryonic and postnatal development, microglia can remove apoptotic neurons, support neuronal survival, guide vascularization, and regulate synaptic pruning. In the adult brain, microglial cells are necessary for adult neurogenesis, skill learning, memory formation, and normal behaviors (Kierdorf and Prinz, 2017). Under pathologic conditions, microglial cells undergo the transformation from the resting state to an activated state (Hanisch and Kettenmann, 2007). M1 microglia (classical activation) can release pro-inflammatory factors, such as tumor necrosis factor-α (TNF-α), interleukin-1 (IL-1), IL-6, IL-12, inducible nitric oxide synthase (iNOS). M2 microglia (alternative activation) can produce anti-inflammatory factors, including transforming growth factor-β, IL-4, and IL-10. M1 microglia can promote inflammation and delay tissue repair. While M2 microglia can attenuate inflammation and promote tissue regeneration (Hu et al., 2015). Plenty of works suggested that microglial dysfunction participates in several neurological disorders, including SCI, brain injury, depression, stroke, epilepsy, neuropathic pain, Parkinson’s disease (PD), multiple sclerosis (MS), and Alzheimer’s disease (AD) (Wolf et al., 2017; Araki et al., 2020; Tripathi et al., 2021).
Astrocytes
Astrocytes are the most abundant glial cells within the central nervous system. Astrocytes can interact with brain endothelial cells to form the blood-brain barrier (Abbott et al., 2006). Besides, astrocytes are also essential for synaptic plasticity, memory consolidation, cognitive function, and neuronal survival (Santello et al., 2019). Under pathological conditions (e.g., stroke, infection, ischemia, cancer, etc.), astrocytes become activated (Pekny and Nilsson, 2005). Activated astrocytes can be classified into A1 astrocytes (pro-inflammatory phenotype) and A2 astrocytes (anti-inflammatory phenotype) (Liu L. R. et al., 2020). A2 astrocytes are helpful for neurons and can promote neuron survival and growth. While A1 astrocytes are neurotoxic and stimulate neuron death (Liddelow and Barres, 2017). Activated astrocytes are involved in various neurological diseases, like stroke, epilepsy, SCI, brain injury, and neurodegenerative diseases (Karthikeyan et al., 2016; Nutma et al., 2020).
Long Non-Coding RNAs in Microglia Mediated Neurological Diseases
Spinal Cord Injury
Spinal cord injury is one neurological disease with impairment of sensory and motor function. The SCI pathological process includes primary and secondary injury (Anjum et al., 2020). Primary SCI occurs owing to mechanical damage, for instance, compression or contusion in the spinal cord. Secondary SCI is caused by pathological changes after primary injury, which can result in inflammation, oxidative stress, glial scar formation, neuronal apoptosis, and neuronal necrosis (Anjum et al., 2020; Wang et al., 2020d). Recently, SCI models induced by lipopolysaccharide (LPS) are widely used to explore SCI pathogenesis and find new therapeutic medicine to treat SCI (Xiao et al., 2021).
MiR-382-5p expression is significantly induced by LPS in BV2 cells (mouse microglial cell lines). MiR-382-5p targets 3′UTR of Neuregulin-1 (Nrg1). MiR-382-5p deletion stimulated by its inhibitor can remarkably increase Nrg1 expression in BV2 cells (Xiang et al., 2021). LncRNA Ftx expression is markedly decreased in tissues collected from SCI mice and in LPS-stimulated BV2 cells. Ftx targets miR-382-5p and represses its expression. Besides, Ftx also competes with Nrg1 for miR-382-5p binding, resulting in suppressing miR-382-5p repression on Nrg1 expression (Xiang et al., 2021). Ftx over-expression increases Nrg1 expression levels through miR-382-5p. Increased Nrg1 attenuates the expressions of inflammation factors (e.g., iNOS, IL-6, TNF-α, and IL-1β) in LPS-treated BV2 cells. As a result, increased Ftx relieves microglia inflammation response through the miR-382-5p-Nrg1 axis (Xiang et al., 2021). In addition, this study also indicated that over-expressed Ftx can suppress inflammation response and improve functional recovery in the SCI mouse model (Xiang et al., 2021). Exosomes, which belong to extracellular vesicles (EVs), can be secreted by all cells and carry various materials, including proteins, lipids, mRNA, ncRNAs, etc. And exosomes derived from mesenchymal stem cells possess the potential for SCI treatment (Ren et al., 2020). It is reported that peroxisome proliferator-activated receptor γ (PPARγ) can suppress inflammatory phenotype in macrophages (Nelson et al., 2018). MiR-130b-3p can interact with 3′UTR of PPARγ and reduce its expression. LncGm37494 is reported to be abundant in exosomes originated from hypoxia-treated adipose-derived stem cells (ADSCs). Exosomal lncGm37494 from ADSCs can shift microglia polarization from M1 to M2 via decreasing miR-130b-3p and increasing PPARγ. Finally, exosomal lncGm37494 contributes to improving functional recovery following SCI in mice (Shao et al., 2020). In the SCI rat model, receptor-activity modifying proteins 3 (RAMP3) is up-regulated and miR-222-5p is decreased. MiR-222-5p can target RAMP3 3′UTR and inhibit RAMP3 expression. Enhanced RAMP3 expression or miR-222-5p inhibitor can promote cell apoptosis and increase TNF-α and IL-6 expressions in LPS-treated microglia (Cui et al., 2021). LncRNA lymphoid enhancer-binding factor 1 (LEF1) antisense RNA 1 (LEF1-AS1) is up-regulated in LPS-treated microglia cells that are collected from the central nervous system of normal rats. LEF1-AS1 acts as a ceRNA via sponging miR-222-5p. LEF1-AS1 small interfering RNA (siRNA) can augment miR-222-5p expression. Knocking down LEF1-AS1 dampens microglia apoptosis and promotes microglial cell viability through the miR-222-5p-RAMP3 axis. LEF1-AS1 silence can impede SCI progression in rats (Cui et al., 2021). Pyroptosis is a type of programmed cell death involved in neuroinflammation (Dai et al., 2019). And it is proved that inhibiting microglial pyroptosis can promote motor function recovery following SCI (Xu S. et al., 2020). F630028O10Rik levels are higher in the peripheral blood from SCI patients compared with normal people. F630028O10Rik can sponge miR-1231-5p to up-regulate Col1a1. Subsequently, increased Colla1 can enhance microglial pyroptosis through the activation of phosphoinositide 3-kinase (PI3K)/protein kinase B (AKT) pathway. Increased F630028O10Rik expression can indicate worse functional recovery after SCI (Xu S. et al., 2020). Smad ubiquitination regulatory factor 1 (Smurf1) is a downstream target of miR-27a and its expression can be attenuated by miR-27a. Smurf1 over-expression or miR-27a down-regulation can promote TNF-α and IL-6 secretion in LPS-treated microglia (Zhao Q. et al., 2020). LncRNA X-inactive specific transcript (XIST) expression is elevated in LPS-treated microglial cells and in injured rat spinal cord tissues. XIST sponges miR-27a and negatively mediates miR-27a expression. XIST deletion abrogates SCI-evoked microglia apoptosis and inflammatory injury through the miR-27a up-regulation and Smurf1 down-regulation. Moreover, the deficiency of XIST can relieve SCI (Zhao Q. et al., 2020). The expression of lncRNA metastasis-associated lung adenocarcinoma transcript 1 (MALAT1) is higher in SCI rats compared to normal rats. Over-expressed MALAT1 activates the IkappaB kinase β (IKKβ)/nuclear factor kappa B (NF-κB) signaling pathway by down-regulating miR-199b. Eventually, MALAT1 over-expression promotes pro-inflammatory cytokine production in LPS-treated microglial cells (Zhou et al., 2018). Zhou et al. (2018) also revealed that MALAT1 silence can alleviate SCI through inhibiting microglial inflammatory responses.
Traumatic Brain Injury
Traumatic brain injury (TBI) is recognized as a primary reason for high morbidity and high disability around the world (Jamjoom et al., 2021). The primary injury of TBI occurs directly in response to mechanical forces, like contusion, hemorrhage, hypoxia, etc. The primary injury can trigger the secondary injury that relates to several biochemical alterations, such as inflammation, nerve cell damage, and cerebral edema, contributing to exacerbating TBI progression (Alam et al., 2020; Gasco et al., 2021). Tumor necrosis factor receptor-related factor 6 (TRAF6) is a target of miR-873-5p. LncRNA KCNQ1 overlapping transcript 1 (KCNQ1OT1) is up-regulated in the cerebral lesions of TBI mice. KCNQ1OT1 down-regulation can increase miR-873-5p expression. Reducing KCNQ1OT1 blocks microglial inflammation through the miR-873-5p-TRAF6 axis (Liu N. et al., 2021). Liu N. et al. (2021) revealed that KCNQ1OT1 depletion can mitigate TBI-induced injuries, including neurological deficits, neuron loss, brain edema, and blood-brain barrier damage (Liu N. et al., 2021). Many studies revealed that inhibiting microglial activation and neuroinflammation can hamper TBI development (Kumar et al., 2017). The E3 ubiquitin ligase Nrdp1 can interact with myeloid differentiation factor-88 adaptor protein (MYD88) and decrease MYD88 protein levels (Cheng et al., 2021). LncRNA HOX antisense intergenic RNA (HOTAIR) is up-regulated in the cerebral cortex of TBI mice and in LPS-treated BV2 cells. HOTAIR up-regulation attenuates the interaction between Nrdp1 and MYD88, thus, to increase MYD88 protein expression. HOTAIR knockout enhances Nrdp1-mediated the down-regulation of MYD88 to suppress microglial activation and microglia inflammatory factor (e.g., TNF-α, IL-1β, and IL-6) release, which can ultimately repress TBI (Cheng et al., 2021). Nod-like receptor protein 3 (NLRP3) is a target of miR-7a-5p. NLRP3 absence decreases iNOS expression, nitric oxide (NO) production, and inflammatory cytokine (e.g., IL-1β, IL-6, and TNF-α) expression (Meng et al., 2021). LncRNA maternally expressed gene 3 (MEG3) is over-expressed in the TBI cell model (LPS + ATP induced microglial cells that are obtained from normal mice). MEG3 targets miR-7a-5p and reduces its expression. MEG3 also functions as a ceRNA for miR-7a-5p and represses miR-7a-5p inhibition on NLRP3. Functionally, MEG3 up-regulation enhances microglial activation and inflammation through the miR-7a-5p-NLRP3 axis in the TBI in vitro model (Meng et al., 2021).
Ischemia-Reperfusion Injury
More and more evidence revealed that recovery of blood flow in a short time after cerebral ischemia can lead to cerebral IRI (Pan et al., 2007). IRI gives rise to reactive oxygen species (ROS), calcium overload, neuron death, platelet activation, and inflammation, which can ultimately cause irreversible damage to the brain (Wu et al., 2010; Bai and Lyden, 2015). In research studies, middle cerebral artery occlusion (MCAO)/reperfusion (MCAO/R) treatment in vivo and oxygen-glucose deprivation (OGD)/reoxygenation (OGD/R) treatment in vitro are used to mimic cerebral IRI (Zheng et al., 2021). It is reported that up-regulated Krüppel-like factor 4 (KLF4) decreases M1 marker (CD86 and iNOS), TNF-α, and IL-1β levels, while increases M2 marker (CD206 and Arg1), IL-10, and IL-4 expressions in OGD/R-induced BV2 cells (Li T. et al., 2020). MEG3 is elevated in the brain tissues from MCAO/R mice and in OGD/R-induced BV2 cells. MEG3 binds to KLF4 and represses its protein progression. MEG3 absence enhances M2 polarization and reduces M1 polarization in microglial cells by targeting KLF4. Eventually, MEG3 knockdown decreases neuroinflammation to lessen cerebral IRI (Li T. et al., 2020).
Long non-coding RNA small nucleolar RNA host gene 4 (SNHG4) is remarkably down-regulated in blood and cerebrospinal fluid samples obtained from patients with acute cerebral infarction. Enhanced SNHG4 expression can facilitate microglia M2 polarization and promote anti-inflammatory factor release (Zhang S. et al., 2020). MiR-449c-5p can bind with the 3′UTR of signal transducers and activators of transcription 6 (STAT6). MiR-449c-5p inhibitor increases STAT6 expression. SNHG4 targets miR-449c-5p and inhibits its expression. Up-regulated SNHG4 activates the STAT6 signaling pathway by suppressing miR-449c-5p. Therefore, SNHG4 up-regulation attenuates microglial inflammatory responses and microglia-mediated neuronal damage during cerebral IRI (Zhang S. et al., 2020). LncRNA SNHG3 is elevated in OGD/R-stimulated BV2 cells. Interference with SNHG3 impedes microglial activation and dampens the release of pro-inflammatory factors (TNF-α and IL-6) through abrogating histone deacetylase 3 (HDAC3) expression (Huang D. et al., 2021). In transient MCAO mice, SNHG3 deletion weakens cerebral IRI (Huang D. et al., 2021). Diabetes mellitus (DM) is characterized by high sugar in the blood and can worsen cerebral IRI (Wang and Zhou, 2018). MALAT1 is highly expressed in cortical tissues of diabetic rats suffering the IRI and in HAPI cells (rat immortalized microglia cell line) undergoing high glucose and hypoxia-reoxygenation. Over-expressed MALAT1 enhances microglia inflammatory response through activating the MyD88/IRAK1 (IL-1 receptor-associated kinase 1)/TRAF6 signaling pathway. Finally, increased MALAT1 aggravates cerebral IRI in diabetic rats (Wang and Zhou, 2018). LncRNA nuclear enriched abundant transcript 1 (NEAT1) expresses highly in the blood of ischemic stroke patients and in OGD/R-induced BV2 cells (Ni et al., 2020). One research reported that NEAT1 can serve as a ceRNA for miR-224 and thus enhance IL-33 production and promote M2a polarization in macrophages. NEAT1 can also hinder A1 astrocyte activation (Liu D. et al., 2021). Ni et al. (2020) found that suppressing NEAT1 abrogates microglial M1 polarization to reduce neuronal apoptosis after cerebral IRI.
Stroke
Stroke is a severe disorder with high rates of mortality and disability around the world. Stroke can be divided into ischemic stroke (major type) and hemorrhagic stroke (Xu et al., 2021). Ischemic stroke is mainly due to atherosclerosis in large vessels and atherosclerotic plaque ruptures. MCAO models in rodents and OGD in vitro models are usually used for experimental ischemic stroke researches (Sommer, 2017).
Malate dehydrogenase 2 (MDH2) binds to the mRNA of neutrophil chemoattractant C-X-C motif ligand 2 (CXCL2) and reduces CXCL2 mRNA levels (Chen J. et al., 2021). LncRNA U90926 is highly increased in microglia isolated from the ischemic hemispheres in C57BL/6J mice. U90926 interacts with MDH2 to impair the interaction between MDH2 and CXCL2 mRNA. Increased microglial U90926 enhances CXCL2 protein levels. Increased CXCL2 release from microglia can ultimately stimulate neutrophil infiltration, which can exacerbate brain injury during ischemic stroke. Once microglial U90926 is silenced, the neurological function is improved and infarct volume is diminished in ischemic stroke mice (Chen J. et al., 2021). LncRNA opa-interacting protein 5 antisense RNA 1 (OIP5-AS1) lowly expresses in the blood of stroke patients. It is shown that OIP5-AS1 down-regulation increases miR-186-5p levels (Chen Y. et al., 2021). Over-expressed miR-186-5p leads to microglia inflammation and oxidative stress via blocking the expression of C1q/tumor necrosis factor-related protein 3 (CTRP3), resulting in neuron damage during ischemic stroke. Further, OIP5-AS1 up-regulation lessens infarct volume and weakens neuron apoptosis and loss in rats with ischemic stroke (Chen Y. et al., 2021). One research demonstrated that lincRNA-EPS can be loaded in leukosomes (biomimetic vesicles). And these leukosomes carrying lincRNA-EPS can target the ischemic area and co-localize with microglia in ischemic stroke mice (Zhang B. et al., 2020). LincRNA-EPS from leukosomes impedes microglia activation and microglia infiltration in vivo. In addition, leukosomes-loaded lincRNA-EPS can also accelerate neuron regeneration via hindering microglia inflammation in cerebral infarction (Zhang B. et al., 2020). It is said that sirtuin1 activation can abolish NF-κB signaling (Kauppinen et al., 2013). MiR-425-5p can target sirtuin1. In brain tissues of MCAO mice, SNHG8 expression is down-regulated. MiR-425-5p can share binding sites with SNHG8 and sirtuin1. SNHG8 functions as a ceRNA for miR-425-5p and inhibits miR-425-5p effects on sirtuin1 (Tian et al., 2021). SNHG8 over-expression can attenuate miR-425-5p to increase sirtuin1 expression and suppress NF-κB phosphorylation. SNHG8 amplification suppresses microglial activation and inflammation through the miR-425-5p/sirtuin1/NF-κB pathway, resulting in diminishing brain microvascular endothelial cell (BMEC) damage. Furthermore, after ischemic stroke, over-expressed SNHG8 dampens neuron damage, cerebral edema, and neurological function loss in mice (Tian et al., 2021). H19 levels are significantly raised in the plasma isolated from stroke patients compared to normal people. Repressing H19 reduces HDAC1 to facilitate microglial polarization from M1 to M2. H19 inhibition promotes cerebral IL-10 production and decreases cerebral TNF-α and IL-1β levels in ischemic mice. Therefore, H19 reduction can inhibit neuroinflammation in ischemic stroke (Wang et al., 2017). In ischemic stroke mice, H19 siRNA decreases cerebral ischemic injury and attenuates neurological deficits. Moreover, H19 would be a promising biomarker to diagnose ischemic stroke (Wang et al., 2017). LncRNA Nespas is greatly expressed in ischemic cerebral tissues of MCAO mice and in BV2 cells subjected to OGD. High Nespas expression hinders transforming growth factor-beta-activated kinase 1 (TAK1) activation via abolishing the interaction between tripartite motif 8 (TRIM8) and TAK1 (Deng et al., 2019). Over-expressed Nespas limits NF-κB activation through TAK1, which can restrain microglia death and neuroinflammation in the ischemic microenvironment. Moreover, Nespas silence in MCAO mice deteriorates ischemic brain injury (Deng et al., 2019). LncRNA 1810034E14Rik declines markedly in microglial cells after the OGD exposure. Elevated 1810034E14Rik expression impedes microglia activation and microglia inflammation in vitro and in vivo (Zhang et al., 2019b). In addition, up-regulated 1810034E14Rik can abrogate neuronal damage caused by OGD-treated microglia. Further, enhanced 1810034E14Rik expression promotes motor function recovery and decreases infarct volumes in MCAO mice (Zhang et al., 2019b). SNHG14 and cytosolic phospholipase A2 (cPLA2) group IVA (PLA2G4A) are notably up-regulated in ischemic cerebral tissues and in OGD-treated BV2 cells. MiR-145-5p is down-regulated in MACO mice. PLA2G4A is a downstream target of miR-145-5p. The miR-145-5p inhibitor can increase TNF-α, and nitrate levels in OGD-treated BV2 cells (Qi et al., 2017). SNHG14 elevates PLA2G4A expression and abrogates miR-145-5p expression. Functionally, SNHG14 can facilitate microglia activation and microglia-mediated neuron apoptosis through the miR-145-5p-PLA2G4A axis in response to ischemic stroke conditions. Further, highly expressed SNHG14 can act as an induction effector of cerebral infarction severity (Qi et al., 2017). MiR-96-5p abrogates NF-κB signaling and TNF-α production via suppressing IKKβ expression in OGD-stimulated BV2 cells (Zhang M. et al., 2021). LncRNA XIST is highly expressed in BV2 cells exposed to OGD treatment. XIST serves as a sponge for miR-96-5p to antagonize inhibitory effects of miR-96-5p on the TNF-α expression. XIST up-regulation increases TNF-α expression through the miR-96-5p-IKKβ-NF-κB axis (Zhang M. et al., 2021). Moreover, TNF-α stimulation can increase XIST mRNA levels in BV2 cells. The XIST-TNF-α feedback enhances the pro-inflammatory polarization of microglial cells, leading to exacerbating cerebral neuron apoptosis in cerebral infarction (Zhang M. et al., 2021).
Neuropathic Pain
Accumulating evidence reveals that neuropathic pain develops owing to the diseases or damaging stimuli in the nervous system. Neuropathic pain includes postherpetic neuralgia, trigeminal neuralgia, and nerve injury pain. Patients with neuropathic pain usually suffer from allodynia and hyperalgesia (Jensen and Finnerup, 2014). It is investigated that enhancer of zeste homolog 2 (EZH2), one RNA-binding protein of Lncenc1, can promote histone methylation in the promoter of brain-specific angiogenesis inhibitor 1 (BAI1) and decrease BAI1 expression (Zhang Z. et al., 2021). After nerve injury, lncRNA embryonic stem cells expressed 1 (Lncenc1) is notably increased in dorsal root ganglion in mice. Increased Lncenc1 enhances EZH2 expression to down-regulate BAI1, which can accelerate microglia activation and microglial inflammatory response. Decreasing Lncenc1 can impede neuropathic pain development in rats (Zhang Z. et al., 2021).
Epilepsy
Epilepsy is a neurological disorder characterized by a persistent predisposition to produce epileptic seizures (Fisher et al., 2014). Epilepsy includes focal epilepsy, generalized epilepsy, combined generalized and focal epilepsy, and unknown epilepsy (the patient has epilepsy but the clinician cannot determine if the epilepsy is focal epilepsy or generalized epilepsy) (Scheffer et al., 2017). Temporal lobe epilepsy is the most common form of focal epilepsy. And patients with temporal lobe epilepsy usually experience hippocampal sclerosis (Lévesque and Avoli, 2013). Epilepsy can be caused by genetic mutations, stroke, TBI, infection, cancers, etc. Although there are many anti-seizure drugs, approximately one-third of patients can’t achieve seizure control (Devinsky et al., 2018). Recently, rodents with spontaneous seizures that mimic human epilepsy can be used to study epilepsy. For example, the kainic acid (KA)-induced epilepsy model is widely used to explore pathogenetic mechanisms of temporal lobe epilepsy (Lévesque and Avoli, 2013). H19 expression is notably higher in the hippocampus in epileptic rats compared to normal rats (Han C. L. et al., 2020). Han C. L. et al. (2018) showed that H19 can stimulate hippocampal microglia and astrocyte activation through the Janus kinase (JAK)/STAT3 signaling pathway in a KA-induced rat model of temporal lobe epilepsy. Another research investigated that H19 can bind to miRNA let-7b and restrain its expression. Increased H19 promotes STAT3 mRNA expression levels via sponging let-7b. Subsequently, up-regulated STAT3 results in microglia and astrocyte activation during epileptogenesis. Eventually, the activation of both microglia and astrocytes can aggravate epileptic seizures in rats undergoing temporal lobe epilepsy induced by KA (Han C. L. et al., 2020). Feng et al. (2020) revealed that up-regulated 26S proteasome non-ATPase regulatory subunit 11 (Psmd11) can not only inactivate microglia and astrocytes but also abrogate neuron injury and inflammation in the epileptic hippocampus in mice. In addition, Psmd11 is a downstream target of miR-490-3p (Feng et al., 2020). LncRNA Peg13 is lowly expressed in epileptic hippocampal tissues from mice. Peg13 acts as a ceRNA for miR-490-3p. Peg13 competitively binds with miR-490-3p to elevate Psmd11 expression. Ultimately, in epileptic mice, high Peg13 expression suppresses epilepsy progression (Feng et al., 2020).
Depression
Depression is a mood disorder affecting human beings at any age. Depressed people usually experience persistent sadness, decreased interest, loss of pleasure, diminished or increased sleep, etc. Childhood maltreatment (e.g., abuse, loss, and neglect) and stressful life can increase the risk of depression (Malhi and Mann, 2018). It is indicated that 2007 lncRNAs were differentially expressed in peripheral blood of major depressive disorder (MDD) patients compared to that in normal people, including 1,556 up-regulated lncRNAs and 441 down-regulated lncRNAs (Liu et al., 2014). LncRNAs related to cognitive function, synaptic plasticity, and psychiatry diseases (e.g., schizophrenia) potentially contribute to MDD (Huang X. et al., 2017). LncRNA uc.80- is down-regulated in the hippocampus obtained from depressed rats induced by chronic unpredictable mild stress (CUMS) regiment. Over-expressed uc.80- inhibits microglia M1 polarization and facilitates microglia M2 polarization. In addition, uc.80- up-regulation can attenuate neuron apoptosis mediated by microglia under inflammation exposure. Functionally, uc.80- over-expression ameliorates depression via enhancing M2 polarization of microglial cells in CUMS-treated rats (Gu et al., 2020).
Parkinson’s Disease
Parkinson’s disease, the second most common neurodegenerative disorder after AD, is characterized by the loss of dopaminergic neurons within the substantia nigra pars compacta (Jankovic and Tan, 2020). The clinical features in PD patients include classical motor symptoms, cognitive impairment, pain, psychiatric symptoms, etc. (Kalia and Lang, 2015). LncRNA HOXA11-AS is detected to be up-regulated in the substantia nigra area in PD mice. HOXA11-AS over-expression can elevate the levels of inflammatory factors, including, IL-1β, IL-18, IL-6, and TNF-α in LPS-mediated BV2 cells (Cao et al., 2021). HOXA11-AS can target miR-124-3p that can target follistatin-like 1 (FSTL1). Down-regulated HOXA11-AS enhances miR-124-3p expression to inhibit FSTL1 and NF-κB expressions. The inactivation of the FSTL1/NF-κB pathway can dampen neuron damage and inhibit microglial inflammation. Ultimately, HOXA11-AS down-regulation mitigates PD progression in PD mice (Cao et al., 2021). MALAT1 highly expresses in 1-Methyl-4-phenyl-1,2,3,6-tetrahydropyridine (MPTP)-induced PD mouse brain tissues and in and in LPS/ATP-induced BV2 cells. MALAT1 can recruit the EZH2 to the promoter of nuclear factor (erythroid-derived 2)-like-2 factor (NRF2) and then inhibit NRF2 expression. Repressing NRF2 decreases IL-1β, IL-18, and TNF-α in vitro (Cai et al., 2020b). MALAT1 deficiency can abrogate inflammasome activation and ROS generation through NRF2 in microglia. The MALAT1 elimination in PD mice blocks neuron damage by reducing microglia inflammation (Cai et al., 2020b). LincRNA-p21 is shown to be induced by p53 in LPS-treated BV2 cells. LincRNA-p21 acts as a ceRNA for the miR-181 family (miR-181a, miR-181b, miR-181c, and miR-181d), thus protecting protein kinase C δ (PKC-δ) from miR-181 family-regulated suppression. Increased lincRNA-p21 can augment PKC-δ by competitively binding with the miR-181 family (Ye et al., 2018). In turn, increased PKC-δ augments the levels of p53 and lincRNA-p21. The lincRNA-p21 mediated regulatory loop with miR-181 family and PKC-δ can contribute to microglia activation and inflammation. Besides, increased lincRNA-p21 also accelerates neuron degeneration caused by microglia inflammation in PD mice (Ye et al., 2018). The expression levels of SNHG1 are increased in LPS-induced BV2 cells and in brain tissues in PD patients. SNHG1 is a ceRNA for miR-7. Knocking down SNHG1 can increase miR-7 to down-regulate NLRP3 expression. Then decreased NLRP3 reduces microglial activation and inflammation. Eventually, SNHG1 knockdown attenuates neuronal apoptosis in PD mice (Cao et al., 2018). It is shown that miR-223-3p can target NLRP3 to impair its expression. Over-expressed miR-223-3p can impede NLRP3 inflammasome activation and decrease inflammatory cytokine expressions, including IL-1β, IL-6, and TNF-α (Xu W. et al., 2020). LncRNA growth arrest-specific 5 (GAS5) is significantly over-expressed in PD mice. GAS5 can repress miR-223-3p expression and abrogates miR-223-3p suppression on NLRP3. Elevated GAS5 can trigger microglia inflammation via the miR-223-3p-NLRP3 axis. Silencing GAS5 can hamper behavioral impairments in the PD mouse model (Xu W. et al., 2020).
Multiple Sclerosis
Multiple sclerosis is a chronic inflammatory disease in the central nervous system. The MS pathological process consists of multifocal inflammation, demyelination, reactive gliosis, and axonal degeneration (Baecher-Allan et al., 2018). GAS5 is significantly increased in microglia from experimental autoimmune encephalomyelitis (EAE) mice (an animal MS model). Up-regulated GAS5 attenuates the expression of interferon-regulatory factor 4 (IRF4) via binding with EZH2, which can impede microglia M2 polarization. Besides, enhanced microglial GAS5 can exacerbate myelin loss in EAE mice (Sun et al., 2017).
Long Non-Coding RNAs in Astrocyte Mediated Neurological Diseases
Spinal Cord Injury
Long non-coding RNA SNHG5 expression is increased in the astrocytes and microglia isolated from SCI rats. High SNHG5 expression elevates KLF4 expression. Enhanced SNHG5 expression improves cell viability and hampers apoptosis through KLF4 in astrocytes and microglia. In SCI rats, SNHG5 up-regulation promotes SCI progression (Jiang and Zhang, 2018).
Traumatic Brain Injury
Extracellular vesicles are cargo-carrying vesicles that can affect cell communication (Teng and Fussenegger, 2020). MiR-195 can target nucleotide-binding leucine-rich repeat containing family member X1 (NLRX1). LncRNA nuclear transcription factor NF-κB interacting lncRNA (NKILA) can be transferred from astrocytes into neurons through EVs. NKILA functions as a ceRNA for miR-195. NKILA competitively binds to miR-195 and counteracts miR-195 inhibitory effect on NLRX1 (He et al., 2021). NKILA from astrocyte-derived EVs diminishes miR-195 to augment NLRX1 expression. Subsequently, increased NLRX1 enhances proliferation and declines apoptosis in injured neurons. Moreover, in TBI mice, NKILA-enriched EVs from astrocytes accelerate brain recovery (He et al., 2021). It is reported that astrocyte swelling is one of the early consequences of TBI-induced brain edema. When astrocyte swelling occurs, the astrocyte volume is increased (Zhang et al., 2019d). MALAT1 expression is decreased in the edematous brain after TBI. Over-expressed MALAT1 abrogates astrocyte swelling through attenuating aquaporin 4 (AQP4), NF-κB, and IL-6 expressions in response to trauma. This study suggests the negative relationship between MALAT1 and TBI-related brain edema (Zhang et al., 2019d).
Ischemia-Reperfusion Injury
Metastasis-associated lung adenocarcinoma transcript 1 is up-regulated in OGD/R-treated MA-C cells (astrocyte cells). AQP4 is a downstream target of miR-145. MALAT1 suppression can reduce AQP4 via stimulating miR-145 expression. AQP4 silence attenuates astrocyte damage under ischemia-reperfusion conditions in vitro (Wang et al., 2020c). In MACO/R mice, MALAT1 deficiency decreases cell apoptosis and alleviates neurological deficits. This research revealed that MALAT1 inhibition can attenuate cerebral IRI through the miR-145-AQP4 axis (Wang et al., 2020c). LncRNA taurine upregulated gene 1 (TUG1) is highly expressed in OGD/R-treated MA-C cells. MiR-142-3p binds to TUG1 3′UTR. TUG1 is a miRNA sponge of miR-142-3p (Li et al., 2021). TUG1 down-regulation increases astrocyte cell viability and hinders astrocyte cell apoptosis via increasing miR-142-3p expression. This study revealed a positive relationship between TUG1 and cerebral IRI (Li et al., 2021).
Neuropathic Pain
It is investigated that SNHG5 levels are remarkably increased in mouse spinal cord after spinal nerve ligation (SNL). In SNL mice, miR-154-5p elevation restrains CXCL13 protein levels, and inhibiting SNHG5 augments miR-154-5p levels (Chen M. et al., 2020). Knocking down SNHG5 dampens astrocyte activation and microglia activation via the miR-154-5p-CXCL13 axis. Repressing astrocyte activation and microglia activation can lessen neuropathic pain in SNL mice. This study revealed that SNHG5 deficiency alleviates neuropathic pain through the miR-154-5p-CXCL13 axis (Chen M. et al., 2020).
Epilepsy
It is said that adenosine deficiency is a hallmark of epilepsy. And therapeutic adenosine augmentation can be a promising approach for epileptic seizures (Boison, 2012). LncRNA cancer susceptibility candidate 2 (CASC2) is lowly expressed in the hippocampus in epileptic rats. Elevated CASC2 hinders astrocyte activation and restrains astrocyte adenosine metabolism via triggering phosphatase and tensin homolog (PTEN) expression during epilepsy. Increased CASC2 can also repress epilepsy seizures in epileptic rats (Zhu et al., 2020). LncRNA urothelial cancer-associated 1 (UCA1) expression is down-regulated in the hippocampus of temporal lobe epilepsy rats. UCA1 over-expression abrogates astrocyte activation and suppresses the expression of astrocyte glutamate aspartate transporter (GLAST) (one factor related to epilepsy) in vivo. And UCA1 up-regulation reduces the frequency of epilepsy seizures and promotes learning and memory in temporal lobe epilepsy rats (Wang et al., 2020b). Yu et al. (2020) revealed that up-regulated UCA1 can enhance myocyte enhancer factor 2C (MEF2C) expression in IL-1β-treated CTX-TNA2 (the epilepsy cell models). MEF2C declines inflammatory effector [e.g., IL-6, TNF-α, and cyclooxygenase 2 (COX-2)] expression via blocking NF-κB signal in IL-1β-treated astrocytes. Increased UCA1 ultimately attenuates astrocyte inflammation through MEF2C/NF-κB pathway, which can hinder epilepsy progression (Yu et al., 2020). It is indicated that miR-129-5p can diminish Notch1 mRNA levels. LncRNA NEAT1 expression levels are elevated in the temporal lobe tissues in epilepsy patients compared to normal people. NEAT1 can target miR-129-5p and inhibit its expression (Wan and Yang, 2020). Increased NEAT1 activates the Notch1 signaling pathway by suppressing miR-129-5p. Finally, NEAT1 up-regulation can enhance astrocyte inflammatory responses in IL-1β-induced epilepsy cell models (Wan and Yang, 2020). In the brain, the brain-derived neurotrophic factor (BDNF) is essential for plasticity, neuronal survival, dendritic branching, etc. (Lima Giacobbo et al., 2019). LncRNA plasmacytoma variant translocation 1 (PVT1) is elevated in hippocampus tissues collected from epileptic rats. PVT1 down-regulation attenuates the Wnt signaling pathway, which can boost BDNF expression, impede neuronal loss, repress astrocyte activation, and decrease pro-inflammatory cytokine (e.g., TNF-α, IL-1β, and IL-6) expressions. PVT1 silence in epileptic rats also promotes spatial memory and learning (Zhao et al., 2019).
Depression
Postpartum depression (PPD) remains one of the most common complications caused by childbirth. Stress and adverse life events can increase the risk of PPD (Payne and Maguire, 2019). The hormone-simulated pregnancy-induced PPD mouse model is used to explore the cellular mechanisms of PPD. It is indicated that oxytocin receptors play protective roles in PPD (Zhu and Tang, 2020). LncRNA Gm14205 is noticed to be up-regulated in the hippocampus in PPD mice. Up-regulated Gm14205 decreases oxytocin receptors, resulting in activating the NLRP3 inflammasome in hippocampal astrocytes. This study suggests a positive relationship between Gm14205 and PPD progression (Zhu and Tang, 2020).
Multiple Sclerosis
Long non-coding RNA Gm13568 expression is stimulated in astrocytes after IL-9 treatment. Gm13568 interacts with CREB-binding protein (CBP)/P300 to facilitate the expression and activation of Notch1 (Liu X. et al., 2021). The activated Notch1/STAT3 pathway contributes to the secretion of inflammatory cytokines (e.g., IL-6, TNF-α, and interferon-inducible protein-10) in activated astrocytes. As a result, Gm13568 promotes astrocyte inflammation to worsen the EAE process (Liu X. et al., 2021).
Alzheimer’s Disease
Alzheimer’s disease, a frequent neurodegenerative disorder, is the leading cause of dementia among elderly individuals. AD patients usually suffer from memory impairment, executive dysfunction, language problems, etc. (Scheltens et al., 2016). MEG3 expression is declined in the hippocampus tissues in AD rats. Up-regulated MEG3 suppresses astrocyte activation and neuronal damage via abrogating PI3K/AKT signaling pathway. Moreover, increased MEG3 can relieve cognitive impairment in AD rats (Yi et al., 2019).
Circular RNAs in Microglia and Astrocyte Mediated Neurological Diseases
Spinal Cord Injury
It is reported that treating astrocytes with TNF-α in vitro can induce the inflammatory response after SCI. Elevated miR-488 attenuates inflammatory cytokine secretion in TNF-α-treated astrocytes. Chemokine CC motif ligand 2 (CCL2) is a downstream target of miR-488 (Chen et al., 2022). CircPrkcsh is up-regulated in mouse injured spinal cords and in TNF-α-treated astrocytes. CircPrkcsh serves as a miRNA sponge for miR-488. Silencing circPrkcsh decreases CCL2 expression via miR-488 up-regulation (Chen et al., 2022). Chen et al. (2022) revealed that circPrkcsh knockout represses inflammatory response after SCI through the miR-488-CCL2 axis.
Ischemia-Reperfusion Injury
Neural stem cells (NSCs) can differentiate into neurons and astrocytes. The NSCs are important for brain tissue regeneration and tissue repair after injury (Gage and Temple, 2013). The expression of circRNA TTC3 (circTTC3) is up-regulated in MCAO/R mice. CircTTC3 can sponge miR-372-3p that targets Toll-like receptor 4 (TLR4). Depleting circTTC3 suppresses OGD-induced astrocyte injury and promotes NSC proliferation and differentiation via the miR-372-3p-TLR4 axis. The circTTC3 knockdown in MCAO/R mice can alleviate cerebral IRI (Yang et al., 2021).
Stroke
The expression levels of circHECTD1 are over-expressed in the plasma collected from acute ischemic stroke patients. TCDD inducible poly (ADP-ribose) polymerase (TIPARP) is a downstream target of miR-142. CircHECTD1 can bind to miR-142 and function as a miR-142 sponge to inhibit miR-142 repression on TIPARP. Down-regulating circHECTD1 eliminates TIPARP through miR-142 (Han B. et al., 2018). Knockdown of circHECTD1 can attenuate astrocyte autophagy via the miR-142-TIPARP axis, resulting in blocking astrocyte activation. Decreased circHECTD1 can lessen cerebral ischemic stroke injury in MCAO mice (Han B. et al., 2018). This research also revealed that circHECTD1 could be a promising biomarker for acute ischemic stroke diagnoses (Han B. et al., 2018). CircSHOC2 is found to be abundant in exosomes derived from ischemic-preconditioned astrocytes. MiR-7670-3p can bind to sirtuin 1 (SIRT1) 3′UTR. CircSHOC2 serves as a miRNA sponge for miR-7670-3p and attenuates miR-7670-3p inhibitory effects on SIRT1 expression (Chen W. et al., 2020). Exosomal circSHOC2 from astrocytes can abrogate neuron apoptosis and neuron damage through the miR-7670-3p-SIRT1 axis. Finally, exosomal circSHOC2 ameliorates ischemic stroke-associated brain injuries (Chen W. et al., 2020).
Epilepsy
CircHivep2 is shown to be notably diminished in KA-treated BV2 cells and in hippocampus tissues of epilepsy mice. Suppressor of cytokine signaling-2 (SOCS2) is a target of miR-181a-5p. CircHivep2 binds to and sponges miR-181a-5p. CircHivep2 can decline miR-181a-5p to increase SOCS2 in KA-treated BV2 cells (Xiaoying et al., 2020). CircHivep2 elevation can attenuate microglia activation and microglia inflammation via the miR-181a-5p-SOCS2 axis. Over-expressed circHivep2 can ameliorate epileptic seizures in epilepsy mice (Xiaoying et al., 2020). CircIgf1r is up-regulated in the brain tissues in mice with status epilepticus. Inhibiting circIgf1r drives astrocyte A2 polarization via promoting autophagy. Therefore, circIgf1r inhibition reduces astrocyte-associated neuronal toxicity in the epilepsy cell model. Moreover, circIgf1r deficiency in epilepsy mice can impede neuronal damage and dampen epileptic discharge (Shao et al., 2021).
Depression
CircSTAG1 is significantly declined in the plasma and whole blood in depressed patients. In astrocytes, over-expressed circSTAG1 increases the demethylase alkB homolog 5 (ALKBH5) levels in the cell cytoplasm and reduces ALKBH5 translocation into the cell nucleus (Huang et al., 2020). Thus, circSTAG1 over-expression facilitates the methylation of fatty acid amide hydrolase (FAAH) mRNA and inhibits FAAH expression. Increased circSTAG1 ultimately hampers astrocyte dysfunction and thereby hinders depressive-like behaviors in mice with MDD (Huang et al., 2020). In the plasma and hippocampi of depressed mice, circHIPK2 expression levels are increased (Zhang et al., 2019c). One study indicated that circHIPK2 deficiency can inhibit astrocyte activation via reducing autophagy (Huang R. et al., 2017). Zhang et al. revealed that circHIPK2 expression can be attenuated by the transplantation of the NLRP3-deficient gut microbiota in depressed mice. Suppressing circHIPK2 can block astrocyte dysfunction and therefore reduce depression in mice (Zhang et al., 2019c). It is shown that increased HECT domain E3 ubiquitin protein ligase 1 (HECTD1) can reduce 90-kDa heat shock protein (HSP90) expression via ubiquitination. MiR-9 can bind with the 3′UTR of HECTD1 and repress HECTD1 expression (Zhang Y. et al., 2020). CircRNA DYM (circDYM) expression is down-regulated in the peripheral blood in patients with MDD CircDYM functions as a molecular sponge to interfere with miR-9 activity, leading to increased HECTD1. CircDYM over-expression inhibits HSP90 expression through the miR-9-HECTD1 axis, which can reduce microglial activation. Therefore, increased circDYM restrains depression in depressed mice (Zhang Y. et al., 2020).
Alzheimer’s Disease
The circNF1-419 expression levels are up-regulated in senescent astrocytes. Up-regulated circNF1-419 promotes autophagy in astrocytes in vitro (Diling et al., 2019). In AD mice, astroglial circNF1-419 over-expression enhances autophagy via binding to Dynamin-1 and Adaptor protein 2 B1 proteins. Eventually, circNF1-419 up-regulation leads to repressing senile dementia in AD mice (Diling et al., 2019).
Targeted Therapy
Long non-coding RNAs and circular RNAs participate in various neurological diseases, suggesting their potential to act as therapeutic targets. Bexarotene, which is a selective agonist of retinoid X receptor, can suppress TBI progression in mice (Zhong et al., 2017a). Bexarotene can stimulate NEAT1 expression to inhibit neuron apoptosis and microglial inflammation, resulting in promoting motor and cognitive function recovery in TBI mice (Zhong et al., 2017b). Curcumin, an anti-inflammatory agent, can be delivered into microglial cells via exosomes. Exosomal curcumin can repress LPS-induced brain inflammation and myelin oligodendrocyte glycoprotein (MOG)-induced EAE development in mice (Zhuang et al., 2011). Moreover, one research suggested that curcumin can increase GAS5 expression to alleviate post-stroke depression in rats (Cai et al., 2020a). Berberine belongs to traditional Chinese medicine. Berberine can elevate circHDAC9 expression in human neurons. Elevated circHDAC9 protects human neuron cells from 42-residue β-amyloid (Aβ42)-triggered neuronal damage via sponging miR-142-5p (Zhang N. et al., 2020). As mentioned above, targeting lncRNAs and circRNAs will be a promising strategy for the treatment of neurological diseases.
Dexmedetomidine
Dexmedetomidine (DEX), an anesthetic adjuvant, has various advantages in clinical applications, including hemodynamic stability, the ability to promote postoperative recognition, sedative and analgesic effects (Zhao Y. et al., 2020). DEX reduces high-mobility group box 1 (HMGB1) via eliminating SNHG14, resulting in attenuating microglial activation. Subsequently, DEX restrains neurological deficits induced by IRI in vivo (Ta Na et al., 2020). Deng et al. indicated that DEX can increase lncRNA LOC102546895 levels in hippocampus tissues in rats undergoing postoperative cognitive dysfunction (POCD). LOC102546895 promotes the expression of Neuronal Per-Arnt-Sim domain protein 4 (Npas4) (one protective neuronal transcription factor), impedes proliferation, and enhances apoptosis in microglial cells (Deng et al., 2020). DEX treatment hampers inflammatory response and neuron injury in the hippocampus. DEX can also ameliorate cognitive deficits in POCD rats (Deng et al., 2020).
Valproate
Valproate is an eight-carbon branched-chain fatty acid and possesses anticonvulsant properties. Valproate is approved for the management of epilepsy, bipolar disorders, and migraine (Löscher, 1999). Li et al. reported that valproate can decline lncRNA RMRP levels to activate PI3K/AKT pathway, which can promote proliferation and repress apoptosis in microglial cells in the ischemic stroke cell model (Li and Sui, 2020). In MACO rats, valproate treatment represses ischemic stroke-induced disorders, including cell apoptosis and cerebral infarction (Li and Sui, 2020).
Isosteviol Sodium
It is said that isosteviol sodium has neuroprotective effects and can block brain damage after ischemic stroke (Rösing et al., 2020). GAS5 over-expression diminishes miR-146a-5p to increase Notch1 expression, leading to promoting microglial M1 polarization and reducing microglial M2 polarization (Zhang et al., 2019a). Isosteviol sodium increases miR-146a-5p expression and declines the expression of GAS5 and Notch1. Functionally, isosteviol sodium enhances microglia M2 polarization through the GAS5-miR-146a-5p-Notch1 axis, which can ultimately attenuate brain injury caused by ischemic stroke in mice (Zhang et al., 2019a).
Sulfasalazine
Sulfasalazine is a prodrug used for the treatment of autoimmune diseases, including rheumatoid arthritis and ulcerative colitis (Choi and Fenando, 2021). HOTAIR serves as a molecular sponge for miR-136-5p to promote AKT2 expression. Down-regulated HOTAIR suppresses the AKT2-NF-κB axis via increasing miR-136-5p (Duan et al., 2018). Sulfasalazine can decrease HOTAIR expression by inhibiting c-Myc in microglia. Sulfasalazine represses the microglial M1-like phenotype through the HOTAIR-miR-136-5p-AKT2-NF-κB axis, contributing to suppressing demyelination (Duan et al., 2018).
Discussion and Conclusion
Microglia and astrocytes are glial cells that maintain tissue homeostasis in the nervous system. Accumulating evidence reports that both microglia and astrocytes have pro-inflammatory phenotype (M1/A1) and anti-inflammatory phenotype (M2/A2). Activated microglia and activated astrocytes can lead to inflammation, and the inflammation can contribute to microglial injuries, astrocyte injuries, and neuron damages. Inflammatory conditions also accelerate disease progression. LncRNAs and circRNAs are two ncRNAs involved in neural cell behaviors and nerve system development. For instance, up-regulated H19 increases CCL2 expression via sponging miR-1-3p, which can enhance astrocyte proliferation (Li P. et al., 2020). Knockdown of circHIPK2 can enhance NSC differentiation into neurons and promote neuronal plasticity (Wang et al., 2020a). LncRNA BDNF-antisense (BDNF-AS) is increased in the human amygdala of alcohol use disorder patients who started to drink before 21 years old. Up-regulated BDNF-AS reduces BDNF signaling, resulting in inhibiting synaptic plasticity in the amygdala (Bohnsack et al., 2019). Xu K. et al. (2020) proved that circGRIA1 deficiency contributes to the improvement of synaptogenesis and synaptic plasticity. LncRNA AtLAS is down-regulated in the dorsal medial prefrontal cortex in dominant mice. AtLAS can increase synapsin 2b (syn2b) expression. Syn2b binds with a-amino-3-hydroxy-5-methyl-4-isoxazolepropionic acid receptors (AMPAR) to restrain AMPAR membrane expression. Therefore, syn2b reduces AMPAR-correlated synaptic transmission. Decreased AtLAS contributes to enhanced synaptic strength in dominant mice (Ma et al., 2020).
Here, we found that lncRNAs and circRNAs can display positive or negative roles in microglia (Table 1) and astrocyte (Table 2) mediated neurological diseases. And the regulatory mechanisms of lncRNAs and circRNAs are related to microglia dysfunction, astrocyte dysfunction, neuron damage, and inflammation. It is widely indicated that both lncRNAs and circRNAs can serve as ceRNAs to inhibit miRNA activity. In this review, the lncRNA/circRNA-miRNA-mRNA regulatory network also exists in microglia and astrocyte mediated neurological diseases, including SCI (Figure 1; Shao et al., 2020; Xu S. et al., 2020; Zhao Q. et al., 2020; Cui et al., 2021; Xiang et al., 2021; Chen et al., 2022), TBI (He et al., 2021; Liu N. et al., 2021; Meng et al., 2021), cerebral IRI (Figure 1; Wang et al., 2020c; Zhang S. et al., 2020; Yang et al., 2021), stroke (Figure 1; Qi et al., 2017; Han B. et al., 2018; Chen W. et al., 2020; Chen Y. et al., 2021; Tian et al., 2021; Zhang M. et al., 2021), epilepsy (Figure 1; Feng et al., 2020; Han C. L. et al., 2020; Wan and Yang, 2020; Xiaoying et al., 2020), neuropathic pain (Figure 1; Chen M. et al., 2020), PD (Figure 1; Cao et al., 2018, 2021; Xu W. et al., 2020), and depression (Figure 1; Zhang Y. et al., 2020). We also found that some therapies can impair the ncRNA-glial cell axis and thus inhibit disease development. DEX can suppress SNHG14 to attenuate microglial activation, thus, to repress IRI (Ta Na et al., 2020). Valproate can decline RMRP to decrease microglia injuries. Isosteviol sodium promotes microglia M2 polarization via suppressing GAS5. Valproate and isosteviol sodium eventually hinder ischemic stroke (Zhang et al., 2019a; Li and Sui, 2020). Sulfasalazine reduces HOTAIR expression and therefore represses the microglial M1-like phenotype, which can finally suppress demyelination (Duan et al., 2018). These results suggest a promising treatment strategy based on the ncRNA-glial cell axis for neurological diseases. In this review, we collected evidence to reveal the relationships between lncRNAs/circRNAs and neurological diseases. We hope that this review will provide new sights for more clinical therapies, thereby allowing us to treat neurological diseases more effectively.
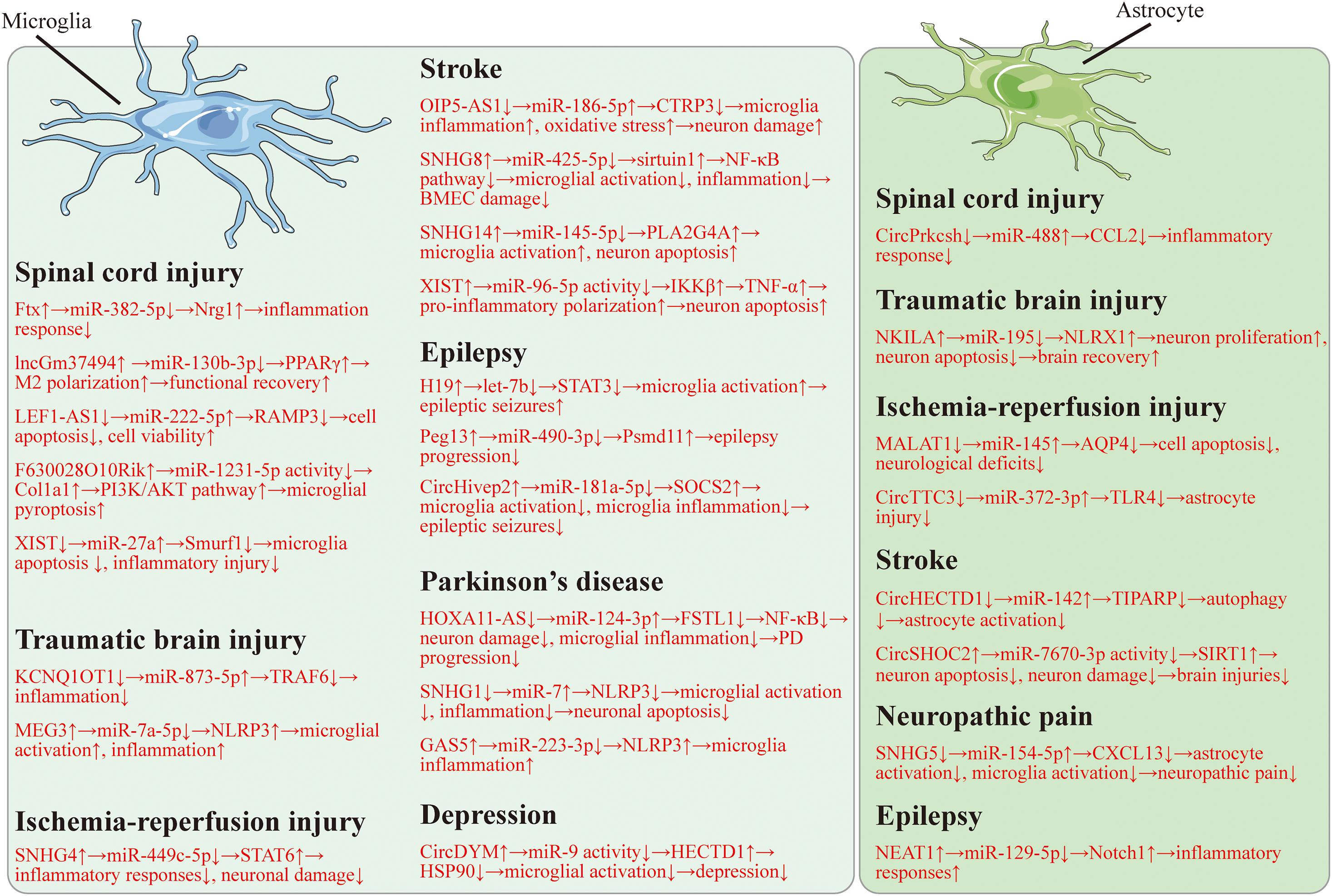
Figure 1. The lncRNA/circRNA-miRNA-mRNA regulatory network in microglia and astrocyte regulated neurological diseases.
Recently, increasing evidence reveals that lncRNAs and circRNAs can be transferred into recipient cells via EVs and control disease development. For example, astrocytes can transfer lncRNAs and circRNAs to neurons through EVs and repress neuron injuries, leading to alleviating nervous system damage (Figure 2; Chen W. et al., 2020; He et al., 2021). Shao et al. (2020) found that exosomal lncGm37494 from ADSCs stimulates microglia M2 polarization to enhance functional recovery after SCI. One study reported that intranasally delivered lincRNA-Cox2-siRNA loaded EVs can suppress microglia proliferation upon LPS treatment in mice (Liao et al., 2020). And Zhang et al. investigated that lincRNA-EPS can be loaded in biomimetic vesicles targeting microglia. And lincRNA-EPS-loaded biomimetic vesicles abolish microglial inflammation to promote neuron regeneration in vivo (Zhang B. et al., 2020). These suggest that EV-loaded ncRNAs targeted glial cells could be promising therapeutic therapies for the treatment of neurological disorders.
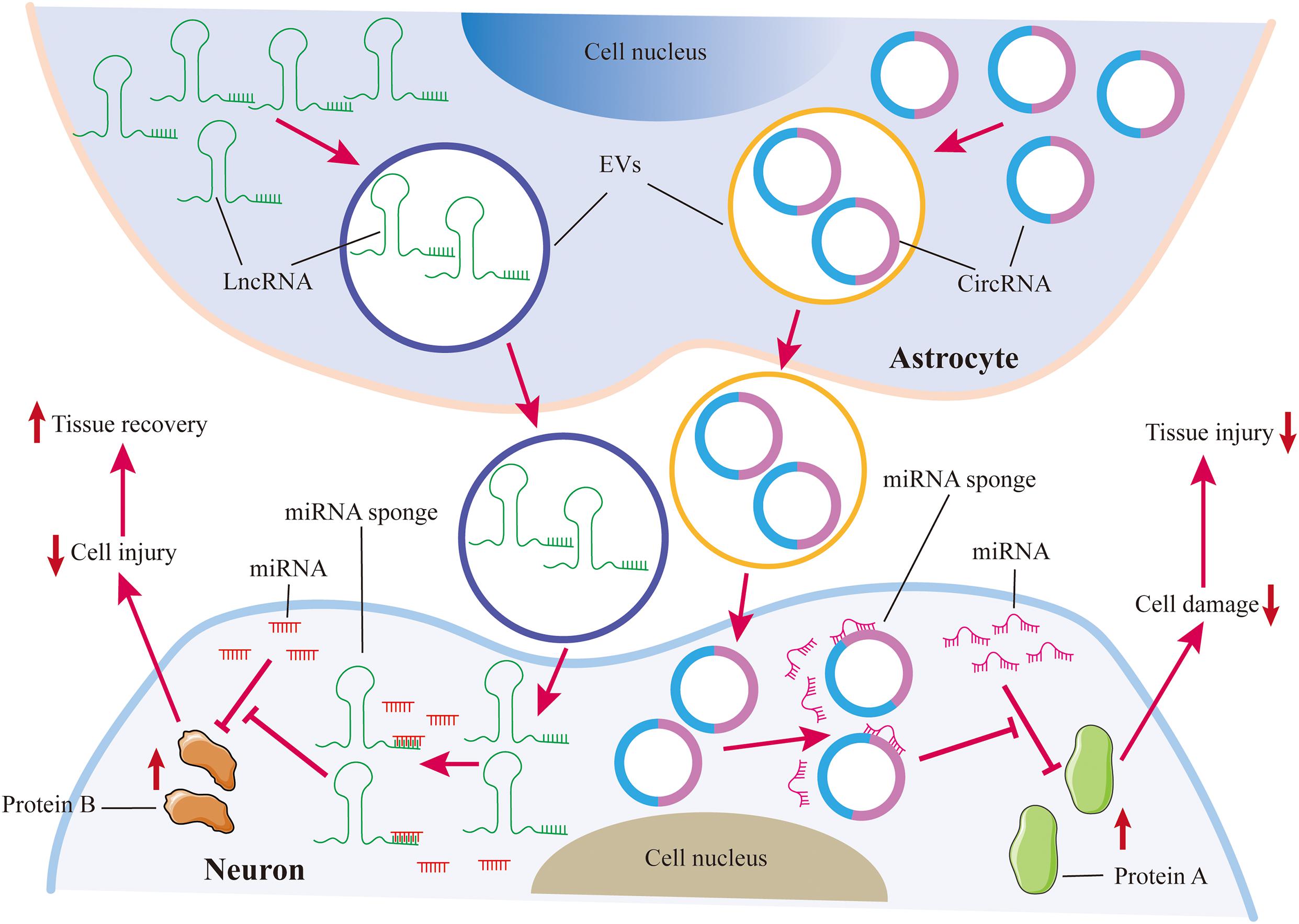
Figure 2. Astrocytes protect neurons against injuries via EVs. Astrocytes transfer lncRNAs and circRNAs to neurons through EVs. Both lncRNAs and circRNAs can act as miRNA sponges to inhibit miRNA repression on target proteins, which can restrain neuron damage. LncRNAs and circRNAs from astrocyte-derived EVs are beneficial for tissue repair in the nervous system.
Presently, despite the great progress that has been made in neurodegeneration, the researches based on the ncRNA-glial cell axis are still lacking. It is widely suggested that LPS-induced neuroinflammation can mimic the microenvironment in neurodegenerative diseases (Hu et al., 2018). LPS-treated models may be useful to explore the pathological mechanism in neurodegeneration.
Author Contributions
All authors contributed to the study conception and design, read, and agreed to the published version of the manuscript.
Funding
This research was supported by grants from the National Natural Science Foundation of China (81760261 and 82060219), Provincial Science Foundation of Jiangxi (20192BCB23024 and 20202BABL206016), and Youth Team Project of the Second Affiliated Hospital of Nanchang University (2019YNTD12003).
Conflict of Interest
The authors declare that the research was conducted in the absence of any commercial or financial relationships that could be construed as a potential conflict of interest.
Publisher’s Note
All claims expressed in this article are solely those of the authors and do not necessarily represent those of their affiliated organizations, or those of the publisher, the editors and the reviewers. Any product that may be evaluated in this article, or claim that may be made by its manufacturer, is not guaranteed or endorsed by the publisher.
References
Abbott, N. J., Rönnbäck, L., and Hansson, E. (2006). Astrocyte-endothelial interactions at the blood-brain barrier. Nat. Rev. Neurosci. 7, 41–53. doi: 10.1038/nrn1824
Alam, A., Thelin, E. P., Tajsic, T., Khan, D. Z., Khellaf, A., Patani, R., et al. (2020). Cellular infiltration in traumatic brain injury. J. Neuroinflamm. 17:328. doi: 10.1186/s12974-020-02005-x
Anjum, A., Yazid, M. D., Fauzi Daud, M., Idris, J., Ng, A. M. H., Selvi Naicker, A., et al. (2020). Spinal cord injury: pathophysiology, multimolecular interactions, and underlying recovery mechanisms. Int. J. Mol. Sci. 21:7533. doi: 10.3390/ijms21207533
Araki, T., Ikegaya, Y., and Koyama, R. (2020). The effects of microglia- and astrocyte-derived factors on neurogenesis in health and disease. Eur. J. Neurosci. 54, 5880–5901. doi: 10.1111/ejn.14969
Baecher-Allan, C., Kaskow, B. J., and Weiner, H. L. (2018). Multiple sclerosis: mechanisms and immunotherapy. Neuron 97, 742–768. doi: 10.1016/j.neuron.2018.01.021
Bai, J., and Lyden, P. D. (2015). Revisiting cerebral postischemic reperfusion injury: new insights in understanding reperfusion failure, hemorrhage, and edema. Int. J. Stroke 10, 143–152. doi: 10.1111/ijs.12434
Bohnsack, J. P., Teppen, T., Kyzar, E. J., Dzitoyeva, S., and Pandey, S. C. (2019). The lncRNA BDNF-AS is an epigenetic regulator in the human amygdala in early onset alcohol use disorders. Transl. Psychiatry 9:34. doi: 10.1038/s41398-019-0367-z
Braga, E. A., Fridman, M. V., Moscovtsev, A. A., Filippova, E. A., Dmitriev, A. A., and Kushlinskii, N. E. (2020). LncRNAs in ovarian cancer progression, metastasis, and main pathways: ceRNA and alternative mechanisms. Int. J. Mol. Sci. 21:8855. doi: 10.3390/ijms21228855
Cai, L. J., Tu, L., Huang, X. M., Huang, J., Qiu, N., Xie, G. H., et al. (2020b). LncRNA MALAT1 facilitates inflammasome activation via epigenetic suppression of Nrf2 in Parkinson’s disease. Mol. Brain 13:130. doi: 10.1186/s13041-020-00656-658
Cai, L. J., Li, W. T., Zhang, L. L., Lu, X. Q., Chen, M., and Liu, Y. (2020a). Long noncoding RNA GAS5 enhanced by curcumin relieves poststroke depression by targeting miR-10b/BDNF in rats. J. Biol. Regul. Homeost. Agents 34, 815–823. doi: 10.23812/20-113-a-25
Cao, B., Wang, T., Qu, Q., Kang, T., and Yang, Q. (2018). Long noncoding RNA SNHG1 promotes neuroinflammation in Parkinson’s disease via regulating miR-7/NLRP3 pathway. Neuroscience 388, 118–127. doi: 10.1016/j.neuroscience.2018.07.019
Cao, H., Han, X., Jia, Y., and Zhang, B. (2021). Inhibition of long non-coding RNA HOXA11-AS against neuroinflammation in Parkinson’s disease model via targeting miR-124-3p mediated FSTL1/NF-κB axis. Aging 13, 11455–11469. doi: 10.18632/aging.202837
Chen, J., Jin, J., Zhang, X., Yu, H., Zhu, X., Yu, L., et al. (2021). Microglial lnc-U90926 facilitates neutrophil infiltration in ischemic stroke via MDH2/CXCL2 axis. Mol. Ther. [Epub ahead of print]. doi: 10.1016/j.ymthe.2021.04.025
Chen, J. N., Zhang, Y. N., Tian, L. G., Zhang, Y., Li, X. Y., and Ning, B. (2022). Down-regulating Circular RNA Prkcsh suppresses the inflammatory response after spinal cord injury. Neural Regen. Res. 17, 144–151. doi: 10.4103/1673-5374.314114
Chen, L. L., and Yang, L. (2015). Regulation of circRNA biogenesis. RNA Biol. 12, 381–388. doi: 10.1080/15476286.2015.1020271
Chen, M., Yang, Y., Zhang, W., Li, X., Wu, J., Zou, X., et al. (2020). Long noncoding RNA SNHG5 knockdown alleviates neuropathic pain by targeting the miR-154-5p/CXCL13 axis. Neurochem. Res. 45, 1566–1575. doi: 10.1007/s11064-020-03021-2
Chen, W., Wang, H., Zhu, Z., Feng, J., and Chen, L. (2020). Exosome-Shuttled circSHOC2 from IPASs Regulates Neuronal Autophagy and Ameliorates Ischemic Brain Injury via the miR-7670-3p/SIRT1 Axis. Mol. Ther. Nucleic Acids 22, 657–672. doi: 10.1016/j.omtn.2020.09.027
Chen, Y., Liu, W., Chen, M., Sun, Q., Chen, H., and Li, Y. (2021). Up-regulating lncRNA OIP5-AS1 protects neuron injury against cerebral hypoxia-ischemia induced inflammation and oxidative stress in microglia/macrophage through activating CTRP3 via sponging miR-186-5p. Int. Immunopharmacol. 92:107339. doi: 10.1016/j.intimp.2020.107339
Cheng, D. L., Xiang, Y. Y., Ji, L. J., and Lu, X. J. (2015). Competing endogenous RNA interplay in cancer: mechanism, methodology, and perspectives. Tumour Biol. 36, 479–488. doi: 10.1007/s13277-015-3093-z
Cheng, S., Zhang, Y., Chen, S., and Zhou, Y. (2021). LncRNA HOTAIR participates in microglia activation and inflammatory factor release by regulating the ubiquitination of MYD88 in traumatic brain injury. J. Mol. Neurosci. 71, 169–177. doi: 10.1007/s12031-020-01623-7
Choi, J., and Fenando, A. (2021). Sulfasalazine. Treasure Island, FL: StatPearls Publishing Copyright.
Cuevas-Diaz Duran, R., Wei, H., Kim, D. H., and Wu, J. Q. (2019). Invited review: long non-coding RNAs: important regulators in the development, function and disorders of the central nervous system. Neuropathol. Appl. Neurobiol. 45, 538–556. doi: 10.1111/nan.12541
Cui, S. Y., Zhang, W., Cui, Z. M., Yi, H., Xu, D. W., Liu, W., et al. (2021). Knockdown of long non-coding RNA LEF1-AS1 attenuates apoptosis and inflammatory injury of microglia cells following spinal cord injury. J. Orthop. Surg. Res. 16:6. doi: 10.1186/s13018-020-02041-6
Dai, W., Wang, X., Teng, H., Li, C., Wang, B., and Wang, J. (2019). Celastrol inhibits microglial pyroptosis and attenuates inflammatory reaction in acute spinal cord injury rats. Int. Immunopharmacol. 66, 215–223. doi: 10.1016/j.intimp.2018.11.029
Deng, F., Cai, L., Zhou, B., Zhou, Z., and Xu, G. (2020). Whole transcriptome sequencing reveals dexmedetomidine-improves postoperative cognitive dysfunction in rats via modulating lncRNA. 3 Biotech 10:202. doi: 10.1007/s13205-020-02190-9
Deng, Y., Chen, D., Wang, L., Gao, F., Jin, B., Lv, H., et al. (2019). Silencing of long noncoding RNA nespas aggravates microglial cell death and neuroinflammation in ischemic stroke. Stroke 50, 1850–1858. doi: 10.1161/strokeaha.118.023376
Devinsky, O., Vezzani, A., O’Brien, T. J., Jette, N., Scheffer, I. E., de Curtis, M., et al. (2018). Epilepsy. Nat. Rev. Dis. Primers 4:18024. doi: 10.1038/nrdp.2018.24
Diling, C., Yinrui, G., Longkai, Q., Xiaocui, T., Yadi, L., Xin, Y., et al. (2019). Circular RNA NF1-419 enhances autophagy to ameliorate senile dementia by binding Dynamin-1 and Adaptor protein 2 B1 in AD-like mice. Aging 11, 12002–12031. doi: 10.18632/aging.102529
Duan, C., Liu, Y., Li, Y., Chen, H., Liu, X., Chen, X., et al. (2018). Sulfasalazine alters microglia phenotype by competing endogenous RNA effect of miR-136-5p and long non-coding RNA HOTAIR in cuprizone-induced demyelination. Biochem. Pharmacol. 155, 110–123. doi: 10.1016/j.bcp.2018.06.028
Dykes, I. M., and Emanueli, C. (2017). Transcriptional and post-transcriptional gene regulation by long non-coding RNA. Genom. Proteom. Bioinform. 15, 177–186. doi: 10.1016/j.gpb.2016.12.005
Feng, H., Gui, Q., Zhu, W., Wu, G., Dong, X., Shen, M., et al. (2020). Long-noncoding RNA Peg13 alleviates epilepsy progression in mice via the miR-490-3p/Psmd11 axis to inactivate the Wnt/β-catenin pathway. Am. J. Transl. Res. 12, 7968–7981.
Fisher, R. S., Acevedo, C., Arzimanoglou, A., Bogacz, A., Cross, J. H., Elger, C. E., et al. (2014). ILAE official report: a practical clinical definition of epilepsy. Epilepsia 55, 475–482. doi: 10.1111/epi.12550
Fu, Y., and Sun, H. (2021). Biogenesis, cellular effects, and biomarker value of circHIPK3. Cancer Cell Int. 21:256. doi: 10.1186/s12935-021-01956-2
Gage, F. H., and Temple, S. (2013). Neural stem cells: generating and regenerating the brain. Neuron 80, 588–601. doi: 10.1016/j.neuron.2013.10.037
Gao, S., Cheng, Q. C., Hu, Y. G., Tan, Z. Z., Chen, L., Liu, S. W., et al. (2021). LncRNA AK148321 alleviates neuroinflammation in LPS-stimulated BV2 microglial cell through regulating microRNA-1199-5p/HSPA5 axis. Life Sci. 266:118863. doi: 10.1016/j.lfs.2020.118863
Gao, Z., Zhu, Q., Zhang, Y., Zhao, Y., Cai, L., Shields, C. B., et al. (2013). Reciprocal modulation between microglia and astrocyte in reactive gliosis following the CNS injury. Mol. Neurobiol. 48, 690–701. doi: 10.1007/s12035-013-8460-4
Gasco, V., Cambria, V., Bioletto, F., Ghigo, E., and Grottoli, S. (2021). Traumatic brain injury as frequent cause of hypopituitarism and growth hormone deficiency: epidemiology, diagnosis, and treatment. Front. Endocrinol. 12:634415. doi: 10.3389/fendo.2021.634415
Greenhalgh, A. D., David, S., and Bennett, F. C. (2020). Immune cell regulation of glia during CNS injury and disease. Nat. Rev. Neurosci. 21, 139–152. doi: 10.1038/s41583-020-0263-9
Gu, X. H., Xu, L. J., Zheng, L. L., Yang, Y. J., Tang, Z. Y., Wu, H. J., et al. (2020). Long non-coding RNA uc.80- overexpression promotes M2 polarization of microglias to ameliorate depression in rats. IUBMB Life 72, 2194–2203. doi: 10.1002/iub.2353
Han, B., Zhang, Y., Zhang, Y., Bai, Y., Chen, X., Huang, R., et al. (2018). Novel insight into circular RNA HECTD1 in astrocyte activation via autophagy by targeting MIR142-TIPARP: implications for cerebral ischemic stroke. Autophagy 14, 1164–1184. doi: 10.1080/15548627.2018.1458173
Han, C. L., Ge, M., Liu, Y. P., Zhao, X. M., Wang, K. L., Chen, N., et al. (2018). LncRNA H19 contributes to hippocampal glial cell activation via JAK/STAT signaling in a rat model of temporal lobe epilepsy. J. Neuroinflammation 15:103. doi: 10.1186/s12974-018-1139-z
Han, C. L., Liu, Y. P., Guo, C. J., Du, T. T., Jiang, Y., Wang, K. L., et al. (2020). The lncRNA H19 binding to let-7b promotes hippocampal glial cell activation and epileptic seizures by targeting Stat3 in a rat model of temporal lobe epilepsy. Cell Prolif. 53:e12856. doi: 10.1111/cpr.12856
Han, T. S., Hur, K., Cho, H. S., and Ban, H. S. (2020). Epigenetic associations between lncRNA/circRNA and miRNA in hepatocellular carcinoma. Cancers 12:2622. doi: 10.3390/cancers12092622
Hanisch, U. K., and Kettenmann, H. (2007). Microglia: active sensor and versatile effector cells in the normal and pathologic brain. Nat. Neurosci. 10, 1387–1394. doi: 10.1038/nn1997
He, B., Chen, W., Zeng, J., Tong, W., and Zheng, P. (2021). Long noncoding RNA NKILA transferred by astrocyte-derived extracellular vesicles protects against neuronal injury by upregulating NLRX1 through binding to mir-195 in traumatic brain injury. Aging 13, 8127–8145. doi: 10.18632/aging.202618
Hu, G., Liao, K., Niu, F., Yang, L., Dallon, B. W., Callen, S., et al. (2018). Astrocyte EV-induced lincRNA-Cox2 regulates microglial phagocytosis: implications for morphine-mediated neurodegeneration. Mol. Ther. Nucleic Acids 13, 450–463. doi: 10.1016/j.omtn.2018.09.019
Hu, X., Leak, R. K., Shi, Y., Suenaga, J., Gao, Y., Zheng, P., et al. (2015). Microglial and macrophage polarization—new prospects for brain repair. Nat. Rev. Neurol. 11, 56–64. doi: 10.1038/nrneurol.2014.207
Huang, D., Cao, Y., Zu, T., and Ju, J. (2021). Interference with long noncoding RNA SNHG3 alleviates cerebral ischemia-reperfusion injury by inhibiting microglial activation. J. Leukoc. Biol. [Epub ahead of print]. doi: 10.1002/jlb.1a0421-190r
Huang, R., Zhang, Y., Bai, Y., Han, B., Ju, M., Chen, B., et al. (2020). N(6)-methyladenosine modification of fatty acid amide hydrolase messenger RNA in circular RNA STAG1-regulated astrocyte dysfunction and depressive-like behaviors. Biol. Psychiatry 88, 392–404. doi: 10.1016/j.biopsych.2020.02.018
Huang, R., Zhang, Y., Han, B., Bai, Y., Zhou, R., Gan, G., et al. (2017). Circular RNA HIPK2 regulates astrocyte activation via cooperation of autophagy and ER stress by targeting MIR124-2HG. Autophagy 13, 1722–1741. doi: 10.1080/15548627.2017.1356975
Huang, X., Luo, Y. L., Mao, Y. S., and Ji, J. L. (2017). The link between long noncoding RNAs and depression. Prog. Neuropsychopharmacol. Biol. Psychiatry 73, 73–78. doi: 10.1016/j.pnpbp.2016.06.004
Huang, X., You, W., Zhu, Y., Xu, K., Yang, X., and Wen, L. (2021). Microglia: a potential drug target for traumatic axonal injury. Neural Plast. 2021:5554824. doi: 10.1155/2021/5554824
Jamjoom, A. A. B., Rhodes, J., Andrews, P. J. D., and Grant, S. G. N. (2021). The synapse in traumatic brain injury. Brain 144, 18–31. doi: 10.1093/brain/awaa321
Jankovic, J., and Tan, E. K. (2020). Parkinson’s disease: etiopathogenesis and treatment. J. Neurol. Neurosurg. Psychiatry 91, 795–808. doi: 10.1136/jnnp-2019-322338
Jensen, T. S., and Finnerup, N. B. (2014). Allodynia and hyperalgesia in neuropathic pain: clinical manifestations and mechanisms. Lancet Neurol. 13, 924–935. doi: 10.1016/s1474-4422(14)70102-4
Jha, M. K., Jo, M., Kim, J. H., and Suk, K. (2019). Microglia-astrocyte crosstalk: an intimate molecular conversation. Neuroscientist 25, 227–240. doi: 10.1177/1073858418783959
Jiang, Z. S., and Zhang, J. R. (2018). LncRNA SNHG5 enhances astrocytes and microglia viability via upregulating KLF4 in spinal cord injury. Int. J. Biol. Macromol. 120(Pt A), 66–72. doi: 10.1016/j.ijbiomac.2018.08.002
Kalia, L. V., and Lang, A. E. (2015). Parkinson’s disease. Lancet 386, 896–912. doi: 10.1016/s0140-6736(14)61393-3
Kalpachidou, T., Kummer, K. K., and Kress, M. (2020). Non-coding RNAs in neuropathic pain. Neuronal Signal. 4:Ns20190099. doi: 10.1042/ns20190099
Karthikeyan, A., Patnala, R., Jadhav, S. P., Eng-Ang, L., and Dheen, S. T. (2016). MicroRNAs: key players in microglia and astrocyte mediated inflammation in CNS pathologies. Curr. Med. Chem. 23, 3528–3546. doi: 10.2174/0929867323666160814001040
Kauppinen, A., Suuronen, T., Ojala, J., Kaarniranta, K., and Salminen, A. (2013). Antagonistic crosstalk between NF-κB and SIRT1 in the regulation of inflammation and metabolic disorders. Cell. Signal. 25, 1939–1948. doi: 10.1016/j.cellsig.2013.06.007
Kierdorf, K., and Prinz, M. (2017). Microglia in steady state. J. Clin. Invest. 127, 3201–3209. doi: 10.1172/jci90602
Kumar, A., Stoica, B. A., Loane, D. J., Yang, M., Abulwerdi, G., Khan, N., et al. (2017). Microglial-derived microparticles mediate neuroinflammation after traumatic brain injury. J. Neuroinflam. 14:47. doi: 10.1186/s12974-017-0819-4
Kwon, H. S., and Koh, S. H. (2020). Neuroinflammation in neurodegenerative disorders: the roles of microglia and astrocytes. Transl. Neurodegener. 9:42. doi: 10.1186/s40035-020-00221-2
Leng, F., and Edison, P. (2021). Neuroinflammation and microglial activation in Alzheimer disease: where do we go from here? Nat. Rev. Neurol. 17, 157–172. doi: 10.1038/s41582-020-00435-y
Lévesque, M., and Avoli, M. (2013). The kainic acid model of temporal lobe epilepsy. Neurosci. Biobehav. Rev. 37(10 Pt 2), 2887–2899. doi: 10.1016/j.neubiorev.2013.10.011
Li, L., Zhang, Q., Wang, Y., Yin, S., Chi, S., Han, F., et al. (2021). Knockdown of lncRNA TUG1 attenuates cerebral ischemia/reperfusion injury through regulating miR-142-3p. Biofactors [Epub ahead of print]. doi: 10.1002/biof.1765
Li, P., Li, Y., Dai, Y., Wang, B., Li, L., Jiang, B., et al. (2020). The LncRNA H19/miR-1-3p/CCL2 axis modulates lipopolysaccharide (LPS) stimulation-induced normal human astrocyte proliferation and activation. Cytokine 131:155106. doi: 10.1016/j.cyto.2020.155106
Li, T., Luo, Y., Zhang, P., Guo, S., Sun, H., Yan, D., et al. (2020). LncRNA MEG3 regulates microglial polarization through KLF4 to affect cerebral ischemia-reperfusion injury. J. Appl. Physiol. 129, 1460–1467. doi: 10.1152/japplphysiol.00433.2020
Li, X., and Sui, Y. (2020). Valproate improves middle cerebral artery occlusion-induced ischemic cerebral disorders in mice and oxygen-glucose deprivation-induced injuries in microglia by modulating RMRP/PI3K/Akt axis. Brain Res. 1747, 147039. doi: 10.1016/j.brainres.2020.147039
Liao, K., Niu, F., Dagur, R. S., He, M., Tian, C., and Hu, G. (2020). Intranasal delivery of lincRNA-Cox2 siRNA loaded extracellular vesicles decreases lipopolysaccharide-induced microglial proliferation in mice. J. Neuroimmune Pharmacol. 15, 390–399. doi: 10.1007/s11481-019-09864-z
Liddelow, S. A., and Barres, B. A. (2017). Reactive astrocytes: production, function, and therapeutic potential. Immunity 46, 957–967. doi: 10.1016/j.immuni.2017.06.006
Lima Giacobbo, B., Doorduin, J., Klein, H. C., Dierckx, R., Bromberg, E., and de Vries, E. F. J. (2019). Brain-derived neurotrophic factor in brain disorders: focus on neuroinflammation. Mol. Neurobiol. 56, 3295–3312. doi: 10.1007/s12035-018-1283-6
Liu, D., Wei, Y., Liu, Y., Wu, T., Hu, J., and Lu, H. (2021). The long non-coding RNA NEAT1/miR-224-5p/IL-33 axis modulates macrophage M2a polarization and A1 astrocyte activation. Mol. Neurobiol. [Epub ahead of print]. doi: 10.1007/s12035-021-02405-x
Liu, L. R., Liu, J. C., Bao, J. S., Bai, Q. Q., and Wang, G. Q. (2020). Interaction of microglia and astrocytes in the neurovascular unit. Front. Immunol. 11:1024. doi: 10.3389/fimmu.2020.01024
Liu, N., Sun, H., Li, X., Cao, W., Peng, A., Dong, S., et al. (2021). Downregulation of lncRNA KCNQ1OT1 relieves traumatic brain injury induced neurological deficits via promoting “M2” microglia polarization. Brain Res. Bull. 171, 91–102. doi: 10.1016/j.brainresbull.2021.03.004
Liu, X., Zhou, F., Wang, W., Chen, G., Zhang, Q., Lv, R., et al. (2021). IL-9-triggered lncRNA Gm13568 regulates Notch1 in astrocytes through interaction with CBP/P300: contribute to the pathogenesis of experimental autoimmune encephalomyelitis. J. Neuroinflam. 18:108. doi: 10.1186/s12974-021-02156-5
Liu, Z., Li, X., Sun, N., Xu, Y., Meng, Y., Yang, C., et al. (2014). Microarray profiling and co-expression network analysis of circulating lncRNAs and mRNAs associated with major depressive disorder. PLoS One 9:e93388. doi: 10.1371/journal.pone.0093388
Löscher, W. (1999). Valproate: a reappraisal of its pharmacodynamic properties and mechanisms of action. Prog. Neurobiol. 58, 31–59. doi: 10.1016/s0301-0082(98)00075-6
Ma, M., Xiong, W., Hu, F., Deng, M. F., Huang, X., Chen, J. G., et al. (2020). A novel pathway regulates social hierarchy via lncRNA AtLAS and postsynaptic synapsin IIb. Cell Res. 30, 105–118. doi: 10.1038/s41422-020-0273-1
Malhi, G. S., and Mann, J. J. (2018). Depression. Lancet 392, 2299–2312. doi: 10.1016/s0140-6736(18)31948-2
Meng, J., Ding, T., Chen, Y., Long, T., Xu, Q., Lian, W., et al. (2021). LncRNA-Meg3 promotes Nlrp3-mediated microglial inflammation by targeting miR-7a-5p. Int. Immunopharmacol. 90:107141. doi: 10.1016/j.intimp.2020.107141
Meng, S., Zhou, H., Feng, Z., Xu, Z., Tang, Y., Li, P., et al. (2017). CircRNA: functions and properties of a novel potential biomarker for cancer. Mol. Cancer 16:94. doi: 10.1186/s12943-017-0663-2
Moreno-García, L., López-Royo, T., Calvo, A. C., Toivonen, J. M., de la Torre, M., Moreno-Martínez, L., et al. (2020). Competing Endogenous RNA networks as biomarkers in neurodegenerative diseases. Int. J. Mol. Sci. 21:9582. doi: 10.3390/ijms21249582
Nelson, V. L., Nguyen, H. C. B., Garcìa-Cañaveras, J. C., Briggs, E. R., Ho, W. Y., DiSpirito, J. R., et al. (2018). PPARγ is a nexus controlling alternative activation of macrophages via glutamine metabolism. Genes Dev. 32, 1035–1044. doi: 10.1101/gad.312355.118
Ni, X., Su, Q., Xia, W., Zhang, Y., Jia, K., Su, Z., et al. (2020). Knockdown lncRNA NEAT1 regulates the activation of microglia and reduces AKT signaling and neuronal apoptosis after cerebral ischemic reperfusion. Sci. Rep. 10:19658. doi: 10.1038/s41598-020-71411-1
Nutma, E., van Gent, D., Amor, S., and Peferoen, L. A. N. (2020). Astrocyte and oligodendrocyte cross-talk in the central nervous system. Cells 9:600. doi: 10.3390/cells9030600
Pan, J., Konstas, A. A., Bateman, B., Ortolano, G. A., and Pile-Spellman, J. (2007). Reperfusion injury following cerebral ischemia: pathophysiology, MR imaging, and potential therapies. Neuroradiology 49, 93–102. doi: 10.1007/s00234-006-0183-z
Payne, J. L., and Maguire, J. (2019). Pathophysiological mechanisms implicated in postpartum depression. Front. Neuroendocrinol. 52:1. doi: 10.1016/j.yfrne.2018.12.001
Pekny, M., and Nilsson, M. (2005). Astrocyte activation and reactive gliosis. Glia 50, 427–434. doi: 10.1002/glia.20207
Peterson, A. R., and Binder, D. K. (2020). Astrocyte glutamate uptake and signaling as novel targets for antiepileptogenic therapy. Front. Neurol. 11:1006. doi: 10.3389/fneur.2020.01006
Pietrowski, M. J., Gabr, A. A., Kozlov, S., Blum, D., Halle, A., and Carvalho, K. (2021). Glial purinergic signaling in neurodegeneration. Front. Neurol. 12:654850. doi: 10.3389/fneur.2021.654850
Qi, X., Shao, M., Sun, H., Shen, Y., Meng, D., and Huo, W. (2017). Long non-coding RNA SNHG14 promotes microglia activation by regulating miR-145-5p/PLA2G4A in cerebral infarction. Neuroscience 348, 98–106. doi: 10.1016/j.neuroscience.2017.02.002
Qian, X., Zhao, J., Yeung, P. Y., Zhang, Q. C., and Kwok, C. K. (2019). Revealing lncRNA structures and interactions by sequencing-based approaches. Trends Biochem. Sci. 44, 33–52. doi: 10.1016/j.tibs.2018.09.012
Ren, Z., Qi, Y., Sun, S., Tao, Y., and Shi, R. (2020). Mesenchymal stem cell-derived exosomes: hope for spinal cord injury repair. Stem Cells Dev. 29, 1467–1478. doi: 10.1089/scd.2020.0133
Rösing, N., Salvador, E., Güntzel, P., Kempe, C., Burek, M., Holzgrabe, U., et al. (2020). Neuroprotective effects of isosteviol sodium in murine brain capillary cerebellar endothelial cells (cerebEND) after hypoxia. Front. Cell Neurosci. 14:573950. doi: 10.3389/fncel.2020.573950
Salim, U., Kumar, A., Kulshreshtha, R., and Vivekanandan, P. (2021). Biogenesis, characterization, and functions of mirtrons. Wiley Interdiscip. Rev. RNA [Epub ahead of print]. doi: 10.1002/wrna.1680
Salter, M. W., and Stevens, B. (2017). Microglia emerge as central players in brain disease. Nat. Med. 23, 1018–1027. doi: 10.1038/nm.4397
Santello, M., Toni, N., and Volterra, A. (2019). Astrocyte function from information processing to cognition and cognitive impairment. Nat. Neurosci. 22, 154–166. doi: 10.1038/s41593-018-0325-8
Scheffer, I. E., Berkovic, S., Capovilla, G., Connolly, M. B., French, J., Guilhoto, L., et al. (2017). ILAE classification of the epilepsies: position paper of the ILAE commission for classification and terminology. Epilepsia 58, 512–521. doi: 10.1111/epi.13709
Scheltens, P., Blennow, K., Breteler, M. M., de Strooper, B., Frisoni, G. B., Salloway, S., et al. (2016). Alzheimer’s disease. Lancet 388, 505–517. doi: 10.1016/s0140-6736(15)01124-1
Shao, L., Jiang, G. T., Yang, X. L., Zeng, M. L., Cheng, J. J., Kong, S., et al. (2021). Silencing of circIgf1r plays a protective role in neuronal injury via regulating astrocyte polarization during epilepsy. FASEB J. 35:e21330. doi: 10.1096/fj.202001737RR
Shao, M., Jin, M., Xu, S., Zheng, C., Zhu, W., Ma, X., et al. (2020). Exosomes from long noncoding RNA-Gm37494-ADSCs repair spinal cord injury via shifting microglial M1/M2 polarization. Inflammation 43, 1536–1547. doi: 10.1007/s10753-020-01230-z
Shi, Z., Pan, B., and Feng, S. (2018). The emerging role of long non-coding RNA in spinal cord injury. J. Cell Mol. Med. 22, 2055–2061. doi: 10.1111/jcmm.13515
Sommer, C. J. (2017). Ischemic stroke: experimental models and reality. Acta Neuropathol. 133, 245–261. doi: 10.1007/s00401-017-1667-0
Sun, D., Yu, Z., Fang, X., Liu, M., Pu, Y., Shao, Q., et al. (2017). LncRNA GAS5 inhibits microglial M2 polarization and exacerbates demyelination. EMBO Rep. 18, 1801–1816. doi: 10.15252/embr.201643668
Ta Na, H. S., An, M., Zhang, T., Deni, W., Hou, L., and Jin, K. (2020). Dexmedetomidine inhibits microglial activation through SNHG14/HMGB1 pathway in spinal cord ischemia-reperfusion injury mice. Int. J. Neurosci. [Epub ahead of print]. doi: 10.1080/00207454.2020.1835901
Teng, F., and Fussenegger, M. (2020). Shedding light on extracellular vesicle biogenesis and bioengineering. Adv. Sci. 8:2003505. doi: 10.1002/advs.202003505
Tian, J., Liu, Y., Wang, Z., Zhang, S., Yang, Y., Zhu, Y., et al. (2021). LncRNA Snhg8 attenuates microglial inflammation response and blood-brain barrier damage in ischemic stroke through regulating miR-425-5p mediated SIRT1/NF-κB signaling. J. Biochem. Mol. Toxicol. 35:e22724. doi: 10.1002/jbt.22724
Tripathi, S., Shree, B., Mohapatra, S., Swati, B., Basu, A., and Sharma, V. (2021). The expanding regulatory mechanisms and cellular functions of long non-coding RNAs (lncRNAs) in neuroinflammation. Mol. Neurobiol. 58, 2916–2939. doi: 10.1007/s12035-020-02268-8
Vainchtein, I. D., and Molofsky, A. V. (2020). Astrocytes and microglia: in sickness and in health. Trends Neurosci. 43, 144–154. doi: 10.1016/j.tins.2020.01.003
Wan, Y., and Yang, Z. Q. (2020). LncRNA NEAT1 affects inflammatory response by targeting miR-129-5p and regulating Notch signaling pathway in epilepsy. Cell Cycle 19, 419–431. doi: 10.1080/15384101.2020.1711578
Wang, H., Zhou, W. X., Huang, J. F., Zheng, X. Q., Tian, H. J., Wang, B., et al. (2020d). Endocrine therapy for the functional recovery of spinal cord injury. Front. Neurosci. 14:590570. doi: 10.3389/fnins.2020.590570
Wang, G., Han, B., Shen, L., Wu, S., Yang, L., Liao, J., et al. (2020a). Silencing of circular RNA HIPK2 in neural stem cells enhances functional recovery following ischaemic stroke. EBioMedicine 52:102660. doi: 10.1016/j.ebiom.2020.102660
Wang, H., Yao, G., Li, L., Ma, Z., Chen, J., and Chen, W. (2020b). LncRNA-UCA1 inhibits the astrocyte activation in the temporal lobe epilepsy via regulating the JAK/STAT signaling pathway. J. Cell. Biochem. 121, 4261–4270. doi: 10.1002/jcb.29634
Wang, H., Zheng, X., Jin, J., Zheng, L., Guan, T., Huo, Y., et al. (2020c). LncRNA MALAT1 silencing protects against cerebral ischemia-reperfusion injury through miR-145 to regulate AQP4. J. Biomed. Sci. 27:40. doi: 10.1186/s12929-020-00635-0
Wang, J., Zhao, H., Fan, Z., Li, G., Ma, Q., Tao, Z., et al. (2017). Long noncoding RNA H19 promotes neuroinflammation in ischemic stroke by driving histone deacetylase 1-dependent M1 microglial polarization. Stroke 48, 2211–2221. doi: 10.1161/strokeaha.117.017387
Wang, L. Q., and Zhou, H. J. (2018). LncRNA MALAT1 promotes high glucose-induced inflammatory response of microglial cells via provoking MyD88/IRAK1/TRAF6 signaling. Sci. Rep. 8:8346. doi: 10.1038/s41598-018-26421-5
Wolf, S. A., Boddeke, H. W., and Kettenmann, H. (2017). Microglia in physiology and disease. Annu. Rev. Physiol. 79, 619–643. doi: 10.1146/annurev-physiol-022516-034406
Wu, P. F., Zhang, Z., Wang, F., and Chen, J. G. (2010). Natural compounds from traditional medicinal herbs in the treatment of cerebral ischemia/reperfusion injury. Acta Pharmacol. Sin. 31, 1523–1531. doi: 10.1038/aps.2010.186
Xiang, W., Jiang, L., Zhou, Y., Li, Z., Zhao, Q., Wu, T., et al. (2021). The lncRNA Ftx/miR-382-5p/Nrg1 axis improves the inflammation response of microglia and spinal cord injury repair. Neurochem. Int. 143:104929. doi: 10.1016/j.neuint.2020.104929
Xiao, S., Wang, C., Yang, Q., Xu, H., Lu, J., and Xu, K. (2021). Rea regulates microglial polarization and attenuates neuronal apoptosis via inhibition of the NF-κB and MAPK signalings for spinal cord injury repair. J. Cell Mol. Med. 25, 1371–1382. doi: 10.1111/jcmm.16220
Xiaoying, G., Guo, M., Jie, L., Yanmei, Z., Ying, C., Shengjie, S., et al. (2020). CircHivep2 contributes to microglia activation and inflammation via miR-181a-5p/SOCS2 signalling in mice with kainic acid-induced epileptic seizures. J. Cell Mol. Med. 24, 12980–12993. doi: 10.1111/jcmm.15894
Xu, K., Zhang, Y., Xiong, W., Zhang, Z., Wang, Z., Lv, L., et al. (2020). CircGRIA1 shows an age-related increase in male macaque brain and regulates synaptic plasticity and synaptogenesis. Nat. Commun. 11:3594. doi: 10.1038/s41467-020-17435-7
Xu, Q., Zhao, B., Ye, Y., Li, Y., Zhang, Y., Xiong, X., et al. (2021). Relevant mediators involved in and therapies targeting the inflammatory response induced by activation of the NLRP3 inflammasome in ischemic stroke. J. Neuroinflam. 18:123. doi: 10.1186/s12974-021-02137-8
Xu, S., Wang, J., Jiang, J., Song, J., Zhu, W., Zhang, F., et al. (2020). TLR4 promotes microglial pyroptosis via lncRNA-F630028O10Rik by activating PI3K/AKT pathway after spinal cord injury. Cell Death Dis. 11:693. doi: 10.1038/s41419-020-02824-z
Xu, W., Zhang, L., Geng, Y., Liu, Y., and Zhang, N. (2020). Long noncoding RNA GAS5 promotes microglial inflammatory response in Parkinson’s disease by regulating NLRP3 pathway through sponging miR-223-3p. Int. Immunopharmacol. 85:106614. doi: 10.1016/j.intimp.2020.106614
Yang, B., Zang, L., Cui, J., and Wei, L. (2021). Circular RNA TTC3 regulates cerebral ischemia-reperfusion injury and neural stem cells by miR-372-3p/TLR4 axis in cerebral infarction. Stem Cell Res. Ther. 12:125. doi: 10.1186/s13287-021-02187-y
Yang, J., Chen, M., Cao, R. Y., Li, Q., and Zhu, F. (2018). The role of circular RNAs in cerebral ischemic diseases: ischemic stroke and cerebral ischemia/reperfusion injury. Adv. Exp. Med. Biol. 1087, 309–325. doi: 10.1007/978-981-13-1426-1_25
Ye, Y., He, X., Lu, F., Mao, H., Zhu, Z., Yao, L., et al. (2018). A lincRNA-p21/miR-181 family feedback loop regulates microglial activation during systemic LPS- and MPTP- induced neuroinflammation. Cell Death Dis. 9:803. doi: 10.1038/s41419-018-0821-5
Yi, J., Chen, B., Yao, X., Lei, Y., Ou, F., and Huang, F. (2019). Upregulation of the lncRNA MEG3 improves cognitive impairment, alleviates neuronal damage, and inhibits activation of astrocytes in hippocampus tissues in Alzheimer’s disease through inactivating the PI3K/Akt signaling pathway. J. Cell. Biochem. 120, 18053–18065. doi: 10.1002/jcb.29108
Yu, Q., Zhao, M. W., and Yang, P. (2020). LncRNA UCA1 suppresses the inflammation via modulating miR-203-mediated regulation of MEF2C/NF-κB signaling pathway in epilepsy. Neurochem. Res. 45, 783–795. doi: 10.1007/s11064-019-02952-9
Zhang, B., Li, Q., Jia, S., Li, F., Li, Q., and Li, J. (2020). LincRNA-EPS in biomimetic vesicles targeting cerebral infarction promotes inflammatory resolution and neurogenesis. J. Transl. Med. 18:110. doi: 10.1186/s12967-020-02278-z
Zhang, X., Zhu, X. L., Ji, B. Y., Cao, X., Yu, L. J., Zhang, Y., et al. (2019b). LncRNA-1810034E14Rik reduces microglia activation in experimental ischemic stroke. J. Neuroinflam. 16:75. doi: 10.1186/s12974-019-1464-x
Zhang, H., Lu, M., Zhang, X., Kuai, Y., Mei, Y., Tan, Q., et al. (2019a). Isosteviol sodium protects against ischemic stroke by modulating microglia/macrophage polarization via disruption of GAS5/miR-146a-5p sponge. Sci. Rep. 9:12221. doi: 10.1038/s41598-019-48759-0
Zhang, Y., Huang, R., Cheng, M., Wang, L., Chao, J., Li, J., et al. (2019c). Gut microbiota from NLRP3-deficient mice ameliorates depressive-like behaviors by regulating astrocyte dysfunction via circHIPK2. Microbiome 7:116. doi: 10.1186/s40168-019-0733-3
Zhang, Y., Wang, J., Zhang, Y., Wei, J., Wu, R., and Cai, H. (2019d). Overexpression of long noncoding RNA Malat1 ameliorates traumatic brain injury induced brain edema by inhibiting AQP4 and the NF-κB/IL-6 pathway. J. Cell. Biochem. 120, 17584–17592. doi: 10.1002/jcb.29025
Zhang, M., Yang, J. K., and Ma, J. (2021). Regulation of the long noncoding RNA XIST on the inflammatory polarization of microglia in cerebral infarction. Exp. Ther. Med. 22:924. doi: 10.3892/etm.2021.10356
Zhang, N., Gao, Y., Yu, S., Sun, X., and Shen, K. (2020). Berberine attenuates Aβ42-induced neuronal damage through regulating circHDAC9/miR-142-5p axis in human neuronal cells. Life Sci. 252:117637. doi: 10.1016/j.lfs.2020.117637
Zhang, S., Sun, W. C., Liang, Z. D., Yin, X. R., Ji, Z. R., Chen, X. H., et al. (2020). LncRNA SNHG4 attenuates inflammatory responses by sponging miR-449c-5p and up-regulating STAT6 in microglial during cerebral ischemia-reperfusion injury. Drug Des. Devel. Ther. 14, 3683–3695. doi: 10.2147/dddt.s245445
Zhang, Y., Du, L., Bai, Y., Han, B., He, C., Gong, L., et al. (2020). CircDYM ameliorates depressive-like behavior by targeting miR-9 to regulate microglial activation via HSP90 ubiquitination. Mol. Psychiatry 25, 1175–1190. doi: 10.1038/s41380-018-0285-0
Zhang, Z., Sun, X., Zhao, G., Ma, Y., and Zeng, G. (2021). LncRNA embryonic stem cells expressed 1 (Lncenc1) is identified as a novel regulator in neuropathic pain by interacting with EZH2 and downregulating the expression of Bai1 in mouse microglia. Exp. Cell Res. 399:112435. doi: 10.1016/j.yexcr.2020.112435
Zhao, Q., Lu, F., Su, Q., Liu, Z., Xia, X., Yan, Z., et al. (2020). Knockdown of long noncoding RNA XIST mitigates the apoptosis and inflammatory injury of microglia cells after spinal cord injury through miR-27a/Smurf1 axis. Neurosci. Lett. 715:134649. doi: 10.1016/j.neulet.2019.134649
Zhao, T., Ding, Y., Li, M., Zhou, C., and Lin, W. (2019). Silencing lncRNA PVT1 inhibits activation of astrocytes and increases BDNF expression in hippocampus tissues of rats with epilepsy by downregulating the Wnt signaling pathway. J. Cell Physiol. [Epub ahead of print]. doi: 10.1002/jcp.28264
Zhao, Y., He, J., Yu, N., Jia, C., and Wang, S. (2020). Mechanisms of dexmedetomidine in neuropathic pain. Front. Neurosci. 14:330. doi: 10.3389/fnins.2020.00330
Zheng, W. X., He, W. Q., Zhang, Q. R., Jia, J. X., Zhao, S., Wu, F. J., et al. (2021). Baicalin inhibits NLRP3 inflammasome activity via the AMPK signaling pathway to alleviate cerebral ischemia-reperfusion injury. Inflammation [Epub ahead of print]. doi: 10.1007/s10753-021-01486-z
Zhong, J., Cheng, C., Liu, H., Huang, Z., Wu, Y., Teng, Z., et al. (2017a). Bexarotene protects against traumatic brain injury in mice partially through apolipoprotein E. Neuroscience 343, 434–448. doi: 10.1016/j.neuroscience.2016.05.033
Zhong, J., Jiang, L., Huang, Z., Zhang, H., Cheng, C., Liu, H., et al. (2017b). The long non-coding RNA Neat1 is an important mediator of the therapeutic effect of bexarotene on traumatic brain injury in mice. Brain Behav. Immun. 65, 183–194. doi: 10.1016/j.bbi.2017.05.001
Zhou, H. J., Wang, L. Q., Wang, D. B., Yu, J. B., Zhu, Y., Xu, Q. S., et al. (2018). Long noncoding RNA MALAT1 contributes to inflammatory response of microglia following spinal cord injury via the modulation of a miR-199b/IKKβ/NF-κB signaling pathway. Am. J. Physiol. Cell Physiol. 315, C52–C61. doi: 10.1152/ajpcell.00278.2017
Zhu, H., Xu, H., Ma, H., Luo, L., Yang, L., Chen, F., et al. (2020). LncRNA CASC2 inhibits astrocytic activation and adenosine metabolism by regulating PTEN in pentylenetetrazol-induced epilepsy model. J. Chem. Neuroanat. 105:101749. doi: 10.1016/j.jchemneu.2020.101749
Zhu, J., and Tang, J. (2020). LncRNA Gm14205 induces astrocytic NLRP3 inflammasome activation via inhibiting oxytocin receptor in postpartum depression. Biosci. Rep. 40:BSR20200672. doi: 10.1042/bsr20200672
Keywords: lncRNA, circRNA, microglia, astrocyte, neurological diseases
Citation: Chen M, Lai X, Wang X, Ying J, Zhang L, Zhou B, Liu X, Zhang J, Wei G and Hua F (2021) Long Non-coding RNAs and Circular RNAs: Insights Into Microglia and Astrocyte Mediated Neurological Diseases. Front. Mol. Neurosci. 14:745066. doi: 10.3389/fnmol.2021.745066
Received: 21 July 2021; Accepted: 14 September 2021;
Published: 05 October 2021.
Edited by:
George Smith, Temple University, United StatesReviewed by:
Gerald Seifert, University Hospital Bonn, GermanyKai K. Kummer, Innsbruck Medical University, Austria
Copyright © 2021 Chen, Lai, Wang, Ying, Zhang, Zhou, Liu, Zhang, Wei and Hua. This is an open-access article distributed under the terms of the Creative Commons Attribution License (CC BY). The use, distribution or reproduction in other forums is permitted, provided the original author(s) and the copyright owner(s) are credited and that the original publication in this journal is cited, in accordance with accepted academic practice. No use, distribution or reproduction is permitted which does not comply with these terms.
*Correspondence: Fuzhou Hua, huafuzhou@126.com
†These authors have contributed equally to this work